- 1Guangzhou Institute of Energy Conversion, Chinese Academy of Sciences, Guangzhou, China
- 2Key Laboratory of Ministry of Education for Water Quality Security and Protection in Pearl River Delta, Guangdong Provincial Key Laboratory of Radionuclides Pollution Control and Resources, School of Environmental Science and Engineering, Guangzhou University, Guangzhou, China
- 3CAS Key Laboratory of Renewable Energy, Guangzhou, China
- 4Guangdong Provincial Key Laboratory of New and Renewable Energy Research and Development, Guangzhou, China
- 5University of Chinese Academy of Sciences, Beijing, China
This study investigated the effects of organic loading rates (OLRs) and temperature on the performance and stability of anaerobic digestion treating the organic fraction of municipal solid waste (OFMSW) in a semi-continuous mode. Furthermore, a preliminary energy analysis was conducted to determine the optimal application parameters for anaerobic digestion of OFMSW. Results showed that the maximum achievable OLR was 9.00 g VS/(L·d), with a volumetric biogas yield of 4.03 L/(L·d) and 4.31 L/(L·d) for mesophilic and thermophilic systems, respectively. However, the preliminary energy analysis showed higher energy conversion efficiency was achieved for the system operated at an OLR of 7.50 g VS/(L·d). The system operated at the thermophilic condition had a higher total energy due to more electricity and heat energy generated than the mesophilic one. Therefore, the recommended operating conditions for the anaerobic digestion of OFMSW is an OLR of 7.50 g VS/(L·d) and thermophilic condition. Based on the annual yield of OFMSW in China, the estimated net output energy was 69970.61 GWh per year.
Introduction
With the rapid development of urbanization and industrialization, the output of municipal solid waste (MSW) has increased dramatically. For example, the total production of MSW in China was ~192 million tons in 2015 (Liu et al., 2017c), resulting in serious environmental and health problems. Current methods for the disposal of MSW include landfill, incineration, and biological processing. Landfill is an undesirable technology due to its requirement for land resources, and environmental issues (Mu et al., 2018). Incineration is often affected by the chemical composition of MSW. In China, typical MSW has the characteristics of high moisture content (>50%) and high organic fractions (60–70%) (Liu et al., 2017b), which lowers the energy recovery rate, and thus necessitates supplementation with auxiliary fuel. The high organic component in MSW favors biological treatment, and anaerobic digestion can achieve better environmental performance than incineration, bio-landfills or composting (Arafat et al., 2015). Liu et al. (2017c) concluded that the “best” treatment scenario for MSW is biological treatment of the biodegradable fractions and incineration of the high calorific value components, followed by landfill of the remaining residues if the land application of digestive residue become feasibility. Therefore, for the organic fraction of MSW (OFMSW), anaerobic digestion may be an alternative treatment method.
The anaerobic digestion of MSW is receiving increasing attention, with the reported specific methane yields of MSW of 158–553 mL/g vs. (Mu et al., 2018). Temperature and OLRs are one of the most important parameters of anaerobic digestion (Wirth et al., 2015; Hu et al., 2018). Although thermophilic condition can increase the performance of anaerobic digestion, its energy requirement and energy loss accordingly increase (Siqueiros et al., 2019). On the other hand, higher organic loading rates (OLRs) could improve the processing efficiency of anaerobic digestion, while it might inhibit the biogas production (Pearse et al., 2018; Rattanapan et al., 2019). Thus, both OLRs and temperature can be considered key parameters for digestion, and their suitable application will determine the outcome in terms of energy feasibility (Barbot et al., 2016; Ganesh Saratale et al., 2018). The methane yield is considered as one of the key parameters affecting output energy (thermal energy and electricity energy) (Abad et al., 2019). However, limited literatures focused on OFMSW treatment and management with considering energy benefits (Callan and Thomas, 2001; Martinez-Sanchez et al., 2016). Therefore, an energy analysis is required to evaluate before determining optimal operating conditions.
The present study aims to: (1) assess the biogas production performance and stability of anaerobic digestion system feeding OFMSW at different temperatures and OLRs; (2) calculate the potential biogas production based on the annual production of OFMSW in China; (3) conduct a preliminary energy analysis to determine the optimal temperature and the maximum operating OLR for OFMSW.
Materials and Methods
Feedstock and Inoculum
Municipal solid waste was collected four times from the Heyuan district, Guangdong, China. OFMSW was obtained by removing impurities such as plastic, foam, stone, glass and metal. The collected OFMSW was milled and stored in a refrigerator prior to further utilization and analysis. The materials from the 1st, 2nd, 3rd, and 4th batches were used for the OLRs of 0.75–1.00 g VS/(L·d), 1.25–2.00 g VS/(L·d), 2.50–3.50 g VS/(L·d), and 4.00–11.00 g VS/(L·d), respectively.
The inoculum was taken from existing CSTR reactors in the lab, which were fed an artificially prepared substrate composed of peptone, yeast powder, xylose, cellulose, starch, and glucose. The characteristics of the feedstock and inoculum were listed in Table 1.
Experimental Setup and Procedure
Two CSTR-type reactors (2 L) were operated semi-continuously at a temperature of 35 ± 1°C for mesophilic conditions, and 55 ± 1°C for thermophilic conditions. The biogas produced was collected in a gas sampling bag (5 L, Dalian Hede Technologies Co., Ltd.).
Each reactor was filled with 1800 mL of inoculum, and fed material at different weights to achieve the required OLRs, which ranged from 0.75 g VS/(L·d) to 11.00 g VS/(L·d). A 30-day hydraulic retention time was used for OLRs of 0.75–6.50 g VS/(L·d), coupled with a wet weight of 4.45–59.98 g/d. For OLRs of 7.50, 9.00, and 11.00 g VS/(L·d), the average weights were 69.21, 82.96 and 101.50 g/d, respectively, and the hydraulic retention times were 26, 22 and 18 days, respectively. Each OLR was run for 22–40 d except for the OLR of 11.00 g VS/(L·d). The experimental lasted for 402–410 days.
Analytical Methods
The values of total solids (TS) content, violate solids (VS) content, pH, total ammonia nitrogen (TAN), partial alkalinity (PA), intermediate alkalinity (IA), volatile fatty acids (VFAs), and methane content (CH4) were measured according to previously described methods (Li et al., 2012, 2018a). Elemental carbon (C), nitrogen (N), and hydrogen (H) were measured using a Vario EL element analyzer (Elementar Analysensysteme GmbH, Hanau, Germany). Oxygen (O) content was calculated by subtracting the C, H and N content from 100%.
Calculation Methods
Following Buswell and Boruff (1932), theoretical biogas potential (TBP) was calculated based on the elemental contents (Equation 1).
Statistical Analysis
The physical and chemical properties of OFMSW were statistically analyzed using IBM SPSS Statistics 25 software with one-way analysis of variance (ANOVA) at a probability level of 0.05.
Preliminary Energy Analysis
A preliminary energy analysis was conducted to compare the energy revenue under different conditions. The designed anaerobic reactor was set to 3000 m3, a typical volume of large-scale commercial reactors (Milledge and Heaven, 2017). When the height is equal to the diameter, the reactor has minimal heat loss (Taricska et al., 2011). The number of anaerobic reactors used to treat OFMSW produced in China was calculated according to Equation (2):
Where N = number of anaerobic reactors; M = OFMSW produced in China per day, 144,123 kilotons, which was calculated in section 4.2; VS = volatile solid content of OFMSW, 21.07% which was the average of VS of material in this study; VR = volume of reactor for OFMSW digestion, 3000 m3; K = the utilization rate of VR, 80%; OLR = organic loading rate.
For anaerobic digestion of OFMSW at mesophilic and thermophilic conditions, the input energy includes energy for feedstock transportation, feedstock separation, feedstock heating (Qheating), pumping (Qpumping), mixing (Qmixing), and heat loss of reactors (Qheatloss) (Milledge and Heaven, 2017; Mu et al., 2018). The output energy (biogas) from anaerobic digestion can be transferred into thermal (Qthermal energy) and electricity (Qelectricity energy) energy through a combined heat and power unit (CHP) (Zema, 2017; Li et al., 2019).
Related calculations involved in the preliminary energy analysis are shown in Figure 1, where C = specific heat of influent, which approximated to water, 4.18 KJ/(kg °C); VI = the flow volume fed to the digester per day; ϕ = the electricity energy used for pumping, 0.5 KWh/m3 (Lu et al., 2008; Mu et al., 2018); ω = the electricity energy used for stirring, 0.08 KWh/(m3·d) (Lu et al., 2008; Mu et al., 2018); The material used for the designed reactor was 300 mm thick insulated concrete wall, U = heat transfer coefficient, 1.99 W/(m2·°C) (Liu et al., 2017a); A = superficial area of reactor, which was calculated according to the calculation formula, 1152 m2; T1 = ambient temperature, which is close the average annual temperature in China, 10.3°C; T2 = reactor temperature, 35°C for mesophilic condition or 55°C for thermophilic condition; The efficiency of the CHP in converting biogas into thermal and electricity energy were 33 and 50%, respectively (Feiz et al., 2019; Valentino et al., 2019), HM = calorific value of methane, 35.8 kJ/L (Chen et al., 2019), and VM = volume of methane produced.
Results
Chemical Composition of the Material
The TS content of OFMSW ranged from 26.82 to 32.62%, and the VS/TS ratio was 68.69–73.64%, suggesting that the major part of the feedstock was biodegradable. The C, N, and H contents were in the range of 32.84–37.13%, 0.92–1.75%, and 5.33–5.53%, respectively. Correspondingly, the C/N ratios were in the range of 20.16–41.01. In general, a C/N ratio of 15–35 is suitable for anaerobic digestion (Silvestre et al., 2015; Capson-Tojo et al., 2016; Li et al., 2018b). While, the material with C/N ratio of 2.3–152 was used for anaerobic digestion (Xu et al., 2018), indicating that the effect of C/N ratio on the performance of anaerobic digestion influenced by other factors, such as carbon type (Choi et al., 2020). No obvious difference was observed for the TS and VS contents, while elemental composition significantly changed. The chemical composition of MSW is site- and season-specific (Breitenmoser et al., 2018), which affects the performance and stability of anaerobic digestion. The specific biogas yields for the component of MSW, such as fruit, vegetable and yard wastes from different seasons, were between 360 and 620 mL/g VS for mono- or co-digested systems (Korai et al., 2018). The reported specific methane yields for meat/fish and paper waste were 293 ± 3 mL/g VS and 217 ± 5 mL/g VS, respectively (Nielfa et al., 2015).
Performance of Anaerobic Digestion of OFMSW in Semi-Continuous Mode
The Performance at Mesophilic Condition
For the system operated at mesophilic condition, the volumetric biogas yields increased from 0.17 ± 0.03 to 4.03 ± 0.42 L/(L·d) with the OLRs increased from 0.75 to 9.00 g VS/(L·d) (Figure S1 and Table 2). Meanwhile, the specific biogas and methane yields varied from 335–495 to 196–278 mL/g VS, respectively, with the methane contents of 55.23–59.97%. During the process, the pH maintained at a range of 6.49–7.73 (Figure 2A), and the concentrations of TAN, acetic acid and propionic acid were <860, 596, and 340 mg/L, respectively (Figures 2A,B), below the reported inhibition values (Zhang et al., 2014; Meng et al., 2018). In addition, the values of stability indicator, IA/PA ratio, was within the range of 0.03–0.51 (Figure 3), suggesting that the system well-performed at an OLR of 0.75–9.00 g VS/(L·d). An IA/PA ratio of <0.9 indicated that the system is in a stable condition (Ferrer et al., 2010).
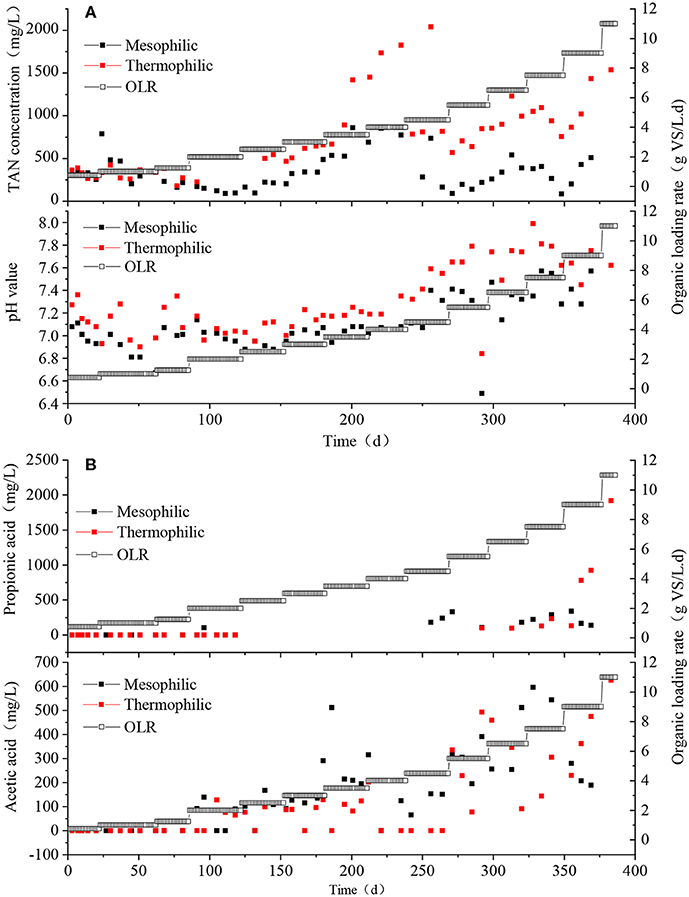
Figure 2. (A) TAN concentration and pH value of anaerobic digestion under different OLR and temperature conditions; (B) propionic acid and acetic acid of anaerobic digestion under different OLR and temperature conditions.
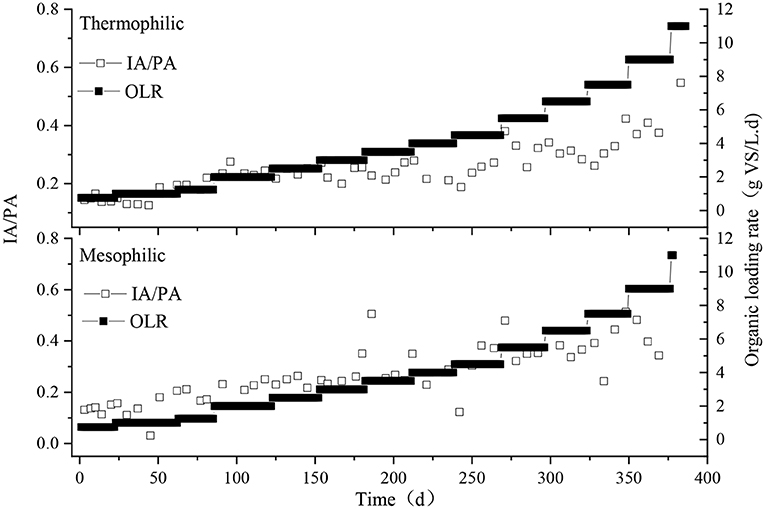
Figure 3. The stability indicator of anaerobic digestion under different OLR and temperature conditions.
Increasing the OLR to 11.00 g VS/(L·d), the volumetric biogas yield decreased to 3.80 ± 0.42 L/(L·d), and correspondingly the specific biogas yield decreased to 346 ± 38 mL/g VS. At the OLR, the system became hard to operate due to overloading. Rincón et al. (2008) revealed that OFMSW anaerobic digestion failed at an OLR of 11.00 g COD/L due to the sudden drop in alkalinity and TVFA accumulation. Under a high OLR, the system has a high solid content and a low moisture content, which hinder the conversion of VFAs (Veeken and Hamelers, 1999).
The Performance at Thermophilic Condition
For the system operated at thermophilic condition, the volumetric biogas yields ranged from 0.19 ± 0.02 to 4.31 ± 0.43 L/(L·d) within the OLRs of 0.50–9.00 g VS/(L·d). Meanwhile the specific biogas and methane yields ranged in 370–553 and 214–302 mL/g VS, respectively, with a methane content of 51.81–60.09%. With respect to the parameters of the process, the pH was 6.84–7.99, and the concentrations of acetic acid and propionic acid were <493 and 923 mg/L, respectively (Figures 2A,B). The TAN concentrations ranged between 180 and 2040 mg/L. The IA/PA ratio ranged in 0.11–0.42. These results indicated stable operation was achieved for the system operated at the OLRs of 0.75–9.00 g VS/(L·d).
Compared with the volumetric biogas yield obtained at the OLR of 9.00 g VS/(L·d), a slight decrease was observed at an OLR of 11.00 g VS/(L·d), coupled with a decrease of 21.29 and 19.05% in specific biogas and methane yields, respectively. In addition, higher concentrations in propionic acid (1921 mg/L) was observed, suggesting that an accumulation in VFAs had occurred due to overloading.
Discussion
The Variation in Biogas Yield at Different OLRs and Temperature Conditions
One of the critical operational parameters of anaerobic digestion is OLR, which is determined by the material characteristics and operation conditions. Generally, improved biogas yields appeared with the OLRs increased. An optimum OLR is beneficial in reducing the capital cost of the plants, improving the biogas yield, and ensuring stability in operation (Tan et al., 2018). For both reactors operating at mesophilic or thermophilic conditions, the volumetric biogas yields increased with the OLRs, and obtained their maximum values at OLRs of 9.00 g VS/(L·d) (Figure 4); the performance then decreased due to overloading. The results indicated that the biogas yield increased with the OLRs within the appropriate range (Li et al., 2015; Shen et al., 2018).
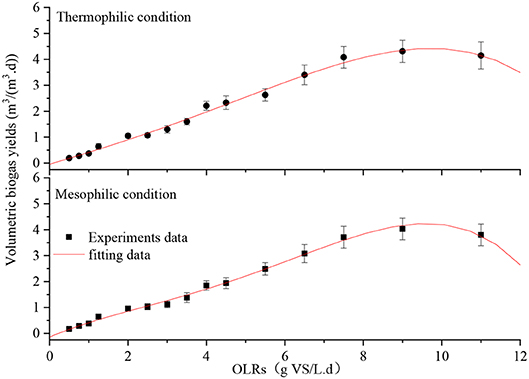
Figure 4. Relationship of OLRs and volumetric biogas yields under mesophilic and thermophilic conditions.
Temperature is another key parameter which influences the biochemical conversion pathway, reaction rate, and input energy. Generally, the optimum temperature is mesophilic (30–40°C) or thermophilic (50–60°C) (Hupfauf et al., 2018; Pearse et al., 2018). In this study, difference analysis showed that when the OLRs were 0.75–1.25 g VS/(L·d), the difference in specific biogas yields at mesophilic and thermophilic condition was not significant. When the OLRs were at 2.00–9.00 g VS/(L·d), the thermophilic reactor obtained higher specific biogas yields, with the specific biogas yields increased by 4.13–19.91% compared to that of the mesophilic reactor. The biogas conversion rate showed similar results, which were 49.92–75.82% and 54.39–84.00% for mesophilic and thermophilic conditions, respectively. This might be related to metabolic activities of microorganisms which increased with the temperature increased (Lu et al., 2008; Ganesh Saratale et al., 2018).
Preliminary Energy Analysis of OFMSW in China
According to the China Statistical Yearbook (State Statistical Bureau, 2015), total MSW generation was about 215,209 kilotons in 2017. From the view of total yield of MSW, the districts of Guangdong, Jiangsu, Shandong, and Zhejiang produces more than 10,000 kilotons per year (Figure 5A). From a perspective of density, that is yields per area, the district of Shanghai obtains the highest value of 1.18 kilotons/km2. While, the densities of Guangdong, Jiangsu, Shandong, and Zhejiang provinces were lower Shanghai, which were 0.15, 0.17, 0.10 and 0.14 kilotons/ km2, respectively. To obtain the organic fraction of MSW, several separation technologies have been established, such as water-assisted sorting treatment, and extrusion treatment. The extrusion treatment could potentially recover about 67% (w/w) of the organic fraction from the raw MSW (Mu et al., 2018). Accordingly, the mass available for anaerobic digestion would be ~144,123 kilotons.
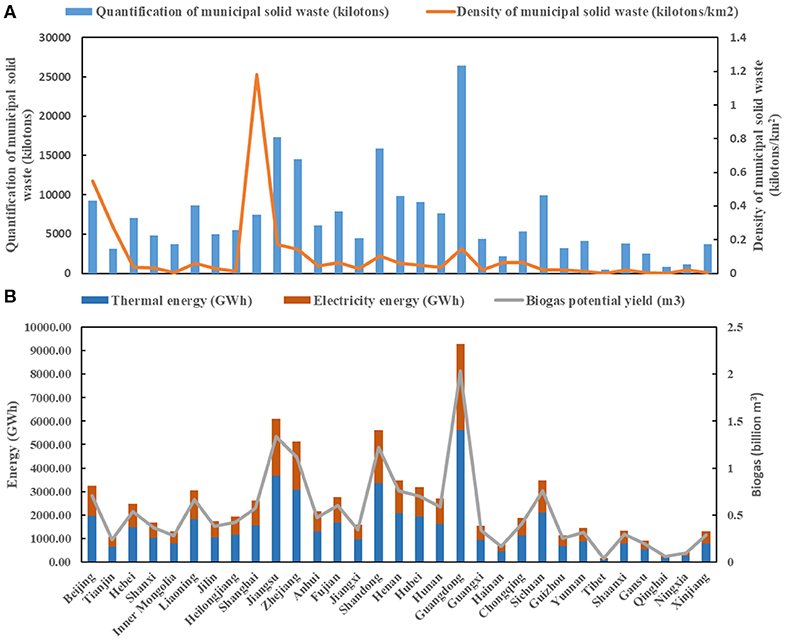
Figure 5. (A) Annual production of municipal solid waste in China; (B) Biogas and energy potential yield of municipal solid waste in China.
Accordingly to the yields of OFMSW in China, the energy revenues at different temperatures and OLRs were analyzed (Table 3). The results showed that the maximum total output energy of 191.70 GWh/day was obtained at the OLR of 7.50 g VS/(L·d) and thermophilic condition. At the condition, the required number of reactors for digested OFMSW was 4,622, which was 20.99% more than that of OLR of 9.00 g VS/(L·d). The increase in number of reactors led to the increase of energy consumption in mixing and heat loss of reactors. However, the higher output energy of this condition makes up for this shortcoming. Compared to other scenarios, an OLR of 7.50 g VS/(L·d) and thermophilic condition generated more thermal energy (124.93 GWh/day) and electricity energy (82.45 GWh/day), which allows it to maintain the best total output energy under high energy consumption conditions. Therefore, the condition of an OLR of 7.50 g VS/(L·d) and thermophilic condition was recommended for the anaerobic digestion of OFMSW.
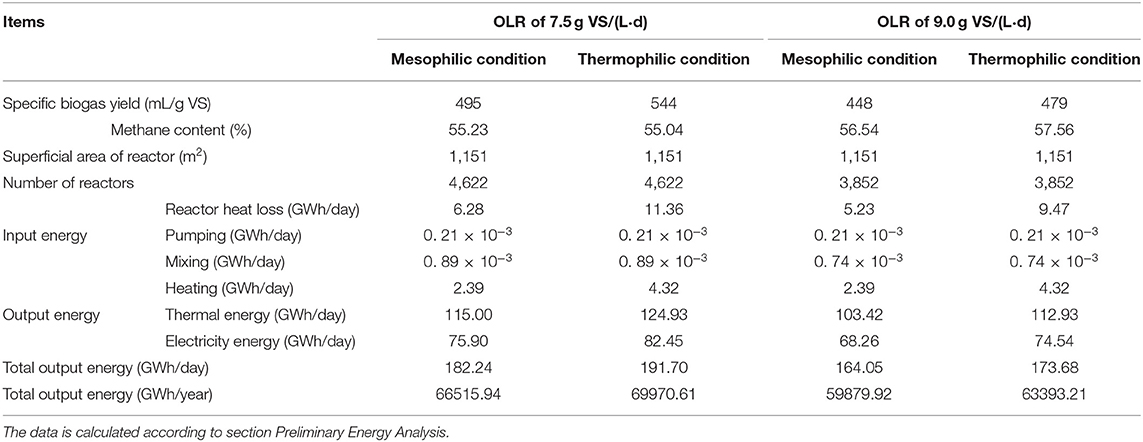
Table 3. Comparison of total output energy between OLR of 7.5 and 9.0 g VS/(L·d) at mesophilic and thermophilic condition.
Based on the specific biogas yield at the OLR of 7.50 g VS/(L·d) and thermophilic condition of this study, the MSW produced in China every year could produce 16.52 billion m3 of biogas. The thermal energy and electricity energy converted from biogas were 45599.00 GWh and 30095.34 GWh, respectively. The net total output energy is ~69970.61 GWh. Accodingly, the output energy varied with the yield of MSW (Figure 5B). Among them, the province of Guangdong had the largest energy production potential of 9305.69 GWh, with the output thermal and electricity energy of 5605.84 and 3699.85 GWh, respectively. Meanwhile, the districts of Jiangsu, Zhejiang and Shandong had the energy production potential of 6104.21, 5118.57, and 5599.60 GWh, respectively. Whereas, the potential in output energy of Hainan, Tibet, Gansu, Qinghai, and Ningxia was <1000.00 GWh.
Conclusions
The semi-continuous experiments, under mesophilic and thermophilic conditions, showed that the anaerobic digestion of OFMSW was stable at OLRs of 0.75–9.00 g VS/(L·d). The volumetric biogas production increased with OLR during stable operation. At an OLR of 7.5 g VS/(L·d), the system exhibited the maximum specific biogas yield, recorded at 495 ± 56 and 544 ± 55 mL/g VS for mesophilic, and thermophilic conditions, respectively. Based on the annual quantity of OFMSW of 144,123 kilotons produced in China, the potential for biogas production is about 16.52 billion m3, corresponding to a net total output energy of 69970.61 GWh.
Data Availability Statement
The data used to support the findings of this study are available from the corresponding author upon request.
Author Contributions
JJ, SH, TX, and XK performed the experiments and analyzed the data. LL, YG, YS, and ZY designed the experiment, prepared and revised the manuscript.
Funding
This work was supported financially by the Strategic Priority Research Program of the Chinese Academy of Sciences [Grand No. XDA21050400]; the Science and Technology Planning Project of Guangdong Province [Grant No. 2017A050501049].
Conflict of Interest
The authors declare that the research was conducted in the absence of any commercial or financial relationships that could be construed as a potential conflict of interest.
Supplementary Material
The Supplementary Material for this article can be found online at: https://www.frontiersin.org/articles/10.3389/fenrg.2020.00089/full#supplementary-material
References
Abad, V., Avila, R., Vicent, T., and Font, X. (2019). Promoting circular economy in the surroundings of an organic fraction of municipal solid waste anaerobic digestion treatment plant: Biogas production impact and economic factors. Bioresour. Technol. 283, 10–17. doi: 10.1016/j.biortech.2019.03.064
Arafat, H. A., Jijakli, K., and Ahsan, A. (2015). Environmental performance and energy recovery potential of five processes for municipal solid waste treatment. J. Clean. Prod. 105, 233–240. doi: 10.1016/j.jclepro.2013.11.071
Barbot, Y. N., Al-Ghaili, H., and Benz, R. (2016). A review on the valorization of macroalgal wastes for biomethane production. Mar. Drugs. 14:E120. doi: 10.3390/md14060120
Breitenmoser, L., Dhar, H., Gross, T., Bakre, M., Huesch, R., Hugi, C., et al. (2018). Methane potential from municipal biowaste: Insights from six communities in Maharashtra, India. Bioresour. Technol. 254, 224–230. doi: 10.1016/j.biortech.2018.01.074
Buswell, A. M., and Boruff, C. S. (1932). The relation between the chemical composition of organic matter and the quality and quantity of gas produced during sludge digestion. Sewage Work. J. 4, 454–460.
Callan, S. J., and Thomas, J. M. (2001). Economies of scale and scope: a cost analysis of municipal solid waste services. Land Econ. 77:548. doi: 10.2307/3146940
Capson-Tojo, G., Rouez, M., Crest, M., Steyer, J.-P., Delgenès, J.-P., and Escudié, R. (2016). Food waste valorization via anaerobic processes: a review. Rev. Environ. Sci. Bio. 15, 499–547. doi: 10.1007/s11157-016-9405-y
Chen, R., Wen, W., Jiang, H., Lei, Z., Li, M., and Li, Y. Y. (2019). Energy recovery potential of thermophilic high-solids co-digestion of coffee processing wastewater and waste activated sludge by anaerobic membrane bioreactor. Bioresour. Technol. 274, 127–133. doi: 10.1016/j.biortech.2018.11.080
Choi, Y., Ryu, J., and Lee, S. R. (2020). Influence of carbon type and carbon to nitrogen ratio on the biochemical methane potential, pH, and ammonia nitrogen in anaerobic digestion. J. Anim. Sci. Technol. 62, 74–83. doi: 10.5187/jast.2020.62.1.74
Feiz, R., Ammenberg, J., Björn, A., Guo, Y., Karlsson, M., Liu, Y., et al. (2019). Biogas potential for improved sustainability in guangzhou, China—a study focusing on food waste on Xiaoguwei Island. Sustainability 11:1556. doi: 10.3390/su11061556
Ferrer, I., Vazquez, F., and Font, X. (2010). Long term operation of a thermophilic anaerobic reactor: Process stability and efficiency at decreasing sludge retention time. Bioresour. Technol. 101, 2972–2980. doi: 10.1016/j.biortech.2009.12.006
Ganesh Saratale, R., Kumar, G., Banu, R., Xia, A., Periyasamy, S., and Dattatraya Saratale, G. (2018). A critical review on anaerobic digestion of microalgae and macroalgae and co-digestion of biomass for enhanced methane generation. Bioresour. Technol. 262, 319–332. doi: 10.1016/j.biortech.2018.03.030
Hu, Y., Kobayashi, T., Qi, W., Oshibe, H., and Xu, K. Q. (2018). Effect of temperature and organic loading rate on siphon-driven self-agitated anaerobic digestion performance for food waste treatment. Waste Manag. 74, 150–157. doi: 10.1016/j.wasman.2017.12.016
Hupfauf, S., Plattner, P., Wagner, A. O., Kaufmann, R., Insam, H., and Podmirseg, S. M. (2018). Temperature shapes the microbiota in anaerobic digestion and drives efficiency to a maximum at 45 degrees C. Bioresour. Technol. 269, 309–318. doi: 10.1016/j.biortech.2018.08.106
Korai, M. S., Mahar, R. B., and Uciaili, M. A. (2018). The seasonal evolution of fruit, vegetable and yard wastes by mono, co and tri-digestion at Hyderabad, Sindh Pakistan. Waste Manag. 71, 461–473. doi: 10.1016/j.wasman.2017.09.038
Li, D., Liu, S., Mi, L., Li, Z., Yuan, Y., Yan, Z., et al. (2015). Effects of feedstock ratio and organic loading rate on the anaerobic mesophilic co-digestion of rice straw and pig manure. Bioresour. Technol. 187, 120–127. doi: 10.1016/j.biortech.2015.03.040
Li, L., Kong, X., Yang, F., Li, D., Yuan, Z., and Sun, Y. (2012). Biogas production potential and kinetics of microwave and conventional thermal pretreatment of grass. Appl. Biochem. Biotechnol. 166, 1183–1191. doi: 10.1007/s12010-011-9503-9
Li, L. H., Li, Y., Sun, Y. M., Yuan, Z. H., Lv, P. M., Kang, X. H., et al. (2018a). Influence of the feedstock ratio and organic loading rate on the co-digestion performance of pennisetum hybrid and cow manure. Energ. Fuel. 32, 5171–5180. doi: 10.1021/acs.energyfuels.8b00015
Li, Y., Jin, Y., Borrion, A., and Li, H. (2019). Current status of food waste generation and management in China. Bioresour. Technol. 273, 654–665. doi: 10.1016/j.biortech.2018.10.083
Li, Y., Li, L., Sun, Y., and Yuan, Z. (2018b). Bioaugmentation strategy for enhancing anaerobic digestion of high C/N ratio feedstock with methanogenic enrichment culture. Bioresour. Technol. 261, 188–195. doi: 10.1016/j.biortech.2018.02.069
Liu, J., Zhou, X., Wu, J., Gao, W., and Qian, X. (2017a). Heat transfer analysis of cylindrical anaerobic reactors with different sizes: a heat transfer model. Environ. Sci. Pollut. Res. Int. 24, 23508–23517. doi: 10.1007/s11356-017-9943-z
Liu, Y., Xing, P., and Liu, J. (2017b). Environmental performance evaluation of different municipal solid waste management scenarios in China. Resour. Conserv. Recycl. 125, 98–106. doi: 10.1016/j.resconrec.2017.06.005$$
Liu, Y. L., Xing, P. X., and Liu, J. G. (2017c). Environmental performance evaluation of different municipal solid waste management scenarios in China. Resour. Conserv. Recycl. 125, 98–106. doi: 10.1016/j.resconrec.2017.06.005
Lu, J., Gavala, H. N., Skiadas, I. V., Mladenovska, Z., and Ahring, B. K. (2008). Improving anaerobic sewage sludge digestion by implementation of a hyper-thermophilic prehydrolysis step. J. Environ. Manage. 88, 881–889. doi: 10.1016/j.jenvman.2007.04.020
Martinez-Sanchez, V., Hulgaard, T., Hindsgaul, C., Riber, C., Kamuk, B., and Astrup, T. F. (2016). Estimation of marginal costs at existing waste treatment facilities. Waste Manag. 50, 364–375. doi: 10.1016/j.wasman.2016.02.032
Meng, L., Xie, L., Kinh, C. T., Suenaga, T., Hori, T., Riya, S., et al. (2018). Influence of feedstock-to-inoculum ratio on performance and microbial community succession during solid-state thermophilic anaerobic co-digestion of pig urine and rice straw. Bioresour. Technol. 252, 127–133. doi: 10.1016/j.biortech.2017.12.099
Milledge, J., and Heaven, S. (2017). Energy balance of biogas production from microalgae: effect of harvesting method, multiple raceways, scale of plant and combined heat and power generation. J. Mar. Sci. Eng. 5:9. doi: 10.3390/jmse5010009
Mu, L., Zhang, L., Zhu, K., Ma, J., and Li, A. (2018). Semi-continuous anaerobic digestion of extruded OFMSW: Process performance and energetics evaluation. Bioresour. Technol. 247, 103–115. doi: 10.1016/j.biortech.2017.09.085
Nielfa, A., Cano, R., Vinot, M., Fernandez, E., and Fdz-Polanco, M. (2015). Anaerobic digestion modeling of the main components of organic fraction of municipal solid waste. Process. Saf. Environ. Prot. 94, 180–187. doi: 10.1016/j.psep.2015.02.002
Pearse, L. F., Hettiaratchi, J. P., and Kumar, S. (2018). Towards developing a representative biochemical methane potential (BMP) assay for landfilled municipal solid waste – a review. Bioresour. Technol. 254, 312–324. doi: 10.1016/j.biortech.2018.01.069
Rattanapan, C., Sinchai, L., Tachapattaworakul Suksaroj, T., Kantachote, D., and Ounsaneha, W. (2019). Biogas production by co-digestion of canteen food waste and domestic wastewater under organic loading rate and temperature optimization. Environments 6:16. doi: 10.3390/environments6020016
Rincón, B., Borja, R., González, J. M., Portillo, M. C., and Sáiz-Jiménez, C. (2008). Influence of organic loading rate and hydraulic retention time on the performance, stability and microbial communities of one-stage anaerobic digestion of two-phase olive mill solid residue. Biochem. Eng. J. 40, 253–261. doi: 10.1016/j.bej.2007.12.019
Shen, F., Li, H., Wu, X., Wang, Y., and Zhang, Q. (2018). Effect of organic loading rate on anaerobic co-digestion of rice straw and pig manure with or without biological pretreatment. Bioresour. Technol. 250, 155–162. doi: 10.1016/j.biortech.2017.11.037
Silvestre, G., Fernandez, B., and Bonmati, A. (2015). Addition of crude glycerine as strategy to balance the C/N ratio on sewage sludge thermophilic and mesophilic anaerobic co-digestion. Bioresour. Technol. 193, 377–385. doi: 10.1016/j.biortech.2015.06.098
Siqueiros, E., Lamidi, R. O., Pathare, P. B., Wang, Y., and Roskilly, A.P. (2019). Energy recovery from brewery waste: experimental and modelling perspectives. Energy Procedia 161, 24–31. doi: 10.1016/j.egypro.2019.02.054
State Statistical Bureau (2015). Guangdong Statistical Yearbook. Available online at: http://www.gdstats.gov.cn/tjnj/2016/directory/content.html?03-05
Tan, L., Nishimura, H., Wang, Y. F., Sun, Z. Y., Tang, Y. Q., Kida, K., et al. (2018). Effect of organic loading rate on thermophilic methane fermentation of stillage eluted from ethanol fermentation of waste paper and kitchen waste. J. Biosci. Bioeng. 3, 2976–2984. doi: 10.1016/j.jbiosc.2018.10.006
Taricska, J. R., Long, D. A., Chen, J. P., Hung, Y. T., and Zou, S. W. (2011). Anaerobic digestion. Handbook Environ. Eng. 8, 589–634. doi: 10.1007/978-1-60327-156-1_14
Valentino, F., Moretto, G., Gottardo, M., Pavan, P., Bolzonella, D., and Majone, M. (2019). Novel routes for urban bio-waste management: a combined acidic fermentation and anaerobic digestion process for platform chemicals and biogas production. J. Clean. Prod. 220, 368–375. doi: 10.1016/j.jclepro.2019.02.102
Veeken, A., and Hamelers, B. (1999). Effect of temperature on hydrolysis rates of selected biowaste components. Bioresour. Technol. 69, 249–254. doi: 10.1016/S0960-8524(98)00188-6
Wirth, B., Reza, T., and Mumme, J. (2015). Influence of digestion temperature and organic loading rate on the continuous anaerobic treatment of process liquor from hydrothermal carbonization of sewage sludge. Bioresour. Technol. 198, 215–222. doi: 10.1016/j.biortech.2015.09.022
Xu, R., Zhang, K., Liu, P., Khan, A., Xiong, J., Tian, F., et al. (2018). A critical review on the interaction of substrate nutrient balance and microbial community structure and function in anaerobic co-digestion. Bioresour. Technol. 247, 1119–1127. doi: 10.1016/j.biortech.2017.09.095
Zema, D. A. (2017). Planning the optimal site, size, and feed of biogas plants in agricultural districts. Biofuel. Bioprod. Biorefin. 11, 454–471. doi: 10.1002/bbb.1757
Keywords: anaerobic digestion, municipal solid wastes, temperature, OLRs, biogas yield
Citation: Jiang J, He S, Kang X, Sun Y, Yuan Z, Xing T, Guo Y and Li L (2020) Effect of Organic Loading Rate and Temperature on the Anaerobic Digestion of Municipal Solid Waste: Process Performance and Energy Recovery. Front. Energy Res. 8:89. doi: 10.3389/fenrg.2020.00089
Received: 22 December 2019; Accepted: 28 April 2020;
Published: 29 May 2020.
Edited by:
Karthik Rajendran, SRM University, IndiaReviewed by:
Mohanakrishna Gunda, Qatar University, QatarQaisar Mahmood, COMSATS University, Islamabad Campus, Pakistan
Copyright © 2020 Jiang, He, Kang, Sun, Yuan, Xing, Guo and Li. This is an open-access article distributed under the terms of the Creative Commons Attribution License (CC BY). The use, distribution or reproduction in other forums is permitted, provided the original author(s) and the copyright owner(s) are credited and that the original publication in this journal is cited, in accordance with accepted academic practice. No use, distribution or reproduction is permitted which does not comply with these terms.
*Correspondence: Yongming Sun, sunym@ms.giec.ac.cn; Lianhua Li, lilh@ms.giec.ac.cn