- 1Center For Energy and Environment, Malaviya National Institute of Technology, Jaipur, India
- 2Biotechnology Division, Protein and Carbohydrate Research Laboratory, Department of Engineering Technology, College of Technology, University of Houston, Houston, TX, United States
- 3Anaerobic Digestion Research and Education Center, Biosystems and Agricultural Engineering, Michigan State University, East Lansing, MI, United States
- 4Department of Microbiology, School of Life Sciences, Central University of Rajasthan Bandarsindri, Kishangarh, India
Organic fraction of municipal solid waste and its proper disposal is becoming a serious challenge around the world. Environmental pollution, public health risk, and scarcity of dumping land are the aftereffects of its improper disposal. Embodied energy recovery associated with the organic waste along with waste minimization may be achieved using anaerobic digestion. The chemical composition of the substrate plays a crucial role among the factors responsible for digestion performance and cumulative methane production. Treatment of substrate to enhance the digestion performance is gaining momentum in the recent years. This review provides an overview of different treatment methodologies including mechanical, thermal, chemical, biological, ultrasonic, and microwave approaches to enhance methane yield of anaerobic digestion of organic fraction of municipal solid waste (OFMSW). Environmental impact analysis of treatment techniques, along with comparison of treatment methodologies and techno-economic assessment, has also been discussed to provide a proper insight into the various processing methods.
Introduction
The organic fraction of municipal solid waste (OFMSW) is widely used as a feedstock for anerobic digestion (AD) and promising source for biogas generation (van Lier et al., 2001; Abudi et al., 2016). AD is a natural practice that breaks feedstock into renewable fuel (here biogas) and digestate by microbial consortium in absence of oxygen. The biogas from AD comprises of CH4 (50–70%), CO2 (30–50%) and few notable impurities such as NH3, H2S, siloxane, halides and water vapors (Bhakov et al., 2014). Biogas generated by digesting OFMSW anaerobically may be utilized directly in combined heat and power (CHP) units, cooking or may be purified for transportation purpose (Parthiba Karthikeyan et al., 2017). The anaerobic treatment of digestion run-off can produce a sustainable residue to be employed as a soil amendment or re-condition certain type of soil (Castillo et al., 2006). Furthermore, another motivation to apply AD to treat the waste is that the producers can acquire emission reduction (CER) credits by clean development mechanism (CDM) defined in the Kyoto Protocol (International Panel on Climate Change (IPCC) Guidelines, 2006; Tong and Jaafar, 2006). Besides the environmental benefits of waste treatment (Edelmann et al., 2005), government agencies and private companies have extensively invested in AD technologies due to the increasing energy demand and environmental concerns such as global warming. Emphasis is mainly given to increase the generation of biogas, accomplish better rate of breakdown in the reactor, and subsequently minimized organic waste residue (Sonesson et al., 2000) to make the process economical.
Since the substrates used in AD are relatively complex, it has been reported that the hydrolysis is the rate-limiting phase among the four phases that take place during AD namely hydrolysis, acidogenesis, acetogenesis, and methanogenesis (Rafique et al., 2010; Ma et al., 2011). This remark was based on characterizing individual biodegradation kinetics of carbohydrate, lipids, and protein (Table 1). Hydrolysis step also generates by-products like volatile fatty acids (VFAs) that are undesirable for the bioreactor in excess amount (Neves et al., 2006; Lu et al., 2008) that inhibit methanogenic bacteria (Lu et al., 2008). Numerous studies have been reported using different treatment techniques to accelerate the hydrolysis phase (Val del Rio et al., 2011; Carlsson et al., 2012) without producing toxic by-products (Neves et al., 2006). Several methods have also been reported to recover components such as nitrogen and phosphorus from the waste and using them as soil amendments (Müller, 2001).

Table 1. Hydrolysis rate constant for primary substrates (APAT manual, 2005).
Complexity of organic waste may hinder the overall methanogenesis process (Kumar et al., 2018). This complexity may be altered by different pretreatment (Mechanical, thermal, biological, chemical, etc.) technique by breaking the molecular fencing around it. In a study performed by Cano et al. (2014), thermal hydrolysis of biological sludge, cow manure, grease waste, spent grains and OFMSW were attempted before AD. Results showed that methane production increased by 1.5-folds. Also, it was concluded theoretically, that integration of thermal hydrolysis may enhance up to 40% of plant's income. Biological co—pretreatment was attempted by Zhang et al. (2017) for enhanced methanogenesis. Food waste and waste activated sludge were investigated by the research group and results showed an increment of 25% in methane yield.
The objective of this paper is to discuss the complexity of the OFMSW and feasibility of various pretreatment methodologies to overcome its complex nature. Techno-economic aspects as well as environmental impacts of pretreatment are also discussed to explore the relation between pretreatment technique and its impact on the environment. Comparison of pretreatment is provided for the readers to get a deep insight of pretreatment methodology. At last, challenges associated with the biogas and its uses are discussed in detail for a deeper comprehension.
Categorization of the OFMSW
AD has been inclusive for the handling of the OFMSW from last few decades (Luning et al., 2003). Contemporary trend is focused toward the linking of anaerobic and aerobic processes (Pognani et al., 2009), to acquire a net energy gain by methane and the production of soil conditioner from the substrates. The OFMSW is assorted based on its composition, source and biological structure (Tables 1–4) (Abdullah et al., 2008) and hence, tremendous variations have been observed in its specific content depending on the source. The basis of categorization should include regional, seasonal and socio-economic impacts (Tyagi et al., 2018). OFMSW includes food waste, kitchen waste, leaf, grass clippings, flower trimmings and yard waste. Wasted food epitomizes a noteworthy proportion of organic material. Colonized and commercial kitchens leftovers also comprise of a considerable portion of OFMSW. Yard waste involves lignocellulosic-based materials explicitly grass off cuts and straw, leaves, weeds, bush and tree trimmings, whose production varies depending on the season.
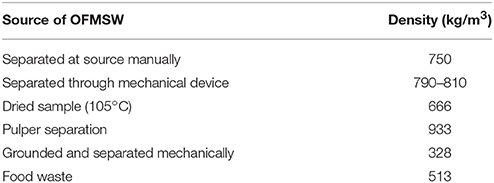
Table 2. Density of OFMSW based on the source (Campuzano and Simón, 2016).
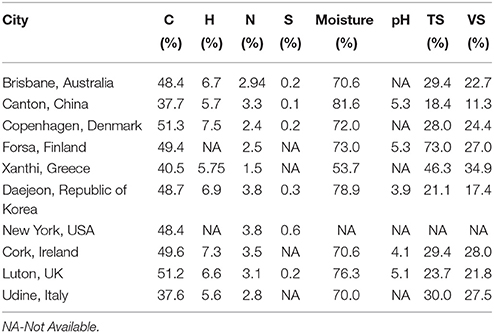
Table 3. Ultimate analysis of OFMSW at globe level (Campuzano and Simón, 2016).
Different Methods Used to Treat OFMSW
Improvement of AD process depends on treatment that is a fundamental step and alters the substrate features. The biogas yield of OFMSW through AD is considerably affected by the substrate availability and mass transfer (Li and Noike, 1992). Treatment ensures increased accessibility of microbes to the nutrients and subsequent product generation (Naran et al., 2016). The basic target of treatment techniques i.e., mechanical, thermal, chemical, biological is to break the barrier (Figure 1) between the microbes and nutrient to accelerate the chemical reaction (Figure 2). Researchers associated with the treatment techniques of OFMSW have mostly opted for thermal, chemical and mechanical processes accounting for 24, 21, and 33% respectively (Mata-Alvarez et al., 2014). Hydrolysis phase is the most decisive step for the overall performance of the biogas digester as in this phase conversion of complex compounds to oligomeric and monomeric units occurs (Rafique et al., 2010; Paritosh et al., 2017a) and it may limit the rate of the subsequent reactions.
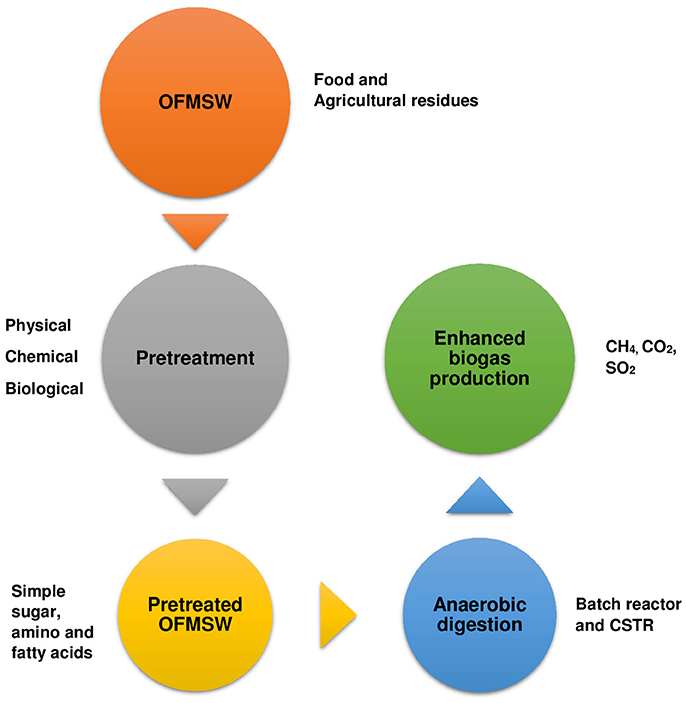
Figure 2. Process flow diagram for treatment and anaerobic digestion of organic fraction of municipal solid waste by treatment.
Also, bromatology of OFMSW plays a substantial role in the hydrolysis rate owing to varying properties of diverse substrates (Table 1).
Mechanical Treatment
Mechanical treatment crumbles compact assembly (Figure 3) of the substrates, consequently liberating OFMSW cell wall. Liberated cell wall has increased surface area for improved contact with microorganism for enhanced biomethanation (Kim et al., 2000; Elliot and Mahmood, 2012). Thus, mechanical treatment techniques such as (i) sonication, (ii) high-pressure homogenization and (iii) steeping or maceration are conducted to reduce the particle size of the substrate. It has been reported by Kim et al. (2000) that rate of utilization of feedstock is firmly depends on its size inversely for microbial consumption. Also, if the size of substrate is large, chemical oxygen demand (COD) solubilization decreases and it may result in declined production of biogas (Esposito et al., 2011).
Mechanical pretreatment strategy also affects the utilization pattern of substrate by microbes. Hartmann et al. (2000), discovered that while steeping the feedstocks, shearing effect played crucial role in increasing the gas production to the cutting of substrate fiber. In another reported study, the cell structure of the substrate was interrupted by sonication (Elliott and Mahmood, 2007). A group of researchers used Hollander beater to treat waste paper. The findings revealed that treating waste paper for 30 min had no significant impact on biogas yield. However, increasing time to 60 min augmented the biogas yield by 21% compared to untreated sample (Rodriguez et al., 2017). Ultrasonic treatment has also been reported to perform the particle size reduction at a low frequency ranging from 20 to 40 kHz of sound waves, and enhance microbial penetration to the substrates (Chua et al., 2002).
A number of studies have verified that reduced particle size enhances the surface area accessibility to the microbes, resulting in improved nutrient availability and boosts up the anaerobic process (Mshandete et al., 2006). On the contrary, a decrease in particle size may increase the hydrolysis and acidogenesis that led to the generation of soluble organic material as VFAs, resulting in excessively high organic loads in AD reactor. Izumi et al. (2010), studied the effect of particle size reduction and solubilization on biogas production from food waste (FW). The study showed that grinding FW by bead milling (Figure 4) led to 40% more solubilisation of COD; leading to 28% enhanced biogas production compared to untreated FW. However, extreme size reduction was reported to trigger VFA accumulation with decreased solubility as well as methane production. Also, the robust structure of lignocellulosic fraction of OFMSW makes its size reduction energy intensive. Accompanying chemical treatment processes prior to wood size reduction may provide a better alternative (Zhu and Pan, 2010).
High-pressure homogenizer expands the pressure up to a few hundred bars, prior to the homogenization of the substrate under depressurization step (Mata-Alvarez et al., 2000). The formed cavitation prompts internal energy, which disturbs the cell layers (Barjenbruch and Kopplow, 2003). These treatment techniques have been employed on different substrates including lignocellulosic biomass, excrement and wastewater treatment plant discharge. Some of the benefits of mechanical treatment include, no unpleasant odor problem, simple execution, better dewaterability of the anaerobic deposit and direct energy utilization (Perez-Elvira et al., 2006; Toreci et al., 2009). Mechanical treatment process like electroporation and liquefaction also aid in disrupting the cell wall (Shepherd, 2006) of OFMSW residues and have been demonstrated at lab scale. While, other mechanical treatments such as revolving drum, plate screen shredder and cylinder squeeze were effectively demonstrated at pilot scale (Carlsson and Anox Kaldnes, 2008). Some mechanical treatment practices involve crushing of the waste with high weight into an expulsion chamber to press-out the wet portion from the expulsion openings (Gonella, 2011). This technique has been in practice and is incorporated in an AD plant in Salerno (Italy) for treating OFMSW (Nayono et al., 2010).
Mechanical treatment viz. the rotational drum (Figure 4) was utilized as a compelling innovation for OFMSW, which improves the biogas generation in the range of 18–36% (Zhu et al., 2009; Subramani and Ponkumar, 2012). Both improvements; i.e., methane yield per gram of volatile solids and amount of methane in biogas have been reported (Davidson et al., 2007). However, Zhang and Banks (2013) reported no significant improvement in biogas yield employing rotational drum treatment. Hansen et al. (2007) reported the impacts of the similar treatment approaches on the nature and source of OFMSW. Interestingly, Bernstad et al. (2013) reported that the screw press treatment results in significant loss of biodegradable substrate after processing. Fantozzi and Buratti (2011) highlighted the significance of the inoculation mechanically treated waste substrates that provide large accessible surface area for microbes.
Thermal Treatment
Thermal treatment is often referred as molding procedure for raw or processed waste as it enhances the dewater ability properties of the waste (Bougrier et al., 2007). Thermal treatment modifies the structure of the insoluble part of the substrate and makes them amenable for biodegradation. Kuo and Cheng (2007) contemplated thermal treatment of kitchen waste at different temperatures (37, 50, and 60°C), to assess its impacts on hydrolysis. The treatment at 60°C showed best results, accomplishing a hydrolytic proficiency of 27.3% and fat removal of 37.7%, as compared to untreated sample. The study demonstrated stable operation and positive execution with respect to treatment of kitchen waste. Comparable results were reported by Komemoto et al. (2009).
Thermal treatment aids into pathogen expulsion, enhances dewatering and reduces thickness of the digestate (Liu et al., 2012). A wide range of temperatures (50–250°C) to upgrade the AD of diverse organic fraction of solid wastes have been worked-out by various groups of reseachers. The fundamental impact of thermal treatment is the disruption of cell wall and aiding into solubilization of organic material (Marin et al., 2010; Protot et al., 2011). COD solubilization and temperature have direct impact on biogas yield. Higher solubilization may likewise be accomplished at lower temperatures; however, the longer treatment time is required. Mottet et al. (2009) studied diverse thermal treatment techniques and reported no considerable distinction amongst steam and electric heating, while microwave heating has been observed to solubilize more biopolymers. The higher rate of solubilization with microwave treatment was due to the phenomenon of polarization of macromolecules present in the feedstock (Toreci et al., 2009; Marin et al., 2010). Thermal treatment at significantly high temperatures (>170°C) may result into formation of bonds and agglomeration of the particles (Bougrier et al., 2006). Maillard reaction is reported to produce complex recalcitrant substrates from starch and amino acids, when subjected to high thermal treatment (150°C) or longer treatment time at lesser temperature (<100°C) (Carrere et al., 2010; Elliot and Mahmood, 2012).
Protot et al. (2011) recommended that thermal treatment at temperatures lower to 100°C did not bring about the debasement of complex particles, rather it essentially incites the separation of macromolecules. Barjenbruch and Kopplow (2003) acquired a comparable conclusion with treatment at 90°C. The outcomes from these experiments demonstrated that the fibers were not broken down, rather, they were just mugged with thermal treatment.
Release of particulate sugars and solubilization of proteins was reported by Neyens and Baeyens (2003) by employing thermal treatment.
The impacts of thermal treatment on the chemical and physical properties of kitchen waste, vegetable waste and waste activated sludge were explored by Liu et al. (2012). Outcomes revealed that thermal treatment (175°C, 60 min) diminished the viscosity and increased COD, dissolvable sugar and proteins. A reduction of 7.9 and 11.7% of methane was observed for kitchen and vegetable waste respectively. The authors ascribed this phenomenon to the arrangement of an intractable copolymer and melanoidin (Maillard reaction products). Under related working conditions (170°C, 1 h), Qiao et al. (2011) observed that both biogas and methane generation from anaerobically treated FW and cow manure were diminished by 3.4 and 7.5% respectively as a result of lower pH and higher VFAs. Ma et al. (2011) reported a 24% enhancement in CH4 production when FW was treated at 120°C.
Chemical Treatment
A range of chemicals viz. acids, base, and oxidants (e.g., ozone, peroxide) have been successfully employed to break down natural constituents (Carrere et al., 2010). Chemical treatment is utilized to breakdown the linkages in plant cell wall by employing strong acids, alkali or oxidants. Alkali treatment is considered as the favored chemical treatment when compared to other treatment methods (Li et al., 2012). During alkali treatment, the primary responses that happen to the substrate are solvation and saponification, which instigates the swelling of solids (Carlsson et al., 2012). Subsequently, the surface region is expanded, and the substrates are effectively available to anaerobes (López Torres and Espinosa Lloréns Mdel, 2008; Modenbach and Nokes, 2012).
Ozonation is a technique by which biogas upgradation may be achieved along with enhanced hydrolysis rate. However, chemical treatment is believed to be less appropriate for easily biodegradable or less recalcitrant substrates. Feedstocks encompassing high amounts of starch, showed accelerated biodegradation resulting in access VFA, which may inhibit methanogenesis step (Wang L. et al., 2011). However, it may have beneficial outcomes on lignin rich substrates that has complicated network of lignin carbohydrate complex linkages (Fernandes et al., 2009). López Torres and Espinosa Lloréns Mdel (2008) reported an increased OFMSW AD efficacy after a primary treatment with Ca(OH)2. The addition of 62.0 mEq Ca(OH)2/L along with stirring for 6 h resulted in 11.5% increment in the soluble COD (sCOD) out of the aggregate COD. Moreover, the addition of the Ca(OH)2 showed reduction in biomass solubilization, which could be ascribed to the arrangement of complex, non-dissolvable compounds. Neves et al. (2006) reported that both; a basic hydrolysis treatment and co-assimilation with kitchen waste were positive to upgrade the methane generation from grain (barley) waste, yet the best results have been observed with alkaline hydrolysis in terms of decrease in total solid (TS) and volatile solid (VS). Maintaining pH during AD process may be an expensive procedure while treating large amounts of waste. This is considered as one of the possible obstacles toward employment of alkaline treatment at large-scale operations. In addition, using alkaline treated samples, in continuous stirred type reactors (CSTRs) has resulted in decreasing acetate and glucose consumption by 5 and 50% respectively because of generation of toxic compounds during the saponification in spite of the fact that sodium or potassium were 0.21 mol/L in the medium (Ward et al., 2008).
In recent years, the utilization of ozone as treatment reagent is gaining a large attention due to its very powerful oxidant property, with a redox potential of 2.07. In addition, it has several advantages, which include: (i) on-site generation, (ii) leaving no traces in the substrate and (iii) do not produce toxic halogenated compounds. However, the adequately high ozone dose promotes, mineralization of the released cell compounds (Elliott and Mahmood, 2007). The effectiveness of ozone relies on the type of biodegradable waste, ozone concentration and pH. Most studies allude to the utilization of ozone for sludge treatment and then subjecting to AD process (Delgenès et al., 2003; Chu et al., 2008).
Solid waste ozonation was performed by Cesaro and Belgiorno (2013) and reported that an ozone dosage of 0.16 g O3/gTS could increase sCOD by 55%, and bringing about 37% increment in biogas production levels. However, higher ozone concentration showed no increase in methane production irrespective of substrate solubilisation (Bougrier et al., 2006; Baratharaj, 2013). Bioconversion process requires stable pH for optimal performance during AD (Pavlostathis and Gosset, 1985). However, chemical treatment employing ozonation have resulted into decrease in pH, hence use of alkali to neutralize the pH is considered as an essential step before the start of AD process.
Acid treatment is attractive for lignocellulosic substrates since it solubilizes hemicelluloses, condenses lignin that precipitates and aids in hydrolysis which functions optimally in acidic conditions (Mata-Alvarez, 2003; Mussoline et al., 2012). One major drawback of acid treatment is that employment of strong acids may result in the formation of inhibitory products such as furfural and hydroxymethylfurfural (HMF) (López Torres and Espinosa Lloréns Mdel, 2008; Mussoline et al., 2012). Other drawback associated with acidic treatment includes loss of fermentable sugars because of the continued degradation of complex substrates, the high cost of acids. Additional cost associated with base that is needed to neutralize treated substrate before the AD process has been reported to be a matter of concern (López Torres and Espinosa Lloréns Mdel, 2008; Kumar and Murthy, 2011). In few cases, adding acids can be effective for the anaerobic absorption of protein-rich substrates by generation of ammonia. However, this may hinder the biogas production (Hansen et al., 1998) due to the fact that microbes get inhibited in the presence of ammonia. In spite of the interesting results from anaerobic trials of chemically treated waste, plenty of room is available for improvement in pH restoration during pilot scale applications for economic viability as well as sustainability of the process.
Biological and Biochemical Treatment
Promoting microbial growth on biomass could notably enhance hydrolysis of the substrate. This could be accomplished by the application of commercially available enzymes as well as growing microbes on it (Yin et al., 2016). Fdéz-Güelfo et al. (2011a) reported that the addition of compost (made from manure) to industrial OFMSW helps in reducing the dissolved organic compound (DOC) by 61% and VS by 35% compared to control. As a result, the biogas and methane generation increased up to 60 and 73%, respectively. While comparing the use of manure compost, biological treatment (using fungus Aspergillus awamori) showed better hydrolysis results (Fdéz-Güelfo et al., 2011b). Several other studies concluded the superiority of employing biological treatment compared to thermochemical-treated substrates for increased AD rate (Fdéz-Güelfo et al., 2011b; Fdez-Güelfo et al., 2012).
Charles et al. (2009) studied the impacts of pre-air circulation to OFMSW and its effect on AD and concluded that aerobic treatment constrained the amount of methane generated as opposed to fortifying the process. The term “micro aeration” was defined as the slightly introducing oxygen into an anaerobic process to empower aerobic organisms to act on organic substrates inside bioreactor. Researchers revealed that increased solubilization and fermentation was accomplished with micro aeration at 37.5 mLO2/d. About 21 and 10% higher methane generation was observed during anaerobic co-processing of brown water and FW respectively in the reactor when infused with sludge. Lim and Wang (2013) reported that oxygen introduction could stimulate VFA production due to improved activity of hydrolytic and acidogenic microbes. Pre-composting of OFMSW resulted in the significant loss in methane production as reported by Brummeler ten and Koster (1990) and Mshandete et al. (2005). On the other hand, Miah et al. (2005) studied the biogas generation from sewage sludge treated with aerobic thermophilic microbes, Geobacillus thermodenitrificans. The study reported significant production of biogas (70 ml/gVS) with 80–90% methane content in AD at 65°C.
Few authors considered the hydrolysis and acidogenisis steps (first and second steps) of a two-stage AD process as a biological treatment option (Carrere et al., 2010; Ge et al., 2010, 2011a,b), while others consider it as a general setup of AD bioreactor and not an alternative of pretreatment (Carlsson et al., 2012). Physically isolating the acidogenic microbes from the methanogenic microbes may bring about increased methane generation. It has been stated that enhancing the hydrolysis stage of an AD bioreactor could excite the acidogens to produce enzymes with higher specificity for prolonged degradation of substrates (Parawira et al., 2005). In a study performed by Verrier et al. (1987), 2–phase biomethanation of vegetable waste was compared with mesophilic and thermophilic single stage CSTRs respectively. It was concluded that a two-stage reactor converted over 90% of easily biodegradable waste to biogas. Also, the mesophilic and thermophilic single stage CSTR's was not able to handle higher organic loading as compared to 2-phased one (Verrier et al., 1987).
Impact of pH value on two-stage AD was studied by Zhang et al. (2005). The research group recommended maintaining the pH at 7 while hydrolysis is being carried out in the reactor. This would enhance the overall organic solid consumption and help to enhance the biogas production. White rot fungi, known to consume lignin, leaving behind cellulose may be effectively applied for biological treatment. Group of researchers have already reported the high de-lignification productivity of various white rot fungi on different lignocellulosic biomass (Keller et al., 2003; Shi et al., 2009; Kumar and Wyman, 2010). Keller et al. (2003) concluded that treating lignocellulosic residues using fungi could bring several benefits such as (i) eco-friendly procedure, (ii) no chemical requirement, (iii) decreased energy input, (iii) operating at ambient conditions, (iv) inexpensive unit operations, (v) less by-product generation, (vi) no washing step and (vii) negligible inhibiting agent production. However, Shi et al. (2009) contemplated that only drawback of fungal treatment is that it's time-consuming process and require considerable amount of space and additional infrastructure to hold the substrate for a period of 20–30 days. Pasteurization of substrate prior to fungal mycelium inoculation will add additional cost to the process.
Researchers have also evaluated the role of extracellular hydrolytic enzymes to increase the yield and the rate of organic matter solubilization of lignocellusolic biomass during AD. Moreover, the possible positive effects of the hydrolytic enzymes by adding to municipal sludge for solubilization were known since 1999 (Delgenès et al., 2003). Nevertheless, selection of appropriate commercial enzyme preparation is a prerequisite to achieve noteworthy outcomes (Mallick et al., 2010). However, the cost of commercial enzymes is a potential barrier to make the process economically viable.
Enzymatic hydrolysis of OFMSW is quite challenging due to its heterogeneous and inconsistent composition. Hence, development and utilization of enzyme cocktails that break down complex substrates i.e. carbohydrates (cellulase, hemicellulase, pectinase), lipids (lipase, lipolytic acyl hydrolase, lipoxygenase) and proteins (protease) are required. The resultant products are simple molecules like sugars, fatty acids and amino acids, which may further be utilized as nutrient source by microbes. Masse et al. (2003) and Valladão et al. (2007) evaluated the impacts of enzymatic hydrolysis on the anaerobic digestion of fat present in poultry waste. Masse et al. (2003) reported that a little impact on fat molecule assimilation at 25°C occurred by enzyme addition, while Valladão et al. (2007) reported enhancement in crude effluent anaerobic treatment by employing biomass degrading microbes as a part of the pre-hydrolysis step. Lipase was successfully employed to hydrolyze the fats present in dairy wastewater by Cammarota et al. (2001) using a bench scale UASB reactor. Leal et al. (2002), reported that utilizing the blend of enzymes and microbes in bioreactors to treat the fat was viable and hybrid treatment. Mendes et al. (2006) employed a cost efficient and easily accessible lipase preparation derived from an animal source to perform enzymatic hydrolysis of lipid-rich wastewater from a dairy farm. The hydrolysis was performed at varying incubation periods ranging from 4 to 24 h at a fixed temperature (35 ± 1°C) and the treatment proficiency was confirmed by running relative biodegradability tests. All treated measures indicated higher response rate when compared to crude wastewater examined, as evident by enhanced levels of biogas generation and higher removal of organic matter.
Currently, researchers are focusing on development of temperature phased anaerobic digestion (TPAD) (Figure 5). This strategy often comprises of an essential reactor at thermophilic (or hyperthermophilic) temperature trailed by using mesophilic auxiliary rectors. Advantages using TPAD include, higher methane yield as well as a pathogen free high quality nutrient rich digestate that may be utilized as soil conditioner (Sung and Harikashan, 2001; Riau et al., 2010). Schmit and Ellis (2001) has reported that conventional AD processes was outperformed by TPAD. Lee et al. (2009) utilized FW and excess sludge using TPAD at 70°C for 4 days in the main bioreactor, followed by a subordinate bioreactor that operates at different temperatures viz. 35, 55, and 65°C. Wang F. et al. (2011) compared treatment of FW with polylactide using TPAD processing at two different conditions 80°C (hyperthermophiles) and 55°C (thermophiles) followed by digesting the materials using mesophilic reactor.
Ultrasound Treatment
Ultrasound treatment (Figure 6) depends on stable cavitation along with physical and chemical impacts in fluid dynamics (Dehghani, 2005). The physical impacts are created by the breakdown of cavitation air pockets, which thus delivers a lifted change in the chemical nature through the development of free radicals (Mason and Peters, 2002). These impacts may enhance anaerobic assimilation yields by physical crumbling and improvement of microbial pool (Kwiatowska et al., 2011), contingent upon the treatment conditions. It has been demonstrated that higher ultrasound power may denature the enzymes, while lesser ultrasound span brought better responses of the same enzymes (Yu et al., 2009). Chen L. et al. (2008) examined impacts of ultrasound procedure on hydrolysis and acidogenesis of organic waste and reported 53% degradation in volatile solids as compared to the control. The improvement could be attributed to desorption of VFA's that are present on substrate surfaces. Comparative results were obtained by treating a blend of mechanically sorted OFMSW and sewage waste with ultrasound, before anaerobic absorption (Cesaro et al., 2012). Elbeshbishy et al. (2011a) observed 27% improvement in hydrogen generation in a CSTR reactor using sonicated FW when compared to control. In another study (Elbeshbishy and Nakhla, 2011), enhanced performance could be achieved by incorporating ultrasound inside the hydrogen reactor. In this study, isolated organics were sonicated for 24 min using a lab-scale ultrasonic test followed by single-stage and two-stage anaerobic digestion. In another report, sonicated biological hydrogen reactor was setup in the first stage for generating hydrogen, followed by blended tank reactor for methane production as the second stage.
The most astounding overall COD diminishing proficiency of 9.3% was achieved in sonicated biological hydrogen reactor (SBHR), while a total COD reduction of 6.4% was achieved in both reactors with either non-sonicated or sonicated feedstocks. Applying sonication inside the reactor demonstrated better results than sonication of the feedstock outside the reactor at a similar specific energy of 5,000 kJ/kg TS. The noteworthy increment in yield was observed when ultra-sonication was carried out inside the reactor such as (i) solubilisation of the particulate organics, (ii) expulsion of the dissolved gases, (iii) change in mass transfer and (iv) increment in the growth rate of the microbes. Ultrasound process ended up being the most adaptable, as it was compelling with various sort of fat-dominated substrates, originated from meat processing units when compared to thermal, alkaline, acid and biological treatment processes (Luste et al., 2009). In a study performed by Cesaro and Belgiorno (2013), ultrasound appeared comparatively suitable for OFMSW when compared with ozonation in terms of 9% higher solubilization of organic matter with ultrasound treatment. Elbeshbishy et al. (2011b) reported about 16% higher biogas volume when subjected OFMSW to ultrasound treatment.
Shanthi et al. (2018) examined the effect of ultrasonication on fruit and vegetable waste assisted with sodium dodecyl sulfate. Result showed that optimum dose of sodium dodecyl sulfate was 0.035 g/g of suspended solid. Also, the energy ratio 0.9 for sodium dodecyl sulfate coupled ultrasonic treatment, which showed its energy efficiency.
Microwave Treatment
Microwave utilizes the capacity of direct interaction between an object and a connected electromagnetic field to expand heat (Figure 7; Hu and Wen, 2008). Due to this both thermal and non-thermal impacts were created in the aqueous solution. The electromagnetic field ruptures the crystalline structures and changes the super atomic structure of lignocellulosic materials, enhancing their reactivity (Tomas-Pejo et al., 2011).
The impact of microwave treatment on the anaerobic biodegradability of kitchen waste was contemplated by Marin et al. (2010). In this study, FW was treated at 175°C to solubilise the sugars and proteins present. However, enhanced solubilisation did not result into improved anaerobic biodegradability, contingent upon the rate of heating. Pecorini et al. (2016) reported that microwave treatment prompted to a methane generation increment of 8.5% for OFMSW with lignocellulosic materials; while autoclave treatment had an expansion extending from 1.0 to 4.4%. Results exposed an upsurge of the soluble fraction after treatments. A significant increase was detected in sCOD for treated substrates (up to 219. 8%). Significant improvement in methane generation prompted to the conclusion that subject the OFMSW to autoclave and microwave brought about the hydrolysis of a noteworthy fraction of non-biodegradable natural substances recalcitrance to anaerobic processing.
Shahriari et al. (2012) explored the impacts of microwave treatment, even in a blend with hydrogen peroxide, on OFMSW bound to anaerobic treatment. Experiments were performed by heating samples from room temperature to 115, 145, and 175°C at a consistent temperature slope time of 40 min. For the consolidated microwave-chemical treatment, tests were blended with 0.38 and 0.66 gH2O2/gTS and heated up to 85°C. Biochemical methane potential (BMP) was analyzed to appraise a definitive methane generation and also to assess any potential hindrance on anaerobic assimilation. Higher sCOD concentration was detected for substrates treated with microwave up to 175°C, H2O2 and joined alkaline/microwave process. These treatment conditions did not bring any subtle change in anaerobic absorption rate or in stabilization degree proposing that the solubilized compounds were less biodegradable (Marin et al., 2010).
High Voltage Pulse Discharge Treatment
High voltage pulse discharge (HYPD) has been utilized to treat biodegradable waste over the years (Lee and Chang, 2014). Zou et al. (2016) attempted HYPD treatment on FW. They discovered that the cumulative methane yield increased after the treatment. A total of 134% increase was recorded as compared to untreated one. The VS reduction was also improved after HYPD treatment. The FW treated with HYPD showed 54.3% VS reduction while VS reduction in untreated FW was 32.3%. In another study, grape pomace was treated with HYPD and compared with alkali, ultrasonic and acid treatments for enhanced methanogenesis by El Achkar et al. (2018) in a batch mode. Results showed that alkali treatment yielded highest methane.
Effect of Additive and Trace Metals on Anaerobic Digestion
With a specific end goal to guarantee rational hydrolysis improvement, it is important to keep a dynamic harmony between every progression. Additives may help to achieve better dynamic stability in methanogenesis (Milán et al., 2001). Some of the literature reported the management and utilization of various additives. Zeolites have been considered as the most preferred additive among the widely recognized ones (Yadvika et al., 2004). Zeolites are small permeable crystalline solids with well-characterized structure; contain silicon, aluminum and oxygen, water and additionally different atoms inside the pores. Most of the present forms of zeolites are naturally occurring minerals and are widely mined all through the world, while others are manufactured and arranged for specific affairs and as per requirement (Montalvo et al., 2006). On account of their novel permeable properties, zeolites are utilized as a part of an assortment of utilizations with a worldwide market of million tons/year. The fervor of zeolites concerning anaerobic assimilation procedures is their ability to adsorb salts especially ammonia, which acts as an inhibitor in the AD process (Tada et al., 2005; Chen Y. et al., 2008).
Kim et al. (2000) contemplated hindrance because of the sodium particle dose on the AD bioreactor running on thermophilic zone of FW and stated that sodium at a concentration of >5 g/L brought about decrease in biogas generation. Sodium is more lethal to propionic acid utilizing microorganisms when contrasted with other VFA debasing microorganisms in the AD system (Soto et al., 1993). The inhibitory effect of potassium begins at the level of 400 mg/L. Interstingly, anaerobic microbes can endure up to 8 g/L of potassium (Bashir and Matin, 2004). It has been reported that the potassium is harmful to thermophilic microbes but may not endanger the reactors running on mesophilic or psychrophilic temperatures (Chen Y. et al., 2008).
The ideal quantity of calcium and magnesium ions has been accounted to be 200 and 720 mg/L, respectively (Kugelman and McCarty, 1965; Schmidt and Ahring, 1993). Higher amounts of calcium may result into the scaling in the reactor because of precipitation of salts, and subsequently decrease the methanogenic process (Zhang et al., 2005). Additionally, a high concentration of the magnesium (100 mM) can interrupt methanogenesis followed by hindrance to the transformation of acetic acid (Schmidt and Ahring, 1993).
Moreover, AD could be improved by supplementation of a range of metals ions viz cobalt, molybdenum, selenium, iron, tungsten, copper and nickel, which assume a part in numerous biochemical responses of the anaerobic process and also play role of co-factors for enzymes involved in AD process. Zhang and Jahng (2012) utilized supplements of Fe, Co, Mo, and Ni to balance out a single stage reactor treating FW and reported that Fe was the best metal for adjustment of the AD procedure. Facchin et al. (2013) supplemented a metal cocktail (Co, Mo, Ni, Se, and W) into AD reactor and achieved 45–65% higher methane yield from FW. Supplementing trace metals to organic waste AD may help to achieve higher biogas generation rates with elevated methane composition in biogas (Paritosh et al., 2017b).
Comparison of Treatment Methods
Efficiencies, monetary attainability and ecological impacts are criteria for the selection of required treatment strategy prior to AD of OFMSW. There are different types of treatment technologies available based on the origin and variety of biomass. These technologies may be physical, mechanical, chemical, biochemical, thermal as well as combination of the treatment methods for disrupting the cell walls. Every technology has their own pros and cons and it may not be possible to recommend the treatment technology, which is suitable for all types of biomass, as every biomass is unique in terms of its chemical composition and hence a specific treatment method may be required for its disruption and obtain maximum energy. The effectiveness of any treatment strategy can be assessed through the increased methane yield and VS reduction. Table 5 illustrates the comparative proficiency of treatment methods including physical, biological, chemical and use of the additives for improving the AD of OFMSW.
Environmental Impacts of Treatment
Besides the energy balance and techno-economic investigation, environmental aspects i.e., pathogen expulsion, utilization of chemicals, the likelihood for a manageable utilization of the deposits and impacts on human health has to be considered while opting a treatment process (Stabnikova et al., 2008; Thorin et al., 2012; Di Matteo et al., 2017). The solids that are generated after AD process has the potential to be used as soil amendments, which is good for the environment. Life cycle assessments (LCA) will help to assess the efficiency and environmental impact of AD process. Only few researchers have assessed the environmental impacts of using treatment technologies before AD of solid wastes. Carballa et al. (2011) have performed the LCA to evaluate environmental attributes associated with the use of seven treatment technologies (alkaline, acid, thermal, thermal acid, freeze-thaw, pressurize-depressurize, and ozonation) for kitchen waste and sewage sludge. Impacts were analyzed with respect to the potential of abiotic resource depletion, eutrophication, global warming, human toxicity and terrestrial ecotoxicity. The researchers suggested that pressurize-depressurize and chemical treatment techniques outstripped ozonation, freeze–thaw and thermal strategies by having a minimum adverse environmental impact. Nwaneshiudu et al. (2016) assessed the environmental impact of mild bi-sulphite treatment of forest residues. The study reported less eutrophication impact of forest residues as compared to the effects from traditional beet and cane sugars, while the global warming impact falls within the range of conventional processes. However, the scarcity of literature regarding the evaluation of environmental impacts of treatment methodologies is inevitable. Adding environmental impact assessment in addition to technical and economic evaluation of treatment will help biogas production environmentally sustainable.
Techno-Economic Traits of Treatment
Development of different treatment methodologies has dramatically increased in the recent years. Mechanical innovations such as robotics have also been employed to minimize human contact with the waste. Combining with treatment, a segregation zone for workers to remove the inorganic materials manually is been practiced. Once the OFMSW has been stacked into the mechanical parcel system, human contact will be insignificant. OFMSW portion is easily breakable in diminutive particles compared to the inorganic materials. Balsari and Menardo (2011) reported that depending on lignocellulosic biomass composition, the energy consumption may differ between 5 and 80 kWh/ton. However, the volume of methane production from these treated wastes fluctuates between 14 and 26%.
The volume of methane production alone will not decide the profitability of the operation. One has to take into account the net energy gained (i.e., energy generated from methane, subtracted by amount of energy spent) and other costs associated with the processing of OFMSW. Ma et al. (2011) reported the effects of different types of treatments (viz. acid, thermal, freez-thaw etc.) on kitchen leftover and methane generation. The results recommended that nevertheless the sort of substrate, reduced energy utilization provide more financial advantage. In thermal treatment, energy demand relies on the required treatment temperature. It has been reported that microwave heating has advantages over conventional heating due to its ability of the direct core heating (Bordeleau and Droste, 2011). Yang et al. (2010) observed higher carbon and VS removal after thermal treatment of sewage sludge in two stage AD. Authors have also concluded that net energy profit decreased as temperature of thermal treetmement increased.
Nasr et al. (2012) evaluated the balance of energy in two-phase AD of thin stillage and reported that optimization of the two-step AD could result into an increase (~18%) in energy balance. Lu et al. (2008) reported 2.17 kJ/day of energy surplus in a two-step reactor when compared with a single stage framework for treating sewage sludge. In UK (Avonmouth), Wessex water introduced an ultrasound framework for treating the domestic and industrial sludge with a population equivalent of 1,200,000. TS and VS decrease of the untreated waste sludge in the digesters was 40 and 50% as compared to that of sonicated sludge, which was 60 and 70%, respectively. The above-mentioned framework was introduced in numerous plants in the UK, USA, and Australia (Hogan et al., 2004). On the contrary, Mottet et al. (2009) reported that neither microwave nor ultrasound treatment was energy friendly for treating blended waste, as the improved methane yields were insufficient to remunerate the required energy and maintain the energy balance of the system.
Biogas and Challenges
The intricacies of AD process and the process instability that involved in modern technologies are two major constraints affecting AD improvement. The prime goal of research and developments to produce bioenergy around the globe is to develop and establish mature AD technology as source of renewable energy in various sectors (viz. CHP, transportation etc.). Figure 8 describes possible strategies and the importance of relation between industries and academic institutions for developing technology on biomethanation. For cost reduction, it is essential to identify the crucial steps and type (single-phase, two-phase, hybrid) of technology in AD process by taking into account; targeted substrate as well as cost of enzymes employed in the process (IEA Bioenergy Update, 37, 2008).
Rate of biomass conversion and its digestion process mainly affected by microbial community and biocatalyst present in the system. Microorganism and biocatalyst having low adoptability to the AD environment are supposed to be stressed and reduce the total output in a biogas plant. This results in several changes to develop integrated process to convert waste to energy that is economically viable. During enzymatic hydrolysis, different enzyme are required as the OFMSW has a complex composition. Utility requirements viz. heat, electricity, power is also of great concern; and its optimal use in the process is an engineering issue by nature. By-products such as digestate if used or sold as soil amendment to generate revenue may meet the partial utility costs.
Keys to cost effective biomethanation process are; combination of appropriate treatment methodology, presence of robust and dynamic microbial community, quality of substrate and reactor operating conditions. Incorporation of engineering and biology in unison may play a futuristic encouraging role to bring higher yield of methane at competitive price. Other opportunities to overcome the challenges include, developing biocatalysts at low production cost, integration of solar photovoltaics with the biogas plants for energy generation, cost effective treatment and enriched methane for use as bio-CNG. Developing fundamental knowledge on the above mentioned topics may fetch a better process stability for biogas production and utilization.
The very concept of biogas economy driven by biogas inherently depends on feedstock availability, logistics and government policy. Converting industrial waste to biogas and utilizing them for industrial energy will benefit both industry and environment. It is widely accepted that AD technology has robust commercial applicability around the globe. Present energy utilization behavior encompasses dispatched energy sources such as thermal power plants, solar photovoltaics, etc. In dispatched energy source, the required energy can be sent as per requirement at any stipulated time. The present energy supply is in transition period. A major driver for development of bioenergy market is greenhouse gas mitigation. Prior to this, main argument was logistics, storage and biomass supply security. Biomass storage and its improved logistics may address the energy demand renewably. India, on the other hand, initiated various programs related to biomass power. Ministry of new and renewable energy (MNRE), Government of India, administrating a MNRE–UNDP /GFF assisted project on “Removal of Barriers to Biomass Power Generation in India.” Total installed biomass power in different states of India is shown in Figure 9. The primary goal of the project is to increase the use of biomass power and cogenerations based technologies in the country and enhance electricity supply through renewable energy sources. Central finance assistance (CFA) in the form of capital subsidy and financial incentives are also provided by MNRE.
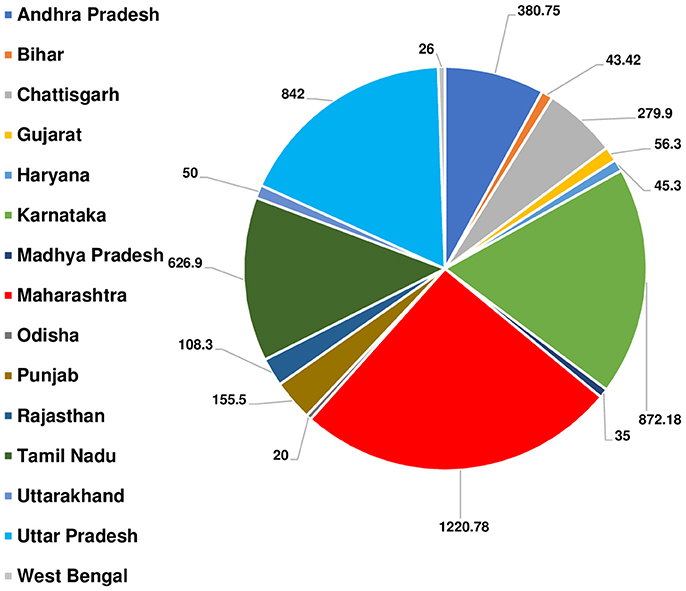
Figure 9. Total installed biomass power (Mega Watt) capacity in India (MNRE, 2016).
Conclusion and Future Aspects
The demand for renewable energy and rise in global warming due to greenhouse gas emission has motivated many government agencies to identify new methods to sustainably produce biogas using AD system. Most treatment techniques have been assessed at batch scale to evaluate the biogas potential from treated OFMSW. However, very limited reports are available that demonstrate biomethanation process at pilot scale using newly developed methods and technologies. Several scientific modifications have to be encountered when the lab scale experiments are translated to large/industrial scale operations. Also, anaerobic biomethanation plants should be operated 24 × 7 in contrast to the one under the controlled lab-scale conditions. Generating energy from OFMSW using anaerobic assimilation research is mainly in two areas, (i) understanding the fundamental of treatment systems and (ii) assessment of the technical and monetary attainability of the joined treatment/anaerobic processing framework. Promising treatment method that is developed in lab scale should be further scaled-up in order to evaluate the overall cost of processing and sustainability. Different treatment procedures viz. physical, thermal, combined, organic or chemical have extensively been attempted at lab scale under defined conditions. Other processes that have been demonstrated at large scale include mechanical, thermal and thermochemical techniques. Anaerobic digestion offers benefits compared to the other disposal procedures for OFMSW considering a fundamental sensibility assessment. Treatment technologies still require advancements at different faces viz. higher biogas yield, efficient management of pathogen, reduction of digestate and reducing the hydraulic retention time as well as the techno-economic viability. The OFMSW offers huge biogas potential and promising opportunity for renewable energy generation, nutrient recovery as well as future research avenues in the area of sustainable waste management and treatment.
Author Contributions
KP, VB, and VV conceived the idea for writing the review and prepared basic content of the manuscript. MY tabulated the data from various references. SM contributed for Environmental Impact Assessment area of treatment. VB contributed for OFMSW information. WL helped in anaerobic digestion aspects. NP drew the figures. VV contributed in treatment ideas. KP and VV clubbed all the information together. All authors worked on text and language correction and reviewed the manuscript.
Funding
VV would like to gratefully acknowledge and thank Department of Biotechnology, Government of India (No. BT/RLF/Re-entry/04/2013) for financially supporting this work. VB thanks University of Houston for supporting his research with start-up funds. WL thanks Michigan State AgBioReserach for supporting his research.
Conflict of Interest Statement
The authors declare that the research was conducted in the absence of any commercial or financial relationships that could be construed as a potential conflict of interest.
Acknowledgments
KP and MY would like to thank MNIT Jaipur for financial support. Authors would like to thank reviewers for their comments and suggestions that has improved the quality of manuscript.
References
Abdullah, Y. A., Akunna, J. C., White, N. A., Hallet, P. D., and Wheatly, R. (2008). Investigating the effects of anaerobic and aerobic posttreatment on quality and stability of organic fraction of municipal solid waste as soil amendment. Bioresour. Technol. 99, 8631–8636. doi: 10.1016/j.biortech.2008.04.027
Abudi, Z. N., Hu, Z., Sun, N., Xiao, B., Rajaa, N., Liu, C., et al. (2016). Batch anaerobic co-digestion of OFMSW (organic fraction of municipal solid waste), TWAS (thickened waste activated sludge) and RS (rice straw): influence of TWAS and RS pretreatment and mixing ratio. Energy 107, 131–140. doi: 10.1016/j.energy.2016.03.141
Agyeman, F. O., and Tao, W. (2014). Anaerobic co-digestion of food waste and dairy manure: effects of food waste particle size and organic loading rate. J. Environ. Manage. 133, 268–274. doi: 10.1016/j.jenvman.2013.12.016
APAT manual (2005). Digestione Anaerobica Della Frazione Organica dei Rifiuti Solidi. Aspetti Fondamentali, Progettuali, Gestionali, di Impatto Ambientale ed Integrazione con la Depurazione Delle Acque Reflue. Rome: Manuale APAT.
Alibardi, L., and Cossu, R. (2015). Composition variability of the organic fraction of municipal solid waste and effects on hydrogen and methane production potentials. Waste Manage. 36, 147–155. doi: 10.1016/j.wasman.2014.11.019
Balsari, P., and Menardo, S. (2011). “Pre-trattamenti dei substrati,” in Biogas da Agrozootecnica e Agroindustria, ed F. Priulla (Palerno: Dario Flaccovio Editore), 183–202.
Barjenbruch, M., and Kopplow, O. (2003). Enzymatic, mechanical and thermal pretreatment of surplus sludge. Adv. Environ. Res. 7, 715–720. doi: 10.1016/S1093-0191(02)00032-1
Bashir, B. H., and Matin, A. (2004). Sodium Toxicity control by the use of magnesium in an anaerobic reactor. J. Appl. Sci. Environ. Mgt. 8, 17–21.
Bernstad, A., Malmquist, L., Truedsson, C., and la Cour Jansen, J. (2013). Need for improvements in physical pretreatment of source-separated household food waste. Waste Manage. 33, 746–754. doi: 10.1016/j.wasman.2012.06.012
Bhakov, Z. K., Korazbekova, K. U., and Lakhanova, K. M. (2014). The kinetics of biogas production from codigestion of cattle manure. Pak. J. Biol. Sci. 17, 1023–1029. doi: 10.3923/pjbs.2014.1023.1029
Bordeleau, É. L., and Droste, R. L. (2011). Comprehensive review and compilation of pretreatments for mesophilic and thermophilic anaerobic digestion. Water Sci. Technol. 63, 291–296. doi: 10.2166/wst.2011.052
Bougrier, C., Albasi, C., Delgenes, J. P., and Carrere, H. (2006). Effect of ultrasonic, thermal and ozone pretreatments on waste activated sludge solubilization and anaerobic biodegradability. Chem. Eng. Process. 45, 711–718. doi: 10.1016/j.cep.2006.02.005
Bougrier, C., Delgenès, J. P., and Carrère, H. (2007). Impacts of thermal pretreatments on the semi-continuous anaerobic digestion of waste activated sludge. Biochem. Eng. J. 34, 20–27. doi: 10.1016/j.bej.2006.11.013
Browne, J. D., and Murphy, J. D. (2013). Assessment of the resource associated with biomethane from food waste. Appl. Energy 104, 170–177. doi: 10.1016/j.apenergy.2012.11.017
Brummeler ten, E., and Koster, I. W. (1990). Enhancement of dry anaerobic batch digestion of the organic fraction of municipal solid waste by anaerobic pretreatment step. Biol. Wastes 31, 199–210.
Cabbai, V., Ballico, M., Aneggi, E., and Goi, D. (2013). BMP tests of source selected OFMSW to evaluate anaerobic codigestion with sewage sludge. Waste Manage. 33, 1626–1632. doi: 10.1016/j.wasman.2013.03.020
Cammarota, M. C., Teixeira, G. A., and Freire, D. M. G. (2001). Enzymatic pre-hydrolysis and anaerobic degradation of wastewaters with high fat content. Biotechnol. Lett. 23, 1591–1595. doi: 10.1023/A:1011973428489
Campuzano, R., and Simón, G. (2015). Extraction of soluble substances from organic solid municipal waste to increase methane production. Bioresour. Technol. 178, 247–253. doi: 10.1016/j.biortech.2014.08.042
Campuzano, R., and Simón, G. (2016). Characteristics of the organic fraction of municipal solid waste and methane production: a review. Waste Manage. 54, 3–12. doi: 10.1016/j.wasman.2016.05.016
Cano, R., Nielfa, A., and Fdz-Polanco, M. (2014). Thermal hydrolysis integration in the anaerobic digestion process of different solid wastes: energy and economic feasibility study. Biores. Technol. 168, 14–22. doi: 10.1016/j.biortech.2014.02.007
Carballa, M., Duran, C., and Hospido, A. (2011). Should we pretreat solid waste prior to anaerobic digestion? an assessment of its environmental cost. Environ. Sci. Technol. 45, 10306–10314. doi: 10.1021/es201866u
Carlsson, M., and Anox Kaldnes, A. D. (2008). “Electroporation for enhanced methane yield from municipal solid waste,” in ORBIT 2008 (Wageningen), 13–15.
Carlsson, M., Lagerkvist, A., and Morgan-Sagastume, F. (2012). The effects of substrate pretreatment on anaerobic digestion: a review. Waste Manage. 32, 1634–1650. doi: 10.1016/j.wasman.2012.04.016
Carrere, H., Dumas, C., Battimelli, A., Batstone, D. J., delgenes, J. P., Steyer, J. P., et al. (2010). Pretreatment methods to improve sludge anaerobic digestion degradability: a review. J. Hazard Mater. 183, 1–15. doi: 10.1016/j.jhazmat.2010.06.129
Castillo, M. E. F., Cristancho, D. E., and Arellano, A. V. (2006). Study of the operational conditions for anaerobic digestion of urban solid wastes. Waste Manage. 26, 546–556. doi: 10.1016/j.wasman.2005.06.003
Cekmecelioglu, D., and Uncu, O. N. (2013). Kinetic modeling of enzymatic hydrolysis of pretreated kitchen wastes for enhancing bioethanol production. Waste Manage. 33, 735–739. doi: 10.1016/j.wasman.2012.08.003
Cesaro, A., and Belgiorno, V. (2013). Sonolysis and ozonation as pretreatment for anaerobic digestion of solid organic waste. Ultrason. Sonochem. 20, 931–936. doi: 10.1016/j.ultsonch.2012.10.017
Cesaro, A., Naddeo, V., Amodio, V., and Belgiorno, V. (2012). Enhanced biogas production from anaerobic codigestion of solid waste by sonolysis. Ultrason. Sonochem. 19, 596–600. doi: 10.1016/j.ultsonch.2011.09.002
Charles, W., Walker, L., and Cord-Ruwisch, R. (2009). Effect of pre-aeration and inoculum on the start up of bathc termophilic anaerobic digestion of municipal solid waste. Bioresour. Technol. 100, 2329–2335. doi: 10.1016/j.biortech.2008.11.051
Chen, L., Li, B., Li, D., Gan, J., and Kitamura, Y. (2008). Ultrasound-assisted hydrolysis and acidogenesis of solid organic wastes in a rotational drum fermentation system. Bioresour. Technol. 99, 8337–8343. doi: 10.1016/j.biortech.2008.02.043
Chen, Y., Chen, J. J., and Creamer, K. S. (2008). Inhibition of anaerobic digestion process: a review. Bioresour. Technol.. 99, 4044–4064. doi: 10.1016/j.biortech.2007.01.057
Chu, L., Yan, S. T., Xing, X. H., Yu, A. F., Sun, X. L., and Jurcik, B. (2008). Enhanced sludge solubilisation by microbubble ozonation. Chemosphere 72, 205–212. doi: 10.1016/j.chemosphere.2008.01.054
Chua, C. P., Lee, D. J., Chang, B., You, C. S., and Tay, J. H. (2002). Weak ultrasonic pretreatment on anaerobic digestion of flocculated activated biosolids. Water Res. 36, 2681–2688. doi: 10.1016/S0043-1354(01)00515-2
Davidson, A., Gruvberger, C., Christensen, T. H., Hansen, T. L., and Jansen, J. C. (2007). Methane yield in source-sorted organic fraction of municipal solid waste. Waste Manage. 27, 406–414. doi: 10.1016/j.wasman.2006.02.013
Dehghani, H. M. (2005). Effectiveness of ultrasound on the destruction of Escherichia coli. Am. J. Environ. Sci. 1, 187–189. doi: 10.3844/ajessp.2005.187.189
Delgenès, J. P., Penaud, V., and Moletta, R. (2003). “Pretreatments for the enhancement of anaerobic digestion of solid waste,” in Biomethanization of the Organic Fraction of Municipal Solid Waste, ed J Mata-Alvarez (Padstow: IWA Publishing), 201–228.
Di Matteo, U., Nastasi, B., Albo, A., and Garcia, A. D. (2017). Energy contribution of OFMSW (Organic Fraction of Municipal Solid Waste) to energy-environmental sustainability in urban areas at small scale. Energies 10:229. doi: 10.3390/en10020229
Dong, L., Zhenhong, Y., and Yongming, S. (2010). Semi-dry mesophilic anaerobic digestion of water sorted organic fraction of municipal solid waste (WS-OFMSW). Bioresour. Technol. 101, 2722–2728. doi: 10.1016/j.biortech.2009.12.007
Edelmann, W., Baier, U., and Engeli, H. (2005). Environmental aspects of the anaerobic digestion of the OFMSW and agricultural wastes. Water. Sci. Technol. 52, 553–559.
El Achkar, J. H., Lendormi, T., Salameh, D., Louka, N., Maroun, R. G., Lanoisellé, J. L., et al. (2018). Influence of pretreatment conditions on lignocellulosic fractions and methane production from grape pomace. Bioresour. Technol. 247, 881–889. doi: 10.1016/j.biortech.2017.09.182
Elbeshbishy, E., and Nakhla, G. (2011). Comparative study of the effect of ultrasonication on the anaerobic biodegradability of food waste in single and two-stage systems. Bioresour. Technol. 102, 6449–6457. doi: 10.1016/j.biortech.2011.03.082
Elbeshbishy, E., Hafez, H., Dhar, B. R., and Nakhla, G. (2011a). Single and combined effect of various pretreatment methods for biohydrogen production from food waste. Int. J. Hydrogen Energy 36, 1379–1387. doi: 10.1016/j.ijhydene.2011.02.067
Elbeshbishy, E., Hafez, H., and Nakhla, G. (2011b). Ultrasonication for biohydrogen production from food waste. Int. J. Hydrogen Energy 36, 2896–2903. doi: 10.1016/j.ijhydene.2010.12.009
Elliot, A., and Mahmood, T. (2012). Comparison of mechanical pretreatment methods for the enhancement of anaerobic digestion of pulp and paper waste. Water Sci. Technol. 84, 497–505. doi: 10.2175/106143012X13347678384602
Elliott, A., and Mahmood, T. (2007). Pretreatment technologies for advancing anaerobic digestion of pulp and paper biotreatment residues. Water Res. 41, 4273–4286. doi: 10.1016/j.watres.2007.06.017
Esposito, G., Frunzo, L., Panico, A., and Pirozzi, F. (2011). Modeling the effect of the OLR and OFMSW particle size on the performances of an anaerobic co-digestion reactor. Process Biochem. 46, 557–565. doi: 10.1016/j.procbio.2010.10.010
Facchin, V., Cavinato, C., Fatone, F., Pavan, P., Cecchi, F., and Bolzonella, D. (2013). Effect of trace elemet supplementation on the mesophilic anaerobic digestion of food waste in batch trials: the influence of inoculum origin. Biochem. Eng. J. 70, 71–77. doi: 10.1016/j.bej.2012.10.004
Fantozzi, F., and Buratti, C. (2011). Anaerobic digestion of mechanically treated OFMSW: experimental data on biogas/methane production and residues characterization. Bioresour. Technol. 102, 8885–8892. doi: 10.1016/j.biortech.2011.06.077
Fdez-Güelfo, A., Álvarez-Gallego, C., Sales-Márquez, D., Romero, L. I., and García (2012). New parameters to determine the optimum pretreatment for improving the biomethanization performances. Chem. Eng. J. 198–199, 81–86. doi: 10.1016/j.cej.2012.05.077
Fdéz-Güelfo, A., Álvarez-Gallego, C., Sales-Márquez, D., and Romero García, L. I. (2011a). Biological pretreatment applied to industrial organic fraction of municipal solid waste (OFMSW): effect on anaerobic digestion. Chem. Eng. J. 172, 321–325. doi: 10.1016/j.cej.2011.06.010
Fdéz-Güelfo, A., Álvarez-Gallego, C., Sales-Márquez, D., and Romero García, L. I. (2011b). The effect of different pretreatments on biomethanation kinetics of industrial Organic Fraction of Municipal Solid Waste (OFMSW). Chem. Eng. J. 171, 411–417. doi: 10.1016/j.cej.2011.03.095
Fernandes, T. V., Klaasse Bos, G. J., Zeeman, G., Sander, J. P. M., and Lier, J. B. (2009). Effects of thermo-chemical pretreatment on anaerobic biodegradability and hydrolysis of lignocellulosic biomass. Bioresour. Technol. 100, 2575–2579. doi: 10.1016/j.biortech.2008.12.012
Ge, H., Jensen, P. D., and Batsone, D. J. (2010). Pretreatment mechanisms during thermophilic mesophilic temperature phased anaerobic digestion of primary sludge. Water Res. 44, 123–130. doi: 10.1016/j.watres.2009.09.005
Ge, H., Jensen, P. D., and Batsone, D. J. (2011a). Increased temperature in thermophilic stage in temperature phased anaerobic digestion (TPAD) improves degradability of waste activated sludge. Hazard Mater. 187, 355–361. doi: 10.1016/j.jhazmat.2011.01.032
Ge, H., Jensen, P. D., and Batsone, D. J. (2011b). Temperature phased anaerobic digestion increases apparent hydrolysis rate for waste activated sludge. Water Res. 45, 1597–1606. doi: 10.1016/j.watres.2010.11.042
Gonella, A. (2011). “Preparation of biological waste and municipal solid waste using the press-extrusion process,” in Proceedings Sardinia, 13th International Waste Management and Landfill Symposium (Cagliari).
Hansen, K., Angelidaki, I., and Ahring, B. (1998). Anaerobic digestion of swine manure: inhibition by ammonia. Water Res. 32, 5–12. doi: 10.1016/S0043-1354(97)00201-7
Hansen, T. L., Jansen, J. C., Davidsson, A., and Christensen, T. H. (2007). Effects of pretreatment technologies on quantity and quality of source-sorted municipal organic waste for biogas recovery. Waste Manage. 27, 389–405. doi: 10.1016/j.wasman.2006.02.014
Hartmann, H., Angelidaki, I., and Ahring, B. K. (2000). Increase of anaerobic degradation of particulate organic matter in full-scale biogas plants by mechanical maceration. Water Sci. Technol. 41, 145–153. doi: 10.2166/wst.2000.0066
Hogan, F., Mormede, S., Clark, P., and Crane, M. (2004). Ultrasound sludge treatment for enhanced anaerobic digestion. Water Sci. Technol. 50, 25–32.
Hu, Z., and Wen, Z. (2008). Enhancing enzymatic digestability of switchgrass by microwave-assisted alkali treatment. Biochem. Eng. J. 38, 369–378. doi: 10.1016/j.bej0.2007.08.001
International Panel on Climate Change (IPCC) Guidelines (2006). Waste Composition, Generation and Management Data.
Izumi, K., Okishio, Y., Nagao, N., Niwa, C., Yamamoto, S., and Toda, T. (2010). Effects of particle size on anaerobic digestion of food waste. Int. Biodeterior. Biodegr. 64, 601–608. doi: 10.1016/j.ibiod.2010.06.013
Keller, F. A., Hamilton, J., and Nguyen, Q. A. (2003). Microbial pretreatment of biomass. Appl. Biotechnol. Bioeng. 105, 27–41. doi: 10.1007/978-1-4612-0057-4_3
Kim, I. S., Kim, D. H., and Hyun, S. H. (2000). Effect of particle size and sodium ion concentration on anaerobic thermophilic food waste digestion. Water Sci. Technol. 41, 67–73. doi: 10.2166/wst.2000.0057
Komemoto, K., Lim, Y. G., Nagao, N., Onoue, Y., Niwa, C., and Toda, T. (2009). Effect of temperature on VFA's and biogas production in anaerobic solubilisation of food waste. Waste Manage. 29, 2950–2955. doi: 10.1016/j.wasman.2009.07.011
Kugelman, I. J., and McCarty, P. L. (1965). Cation toxicity and stimulation in anaerobic waste treatment. J. Water Pollut. Control. Fed. 37, 97–116.
Kumar, D., and Murthy, G. S. (2011). Impact of pretreatment and downstream processing technologies on economics and energy in cellulosic ethanol production. Biotechnol. Biofuels 4:27. doi: 10.1186/1754-6834-4-27
Kumar, R., and Wyman, C. E. (2010). “Key features of pretreated lignocellulosic biomass solids and their impact on hydrolysis,” in Bioalcohol Production. Biochemical Conversion of Lignocellulosic Biomass (Cambridge: Woodhead Publishing), 73–121.
Kumar, S., Paritosh, K., Pareek, N., Chawade, A., and Vivekanand, V. (2018). De-construction of major Indian cereal crop residues through chemical pretreatment for improved biogas production: an overview. Renew. Sustain. Energy Rev. 90, 160–170 doi: 10.1016/j.rser.2018.03.049
Kuo, W. C., and Cheng, K. Y. (2007). Use of respirometer in evaluation of process and toxicity of termophilic anaerobic digestion for treating kitchen waste. Bioresour. Technol. 98, 1805–1811. doi: 10.1016/j.biortech.2006.06.016
Kwiatowska, B., Bennett, J., Akunna, J., Walker, G. M., and Bremner, D. H. (2011). Stimulation of bioprocess by ultrasound. Biotechnol. Adv. 29, 768–780. doi: 10.1016/j.biotechadv.2011.06.005
Leal, M. C. M. R., Cammarota, M. C., Freire, D. M. G., and Sant'Anna, Jr. G. L. (2002). Hydrolytic enzymes as coadjuvants in the anaerobic treatment of dairy wastewaters. Braz. J. Chem. Eng. 19, 175–180. doi: 10.1590/S0104-66322002000200013
Lee, J. S., and Chang, I. S. (2014). Membrane fouling control and sludge solubilization using high voltage impulse (HVI) electric fields. Process Biochem. 49, 858–862. doi: 10.1016/j.procbio.2014.03.001
Lee, M., Hidaka, T., Hagiwara, W., and Tsuno, H. (2009). Comparative performance and microbial diversity of hyper-thermophilic and thermophilic co-digestion of kitchen garbage and excess sludge. Bioresour. Technol. 100, 578–585. doi: 10.1016/j.biortech.2008.06.063
Li, H., Li, C., Liu, W., and Zou, S. (2012). Optimized alkaline pretreatment of sludge before anaerobic digestion. Bioresour. Technol. 123, 189–194. doi: 10.1016/j.biortech.2012.08.017
Li, Y. Y., and Noike, T. (1992). Upgrading of anaerobic digestion of waste activated sludge by thermal pretreatment. Water Sci. Technol. 26, 857–866.
Lim, J. W., and Wang, J. Y. (2013). Enhanced hydrolysis and methane yield by applying microaeration pretreatment to the anaerobic co-digestion of brown water and food waste. Waste Manage. 33, 813–819. doi: 10.1016/j.wasman.2012.11.013
Liu, X., Wang, W., Gao, X., Zhou, Y., and Shen, R. (2012). Effect of thermal pretreatment on the physical and chemical properties of municipal biomass waste. Waste Manage. 32, 249–255. doi: 10.1016/j.wasman.2011.09.027
López Torres, M., and Espinosa Lloréns Mdel, C. (2008). Effect of alkaline pretreatment on anaerobic digestion of solid wastes. Waste Manage. 28, 2229–2234. doi: 10.1016/j.wasman.2007.10.006
Lu, J., Gavala, H. N., Skiadas, I. V., Mladenovska, Z., and Ahrin, B. K. (2008). Improving anaerobic sewage sludge digestion by implementation of a hyper-thermophilic prehydrolysis step. J. Environ. Manag. 88, 881–889. doi: 10.1016/j.jenvman.2007.04.020
Luning, L., van Zundert, E. H., and Brinkmann, A. J. (2003). Comparison on dry and wet digestion for solid waste. Water Sci. Technol. 48, 15–20.
Luste, S., Luostarinen, S., and Sillanpää, M. (2009). Effect of pretreatments on hydrolysis and methan epotentials of by-products from meat-processing industry. J. Hazard. Mater. 164, 247–255. doi: 10.1016/j.jhazmat.2008.08.002
Ma, J., Duong, T. H., Smits, M., Vestraete, W., and Carballa, M. (2011). Enhanced biomethanation of kitchen waste by different pretreatments. Bioresour. Technol.. 102, 592–599. doi: 10.1016/j.biortech.2010.07.122
Mallick, P., Akunna, J. C., and Walker, G. M. (2010). Anaerobic digestion of distillery spent wash: influence of enzymatic pretreatment of intact yeast cells. Bioresour. Technol. 101, 1681–1685. doi: 10.1016/j.biortech.2009.09.089
Marin, J., Kennedy, K. J., and Eskicioglu, C. (2010). Effect of microwave irradiation on anaerobic degradability of model kitchen waste. Waste Manag. 30, 1772–1779. doi: 10.1016/j.wasman.2010.01.033
Mason, T. J., and Peters, D. (2002). Practical Sonochemistry: Power Ultrasound and Applications, 2nd edn. Chichester: Woodhead Publishing.
Masse, L., Masse, D. I., and Kennedy, K. J. (2003). Effect of enzymatic pretreatment on fat degradation during anaerobic digestion of slaughterhouse wastewater. Process Biochem. 38, 1365–1372. doi: 10.1016/s0032-9592(03)00020-7
Mata-Alvarez, J. (2003). Biomethanation of the Organic Fraction of Municipal Solid Wastes. ISBN: 1 900222 14 0.
Mata-Alvarez, J., Dosta, J., Romero-Güiza, M. S., Fonoll, X., Peces, M., and Astals, S. (2014). A critical review on anaerobic co-digestion achievements between 2010 and 2013. Renew. Sustain. Energy Rev. 36, 412–427. doi: 10.1016/j.rser.2014.04.039
Mata-Alvarez, J., Mace, S., and Llabres, P. (2000). Anaerobic digestion of organic solid wastes. an overview of research achievements and perspectives. Bioresour. Technol. 74, 3–16. doi: 10.1016/S0960-8524(00)00023-7
Mendes, A. A., Pereira, E. B., and de Castro, H. F. (2006). Effect of enzymatic hydrolysis pretreatment of lipids-rich wastewater on the anaerobic digestion. Biochem. Eng. J. 32, 185–190. doi: 10.1016/j.bej.2006.09.021
Miah, M. S., Tada, C., and Yang, Y. (2005). Aerobic thermophilic bacteria enhance biogas production. J. Mater. Cycl. Waste Manag. 7, 48–54. doi: 10.1007/s10163-004-0125-y
Milán, Z., Sánchez, E., Weiland, P., Borja, R., Martín, A., and Ilangovan, K. (2001). Influence of different natural zeolite concentrations on the anaerobic digestion of piggery waste. Bioresour. Technol. 80, 37–43. doi: 10.1016/S0960-8524(01)00064-5
Modenbach, A. A., and Nokes, S. E. (2012). The use of high-solids loading in biomass pretreatment – a review. Biotechnol. Bioeng. 109, 1430–1442. doi: 10.1002/bit.24464
Montalvo, S., Guerrero, L., Borja, R., Travieso, L., Sánchez, E., and Díaz, D. (2006). Use of natural zeolite at different doses and dosage procedures in batch and continuous anaerobic digestion of synthetic and swine wastes. Resour. Conserv. Recycle 47, 26–41. doi: 10.1016/j.resconrec.2005.10.001
Mottet, A., Steyera, J. P., Délérisb, S., Vedrenneb, F., Chauzyc, J., and Carrère, H. (2009). Kinetics of thermophilic batch anaerobic digestion of thermal hydrolysed waste activated sludge. Biochem. Eng. J. 46, 169–175. doi: 10.1016/j.bej.2009.05.003
Mshandete, A., Bjornsson, L., Kivaisi, A. K., Rubindamayugi, M. S. T., and Mattiasson, B. (2006). Effect of particle size on biogas yield from sisal fiber waste. Renew. Energy 31, 2385–2392. doi: 10.1016/j.renene.2005.10.015
Mshandete, A., Bjornsson, L., Kivaisi, A. K., Rubindamayugi, S. T., and Mattiasson, B. (2005). Enhancement of anaerobic batch digestion of sisal pulp waste by mesophilic aerobic pretreatment. Water Res. 39, 1569–1575. doi: 10.1016/j.watres.2004.11.037
Müller, J. A. (2001). Prospects and problems of sludge pretreatment processes. Water Sci. Technol. 44, 121–128. doi: 10.2166/wst.2001.0598
Mussoline, W., Esposito, G., Giordano, A., and Lens, P. (2012). Anaerobic digestion of rice straw: a review. Crit. Rev. Environ. Sci. Technol. 43, 895–915. doi: 10.1080/10643389.2011.627018
Naran, E., Toor, U. A., and Kim, D.-J. (2016). Effect of pretreatment and anaerobic co-digestion of food waste and waste activated sludge on stabilization and methane production. Int. Biod. Biodet. 113, 17–21. doi: 10.1016/j.ibiod.2016.04.011
Nasr, N., Elbeshbishy, E., Hafez, H., Nakhla, G., and El Naggar, M. H. (2012). Comparative assessment of single-stage and two-stage anaerobic digestion for the treatment of thin stillage. Biores. Technol. 111, 122–126. doi: 10.1016/j.biortech.2012.02.019
Nayono, S. E., Winter, J., and Gallert, C. (2010). Anaerobic digestion of pressed off leachate from the organic fraction of municipal solid waste. Waste Manag. 30, 1828–1833. doi: 10.1016/j.wasman.2009.09.019
Neves, L., Ribeiro, R., Oliveira, R., and ALves, M. M. (2006). Enhancement of methane production from barley waste. Biomass Bioenergy 30, 599–603. doi: 10.1016/j.biombioe.2005.12.003
Neyens, E., and Baeyens, J. (2003). A review of thermal sludge pretreatment processes to improve dewaterability. Hazard. Mater. 98, 51–67. doi: 10.1016/S0304-3894(02)00320-5
Nwaneshiudu, I. C., Ganguly, I., Pierobon, F., Bowers, T., and Eastin, I. (2016). Environmental assessment of mild bisulfite pretreatment of forest residues into fermentable sugars for biofuel production. Biotech. Biofuels 9:15. doi: 10.1186/s13068-016-0433-1
Parawira, W., Murto, M., Read, J. S., and Mattiasson, B. (2005). Profile of hydrolases and biogas production during two-stage mesophilic anaerobic digestion of solid potato waste. Process Biochem. 40, 2945–2952. doi: 10.1016/j.procbio.2005.01.010
Paritosh, K., Kushwaha, S. K., Yadav, M., Pareek, N., Chawade, A., and Vivekanand, V. (2017a). Food waste to energy: an overview of sustainable approaches for food waste management and nutrient recycling. BioMed. Res. Int. 2017:2370927. doi: 10.1155/2017/2370927
Paritosh, K., Mathur, S., Pareek, N., and Vivekanand, V. (2017b). Feasibility study of waste (d) potential: co-digestion of organic wastes, synergistic effect and kinetics of biogas production. Int. J. Environ. Sci. Technol. 15, 1009–1018. doi: 10.1007/s13762-017-1453-5
Parthiba Karthikeyan, O., Trably, E., Mehariya, S., Bernet, N., Wong, J. W. C., and Carrere, H. (2017). Pretreatment of food waste for methane and hydrogen recovery: a review. Bioresour. Technol. 249, 1025–1039. doi: 10.1016/j.biortech.2017.09.105
Pavlostathis, S. G., and Gosset, J. M. (1985). Alkaline treatment of wheat straw for increasing anaerobic digestion. Biotechnol. Bioeng. 27, 334–344. doi: 10.1002/bit.260270319
Pecorini, I., Baldi, F., Carnevale, E. A., and Corti, A. (2016). Biochemical methane potential tests of different autoclaved and microwaved lignocellulosic organic fractions of municipal solid waste. Waste Manag. 56, 143–150 doi: 10.1016/j.wasman.2016.07.006
Perez-Elvira, S. I., Nieto Diez, P., and Fdz-Polanco, F. (2006). Sludge minimization technologies. Rev. Environ. Sci. Bio/Technol. 5, 375–398. doi: 10.1007/s11157-005-5728-9
Pognani, M., D'Imporzano, G., Scaglia, B., and Adani, F. (2009). Substituting energy crops with organic fraction of municipal solid waste for biogas production at farm level: a full-scale plant study. Process Biochem. 44, 817–821. doi: 10.1016/j.procbio.2009.03.014
Protot, A., Julien, L., Christophe, D., and Partick, L. (2011). Sludge disintegration during heat treatment at low temperature: a better understanding of involved mechanisms with a multi-parametric approach. Biochem. Eng. J. 54, 178–184. doi: 10.1016/j.bej.2011.02.016
Qiao, W., Yan, X., Ye, J., Sun, Y., Wang, W., and Zhang, Z. (2011). Evaluation of biogas production from different biomass wastes with/without hydrothermal pretreatment. Renew. Energy 36, 3313–3318. doi: 10.1016/j.renene.2011.05.002
Rafique, R., Poulse, T. G., Nizami, A. S., Asam, Z. Z., Murphy, J. D., and Kiely, G. (2010). Effect of thermal, chemical and thermo-chemical pretreatments to enhance methane production. Energy 35, 4556–4561. doi: 10.1016/j.energy.2010.07.011
Riau, V., Rubia, M. A., and Perez, M. (2010). Temperature-phased anaerobic digestion (TPAD) to obtain Class A biosolids. A discontinuous study. Biores. Technol. 101, 65–70. doi: 10.1016/j.biortech.2009.07.072
Rodriguez, C., Alaswad, A., El-hassan, Z., and Olabi, A. G. (2017). Mechanical pretreatment of waste paper for biogas production. Wast. Manag. 68, 157–164. doi: 10.1016/j.wasman.2017.06.040
Schmidt, J. E., and Ahring, B. K. (1993). Effects of magnesium on thermophilic acetate degrading granules in upflow anaerobic sludge blanket (UASB) reactors. Enzyme Microb. Technol. 15, 304–310. doi: 10.1016/0141-0229(93)90155-U
Schmit, K. H., and Ellis, T. G. (2001). Comparison of temperature phased and other stage of the art process for anaerobic digestion of municipal solid waste. Water Environ. Res. 73, 314–321. doi: 10.2175/106143001X139335
Shahriari, H., Warith, M., Hamoda, M., and Kennedy, K. J. (2012). Anaerobic digestion of organic fraction of municipal solid waste combining two pretreatment modalities, high temperature microwave and hydrogen peroxide. Waste Manag. 32, 41–52. doi: 10.1016/j.wasman.2011.08.012
Shanthi, M., Rajesh Banu, J., and Sivashanmugam, P. (2018). Effect of Surfactant assisted sonic pretreatment on liquefaction of fruits and vegetable residue: characterization, acidogenesis biomethane yield and energy ratio. Biores. Technol. 264, 35–41. doi: 10.1016/j.biortech.2018.05.054
Shepherd, T. A. (2006). Pretreatment Technologies for Increasing Biogas Potential of Agricultural Wastes. Available online at: http://home.eng.iastate.edu/~tge/ce421-521/tshep.pdf
Shi, J., Sharma-Shivappa, R. R., Chinn, M., and Howell, N. (2009). Effect of microbial pretreatment on enzymatic hydrolysis and fermentation of cotton stalks for ethanol production. Biomass Bioenergy 33, 88–96. doi: 10.1016/j.biombioe.2008.04.016
Sonesson, U., Bjorklund, A., Carlsson, M., and Dalemo, M. (2000). Environmental and economic analysis of management systems for biodegradable waste. Res. Conserv. Recycle 28, 29–53. doi: 10.1016/S0921-3449(99)00029-4
Soto, M., Mendez, R., and Kema, J. M. (1993). Sodium inhibition and sulphate reduction in the anaerobic treatment of mussel processing wastewaters. J. Chem. Technol. Biotechnol. 58, 1–7. doi: 10.1002/jctb.280580102
Stabnikova, O., Liu, X. Y., and Wang, J. Y. (2008). Digestion of frozen/thawed food waste in the hybrid anaerobic solid-liquid system. Waste Manag. 28, 1654–1659. doi: 10.1016/j.wasman.2007.05.021
Subramani, T., and Ponkumar, S. (2012). Anaerobic digestion of aerobic pretreated organic waste. Int. J. Modern Eng. Res. 2, 607–611.
Sung, S., and Harikashan, S. (2001). Performance of temperature-phased anaerobic digestion (TPAD) system treating dairy cattle wastes. Tamkang. J. Sci. Eng. 4, 301–310. doi: 10.1016/S0043-1354(02)00498-0
Tada, C., Yang, Y., Hanaoka, T., Sonoda, A., Ooi, K., and Sawayama, S. (2005). Effect of natural zeolite on methane production for anaerobic digestion of ammonium rich organic sludge. Bioresour. Technol. 96, 459–464. doi: 10.1016/j.biortech.2004.05.025
Thorin, E., Lindmark, J., Nordlander, E., Odlare, M., Dahlquist, E., Kastensson, J., et al. (2012). Performance optimization of the Vaxtkraft biogas production plant. Appl. Energy 97, 503–508. doi: 10.1016/j.apenergy.2012.03.007
Tomas-Pejo, E., Alvira, P., Ballesteros, M., and Negro, M. J. (2011). “Pretreatment technologies for lignocellulose-to-bioethanol conversion,” in Biofuel: Alternative Feedstocks and Conversion Processes, eds S. C. Ricke, C.-G. Dussap, A. Pandey, C. Larroche, and E. Gnansounou (Amsterdam: Elsevier Inc.), 149–176.
Tong, S. L., and Jaafar, A. B. (2006). POME biogas capture, upgrading and utilization. Palm Oil Eng. Bull. 78, 11–17.
Toreci, I., Kennedy, K. J., and Droste, R. L. (2009). Evaluation of continuous mesophilic anaerobic sludge digestion after high temperature microwave pretreatment. Water Res. 43, 1273–1284. doi: 10.1016/j.watres.2008.12.022
Tyagi, V. K., Fdez-Güelfoc, L. A., Zhoud, Y., Álvarez-Gallegof, C. J., Romero Garciaf, L. I., and Ng, W. J. (2018). Anaerobic co-digestion of organic fraction of municipal solid waste (OFMSW): progress and challenges. Renew. Sustain. Energy Rev. 93 380–399. doi: 10.1016/j.rser.2018.05.051
van Lier, J. B., Tilche, A., Ahring, B. H., Macarie, H., Moletta, R., Dohanyos, M., et al. (2001). New perspectives in anaerobic digestion. Water Sci. Technol. 43, 1–18.
Val del Rio, A., Morales, N., Isanta, E., Mosquera-Corral, A., Campos, J. L., Steyer, J. P., et al. (2011). Thermal pretreatment of aerobic granular sludge: impact on anaerobic biodegradability. Water Res. 45, 6011–6020. doi: 10.1016/j.watres.2011.08.050
Valladão, A., Freire, D., and Cammarota, M. (2007). Enzymatic pre-hydrolysis to the anaerobic treatment of effluents from poultry slaughterhouses. Int. Biodeterior. Biodegr. 60, 219–225. doi: 10.1016/j.ibiod.2007.03.005
Verrier, D., Roy, F., and Albagnac, G. (1987). Two-phase methanization of solid vegetable wastes. Biol. Waste 22, 163–177. doi: 10.1016/0269-7483(87)90022-X
Wang, F., Hidaka, T., Tsuno, H., and Tsubota, J. (2011). Co-digestion of polylactide and kitchen garbage in hyper-thermophilic and thermophilic continuous anaerobic process. Bioresour. Technol. 112, 67–74. doi: 10.1016/j.biortech.2012.02.064
Wang, L., Mattsson, M., Rundstedt, J., and Karlsson, N. (2011). Different pretreatments to enhance biogas production. Master of Science Thesis, Halmstad University.
Ward, A. J., Hobbs, P. J., Holliman, P. J., and Jones, D. L. (2008). Optimisation of the anaerobic digestion of agricultural resources. Bioresour. Technol. 99, 7928–7940. doi: 10.1016/j.biortech.2008.02.044
Yadvika, Santosh, Sreekrishnan, T. R., Kohli, S., and Rana, V. (2004). Enhancement of biogas production from solid substrates using different techniques – a review. Biores. Technol. 95, 1–10. doi: 10.1016/j.biortech.2004.02.010
Yang, X., Wang, X., and Wang, L. (2010). Transferring of components and energy putput in industrial sewage sludge disposal by thermal pretreatment and two-phase anaerobic process. Biores. Technol. 101, 2580–2584. doi: 10.1016/j.biortech.2009.10.055
Yin, Y., Liu, Y.-J., Meng, S.-J., Kiran, E. U., and Liu, Y. (2016). Enzymatic pretreatment of activated sludge, food waste and their mixture for enhanced bioenergy recovery and waste volume reduction via anaerobic digestion. Appl. Energy 179, 1131–1137. doi: 10.1016/j.apenergy.2016.07.083
Yu, G., He, P., Shao, L., and Zhu, Y. (2009). Enzyme extraction by ultrasound from sludge flocs. J. Environ. Sci. 21, 204–210. doi: 10.1016/S1001-0742(08)62252-4
Zhang, B., Zhang, L. L., Zhang, S. C., Shi, H. Z., and Cai, W. M. (2005). The influence of pH on hydrolysis and acidnogenesis of kitchen wastes in two-phase anaerobic digestion. Environ. Technol. 26, 329–339. doi: 10.1080/09593332608618563
Zhang, J., Li, W., Lee, J., Loh, K. C., Dai, Y., and Tong, Y. W. (2017). Enhancement of biogas production in anaerobic co-digestion of food waste and waste activated sludge by biological co-pretreatment. Energy 137, 479–486. doi: 10.1016/j.energy.2017.02.163
Zhang, L., and Jahng, D. (2012). Long term anaerobic digestion of food waste stabilized by trace elements. Waste Manag. 32, 1509–1515. doi: 10.1016/j.wasman.2012.03.015
Zhang, Y., and Banks, C. J. (2013). Impact of different particle size distributions on anaerobic digestion of the organic fraction of municipal solid waste. Waste Manag. 33, 297–307. doi: 10.1016/j.wasman.2012.09.024
Zhu, B., Gikas, P., Zhang, R., Lord, J., Jenkins, B., and Li, X. (2009). Characteristics and biogas production potential of municipal solid wastes pretreated with rotary drum reactor. Bioresour. Technol. 100, 1122–1129. doi: 10.1016/j.biortech.2008.08.024
Zhu, J. Y., and Pan, X. J. (2010). Woody biomass pretreatment for cellulosic ethanol production: technology and energy consumption evaluation. Bioresour. Technol. 101, 4992–5002. doi: 10.1016/j.biortech.2009.11.007
Keywords: organic waste, biomass treatment, biogas, anaerobic digestion, environmental impact assessment
Citation: Paritosh K, Yadav M, Mathur S, Balan V, Liao W, Pareek N and Vivekanand V (2018) Organic Fraction of Municipal Solid Waste: Overview of Treatment Methodologies to Enhance Anaerobic Biodegradability. Front. Energy Res. 6:75. doi: 10.3389/fenrg.2018.00075
Received: 11 April 2018; Accepted: 12 July 2018;
Published: 03 August 2018.
Edited by:
J. Richard Hess, Idaho National Laboratory (DOE), United StatesReviewed by:
Abu Yousuf, Shahjalal University of Science and Technology, BangladeshMuhammad Aziz, Tokyo Institute of Technology, Japan
Copyright © 2018 Paritosh, Yadav, Mathur, Balan, Liao, Pareek and Vivekanand. This is an open-access article distributed under the terms of the Creative Commons Attribution License (CC BY). The use, distribution or reproduction in other forums is permitted, provided the original author(s) and the copyright owner(s) are credited and that the original publication in this journal is cited, in accordance with accepted academic practice. No use, distribution or reproduction is permitted which does not comply with these terms.
*Correspondence: Vivekanand Vivekanand, vivekanand.cee@mnit.ac.in