- 1Department of Green Chemistry and Technology, Faculty of Bioscience Engineering, Ghent University, Ghent, Belgium
- 2Department of Agricultural Economics, Faculty of Bioscience Engineering, Ghent University, Ghent, Belgium
- 3Inagro vzw, Research and Advice in Agriculture and Horticulture, Rumbeke-Beitem, Belgium
- 4Innolab, Oostkamp, Belgium
Anaerobic pig manure digestion holds potential to contribute to a bio-based economy. This work assesses the stability of the thermophilic mono-digestion process. Thermophilic mono-digestion experiments with (i) fresh liquid pig manure and (ii) the fresh fecal fraction from source separation by a pig housing construction were conducted in semi pilot-scale continuous stirred tank reactors. The effect of separation on the digestion stability was studied by comparing thermophilic mono-digestion of fresh liquid (unseparated) and fresh source separated pig manure. Influencing factors and inhibitors were identified during the experiments. An unstable thermophilic mono-digestion process was observed for fresh liquid pig manure at a digester retention time of 60 days, due to high levels of ammonia and sulfur-containing components. Thermophilic mono-digestion of the fresh fecal fraction was more promising in terms of stability, provided enough time for digestion. In addition, the effect of low temperature (70°C) thermal pre-treatment of manure on the digestion stability was investigated. In the case of liquid pig manure, no improvement in the digestion stability was noted upon thermal pre-treatment. For the fecal fraction, the stability of the thermophilic mono-digestion process did improve. Moreover, thermal treatment and subsequent thermophilic mono-digestion of the fresh fecal fractions from two different farms with a similar pig housing construction suggested an effect of the (organic) dry matter content on the process stability.
Introduction
Pig production in Flanders, the Northern part of Belgium, has a very high density of animals per surface area. The more than 5.8 million animals in 2018 accounted for 90% of the total pig population in Belgium and were divided over approximately 3,750 farms1 Given the actual annual manure production per pig category in terms of nitrogen (VLM, 2019b) and considering the fixed composition of liquid manure from these pig categories (VLM, 2019c), the estimated annual mass of pig manure produced is close to 10 million tons.
Manure can be valorized to energy through the process of anaerobic digestion. Under anaerobic conditions, organic substrate such as manure is converted to biogas, i.e., a mixture of mainly carbon dioxide and methane, and digestate by a wide diversity of micro-organisms (Pavlostathis and Giraldo-Gomez, 1991). The produced biogas can be burned in a combined heat and power unit to supply electricity and heat, while digestate can serve as an organic fertilizer. The World Biogas Association recently stated that the total agricultural energy demand could be met by renewable energy generated from only livestock manure (Jain et al., 2019). Scarlat et al. (2018) estimated the electricity production potential by digestion of manure from livestock and poultry in Europe to be close to 58 TWh per year, only taking into account collectable manure. For the specific case of Belgium, it was nearly 1.5 TWh.
Despite the high potential for anaerobic manure digestion, this technology is not yet fully exploited in Flanders, especially not in the pig sector. If pig manure is digested, it is almost exclusively in large, centralized installations within the concept of co-digestion. In addition, the manure is usually not collected fresh, leading to a reduced biogas potential (de Buisonjé and Verheijen, 2014). About a decade ago, small-scale, decentralized digestion on farms was introduced in Flanders (Decorte et al., 2019). Farm-scale digestion comprises the conversion of on-farm organic substrate to on-farm energy in a dedicated digestion reactor, allowing digester-owning farmers to become (partly) energy self-sufficient (De Dobbelaere et al., 2015). Furthermore, digestion of fresh manure has potential to reduce the overall carbon footprint of farms, as was demonstrated for the dairy sector by Vergote et al. (2019). Note that the term farm-scale anaerobic digestion applies in this case to digesters treating <5,000 tons of proprietary organic substrates per year and having a maximum electrical capacity of 200 kW (De Dobbelaere et al., 2015).
In 2018, the majority of the 53 active farm-scale digesters in Flanders were mono-digesters treating dairy manure (Decorte et al., 2019). Most of these digesters were operated under mesophilic conditions, i.e., at temperatures ranging from 30 to 40°C (Parkin and Owen, 1986; van Lier, 1995). However, thermophilic digestion (>50°C) poses some advantages, among which an increased microbial growth rate, and thus an accelerated energy generation, as well as an enhanced digestibility (Al Seadi et al., 2008), which is important for substrates such as manure, mainly consisting of non-readily biodegradable compounds (Vavilin et al., 1996). Furthermore, despite the large pig population in Flanders, farm-scale anaerobic digesters only treating pig manure are rare, due to the challenges that arise not only during the collection of fresh pig manure but also during the digestion process itself. Pig manure is rich in nitrogen, thereby inducing a high risk for ammonia inhibition (Hashimoto, 1983; Chen et al., 2008). Furthermore, it can contain a lot of sulfuric components depending on the pig's feed ration (Chen et al., 2008; Trabue et al., 2019) and it is sensitive to foam formation (Lindorfer and Demmig, 2016). All these aspects can cause the digestion process to become unstable, resulting in a low, non-constant production of methane or even complete termination of the process. As long as process stability cannot be guaranteed, investments in this technology will be limited, leaving the renewable energy potential by pig manure digestion unused.
In Flanders, pig manure is mostly present in liquid form, with a low dry matter content and a low methane potential in terms of fresh mass (Møller et al., 2007). Thermal pre-treatment could enhance methane production by increasing the soluble organic matter content of the substrate to be digested. Both high (>100°C) and low temperature (<100°C) thermal treatment prior to anaerobic digestion have already been frequently studied for wastewater treatment excess sludge, in batch and small-scale continuous mode, ranging treatment times from several minutes to multiple days (Wang et al., 1997; Gavala et al., 2003; Valo et al., 2004; Skiadas et al., 2005; Climent et al., 2007; Appels et al., 2010). For liquid pig manure, some studies are available focusing on possible improvement of the methane production (potential) by thermal pre-treatment (Bonmatí et al., 2001; González-Fernández et al., 2008; Carrère et al., 2009; Sutaryo et al., 2014). However, thermal pre-treatment could also have a (positive) effect on digestion stability, i.e., the continuation of the anaerobic digestion process under normal conditions, without sudden changes in gas production or methane content in the gas due to limiting or inhibitory components (Drosg, 2013). Indeed, there could be hypothesized that thermal treatment partially converts foam forming agents and other (inhibitory) components before digestion, thereby limiting the risk for instability during digestion. None of the mentioned studies specifically investigated this hypothesis, while process stability is an essential prerequisite for the implementation in practice.
Manure can be separated mechanically into a liquid and solid fraction or by specific animal housing constructions into a fecal and urine fraction (Hjorth et al., 2010). In Flanders, interest in separation techniques has recently been growing because of their possible role in optimizing manure disposal (by mechanical separation; Vannecke et al., 2018) and in the reduction of ammonia emissions and odor nuisance (by source separation in the animal housing; Vermeulen, 2019). Soluble minerals, such as ammonium nitrogen, mainly accumulate in the liquid or urine fraction. Most of the organic matter is present in the solid or fecal fraction, which thus can be useful for digestion (Vannecke et al., 2018). Due to partial removal of the liquid fraction or urine, the remaining fraction has a much higher methane potential and thus energy content in terms of mass than unseparated pig manure, if fresh (Asam et al., 2011; Deng et al., 2014). However, the higher methane potential is only beneficial toward digestion if the process can be kept stable. Available results from experimental research on inhibition and stability are contradictory. Sutaryo et al. (2013) found that raw acidified liquid sow and pig slurry were inhibited by sulfide during mesophilic digestion, while this was not the case for the solid fraction obtained through mechanical separation. In contrast, Møller et al. (2007) obtained a stable thermophilic digestion process for fresh liquid pig manure as such and for fresh liquid pig manure partly substituted by its solid fraction (up to 60% on mass base), though latter process was inhibited by ammonia. Other studies on mono-digestion of (fresh) separated pig manure have mostly been conducted in view of identifying the influence of thermal pre-treatment on methane production (Rafique et al., 2010; Raju et al., 2013; Sutaryo et al., 2014). Available research is mainly focused on the solid fraction of separated pig manure instead of the fecal fraction resulting from source separation by a pig housing construction. However, such construction meets an important condition for valuable anaerobic digestion: fresh substrate (collection). Furthermore, due to inconsistency in results from previous research, it is clear that more studies on mono-digestion of fresh separated pig manure are required to assess its process stability. Moreover, the effect of thermal pre-treatment on this stability has not yet been clarified.
The objective of the current work is to study the stability of thermophilic mono-digestion of fresh pig manure, using semi pilot-scale digesters. Both fresh liquid pig manure and the fresh fecal fraction from source separation by a specific pig housing construction are digested, thereby assessing the effect of separation on the process stability. In addition, the effect of thermal pre-treatment on the digestion stability is investigated. Low temperature thermal pre-treatment (70°C) with a short treatment time (1 h) is studied to ensure high probability for an economically feasible farm-scale implementation. Influencing factors and inhibitors are identified during the experiments. In general, this study aims to gain insight into the conditions necessary for stable mono-digestion of pig manure in order to support potential future implementations on pig farms.
Materials and Methods
Substrate and Inoculum
Fresh liquid (LPM) and separated pig manure (SPM) were obtained periodically from different Flemish pig farms. Liquid pig manure is present on standard pig farms, in the manure pit below the slatted floor of the animal housing. As this specific pig housing construction does not allow for collection of fresh manure, collection took place at a more advanced facility in a manure pit with separate manure and water channels. The water channel mainly contains feed residuals, besides some manure. Such manure pit construction reduces the ammonia emitting surface and thus limits ammonia emissions. Furthermore, it enables fresh manure collection, as the channels are connected to a central pipe and can be flushed (Van Overbeke et al., 2010). Fresh excreta from the manure and water channels were collected and mixed proportionally, more specifically 4 to 1 L, to obtain representative fresh LPM for the experiments. SPM was in this case the fresh fecal fraction collected from a VeDoWS (Vermeulen Dobbelaere Welfare System) pig housing construction. Its design, a shallow manure pit with gutters and a manure scraper below the slatted floor, allows for source separation, i.e., primary separation of freshly excreted manure into a fecal and urine fraction2 This specific pig housing construction is recognized as an ammonia-reducing technique in Flanders (VLM, 2019a). Note that the substrate choice was driven by the current animal housing situation, and thus manure collection systems, in the Flemish pig sector and was aimed at enlarging pig manure digestion possibilities.
Manure was collected as fresh as possible (<2 days old) to avoid any effect of pre-digestion. The collected substrates were stored at 4°C prior to the digestion experiments. The anaerobic digestate used as inoculum was obtained from a properly running thermophilic reactor in Flanders, co-digesting mainly organic biological waste and a small fraction of manure (up to 10% of the total mass fed per year). Inoculum is necessary to expedite process start-up in the reactors (Liebetrau et al., 2019). Furthermore, a volume of inoculum (Table 2), which amounted to 10%of the total reactor volume, was added to the reactor when a temporary stop of the feeding was not sufficient for recovery and continuation of the process after instability.
Experimental Set-Up
Experiments were conducted in semi pilot-scale continuous stirred tank reactors (CSTRs) at the Flemish biogas laboratory Innolab. Four reactors were used, two in vertical configuration (CSTR 1 and 2) and two in horizontal configuration (CSTR 3 and 4) (Supplementary Figure A.1). The working volume of the CSTRs was respectively, 72 and 33 L. Every 10 min, the CSTRs were mixed for 1 min by a central shaft, rotating at 20 or 15 rpm depending on the reactor. All CSTRs were operated under thermophilic conditions (50–52°C). The reactors were fed manually once per day and up to seven days per week. Before feeding, the biogas production was read from a Drum-type gas meter (Ritter, TG 0.5 model) connected to the reactor. The gas composition, including the percentage of methane, carbon dioxide and oxygen in the gas as well as the hydrogen sulfide content, was measured by a portable gas analyzer (Geotech, Biogas 5000 model). The specific time and mass of ingoing manure were recorded at each feeding. Excess digestate was periodically removed via the overflow. The reactor set-up is further elaborated in Supplementary Material A.2.
The stability of thermophilic pig manure mono-digestion was investigated based on three experiments (Table 1). The effect of low temperature thermal pre-treatment on the digestion stability was studied in experiment 1 and 2, for LPM from farm A and SPM from farm B, respectively, by simultaneously digesting raw and pre-treated substrate. The hydraulic retention time (HRT) was varied during the experiments with SPM B1. Experiment 3 studied the digestion stability of SPM, from two different farms (farm B and C) with the same pig housing construction, after thermal treatment. By comparing the results for LPM (experiment 1) and SPM (experiment 2 and 3), the possible influence of separation on the process stability was assessed. The digestion process was defined as unstable when the relative biogas or methane production on organic dry matter base (Equation 2) or the methane content in the biogas suddenly dropped without fast recovery (Drosg, 2013), or when the biogas production process (almost) completely stopped despite daily feeding.
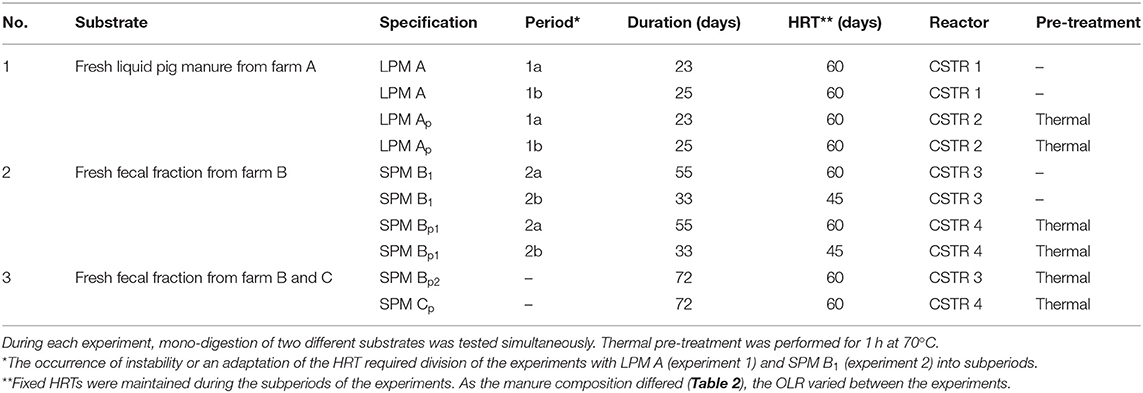
Table 1. Overview of the experiments performed to investigate the stability of thermophilic pig manure mono-digestion.
A thermal pre-treatment was performed under the hypothesis that it will partially convert foam forming agents and other (inhibitory) components before digestion, thereby limiting the risk for instability during digestion. Low temperature thermal pre-treatment with a short treatment time was studied to increase the probability for an economically feasible implementation on farm-scale. Manure was heated in an oven to a temperature of 70°C after which it was kept at that temperature for 1 h. At a temperature of 70°C, denaturation can be induced (Ichimura, 1991; Ahmed et al., 2007). Moreover, the activity of part of the thermophilic bacteria population may be improved by thermal pre-treatment at 70°C (Climent et al., 2007). During heating, manure was mixed manually every 20–30 min. Each time, about 15 kg of manure was pre-treated at once.
An HRT of 100 days was set at the beginning of the experiments to ensure good start-up and proper adaptation of the microbiology. The HRT was decreased to 60 days by increasing the daily ingoing mass of manure. A change in HRT happened gradually, meaning that the ingoing mass of manure was daily increased by 10% until the desired HRT was reached. This approach allowed for steady adaptation of the microbial community to higher loading rates.
Chemical Analysis
Raw and pre-treated manure samples were analyzed prior to reactor feeding each time after a pre-treatment was performed, i.e., up to five times depending on the length of the experiment and the necessary mass for feeding. A representative digestate sample was taken every week from each CSTR for analysis.
The substrate and digestate samples were analyzed for dry matter (DM), i.e., total solids, organic dry matter (oDM), i.e., volatile solids, total nitrogen (TN), total ammonia nitrogen (TAN) and pH. The DM content was determined gravimetrically after drying at 105°C for 24 h. The oDM content was measured based on the dried sample after incineration at 550°C for 4 h in a muffle furnace. TN was determined by Kjeldahl destruction, TAN by steam distillation, after addition of MgO to the sample, and subsequent titration (Van Ranst et al., 1999). The pH was measured with a pH-meter (PCD 6500, Eutech Instruments). Ammonia nitrogen was calculated based on the TAN, pH and temperature (Equation 4).
Besides, manure samples were analyzed for degradable carbohydrates, proteins and lipids based on the Weende analysis (Henneberg and Stohman, 1860). The biogas and methane potential were determined by the EPi test (Flash biochemical methane potential test)3 Digestate samples were additionally analyzed for volatile fatty acids (VFA), including acetic, propionic, (iso)butyric, (iso)valeric, and caproic acid, by extraction with diethyl ether and subsequent separation and detection by the GC-FID. Volatile organic acids (FOS) and total inorganic carbonates (TAC) were determined by the Nordmann method (Nordmann, 1977). Viscosity was measured by using the Brookfield method (ASTM/DIN ISO 2555).
The density of the different substrates was calculated as the ratio between the mass and the volume of a sample. For LPM substrates, the density was directly measured with a graduated cylinder of 0.250 L. For SPM substrates, having a high DM content, a graduated cylinder (0.100 L) containing demineralized water was used. The density was determined based on the added mass of manure and the volume increase due to this addition.
Calculations
The relative methane production CH4,rel (L CH4 kg−1 manure) was calculated based on the measured volume of biogas produced per day Vbiogas, daily (L biogas d−1), the measured methane content in the gas CH4,daily (L CH4 L−1 biogas) and the mass of manure daily fed to the digester mfed, daily (kg manure d−1) (Equation 1). The relative methane production on oDM base CH4,rel,oDM (L CH4 kg−1 oDM) was determined based on CH4,rel, the DM (kg DM kg−1 manure) and oDM (kg oDM kg−1 DM) content of the manure (Equation 2).
The relative efficiency αrel (%) indicates how much the methane production obtained during the experiments deviates from the biochemical methane potential of the substrate. It was calculated based on CH4,rel,oDM and the analyzed biochemical methane potential on oDM base BMPoDM (L CH4 kg−1 oDM) (Equation 3).
The ammonia nitrogen concentration NH3-N (g N kg−1 manure) was calculated based on the measured TAN concentration (g N kg−1 manure), which is the sum of ionized ammonium nitrogen () and unionized ammonia nitrogen (NH3), the prevailing digester temperature T (K) and the measured pH (–) (Equation 4; Hansen et al., 1998).
The organic loading rate (OLR) (kg oDM m−3 d−1) indicates how much organic matter enters the reactor per unit of time. This parameter thus gives an idea about possible overloading of the system. The OLR was calculated based on Equation 5 with V the working volume of the CSTR (m3).
Results and Discussion
Both fresh liquid pig manure and the fresh fecal fraction from source separation by a specific pig housing construction were mono-digested under thermophilic conditions. The average chemical composition of the used substrates and inoculum was analyzed and discussed. The stability of raw substrate mono-digestion was determined and described as such, as well as the effect of a thermal pre-treatment and of separation on the digestion stability. Based on the acquired insights, suggestions were given toward further research, focusing on the possible implementation in practice.
Characterization of Substrates
Table 2 shows the average chemical composition of the used substrates and inoculum. The average density of all substrates was close to 1 kg L−1. In general, the measured parameter values of the substrates were in line with typical values from literature.
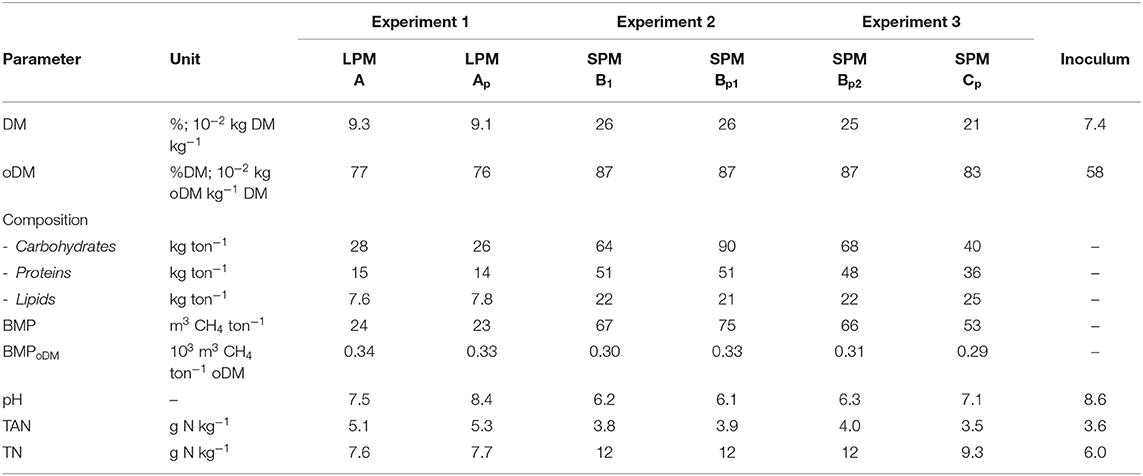
Table 2. Average chemical composition of the substrates, being fresh liquid pig manure (LPM) and the fresh fecal fraction from source separation by a specific pig housing construction (SPM), and the inoculum used for the thermophilic mono-digestion experiments.
LPM is generally characterized by a DM content below 10% and an oDM content ranging from 65 to 85% (Møller et al., 2004; Al Seadi et al., 2008; Coppens, 2009; Deublein and Steinhauser, 2009; Verdoes, 2013; Sommer et al., 2015). The DM content of separated manure depends on the technique used for separation (Hjorth et al., 2010). For the fecal fraction from the pig housing construction considered in this study, the DM content typically varies between 22 and 32%, depending on the feed ration and the season (Vermeulen, 2019). Variability in the DM content is thus common, as was the case for the used source SPM with values ranging from 21 (for SPM C) to 26% (for SPM B) (Table 2 and Supplementary Table A.1). Similar DM contents can be reached with mechanical separators, such as a screw press or decanter, depending on the machine settings and the addition of coagulants and flocculants (Verdoes, 2013; Vannecke et al., 2018). The oDM content of SPM commonly ranges between 75 and 90% (Deublein and Steinhauser, 2009; Sommer et al., 2015). The DM content of SPM was higher than that of LPM due to removal of part of the urine. Because of the difference in DM and oDM content between LPM and SPM (Table 2) and since fixed HRTs were maintained in the reactors during the subperiods (Table 1), the OLR varied during the experiments.
The protein and lipid content were in line with values proposed by Møller et al. (2004) for LPM. The proposed carbohydrate content was almost double the value in this study as besides degradable also non-degradable carbohydrates were included. The lipid content was significantly higher than the values found by Matulaitis et al. (2015) for fresh LPM and the solid fraction of SPM, which amounted to respectively 3.5 and 0.5 kg lipids per ton, probably due to dissimilarities in the pig's feed ration and the separation technique. Manure composition in terms of carbohydrates, proteins and lipids is directly related to the methane potential (Weinrich, 2019). For LPM, the methane potential typically ranges from 150 to 480 m3 per ton of oDM (Al Seadi et al., 2008; Deublein and Steinhauser, 2009; Matulaitis et al., 2015), given a methane content of 60% (Al Seadi et al., 2008). Values for SPM on oDM base are comparable to those for LPM (Deublein and Steinhauser, 2009; Matulaitis et al., 2015; Vermeulen, 2019). However, the methane potential and thus energy content in terms of mass is much higher for SPM due to (partial) removal of the urine, containing a limited amount of organic matter (Vannecke et al., 2018) and therefore having a low methane potential (Asam et al., 2011; Deng et al., 2014).
Pig manure in general has a high nitrogen content, as it is a protein-rich substrate (Chen et al., 2008; Sommer et al., 2013). In this case, 30 to 40% of the degradable fraction was related to proteins. The TAN and TN content of LPM commonly vary from 3.2 to 6.3 g N kg−1 and 6.3 to 9.9 g N kg−1, respectively (Coppens, 2009; de Haan et al., 2013; Verdoes, 2013; Sommer et al., 2015). For SPM, these values range from 2.7 to 6.0 g N kg−1 and 7.4 to 12.0 g N kg−1, respectively (de Haan et al., 2013; Sommer et al., 2015; Vermeulen, 2019). The TN content is in general higher for mechanically SPM since the mass separation efficiency is higher than the nitrogen separation efficiency from raw manure to the solid fraction (Smets et al., 2018). The TAN content is slightly lower for SPM as soluble minerals rather accumulate in the liquid than in the solid fraction (Vannecke et al., 2018). The same trends were found when comparing the values of the LPM and the source SPM used in this study. The pH of the SPM was slightly more acidic than expected, the pH of the LPM was mostly close to neutral (Sommer et al., 2015).
Thermal pre-treatment of LPM (A vs. Ap) did not change the manure composition, taking into account a mass loss of 1–2%. No significant increase in methane potential was thus induced, which contrasts with most results for substrates treated at higher temperatures or longer contact times (Wang et al., 1997; Bonmatí et al., 2001; González-Fernández et al., 2008). The obtained results were however in line with research on low temperature thermal pre-treatment such as the work of Appels et al. (2010), in which no significant solubilization of carbohydrates and proteins was detected after thermal treatment of sludge from a waste water treatment plant during 1 h at 70°C. Thermal pre-treatment of SPM did have an effect on the manure composition, though the effect was variable depending on the used manure. The protein and lipid content remained rather constant after thermal treatment. The change in carbohydrate content differed among the investigated samples (Table 2 and Supplementary Table A.1). The difference in composition between SPM Bp1 and SPM Bp2 could be attributed to the difficulty for homogeneous and representative sampling, due to the high DM content, as the composition of the not pre-treated substrates was comparable.
Stability of Raw Substrate Digestion
Fresh Liquid Pig Manure
Digestion of LPM from farm A (experiment 1) yielded an average methane production of 359 L CH4 kg−1 oDM during period 1a (Table 3). Methane production was higher than the analyzed methane potential of the substrate (Table 2), shown by an average relative efficiency exceeding 100% (Figure 1A and Table 3). This high percentage is due to the residual methane potential of the inoculum, with which the reactors were completely filled at the beginning of the experiments. The methane content remained relatively constant during the digestion period (Supplementary Figure B.1). However, after an operational period of about 1/2 of the HRT, it suddenly dropped from 66 to 55%. Likewise, the biogas production decreased by 33% (Supplementary Figure B.1), resulting in a drop in relative efficiency (Figure 1A). The sudden change in methane content and biogas production is a consequence of digester instability (Drosg, 2013). During period 1b, on average 225 L CH4 kg−1 oDM was produced, which equals to an average relative efficiency of 63% (Figure 1A and Table 3). After an operational period of about 1/3 of the HRT, instability occurred again, characterized by a drop in methane content from 61 to 46% over a few days and a 37% decreased biogas production (Supplementary Figure B.1).
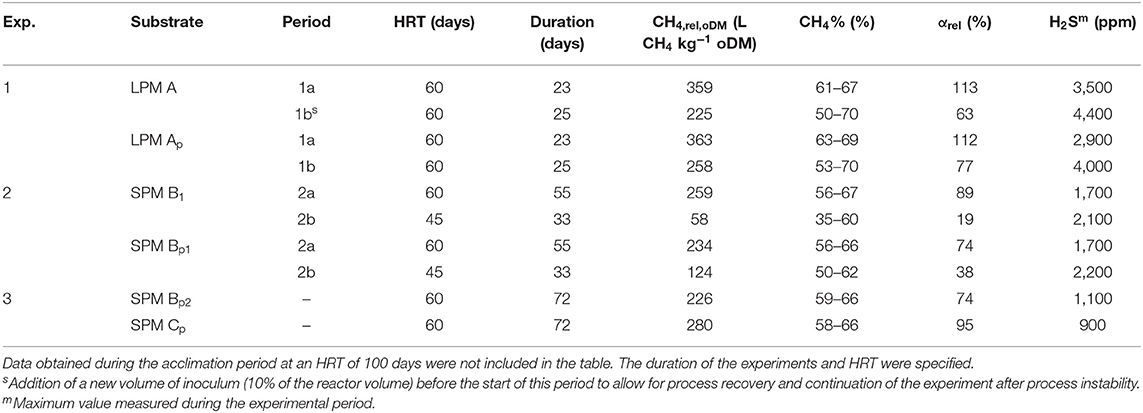
Table 3. (Average of) the measured values of the gas production and composition parameters obtained during the thermophilic mono-digestion experiments with fresh liquid pig manure (LPM) and the fresh fecal fraction from source separation by a specific pig housing construction (SPM), excluding the values before complete process failure.
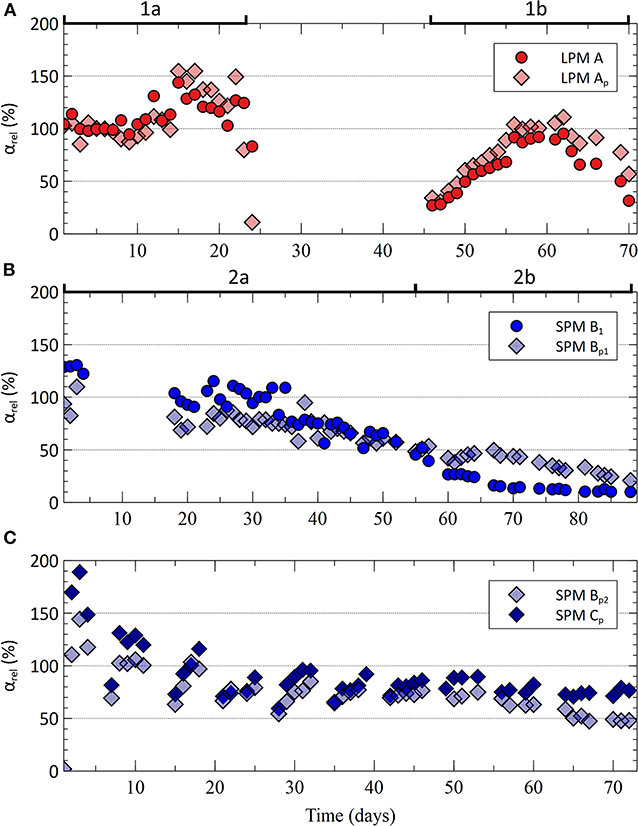
Figure 1. Relative efficiency αrel, defined as the ratio between the methane production obtained during the thermophilic mono-digestion experiments and the biochemical methane potential, on oDM base. (A) represents experiment 1 with the (not) pre-treated fresh liquid pig manure from farm A (LPM A and LPM Ap), digested at an HRT of 60 days (in period 1a and 1b), (B) experiment 2 with the (not) pre-treated fresh fecal fraction from source separation by a specific pig housing construction on farm B (SPM B1 and SPM Bp1), digested at an HRT of 60 (period 2a) and 45 days (period 2b) and (C) experiment 3 with the pre-treated fresh fecal fractions from source separation by a similar pig housing construction on farm B and C (SPM Bp2 and SPM Cp), digested at an HRT of 60 days. Data obtained during the acclimation period at an HRT of 100 days were not included in the figures.
The concentration of TAN and ammonia were high with values of respectively, 3.4 g N kg−1 and 1.5 g NH3-N kg−1 at the end of period 1a (Table 4 and Supplementary Figure B.1). During the experimental period, the levels increased by 30–55% due to mineralization of organic nitrogen and preservation of a rather constant pH (Equation 4). Angelidaki and Ahring (1994) stated that for thermophilic digestion of manure inhibition may happen at an ammonia concentration exceeding 0.7 g NH3-N kg−1. Hansen et al. (1998) found values of 1.1 g NH3-N kg−1 for thermophilic pig manure digestion. Rajagopal et al. (2013) summarized that digestion instability can be the result of TAN concentrations ranging from 1.5 to 7.0 g N kg−1. The experimental values mostly exceeded the maximum values stated in literature, suggesting the occurrence of inhibition or even intoxication. However, ammonia inhibition and intoxication remain case-specific as they depend on pH, temperature, ionic strength, type of micro-organisms and their adaptation (Chen et al., 2008). At the end of period 1b, the concentration of TAN and ammonia were even higher with values of respectively 4.1 g N kg−1 and 2.1 g NH3-N kg−1 (Table 4), resulting in more process inhibition and thus lower methane production values and relative efficiencies than during period 1a (Figure 1A).
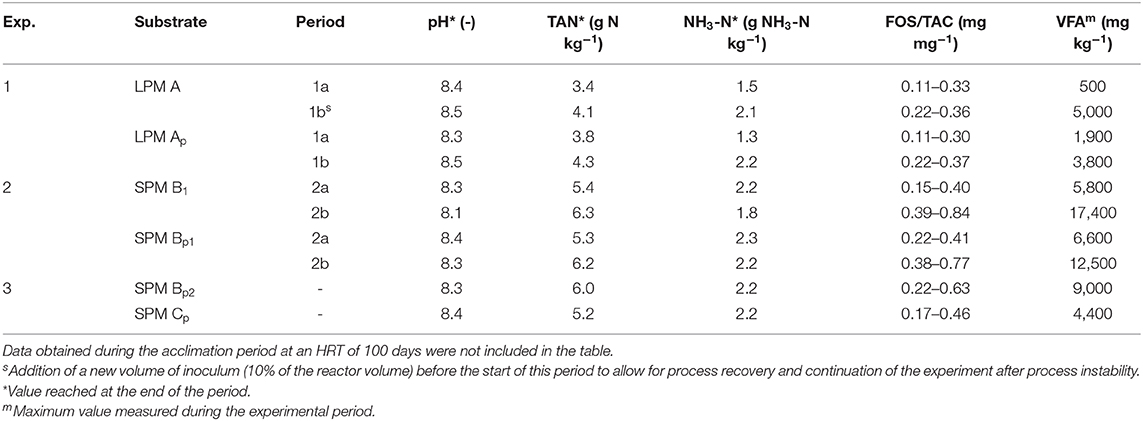
Table 4. Measured values of the digestate parameters obtained during the thermophilic mono-digestion experiments with fresh liquid pig manure (LPM) and the fresh fecal fraction from source separation by a specific pig housing construction (SPM), excluding the values before complete process failure.
H2S concentrations up to 3,500 ppm were measured in the gas during period 1a (Table 3 and Supplementary Figure B.1). An increasing H2S concentration in the gas indicates the presence of sulfur-containing components in the liquid (manure). Sulfate reducing bacteria (SRB) compete with other micro-organisms for substrate to form sulfides (Deublein and Steinhauser, 2009; Chen et al., 2014). With methanogens, they compete for H2 and acetic acid, thereby hindering methane production (Shah et al., 2014). Moreover, sulfides in the liquid, resulting from SRB activity, will at some point become inhibitory or even toxic for both methanogens and SRB (Chen et al., 2014). The inhibitory or toxic effect depends on the prevailing pH and temperature (Koster et al., 1986) as well as on the microbial adaptation (Chen et al., 2008). Not long after values of 1,500–2,000 ppm were reached, methane production started to decrease gradually until the point of failure (Supplementary Figure B.1). Both competition and high sulfide concentrations in the liquid presumably contributed to sudden process instability. In period 1b, H2S concentrations of more than 4,000 ppm were detected in the gas (Table 3). The concentration varied strongly over time (Supplementary Figure B.1). Variation of H2S concentration in the biogas can take place due to unexplainable reasons (Deublein and Steinhauser, 2009). Nonetheless, a sudden decrease in concentration could have been caused by the occurrence of some microaeration during the measurements, inducing biochemical oxidation of sulfide to elemental sulfur (Krayzelova et al., 2015). H2S values were higher during period 1b and thus contributed to the reduced methane production and relative efficiency (Table 3), as was the case for ammonia. Nevertheless, the microbial community seemed to be slightly adapted to these inhibitors, by being subjected to gradually increasing concentrations during period 1a, as the digestion process was not disrupted immediately after exceeding high inhibitor values.
The total VFA concentration during period 1a (Table 4) was low to normal compared to the suggested values for proper digestion by Wiese and König (2007), ranging from 500 to 3,000 mg kg−1. The acid production and consumption were thus in balance, indicating that the instability was not caused by overloading the system. This observation was confirmed by a FOS/TAC ratio lower than 0.4 (Table 4) which shows that the buffer capacity (TAC) was high compared to the FOS content (Wiese and König, 2007; Drosg, 2013). Immediately after instability, the VFA concentration, and more specifically the acetic acid concentration, increased rapidly indicating a disturbed digestion process. Due to (extreme) inhibition and intoxication, methanogenic activity can decrease, leading to an accumulation in VFAs (Parkin and Owen, 1986; Angelidaki and Ahring, 1994). At the beginning of period 1b, the microbiology had to cope with the restart of the feeding after instability, leading to somewhat higher VFA concentrations. However, after a while normal values were again reached (Supplementary Figure B.1). In contrast to period 1a, a constantly higher propionic acid concentration, sometimes exceeding the acetic acid concentration, was observed. Propionic acid accumulation can occur due to inhibition of propionate degrading acetogens by high ammonia concentrations (Calli et al., 2005). Furthermore, conversion of propionic acid is not thermodynamically favorable anymore at a hydrogen partial pressure exceeding values of 10−4 bar (McCarty and Smith, 1986). Such values can be induced by imbalance in the syntrophic relation between hydrogen-producing acetogens and hydrogen-consuming methanogens, due to inhibition of the latter (Parkin and Owen, 1986; Deublein and Steinhauser, 2009).
Fresh Separated Pig Manure
An average methane production of 259 L CH4 kg−1 oDM and a relative efficiency of 89% were obtained during digestion of SPM from farm B (experiment 2) in period 2a (Table 3 and Figure 1B). Note that at the beginning of this period, a temporary stop of the feeding was necessary due to a temperature problem in one of the reactors. This stop was applied to both CSTR 3 and 4 to preserve a correct basis for comparison. After restarting the feeding process, the methane content was rather constant with values ranging from 56 to 64% (Supplementary Figure B.3). At the end of period 2a, the digester was completely run through with manure. A steady state should thus be reached if operation is maintained under the same conditions. Supplementary Figure B.3 shows indeed that methane production decreased gradually tending to go to one value, namely 160 L CH4 kg−1 oDM. This value was only 53% of the methane potential (Table 2), thus indicating process inhibition. The TAN and ammonia concentration were high with values up to 5.4 g N kg−1 and 2.2 g NH3-N kg−1, respectively, at the end of period 2a and mainly caused this inhibition. H2S concentrations in the gas remained within an acceptable range (<1,700 ppm). The OLR reached values up to 4 g oDM L−1 d−1 (Supplementary Figure B.3), which should still be manageable for a microbiology adapted to thermophilic conditions according to Liu et al. (2017).
Despite some inhibition during period 2a, there were no specific signs of instability when assessing the change in methane content and individual VFA concentrations. The methanogenic community seemed to work properly as was demonstrated by the relatively constant methane content and both the rather low acetic acid (<1,000 mg kg−1) and butyric acid concentrations (<20 mg kg−1) at the end of period 2a. However, propionic acid accumulated to values up to 3,700 mg kg−1 (Supplementary Figure B.3). Propionic acid will more likely accumulate at higher DM contents, even though no inhibition due to ammonia or sulfur-containing components takes place, because the hydrogen partial pressure will increase due to the higher resistance to H2-release (Corijn, 2017). The low acetic acid concentration and methane production could thus be the result of less propionic acid conversion. Despite the high VFA concentration, acidification did not occur, shown by the rather constant pH (Supplementary Figure B.3). This phenomenon is common for digestion of nitrogen-rich substrates such as pig manure, since ammonia serves as a strong buffer (Angelidaki and Ahring, 1994; Garcia and Angenent, 2009).
Around the moment of transition from an HRT of 60 days (period 2a) to an HRT of 45 days (period 2b), methane production values and relative efficiencies started to deviate from the steadily decreasing trend, suggesting some process instability (Figure 1B and Supplementary Figure B.3). Due to the set-up of the experiments, it was however not possible to identify with certainty whether instability occurred at the end of period 2a, during digestion at an HRT of 60 days, or at the start of period 2b, when the shift was made to an HRT of 45 days. During period 2b, digestion only yielded an average methane production of 58 L CH4 kg−1 oDM. The average relative efficiency was 19% (Figure 1B and Table 3). The methane content started to decrease once an HRT of 45 days was reached (Supplementary Figure B.3). The increase in OLR up to 5.3 g oDM L−1 d−1 caused overloading of the system, inducing an increase in VFAs. Overloading was confirmed by the FOS/TAC ratio reaching values up to 0.84 (Table 4). The increase in VFAs resulted in acidification, shown by the decrease in pH from the start of period 2b (Supplementary Figure B.3). Methanogenic activity became inhibited by this low pH, demonstrated by the fast increase in acetic acid and butyric acid concentration (Supplementary Figure B.3), leading to even more acidification and ultimately extreme process disturbance. Although during period 2b the digestion process was mainly affected by a pH decrease, ammonia (<2.2 g NH3-N kg−1) (Table 4) and H2S (<2,100 ppm) (Table 3) also (in)directly contributed.
Effect of Thermal Pre-Treatment
Fresh Liquid Pig Manure
Figure 1A demonstrates that the same trend in relative efficiency was obtained for LPM A and LPM Ap over the two periods (experiment 1). This could be expected, as the composition of the not pre-treated and pre-treated manure was similar (Table 2). Instability occurred at the same moments (Figure 1A and Supplementary Figures B.1, B.2), more specifically after an operational period of 1/3–1/2 of the HRT. During period 2b, a slightly higher relative efficiency was obtained for the pre-treated sample (Table 3 and Figure 1A). The change in ammonia and H2S concentration over time was comparable for LPM A and LPM Ap (Supplementary Figures B.1, B.2). For LPM Ap, TAN and ammonia concentrations up to 4.3 g N kg−1 and 2.2 g NH3-N kg−1 (Table 4), respectively, and H2S concentrations close to 4,000 ppm (Table 3) were reached, both suggesting the occurrence of inhibition. After instability at the end of period 2a, a temporary stop of the feeding was sufficient for recovery of the microbiology digesting LPM Ap, while for the case of LPM A addition of inoculum was necessary.
Methane production values and relative efficiencies obtained for LPM Ap were similar to somewhat higher than those for LPM A (Figure 1A). This observation was in agreement with the results found by Appels et al. (2010), who investigated the influence of thermal pre-treatment at for example 70°C on mesophilic anaerobic digestion of sludge from a waste water treatment plant. Via batch anaerobic tests, Bonmatí et al. (2001) examined the effect of thermal pre-treatment at 80°C for 3 h on mesophilic digestion of pig slurry with a low (1.3 g N kg−1) and high (3.7 g N kg−1) TAN concentration. In the case of a low TAN concentration, methane production values were 45% higher upon thermal pre-treatment. Pre-treatment of pig slurry with a high TAN concentration showed to have a negative effect on methane production as ammonia inhibition occurred. Carrère et al. (2009) thermally pre-treated liquid pig manure at 70°C for 3 h, resulting in an increase in methane potential of the soluble fraction up to 70%. At 20 h of pre-treatment instead of 3 h, a 10% increase in methane production was found by Sutaryo et al. (2014) after 11 days of mesophilic digestion. However, the methane potential of the total fraction did not increase in both studies. González-Fernández et al. (2008) obtained a 35% increase in methane production after pre-treatment of pig slurry at 170°C for 30 min. It is clear that the possible increase in methane production by thermal treatment prior to digestion depends on the treatment duration, the applied temperature and the nitrogen content of the substrate. The aim of this study was to improve the process stability by thermal pre-treatment, and not necessarily the methane production. Nonetheless, the performed thermal pre-treatment did not succeed in advancing the LPM digestion stability.
Fresh Separated Pig Manure
Methane production values and relative efficiencies of SPM Bp1 digestion at an HRT of 60 days (experiment 2, period 2a) were similar to those of SPM B1 (Figure 1B and Supplementary Figures B.3, B.4). This is in agreement with the results of Rafique et al. (2010) who did not find an increase in methane production after thermally pre-treating the solid fraction of pig manure for 1 h at 70°C. According to Sutaryo et al. (2014), prolonging this pre-treatment time to 20 h results in an 11% increased methane production after 11 days of mesophilic digestion. Raju et al. (2013) achieved a 29% increase after 27 days of mesophilic digestion by thermal pre-treatment for 15 min at 200°C. Nonetheless, the observed values in the experiment with thermal pre-treatment decreased more steadily with less variation (Figure 1B and Supplementary Figures B.3, B.4). The steady state value was lower than the methane potential (Table 2) due to propionic acid accumulation and inhibition mainly by ammonia (<2.3 g NH3-N kg−1) (Table 4), as was also the case for SPM B1. Some foam was formed during digestion of SPM B1, however not to such extent that it affected the process. Almost no foam was observed when digesting SPM Bp1. Furthermore, values of 4,100 and 930 mPa s were obtained for the digestate viscosity of SPM B1 and SPM Bp1, respectively, implying that digestate becomes less viscous when SPM is thermally pre-treated.
The digestion process with SPM Bp1 was relatively stable and close to the steady state value during the first 2 weeks of period 2b, after transition to an HRT of 45 days and a short adaptation period (Figure 1B and Supplementary Figure B.4). The methane content remained rather constant up to 3 weeks. The buffer capacity was sufficient to cope with the increasing amount of VFAs, reaching values up to 12.5 g kg−1. However, due to the need for such a high buffer capacity, ammonia concentrations remained high (Table 4) leading to further inhibition. In general, a more stable digestion process was observed upon thermal pre-treatment, as this may have induced the higher resistance to inhibiting factors, such as ammonia and sulfur-containing components.
Although there are signs that instability only occurred multiple days after the transition to an HRT of 45 days, some uncertainty remains as the HRT was adapted not long after the reactor was completely run through with SPM Bp1. To elucidate the effect of the HRT, an additional experiment (experiment 3) was conducted in which pre-treated SPM from farm B (SPM Bp2) was again digested at an HRT of 60 days, however over a longer time period. Parallel to this, the pre-treated SPM from another farm with a similar pig housing construction (SPM Cp) was digested. Experiment 3 only focused on pre-treated SPM, as this induced the most promising results in experiment 2.
An average methane production of 226 and 280 L CH4 kg−1 oDM was obtained during digestion of SPM Bp2 and SPM Cp, respectively (Table 3). The average relative efficiency was respectively, 74 and 95% (Figure 1C and Table 3). The former was equal to the value obtained during experiment 2 with manure from the same farm. The lowest methane production values were mostly reached after a non-feeding period of a few days (e.g., weekend). The methane content remained rather constant (Supplementary Figures B.5, B.6).
In general, a gradually decreasing methane production and relative efficiency, with the tendency to go to one value, were observed for both SPM Bp2 and SPM Cp until about one HRT (Figure 1C and Supplementary Figures B.5, B.6). In the case of SPM Cp, a steady-state value of 220 L CH4 kg−1 oDM or 40 L CH4 kg−1 manure (Supplementary Figure B.6) was ultimately reached, indicating a relatively stable digestion of SPM Cp. If the obtained value would be the actual methane production in a farm-scale digester, assuming that the transfer of this fresh manure to the digester is technically feasible, about 17 kWe could be generated given a daily feeding amount of 5 tons, a conversion factor of 10 kWh m−3 methane (Banks, 2009) and an electrical efficiency of 21%. In this experiment, the steady-state value corresponded to a relative efficiency of 75% (Figure 1C), indicating some inhibition. High ammonia levels (>2 g NH3-N kg−1) (Table 4) were the predominant cause of this inhibition. The H2S content in the gas stayed below 900 ppm (Table 3). In the case of SPM Bp2, the methane production decreased further without reaching a clear steady-state value. Ammonia and H2S levels were slightly higher during digestion of SPM Bp2 as well as the OLR, reaching values of 3.6 instead of 3.0 g oDM L−1 d−1 (Supplementary Figures B.5, B.6). The higher OLR caused some overloading of the reactor, shown by a FOS/TAC ratio of 0.63 at the end of the experimental period (Table 4).
VFAs accumulated strongly, especially during digestion of SPM Bp2 (Table 4). Propionic acid concentrations were higher compared to the other measured VFAs due to the high DM content of manure (Supplementary Figures B.5, B.6). The pH decrease by the end of the experiment with SPM Bp2 demonstrated the occurrence of acidification due to VFA accumulation. No significant pH decrease was detected for SPM Cp. Viscosity measurements showed that the digestate resulting from digestion of SPM Cp was less viscous, 550 instead of 1,550 mPa s, thus leading to better H2-release and less propionic acid accumulation. In general, almost no foam was detected during digestion of both pre-treated substrates.
Effect of Separation
Figure 2 shows the effect of DM content, H2S concentration in the gas and ammonia concentration on the methane production during LPM and SPM digestion. The average values were derived from the experiments performed at an HRT of 60 days. Additional values observed after recovery and continuation of the digestion process with (pre-treated) LPM A (experiment 1) and SPM B1 (experiment 2) were included, however were not previously described as they did not provide new perspectives on the digestion stability. Methane production on oDM base by SPM digestion, including results for not pre-treated and pre-treated substrates, was on average 42% higher than by LPM digestion (Figure 2A). Conversion to mass base showed that methane production by SPM digestion was on average over three times higher, indicating the effect of partial removal of the low energy liquid fraction (Asam et al., 2011; Deng et al., 2014), which was in this case mainly urine.
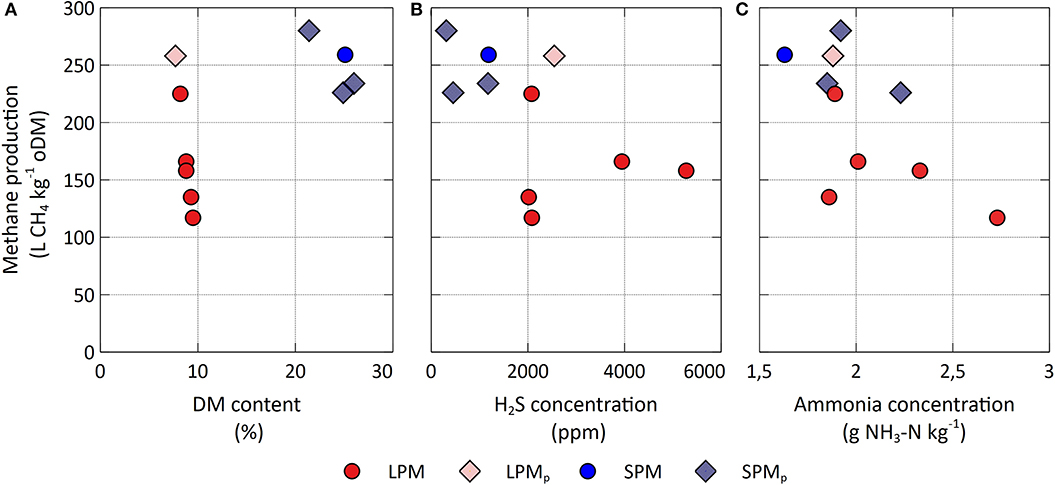
Figure 2. The effect of (A) average DM content (%), (B) H2S concentration in the gas (ppm) and (C) ammonia concentration (g NH3-N kg−1) on the average methane production obtained during thermophilic digestion of (not) pre-treated fresh liquid pig manure (LPM) and of the (not) pre-treated fresh fecal fraction from source separation by a specific pig housing construction (SPM), at an HRT of 60 days. Note that the OLR varied between the experiments. The figure did not include period 1a for LPM A and LPM Ap (experiment 1) due to the effect of the inoculum. The results of all other experimental periods at an HRT of 60 days were shown, including some values observed after continuation of the experiments with (pre-treated) LPM A and SPM B1, which were not specifically mentioned in the main article.
The digestion process proved to be more stable for separated substrates. This is in accordance with previous research which reported (extreme) inhibition and/or instability when the liquid fraction of manure was still present during digestion and when initial TAN and sulfide concentrations were high (Hashimoto, 1983, 1986; Sutaryo et al., 2013). In contrast, Møller et al. (2007) achieved a stable process when liquid pig manure, with or without being partly substituted by its solid fraction (up to 60% on mass base), was digested. In general, the methane production decreased when the concentration of ammonia or H2S in the gas increased (Figure 2). The lower H2S concentrations in the gas when digesting SPM instead of LPM (Figure 2B) were in line with the statement of Deublein and Steinhauser (2009), that the H2S content will be higher when digesting liquid substrates, and indicated the occurrence of less sulfide inhibition during SPM digestion. The ammonia concentration was in general only slightly lower for SPM than LPM substrates (Figure 2C). These findings suggest that the main cause of instability was the excess of sulfur-containing components. High concentrations of ammonia seem to have resulted in inhibition, but not necessarily in instability.
For the case of SPM, the digestion stability seemed to be affected by the (organic) DM content. The most stable digestion process was observed for the thermally pre-treated fecal fraction from farm C, having a DM content of 21% (experiment 3). At DM contents up to 26%, some instability occurred (experiment 2 and 3). In general, a higher OLR and more propionic acid accumulation during digestion were induced for substrates with a higher (organic) DM content and resulted in lower methane production values (Supplementary Figures B.3, B.6). The (organic) DM content of separated manure is directly related to the extent of separation, and more specifically to the remaining fraction of urine in the manure. Besides the (organic) DM content, farm-specific properties could also have contributed to a change in digestion stability.
Perspectives
Liquid Pig Manure Digestion
The experiment with LPM showed that stable thermophilic mono-digestion will be a challenge as inhibition and intoxication by ammonia and sulfur-containing components is facilitated. In the case of mono-digestion, some strategies to reduce ammonia inhibition during digestion are controlling the pH or temperature (Rajagopal et al., 2013), as at lower values, less ammonia is formed (Equation 4). Tackling sulfur issues is not only related to process stability but also to avoiding corrosion of equipment, such as the combustion engine and piping (Wellinger and Lindberg, 2005). In practice, H2S is often dealt with, during or after digestion, through biological desulfurization by air (microaeration) or oxygen dosing, through adsorption on activated carbon or through precipitation by addition of iron salts (Wellinger and Lindberg, 2005; Deublein and Steinhauser, 2009).
Research on substrate pre-treatment aimed at (partial) removal of nitrogen and sulfur-containing components is limited. In the case of sulfur, it could however eliminate undesirable side effects of (partial) removal techniques, which are applied during or after the digestion process. Side effects may be: process disturbance by too much air or oxygen dosing, damage of the equipment by insufficient replacement of the activated carbon or formation of impregnable phosphate components after digestate fertilization. Ammonia could be removed prior to digestion by means of a stripping and scrubbing process (Ghyselbrecht et al., 2018; Bijnagte, 2019) or through gas-permeable membranes (Filho et al., 2018). At this moment, such techniques still come at a high cost. In view of future farm-scale implementations, attention should be given to low-cost inhibitor removal techniques prior to digestion. Besides, the single and combined effect of (partial) removal or avoidance of these inhibitors on the digestion stability as well as the necessary extent of inhibitor removal needs further elaboration.
This study focused on mono-digestion because of its link to decentralized digestion on farms. Pig farmers often have no other agricultural by-products for digestion apart from pig manure. Besides, Flemish legislation does not promote co-digestion of manure and agro-residues at this moment, as the resulting digestate is completely categorized as animal manure, while part originates from agro-residues (Vlaamse Overheid, 2019). However, co-digestion of pig manure with carbon-rich agro-residues could increase the C:N ratio of the digested substrate, thereby lowering the risk for ammonia inhibition (Rajagopal et al., 2013). Co-digestion of pig manure, whether or not combined with a sulfur removal process, could thus possibly induce a (more) stable LPM digestion. However, research should be conducted on which and how much co-substrates should be added in proportion to manure.
In this study, instability of LPM digestion occurred after specific operational periods. Regular addition of inoculum or a regular stop of the feeding could ensure preservation of an active microbiology that can cope with inhibiting and limiting factors under the investigated conditions. In practice, the application of this approach will be difficult, due to the related economic losses and additional work load. Adaptation of the microbiology to its inhibitors can be a solution to some extent (Deublein and Steinhauser, 2009; Chen et al., 2014). However, practical implementation of microbial adaptation before digestion should be evaluated.
Separated Pig Manure Digestion
The experiments with SPM demonstrated the potential for stable thermophilic mono-digestion at an HRT of 60 days. The most stable digestion process was observed for the thermally pre-treated fecal fraction with a DM content of 21%. At higher DM contents, some instability occurred due to an increase in OLR and propionic acid accumulation, suggesting an effect of the (organic) DM content on the digestion stability. Further research, focusing on the 15 to 25% DM range, could explore whether an optimal (organic) DM content for stable thermophilic digestion of SPM with a high nitrogen content exists. Based on the outcome of this research, the required extent of separation can be determined, thereby evaluating if it can be accomplished by both source separation by an animal housing construction and mechanical separation.
Thermal Pre-Treatment
A thermal pre-treatment for 1 h at 70°C was chosen to keep the total thermal demand of decentralized digestion on farms as low as possible, although increased treatment times or temperatures could improve methane production (Wang et al., 1997; Valo et al., 2004; Appels et al., 2010; Rafique et al., 2010; Raju et al., 2013; Sutaryo et al., 2014). Moreover, from practical point of view, a thermal treatment for 1 h at 70°C ensures pasteurization of manure, i.e., the destruction of harmful bacteria and germs (FAVV, 2017), making export possible (European Commission, 2009).
Heat is not only necessary for thermal pre-treatment but also to keep the manure in the digester on temperature (De Dobbelaere et al., 2015; Paterson, 2015). The heat demand will be higher for LPM due to its higher water content (Asam et al., 2011). In this study, the water content of LPM was over 90% of the total mass, for SPM this was up to 79% (Table 2). Excess heat from the combined heat and power unit could be used to perform the thermal pre-treatment. The degree of excess depends on the heat demand of the farm and the digester implementation, and is also directly related to the prevailing outside air temperature. The heat requirement of a well-insulated digester is expected to be low, because heated substrate will be fed daily. Due to the lower water content of SPM, less heat will probably be necessary to cover the demand for pre-treatment and digester, thus making implementation more feasible.
Furthermore, thermal pre-treatment positively affected the digestion stability of the investigated SPM, especially at lower HRTs. Additional experiments are needed, simultaneous to the suggested ones for the determination of an optimal (organic) DM content, to discover whether thermal pre-treatment is always necessary for process stability in that DM range.
Operational Restrictions
The use of fresh pig manure is essential in view of maximizing methane formation and thus renewable energy production through anaerobic digestion. de Buisonjé and Verheijen (2014) found that the biogas potential of liquid pig manure reduces by more than 30% after pre-storage for 1 month. The current standard pig farms, consisting of a manure pit below the slatted floor of the animal housing, do not allow for collection of fresh pig manure. The fecal fraction, resulting from source separation by a specific pig housing construction with manure scrapers, can be collected freshly. Nonetheless, under the current conditions, investments in such pig housing constructions will probably remain limited in Flanders, especially if no stable digestion process can be guaranteed, as long as end-of-pipe techniques (such as air scrubbers) are sufficient for reduction of ammonia emissions and no additional standards are imposed on greenhouse gas emission reduction. Both fresh pig manure collection and stable digestion are thus crucial toward practical implementation and require further investigation.
Besides fresh pig manure collection, attention should be given to the transfer of manure from animal housings to the digester. If digestion takes place in a CSTR, keeping the manure pumpable is recommended to limit costs. No problems in terms of pumpability are expected with liquid pig manure. However, the investigated fecal fractions (SPM B and C), which proved to be more promising in terms of digestion stability, had a DM content up to 26%, suggesting that transfer through piping will be difficult. In the context of practical implementation, this manure-specific property will thus have to be taken into account.
The HRT maintained in the digester is directly related to the digester volume. If long HRTs are required, a larger digester can be implemented. Due to the digester volume increase, the OLR will decrease if the daily feeding amount remains constant (Equation 5). However, a larger digester will induce higher investment costs. Promising results in terms of stability were obtained during the digestion experiments with SPM at an HRT of 60 days. At an HRT of 45 days, methane production values were low. In practice, the HRT of farm-scale mesophilic digesters is recommended to be 30 days (De Dobbelaere and Verleden, 2018). HRTs of thermophilic digesters are expected to be even lower (Al Seadi et al., 2008). In view of cost reduction, achieving stable digestion at lower HRTs seems necessary. Nonetheless, the HRT should be kept high enough, so that the residual methane potential of digestate remains limited (Vergote et al., 2019).
Moreover, this study focused on thermophilic digestion of pig manure. Under thermophilic conditions, the growth rate of the microbiology will increase. Furthermore, the digestibility is enhanced (Al Seadi et al., 2008), which is important for substrates such as manure, mainly consisting of non-readily biodegradable compounds (Vavilin et al., 1996). However, at thermophilic temperatures, the risk for inhibition may be higher than at mesophilic temperatures, if no substrate pre-treatment takes place or under other specific conditions (Al Seadi et al., 2008; Chen et al., 2014). A techno-economic assessment would be valuable to find the most optimal farm-scale implementation taking into account digestion of pig manure at these two temperature regimes.
Conclusions
No stable thermophilic mono-digestion of fresh liquid pig manure was observed at an HRT of 60 days, due to too high concentrations of ammonia and sulfur-containing components. Thermophilic mono-digestion of the fresh fecal fraction from source separation by a specific pig housing construction was more stable, if allowed sufficient time for digestion. The H2S concentration in the gas was much lower when mono-digesting the fecal fraction instead of liquid pig manure, suggesting the excess of sulfur-containing components to be the main driver for instability. The stability of the digestion process with the fecal fraction further improved by thermal pre-treatment at 70°C for 1 h, though seemed to be affected by the (organic) dry matter content. No improvement was noted for liquid pig manure.
In general, (source) separation, possibly followed by a thermal pre-treatment, appeared to be a promising strategy in view of thermophilic pig manure mono-digestion, since it improved the process stability and increased the energy content on mass base. However, toward practical implementation, further elaboration or research is required on the extent of separation, the necessity for thermal pre-treatment and its techno-economic evaluation, (partial) inhibitor removal prior to digestion, fresh manure collection, and manure transfer to the digester.
Data Availability Statement
The datasets generated for this study are available on request to the corresponding author.
Author Contributions
TV, BW, AD, JL, and EM developed the experimental set-up. TV, BW, and AD took care of substrate and inoculum collection. TV and BW were involved in the execution of the experiments with input from AD, JL, and EM. BW supervised the chemical analyses. TV conducted data processing and wrote the manuscript. TV, BW, AD, JL, and EM discussed the results. AD, JL, JB, EV, and EM read and commented on the manuscript.
Funding
This work was supported by the LA project Pocket Power (150913), funded by Flanders Innovation and Entrepreneurship (VLAIO, http://www.vlaio.be).
Conflict of Interest
AD and JL were employed by the company Inagro. BW was employed by the company Innolab.
The remaining authors declare that the research was conducted in the absence of any commercial or financial relationships that could be construed as a potential conflict of interest.
Acknowledgments
The authors thank Innolab for the opportunity to perform experiments at the facility, for the technical support and for sharing knowledge. The owners of the pig farms are acknowledged for giving access to their facility for manure collection.
Supplementary Material
The Supplementary Material for this article can be found online at: https://www.frontiersin.org/articles/10.3389/fenrg.2020.00040/full#supplementary-material
Abbreviations
BMP, Biochemical methane potential; CSTR, Continuous stirred tank reactor; DM, Dry matter; FOS, Volatile organic acids; HRT, Hydraulic retention time; LPM, Liquid pig manure; oDM, Organic dry matter; OLR, Organic loading rate; SPM, Separated pig manure; SRB, Sulfate reducing bacteria; TAC, Total inorganic carbonates; TAN, Total ammonia nitrogen; TN, Total nitrogen; VFA, Volatile fatty acids.
Footnotes
1. ^https://statbel.fgov.be/nl/themas/landbouw-visserij/land-en-tuinbouwbedrijven#figures
2. ^https://www.vermeulenconstruct.be/nl/stalinrichting/
3. ^http://www.innolab.be/nl/productendetail/methaanpotentieel-biogaspotentieel-sneltest
References
Ahmed, J., Ramaswamy, H. S., Alli, I., Raghavan, V. G. S., Ahmed, J., Ramaswamy, H. S., et al. (2007). Protein denaturation, rheology, and gelation characteristics of radio-frequency heated egg white dispersions. Int. J. Food Prop. 10, 145–161. doi: 10.1080/10942910600986970
Al Seadi, T., Rutz, D., Prassl, H., Köttner, M., Finsterwalder, T., Volk, S., et al. (2008). Biogas Handbook. Esbjerg: University of Southern Denmark Esbjerg. doi: 10.1533/9780857097415.1.85
Angelidaki, I., and Ahring, B. K. (1994). Anaerobic thermophilic digestion of manure at different ammonia loads: effect of temperature. Water Res. 28, 727–731.
Appels, L., Degrève, J., Van der Bruggen, B., Van Impe, J., and Dewil, R. (2010). Influence of low temperature thermal pre-treatment on sludge solubilisation, heavy metal release and anaerobic digestion. Bioresour.Technol. 101, 5743–5748. doi: 10.1016/j.biortech.2010.02.068
Asam, Z.-Z., Poulsen, T. G., Nizami, A.-S., Rafique, R., Kiely, G., and Murphy, J. D. (2011). How can we improve biomethane production per unit of feedstock in biogas plants? Appl. Energy 88, 2013–2018. doi: 10.1016/j.apenergy.2010.12.036
Banks, C. (2009). Optimising Anaerobic Digestion - Evaluating the Potential for Anaerobic Digestion to Provide Energy and Soil Amendment. University of Reading.
Bijnagte, J. W. (2019). POULAR - Poultry Manure De-ammonification as Pre-treatment for Anaerobic Digestion.
Bonmatí, A., Flotats, X., Mateu, L., and Campos, E. (2001). Study of thermal hydrolysis as a pretreatment to mesophilic anaerobic digestion of pig slurry. Water Sci. Technol. 44, 109–116. doi: 10.2166/wst.2001.0193
Calli, B., Mertoglu, B., Inanc, B., and Yenigun, O. (2005). Effects of high free ammonia concentrations on the performances of anaerobic bioreactors. Proc. Biochem. 40, 1285–1292. doi: 10.1016/j.procbio.2004.05.008
Carrère, H., Sialve, B., and Bernet, N. (2009). Improving pig manure conversion into biogas by thermal and thermo-chemical pretreatments. Bioresour. Technol. 100, 3690–3694. doi: 10.1016/j.biortech.2009.01.015
Chen, J. L., Ortiz, R., Steele, T. W. J., and Stuckey, D. C. (2014). Toxicants inhibiting anaerobic digestion: a review. Biotechnol. Adv. 32, 1523–1534. doi: 10.1016/j.biotechadv.2014.10.005
Chen, Y., Cheng, J. J., and Creamer, K. S. (2008). Inhibition of anaerobic digestion process: a review. Bioresour. Technol. 99, 4044–4064. doi: 10.1016/j.biortech.2007.01.057
Climent, M., Ferrer, I., Baeza, M., del, M., Artola, A., Vázquez, F., et al. (2007). Effects of thermal and mechanical pretreatments of secondary sludge on biogas production under thermophilic conditions. Chem. Eng. J. 133, 335–342. doi: 10.1016/j.cej.2007.02.020
de Buisonjé, F., and Verheijen, R. (2014). Drijfmest Verliest Snel Zijn Waarde Voor Biogas. V-Focus; Rubriek Onderzoek & Beleid.
De Dobbelaere, A., De Mey, J., Lebuf, V., Ryckaert, B., Schollier, C., and Van Driessche, J. (2015). Kleinschalige vergisting: Praktijkvoorbeelden uit binnen- & buitenland. Inagro.
De Dobbelaere, A., and Verleden, I. (2018). Enquête project Pocketboer - Impact van reactorinstellingen op energieproductie. Unpublished data. Inagro.
de Haan, J., van Geel, W., Paauw, J., van der Burgt, G.-J., Hospers, M., Venhuizen, A., et al. (2013). Databank Organische Meststoffen. Retrieved from: https://www.researchgate.net/publication/289128391_Databank_organische_meststoffen (accessed November 22, 2019).
Decorte, M., Tessens, S., and Winternitz, K. (2019). De Vlaamse biogassector in 2018. Voortgangsrapport Biogas-E.
Deng, L., Li, Y., Chen, Z., Liu, G., and Yang, H. (2014). Separation of swine slurry into different concentration fractions and its influence on biogas fermentation. Appl. Energy. 114, 504–511. doi: 10.1016/j.apenergy.2013.10.018
Deublein, D., and Steinhauser, A. (2009). Biogas From Waste and Renewable Resources (2nd Edn). Weinheim: WILEY-VCH Verlag GmbH & Co. KGaA.
European Commission (2009). Verordening (EG) nr. 1069/2009 van het Europees Parlement en de Raad van 21 Oktober 2009 tot Vaststelling van Gezondheidsvoorschriften Inzake Niet Voor Menselijke Consumptie Bestemde Dierlijke Bijproducten en Afgeleide Producten en Tot Intrekking van Vero. Publicatieblad van de Europese Unie, nr. L 300. Available online at: https://eur-lex.europa.eu/legal-content/NL/ALL/?uri=CELEX%3A32009R1069
FAVV (2017). Risico van de Verspreiding van Mest en Mestdigestaten Gecontamineerd Door Clostridium botulinum. FAVV.
Filho, J., de, S. O., Daguerre-Martini, S., Vanotti, M. B., Saez-Tovar, J., Rosal, A., et al. (2018). Recovery of ammonia in raw and co-digested swine manure using gas-permeable membrane technology. Front. Sustain. Food Syst. 2, 1–9. doi: 10.3389/fsufs.2018.00030
Garcia, M. L., and Angenent, L. T. (2009). Interaction between temperature and ammonia in mesophilic digesters for animal waste treatment. Water Res. 43, 2373–2382. doi: 10.1016/j.watres.2009.02.036
Gavala, H. N., Yenal, U., Skiadas, I. V., Westermann, P., and Ahring, B. K. (2003). Mesophilic and thermophilic anaerobic digestion of primary and secondary sludge. Effect of pre-treatment at elevated temperature. Water Res. 37, 4561–4572. doi: 10.1016/S0043-1354(03)00401-9
Ghyselbrecht, K., Monballiu, A., Somers, M. H., Sigurnjak, I., Meers, E., Appels, L., et al. (2018). Stripping and scrubbing of ammonium using common fractionating columns to prove ammonium inhibition during anaerobic digestion. Int. J. Energy Environ. Eng. 9, 447–455. doi: 10.1007/s40095-018-0283-7
González-Fernández, C., León-Cofreces, C., and García-Encina, P. A. (2008). Different pretreatments for increasing the anaerobic biodegradability in swine manure. Bioresour. Technol. 99, 8710–8714. doi: 10.1016/j.biortech.2008.04.020
Hansen, K. H., Angelidaki, I., and Ahring, B. K. (1998). Anaerobic digestion of swine manure: inhibition by ammonia. Water Res. 32, 5–12. doi: 10.1016/S0043-1354(97)00201-7
Hashimoto, A. G. (1983). Thermophilic and mesophilic anaerobic fermentation of swine manure. Agr. Wastes 6, 175–191.
Hashimoto, A. G. (1986). Ammonia inhibition of methanogenesis from cattle wastes. Agr Wastes 17, 241–261. doi: 10.1016/0141-4607(86)90133-2
Henneberg, W., and Stohman, F. (1860). Begründung einer rationellen Ftterung der Wiederkäuer. Vol. 1. Braunschweig: Schwetschtke u. Sohn.
Hjorth, M., Christensen, K. V., Christensen, M. L., and Sommer, S. G. (2010). Solid-liquid separation of animal slurry in theory and practice. A review. Agron. Sustain. Dev.30, 153–180.
Ichimura, Y. (1991). Thermal Denaturation of Proteins II. Tokyo: Hitachi High-Tech Science Corporation.
Jain, S., Newman, D., Nzihou, A., Dekker, H., Le Feuvre, P., Richter, H., et al. (2019). Global Potential of Biogas. World Biogas Association.
Koster, I. W., Rinzema, A., De Vegt, A. L., and Lettinga, G. (1986). Sulfide inhibition of the methanogenic activity of granular sludge at various pH-levels. Water Res. 20, 1561–1567. doi: 10.1016/0043-1354(86)90121-1
Krayzelova, L., Bartacek, J., Díaz, I., Jeison, D., Volcke, E. I. P., and Jenicek, P. (2015). Microaeration for hydrogen sulfide removal during anaerobic treatment: a review. Rev. Environ. Sci. Bio/Technol. 14, 703–725. doi: 10.1007/s11157-015-9386-2
Liebetrau, J., Sträuber, H., Kretzschmar, J., Denysenko, V., and Nelles, M. (2019). Anaerobic Digestion. Adv. Biochem. Eng. Biotechnol. 166, 281–300. doi: 10.1007/10
Lindorfer, H., and Demmig, C. (2016). Foam formation in biogas plants–a survey on causes and control strategies. Chem. Eng. Technol. 39, 620–626. doi: 10.1002/ceat.201500297
Liu, C., Wang, W., Anwar, N., Ma, Z., Liu, G., and Zhang, R. (2017). Effect of organic loading rate on anaerobic digestion of food waste under mesophilic and thermophilic conditions. Energy Fuels 31, 2976–2984. doi: 10.1021/acs.energyfuels.7b00018
Matulaitis, R., Juškien,e, V., and Juška, R. (2015). Measurement of methane production from pig and cattle manure in Lithuania. Zemdirbyste-Agr. 102, 103–110. doi: 10.13080/z-a.2015.102.013
McCarty, P. L., and Smith, D. P. (1986). Anaerobic wastewater treatment. Environ. Sci. Technol. 20, 1200–1206. doi: 10.1021/es00154a002
Møller, H. B., Nielsen, A. M., Nakakubo, R., and Olsen, H. J. (2007). Process performance of biogas digesters incorporating pre-separated manure. Livest. Sci. 112, 217–223. doi: 10.1016/j.livsci.2007.09.014
Møller, H. B., Sommer, S. G., and Ahring, B. K. (2004). Methane productivity of manure, straw and solid fractions of manure. Biomass Bioenergy. 26, 485–495. doi: 10.1016/j.biombioe.2003.08.008
Nordmann, W. (1977). Die Überwachung der Schlammfaulung. KA-Informationen für das Betriebspersonal, Beilage zur Korrespondenz Abwasser, 3/77.
Parkin, G. F., and Owen, W. F. (1986). Fundamentals of Anaerobic Digestion of Wastewater Sludges. J. Environ. Eng. 112, 867–920. doi: 10.1061/(ASCE)0733-9372(1986)112:5(867)
Paterson, M. (2015). Kleinschalige Vergisting in de Praktijk - Handboek Voor Landbouwers. BioEnergy Farm II Publication; KTBL.
Pavlostathis, S. G., and Giraldo-Gomez, E. (1991). Kinetics of anaerobic treatment: a critical review. Crit. Rev. Environ. Contr. 21, 411–490. doi: 10.1080/10643389109388424
Rafique, R., Poulson, T. G., Nizami, A., Asam, Z., Murphy, J. D., and Kiely, G. (2010). Effect of thermal, chemical and thermo-chemical pre-treatments to enhance methane production. Energy 35, 4556–4561. doi: 10.1016/j.energy.2010.07.011
Rajagopal, R., Massé, D. I., and Singh, G. (2013). A critical review on inhibition of anaerobic digestion process by excess ammonia. Bioresour. Technol. 143, 632–641. doi: 10.1016/j.biortech.2013.06.030
Raju, C. S., Sutaryo, S., and Ward, A. J. (2013). Effects of high-temperature isochoric pre- treatment on the methane yields of cattle, pig and chicken manure. Environ. Technol. 34, 239–244. doi: 10.1080/09593330.2012.689482
Scarlat, N., Fahl, F., Dallemand, J.-F., Monforti, F., and Motola, V. (2018). A spatial analysis of biogas potential from manure in Europe. Renew. Sustain. Energy Rev. 94, 915–930. doi: 10.1016/j.rser.2018.06.035
Shah, F. A., Mahmood, Q., Shah, M. M., Pervez, A., and Asad, S. A. (2014). Microbial ecology of anaerobic digesters: the key players of anaerobiosis. Sci. World J. 2014:183752. doi: 10.1155/2014/183752
Skiadas, I. V., Gavala, H. N., Lu, J., and Ahring, B. K. (2005). Thermal pre-treatment of primary and secondary sludge at 70°C prior to anaerobic digestion. Water Res. 52, 161–166.
Smets, S., Palmans, S., and Luys, L. (2018). Dikke Fractie: Praktijkgids Voor Productie en Aanwending. Uitgegeven door PIBO-Campus vzw; PVL Bocholt vzw en VCM vzw.
Sommer, S. G., Christensen, M. L., Schmidt, T., and Jensen, L. S. (2013). Animal Manure Recycling: Treatment and Management. John Wiley & Sons Ltd.
Sommer, S. G., Hjorth, M., Leahy, J. J., Zhu, K., Christel, W., Sørensen, C. G., et al. (2015). Pig slurry characteristics, nutrient balance and biogas production as affected by separation and acidification. J. Agr. Sci. 153, 177–191. doi: 10.1017/S0021859614000367
Sutaryo, S., Ward, A. J., and Møller, H. B. (2013). Anaerobic digestion of acidified slurry fractions derived from different solid–liquid separation methods. Bioresour. Technol. 130, 495–501. doi: 10.1016/j.biortech.2012.12.037
Sutaryo, S., Ward, A. J., and Møller, H. B. (2014). The effect of low-temperature thermal pre-treatment on methane yield of pig manure fractions. Anim. Prod. 16, 55–62.
Trabue, S. L., Kerr, B. J., and Scoggin, K. D. (2019). Swine diets impact manure characteristics and gas emissions : Part I sulfur level. Sci. Total Environ. 687, 800–807. doi: 10.1016/j.scitotenv.2019.06.130
Valo, A., Carrère, H., and Delgenès, J. P. (2004). Thermal, chemical and thermo-chemical pre-treatment of waste activated sludge. J. Chem. Technol. Biotechnol. 79, 1197–1203. doi: 10.1002/jctb.1106
van Lier, J. B. (1995). Thermophilic anaerobic wastewater treatment; Temperature aspects and process stability (Ph.D. thesis), Wageningen Agricultural University, Wageningen, The Netherlands.
Van Overbeke, P., D'Hoop, M., Van Ransbeeck, N., and Demeyer, P. (2010). Code van Goede Praktijk Voor Emissiearme Stalsystemen in de Varkenshouderij. ILVO.
Van Ranst, E., Verloo, M., Demeyer, A., and Pauwels, J. M. (1999). Manual for the Soil Chemistry and Fertility Laboratory: Analytical Methods of Soils and Plants Equipment and Management of Consumables. Ghent: University of Ghent, International Training Centre for Post-Graduate Soil Scientists.
Vannecke, T., Gorissen, A., and Vanrespaille, H. (2018). Literatuurstudie: Waarde van de Dikke Fractie na Mestscheiding als Bron van Organische stof . Uitgegeven door het Vlaams Coördinatiecentrum voor Mestverwerking vzw te Brugge.
Vavilin, V. A., Rytov, S. V., and Lokshina, L. Y. (1996). A description of hydrolysis kinetics in anaerobic degradation of particulate organic matter. Biores. Technol. 56, 229–237. doi: 10.1016/0960-8524(96)00034-X
Vergote, T. L. I., Vanrolleghem, W. J. C., Van der Heyden, C., De Dobbelaere, A. E. J., Buysse, J., Meers, E., et al. (2019). Model-based analysis of greenhouse gas emission reduction potential through farm-scale digestion. Biosyst. Eng. 181, 157–172. doi: 10.1016/j.biosystemseng.2019.02.005
Vermeulen, G. (2019). Voorstel VeDoWS Vermeulen Dobbelaere Welfare System Duurzaam Emissiearm Systeem. Koplopersbijeenkomst: milieu- en kringloopeconomie in de varkenshouderij.
VLM (2019a). Lijst van Ammoniak-Emissiearme Stalsytemen (AEA-lijst) - Stikstofverliezen en Ammoniakemissiefactor Varkens. Vlaamse Landmaatschappij.
Wang, Q., Noguchi, C., Hara, Y., Sharon, C., Kakimoto, K., and Kato, Y. (1997). Studies on anaerobic digestion mechanism: influence of pretreatment temperature on biodegradation of waste activated sludge. Environ. Technol. 18, 999–1008. doi: 10.1080/09593331808616619
Weinrich, S. (2019). Predicting BMP from substrate composition. In: Workshop “Start!”, 16th IWA World Conference on Anaerobic Digestion.
Wellinger, A., and Lindberg, A. (2005). Biogas upgrading and utilisation. In: IEA Bioenergy Task 24: Energy Form Biological Conversion of Organic Waste.
Keywords: anaerobic digestion, thermophilic mono-digestion, fresh pig manure, process stability, semi pilot-scale digesters, thermal pre-treatment, source separation
Citation: Vergote TLI, De Dobbelaere AEJ, Willems B, Leenknegt J, Buysse J, Volcke EIP and Meers E (2020) Stability of Thermophilic Pig Manure Mono-digestion: Effect of Thermal Pre-treatment and Separation. Front. Energy Res. 8:40. doi: 10.3389/fenrg.2020.00040
Received: 09 December 2019; Accepted: 26 February 2020;
Published: 15 April 2020.
Edited by:
Su Shiung Lam, University of Malaysia Terengganu, MalaysiaReviewed by:
Ao Xia, Chongqing University, ChinaObulisamy Parthiba Karthikeyan, University of Houston, United States
Copyright © 2020 Vergote, De Dobbelaere, Willems, Leenknegt, Buysse, Volcke and Meers. This is an open-access article distributed under the terms of the Creative Commons Attribution License (CC BY). The use, distribution or reproduction in other forums is permitted, provided the original author(s) and the copyright owner(s) are credited and that the original publication in this journal is cited, in accordance with accepted academic practice. No use, distribution or reproduction is permitted which does not comply with these terms.
*Correspondence: Tine L. I. Vergote, Tine.Vergote@UGent.be