- 1Endocrinology and Diabetes Department, Medical Hospital I, Bergmannsheil University Hospitals, Ruhr University of Bochum, Bochum, Germany
- 2Ruhr Center for Rare Diseases (CeSER), Ruhr University of Bochum and Witten/Herdecke University, Bochum, Germany
- 3Private Consultancy, Research and Development, Yandina, QLD, Australia
- 4North Lakes Clinical, Ilkley, United Kingdom
- 5Department of Psychiatry and Psychotherapy, LVR-Klinikum Düsseldorf, Düsseldorf, Germany
- 6Department of Cardiology II, Münster University Hospitals, University of Münster, Münster, Germany
Elevated concentrations of free thyroid hormones are established cardiovascular risk factors, but the association of thyrotropin (TSH) levels to hard endpoints is less clear. This may, at least in part, ensue from the fact that TSH secretion depends not only on the supply with thyroid hormones but on multiple confounders including genetic traits, medication and allostatic load. Especially psychosocial stress is a still underappreciated factor that is able to adjust the set point of thyroid function. In order to improve our understanding of thyroid allostasis, we undertook a systematic meta-analysis of published studies on thyroid function in post-traumatic stress disorder (PTSD). Studies were identified via MEDLINE/PubMed search and available references, and eligible were reports that included TSH or free thyroid hormone measurements in subjects with and without PTSD. Additionally, we re-analyzed data from the NHANES 2007/2008 cohort for a potential correlation of allostatic load and thyroid homeostasis. The available evidence from 13 included studies and 3386 euthyroid subjects supports a strong association of both PTSD and allostatic load to markers of thyroid function. Therefore, psychosocial stress may contribute to cardiovascular risk via an increased set point of thyroid homeostasis, so that TSH concentrations may be increased for reasons other than subclinical hypothyroidism. This provides a strong perspective for a previously understudied psychoendocrine axis, and future studies should address this connection by incorporating indices of allostatic load, peripheral thyroid hormones and calculated parameters of thyroid homeostasis.
Introduction
The prognostic and therapeutic implications of subclinical hypothyroidism remain to be debated (1–4). At least in elderly subjects, the benefits of levothyroxine substitution are questionable (5). While increased and high-normal free thyroxine (FT4) concentration is a well-established risk factor for malignant arrhythmia and sudden cardiac death (6, 7), the association between thyrotropin (TSH) concentration and cardiovascular mortality is less well understood (8–11). Studies reported either no relation at all (12, 13) or a rather complex U-shaped association (14, 15), as has been shown in a recent population study based on the large NHANES datasets (16). Likewise, a recent study observed elevated FT4 concentration in stress cardiomyopathy or Takotsubo syndrome (17). In the same cohort, the distribution of TSH levels was complex and ambiguous, however.
The conclusion by Inoue et al. that subclinical hypothyroidism is a risk factor for cardiovascular death (16) may be premature. High-normal or elevated TSH concentrations do not unequivocally indicate early thyroid failure but may also be reflective of an increased homeostatic set point of the hypothalamus-pituitary-thyroid axis (18). Apart from genetic traits manifestation of a high set point may ensue from chronic psychosocial stress. This is termed type 2 allostatic load and a well-recognized cardiovascular risk indicator (19–24). Set point alterations have been linked to acute and chronic stress situations including psychosis, alcohol withdrawal and post-traumatic stress disorder (PTSD), all of which are known risk factors for cardiovascular mortality (25–30). Here, we present a brief evaluation of the stress-thyroid axis and discuss an alternative explanation.
Methods
Based on previous reports on a possible association of PTSD to thyroid function (19, 31–36) we performed a systematic meta-analysis summarizing the available evidence. Up to July 4th, 2020, a systematic search was executed of PubMed/MEDLINE by using the following search formula: “post-traumatic stress disorder AND (thyroid OR triiodothyronine OR thyrotropin OR TSH OR thyroxine)”. Additionally, we screened the references of the retrieved publications for additional suitable publications. Studies were eligible if they compared TSH, FT4 or free T3 (FT3) concentration in subjects with and without PTSD, and if the definition of the disease was compatible with the criteria provided by the fifth edition of the Diagnostic and Statistical Manual of Mental Disorders (DSM-5). Summary measures were included in random effects meta-analysis. Between-study variance was assessed with the DerSimonian-Laird estimator, Cochran’s Q and tau squared, and heterogeneity with Higgins’ and Thompson’s I squared. Small study effects were estimated with funnel plots and three tests statistics (Egger, Begg and Mazumdar, and Thompson and Sharp) with the null hypothesis of no bias in the meta-analysis. The quality of the studies was assessed with a Newcastle-Ottawa score. Calculations were supported by the packages meta and metaphor (37, 38) for the statistical environment R (39).
Additionally, we analyzed publicly available data from the NHANES 2007/2008 dataset (40) in order to re-assess the association between thyroid function and type 2 allostasis. A summative quantitative allostatic load score (SIQALS 2) was derived from the sum of sub-scores for pulse rate, systolic and diastolic blood pressure, total cholesterol, high density lipoprotein (HDL), body mass index (BMI), HbA1c and c-reactive protein (CRP) concentration according to the procedure of the Scottish Health Survey (20). Each sub-score was graded as 1 if the results were in the upper quartile of the respective population distribution or 0 if they were in any of the lower quartiles, except for HDL, which was scaled in the opposite direction (20). Hence, the possible range for SIQALS is between 0 and 8, where a larger value indicates a higher amount of allostatic load. Analysis was restricted to euthyroid subjects (as defined by TSH and FT4 concentrations within their respective laboratory-defined reference ranges) without known thyroid disease, pregnancy or any major comorbidity potentially leading to non-thyroidal illness syndrome. We relied on Jostel’s TSH index (JTI) as a marker for the location of the pituitary-thyroid set point (41). Additionally, estimates for thyroid’s secretory capacity (SPINA-GT) and total deiodinase activity (SPINA-GD) were calculated in order to provide further insights into the physiological roots of potential variations in hormone levels (41).
We tested for associations between allostatic load and markers of thyroid function with unadjusted ordinary least squares (OLS) regression. In these univariate analyses, the multiple components contributing to type 2 allostatic load were expressed as an equally weighted summary score (SIQALS 2) in order to avoid potential issues with collinearity in multivariable analysis. To statistically account for direction of causality and derive more robust estimators in the possible presence of endogenous regressors and feedback (reversed causality), we relied on instrumental variables estimation (IV regression) (42), using the R package AER (43). We selected parameters from the somatic, psychological and social domain as candidates for instrumental variables. Eligible as instruments were parameters that correlated with both SIQALS 2 and JTI, and whose influence could be assumed to be mediated via allostatic load only (Supplementary Tables 3 and 4). The Durbin-Wu-Hausmann test was used to detect potential endogeneity and to decide if OLS and IV regressions are equally consistent or if IV are to be preferred over OLS models. The Sargan test was performed to test for over-identification, when multiple IVs were used. Additionally, a test for “weak instruments” was performed to decide about the validity of IVs.
Results
We identified 16 studies investigating TSH and/or thyroid hormone concentrations in subjects with and without PTSD. Two older studies were excluded, since they reported a time interval between trauma and evaluation of thyroid function of a month or less, rendering them incompatible with current definitions of PTSD, and one study was excluded, because only total thyroid hormones but neither TSH nor free thyroid hormones were determined. The remaining 13 studies could be included in the meta-analysis (Supplementary Figure 1) (25–27, 44–53).
With respect to TSH and FT3 concentrations the studies were highly heterogeneous, but the findings were unanimous for FT4 concentrations. Combining the results yields a positive association of PTSD with TSH levels in the random effects model, but unchanged FT4 concentrations (Figure 1). FT3 concentration tended to be higher in PTSD.
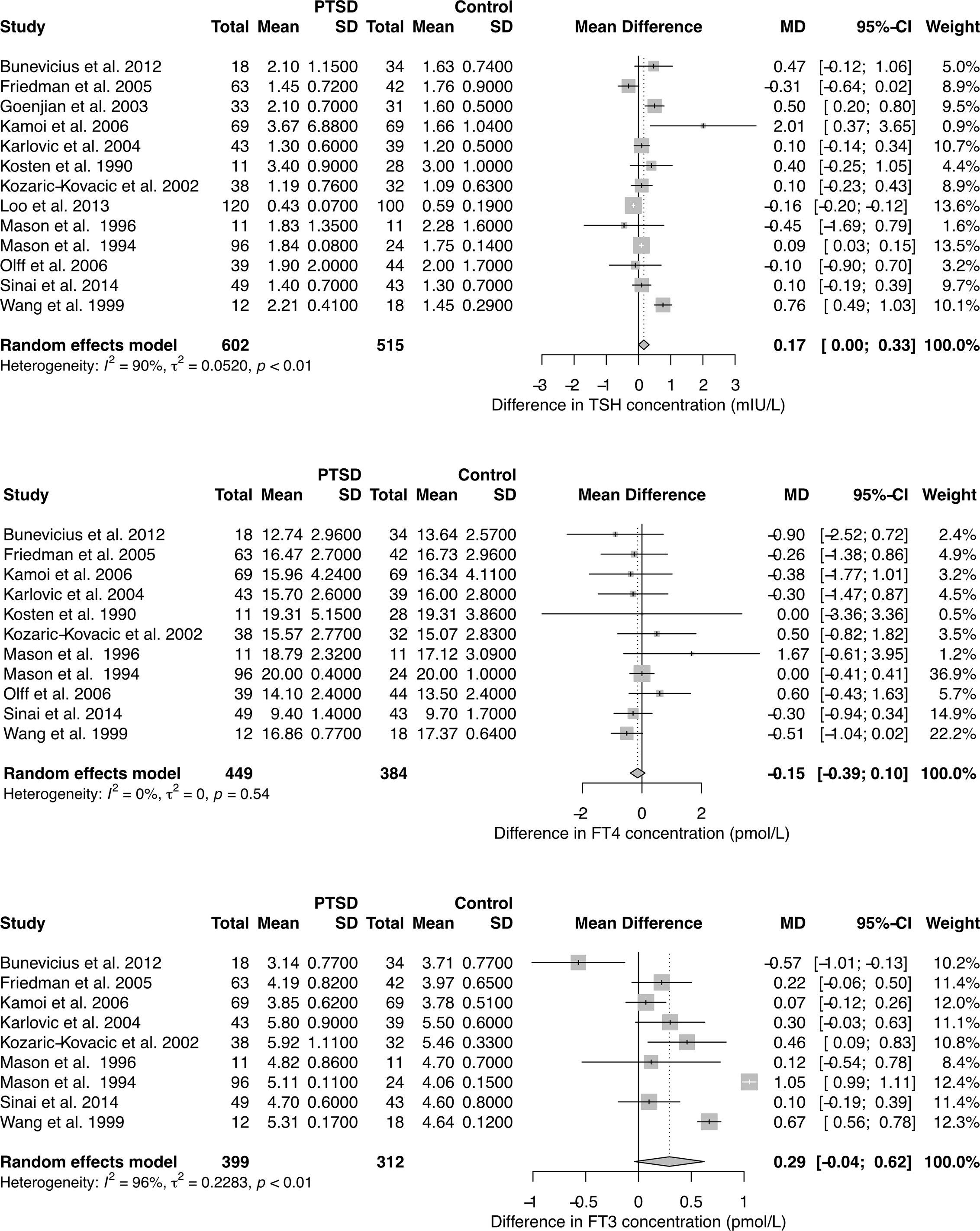
Figure 1 Forest plots showing differences in TSH, FT4, and FT3 concentration between subjects with and without PTSD. See Supplementary Figures 3–5 for results in chronic PTSD and for total T3 (TT3) and total T4 (TT4) concentration.
Meta-regression between concentration differences and time of the exposure to the triggering event revealed a significant correlation for TSH levels (Supplementary Figure 2). Accordingly, the observed pooled study effects were even more pronounced in a subset of results with chronic PTSD (24 months or more since the occurrence of the triggering event, Supplementary Figure 3). Total T3 concentrations were significantly higher in PTSD compared to controls (Supplementary Figures 4 and 5).
Funnel plots (Supplementary Figure 6) and tests for small study effects demonstrated that publication bias resulting from less frequent publication of non-significant results does not play a decisive role.
From the NHANES dataset records of 3386 euthyroid subjects (1579 female, mean ± SD of age 44.0 ± 14.0 years) were eligible. Median and interquartile range (IQR) of SIQALS 2 results were both 2 with a total range between 0 and 7. In the majority of subjects, Jostel’s TSH index was in the reported reference range (1.3 to 4.1) (41, 54) with a mean of 1.75 and SD of 0.57. Allostatic load was significantly positively associated with both TSH concentration and JTI, and negatively with SPINA-GT, as shown in Figure 2 and Supplementary Table 2. JTI weakly, but significantly increased with age, beta coefficient per year ± SE = 3.8e–4 ± 5.9e–5, p < 1e–9. It was also slightly elevated in diabetes and prediabetes (mean ± SEM 1.85 ± 0.03 and 1.88 ± 0.06, resp., vs. 1.74 ± 0.01 and 1.73 ± 0.01, respective p < 0.001 and < 0.02).
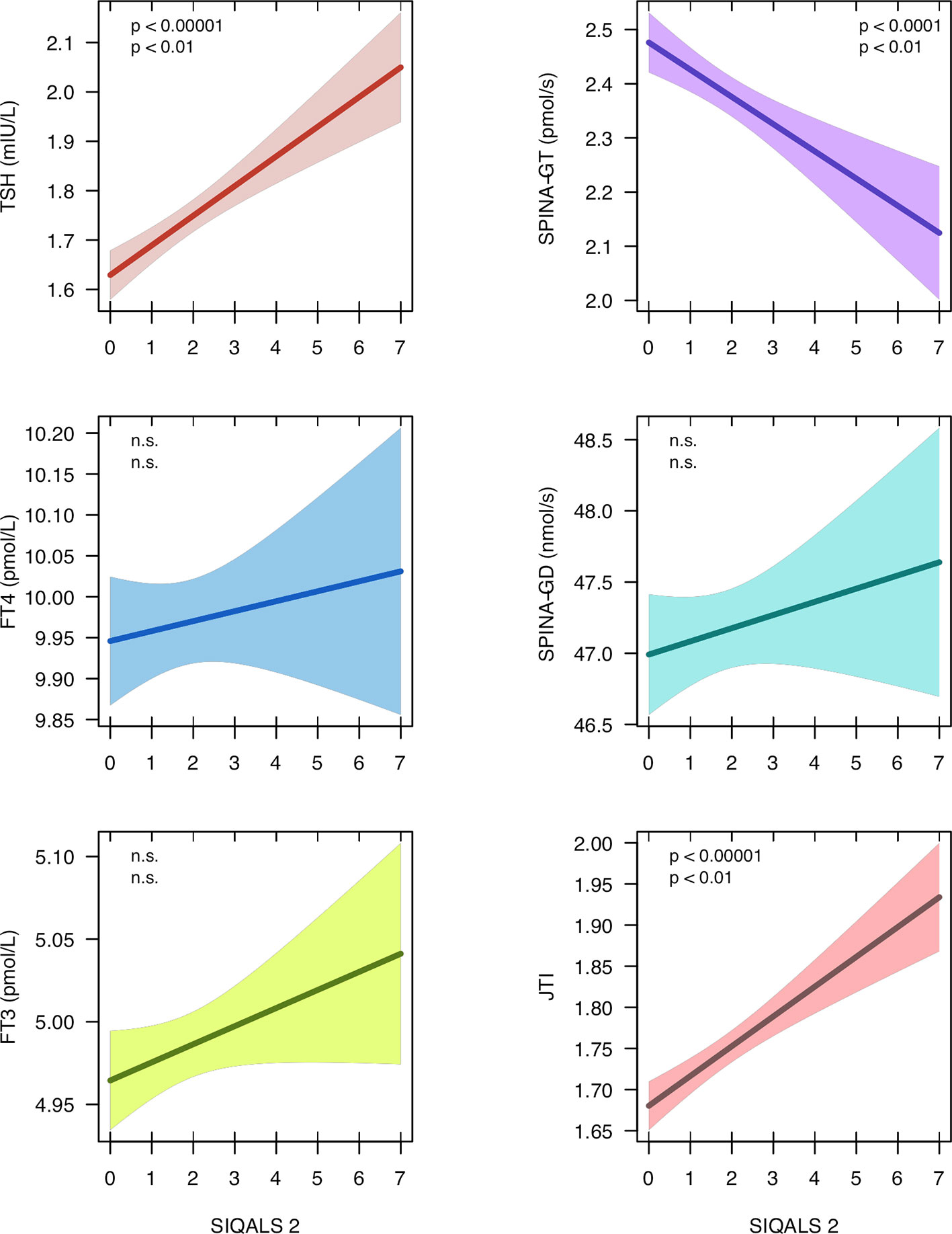
Figure 2 Regression models between type 2 allostatic load (SIQALS 2) and parameters of thyroid homeostasis in 3386 euthyroid subjects. Data were obtained from the National Health and Nutrition Examination Survey (NHANES), 2007 to 2008 (40). Shown is the regression line with 95% confidence bands. In each panel, the first p value refers to unadjusted OLS regression and the second p value to estimates derived from instrumental variable (IV) regression. For regression details, see Supplementary Tables 2 and 6.
Poor socio-economic status (assessed with the family monthly poverty level index) (40, 55), sleep disorder (snoring) and illicit drug usage (cannabis), as reported in the NHANES survey, met above eligibility criteria (Supplementary Tables 3 and 4) and were added as instrumental variables. Since in the multivariable model with 3 IVs the Durbin-Wu-Hausmann test did not reveal an advantage of IV over OLS models and the Sargan test suggested over-identification we simplified the model to two predictors (cannabis and snoring, Supplementary Figure 7). This model with two IVs confirmed the significant relationship between SIQALS 2 and JTI (beta ± SE = 0.09 ± 0.03, p < 0.01), TSH concentration (beta ± SE = 0.13 ± 0.05, p < 0.01) and SPINA-GT (beta ± SE = –0.16 ± 0.06, p < 0.01). No correlations were observed for FT4 or FT3 concentrations and deiodinase activity (SPINA-GD).
Discussion
A high-normal or moderately elevated TSH concentration may arise from different causes including setpoint alterations of the homeostatic system in response to PTSD or other causes of allostatic load, as demonstrated here. High SIQALS 2 scores may raise the TSH concentration by 1.04 mIU/L and Jostel’s TSH index by 0.72, compared to low allostatic load. Given the small dispersion of intra-individual variation in TSH concentration this influence is substantial (56). Therefore, the width of the observed broad interindividual reference ranges for TSH and thyroid hormones in population studies may at least partly result from inter-personal differences in allostatic load, age and other cardiovascular risk factors.
In this secondary analysis, we found allostatic load to be unrelated to FT4 or FT3 concentration, but to negatively correlate with maximum thyroid capacity (SPINA-GT). Likewise, PTSD was accompanied by increased TSH concentration, but did not show a clear association to FT4 levels. Interestingly, the association to TSH even grew stronger with a rising time interval since the traumatic event, possibly due to therapeutic interventions addressing a depression-related component of PTSD.
In summary, high-normal or slightly increased TSH concentration, as typically observed in patients with subclinical hypothyroidism, may ensue from two different etiologies, i.e. very early forms of primary hypothyroidism and set point elevation of the otherwise normal feedback control system. At the population level these two mechanisms may be conflated, so that mean concentrations of peripheral thyroid hormones may remain unaltered, although the underlying causal relationships differ.
Our analysis suggests a possible mechanism linking TSH to cardiovascular complications, which is a sensible alternative to the thyroid failure hypothesis by other authors (16). It also well accounts for the U-shaped relationship between TSH concentration and cardiovascular risk, as elsewhere described (14, 15). Hence, a dual etiology may explain the findings of previous studies. To resolve this ambiguity, measurements of peripheral thyroid hormones are required (11) and should be integrated into future studies—not only for the selection of subjects but also for functional assessment (10, 57, 58). Calculated parameters providing biomarkers for the set point of thyroid homeostasis and peripheral hormone metabolism may provide additional insights, especially in the setting of clinical trials (41). This should avoid ambiguities in the interpretation and provide further direction on potential therapeutic intervention (59).
In conclusion, the link between the central stress response and cardiovascular risk is empirically well established (24, 60, 61). Apparently, as demonstrated here, it also involves the central control of thyroid function. Since thyroid hormones have a profound effect on cardiovascular physiology (62–64), the set point of thyroid homeostasis appears to play a more important role than previously assumed.
Data Availability Statement
The data used for secondary analysis was obtained from the National Health and Nutrition Examination Survey (NHANES) data set, period 2007 to 2008 (https://www.cdc.gov/nchs/nhanes/). The S scripts for generating the figures and meta-analysis, a graphical explanation of instrumental variable regression, supplementary figures and tables with additional results are available as online supplement to this article and from zenodo.org (DOI 10.5281/zenodo.3701232). The protocol for meta-analysis has been registered by PROSPERO with the ID CRD42020208436 and is available from https://www.crd.york.ac.uk/prospero/display_record.php?ID=CRD42020208436.
Author Contributions
JD drafted a first version of the manuscript. RH, JM, FB, and PM edited the text and contributed additional ideas, material, and text passages. All authors contributed to the article and approved the submitted version.
Funding
We acknowledge support by the DFG Open Access Publication Funds of the Ruhr-Universität Bochum.
Conflict of Interest
JD received funding and personal fees by Sanofi-Henning, Hexal AG, Bristol-Myers Squibb, and Pfizer, and is co-owner of the intellectual property rights for the patent “System and Method for Deriving Parameters for Homeostatic Feedback Control of an Individual” (Singapore Institute for Clinical Sciences, Biomedical Sciences Institutes, Application Number 201208940-5, WIPO number WO/2014/088516). The funders had no role in study design, data collection and analysis, decision to publish, or preparation of the manuscript. RH has been paid personal fees in the past for consultancy and code development in general research methods and data analysis.
The remaining authors declare that the research was conducted in the absence of any commercial or financial relationships that could be construed as a potential conflict of interest.
Supplementary Material
The Supplementary Material for this article can be found online at: https://www.frontiersin.org/articles/10.3389/fendo.2020.542710/full#supplementary-material
References
1. Pasqualetti G, Tognini S, Polini A, Caraccio N, Monzani F. Is subclinical hypothyroidism a cardiovascular risk factor in the elderly? J Clin Endocrinol Metab (2013) 98(6):2256–66. doi: 10.1210/jc.2012-3818
2. Rugge JB, Bougatsos C, Chou R. Screening and treatment of thyroid dysfunction: an evidence review for the U.S. Preventive Services Task Force. Ann Intern Med (2015) 162(1):35–45. doi: 10.7326/M14-1456
3. Rodondi N, den Elzen WP, Bauer DC, Cappola AR, Razvi S, Walsh JP, et al. Subclinical hypothyroidism and the risk of coronary heart disease and mortality. JAMA (2010) 304(12):1365–74. doi: 10.1001/jama.2010.1361
4. Biondi B, Cappola AR, Cooper DS. Subclinical Hypothyroidism: A Review. JAMA (2019) 322(2):153–60. doi: 10.1001/jama.2019.9052
5. Stott DJ, Rodondi N, Kearney PM, Ford I, Westendorp RGJ, Mooijaart SP, et al. Thyroid Hormone Therapy for Older Adults with Subclinical Hypothyroidism. N Engl J Med (2017) 376(26):2534–44. doi: 10.1056/NEJMoa1603825
6. Chaker L, van den Berg ME, Niemeijer MN, Franco OH, Dehghan A, Hofman A, et al. Thyroid Function and Sudden Cardiac Death: A Prospective Population-Based Cohort Study. Circulation (2016) 134(10):713–22. doi: 10.1161/CIRCULATIONAHA.115.020789
7. Müller P, Dietrich JW, Lin T, Bejinariu A, Binnebößel S, Bergen F, et al. Usefulness of Serum Free Thyroxine Concentration to Predict Ventricular Arrhythmia Risk in Euthyroid Patients With Structural Heart Disease. Am J Cardiol (2020) 125(8):1162–9. doi: 10.1016/j.amjcard.2020.01.019
8. Li X, Yao Y, Chen Z, Fan S, Hua W, Zhang S, et al. Thyroid-stimulating hormone within the normal range and risk of major adverse cardiovascular events in nonischemic dilated cardiomyopathy patients with severe left ventricular dysfunction. Clin Cardiol (2019) 42(1):120–8. doi: 10.1002/clc.23117
9. Kannan L, Shaw PA, Morley MP, Brandimarto J, Fang JC, Sweitzer NK, et al. Thyroid Dysfunction in Heart Failure and Cardiovascular Outcomes. Circ Heart Fail (2018) 11(12):e005266. doi: 10.1161/CIRCHEARTFAILURE.118.005266
10. Dietrich JW, Makimoto H, Muller P. Letter by Dietrich, et al. Regarding Article, “Thyroid Dysfunction in Heart Failure and Cardiovascular Outcomes”. Circ Heart Fail (2019) 12(4):e005854. doi: 10.1161/CIRCHEARTFAILURE.118.005854
11. Fitzgerald SP, Bean NG, Falhammar H, Tuke J. Clinical Parameters Are More Likely to Be Associated with Thyroid Hormone Levels than with Thyrotropin Levels: A Systematic Review and Meta-analysis. Thyroid (2020). doi: 10.1089/thy.2019.0535
12. Perez AC, Jhund PS, Stott DJ, Gullestad L, Cleland JG, van Veldhuisen DJ, et al. Thyroid-stimulating hormone and clinical outcomes: the CORONA trial (controlled rosuvastatin multinational study in heart failure). JACC Heart Fail (2014) 2(1):35–40. doi: 10.1016/j.jchf.2013.07.008
13. Cappola AR, Fried LP, Arnold AM, Danese MD, Kuller LH, Burke GL, et al. Thyroid status, cardiovascular risk, and mortality in older adults. JAMA (2006) 295(9):1033–41. doi: 10.1001/jama.295.9.1033
14. Rhee CM, Ravel VA, Streja E, Mehrotra R, Kim S, Wang J, et al. Thyroid Functional Disease and Mortality in a National Peritoneal Dialysis Cohort. J Clin Endocrinol Metab (2016) 101(11):4054–61. doi: 10.1210/jc.2016-1691
15. Langen VL, Niiranen TJ, Puukka P, Lehtonen AO, Hernesniemi JA, Sundvall J, et al. Thyroid-stimulating hormone and risk of sudden cardiac death, total mortality and cardiovascular morbidity. Clin Endocrinol (Oxf) (2018) 88(1):105–13. doi: 10.1111/cen.13472
16. Inoue K, Ritz B, Brent GA, Ebrahimi R, Rhee CM, Leung AM. Association of Subclinical Hypothyroidism and Cardiovascular Disease With Mortality. JAMA Netw Open (2020) 3(2):e1920745. doi: 10.1001/jamanetworkopen.2019.20745
17. Aweimer A, El-Battrawy I, Akin I, Borggrefe M, Mügge A, Pataslis PC, et al. Abnormal Thyroid Function is Common in Takotsubo Syndrome and Depends on two Distinct Mechanisms: Results of a Multicenter Observational Study. J Internal Med (2020). doi: 10.1111/joim.13189
18. Hoermann R, Midgley JE, Larisch R, Dietrich JW. Homeostatic Control of the Thyroid-Pituitary Axis: Perspectives for Diagnosis and Treatment. Front Endocrinol (Lausanne) (2015) 6:177. doi: 10.3389/fendo.2015.00177
19. Chatzitomaris A, Hoermann R, Midgley JE, Hering S, Urban A, Dietrich B, et al. Thyroid Allostasis–Adaptive Responses of Thyrotropic Feedback Control to Conditions of Strain, Stress, and Developmental Programming. Front Endocrinol (2017) 8:163. doi: 10.3389/fendo.2017.00163
20. Robertson T, Beveridge G, Bromley C. Allostatic load as a predictor of all-cause and cause-specific mortality in the general population: Evidence from the Scottish Health Survey. PLoS One (2017) 12(8):e0183297. doi: 10.1371/journal.pone.0183297
21. Fava GA, McEwen BS, Guidi J, Gostoli S, Offidani E, Sonino N. Clinical characterization of allostatic overload. Psychoneuroendocrinology (2019) 108:94–101. doi: 10.1016/j.psyneuen.2019.05.028
22. Gillespie SL, Anderson CM, Zhao S, Tan Y, Kline D, Brock G, et al. Allostatic load in the association of depressive symptoms with incident coronary heart disease: The Jackson Heart Study. Psychoneuroendocrinology (2019) 109:104369. doi: 10.1016/j.psyneuen.2019.06.020
23. Mazgelyte E, Karciauskaite D, Linkeviciute A, Mazeikiene A, Burokiene N, Matuzeviciene R, et al. Association of Hair Cortisol Concentration with Prevalence of Major Cardiovascular Risk Factors and Allostatic Load. Med Sci Monit (2019) 25:3573–82. doi: 10.12659/MSM.913532
24. McEwen BS, Akil H. Revisiting the Stress Concept: Implications for Affective Disorders. J Neurosci (2020) 40(1):12–21. doi: 10.1523/jneurosci.0733-19.2019
25. Friedman MJ, Wang S, Jalowiec JE, McHugo GJ, McDonagh-Coyle A. Thyroid hormone alterations among women with posttraumatic stress disorder due to childhood sexual abuse. Biol Psychiatry (2005) 57(10):1186–92. doi: 10.1016/j.biopsych.2005.01.019
26. Mason J, Weizman R, Laor N, Wang S, Schujovitsky A, Abramovitz-Schneider P, et al. Serum triiodothyronine elevation with posttraumatic stress disorder: a cross-cultural study. Biol Psychiatry (1996) 39(10):835–8. doi: 10.1016/0006-3223(95)00310-x
27. Wang S, Mason J. Elevations of serum T3 levels and their association with symptoms in World War II veterans with combat-related posttraumatic stress disorder: replication of findings in Vietnam combat veterans. Psychosom Med (1999) 61(2):131–8. doi: 10.1097/00006842-199903000-00001
28. Armario A, Garcia-Marquez C, Jolin T. The effects of chronic stress on corticosterone, GH and TSH response to morphine administration. Brain Res (1987) 401(1):200–3. doi: 10.1016/0006-8993(87)91184-x
29. Johansson G, Laakso ML, Karonen SL, Peder M. Examination stress affects plasma levels of TSH and thyroid hormones differently in females and males. Psychosom Med (1987) 49(4):390–6. doi: 10.1097/00006842-198707000-00008
30. Schedlowski M, Wiechert D, Wagner TO, Tewes U. Acute psychological stress increases plasma levels of cortisol, prolactin and TSH. Life Sci (1992) 50(17):1201–5. doi: 10.1016/0024-3205(92)90319-k
31. Yehuda R. Psychoneuroendocrinology of post-traumatic stress disorder. Psychiatr Clin North Am (1998) 21(2):359–79. doi: 10.1016/s0193-953x(05)70010-1
32. Newport DJ, Nemeroff CB. Neurobiology of posttraumatic stress disorder. Curr Opin Neurobiol (2000) 10(2):211–8. doi: 10.1016/s0959-4388(00)00080-5
33. Vermetten E, Bremner JD. Circuits and systems in stress. II. Applications to neurobiology and treatment in posttraumatic stress disorder. Depress Anxiety (2002) 16(1):14–38. doi: 10.1002/da.10017
34. Boscarino JA. Posttraumatic stress disorder and physical illness: results from clinical and epidemiologic studies. Ann N Y Acad Sci (2004) 1032:141–53. doi: 10.1196/annals.1314.011
35. Wang S. Traumatic stress and thyroid function. Child Abuse Negl (2006) 30(6):585–8. doi: 10.1016/j.chiabu.2005.11.012
36. Daya SK, Paulus AO, Braxton EE Jr., Vroman PJ, Mathis DA, Lin R, et al. Delayed Diagnosis of TSH-Secreting Adenoma Attributed to Worsening Post-Traumatic Stress Disorder Symptoms in a Military Veteran Because of Provider Anchoring Bias. Mil Med (2017) 182(3):e1849–e53. doi: 10.7205/MILMED-D-16-00241
37. Balduzzi S, Rücker G, Schwarzer G. How to perform a meta-analysis with R: a practical tutorial. Evidence-Based Ment Health (2018) 22(4):153-60. doi: 10.1136/ebmental-2019-300117
38. Viechtauer W. Conducting meta-analyses in R with the metafor package. J Stat Softw (2018) 36(3):1–48. doi: 10.18637/jss.v036.i03
39. R Core Team. R: A Language and Environment for Statistical Computing. Vienna, Austria: R Foundation for Statistical Computing (2018). RRID:SCR_001905.
40. National Center for Health Statistics. National Health and Nutrition Examination Survey: Centers for Diesase Control and Prevention. US Centers for Disease Control and Prevention (CDC) (2020). Available at: https://www.cdc.gov/nchs/nhanes/index.htm.
41. Dietrich JW, Landgrafe-Mende G, Wiora E, Chatzitomaris A, Klein HH, Midgley JE, et al. Calculated Parameters of Thyroid Homeostasis: Emerging Tools for Differential Diagnosis and Clinical Research. Front Endocrinol (Lausanne) (2016) 7:57. doi: 10.3389/fendo.2016.00057
42. Baiocchi M, Cheng J, Small DS. Instrumental variable methods for causal inference. Stat Med (2014) 33(13):2297–340. doi: 10.1002/sim.6128
44. Kosten TR, Wahby V, Giller E Jr., Mason J. The dexamethasone suppression test and thyrotropin-releasing hormone stimulation test in posttraumatic stress disorder. Biol Psychiatry (1990) 28(8):657–64. doi: 10.1016/0006-3223(90)90452-8
45. Mason J, Southwick S, Yehuda R, Wang S, Riney S, Bremner D, et al. Elevation of serum free triiodothyronine, total triiodothyronine, thyroxine-binding globulin, and total thyroxine levels in combat-related posttraumatic stress disorder. Arch Gen Psychiatry (1994) 51(8):629–41. doi: 10.1001/archpsyc.1994.03950080041006
46. Kozaric-Kovacic D, Karlovic D, Kocijan-Hercigonja D. Elevation of serum total triiodothironine and free triiodothironine in Croatian veterans with combat-related post-traumatic stress disorder. Mil Med (2002) 167(10):846–9. doi: 10.1093/milmed/167.10.846
47. Goenjian AK, Pynoos RS, Steinberg AM, Endres D, Abraham K, Geffner ME, et al. Hypothalamic-pituitary-adrenal activity among Armenian adolescents with PTSD symptoms. J Trauma Stress (2003) 16(4):319–23. doi: 10.1023/A:1024453632458
48. Karlovic D, Marusic S, Martinac M. Increase of serum triiodothyronine concentration in soldiers with combat-related chronic post-traumatic stress disorder with or without alcohol dependence. Wien Klin Wochenschr (2004) 116(11-12):385–90. doi: 10.1007/BF03040918
49. Kamoi K, Tanaka M, Ikarashi T, Miyakoshi M. Effect of the 2004 mid niigata prefecture earthquake on patients with endocrine disorders. Endocr J (2006) 53(4):511–21. doi: 10.1507/endocrj.k06-022
50. Olff M, Guzelcan Y, de Vries GJ, Assies J, Gersons BP. HPA- and HPT-axis alterations in chronic posttraumatic stress disorder. Psychoneuroendocrinology (2006) 31(10):1220–30. doi: 10.1016/j.psyneuen.2006.09.003
51. Bunevicius A, Leserman J, Girdler SS. Hypothalamic-pituitary-thyroid axis function in women with a menstrually related mood disorder: association with histories of sexual abuse. Psychosom Med (2012) 74(8):810–6. doi: 10.1097/PSY.0b013e31826c3397
52. Loo WT, Liu Q, Yip MC, Wang M, Chow LW, Cheung MN, et al. Status of oral ulcerative mucositis and biomarkers to monitor posttraumatic stress disorder effects in breast cancer patients. Int J Biol Markers (2013) 28(2):168–73. doi: 10.5301/jbm.5000025
53. Sinai T, Hirvikoski T, Nordström A-L, Nordström P, Nilsonne Å, Wilczek A, et al. Hypothalamic pituitary thyroid axis and exposure to interpersonal violence in childhood among women with borderline personality disorder. Eur J Psychotraumatol (2014) 5(1):23911. doi: 10.3402/ejpt.v5.23911
54. Jostel A, Ryder WD, Shalet SM. The use of thyroid function tests in the diagnosis of hypopituitarism: definition and evaluation of the TSH Index. Clin Endocrinol (Oxf) (2009) 71(4):529–34. doi: 10.1111/j.1365-2265.2009.03534.x
55. ASPE. The poverty guidelines updated periodically in the Federal Register by the U.S. Department of Health and Human Services under the authority of 42 U.S.C. 9902(2). US Department of Health and Human Services (2007).
56. Hoermann R, Midgley JEM, Larisch R, Dietrich JW. Functional and Symptomatic Individuality in the Response to Levothyroxine Treatment. Front Endocrinol (Lausanne) (2019) 10:664. doi: 10.3389/fendo.2019.00664
57. Hoermann R, Midgley JEM, Larisch R, Dietrich JW. Recent Advances in Thyroid Hormone Regulation: Toward a New Paradigm for Optimal Diagnosis and Treatment. Front Endocrinol (Lausanne) (2017) 8:364. doi: 10.3389/fendo.2017.00364
58. Hoermann R, Midgley JEM, Larisch R, Dietrich JW. The role of functional thyroid capacity in pituitary thyroid feedback regulation. Eur J Clin Invest (2018) 48(10):e13003. doi: 10.1111/eci.13003
59. Midgley JEM, Toft AD, Larisch R, Dietrich JW, Hoermann R. Time for a reassessment of the treatment of hypothyroidism. BMC Endocr Disord (2019) 19(1):37. doi: 10.1186/s12902-019-0365-4
60. Sterling P, Eyer J. Biological basis of stress-related mortality. Soc Sci Med Part E: Med Psychol (1981) 15(1):3–42. doi: 10.1016/0271-5384(81)90061-2
61. Tawakol A, Ishai A, Takx RA, Figueroa AL, Ali A, Kaiser Y, et al. Relation between resting amygdalar activity and cardiovascular events: a longitudinal and cohort study. Lancet (2017) 389(10071):834–45. doi: 10.1016/S0140-6736(16)31714-7
62. Vargas-Uricoechea H, Sierra-Torres CH. Thyroid hormones and the heart. Horm Mol Biol Clin Invest (2014) 18(1):15–26. doi: 10.1515/hmbci-2013-0059
63. Jabbar A, Pingitore A, Pearce SH, Zaman A, Iervasi G, Razvi S. Thyroid hormones and cardiovascular disease. Nat Rev Cardiol (2017) 14(1):39–55. doi: 10.1038/nrcardio.2016.174
Keywords: allostatic load, subclinical hypothyroidism, thyroid homeostasis, thyrotropin, malignant arrhythmia, sudden cardiac death, post-traumatic stress disorder (PTSD)
Citation: Dietrich JW, Hoermann R, Midgley JEM, Bergen F and Müller P (2020) The Two Faces of Janus: Why Thyrotropin as a Cardiovascular Risk Factor May Be an Ambiguous Target. Front. Endocrinol. 11:542710. doi: 10.3389/fendo.2020.542710
Received: 13 March 2020; Accepted: 02 October 2020;
Published: 26 October 2020.
Edited by:
Bernadette Biondi, University of Naples Federico II, ItalyReviewed by:
Giuseppe Pasqualetti, University of Pisa, ItalyCopyright © 2020 Dietrich, Hoermann, Midgley, Bergen and Müller. This is an open-access article distributed under the terms of the Creative Commons Attribution License (CC BY). The use, distribution or reproduction in other forums is permitted, provided the original author(s) and the copyright owner(s) are credited and that the original publication in this journal is cited, in accordance with accepted academic practice. No use, distribution or reproduction is permitted which does not comply with these terms.
*Correspondence: Johannes Wolfgang Dietrich, johannes.dietrich@ruhr-uni-bochum.de