- 1Centre for Energy and Environment, Malaviya National Institute of Technology, Jaipur, India
- 2Department of Plant Breeding, Swedish University of Agricultural Sciences, Alnarp, Sweden
- 3Department of Botany, University of Delhi, New Delhi, India
- 4Department of Civil Engineering, National Institute of Technology, Raipur, India
- 5Department of Microbiology, School of Life Sciences, Central University of Rajasthan, Ajmer, India
Anaerobic digestion is an attractive technology for resource management of organic waste and stubble. The state of biochemical reactions and activities in anaerobic digestion determines the process stability. Biochemical activities, such as syntrophic, metabolic, catalytic, and enzymatic activities, regulate the anaerobic efficiency for improved methane yield. Inhibitors, such as access to volatile fatty acids, ammonia, sulfur, and heavy metals, may slow down the anaerobic digestion and may cause reactor failure. However, additives for various biochemical activities may help to diminish the effect of inhibitors as well as improve process stability for enhanced methane yield. This manuscript presents an overview of various additives for enhancing the biochemical activities. The overview consists of the application of (a) conductive material for improving syntrophic activity, (b) trace metals for metabolic activities, (c) nanoparticles for improving catalytic activity, metabolism, and symbiosis during AD, (d) biological additives for enzymatic action, and (e) application of zeolite for introducing cation exchange properties in anaerobic digestion. Also, a comparison of various additives as per biochemical activity has also been performed for a deeper insight into the application of additives in anaerobic digestion.
Introduction
Anaerobic digestion (AD) is practiced extensively for treatment of biodegradable waste for energy generation (here methane) (Lovarelli et al., 2019). This technology has the capability to manage organic waste, such as sludge, lignocellulosic materials, energy crops, food waste, and organic fractions of solid municipal waste, with high efficiency and minimum byproduct generation (Bhatia et al., 2018). The environmentally sound features of AD have attracted research groups worldwide to improve biogas production. Biogas has a calorific value ranging between 20 and 25 MJ/m3 and may be utilized as fuel by replacing the natural and liquid petroleum gas. Also, its application may help to curb 80% of the greenhouse gases emitted into the environment. Biodegradable waste may be handled by other technologies, such as landfills and incineration, but AD is preferred, as it helps in the reduction of waste sludge, kills pathogens, provides essential nutrients, and has less of an energy demand.
AD is a complex microbe-driven, multi-phase biochemical process. It involves mainly four biochemical phases, such as hydrolysis, acidogenesis, acetogenesis, and methanogenesis. In these phases, organic matter is metabolized by bacteria and archaea and is converted into methane and carbon dioxide. However, AD has naturally inherent complications based on feedstock type and process parameters, such as slow mass transfer if total solid is high, volatile fatty acid accumulation, C/N ratio imbalance, recalcitrance of lignocellulosic residues, ammonia inhibition, and micronutrient deficiency (Table 1). These complications may sometimes lead to slower methanogenesis as well as low energy yield apart from hampering the microbial population of the AD process. It was reported by Thanh et al. that AD of rubber processing wastewater is undesirable as a waste treatment process because of the high ammonia content and other residual matters (Thanh et al., 2016). In some cases, digester failure has been reported because of inhibitions and high hydraulic retention time, which limits the biomass washout.
Different strategies have been adopted to mitigate these complications, such as solid concentration optimization in anaerobic digesters for better mass transfer, the addition of buffering agent in the reactor to maintain pH, codigestion of substrates for a balanced C/N ratio, and pretreatment to break the lignin barrier for enhanced methanogenesis. On the other hand, supplementation of various materials as additives to the anaerobic reactors provides an economically viable solution for aforementioned problems of AD for better process stability. For example, additives that are carbon based reinforce direct interspecies electron transfer in the system and enable syntrophic relations in the reactor. Materials such as biochar or activated carbon help speed up the volatile fatty acids (VFA) consumption and provide substrates to methanogens. On the other hand, for the metabolic function of microbial cells, trace metals as an additive to the system do the required job. Elements such as iron, nickel, cobalt, and others enhance metabolic activity of methanogens, which results in enhanced methane yield. Nanoparticles of these elements have been also employed by various studies to enhance anaerobic biodegradability. Apart from these, if immobilization is required of biomass in the system, zeolite may help to achieve the goal if applied as additive to anaerobic reactor.
Additives for Improving Syntrophic Activity
In an anaerobic reactor, a balanced syntrophic relation between acidogenesis and methanogenesis provides a thermodynamically stable environment for VFA conversion (Dang et al., 2016). This conversion of VFA to lactate, acetate, ethanol, butyrate, and other intermediates by syntrophic microbes incites methanogens to consume these as substrates for methane formation along with consuming CO2/H2. Carbon-based conducting materials, on the other hand, were reported to improve the syntrophic relation between acid formation by acidogens and methane-forming microbes by interspecies electron transfer, which encompasses indirect interspecies electron transfer (IIET) and direct interspecies electron transfer (DIET) (Lovley, 2017) (Table 2). In bioreactors, H2 and HCOOH could easily be consumed by methanogens to produce methane via IIET provided partial pressure is favorable to IIET. The syntrophic environment may get hampered if partial pressure of H2 is high, and it may stop the IIET, leading to VFA accumulation and process instability. DIET, on the other hand, when it exists in the reactor, can replace H2 as the only route of interspecies electron transfer in the reactor and helps to maintain syntrophic relations between acetogens and methanogens (Viggi et al., 2017).
In DIET, the electron released by the microbes is directly captured by electron-seeking microorganisms via electrical connections established by cytochromes and conductive nanowires generated by microbes. Moreover, the addition of conductive substances into the anaerobic bioreactor may help to stimulate the DIET process for those microbes that are not able to generate nanowires for electron transfer (Figure 1). It was also reported that the speed of the electron transfer in the DIET process is around 106 times more to that in IIET (Capson-Tojo et al., 2018). In this regard, instability triggered by accumulation of VFA at a high solid concentration or organic loading rate (OLR) in the anaerobic process may be improved by DIET. Furthermore, carbon-based conducting materials, such as biochar, activated carbon, magnetite, carbon cloth, and graphene, can act as a precursor to DIET in the anaerobic reactor (Mumme et al., 2014; Lin et al., 2017). Various researchers have reported a positive impact on both methanogenesis and process stability after addition of carbon-based conducting materials for AD of food waste, ethanol, acetate, sewage sludge, and glucose (Tian et al., 2017; Baek et al., 2018; Wang et al., 2018).
Biochar
Lü et al. (2016) observed that employing biochar to an anaerobic medium helped to mitigate ammonium inhibition and enhanced the microbial growth of methanogens (Lü et al., 2016). As compared to the control, reactors using biochar as an external additive in three different size group (2–5, 0.5–1 mm, and 75–150 μm) helped to achieve reduced lag phase by 23, 23, and 6%, respectively. Also, it was observed that maximum methane production rate was 47, 23, and 44% more to that control reactor. It was concluded that selection of biochar particle size is important for enhanced methanogenesis and a rich microbial community. Mumme et al. studied the effect of the addition of biochar from pyrolysis or pyrochar and hydrothermal carbonization or hydrochar on methane production and ammonia inhibition (Mumme et al., 2014). A 32% increase in methane yield was observed in the case of hydrochar, while pyrochar showed no effect on methane yield. Also, results revealed that mild ammonia inhibition up to 2.1 g of the total ammonia nitrogen (TAN)/kg may be prevented by addition of biochar. However, both the pyrochar and hydrochar were unable to stop the strong inhibition level of ammonia in the anaerobic reactor. Citrus peel waste was reported to produce D—Limonene under the AD process, which may hamper process stability (Fagbohungbe et al., 2016). In this regards, citrus peel waste was investigated through an anaerobic digestion methane potential test by Fagbohungbe et al. (2016) by employing a biochar and various biochar mixing ratio. The coconut shell biochar, wood biochar, and rice husk biochar were mixed with citrus peel waste for the biomethane potential test for 30 days retention time. Maximum methane yield was observed to be 186 L/kg VS when the citrus peel was mixed with the coconut shell biochar, which was 21 units more to that of AD of citrus peel waste alone. Biochar prepared from dairy manure was investigated as an additive by Jang et al. (2018) for AD of dairy manure. Three different temperature regions (psychrophilic, mesophilic, and thermophilic) and three different concentration of biochar (0, 1, and 10 g/L) were selected for the experimental evaluation. Results revealed that the addition of biochar helped to lower the VFA concentration and reduced the lag phase. It was concluded that nutrients present in the dairy-manure-derived biochar, such as Ca, Mg, N, and P, may help to reduce the lag phase and enhanced methane yield. Wang et al. prepared biochar from saw dust to increase the syntrophic methane yield from dewatered activated sludge and food waste (Wang et al., 2018). Results showed that saw dust biochar helped to reduce the lag phase by 27–64% and enhanced the methanogenesis by 22–40%. In addition to these, the application of biochar alleviated the organic loading rate up to 3 g of feedstock to 1 g of inoculum and showed effective buffering capacity to VFA accumulation and pH decrease.
Crushed granules, such as 1 g VS/L, have been incorporated as an inoculation medium with 0.5–1 mm biochar as an additive and glucose (4, 6, and 8 g/L) as a substrate in the AD process by Luo et al. (2015). Results revealed that the methanogenic lag phase was reduced up to 30% for 6 g/L of glucose, and an 88% increase was observed for methane when glucose concentration was 4 g/L. Simultaneous degradation of intermediate acids has also been reported by adding biochar in anaerobic medium.
Activated Carbon
Activated carbon, a carbonaceous matter, has an amorphous structure along with high porosity and high adsorption capacity. In an anaerobic medium, activated carbon may act as an electron acceptor and may be able to endorse direct interspecies electron transfer in the reactor (Liu F. et al., 2012). Zhang et al. studied the effect of activated carbon supplementation on AD of food waste (Zhang et al., 2017). Results showed that the reactor fed with food waste and activated carbon kept a stable digestion process. Also, increasing the organic loading from 1.5 to 4.38 g VS/L/d created instability in the control reactor, while a reactor fed with activated charcoal along with food waste showed no disability during the run. Zhang et al. (2018) discovered that 15 g/L of activated carbon can provide a stable digester along with improved methane yield if the feedstock used is food waste. Also, a pilot scale AD of food waste showed 41% less methane yield when run without the addition of activated charcoal. In addition to these, Zhang et al. also revealed that abundance of Firmicutes, Elusimicrobia, and Proteobacteria increased by 70, 190, and 110%, respectively, in activated carbon added to an anaerobic reactor (Zhang et al., 2018).
Granular Activated Carbon
Accumulation of VFA is an inevitable problem during AD of complex substrates. Consumption of accumulated VFA may help to stabilize the AD process. Granular activated carbon has been reported as an adsorbing material for inhibitors and a precursor for increasing the syntrophic mechanism in the anaerobic reactors (Capson-Tojo et al., 2018). Moreover, the high electrical conductivity of granular activated carbon enables it to act as an electron acceptor in the anaerobic bioreactor as well as an electron donor after reduction (Lee et al., 2016). Capson-Tojo et al. investigated the effect of granular activated carbon on AD of food waste (Capson-Tojo et al., 2018). A total of 10 g/L of granular activated carbon was supplemented with food waste to enhance the anaerobic biodegradability. It was reported that, while the lag phase was 10 days for the reactor having used food waste as a feedstock, granular activated carbon added to the reactor had resulted in only a 2-day lag phase. This shows that consumption of acetic acid was prevalent in the presence of granular activated carbon. Also, a microbial analysis revealed the predominance of hydrogenotrophic microbes.
Effective direct interspecies electron transfer was observed by Lee et al. after adding granular activated carbon to an anaerobic reactor (Lee et al., 2016). It was reported that the methane production was 1.8 times higher when the anaerobic rector was fed with granular activated carbon. Furthermore, the pyrosequencing of the 16S rRNA gene showed enrichment of the hydrogenotrophic microorganism. Supplementation of granular activated sludge with magnetite in AD of sludge was investigated by Peng et al. (2018). It was reported that, while magnetite augmented the iron reducing bacteria in an anaerobic reactor, granular activated carbon increased the syntrophic activity between methanogenic microbes and iron-reducing bacteria. Apart from these, the total chemical oxygen demand in granular activated carbon and the magnetite fed reactor was 10 g/L less as compared to the control after 56 days of digestion period. Wastewater activated sludge shows resistance against AD because of its intrinsic property of slow fermentation. However, carbon-based materials may boost up the fermentation process of activated sludge because of their enhanced syntrophic mechanism. Yang et al. (2017) studied the effect of granular activated carbon for the AD of activated sludge. Direct interspecies electron transfer was reported to be increased after the addition of granular activated carbon to batch mode along with 31% increase in methane yield.
Carbon Cloth
In a co-culture medium, the electrical conductivity of a carbon cloth may enhance direct interspecies electron transfer (Chen et al., 2014). Zhao et al. (2017) experienced potential shifting of IHT to DIET using a carbon cloth and reported a reduced impact of acid on the AD. In another study, methanogenesis simulation has been attempted in an up flow anaerobic sludge blanket (UASB) reactor by employing a carbon cloth (Lei et al., 2016). Results showed that reactors with a carbon cloth enabled 1.34-fold more organic loading than that of the control. Also, transfer of VFA was effective in the presence of a carbon cloth. A microbial community analysis of the reactor with carbon cloth revealed that extracellular bacteria and methanogenic microbes were enriched, which enables reactor to be capable of a higher organic load. In a similar study, carbon cloth used to enhance the treatment of brewery wastewater in anaerobic membrane bioreactors (Jia et al., 2020). A highly conductive carbon cloth was tested against a polyester cloth and showed that it can sustain high organic loading (up to 10,000 mg/L COD). Also, it was thought that carbon cloth supported the growth of Methanothrix in the reactor.
Iron Oxide (Magnetite)
Magnetite (Fe3O4), an iron oxide particle that is known to have conductive properties and may help to enable the interspecies electron transfer between bacteria and archaea. Cruz Viggi et al. examined micro-sized magnetite for sludge degradation via a robust syntrophic route (Viggi et al., 2017). Results showed that methane production was increased by 33% when using propionate as a substrate. In another study, acetate and propionate have been investigated as substrates in combination with magnetite at a thermophilic temperature (Yamada et al., 2015). Results of the microbial community analysis showed that the bacterial population in the reactor changed considerably. These outcomes endorse the fact that addition of magnetite will enhance the capacity of anaerobic rector to consume VFA rapidly and may help to reduce the acid stress within. In this regard, dairy wastewater was investigated for methane production, and magnetite was used as additive for the process (Baek et al., 2015). As per our results, the removal of chemical oxygen demand (COD) in the magnetite fed batch reactor was 82.5%, while in control it was 31.9%, and all the removed COD was recovered as biogas in the magnetite fed reactor. A total of 65.5 kJ of extra energy was produced from magnetite fed reactor as compared to the control. It was anticipated by the research group that the syntrophic relation between Methanosaeta concilii and Trichococcus was responsible for the enhanced methanogenic activity in the reactor. In the continuation of above discussed batch experiment, Baek et al. (2016) examined the syntrophic relation between exoelectrogenic microbes and methanogenic microbes in a continuous reactor utilizing cheese whey as a substrate for 376 days. Results showed that the addition of magnetite significantly helped to improve the process stability and methane yield. Also, after the addition of magnetite, VFA concentration was nearly zero throughout the process, which shows that conducting material consumes VFA as a substrate and provides a substrate for methanogenic microbes. Methanosaeta was the predominant methanogen in the reactor fed with magnetite.
AD of incineration leachate waste may be detrimental since it encompasses toxic compounds and salts, which may inhibit the microbial population. Adsorption of toxic compounds of incineration waste leachate may be performed by magnetite. Lei et al. (2018) employed magnetite as an additive while digesting incinerated municipal solid waste leachate anaerobically for methanogenesis. Results showed that addition of magnetite enhanced the microbial diversity in the reactor. As per results, Methanosarcina and Methanosaeta were discovered in abundance in a magnetite fed reactor along with improved COD removal and methane yield.
Additives for Improving Metabolic Activity
The most critical phase involved in AD out of four other phases is methanogenesis, which is accomplished by a pool of microbes termed as archaea. These pools of microbes require the presence of metals as micronutrients for their cell metabolic function (Figure 2A). Apart from major elements such as carbon, hydrogen, nitrogen, and phosphorus, other elements like iron, nickel, cobalt, zinc, molybdenum, and copper help to achieve effective metabolic activity within the cell of these anaerobes (Table 3). Elemental configuration of various methanogens was studied by Scherer et al. (1983), and results revealed that trace elements are required as building blocks for these microbes. Scherer et al. (1983) recommended an order of these elements as Fe >> Zn > Ni > Cu ≈ Co ≈ Mo > Mn for metabolic activity of methanogenic microbes. Zhang et al. (2003) conducted an experiment on the minimum requirement of elements for the optimum cell density of methanogens. Results showed that when the mass of methanogens was 1.32 g/L, a scarcity of Fe was observed. Also, when methanogenic microbes will cross a threshold of mass in the liquid medium, elemental shortage will be experienced in the cell mass. For instance, it was 30, 4.8, 0.12, and 1.13 g/L for Co, Ni, Cu, and Zn respectively.
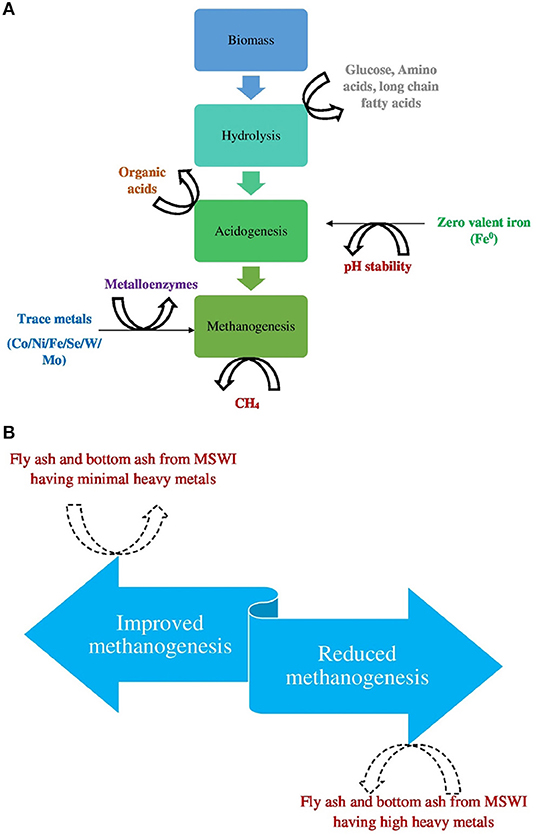
Figure 2. (A) Mechanism of metal-based additives in anaerobic digestion. (B) Municipal solid waste incineration ash as additive in anaerobic digestion.
Biochemical routes for methanogenic activity consist of the acetoclastic route or CO2/H2 route. The acetoclastic route in which acetate works as feedstock for methane formation accounts for two-thirds of methanogenesis while one-third part of methanogenesis is carried out by CO2/H2 route. These biochemical routes for methane production require metals for enzymatic activity, which may differ as per the adopted biochemical route inside the anaerobic digester, and the metal requirement may summed up as Fe >> Ni > Co > Zn = Mo/W (Glass and Orphan, 2012).
Nickel
Nickel is required for the growth of methanogenic microbes for enhanced methane production. Diekert et al. (1981) investigated methanogenic microbes for nickel requirement. Results showed that all the methanogenic microbes examined exploited nickel for their growth and synthesized factor F430. In addition to this, Speece et al. (1983) also reported a positive relationship between nickel and Methanosarcina while assaying acetate-enriched methanogens. It was also reported that, in the absence of nickel, the acetate utilization rate was around 3 g/g VSS/day, while this utilization rate increased up to 10 g/g VSS/day in the presence of nickel.
Source-segregated food waste was tested in batch assay mode along with trace elements supplementation (Facchin et al., 2013). Four different concentrations of nickel were added to the batch trials as 5,10, 20, and 100 mg/kg total solid (TS). Results showed that when nickel was present in the inoculation medium, the addition of nickel as a trace element showed negative impact on methane beyond 5 mg/kg TS to the bath bioreactor, and a 6% decrease was observed in the specific methane yield at 100 mg/kg TS concentration. On the other hand, when nickel was less in the inoculation medium, it showed an increase of 15% to the specific methane yield at concentration of 100 mg/kg TS. Batch and continuous AD of food waste was attempted by Qiang et al. (2012) with nickel as an additive. It was reported that 1 mg/L of nickel along with iron and cobalt showed improvement on methane yield. Required value of nickel was reported in association with COD of food waste as Ni/COD, and the value was 5.70 mg/kg for enhanced methanogenesis of food waste. Apart from food waste, Maze silage was supplemented with nickel in AD system for improved methane yield by Evranos and Demirel (2015). Addition of 0.1 mg/L of nickel showed positive impact on TS and VS removal (0.2 and 0.5% increase) of maze silage. Also, a total of 27% increase was observed in methane yield of maze silage.
Cobalt
Cobalt has been reported as a growing factor of acetogenic microbes by which the acetogenesis process may boost ending with enhanced overall reactor performance (Boonyakitsombut et al., 2002). For efficient AD of grass silage, Jarvis et al. (1997) discovered that 0.2 mg/L concentration of copper was essential. It was also reported that problem of low specific methane yield and decrease in pH could substantially be improved if trace elements were added daily in very small amount. Also, the addition of cobalt as an additive helped to increase organic loading rate up to 7 g VS/L/d.
Gustavsson et al. (2013) examined the effect of cobalt addition and its bioavailability for AD of silage, a sulfur-rich feedstock for biomethane production. A total of six semi-continuous anaerobic reactors were tested for the experiment. Results showed that around 20% of cobalt was easily available to the microbes for enzymatic activity. Also, a microbial community analysis revealed that supplementation of cobalt enhanced the acetate utilizing activity, while its limitation endorsed VFA accumulation and hydrogenotrophic action. Pobeheim et al. (2010) tested xylan and starch as feedstock for AD along with copper as trace elements under a mesophilic condition. As per the experimental result, an acceptable concentration of copper was 0.4 to 2 μM for the significant enhancement of methane production to be greater than that of the control. Bougrier et al. (2018) suggested that cobalt is not necessary for the optimum performance of anaerobic rector digesting brewery spent waste. This experiment advocates that if feedstock is rich in cobalt then addition of cobalt may inhibit the overall process, as brewery spent waste is rich in cobalt, and the concentration was around 0.5 mg/kg TS.
Iron
The economic viability and conductivity of iron as an additive to anaerobic digesters makes it the most prominent trace element for improved methane yield (Kato et al., 2012). Liu Y. et al. (2012) examined the effect of adding iron powder to a lab-scale anaerobic wastewater treatment unit. It was reported that addition of iron powder enhanced the activity of pyruvate-ferrodoxin oxidoreductase, a key enzyme in AD. This enhanced enzymatic activity helped to reduce hydraulic retention time (HRT) from 6 to 2 h by increasing the population of acidogens. Apart from improved enzyme biochemistry, Liu et al. (2011) reported that addition of iron to anaerobic reactors creates amenable condition for better process stability by decreasing the oxidation-reduction potential (ORP).
Various iron forms have been examined for an optimized AD reactor performance, and forms of iron additives have key role in the AD process (Karri et al., 2005; Zhang et al., 2009; Park and Novak, 2013). AD efficiency and the odor problem of sewage sludge has been optimized by Park and Novak (2013) as they adopted the direct addition of iron (III). Under a mesophilic condition, the addition of ferric chloride (1.25% w/w) assisted higher VS consumption and reduced odor-causing H2S by 65%. This was explained by the fact that the metabolite bisulfide, a product of sulfate-reducing microbes, was consumed by precipitation of FeS in the anaerobic reactor. The precipitation of FeS in anaerobic reactors was also examined by Gustavsson et al. (2013). Results revealed that FeS precipitation affects the solubility of cobalt and nickel. It was suggested that bioavailability of other additives should be considered if iron is also added to the bioreactor.
Apart from enhancing the anaerobic biodegradability, iron (III) may even hamper the methanogenesis process, as revealed when stating the fact that reduction of iron (III) is thermodynamically favorable to that methanogenesis (Zhang et al., 2009). These results enforce the idea that dosing of iron (III) plays a vital role in upliftment of anaerobic reactors. In a study performed by Van Bodegom et al. (2004), inhibition of methanogenesis was observed by amorphous ferric hydroxide (Fe(OH)3). A pure culture of methanogens was adopted, and the dosing of FeOH3 ranged between 0 to 10 mM. The level of inhibition in methane production was 14 to 99%, and it was contemplated that methanosarcina barkeri demonstrated ability to reduce Fe (III). Cheng et al. (2020) studied and compared the effect of rusted iron shavings to clean iron shavings on AD of food waste and municipal sludge. Results revealed that the group having rusted iron shavings shoed 41% more methane to that of control. Also, the content of Fe (II) in the rusted iron shaving supernatant was 132% higher compared to clean iron shavings on the fifth day. So, it was thought that rusted iron could enhance the ferrous ions in the supernatant. Yu et al. (2015) investigated the effect of ferric salts (chloride, nitrate, and sulfate) on thermophilic AD of waste-activated sludge. Results revealed that FeCl3 increased the methane yield by 79%, while Fe2(SO4)3 showed no significant impact and Fe (NO3)3 inhibited the AD process. Furthermore, pyrosequencing revealed that FeCl3 dosing enriched methanosarcina by 63% along with enriching Coprothermobacter for protein degradation as compared to the control.
On the other hand, iron (0) or zero-valentiron (ZVI) may enhance the anaerobic process since it acts as an electron donor (Karri et al., 2005). Results showed that a 0.01 mm size ZVI assisted the bioreactor to yield maximum methane yield along with sulfate reduction. AD of sludge has been attempted by various researchers since it requires some sort of pretreatment to increase its bioavailability for the digestion process (Zhang et al., 2014; Liu L. et al., 2015). Different forms of ZVI have been tested for the improvement of methane yield (Liu et al., 2015b). Iron powder, clean scrap, and rusty scrap ZVI (10 g/L each) have been supplemented to an anaerobic reactor containing waste-activated sludge. Rusty scrap ZVI showed maximum improvement in methane yield, which was 30% more to that of the control bioreactor. The performances of clean iron scrap and rusty iron scrap have been compared to iron powder for the AD of waste-activated sludge (Zhang et al., 2014). The rationale behind adding iron scrap was its ability of better mass transformation as compared to iron powder. It was reported that, while iron powder increased methane yield by 14.46%, clean and rusty iron scrap increased methane by 21.28 and 29.51%, respectively. Also, a PCR–DGGE test exposed that the addition of rusty iron scrap helped to increase acetobateria along with iron-reducing bacteria. Zhen et al. (2015) also revealed that adding scrap iron (zero valent) stimulated the anaerobic digestion of waste-activated sludge. Results showed an increment of 38.3% in methane yield when 1 g/g VSS of zero-valentscrap iron was added to the reactor. Also, the rate of hydrolysis, acidogenesis, and methanogenesis improved by 7, 4, and 11%, respectively, as compared to the control.
AD of food waste may be inhibited by excess VFA and pH drop (Paritosh et al., 2018). Utilizing ZVI as VFA remediation in AD of food waste may enhance overall process stability and methane yield. Kong et al. (2016) examined the effect of adding ZVI with mixed food waste for enhanced methane yield. Both the ZVI powder (0.2 mm diameter) and ZVI scrap (2.0 cm length and 1.0 cm in width) have been tested as acid inhibitor in the reactor. Results showed that butyric acid in the reactor decreased to 0% from 30 to 40% after addition of ZVIs. Also, the VFA/alkalinity ratio was <0.1 after the addition of ZVI to the reactor. Table 4 shows summary of some studies about iron used as additives in various forms.
Molybdenum
Feedstocks selected for the AD and methane production may not have significant amounts of trace elements, and supplementation of the elements may be fruitful for the anaerobic process (Qiang et al., 2012). Maze silage was characterized prior to the AD process by Qiang et al. (2012) for trace element composition. As per the results, molybdenum in the maze silage was discovered to be 0.5 μg/g, and supplementation of molybdenum was attempted as 0.05 mg/L. Results showed that molybdenum as an additive did not show any positive impact on methanogenesis in first 30 days. However, between the time span of 30–45 days, higher methane production was observed in molybdenum fed reactor. On the contrary, in the case of food waste, the addition of molybdenum (6 mg/kg TS) to the anaerobic reactor showed significant increase (42.9%) in methane yield (Speece et al., 1983).
Metals in Combination
The addition of multiple trace elements may be fruitful for methane production because of the synergistic behavior of these elements in the anaerobic bioreactor (Speece et al., 1983; Kong et al., 2016). In a study performed by Moestedt et al. (2016), the additions of copper, nickel, iron, and their combination have been tested for enhanced methanogenesis of organic fraction of municipal solid waste (OFMSW) and slaughterhouse waste. Results showed that cobalt alone increased the acetogenesis rate and may affect the process stability in the absence of nickel. Furthermore, the addition of nickel to that reactor after 90 days stabilized the anaerobic process within 5 days by reducing VFA to 0 from 7 mg/L. VFA accumulation is detrimental to enhanced methanogenesis as well as the stable performance of the reactor, and its degradation is needed at higher rate if accumulated in the reactor. Trace elements consortia as additives may increase the consumption rate of VFA and stabilize the process (Feng et al., 2010; Wei et al., 2014; Kong et al., 2016). In this regard, Zhang et al.'s (Kong et al., 2016) anaerobic digester has been operated for 400 days along with trace element supplementation. Results showed that, in the absence of trace elements, the reactor was severely affected by VFA accumulation (30,000 mg/L at OLR of 4 g VS/L/d). On the contrary, the reactor was supplemented with trace elements in combination and showed stable performance. The methane yield observed in the reactor with trace elements was 465.4 L/kg VS, and there was no VFA accumulation. Along with VFA consumption, higher OLR may also be achieved by trace elements addition. Wei et al. (2014) discovered that addition of trace elements in combination showed higher biomass yield (3-fold) vis-à-vis the control reactor. Also, the inhibited reactor recovered after adding the trace elements as methane yield increased to 440 L/kg VS from 130 L/kg VS. This proves the fact that adding trace elements may enhance the ability of reactor to consume access VFA and enhance methanogenesis. Diekert et al. (1981) also suggested the addition of combined trace elements (combination of nickel, iron, and cobalt) for a higher VSS in the reactor. Apart from testing the effect of trace elements on mesophilic conditions, supplementing these elements in thermophilic conditions may enhance the process output. Yirong et al. (2015) conducted a test of trace element supplementation on thermophilic AD of source-segregated food waste. As per the results obtained, the reactors without trace elements showed VFA accumulation after 3–4 months of operation. Although the addition of trace elements helped to mitigate VFA accumulation, significant improvement in methane was not observed. The effect of the addition of combined trace elements has also been investigated on AD and microbial community by Feng et al. (2010) for food industry waste. The combination of nickel/molybdenum/boron and selenium/tungsten was reported as a precursor for archaeal population and Methanosarcina sp., respectively, for AD of food industrial waste. Also, an increment of 15% in methane was observed in the case of selenium/tungsten addition to the bioreactor.
Incineration waste, on the other hand, may also be used as metal combination in AD (Figure 2B). Incineration, if adopted for municipal waste, generates two types of ashes: fly ash and bottom ash, which is high in calcium oxide, silica, and minimal proportion of heavy metals (Lo et al., 2012). The addition of municipal solid waste incineration (MSWI) ash to an anaerobic reactor may reduce its performance by providing heavy metals to the system. Lo et al. (2012) studied the effect of mixing the ash of the MSW incineration with MSW in a micro form for enhanced methane yield. The proportion of ash was fixed between 3 and 20 g/g VS of MSW for the AD. Results showed that addition of 36 of bottom ash per g VS of MSW helped to enhance the methane yield by 3.5-folds. In another study, Lo et al. (2010) reported that the addition of fly and bottom ash to the anaerobic system enhanced the anaerobic performance of MSW. The bioreactors with ash showed sorter digestion time and enhanced methane yield as compared to controls. The maximum biogas yield was observed at the 20 g/L loading of fly ash, which was 222 L/kg VS. The control showed 209 L/kg VS of biogas yield. Also, the peak production rate of biogas was 6.5 and 5.5 l/kg VS for fly and bottom ash, respectively, which was 3–4 unit more compared to the control. These results showed that controlled use of incineration waste may enhance the methane production. However, sometimes the bottom ash could consist of heavy metals, which may hinder the AD process (del Valle-Zermeño et al., 2014).
Additives for Catalytic Activity
Particles within a nanometric size range are called nanoparticles and can be synthesized by many methods, such as the fragmentation of bulk materials and seed growth using chemical processes (Kiser et al., 2010). In recent years, the application of nanoparticles has been increased by their incorporation into the commercial products. The high energy storage capacity and the ability to provide more surface area has increased the research interest for nanomaterials in energy applications. The nanotechnology has found applications in energy conversion devices and integrated circuits. Due to their ability to enhance catalytic ability through a larger surface area for reactions, the nanoparticles have also garnered much interest in the field of bioenergy (Abdelsalam et al., 2017a). The addition of nano additives during the AD process (Figure 3) and its impact on methane production has been explored frequently in the recent years. These nano additives include nanoparticles of metal oxides, zero-valent nanomaterials, and carbon-based nanomaterials. Barrena et al. (2009) studied the AD test by providing the nanoparticles at a dose of 10, 16, and 18 μg mL−1 for Au, Ag, and Fe3O4 in a mixture of cellulose, as substrate, and inoculum. Results showed that Ag and Au had almost no significant effect on the bacterial activity, but Fe3O4 has a positive effect on the anaerobic bacterial group, which increased the biogas production to 557.1 L kg−1 dry matter as compared to 471 L kg−1 dry matter. Another study from Yang et al. (2012) related the effect of different concentrations (0.010 and 0.040 gL−1) of Ag element to the methane yield (0.196 and 0.185 L) from the mixture of sewage sludge and glucose. Similar results have been observed by Abdelsalam et al. (2017b) when nanoparticles of Fe and Fe3O4 were applied to digest cattle dung slurry at different concentrations (5, 10, and 20 mg L−1), this leads to the enhancement of biogas volume by 1.45 and 1.66 times at a concentration 20 mg L−1 for both Fe and Fe3O4, respectively. Otero-González et al. (2014) compared the methane production in laboratory-scale reactors with and without the addition of CuO nanoparticles. The addition of CuO nanoparticles resulted in a 15% decrease in the methane yield. The study revealed the inhibitory effect of CuO nanoparticles on the acetoclastic microbes while having no significant effect on hydrogenotrophic activities in the reactor. Luna-delRisco et al. (2011) investigated the impact of CuO and ZnO nanoparticles on biogas yield through the AD of cattle manure for 14 days. Both type of nanoparticles showcased negative implications on the biogas yield. The % decrease was enhanced with the increase in the doses of added nanoparticles. On the other hand, micro particles did not show any significant reduction in biogas yield. In this study, an increase in biogas was also observed during the last days of the experiment, which indicates that, after some days, the microbial community is acquainted with the presence of nanoparticles and their toxic effects. Furthermore, Mu and Chen (2011) investigated long-term effects of ZnO nanoparticles on the AD process for 105 days and observed the same trend of decreasing the biogas yield. The study revealed the inhibitory effects of nanoparticles on hydrolysis and the methanogenesis process, while no effect was reported on acidification stage of anaerobic digestion. Gonzalez-Estrella et al. (2013) studied the effect of CuO, ZnO, Al2O3, CeO2, Mn2O3, Fe2O3, TiO2, and SiO2 ranging from 10 to 100 nm on the acetoclastic and hydrogentrophic methanogenesis during AD of anaerobic granular sludge. The observations revealed that Mn2O3, ZnO, CeO2, and CuO reduced the methanogenesis, activities while Al2O3, Fe2O3, TiO2, and SiO2 exhibited no significant effect on the process. On the other hand, hydrogentrophic methanogenesis was negatively affected by Mn2O3, ZnO, Al2O3, Fe2O3, and CeO2 with remaining nanoparticles having no significant impact.
In contrast to the above-mentioned studies, some researchers also observed positive impacts of metal-oxide nanoparticle addition on biogas production. García et al. (2012) reported a 10% increase in biogas production after the addition of TiO2 nanoparticles of a size of 7.5 nm. Casals et al. (2014) employed the Fe3O4 nanoparticles of 7 nm, which provided the required iron ions to the microorganisms without causing any toxicity. The addition of these nanoparticles in a 100 ppm concentration resulted in a 180 and 234% increase in biogas and methane yield, respectively.
Contrary to the metal oxide nanomaterials, the zero-valent nanomaterials are reported to affect the biogas production positively by releasing electron donors that are helpful for the methanogenesis process. Su et al. (2013) enhanced the methane yield by 40.4% after the addition of 0.1% (w/w) of zero-valent iron nanoparticles of 20 nm in size during AD of activated waste sludge. The study also reported the significant improvement in biogas yield by addition of micro sized zero-valent iron particles. On the other hand, Yang et al. (2013) employed ZVI nanoparticles of a 55 nm size and observed a 20% decrease in the methane production. The % decrease in methane production reached to 70% after increasing the concentration from 1 to 30 mM. However, Gonzalez-Estrella et al. (2013) observed the inhibitory effect of zero-valent copper nano particles (1,500 mg/L) on both acetoclastic and hydrogentrophic methanogenesis.
Encapsulating metal nanoparticles with SiO2 provided positive outcomes in some studies. Al-Ahmad et al. (2014) studied the effect of encapsulated Fe, Pd, Pt, Ni, Co, Ag, and Cu nanoparticles. The highest increase of 70% was observed by adding Ni nanoparticles encapsulated with SiO2 followed by Co nanoparticles.
All these reports suggest that the size of the nanoparticles and the concentration have the greatest impact on the biogas yield where larger size and higher concentrations often cause toxicity that inhibits the methanogenesis activity.
Additives for Enhancing Enzymatic Activity
Additions of microorganisms or enzymatic preparations for replacing the physical and chemical pretreatments carried out before AD have been explored intensively in recent years. However, the additions of microorganisms or enzymes simultaneously during the AD process have been studied comparatively less. When the process of AD is improved by the microbes, it is referred to as bioaugmentation. The microbial community composition has a direct impact on the behavior and deliverables of the AD system, as the execution of all stages of AD depends on the activity of the microbes secreting the required enzymes, including the multienzyme complex of cellulosomes, which bind to the cell wall of substrates, thus causing hydrolysis of cellulose. Therefore, the performance of AD process can be regulated by manipulating or enriching the microbial community present in the digesters. When there is a process failure due to the microbial community shifts, bioaugmentation with newly added microbes can be performed to restore adequate process performance. These microbial shifts may occur during transitional stages and stress situations, such as pH drop and changes in temperature. In these cases, higher process performance can be achieved through enriching the microbial community or adding microbes with new capabilities. However, the selection of inoculum as microbial source and its loading rate is a crucial step for achieving the higher abundance of designated microbial population. Researchers mostly emphasize establishing the methanogenic community during the early stage of the AD process to achieve a stable process (Pandey et al., 2011; Heerenklage et al., 2019). The abundance of archaea responsible for the methanogenesis is considered as a key factor to improve biogas production (Traversi et al., 2012). Therefore, many studies targeted the methanogenic community to stabilize the AD process during early stages. The addition of a community rich in microbes, such as Methanosarcina, was suggested to improve the performance of the AD system during the start-up phase (Lins et al., 2014). The augmentation may be enhanced further by employing microbial cultures immobilization through various support materials such as membrane filters (Youngsukkasem et al., 2011). Apart from methanogenic microbes, hydrolytic microorganisms may also be added during AD to augment the hydrolysis of complex molecules in substrates (Costa et al., 2012). Lü, et al. (2014) investigated the impact of adding Coprothermobacter proteolyticus and methanogenic granular sludge to the hydrolysis process. The addition of Coprothermobacter proteolyticus (10% v/v) resulted in improved hydrolysis and fermentation of polysaccharides and proteins under thermophillic conditions and enhanced methane yield by stimulating syntrophy with methanogenic granulate sludge. Lignocellulolytic microorganisms were also suggested to enhance the biogas production by simultaneously treating the biomass during AD process. Tuesorn et al. (2013) employed the lignocellulolytic microbial consortium obtained from microflora present in sugarcane bagasse compost. The addition of consortium to AD resulted in a 55% increase in methane production under mesophillic conditions. Furthermore, rumen fluid was also investigated for enhancing the hydrolysis of MSW in bench scale reactor (Ganesh et al., 2010). Rumen fluid (3, 5, and 10% v/v) was added daily and on alternate days. The hydrolysis of MSW was observed to be highest after daily addition of 5% rumen fluid.
Bioaugmentation through microorganisms was also studied to handle the stress conditions during AD. Researches were specifically targeted toward ammonia stress conditions which is a frequent problem in full scale AD plants. Microbial community analysis revealed that ammonia strongly influence the activity of anaerobic microorganisms—specifically the acetoclastic methanogens (Yenigün and Demirel, 2013). In this scenario, the addition of ammonia-tolerant methanogens may provide a solution to ammonia inhibition in AD plants (Fotidis et al., 2013). Fotidis et al. (2014) added Methanoculleus bourgensis in a steady state continuous stirred tank reactor inhibited at a high ammonia level. The addition of microorganisms resulted in a 31% increase in methane yield.
The other method to augment the AD process is to add enzyme preparations the in reactors rather than the microorganisms (Figure 4). Earlier, several reports revealed improved biogas production from pretreating the lignocellulosic biomass with crude and commercial enzymes (Al-Zuhair et al., 2011; Parawira, 2012; Christy et al., 2014). However, enzymes may also be employed for treating the biomass simultaneously in the AD system (Parawira, 2012; Heerenklage et al., 2019). Adding enzymes to the AD system may be more beneficial due to the facts that it can withstand a wide range of temperature, pH, and salinity and can dodge the adverse conditions that inhibits the activity of microorganisms. Adding enzymes instead of other pretreatment methods will also be beneficial in preventing the negative effect of generated inhibitors on enzymatic activities. For instance, the furfural and phenols generated during chemical pretreatments are reported to inhibit the activity of cellulosomes during AD (Wang et al., 2019). Moreover, the smaller size and higher mobility enable the enzymes to gain higher access to the feedstock as compared to the microorganism. Donoso-Bravo and Fdz-Polanco (2013) employed a lipase enzyme as an additive for simultaneously treating grease trap in AD system. The addition of the enzyme (0.33% v/v) resulted in a 23% increase in methane production compared to the reactors running without lipase. The study also revealed that the methane yield increased with an increase in the concentration of lipase enzyme.
Apart from adding enzymes, biochemical compounds may also be added to enhance the enzymatic activity. In this regard, the addition of organic acid anions viz. acetate, formate, and lactate have been reported to act as promotors to increase the activity of cellulosomes during AD below the respective concentrations of 200, 100, and 50 mM. However, addition beyond these critical concentrations turned out to be inhibitory for the enzymatic activity of cellulosomes (Xu et al., 2010).
Additives for Enhancing Cation Exchange Activity
Surface-active materials, such as zeolite, have the ability to influence the microbial population and its transformation in the anaerobic medium (Milán et al., 2003). Apart from this, zeolite has been discovered as microbial support system in AD of various substrate (Weiß et al., 2011; Liu L. et al., 2015). The primary characteristics of zeolite, which makes it attractive for AD, are immobilizing capacity of microbes and improving the NH3/ equilibrium in the system (Weiß et al., 2011) (Figure 5). Colonization of clinoptilolites, an activated zeolite, has been investigated by Weiß et al. (2011) in AD of grass silage as carriers of microbes. The size of the zeolite introduced to the 500 mL vials was around 2.5 mm. As per the results of single-strand conformation polymorphism, the zeolite was favored by structurally distinct microbes in the system as a favorable environment. Acetate and methanol were employed by Milán et al. (2003) as carbon sources in the AD process in the presence of natural as well as modified zeolite. Results showed that not only methanogenic microbes got enriched, but hydrolytic microorganisms also flourished rapidly in presence of zeolite. Apart from this, the kinetic constant was double to that of control reactors without zeolite. In a second phase of the experiment, Milán et al. (2003) examined the effect of zeolites on AD of piggery waste. As per the results, Magnesium, cobalt, and nickel zeolites showed an increment of 8.5, 4.4, and 2.8 times, respectively, in specific methane yield. The effect of zeolite addition was investigated by Milán et al. (2010) for AD of anaerobic flocculent and granular sludge. It was reported that the sludge selected as a substrate for AD was highly inhibited by ammonia, and the addition of zeolite (0.01 to 0.1 g/g VSS) helped to diminish the effect of ammonia in AD process. A 16S rRNA study showed that Methanococcaceae was a predominant genus, which was followed by Methanosarcina and Methanosaeta in the AD reactor supplemented with zeolite. Montalvo et al. (2012) studied the effect of zeolite addition on AD of synthetic wastewater in a mesophilic temperature zone (25–7°C). Three different runs have been conducted for the COD: N: P ratio of 500:5:1. In the first run, the F/M ratio increased from 0.21 to 0.41, and the temperature was kept constant at 27°C, while in second run, temperature increased from 27 to 37°C with the previous F/M ratio. However, during third run, F/M increased up to 1.92. Results showed that the reactors supplemented with zeolite (3 g each) had high methane yield, COD removal (93%), and ammonia mitigation (70%).
Apart from synthetic substrates, natural feedstocks have also been examined for improved methane yield in presence of zeolite. Wijesinghe et al. (2018) conducted an experiment with Australian zeolite and swine manure for enhanced methane yield. It was reported by the authors that low C/N ratio of swine manure may hamper the anaerobic process, and addition of zeolite may help to curb process instability. A total of 40 g/L of zeolite addition showed maximum methane yield among other concentration (0, 10, 70, and 100 mg/L), and methane yield was 29% higher to that of control reactor. Although the increasing concentration of zeolite showed maximum reduction in (~50% at 100 g/L), the increment in methane was only 12%. As per results of Wijesinghe et al. (2018), it was evident that increasing the dose of zeolite may not be fruitful for methane yield but may have positive effect on ammonia reduction. A similar trend has been observed by Huiliñir et al. (2014) in the case of cardboard and paper waste digestion. The dosing of zeolite was 0.2, 0.5, 1, and 20 g/L and dosing between 0.2 and 1 g/L helped to increase the methane yield by 10–18 units, while 20 g/L reduced the methane yield by four units. In a batch anaerobic experiment, Lin et al. (2013) observed ammonium ion adsorption on zeolite which lead to an increment of 20% in cumulative methane yield. In another experiment, AD of poultry manure showed positive methane yield after zeolite addition despite of being an ammonia-rich feedstock (Fotidis et al., 2013). A total of 10 g/L of zeolite addition showed highest increment (80.3%) in methane yield as compared to the control. Despite adding zeolite to the mono-digestion of substrates, Wang et al. (2015) attempted codigestion of Phragmites australis, feces, and kitchen waste and supplemented clinoptilolite to the reactors. Results showed that addition of 10% of clinoptilolite (v/v) reduced the lag phase up to 0.07/d, and a 44% of increment was observed in methane yield. It was also reported that addition of clinoptilolite helped to diminish the effect of ammonia inhibition in the reactor. As an additive, zeolite may help to instigate granulation of sludge because of its capability of getting set easily and may attach biomass on its surface. In a study, hydrodynamic behavior of the expanded granular sludge bed reactor (EGSB) was improved after the addition of zeolite (Pérez-Pérez et al., 2018). Also, the biofilm agglomeration and microbe-feedstock contact were improved in EGSB because of zeolite addition. Zeolite may also be applied as a biomass immobilizer in fixed bed reactors installed for the AD process (Umaña et al., 2008; Nikolaeva et al., 2009). The AD of dairy manure was performed in a fixed bed reactor packed with a combination of zeolite and rubber column and compared to only a reactor packed with rubber column by Umaña et al. (2008). It was reported that a 40% increment was observed in methane (for HRT of 1 day) when zeolite was used as packing material of column along with rubber. Similar observation was reported by Nikolaeva et al. (2009) in the case of dairy manure where implementation of zeolite as support material improved the kinetics and methane yield by 11 and 29%, respectively.
Perspective on Additives for Biochemical Activity
Additives, if utilized in a planned manner, may alleviate the performance of an anaerobic reactor and may help to achieve financial gains. It is of great importance to look for target activity prior to select the additive (Table 5). If a feedstock is rich in simple sugar and there is a probability of increased acid stress on the bioreactor, carbon-based functional materials may reduce the acid stress via DIET and may help to maintain the microbial flora of the reactor. Apart from the adding carbon-based functional material, proper dosing is also required for the uninterrupted operation of AD reactor. For example, a study showed that, if a biochar addition is beyond 10 g/L, methane yield is decreased (Paritosh and Vivekanand, 2019). In this case, if dosing is high, not only is it a cost to the methane production, but the economy of the system will also be affected. Instead of using one carbon-based functional material, different materials, such as activated carbon or granulated carbon, may be tested for different feedstocks to assess suitability. However, not all the carbon-based functional materials can be used in each AD, such as carbon cloth. Carbon cloth, on the other hand will only be used in submerged condition to acts up to its full potential and may not be helpful in solid state or dry anaerobic digestion. Despite this, the application of carbon-based functional materials could enhance the syntrophic activity, and the specific surface area of AC is greater to that of biochar. This property of AC makes it preferred to biochar for microorganism immobilization (Fagbohungbe et al., 2017). However, when it comes to economics of the AD process, biochar beats the application of AC, and, if reuse of AC could be facilitated, it may be proved economical (Fierro et al., 2014). Biochar, on the other hand, may enhance the digestate property for field application apart from increasing methane yield since it may enhance the water holding capacity and microorganism commotion in the soil (Paritosh and Vivekanand, 2019). Carbon cloth may be used in fabricating the new anaerobic reactor treating waste for stable operation. In this way, regular addition of carbon-based material may not be needed.
Besides carbon cloth in wastewater, zeolite may also be the attractive solution because of its cation exchange property. The physical and structural property of zeolite makes it the best suited candidate for biological augmentation of wastewater. These properties have cavities of diameters ranging from 3 to 10 Å, 25 m2/g of average surface area, minimal bulk density, as well as high cation exchange capacity (Carretero and Pozo, 2009; Marty et al., 2010). Zeolite, as it has a cation exchange property, may exchange Na+ or Mg2+ with ammonium ion, subsequently reducing the proton imbalance in the reactor. However, if oxidation of ammonia is required in high-strength saline wastewater, inorganic salts may be preferred to produce salt precipitates instead of using zeolite to make the process economical and effective (Fernández et al., 2008). If required, zeolite may also be combined with TiO2 to adsorb the H2S for in situ purification of biogas (Hao et al., 2016). Care should be taken, however, if employing zeolite for adsorption of H2S, as the increment in the biomethane was only 16%, and, if feedstock non-sulfuric, it may be uneconomical.
Characterization of feedstock may help to choose required additive for further application. It will reveal the presence of micronutrient in it and required nutrient may be added to the reactor. For example, if the feedstock is rich in nickel, adding Ni to the system may not be helpful and may inhibit the process which would not be economical. Characterization also help to understand the elemental profile of biomass and whether a cocktail of metals needed or not in AD (Demirel and Scherer, 2011). Iron and nickel are the most important micronutrient for improving stimulatory effect in AD. The forms of iron, such as Fe (0) and Fe (III), can both enable the biomass solubilization in AD. Nevertheless, the risk of its precipitation and blockage of the reactor is a great concern at continuous feeding. Other than requirements, bioavailability of the trace metals is a major concern. Ortner et al. (2015) examined that around 67% of iron was theoretically not able for microbial uptake. It was suggested that codigestion of different feedstock may help to balance the trace metals requirement, such as digesting sludge and animal manure.
Nanoparticles, when added to the AD reactor, did more harm than good. The toxicity of oxides of zinc, copper, manganese, and aluminum have shown to be detrimental in AD process. Oxides of titanium and cerium have shown mixed effects on the AD process. However, oxides of the iron aa nanoparticle increased the methane yield by 234% because of the non-toxicity of ferrous and ferric ions (Casals et al., 2014). To overcome the toxic behavior of the nanoparticles, bioactive nanoparticles may be employed to reduce the toxicity on the AD reactor. Also, emphasis may be given to make additives commercially viable, as nanoparticles and biological additives are a costly affair for AD and may be a detriment to the economic feasibility of a full-scale project.
Conclusion
Reactor performance and the process stability of an anaerobic digester may be enhanced by using additives for specific biochemical activity. However, surplus additives in an anaerobic reactor may hinder the process stability. Additives based on carbon-based functional materials has been proved to enhance acid consumption in in AD and thus improve methanogenesis. Similarly, metal-based additives help in enzyme activation. Trace metals, such as Fe, Ni, and Co, have been extensively studied for improving enzyme activation as metalloenzyme. Also, Fe has been reported as cheapest trace metal for improving methane yield and process stability. Apart from trace elements, metal oxide nanoparticles may also enhance catalytic process in AD and provide trace metals for metabolic activity simultaneously. Oxides of Fe, Cu, and Zn have been proven and used for application in AD. However, synthesis of nanoparticles is costly and may not be economical for methane production. Bioaugmentation with biological additives may enhance the process but is hard to control and may severely damage the reactor. Zeolite, on the other hand, may exchange ammonium ion with sodium or magnesium ion and let anaerobic process manifest smoothly. The cation exchange property of zeolite, however, makes it undesirable for solid-state anaerobic digestion. Emphasis may be given using zeolite in dry anaerobic digestion of food, sludge, and slaughterhouse waste, as these feedstocks are rich in nitrogen.
Author Contributions
KP and VV conceptualization, methodology, writing—original draft preparation, formal analysis, investigation, resources. MY, AC, and DS data curation, visualization, reviewing and editing. NK and NP data curation, visualization, reviewing and editing. VV supervision, project administration, funding acquisition conceptualization, methodology, reviewing and editing.
Conflict of Interest
The authors declare that the research was conducted in the absence of any commercial or financial relationships that could be construed as a potential conflict of interest.
Acknowledgments
KP and MY acknowledge the Centre for Energy and Environment, Malaviya National Institute of Technology, Jaipur, for infrastructure, facilities, and their fellowships when carrying out the research. VV would like to thank the Department of Biotechnology, Ministry of Science and Technology, for the Ramalingaswami Re-Entry fellowship (No. BT/RLF/Reentry/04/2013), Government of India, for supporting this work financially.
References
Abdelsalam, E., Samer, M., Attia, Y. A., Abdel-Hadi, M. A., Hassan, H. E., and Badr, Y. (2017a). Effects of Co and Ni nanoparticles on biogas and methane production from anaerobic digestion of slurry. Energy Convers. Manage.141, 108–119. doi: 10.1016/j.enconman.2016.05.051
Abdelsalam, E., Samer, M., Attia, Y. A., Abdel-Hadi, M. A., Hassan, H. E., and Badr, Y. (2017b). Influence of zero-valentiron nanoparticles and magnetic iron oxide nanoparticles on biogas and methane production from anaerobic digestion of manure. Energy 120, 842–853. doi: 10.1016/j.energy.2016.11.137
Al-Ahmad, A. E., Hiligsmann, S., Lambert, S., Heinrichs, B., Wannoussa, W., Tasseroul, L., et al. (2014). “Effect of encapsulated nanoparticles on thermophillic anaerobic digestion,” in 19th National Symposium on Applied Biological Sciences (Liège).
Al-Zuhair, S., Ramachandran, K. B., Farid, M., Aroua, M. K., Vadlani, P., Ramakrishnan, S., et al. (2011). Enzymes in biofuels production. Enzyme Res. 2011:658263. doi: 10.4061/2011/658263
Baek, G., Kim, J., Cho, K., Bae, H., and Lee, C. (2015). The biostimulation of anaerobic digestion with (semi) conductive ferric oxides: their potential for enhanced biomethanation. Appl. Microbiol. Biotechnol. 99, 10355–10366. doi: 10.1007/s00253-015-6900-y
Baek, G., Kim, J., Kim, J., and Lee, C. (2018). Role and potential of direct interspecies electron transfer in anaerobic digestion. Energies 11:107. doi: 10.3390/en11010107
Baek, G., Kim, J., and Lee, C. (2016). A long-term study on the effect of magnetite supplementation in continuous anaerobic digestion of dairy effluent–Enhancement in process performance and stability. Bioresour. Technol. 222, 344–354. doi: 10.1016/j.biortech.2016.10.019
Barrena, R., Casals, E., Colón, J., Font, X., Sánchez, A., and Puntes, V. (2009). Evaluation of the ecotoxicity of model nanoparticles. Chemosphere 75, 850–857. doi: 10.1016/j.chemosphere.2009.01.078
Bhatia, S. K., Joo, H. S., and Yang, Y. H. (2018). Biowaste-to-bioenergy using biological methods–a mini-review. Energy Convers. Manage. 177, 640–660. doi: 10.1016/j.enconman.2018.09.090
Boonyakitsombut, S., Kim, M. I., Ahn, Y. H., and Speece, R. E. (2002). Degradation of propionate and its precursors: the role of nutrient supplementation. KSCE J. Civ. Eng. 6, 379–387. doi: 10.1007/BF02841992
Bougrier, C., Dognin, D., Laroche, C., and Rivero, J. A. (2018). Use of trace elements addition for anaerobic digestion of brewer's spent grains. J. Environ. Manage. 223, 101–107. doi: 10.1016/j.jenvman.2018.06.014
Capson-Tojo, G., Moscoviz, R., Ruiz, D., Santa-Catalina, G., Trably, E., Rouez, M., et al. (2018). Addition of granular activated carbon and trace elements to favor volatile fatty acid consumption during anaerobic digestion of food waste. Bioresour. Technol. 260, 157–168. doi: 10.1016/j.biortech.2018.03.097
Carretero, M. I., and Pozo, M. (2009). Clay and non-clay minerals in the pharmaceutical industry, Part I. Excipients and medical applications. Appl. Clay Sci. 46, 73–80. doi: 10.1016/j.clay.2009.07.017
Casals, E., Barrena, R., García, A., González, E., Delgado, L., Busquets-Fité, M., et al. (2014). Programmed iron oxide nanoparticles disintegration in anaerobic digesters boosts biogas production. Small 10, 2801–2808. doi: 10.1002/smll.201303703
Chen, S., Rotaru, A. E., Liu, F., Philips, J., Woodard, T. L., Nevin, K. P., et al. (2014). Carbon cloth stimulates direct interspecies electron transfer in syntrophic co-cultures. Bioresour. Technol. 173, 82–86. doi: 10.1016/j.biortech.2014.09.009
Cheng, J., Zhu, C., Zhu, J., Jing, X., Kong, F., and Zhang, C. (2020). Effects of waste rusted iron shavings on enhancing anaerobic digestion of food wastes and municipal sludge. J. Clean. Prod. 242:118195. doi: 10.1016/j.jclepro.2019.118195
Choi, G., Kim, J., Lee, S., and Lee, C. (2018). Anaerobic co-digestion of high-strength organic wastes pretreated by thermal hydrolysis. Bioresour. Technol. 257, 238–248 doi: 10.1016/j.biortech.2018.02.090
Christy, P. M., Gopinath, L. R., and Divya, D. (2014). A review on anaerobic decomposition and enhancement of biogas production through enzymes and microorganisms. Renew. Sustain. Energy Rev. 34, 167–173. doi: 10.1016/j.rser.2014.03.010
Costa, J. C., Barbosa, S. G., Alves, M. M., and Sousa, D. Z. (2012). Thermochemical pre-and biological co-treatments to improve hydrolysis and methane production from poultry litter. Bioresour. Technol. 111, 141–147. doi: 10.1016/j.biortech.2012.02.047
Dang, Y., Holmes, D. E., Zhao, Z., Woodard, T. L., Zhang, Y., Sun, D., et al. (2016). Enhancing anaerobic digestion of complex organic waste with carbon-based conductive materials. Bioresour. Technol. 220, 516–522. doi: 10.1016/j.biortech.2016.08.114
del Valle-Zermeño, R., Formosa, J., Prieto, M., Nadal, R., Niubó, M., and Chimenos, J. M. (2014). Pilot-scale road subbase made with granular material formulated with MSWI bottom ash and stabilized APC fly ash: environmental impact assessment. J. Hazard Mater. 266, 132–140. doi: 10.1016/j.jhazmat.2013.12.020
Demirel, B., and Scherer, P. (2011). Trace element requirements of agricultural biogas digesters during biological conversion of renewable biomass to methane. Biomass Bioenergy 35, 992–998. doi: 10.1016/j.biombioe.2010.12.022
Diekert, G. A., Konheiser, U. R., Piechulla, K. A., and Thauer, R. K. (1981). Nickel requirement and factor F430 content of methanogenic bacteria. J. Bacteriol. 148, 459–464. doi: 10.1128/JB.148.2.459-464.1981
Donoso-Bravo, A., and Fdz-Polanco, M. (2013). Anaerobic co-digestion of sewage sludge and grease trap: assessment of enzyme addition. Process Biochem. 48, 936–940. doi: 10.1016/j.procbio.2013.04.005
Evranos, B., and Demirel, B. (2015). The impact of Ni, Co and Mo supplementation on methane yield from anaerobic mono-digestion of maize silage. Environ. Technol. 36, 1556–1562. doi: 10.1080/09593330.2014.997297
Facchin, V., Cavinato, C., Fatone, F., Pavan, P., Cecchi, F., and Bolzonella, D. (2013). Effect of trace element supplementation on the mesophilic anaerobic digestion of food waste in batch trials: the influence of inoculum origin. Biochem. Eng. J. 70, 71–77. doi: 10.1016/j.bej.2012.10.004
Fagbohungbe, M. O., Herbert, B. M., Hurst, L., Li, H., Usmani, S. Q., and Semple, K. T. (2016). Impact of biochar on the anaerobic digestion of citrus peel waste. Bioresour. Technol. 216, 142–149. doi: 10.1016/j.biortech.2016.04.106
Fagbohungbe, M. O., Herbert, B. M. J., Hurst, L., Ibeto, C. N., Li, H., Usmani, S. Q., et al. (2017). The challenges of anaerobic digestion and the role of biochar in optimizing anaerobic digestion. Waste Manage. 61, 236–249. doi: 10.1016/j.wasman.2016.11.028
Feng, X. M., Karlsson, A., Svensson, B. H., and Bertilsson, S. (2010). Impact of trace element addition on biogas production from food industrial waste–linking process to microbial communities. FEMS Microbiol. Ecol. 74, 226–240. doi: 10.1111/j.1574-6941.2010.00932.x
Fernández, I., Vázquez-Padín, J., Mosquera-Corral, A., Campos, J., and Méndez, R. (2008). Biofilm and granular systems to improve Anammox biomass retention. Biochem. Eng. J. 42, 308–313. doi: 10.1016/j.bej.2008.07.011
Fierro, J., Gómez, X., and Murphy, J. D. (2014). What is the resource of second generation gaseous transport biofuels based on pig slurries in Spain? Appl. Energy 114, 783– 789. doi: 10.1016/j.apenergy.2013.08.024
Fotidis, I. A., Karakashev, D., and Angelidaki, I. (2013). Bioaugmentation with an acetate-oxidising consortium as a tool to tackle ammonia inhibition of anaerobic digestion. Bioresour. Technol. 146, 57–62. doi: 10.1016/j.biortech.2013.07.041
Fotidis, I. A., Wang, H., Fiedel, N. R., Luo, G., Karakashev, D. B., and Angelidaki, I. (2014). Bioaugmentation as a solution to increase methane production from an ammonia-rich substrate. Environ. Sci. Technol. 48, 7669–7676. doi: 10.1021/es5017075
Ganesh, G., Karthikeyan, O. P., and Joseph, K. (2010). Enhancing the hydrolysis step in anaerobic digestion of municipal solid waste using rumen fluid. Int. J. Environ. Technol. Manage. 13, 311–321. doi: 10.1504/IJETM.2010.038010
García, A., Delgado, L., Torà, J. A., Casals, E., González, E., Puntes, V., et al. (2012). Effect of cerium dioxide, titanium dioxide, silver, and gold nanoparticles on the activity of microbial communities intended in wastewater treatment. J. Hazard Mater. 199, 64–72. doi: 10.1016/j.jhazmat.2011.10.057
Glass, J., and Orphan, V. J. (2012). Trace metal requirements for microbial enzymes involved in the production and consumption of methane and nitrous oxide. Front. Microbiol. 3:61. doi: 10.3389/fmicb.2012.00061
Gonzalez-Estrella, J., Sierra-Alvarez, R., and Field, J. A. (2013). Toxicity assessment of inorganic nanoparticles to acetoclastic and hydrogenotrophic methanogenic activity in anaerobic granular sludge. J. Hazard Mater. 260, 278–285. doi: 10.1016/j.jhazmat.2013.05.029
Gustavsson, J., Yekta, S. S., Sundberg, C., Karlsson, A., Ejlertsson, J., Skyllberg, U., et al. (2013). Bioavailability of cobalt and nickel during anaerobic digestion of sulfur-rich stillage for biogas formation. Appl. Energy 112, 473–477. doi: 10.1016/j.apenergy.2013.02.009
Hao, X., Hou, G., Zheng, P., Liu, R., and Liu, C. (2016). H2S in-situ removal from biogas using a tubular zeolite/TiO2 photocatalytic reactor and the improvement on methane production. Chem. Eng. J. 294, 105–110. doi: 10.1016/j.cej.2016.02.098
Heerenklage, J., Rechtenbach, D., Atamaniuk, I., Alassali, A., Raga, R., Koch, K., et al. (2019). Development of a method to produce standardised and storable inocula for biomethane potential tests–preliminary steps. Renew. Energy. 143, 753–761. doi: 10.1016/j.renene.2019.05.037
Huiliñir, C., Quintriqueo, A., Antileo, C., and Montalvo, S. (2014). Methane production from secondary paper and pulp sludge: effect of natural zeolite and modelling. Chem. Eng. J. 257, 131–137. doi: 10.1016/j.cej.2014.07.058
Jang, H. M., Choi, Y. K., and Kan, E. (2018). Effects of dairy manure-derived biochar on psychrophilic, mesophilic and thermophilic anaerobic digestions of dairy manure. Bioresour. Technol. 250, 927–931. doi: 10.1016/j.biortech.2017.11.074
Jarvis, Å., Nordberg, Å., Jarlsvik, T., Mathisen, B., and Svensson, B. H. (1997). Improvement of a grass-clover silage-fed biogas process by the addition of cobalt. Biomass Bioenrgy 12, 453–460. doi: 10.1016/S0961-9534(97)00015-9
Jia, R., Sun, D., Dang, Y., Meier, D., Holmes, D. E., and Smith, J. A. (2020). Carbon cloth enhances treatment of high-strength brewery wastewater in anaerobic dynamic membrane bioreactors. Bioresour. Technol. 298:122547. doi: 10.1016/j.biortech.2019.122547
Karri, S., Sierra-Alvarez, R., and Field, J. A. (2005). Zero-valentiron as an electron-donor for methanogenesis and sulfate reduction in anaerobic sludge. Biotechnol. Bioenergy 92, 810–819. doi: 10.1002/bit.20623
Kato, S., Hashimoto, K., and Watanabe, K. (2012). Methanogenesis facilitated by electric syntrophy via (semi) conductive iron-oxide minerals. Environ. Microbiol. 14, 1646–1654. doi: 10.1111/j.1462-2920.2011.02611.x
Kiser, M. A., Ryu, H., Jang, H., Hristovski, K., and Westerhoff, P. (2010). Biosorption of nanoparticles to heterotrophic wastewater biomass. Water Res. 44, 4105–4114. doi: 10.1016/j.watres.2010.05.036
Kong, X., Wei, Y., Xu, S., Liu, J., Li, H., Liu, Y., et al. (2016). Inhibiting excessive acidification using zero-valent iron in anaerobic digestion of food waste at high organic load rates. Bioresour. Technol. 211, 65–71. doi: 10.1016/j.biortech.2016.03.078
Kong, X., Yu, S., Xu, S., Fang, W., Liu, J., and Li, H. (2018). Effect of Fe0 addition on volatile fatty acids evolution on anaerobic digestion at high organic loading rates. Waste Manage. 71, 719–727. doi: 10.1016/j.wasman.2017.03.019
Lee, J. Y., Lee, S. H., and Park, H. D. (2016). Enrichment of specific electro-active microorganisms and enhancement of methane production by adding granular activated carbon in anaerobic reactors. Bioresour. Technol. 205, 205–212. doi: 10.1016/j.biortech.2016.01.054
Lei, Y., Sun, D., Dang, Y., Chen, H., Zhao, Z., Zhang, Y., et al. (2016). Stimulation of methanogenesis in anaerobic digesters treating leachate from a municipal solid waste incineration plant with carbon cloth. Bioresour. Technol. 222, 270–276. doi: 10.1016/j.biortech.2016.10.007
Lei, Y., Wei, L., Liu, T., Xiao, Y., Dang, Y., Sun, D., et al. (2018). Magnetite enhances anaerobic digestion and methanogenesis of fresh leachate from a municipal solid waste incineration plant. Chem. Eng. J. 348, 992–999. doi: 10.1016/j.cej.2018.05.060
Lin, L., Wan, C., Liu, X., Lei, Z., Lee, D. J., Zhang, Y., et al. (2013). Anaerobic digestion of swine manure under natural zeolite addition: VFA evolution, cation variation, and related microbial diversity. Appl. Microbiol. Biotechnol. 97, 10575–10583. doi: 10.1007/s00253-013-5313-z
Lin, R., Cheng, J., Zhang, J., Zhou, J., Cen, K., and Murphy, J. D. (2017). Boosting biomethane yield and production rate with graphene: the potential of direct interspecies electron transfer in anaerobic digestion. Bioresour. Technol. 239, 345–352. doi: 10.1016/j.biortech.2017.05.017
Lins, P., Reitschuler, C., and Illmer, P. (2014). Methanosarcina spp., the key to relieve the start-up of a thermophilic anaerobic digestion suffering from high acetic acid loads. Bioresour. Technol. 152, 347–354. doi: 10.1016/j.biortech.2013.11.020
Liu, F., Rotaru, A. E., Shrestha, P. M., Malvankar, N. S., Nevin, K. P., and Lovley, D. R. (2012). Promoting direct interspecies electron transfer with activated carbon. Energy Environ Sci. 5, 8982–8989. doi: 10.1039/c2ee22459c
Liu, L., Zhang, T., Wan, H., Chen, Y., Wang, X., Yang, G., et al. (2015). Anaerobic co-digestion of animal manure and wheat straw for optimized biogas production by the addition of magnetite and zeolite. Energy Convers. Manage. 97, 132–139. doi: 10.1016/j.enconman.2015.03.049
Liu, Y., Wang, Q., Zhang, Y., and Ni, B.-J. (2015a). Zero-valentiron significantly enhances methane production from waste-activated sludge by improving biochemical methane potential rather than hydrolysis rate. Sci. Rep. 5:8263. doi: 10.1038/srep08263
Liu, Y., Zhang, Y., and Ni, B. J. (2015b). Zero-valentiron simultaneously enhances methane production and sulfate reduction in anaerobic granular sludge reactors. Water Res. 75, 292–300. doi: 10.1016/j.watres.2015.02.056
Liu, Y., Zhang, Y., Quan, X., Chen, S., and Zhao, H. (2011). Applying an electric field in a built-in zero-valentiron–anaerobic reactor for enhancement of sludge granulation. Water Res. 45, 1258–1266. doi: 10.1016/j.watres.2010.10.002
Liu, Y., Zhang, Y., Quan, X., Li, Y., Zhao, Z., Meng, X., et al. (2012). Optimization of anaerobic acidogenesis by adding Fe0 powder to enhance anaerobic wastewater treatment. Chem. Eng. J. 192, 179–185. doi: 10.1016/j.cej.2012.03.044
Lo, H. M., Chiu, H. Y., Lo, S. W., and Lo, F. C. (2012). Effects of micro-nano and non micro-nano MSWI ashes addition on MSW anaerobic digestion. Bioresour. Technol. 114, 90–94. doi: 10.1016/j.biortech.2012.03.002
Lo, H. M., Kurniawan, T. A., Sillanpää, M. E., Pai, T. Y., Chiang, C. F., Chao, K. P., et al. (2010). Modelling biogas production from organic fraction of MSW co-digested with MSWI ashes in anaerobic bioreactors. Bioresour. Technol. 101, 6329–6335. doi: 10.1016/j.biortech.2010.03.048
Lovarelli, D., Falcone, G., Orsi, L., and Bacenetti, J. (2019). Agricultural small anaerobic digestion plants: combining economic and environmental assessment. Biomass Bioenergy 128:105302. doi: 10.1016/j.biombioe.2019.105302
Lovley, D. R. (2017). Syntrophy goes electric: direct interspecies electron transfer. Annu. Rev. Microbiol. 71, 643–664. doi: 10.1146/annurev-micro-030117-020420
Lü, F., Li, T., Wang, T., Shao, L., and He, P. (2014). Improvement of sludge digestate biodegradability by thermophilic bioaugmentation. Appl. Microbiol. Biotechnol. 98, 969–977. doi: 10.1007/s00253-013-4977-8
Lü, F., Luo, C., Shao, L., and He, P. (2016). Biochar alleviates combined stress of ammonium and acids by firstly enriching Methanosaeta and then Methanosarcina. Water Res. 90, 34–43. doi: 10.1016/j.watres.2015.12.029
Luna-delRisco, M., Orupõld, K., and Dubourguier, H. C. (2011). Particle-size effect of CuO and ZnO on biogas and methane production during anaerobic digestion. J. Hazard Mater. 189, 603–608. doi: 10.1016/j.jhazmat.2011.02.085
Luo, C., Lü, F., Shao, L., and He, P. (2015). Application of eco-compatible biochar in anaerobic digestion to relieve acid stress and promote the selective colonization of functional microbes. Water Res. 68, 710–718. doi: 10.1016/j.watres.2014.10.052
Marty, N. C. M., Fritz, B., Clément, A., and Michau, N. (2010). Modelling the long term alteration of the engineered bentonite barrier in an underground radioactive waste repository. Appl. Clay Sci. 47, 82–90. doi: 10.1016/j.clay.2008.10.002
Milán, Z., Montalvo, S., Ilangovan, K., Monroy, O., Chamy, R., Weiland, P., et al. (2010). The impact of ammonia nitrogen concentration and zeolite addition on the specific methanogenic activity of granular and flocculent anaerobic sludges. J. Environ. Sci. Health Part A. 45, 883–889. doi: 10.1080/10934521003709099
Milán, Z., Villa, P., Sánchez, E., Montalvo, S., Borja, R., Ilangovan, K., et al. (2003). Effect of natural and modified zeolite addition on anaerobic digestion of piggery waste. Water Sci. Technol. 48, 263–269. doi: 10.2166/wst.2003.0411
Moestedt, J., Nordell, E., Yekta, S. S., Lundgren, J., Martí, M., Sundberg, C., et al. (2016). Effects of trace element addition on process stability during anaerobic co-digestion of OFMSW and slaughterhouse waste. Waste Manage. 47, 11–20. doi: 10.1016/j.wasman.2015.03.007
Montalvo, S., Gonzalez, P., Mena, C., Guerrero, L., and Borja, R. (2012). Influence of the food to microorganisms (F/M) ratio and temperature on batch anaerobic digestion processes with and without zeolite addition. J. Environ. Sci. Health Part A. 47, 1785–1794. doi: 10.1080/10934529.2012.689235
Mu, H., and Chen, Y. (2011). Long-term effect of ZnO nanoparticles on waste-activated sludge anaerobic digestion. Water Res. 45, 5612–5620. doi: 10.1016/j.watres.2011.08.022
Mumme, J., Srocke, F., Heeg, K., and Werner, M. (2014). Use of biochars in anaerobic digestion. Bioresour. Technol. 164, 189–197. doi: 10.1016/j.biortech.2014.05.008
Nikolaeva, S., Sánchez, E., Borja, R., Raposo, F., Colmenarejo, M. F., Montalvo, S., et al. (2009). Kinetics of anaerobic degradation of screened dairy manure by upflow fixed bed digesters: effect of natural zeolite addition. J. Environ. Sci. Health Part A 44, 146–154. doi: 10.1080/10934520802539715
Ortner, M., Rameder, M., Rachbauer, L., Bochmann, G., and Fuchs, W. (2015). Bioavailability of essential trace elements and their impact on anaerobic digestion of slaughterhouse waste. Biochem. Eng. J. 99, 107–113. doi: 10.1016/j.bej.2015.03.021
Otero-González, L., Field, J. A., and Sierra-Alvarez, R. (2014). Inhibition of anaerobic wastewater treatment after long-term exposure to low levels of CuO nanoparticles. Water Res. 58, 160–168. doi: 10.1016/j.watres.2014.03.067
Pandey, P. K., Ndegwa, P. M., Soupir, M. L., Alldredge, J. R., and Pitts, M. J. (2011). Efficacies of inocula on the startup of anaerobic reactors treating dairy manure under stirred and unstirred conditions. Biomass Bioenergy 35, 2705–2720. doi: 10.1016/j.biombioe.2011.03.017
Parawira, W. (2012). Enzyme research and applications in biotechnological intensification of biogas production. Crit. Rev. Biotechnol. 32, 172–186. doi: 10.3109/07388551.2011.595384
Paritosh, K., and Vivekanand, V. (2019). Biochar enabled syntrophic action: solid state anaerobic digestion of agricultural stubble for enhanced methane production. Bioresour. Technol. 289:121712. doi: 10.1016/j.biortech.2019.121712
Paritosh, K., Yadav, M., Mathur, S., Balan, V., Liao, W., Pareek, N., et al. (2018). Organic fraction of municipal solid waste: overview of treatment methodologies to enhance anaerobic biodegradability. Front. Energy Res. 6:75. doi: 10.3389/fenrg.2018.00075
Park, C. M., and Novak, J. T. (2013). The effect of direct addition of iron (III) on anaerobic digestion efficiency and odor causing compounds. Water Sci. Technol. 68, 2391–2396. doi: 10.2166/wst.2013.507
Peng, H., Zhang, Y., Tan, D., Zhao, Z., Zhao, H., and Quan, X. (2018). Roles of magnetite and granular activated carbon in improvement of anaerobic sludge digestion. Bioresour. Technol. 249, 666–672. doi: 10.1016/j.biortech.2017.10.047
Pérez-Pérez, T., Pereda-Reyes, I., Pozzi, E., Oliva-Merencio, D., and Zaiat, M. (2018). Performance and stability of an expanded granular sludge bed reactor modified with zeolite addition subjected to step increases of organic loading rate (OLR) and to organic shock load (OSL). Water Sci. Technol. 77, 39–50. doi: 10.2166/wst.2017.516
Pobeheim, H., Munk, B., Johansson, J., and Guebitz, G. M. (2010). Influence of trace elements on methane formation from a synthetic model substrate for maize silage. Bioresour. Technol. 101, 836–839. doi: 10.1016/j.biortech.2009.08.076
Qiang, H., Lang, D. L., and Li, Y. Y. (2012). High-solid mesophilic methane fermentation of food waste with an emphasis on iron, cobalt, and nickel requirements. Bioresour. Technol. 103, 21–27. doi: 10.1016/j.biortech.2011.09.036
Scherer, P., Lippert, H., and Wolff, G. (1983). Composition of the major elements and trace elements of 10 methanogenic bacteria determined by inductively coupled plasma emission spectrometry. Biol. Trace Elem. Res. 5, 149–163. doi: 10.1007/BF02916619
Speece, R. E., Parkin, G. F., and Gallagher, D. (1983). Nickel stimulation of anaerobic digestion. Water Res. 17, 677–683. doi: 10.1016/0043-1354(83)90237-3
Su, L., Shi, X., Guo, G., Zhao, A., and Zhao, Y. (2013). Stabilization of sewage sludge in the presence of nanoscale zero-valent iron (nZVI): abatement of odor and improvement of biogas production. J. Mater. Cycl. Waste Manage. 15, 461–468. doi: 10.1007/s10163-013-0150-9
Thanh, P. M., Ketheesan, B., Yan, Z., and Stuckey, D. (2016). Trace metal speciation and bioavailability in anaerobic digestion: a review. Biotechnol. Adv. 34, 122–136. doi: 10.1016/j.biotechadv.2015.12.006
Tian, T., Qiao, S., Li, X., Zhang, M., and Zhou, J. (2017). Nano-graphene induced positive effects on methanogenesis in anaerobic digestion. Bioresour. Technol. 224, 41–47. doi: 10.1016/j.biortech.2016.10.058
Traversi, D., Villa, S., Lorenzi, E., Degan, R., and Gilli, G. (2012). Application of a real-time qPCR method to measure the methanogen concentration during anaerobic digestion as an indicator of biogas production capacity. J. Environ. Manage. 111, 173–177. doi: 10.1016/j.jenvman.2012.07.021
Tuesorn, S., Wongwilaiwalin, S., Champreda, V., Leethochawalit, M., Nopharatana, A., Techkarnjanaruk, S., et al. (2013). Enhancement of biogas production from swine manure by a lignocellulolytic microbial consortium. Bioresour. Technol.144, 579–586. doi: 10.1016/j.biortech.2013.07.013
Umaña, O., Nikolaeva, S., Sánchez, E., Borja, R., and Raposo, F. (2008). Treatment of screened dairy manure by upflow anaerobic fixed bed reactors packed with waste tyre rubber and a combination of waste tyre rubber and zeolite: effect of the hydraulic retention time. Bioresour. Technol. 99, 7412–7417. doi: 10.1016/j.biortech.2008.01.009
Van Bodegom, P. M., Scholten, J. C., and Stams, A. J. (2004). Direct inhibition of methanogenesis by ferric iron. FEMS Microbiol. Ecol. 49, 261–268. doi: 10.1016/j.femsec.2004.03.017
Viggi, C. C., Simonetti, S., Palma, E., Pagliaccia, P., Braguglia, C., Fazi, S., et al. (2017). Enhancing methane production from food waste fermentate using biochar: the added value of electrochemical testing in pre-selecting the most effective type of biochar. Biotechnol. Biofuels 10:303. doi: 10.1186/s13068-017-0994-7
Wang, G., Li, Q., Gao, X., and Wang, X. C. (2018). Synergetic promotion of syntrophic methane production from anaerobic digestion of complex organic wastes by biochar: performance and associated mechanisms. Bioresour. Technol. 250, 812–820. doi: 10.1016/j.biortech.2017.12.004
Wang, X., Zhang, L., Xi, B., Sun, W., Xia, X., Zhu, C., et al. (2015). Biogas production improvement and C/N control by natural clinoptilolite addition into anaerobic co-digestion of Phragmites australis, feces and kitchen waste. Bioresour. Technol. 180, 192–199. doi: 10.1016/j.biortech.2014.12.023
Wang, Y., Leng, L., Islam, M. K., Liu, F., Lin, C. S. K., and Leu, S. Y. (2019). Substrate-related factors affecting cellulosome-induced hydrolysis for lignocellulose valorization. Int. J. Mol. Sci. 20:3354. doi: 10.3390/ijms20133354
Wei, Q., Zhang, W., Guo, J., Wu, S., Tan, T., Wang, F., et al. (2014). Performance and kinetic evaluation of a semi-continuously fed anaerobic digester treating food waste: effect of trace elements on the digester recovery and stability. Chemosphere 117, 477–485. doi: 10.1016/j.chemosphere.2014.08.060
Weiß, S., Zankel, A., Lebuhn, M., Petrak, S., Somitsch, W., and Guebitz, G. M. (2011). Investigation of mircroorganisms colonising activated zeolites during anaerobic biogas production from grass silage. Bioresour. Technol. 102, 4353–4359. doi: 10.1016/j.biortech.2010.12.076
Wijesinghe, D. T., Dassanayake, K. B., Scales, P. J., Sommer, S. G., and Chen, D. (2018). Effect of Australian zeolite on methane production and ammonium removal during anaerobic digestion of swine manure. J. Environ. Chem. Eng. 6, 1233–1241. doi: 10.1016/j.jece.2018.01.028
Wu, D., Zheng, S., Ding, A., Sun, G., and Yang, M. (2015). Performance of a zero-valentiron-based anaerobic system in swine wastewater treatment. J. Hazard Mater. 286, 1–6. doi: 10.1016/j.jhazmat.2014.12.029
Xu, C., Qin, Y., Li, Y., Ji, Y., Huang, J., Song, H., et al. (2010). Factors influencing cellulosome activity in consolidated bioprocessing of cellulosic ethanol. Bioresour. Technol. 101, 9560–9569. doi: 10.1016/j.biortech.2010.07.065
Yamada, C., Kato, S., Ueno, Y., Ishii, M., and Igarashi, Y. (2015). Conductive iron oxides accelerate thermophilic methanogenesis from acetate and propionate. J. Biosci. Bioeng. 119, 678–682. doi: 10.1016/j.jbiosc.2014.11.001
Yang, Y., Chen, Q., Wall, J. D., and Hu, Z. (2012). Potential nanosilver impact on anaerobic digestion at moderate silver concentrations. Water Res. 46, 1176–1184. doi: 10.1016/j.watres.2011.12.024
Yang, Y., Guo, J., and Hu, Z. (2013). Impact of nano zero-valentiron (NZVI) on methanogenic activity and population dynamics in anaerobic digestion. Water Res. 47, 6790–6800. doi: 10.1016/j.watres.2013.09.012
Yang, Y., Zhang, Y., Li, Z., Zhao, Z., Quan, X., and Zhao, Z. (2017). Adding granular activated carbon into anaerobic sludge digestion to promote methane production and sludge decomposition. J. Clean. Prod. 149, 1101–1108. doi: 10.1016/j.jclepro.2017.02.156
Yenigün, O., and Demirel, B. (2013). Ammonia inhibition in anaerobic digestion: a review. Process Biochem. 48, 901–911. doi: 10.1016/j.procbio.2013.04.012
Yirong, C., Heaven, S., and Banks, C. J. (2015). Effect of a trace element addition strategy on volatile fatty acid accumulation in thermophilic anaerobic digestion of food waste. Waste Biomass Val. 6, 1–2. doi: 10.1007/s12649-014-9327-2
Youngsukkasem, S., Rakshit, S. K., and Taherzadeh, M. J. (2011). Biogas production by encapsulated methane-producing bacteria. Bioresources 7, 56–65.
Yu, B., Huang, X., Zhang, D., Lou, Z., Yuan, H., and Zhu, N. (2016). Response of sludge fermentation liquid and microbial community to nano zero-valent iron exposure in a mesophilic anaerobic digestion system. RSC Adv. 6, 24236–24244. doi: 10.1039/C6RA02591A
Yu, B., Lou, Z., Zhang, D., Shan, A., Yuan, H., Zhu, N., et al. (2015). Variations of organic matters and microbial community in thermophilic anaerobic digestion of waste-activated sludge with the addition of ferric salts. Bioresour. Technol. 179, 291–298. doi: 10.1016/j.biortech.2014.12.011
Zhang, J., Zhang, L., Loh, K. C., Dai, Y., and Tong, Y. W. (2017). Enhanced anaerobic digestion of food waste by adding activated carbon: fate of bacterial pathogens and antibiotic resistance genes. Biochem. Eng. J. 5, 19–25. doi: 10.1016/j.bej.2017.09.004
Zhang, L., Keller, J., and Yuan, Z. (2009). Inhibition of sulfate-reducing and methanogenic activities of anaerobic sewer biofilms by ferric iron dosing. Water Res. 43, 4123–4132. doi: 10.1016/j.watres.2009.06.013
Zhang, L., Zhang, J., and Loh, K. C. (2018). Activated carbon enhanced anaerobic digestion of food waste–Laboratory-scale and Pilot-scale operation. Waste Manage. 75, 270–279. doi: 10.1016/j.wasman.2018.02.020
Zhang, Y., Feng, Y., Yu, Q., Xu, Z., and Quan, X. (2014). Enhanced high-solids anaerobic digestion of waste-activated sludge by the addition of scrap iron. Bioresour. Technol. 159, 297–304. doi: 10.1016/j.biortech.2014.02.114
Zhang, Y., Zhang, Z., Suzuki, K., and Maekawa, T. (2003). Uptake and mass balance of trace metals for methane producing bacteria. Biomass Bioenergy 25, 427–433. doi: 10.1016/S0961-9534(03)00012-6
Zhao, Z., Zhang, Y., Li, Y., Dang, Y., Zhu, T., and Quan, X. (2017). Potentially shifting from interspecies hydrogen transfer to direct interspecies electron transfer for syntrophic metabolism to resist acidic impact with conductive carbon cloth. Chem. Eng. J. 313, 10–18. doi: 10.1016/j.cej.2016.11.149
Keywords: additives, syntrophy, methane, biochemical activity, anaerobic digestion, inhibition
Citation: Paritosh K, Yadav M, Chawade A, Sahoo D, Kesharwani N, Pareek N and Vivekanand V (2020) Additives as a Support Structure for Specific Biochemical Activity Boosts in Anaerobic Digestion: A Review. Front. Energy Res. 8:88. doi: 10.3389/fenrg.2020.00088
Received: 09 March 2020; Accepted: 27 April 2020;
Published: 11 June 2020.
Edited by:
Karthik Rajendran, SRM University, IndiaReviewed by:
Yan Dang, Beijing Forestry University, ChinaKeikhosro Karimi, Isfahan University of Technology, Iran
Copyright © 2020 Paritosh, Yadav, Chawade, Sahoo, Kesharwani, Pareek and Vivekanand. This is an open-access article distributed under the terms of the Creative Commons Attribution License (CC BY). The use, distribution or reproduction in other forums is permitted, provided the original author(s) and the copyright owner(s) are credited and that the original publication in this journal is cited, in accordance with accepted academic practice. No use, distribution or reproduction is permitted which does not comply with these terms.
*Correspondence: Vivekanand Vivekanand, Vivekanand.cee@mnit.ac.in