- Department of Environmental Sciences, PMAS Arid Agriculture University, Rawalpind, Pakistan
In the present study, a biorefinery system is proposed using catering and agro industrial waste for biogas and low phytotoxic digestate. Anaerobic co-digestion of catering waste with partially pre-treated (microwave 800 J/g/min + steam 121 °C 40 min) maize crop residues was conducted under different composition (20–50%) of feedstock. The results showed that the biogas production was increased by 2.03 times in co-digestion experiment (40% partially pre-treated maize crop residue + 60% catering waste: TCM3) as compared to catering waste alone (control). The increment in accumulative methane 116.7 m3 t−1 was recorded in TCM3 which is due to improvement in biodegradation under co-digestion process. The post digestion byproduct (residual digestate) was evaluated for its phytotoxicity which is supplied with aerobic post treatment. The post treatment has improved the digestate quality by decreasing VS/TS ratio from 259 to 173 g/L and slightly increase the pH from 7.29 to 8.32. Seed germination assay showed that the germination percentage (G%), germination index (GI) and vigor index (VI) were relatively higher with post treated digesate as compared to un-treated digestate. In the germination test using wheat seeds, the post treated digestate (5% sol. extract) achieved higher values of GI and VI (46 and 609) whereas in un-treated, values for these indices were 14 and 62, respectively. Overall the findings of the present study identify the significance co-digestion based waste biorefinery, in order to development of value added bio-products such as biogas and biofertilizers.
Introduction
The advancement in living standard has risen the generation of organic waste at substantial rates. One of the signific sources of organic wastes in municipalities is from catering services which are generated excessively in hotels, canteens, restaurants, and the aviation industry (Ayomoh et al., 2008; Jiang et al., 2018). Catering waste may contain vegetables, fruit, meat, baked goods, dairy, and animal by-products which are not only rich in nutrients, but also a variety of amino acids, proteins, carbohydrates, and vitamins are also present. According to an estimate of UN Food and Agriculture Organization, about 1.3 billion tons of these kinds of waste are lost during the food supply chain (Jiang et al., 2018). Catering wastes are problematic to treat due their high moisture content, it can involve in the transmission of pathogen microorganisms that possibly cause diseases in humans and also pollute drinking water (Chen et al., 2017). Hence, it requires being dealt in an environmentally safe method (Zhang et al., 2005; Izumi et al., 2010; Anjum et al., 2016). On the other hand, agriculture is another major source of organic waste in the form of crop residues. Maize is known as one of the major crops in Pakistan, which produce thousands of tons of post harvesting residues. These residues are difficult to degrade through a biological process due to high content of lignocellulose (Surra et al., 2018).
The organic waste when disposed in landfills creates problems of leachate and biogas production that not only create nuisance in the landfill area, but also results in the loss of huge resource potential. Thus, an effective management system (biorefinary) is required to ensure the integration of organic waste management with energy recovery and reuse of byproduct formulation. Competence of biological waste treatment in this regard is well established option both in terms of scope and applicability, as it encompasses a wide variety of organic wastes ranging from food waste (Kuczman et al., 2018) and agro-allied industries (Alvarez et al., 2010) to waste activated sludge (Bolzonella et al., 2012; Bundhoo et al., 2016).
Among biological treatment anaerobic digestion has received special interest in the past few years, which results in the production of two byproducts that is biogas and digestate (Chen et al., 2018). However, some interventions are still needed for the provision of balanced nutrients and stable conditions for creating efficient digestion systems (Sosnowski et al., 2003). Recently, anaerobic co-digestion has been reported to improve the digestion process and energy production by improving the nutrient availability and organic load, while lowering the toxicity of the inhibitory compounds by dilution (Anjum et al., 2012, 2016; Serrano et al., 2013).
In anaerobic digestion most of the studies highlighting the anaerobic treatment of organic waste have made significant improvements to enhance the energy recovery as a factor of biogas production. Where mere efforts have been made to utilize anaerobic digestate which is an enriched nutrient source(Abdullahi et al., 2008; Trzcinski and Stuckey, 2011). The digestate effluents carry vital nutrients capable of promoting plant growth (Tornwall et al., 2017). Application of such high nutrient entities to the soil not only ensure the sound disposal of anaerobic byproducts, but also is an appealing solution to declining soil nutrient balance, which has identified as an important soil health problem worldwide (Galvez et al., 2012; Da Ros et al., 2018). However, the characteristic phytotoxicity limits the applications of anaerobic digestate as soil conditioner (Coelho et al., 2018). The fertilizer value of digestate can be enhanced by reducing its phytotoxic effects. Therefore, an appropriate pre-treatment is required that ensures sound application of anaerobic digestate to the soil. Aerobic “polishing” is identified as competent option for the reduction of toxic effects of anaerobic digestate through reduction in moisture, odor, carbon and pathogens in the digestate.
In view of above mention concerns the present study was conducted with the aims to: (1) partial pretreatment of maize crop residue to improve its digestibility in co-digestion with catering waste, (2) Optimizing substrate ratio in co-digestion catering waste and partial pre-treated maize crop residues to maximum digestibility and biogas production, (3) to test and prepare a low pytotoxic digestate as an organic conditioner for plants.
Materials and Methods
Feedstock Material and Physico-Chemical Characterization
The raw materials used for the preparation of digestate were catering waste and crop residues maize crop residues. The catering waste samples were taken from local food restaurants and banquet halls of Rawalpindi city, while the maize residues were collected from PMAS Arid Agriculture University Research Farm located at Koont, Pakistan. The initial physico-chemical characterization of catering waste and maize crop residues are described in Table 1.
Pre-treatment of Maize Crop Residues
Prior to anaerobic digestion maize crop residues were supplied with the pretreatment steps, which were selected based on a partial level of treatment with minimal energy prerequisite, and that avoids the complete degradation (Taherzadeh and Karimi, 2008). Sequential physical pretreatments, microwave irradiation and steam water were applied to the maize crop residues in order to enhance the accessibility of lignocellulose of maize crop residues for biological degradation. For microwave irradiation pretreatment a household-type DW-105-G microwave oven (Dawlance, Pakistan). Maize crop residues (50 g of each) were placed in the microwave oven for 3 min supplying energy of 800 J/g/min. Subsequently, irradiated substrate was placed in a high-pressure steam unit (Nanolytik Nanoclave 1, Germany) for 40 min at 121°C for steam treatment (Anjum et al., 2016). After pretreatments, maize crop residues were dried in air for 6 h, and later in a digital oven for 12 h prior to use in co-digestion.
Anaerobic Co-digestion Experiment
Anaerobic digestion experiments were performed in two steps. First, the optimization of feeding composition was performed and secondly the analysis of optimized co-digestion treatment was conducted during biogas production. At first set of experiment anaerobic co-digestion experiments were performed using catering waste as a main substrate mixed with partially pre-treated maize crop residues at different compositions. Maize crop residues (M) were used in 20, 30, 40, and 50% of total fraction with catering waste, while similar experiments were repeated with untreated maize crop residues. Anaerobic glass batch bottles of 500 ml capacity were used with total substrate volume of 350 and 150 ml empty head space. All mixtures of substrates were prepared on the basis of total solids. The seed anaerobic microorganisms were applied using previously digested organic waste added at a rate of 100 g/L (Anjum et al., 2012). A suitable amount of water was added keeping the moisture content of reactors fixed between ranges of the 70 and 75%. Co-digestion experiment took place for 6 weeks 42 at mesophilic temperature 30 ± 1°C. To examine the degradation efficiency of co-digestion, samples were analyze at different interval changes in COD and VS.
In the second set of experiment, selected treatments (40 % maize crop residues and 60% catering waste) (TEM3) was subjected to biogas production in a lab scale, anaerobic static batch reactor and compared with control. Two similar reactor of dimensions 13 × 30 cm (diameter × width) each, having capacity of 2,500 ml were designed. One reactor was used for co-digestion (TEM3), while the second was used as control reactor having catering waste only. About 2,000 ml of the reactor volume was filled with the substrates, while 500 ml head space was left empty for biogas accumulation. Biogas was collected in biogas collection bag connected at the top biogas valve, through the flow tube built in with an internal valve to control the one-way flow of biogas. The digestate samples were taken from the lower part of the reactor where a sampling valve was set. Both reactors were placed in an incubator at fixed temperature 30 ± 1°C. The biogas was analyzed using liquid displacement apparatus.
Aerobic Post Treatment of Digestate
Two digestate samples were collected from anaerobic co-digestion of catering waste and maize crop residues. One digestate sample is supplied with an aerobic post treatment in order to reduce toxic substances exist in digestate, while second digestate sample did not supply with any post treatment. Aerobic pretreatment was performed using continuous stirred aerobic reactor supplied by continuous air diffusion from the bottom and string of 150 rpm. The temperature was maintained in range of 32–35°C and reaction was continued for 20 days. The digestate quality was analyzed by measuring carbon content, volatile solids, pH and EC using standard methods. The digestate was dried in a digital oven for 24 h at 70°C. The final obtained digestate materials were preserved in the cold storing unit at temperature >50°C for further utilization in experiments.
Phytotoxicity Test
The phytotoxicity test of both digestate was conducted by using 72 h seed germination assay. Germination assay is a rapid and widely applied method for evaluating phytotoxicity of organic product (Da Ros et al., 2018). Three dilution of each digestate material (post treated and untreated digestate) were prepared using 5, 10, and 20 g of dried digestate in 100 ml of distilled water. Wheat seed were used to testing seed germination assay. For the germination assay, Petri dishes of 10 cm diameter were lined with watt man filter paper. Each dish received 5 ml of the digestate solution using a micropipet. Ten wheat seeds (sterilized) were placed in each petri dish and sealed with the parafilm. The petri dishes were incubated at 25°C for 72 h in the dark conditions. The germinated seeds were counted and, root length and shoot length were measured were at regular interval during 72 h. The seed germination percentage (G%), germination index (GI) and vigor index (VI) were analyzed to estimate the phytotoxicity of digestate.
Anatical Methods
Chemical oxygen demand (COD) was analyzed using the closed, reflux titrimetric method applying the standard method of American Public Health Association (APHA (American Public Health Association), 2005). COD was calculated using the following equation (Equation 1):
The factor X-B is ml of FAS used for blank subtracted from ml of FAS used for sample. M denotes the molarity of FAS, whereas the value 8,000 is the weight of oxygen mill equivalent 1,000 ml/l. Solid fractions i.e., TS and VS were analyzed using the standard method # 1684 (US EPA, 2001). TS was determined by measuring the difference in mass of the sample before and after drying in oven at temperature 105°C, whereas VS was measured as an ignition loss at high temperature (550°C) in the muffle furnace. The C:N ratio was calculated by taking the total mass of carbon divided by mass of nitrogen in grams (Smith and Holtzapple, 2011; Anjum et al., 2012). pH, EC, and TDS values were recorded on Crison Multimeter (Model number: “CRISON MM-40þ”). In anaerobic digesters, the biogas production was measured as methane production through liquid a displacement methods for duration of 80 days using the method described by Elaiyaraju and Partha (2012). During phytotoxicity experiment, seed germination assay was conducted where seed germination index, germination percentage, seedling length, and vigor index were measured. The germination index (GI) was calculated using the following formula (Equation 2) (Mitelut and Popa, 2011):
where Lo and Go are root growth and germination percentage. Data interpretation, calculation, averages and standard deviations were calculated using MS Office Excel 2010.
Results and Discussion
Anaerobic Co-digestion Test of Catering Waste With Maize Crop Residues
Optimization of Substrate Ratio and Effect of Pretreatment of Maize Crop Residues
For optimization of the substrate ratio, the co-digestion experiments were conducted using catering waste as main substrate and maize crop residues as co-substrates (untreated and pre-treated with microwave-steam) for a duration of 42 days. The results regarding organic matter removal efficiency (VS content basis) and change in COD are explained as under:
The results regarding VS content and organic matter removal efficiency are described in Figure 1 which showed that the co-digestion experiments with pre-treated maize crop residues showed more reduction in VS as compared to untreated maize crop residues. The maximum reduction in VS was shown by CM4 (50% untreated crop residue ratio) where VS content was lowered to 644 g/kg from 881 g/kg after 42 days of incubation. However, maximum degradation in case of pre-treated maize crop residues was observed in TCM3 (40% ratio), with the VS reduction of 302 g/kg from 798 g/kg. The organic matter removal efficiency was achieved up to maximum 62% in TEM3 compared to the TEM4 (45%). The increased in organic matter removal efficiency is probably due to the increase in lignin loss under the influence of pretreatment of maize crop residues as reported by Yuan et al. (in press). The fact that pre-treatment enhance the degradation of lignocellulose and hemicellulose material of maize crop residues which are actually recalcitrant in nature (Surra et al., 2018). After applying pre-treatment these material partially degraded and become available to microorganisms. Overall, these outcomes revealed that the pretreatment of maize crop residues has enhanced the degradation in co-digestion process with catering waste.
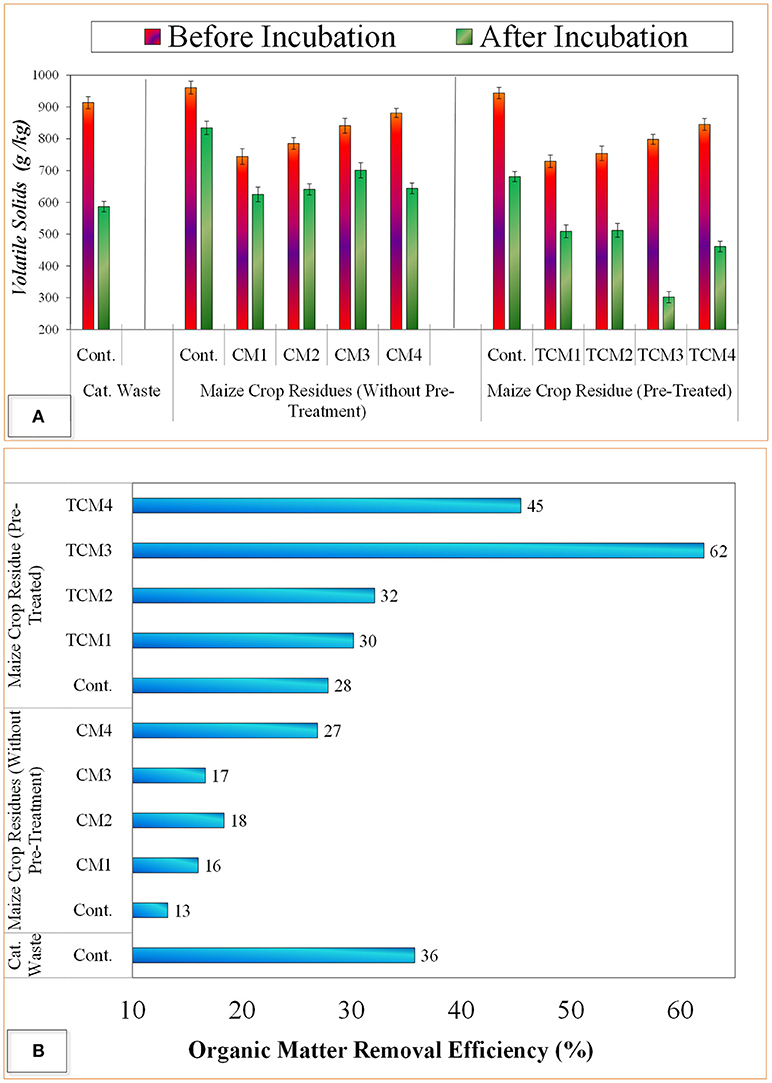
Figure 1. Anaerobic co-digestion test of catering waste with maize crop residues; (A) Effect on VS degradation, (B) Organic matter removal efficiency.
The variation in COD values during anaerobic digestion of catering waste and pre-treated maize crop residues is presented in Figure 2 COD is used as a sole indicator of optimization of anaerobic digestion process. After 42 days of the co-digestion process, the highest decrease in COD (30.2–11.5 g/L) was observed at 40% maize crop residues (TCM3), which means that insoluble COD (51%) was probably transformed soluble organic matter to final mineralized products (Figure 2B). In untreated maize crop residues the maximum degradation COD from 31.2 g/L (day1) to 20.4 g/L (day 42) was achieved at 40% fraction ration with catering waste (CM3). The lowest COD removal (31%) was observed in CM1 and CM4 in case of untreated maize crop residues which is even lower than catering waste alone (control) where COD removal of 46% was found. The improved COD degradation with pre-treated maize crop residue was due to the fact that pretreatment provides more organics in solubilized form (sCOD) and thus more available to anaerobic bacteria (Anjum et al., 2018). Overall, these results showed that use of pre-treated crop residues is more promising with catering waste co-digestion where optimum mixing ration was found at 40% maize crop residue with 60% catering waste and labeled as TCM3.
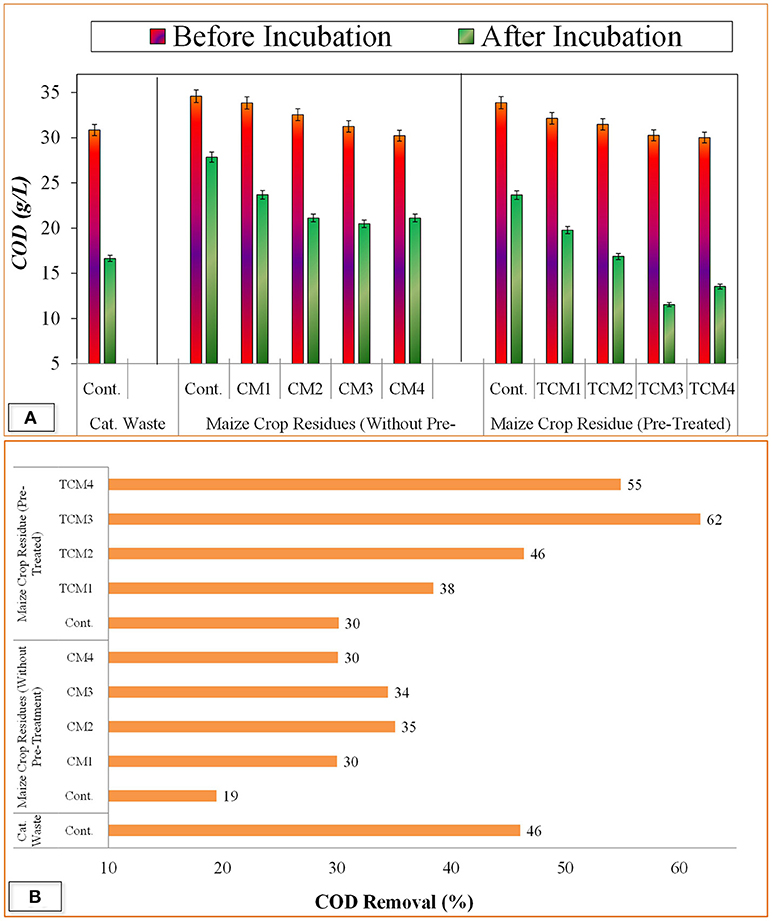
Figure 2. Anaerobic co-digestion test of catering waste with maize crop residues; (A) Effect on COD, (B) COD removal efficiency.
Biogas Production
To observe the biogas potential of mixed catering waste and maize crop residues, anaerobic digestion was performed in two static batch reactors. One is for co-digestion containing both substrates in ratio as optimized in previous experiment (TCM3), while second reactor was taken as control containing catering waste alone. The results showed that the addition of pre-treated maize crop residues in catering waste, significantly increase the methane production potential as compared to that in catering waste alone (Figure 3A). During the anaerobic digestion process of 80 days, TCM3 co-digestion reactor showed an increasing trend in biogas production up 27–42 days where highest methane generation rate of 2.46 m3 d−1 t−1 was recorded. The incremented methane production was due to the influence of pretreatment of lignocellulosic biomass, where the pretreatment degrade the lignocellulose and increase the cellulose component (Rajput and Visvanathan, 2018). Furthermore, it is well reported that the co-digestion has ability to provide more balance nutrients such as optimum C/N (Wang et al., 2014) and increased P (Medvedeff et al., 2014) content which ultimately improve the growth of methanogens and biogas production.
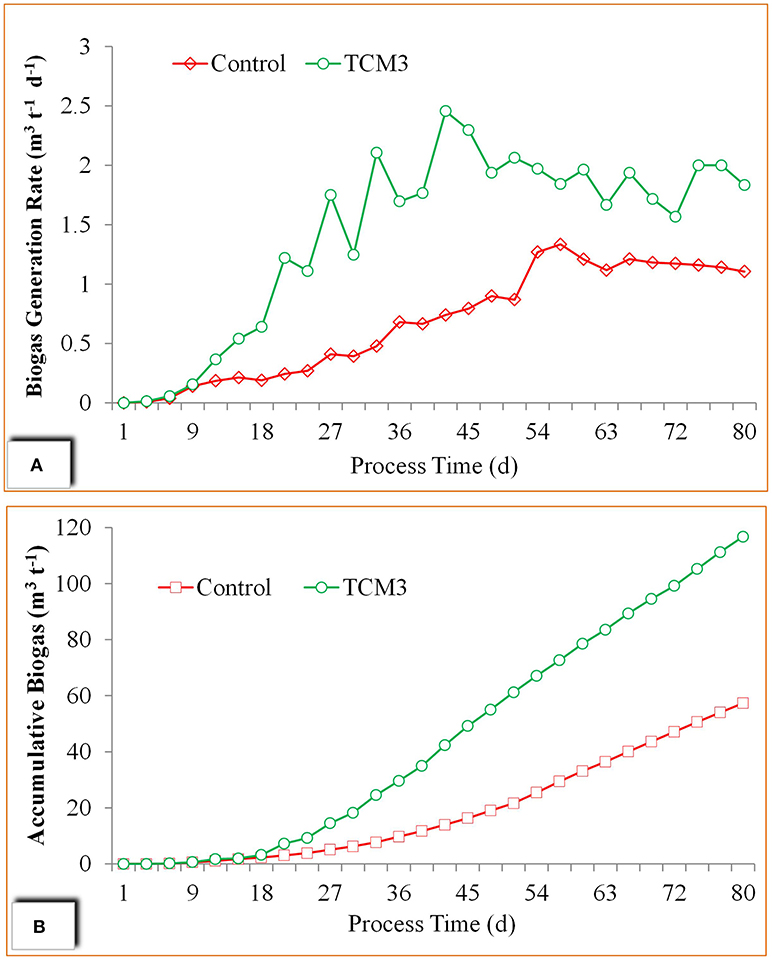
Figure 3. Anaerobic co-digestion of catering waste with partial pre-treated maize crop residues at optimum mixing ratio for biogas production; (A) Biogas production rate, (B) Accumulative biogas production.
On the other hand, control bioreactor showed the highest methane production rate of between after 54–60 days. Thereafter, the methane production rate was become stable in TEM3 co-digestion reactor till 80 days. In control bioreactor the methane production rate was slightly decrease after 60 days, which is due to slow and incomplete degradation rate. The lowering in methane production in catering waste alone may be due the accumulation of volatile fatty acids, as acidic pH range was found during the anaerobic digestion process. Moreover, the inhibition in biogas production may be cause due to accumulation of ammonia within the system which cause lowering in C/N ratio below the optimum range (25–30 required for methanogens Wang et al., 2014. The accumulation of volatile fatty acids inhibit the methanogenic activity and longer the acetogenic phase, thus reduces overall efficiency of the system Chen et al., 2014; Yang et al., 2015; Jiang et al., 2018. Overall, the accumulative methane production was more in TEM3 as compared to catering waste alone (Figure 3B). The maximum accumulative methane of 116.7 m3 t−1 was recorded in TCM3 reactor i.e., 2.03 times more as compared to control reactor. The addition of the co-substrate in anaerobic digestion process has influence the provision of more balanced nutrients such as C/N ratio and, organic load, thus creating more suitable conditions for microbial activity (Sosnowski et al., 2003; Zou et al., 2018).
Effect of Aerobic Post Treatment of Digestate
In order to prepare anaerobic digestate bio-product as low phytotoxic organic fertilizer, aerobic post treatment was applied to the anaerobic digestate of co-digested catering waste and wheat crop residues (TCM3). Figure 4A expressed the results regarding effect on VS and carbon content due aerobic post treatment. VS in the digestate was decreased from 209 g/L to 113 g/L after 20 days where as carbon content was lowered to 17% from 24% in fresh digestate. The lowering in VS and carbon content is attributed degradation of toxic organic compound especially organic acids produced during anaerobic digestion process. The lowering in VS content in post treatment make digestate more stable (Wojnowska-Baryła et al., 2018). These means that aerobic post treatment for 20 days could lower the phytotoxic compounds in digestate and make more feasible bio-product for soil application.
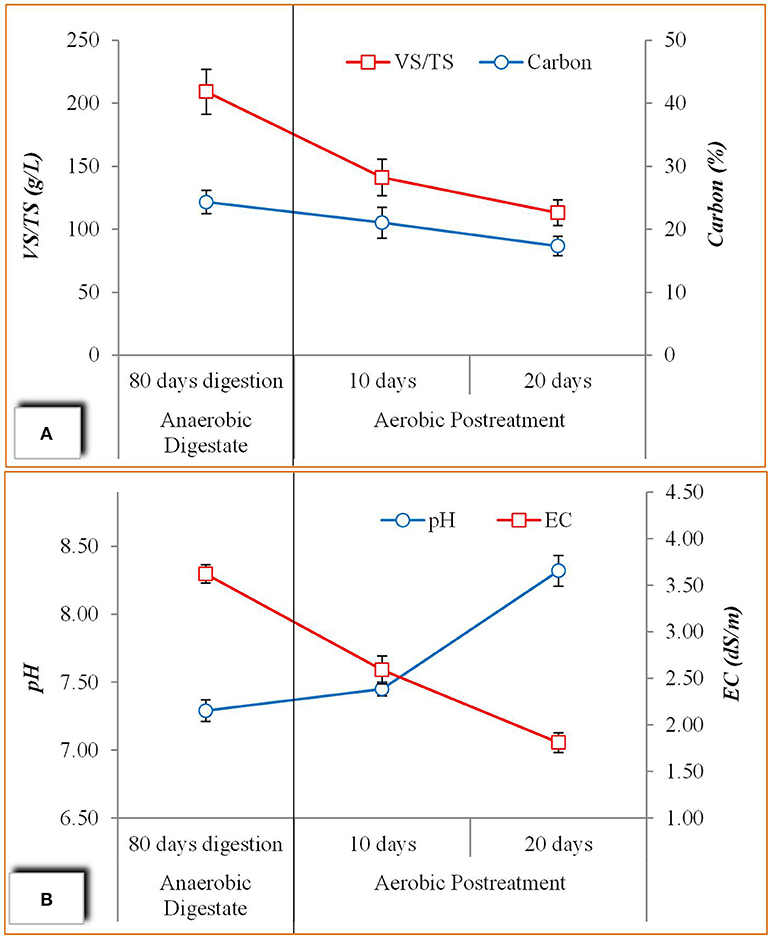
Figure 4. Post treatment of anaerobic co-digested digestate for bio-product formulation; (A) Effect on VS and Carbon content, (B) Effect on EC and pH.
In case of pH, aerobic post treatment increases an overall pH of the digestate up 8.32 which is very favorable for soil application (Figure 4B). The basic reason of low pH in anaerobic digestion production of organic acid in high quantity which inhibit the digestion process (Yang et al., 2015; Zhang et al., 2018), but in the present study the pH of 7.29 showed that there were limit accumulation of organic acids and aerobic post treatment has further improved the pH of the digestate. Electrical conductivity is another important indicator of quality of digestate which mainly represents the concentration of salts and ions. EC values of 3.62 dS/m was found in anaerobic fresh digestate which is further lowered to 1.81 dS/m after aerobic digestion process (Figure 4B). EC can influence seed germination in contact with digestate, where lower EC is more favorable for seed germination (Coelho et al., 2018). This showed that EC of the digestate could be improved by post treatment of digestate in order to cause least negative effect in soil due to higher EC.
Phytotoxicity Analysis of Digestate
Phytotoxicity is widely applied and promising parameter to evaluate digestate application on plants which describes the index its overall eco-toxicological impact (Da Ros et al., 2018). The phytotoxicity test of both untreated and post treated digestate at varying digestate solution (5, 10, and 20 g per 100 ml water) was performed using seed germination assay on wheat seeds. The results regarding seed germination percentage are described in Figure 5. Seed germination was continuously increased in case of control (Water only) where after 72 h 91% of seed were observed fully germinated. When digestate was applied G% was lowered where only 17 % of germination was found even after 72 h in D20 (20 g untreated digestate/100 ml water) (Figure 5A). However, a slightly higher G% (38 and24%) was found in D5 and D10, respectively after 72 h. This shows that the digested without post treatment cause swear toxicity to wheat seed which in increased as the concentration of digestate increase.
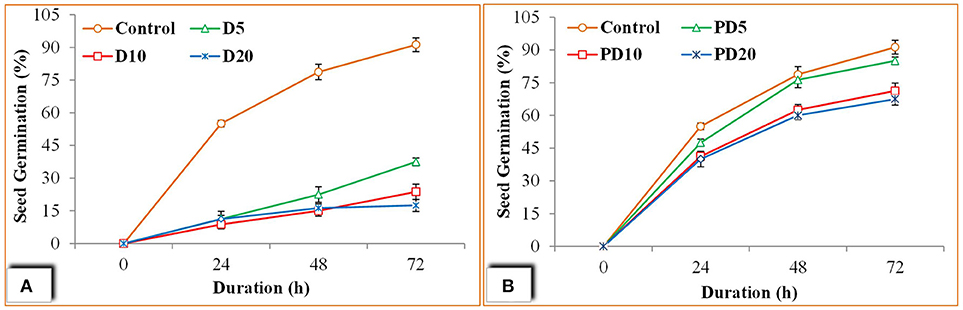
Figure 5. Seed germination assay; (A) Seed germination percentage using un-treated digestate, (B) Seed germination percentage using aerobic post treated digestate.
In case of post treated digestate an improvement in G% was observed with up to 85% seed germination was attained after 72 h in PD5 (5 g/100 ml) (Figure 5B). These finding also confirmed by the Germination Index (GI) values with a maximum GI of 46 was shown by PD5 and followed by PD10 (GI = 39) and PD20 (GI = 37) (Figure 6). However, the lower germination index values in un-treated digestate was observed which is probably due to low high EC values (Tang et al., 2018).
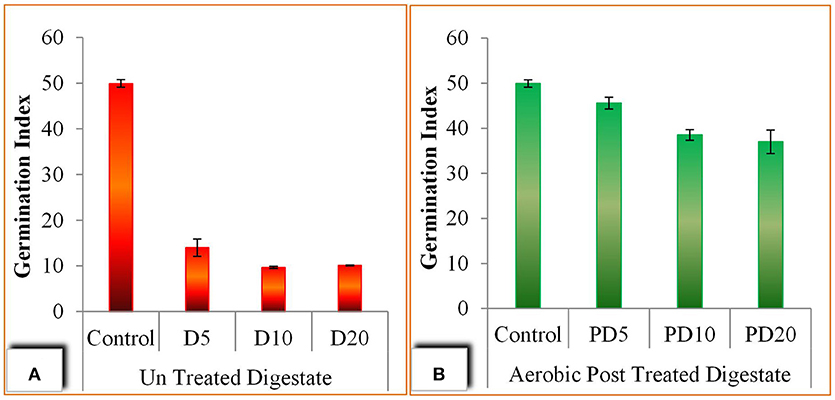
Figure 6. Seed germination assay; (A) Seed germination index using un-treated digestate, (B) Seed germination index using aerobic post treated digestate.
The seedling length was measured after 72 h of incubation and illustrated in Figure 7. Both shoot length and rood length were significantly increased with post treated digestate solution. A maximum root length of 6.01 cm and 5.19 cm were achieved by PD5 and PD10 which were 4.9 and 4.8 times higher than respective treatments with untreated digestate. Similarly, shoot length of 7.34 cm was the highest in PD5 with the slight lowing in PD10 (6.06 cm) and PD20 (5.60 cm). The improvement in seedling length is attributed to the post treatment of digestate which supply more plants essential nutrients [N, P, and K (Coelho et al., 2018)] to seedling with least effect of toxic materials.
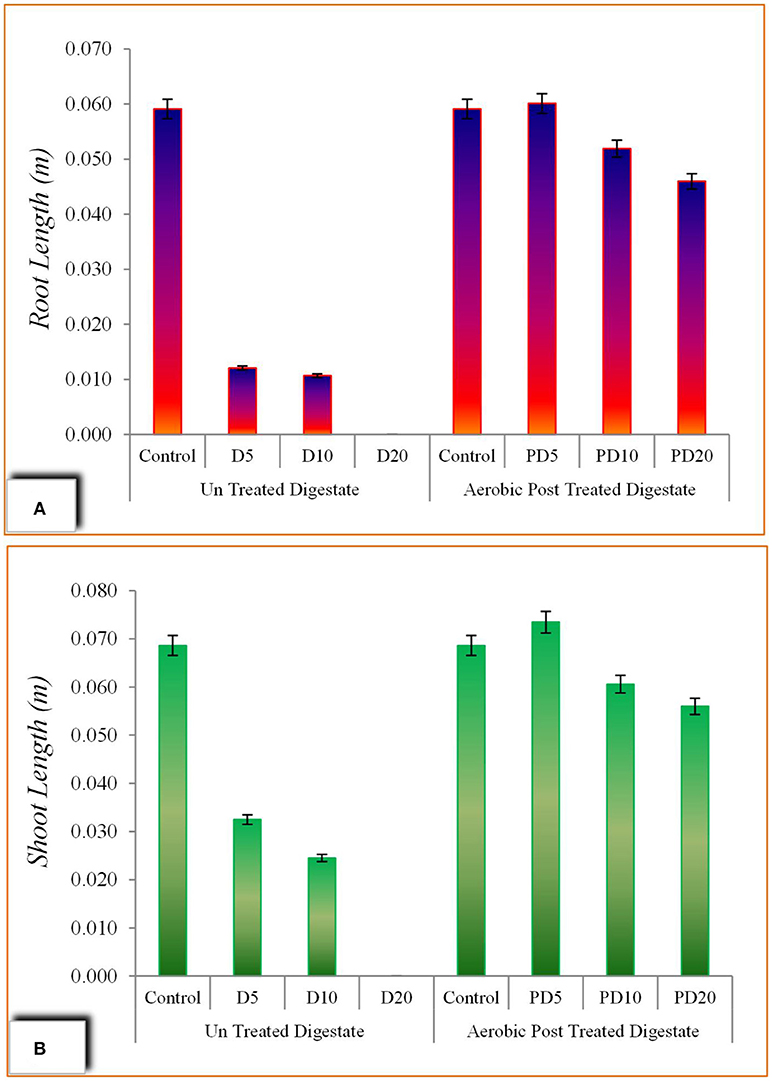
Figure 7. Seed germination assay using un-treated and aerobic post treated digestate; (A) Root length, (B) Shoot length.
Vigor index is one the known indicator used in analyses toxicity in plants (Shaikh et al., 2013). The vigor of seeds was described as a vigor index (VI) as illustrated in Figure 8. Seeds treated with post treated digestate showed the higher VI i.e., up to 609 in PD5, where with the increase in concentration of digestate from 5 g/100 ml to 20 g/100 ml, VI was decreased to 377. On the other hand the seed grown with untreated digestate shows significantly lower vigor i.e., even with a value of zero with D20 (20 g/100 ml).
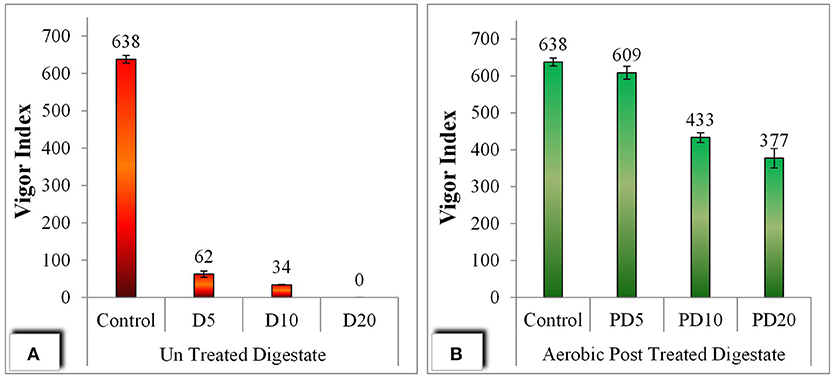
Figure 8. Seed germination assay; (A) Vigor Index with un-treated digestate, (B) Vigor Index with aerobic post treated digestate.
Conclusion
The present study demonstrates the successful application of anaerobic co-digestion process for catering waste with partially pre-treated maize crop residues to produce biogas and biofertilizer production. The co-digestion of catering waste partially pre-treated maize crop residues at 40 % mixing ratio (TCM3) showed a significant degradability, where, 2.03 times higher methane production was achieved compared to control reactor.
Thereafter, the quality of digestate was improved by applying aerobic post treatment. The phytoxic analysis showed that improved G% (85%) while maximum GI of 46 and VI of 609 were achieved. Overall, the present study proposed a biorefinery concept in which co-digestion can be used as waste management approach for multiple outcomes such as energy recovery and biofertilizer production.
Author Contributions
MA conducted the basic experimental work and prepared the initial draft. AK supervised the work and provided technical and scientific assistance. The final draft was revised by both AK and SQ.
Conflict of Interest Statement
The authors declare that the research was conducted in the absence of any commercial or financial relationships that could be construed as a potential conflict of interest.
Acknowledgments
This work was conducted and supported by Environmental Science Lab, Department of Environmental Sciences, PMAS Arid Agriculture University, Rawalpindi Pakistan.
References
Abdullahi, Y. A., Akunna, J. C., White, N. A., Hallett, P. D., Wheatley, R. (2008). Investigating the effects of anaerobic and aerobic post-treatment on quality and stability of organic fraction of municipal solid waste as soil amendment. Bioresour. Technol. 99, 8631–8636. doi: 10.1016/j.biortech.2008.04.027
Alvarez, J. A., Otero, L., Lema, L. J. (2010). A methodology for optimising feed composition for anaerobic co-digestion of agro-industrial wastes. Bioresour. Technol. 101, 1153–1158. doi: 10.1016/j.biortech.2009.09.061
Anjum, M., Khalid, A., Mahmood, T., and Arshad, M. (2012). Anaerobic co-digestion of municipal solid organic waste with melon residues to enhance biodegradability and biogas production. J. Mater. Cycles Waste Manag. 14, 388–395. doi: 10.1007/s10163-012-0082-9
Anjum, M., Khalid, A., Mahmood, T., and Aziz, I. (2016). Anaerobic co-digestion of catering waste with partially pretreated lignocellulosic crop residues. J. Clean. Prod. 117, 56–63. doi: 10.1016/j.jclepro.2015.11.061
Anjum, M., Kumar, R., Al-Talhi, H. A., Mohamed, S. A., and Barakat, M. A. (2018). Valorization of biogas production through disintegration of waste activated sludge using visible light ZnO-ZnS/Ag2O-Ag2S photocatalyst. Proc. Safety Environ. Protect. 119, 330–339. doi: 10.1016/j.psep.2018.08.022
APHA (American Public Health Association) (2005). Standard Methods for the Examination of Water and Wastewater, 21st Edn. Washington, DC: APHA-AWWA-WEF.
Ayomoh, M. K., Oke, S. A., Adedeji, W. O., and Charles-Owaba, O. E. (2008). An approach to tackling the environmental and health impacts of municipal solid waste disposal in developing countries. J. Environ. Manag. 88, 108–114. doi: 10.1016/j.jenvman.2007.01.040
Bolzonella, D., Cavinato, C., Fatone, F., Pavan, P., Cecchi, F. (2012). High rate mesophilic, thermophilic, and temperature phased anaerobic digestion of waste activated sludge: a pilot scale study. Waste Manag. 32, 1196–1201. doi: 10.1016/j.wasman.2012.01.006
Bundhoo, Z. M. A., Mauthoor, S., and Mohee, R. (2016). Potential of biogas production from biomass and waste materials in the Small Island Developing State of Mauritius. Renew. Sustain. Energy Rev. 56, 1087–1100. doi: 10.1016/j.rser.2015.12.026
Chen, H., Shen, H., Su, H. F., Chen, H. Z., Tan, F. R., Lin, J. F. (2017). High-efficiency bioconversion of kitchen garbage to biobutanol using an enzymatic cocktail procedure. Bioresour. Technol. 245 (Pt A) 1110–1121. doi: 10.1016/j.biortech.2017.09.056
Chen, X., Yan, W., Sheng, K., and Sanati, M. (2014). Comparison of high-solids to liquid anaerobic co-digestion of food waste and green waste. Bioresour. Technol. 154, 215–221. doi: 10.1016/j.biortech.2013.12.054
Chen, Y-D., Ho, S-H, Nagarajan, D., Ren N-Q, and, Chang, J. S. (2018). Waste biorefineries—integratanaerobic digestion and microalgae cultivation for bioenergy production. Curr. Opin. Biotechnol. 50, 101–110. doi: 10.1016/j.copbio.2017.11.017
Coelho, J. J., Prieto, M. L., Dowling, S., Hennessy, A., Casey, I., Woodcock, T., et al. (2018). Physical-chemical traits, phytotoxicity and pathogen detection in liquid anaerobic digestates. Waste Manag. 78, 8–15. doi: 10.1016/j.wasman.2018.05.017
Da Ros, C, Libralato, G., Ghirardini, A. V., Radaelli, M., Cavinato, C. (2018). Assessing the potential phytotoxicity of digestate from winery wastes. Ecotoxicol. Environ. Safety 150, 26–33. doi: 10.1016/j.ecoenv.2017.12.029
Elaiyaraju, P., and Partha, N. (2012). Biogas production from co-digestion of orange peel waste and jatropha de-oiled cake in an anaerobic batch reactor. African J. Biotechnol. 11, 3339–3345. doi: 10.5897/AJB11.2622
Galvez, A., Sinicco, T., Cayuela, M. L., Mingorance, M. D., Fornasier, F., and Mondini, C. (2012). Short term effects of bioenergy by-products on soil C and N dynamics, nutrient availability and biochemical properties. Agric. Ecosyst. Environ. 160, 3–14. doi: 10.1016/j.agee.2011.06.015
Izumi, K., Okishio, Y. K., Nagao, N., Niwa, C., Yamamoto, S., and Toda, T. (2010). Effects of particle size on anaerobic digestion of food waste. Int. Biodeterior. Biodegrad. 64, 601–608. doi: 10.1016/j.ibiod.2010.06.013
Jiang, Y., Dennehy, C., Lawlor, P. G., Hu, Z., McCabe, M., Cormican, P., et al. (2018). Inhibition of volatile fatty acids on methane production kinetics during dry co-digestion of food waste and pig manure. Waste Manag. 79, 302–311. doi: 10.1016/j.wasman.2018.07.049
Kuczman, O., Gueri, M. V. D., De Souza, S. N. M., Schirmer, W. N., Alves, H. J., Secco, D., et al. (2018). Food waste anaerobic digestion of a popular restaurant in Southern Brazil. J. Clean. Prod. 196, 382–389. doi: 10.1016/j.jclepro.2018.05.282
Medvedeff, C. A., Inglett, K. S., and Inglett, P. W. (2014). Evaluation of direct and indirect phosphorus limitation of methanogenic pathways in a calcareous subtropical wetland soil. Soil Biol. Biochem. 69, 343–345. doi: 10.1016/j.soilbio.2013.11.018
Mitelut, A. C., and Popa, M. E. (2011). Seed germination bioassay for toxicity evaluation of different composting biodegradable materials. Romanian Biotechnol. Lett. 16, 121–129.
Rajput, A. A., and Visvanathan, C. (2018). Effect of thermal pretreatment on chemical composition, physical structure and biogas production kinetics of wheat straw. J. Environ. Manag. 221, 45–52. doi: 10.1016/j.jenvman.2018.05.011
Serrano, A., Siles, J. A., Chica, A. F., and Martín, M. A. (2013). Agri-food waste valorization through anaerobic co-digestion: fish and strawberry residues. J. Clean. Prod. 54, 125–132. doi: 10.1016/j.jclepro.2013.05.002
Shaikh, I. R., Shaikh, P. R., Shaikh, R. A., and Shaikh, A. A. (2013). Phytotoxic effects of heavy metals (Cr, Cd, Mn and Zn) on wheat (Triticum aestivum L.) seed germination and seedlings growth in black cotton soil of Nanded, India. Res. J. Chem. Sci. 3, 14–23.
Smith, A. D., and Holtzapple, M. T. (2011). Investigation of the optimal carbon–nitrogen ratio and carbohydrate-nutrient blend for mixed-acid batch fermentations. Bioresour. Technol. 102, 5976–5987. doi: 10.1016/j.biortech.2011.02.024
Sosnowski, P., Wieczorek, A., and Ledakowicz, S. (2003). Anaerobic co-digestion of sewage sludge and organic fraction of municipal solid wastes. Adv. Environ. Res. 7, 609–616. doi: 10.1016/S1093-0191(02)00049-7
Surra, E., Bernardo, M., Lapa, N., Esteves, I., Fonseca, I., Motab, J. P., et al. (2018). Maize cob waste pre-treatment to enhance biogas production through co-anaerobic digestion with OFMSW. Waste Manag. 72, 193–205. doi: 10.1016/j.wasman.2017.11.004
Taherzadeh, M. J., and Karimi, K. (2008). Pretreatment of lignocellulosic wastes to improve ethanol and biogas production: a review. Int. J. Mol. Sci. 9, 1621–1651. doi: 10.3390/ijms9091621
Tang, Y., Li, X., Dong, B., Huang, J., Wei, Y., Dai, X., et al. (2018). Effect of aromatic repolymerization of humic acid-like fraction on digestate phytotoxicity reduction during high-solid anaerobic digestion for stabilization treatment of sewage sludge. Water Res. 143, 436–444. doi: 10.1016/j.watres.2018.07.003
Tornwall, E., Pettersson, H., Thorin, E., Schwede, S. (2017). Post-treatment of biogas digestate-an evaluation of ammonium. Energy Procedia 142, 957–963. doi: 10.1016/j.egypro.2017.12.153
Trzcinski, A. P., and Stuckey, D. C. (2011). Parameters affecting the stability of the digestate from a two-stage anaerobic process treating the organic fraction of municipal solid waste. Waste Manag. 31, 1480–1487. doi: 10.1016/j.wasman.2011.02.015
US EPA (2001). Total, Fixed, and Volatile Solids in Water, Solids, and Biosolids (Method 1684). Washington, DC: US Environmental Protection Agency.
Wang, X., Lu, X., Li, F., and Yang, G. (2014). Effects of temperature and carbon-nitrogen (C/N) ratio on the performance of anaerobic co-digestion of dairy manure, chicken manure and rice straw: focusing on ammonia inhibition. PloS ONE 9:97265. doi: 10.1371/journal.pone.0097265
Wojnowska-Baryła, I., Bernat, K., and Sartowska, S. (2018). Biological stability of multi-component agri-food digestates and post-digestates. Waste Manag. 77, 140–146. doi: 10.1016/j.wasman.2018.05.016
Yang, L., Huang, Y., Zhao, M., Huang, Z., Miao, H., Xu, Z., et al. (2015). Enhancing biogas generation performance from food wastes by high-solids thermophilic anaerobic digestion: effect of pH adjustment. Int. Biodeteriorat. Biodegrad. 105, 153–159. doi: 10.1016/j.ibiod.2015.09.005
Yuan, H., Lan, Y., Zhu, J., Wachemo, A. C., Li, X., and Yu, L. (in press). Effect on anaerobic digestion performance of corn stover by freezing-thawing with ammonia pretreatment. Chin. J. Chem. Eng. doi: 10.1016/j.cjche.2018.04.021
Zhang, B., Zhang, L. L., Zhang, S. C., Shi, H. Z., and Cai, W. M. (2005). The influence of pH on hydrolysis and acidogenesis of kitchen wastes in two-phase anaerobic digestion. Environ. Technol. 26, 329–339. doi: 10.1080/09593332608618563
Zhang, W., Xing, W., and Li, R. (2018). Real-time recovery strategies for volatile fatty acid-inhibited anaerobic digestion of food waste for methane production. Bioresour. Technol. 265, 82–92. doi: 10.1016/j.biortech.2018.05.098
Keywords: anaerobic digestion, biorefinery, bioenergy, digestate, phytotoxicity, organic waste
Citation: Anjum M, Qadeer S and Khalid A (2018) Anaerobic Co-digestion of Catering and Agro-Industrial Waste: A Step Forward Toward Waste Biorefinery. Front. Energy Res. 6:116. doi: 10.3389/fenrg.2018.00116
Received: 07 August 2018; Accepted: 18 October 2018;
Published: 08 November 2018.
Edited by:
Abdul-Sattar Nizami, Center of Excellence in Environmental Studies, King Abdulaziz university, Saudi ArabiaReviewed by:
Vivekanand Vivekanand, Malaviya National Institute of Technology, Jaipur, IndiaQaisar Mahmood, COMSATS University Islamabad, Pakistan
Mohammad Rehan, King Abdulaziz University, Saudi Arabia
Copyright © 2018 Anjum, Qadeer and Khalid. This is an open-access article distributed under the terms of the Creative Commons Attribution License (CC BY). The use, distribution or reproduction in other forums is permitted, provided the original author(s) and the copyright owner(s) are credited and that the original publication in this journal is cited, in accordance with accepted academic practice. No use, distribution or reproduction is permitted which does not comply with these terms.
*Correspondence: Muzammil Anjum, muzammilanjum@gmail.com