- 1Department of Clinical Epidemiology, Steno Diabetes Center Copenhagen, Gentofte, Denmark
- 2Steno Diabetes Center Copenhagen, Gentofte, Denmark
- 3Department of Population Health and Morbidity, Health in Greenland, University of Southern Denmark, Odense, Denmark
- 4Institute of Nursing and Health Science, University of Greenland, Nuuk, Greenland
- 5Steno Diabetes Center Aarhus, Aarhus, Denmark
Background: Glycemic variability (GV) may attribute to the pathogenesis of diabetic neuropathy. The aim of this cross-sectional study was to investigate the association between GV and distal symmetric polyneuropathy (DSPN) and cardiovascular autonomic neuropathy (CAN) in a Danish population of young adults with type 1 diabetes.
Methods: Young adults between 18 and 24 years with type 1 diabetes were included in this cross-sectional study. CAN was assessed by cardiovascular autonomic reflex tests (CARTs) and heart rate variability (HRV). DSPN was assessed by light pressure, pain and vibration perception, electrochemical skin conductance, sural nerve conduction velocity (SNCV), and amplitude potential (SNAP). GV were obtained by continuous glucose monitoring including coefficient of variation (CV), SD, continuous overall net glycemic action (CONGA), and mean amplitude of glucose excursions (MAGE).
Results: The study comprised 133 young adults (43.6% males), mean age of 22 years (SD 1.6). Unadjusted, higher CV was associated with a decreased risk of sural nerve conduction (P = 0.03), abnormal SNAP (P = 0.04) and incidents of definite CAN (P = 0.04). Likewise, higher CONGA was associated with increasing incidents of subclinical DSPN (P = 0.03), abnormal SNAP (P = 0.01), and SNCV (P = 0.02). However, both associations were not statistically significant in the fully adjusted model. Higher MAGE was associated with slightly increasing measures of HRV (P = 0.03) but only when fully adjusted. When correcting for multiple tests significance was lost. A significant association was found between HbA1c and measures of both DSPN (P < 0.02) and HRV (P < 0.03) in fully adjusted models.
Conclusions: No significant associations between GV and diabetic neuropathy were found after adjusting for risk factors and multiple tests. This suggests that GV may not be a risk factor for diabetic neuropathy in young adults with type 1 diabetes. However, long-term effects of GV excursions may still play a role in the pathogenic mechanisms leading to neuropathy in later life.
Introduction
Distal symmetric polyneuropathy (DSPN) and cardiovascular autonomic neuropathy (CAN) are severe and common complications of type 1 diabetes (1, 2). DSPN and CAN are usually rare problems during childhood but may be present in adolescents and young adults (3, 4). Improved glycemic control in patients with type 1 diabetes may prevent and revert early stages of DSPN and CAN and slow the progression toward overt neuropathy (5–9). Thus, detecting and preventing diabetic neuropathy at an early stage is essential.
The Diabetes Control and Complications Trial (DCCT) (10) demonstrated that intensive glycemic control in type 1 diabetes monitored by HbA1c reduced the onset and progression of diabetic neuropathy. Glycated hemoglobin (HbA1c) is an integrated assessment marker of glycaemia in diabetes treatment (11) and is associated with increased risk of diabetic complications like neuropathy. However, some limitations arise when using measures of HbA1c to evaluate the role of glucose variability (GV): HbA1c depicts an average of blood glucose over 3 months and does not reflect incidents of hypo- and hyperglycemia on a daily basis (12). Hence HbA1c may be an insufficient tool to monitor and treat dysglycaemia. Diurnal GV may contribute to the risk of diabetic complications beyond HbA1c (13). The international consensus panel from the Advanced Technologies & Treatments for Diabetes Congress in February 2017 recommends using data from continuous glucose monitoring (CGM) and the coefficient of variation (CV) as the primary measure to assess GV.
Data on the association between GV and diabetic neuropathy is inconsistent, scarce, and has not previously been investigated in young adults with type 1 diabetes by applying CGM (14–19). Several studies point to glucose fluctuation as a risk factor for CAN in adults with type 1 diabetes (15–17). However, studies on the association between GV and peripheral neuropathy are conflicting (14, 15), possibly due to inconsistent use of various measures and definitions to assess GV and neuropathy in different studies. While large GV has been associated to oxidative stress, GV may attribute to the pathogenesis of diabetic neuropathy despite conflicting reports (13, 20). Studies on the association between GV and CAN and DSPN in type 2 diabetes are limited. However, they do demonstrate that variability of HbA1c in particular but also measures of GV assessed from CGM are associated with both CAN and DSPN (21–25).
The aim of this study was to investigate the association between modifiable glycaemic risk factors of neuropathy including GV and early and possibly reversible signs of DSPN and CAN early in the life of type 1 diabetes where preventive measures may have a substantial effect later in life. This was done in a Danish population of young adults with type 1 diabetes using the newest recommendations for assessing GV and both novel and established measures for DSPN and CAN.
Materials and Methods
Study Population
The study was designed as a cross-sectional observational study. The structure has been described in detail previously (4).
In order to investigate a population with early signs of diabetic neuropathy a cohort of young adults was assessed. All participants (age between 18 and 24 years) were recruited from the outpatient clinic at Steno Diabetes Center Copenhagen, Gentofte, Denmark. Participants were included regardless of duration of type 1 diabetes. Three hundred and fifty participants received a written invitation to participate and were subsequently contacted by phone. Written informed consent was obtained prior to examination. Ethical approval was obtained from The Danish Research Ethics Committee (project id.: H-15006967) (26).
Participants were excluded from the present study if they were not able to wear the CGM or failed to measure and log their capillary blood glucose during the 5-days of CGM-monitoring. Moreover, examination of CAN was not performed if they were treated with beta blockers.
There was no basis for conducting sensible power calculations to estimate a sample size for the aim of the study, because the investigated associations have not previously been investigated in young adults with type 1 diabetes with the use of CGM. Moreover, previous studies in other patient groups have demonstrated conflicting results.
Assessment of Diabetic Neuropathy
DSPN was assessed and categorized according to recommendations made by the Toronto Diabetic Neuropathy Expert Group (2). Questionnaires were used to assess symptoms of DSPN (see section “Questionnaires on peripheral neuropathy and exposures” for further details).
Signs of DSPN were evaluated by established measures: Light pressure perception was assessed by applying a 10-g monofilament until it buckled (Neuropen®, Owen Mumford Ltd, Oxford, UK) to three points at the distal bilateral foot pads: just proximal to the great, third and fifth toe (27, 28). Pain sensation was evaluated by using a 40-gram pin prick device (Neuropen®, Owen Mumford Ltd, Oxford, UK) applied at the dorsal side of the toes just proximal to the nail on the great, third, and fifth toe. Vibration perception threshold (VPT) was determined using a Bio-Thesiometer (Bio-medical instruments, Ohio, USA) at the distal end of the great toe on both feet, and age stratified perception thresholds were used to assess abnormal results (29).
Novel measures were used to objectively evaluate DSPN: Small autonomic fiber function was assessed using the non-invasive device Sudoscan™ (Impeto Medical, Paris, France) performing an electrochemical skin conductance (ESC) test on the hands and feet (30). Age and gender stratified ESC thresholds were applied (31). Sural nerve conduction velocity (SNCV) and amplitude potential (SNAP) were obtained by the handheld NC-Stat® DPNCheckTM (NeuroMetrix, Inc., Waltham, USA) (32). Age and height stratified SNAP and SNCV thresholds were used (33). Participants were examined for bilateral abnormalities in SNAP and SNCV. A composite measure, “sural nerve conduction” (SNC), was used when abnormalities in either SNAP, SNCV or both bilaterally. DSPN was defined according to four categories. The label “possible DSPN” was given if presence of symptoms of peripheral neuropathy as assessed by questionnaires, or signs as assessed by VPT, light pressure and pain perception were confirmed. If presence of symptoms and signs were confirmed the label “probable DSPN” was added. “Confirmed DSPN” was given if either the test for SNC or ESC was abnormal and if the participants had symptoms or signs. Ultimately, “subclinical DSPN” was defined as presence of abnormal SNC or ESC without symptoms or signs.
To evaluate CAN three standard cardiovascular autonomic reflex tests (CARTs) and measures of 5 min. resting heart rate variability (HRV) were performed in a quiet examination room. HRV was assessed after 5 min of supine rest and analyzed from 5-min resting heart rate (HR). HRV indices were analyzed in time- and frequency-domain. Time-domain included the root mean square of the sum of the squares of differences between consecutive R–R intervals (RMSSD) and standard deviation of normal-to-normal intervals (SDNN). Frequency-domain included low-frequency power band (LF) (0.04–0.15 Hz), high-frequency power band (HF) (0.15–0.4 Hz), total frequency power (Total) and the ratio low-frequency power/high-frequency power (LH/HF-ratio) (34).
The 5-min resting HRV test was followed by the three CARTs including the lying-to-standing test (30:15), the deep breathing test (E:I) and Valsalva Maneuver. “Early CAN” was defined as one out of the three CARTs was abnormal, “definite CAN” was defined as two or three were abnormal. Thresholds for abnormal results were age stratified (35). Resting HRV indices and CARTs were registered by using Vagus™ (Medicus Engineering, Aarhus, Denmark).
In line with the recommended criteria for examination of CAN (26), participants were asked to restrain from vigorous exercise 24 h before examination and from caffeine consumption on the specific day of examination.
Questionnaires on Peripheral Neuropathy and Exposures
Each patient was asked to fill in the questionnaires Brief Pain Inventory (BPI) and Michigan Neuropathy Screening Instrument (MNSI) on the examination day. Participants were diagnosed with painful diabetic neuropathy if they in the BPI questionnaire answered having pain in both legs and/or both arms peripherally (36). A MNSI score of ≥7 was interpreted as presence of neuropathy (37).
Moreover, a questionnaire considering life style factors such as smoking status (current, former, or never) and weekly amount of exercise in hours (pooled light and moderate/vigorous exercise) was filled in.
Assessment of GV Indices
The CGM sensor Enlite™ (Medtronic, Northridge, CA) was inserted into the subcutaneous tissue of the abdomen or alternatively the upper arm. Subsequently the iPro2™ (Medtronic, Northridge, CA) recorder was attached. The sensors should be worn for 5 days and the capillary finger blood glucose monitored four times daily for calibration. The software Medtronic CareLink™ iPro™ was used to generate data from the sensors. Participants were excluded from the study if there were not enough measurements of the capillary blood glucose to run the Medtronic CareLink™ iPro™ software. CV, standard deviation (SD), continuous overall net glycemic action (CONGA), and mean amplitude of glucose excursions (MAGE) were used to quantify GV (38). Time spent in hypo- (<3.0 mmol/l), eu- (≥3.0; ≤ 10.0 mmol/l), and hyperglycemia (>10.0 mmol/l) were calculated (38) and presented in minutes and percentage.
Blood Pressure and Anthropometric Measures
Blood pressure and heart rate (HR) were measured after 10 min of rest and calculated as the mean of three consecutive measures performed with intervals of 1 min. Automated oscillometric blood pressure recorders were used (AND UA-787plus, A&D medical, California, USA).
Height and weight were measured with clothes on but without shoes using a fixed rigid stadiometer (Seca, Chino, USA) and an electronic scale (Mettler Toledo, Glostrup, Denmark), respectively.
Biochemical Measures
All biochemical measures were analyzed from venous blood samples except for urine albumin and creatinine. Blood and urine samples were collected on the same day as the examination. The participants were non-fasting.
HbA1c was analyzed by high performance liquid chromatography on a Tosoh G7 (Tosoh Cooperation, Japan). C-peptide was measured using a Cobas e411 (Roche Diagnostics, Mannheim, Germany). Triglycerides, HDL, and total cholesterol were analyzed by standard enzymatic colorimetry techniques on a Vitros 5600 (Ortho Clinical Diagnostics, France). Serum LDL cholesterol was calculated using the Friedewald equationTriglyceride level did not exceed 4.5 mmol/l in any subject. Hence, no other LDL assessments were deemed relevant. Plasma creatinine was analyzed by two-point rate enzymatic technique. The Chronic Kidney Disease Epidemiology (CKD-EPI) equation was used to estimate eGFR (39). Urinary albumin-to-creatinine ratio was analyzed by quantitative immunological turbidimetry.
Medication
Data on medication were extracted from hospital electronic records and validated by the patient at examination day.
Statistical Analysis
Patient characteristics are presented as means with standard deviation (SD) or in case of skewed distributions as medians with interquartile range [IQR].
Participants were excluded from the analysis of a specific test if the values were missing.
Both GV and HbA1c were examined as determinants for neuropathy. The associations were assessed by logistic regression for the categorical outcomes and presented as odds ratios (OR) with 95% confidence interval (CI). Linear regression analyses were applied for continuous outcomes and presented as estimates with 95% CI. To meet model assumptions outcomes were log-transformed prior to analysis and subsequently back transformed to original scale where appropriate. To avoid small-sample bias, determinants of DSPN and CAN were not included in the analyses if the number of affected participants were <5.
Four models of adjustments were applied: Model 1: Unadjusted; Model 2: Adjusted for age and gender; Model 3: Adjusted as model 2 + diabetes duration, BMI, exercise and HbA1c; Model 4: Adjusted as model 3 + systolic blood pressure, triglycerides, LDL cholesterol and current smoking. HR was included as a confounder in models where HRV indices were determinants.
All analyses used 2-sided P = 0.05 as statistically significant and were adjusted for multiple tests by the Benjamini-Hochberg procedure (40).
Statistical analyses were performed in R version 3.3.3 (The R Foundation for Statistical Computing) and SAS, version 9.4 (SAS Institute, Cary, NC, USA).
Results
Patient Characteristics
Overall, 133 young adults (43.6% male) were included in the study. Twenty-three participants were excluded due to missing or lacking CGM-monitoring including insufficient numbers of capillary finger blood glucose monitoring. Reasons for not wearing a CGM sensor were primarily irritative/allergic reactions to the bandage patches or fear of discomfort. Mean (SD) age was 22 years (1.6), diabetes duration 11 years (5.2), and median (IQR) HbA1c 65.5 mmol/mol (57;74). Mean (SD) BMI was 24.7 kg/m2 (3.8) and 122 (92.4%) participants exercised regularly for an average of 9 h weekly. All participants were treated with insulin. Participant characteristics are presented in Table 1.
Diabetic Neuropathy
The results of the prevalence of DSPN and CAN have been discussed elsewhere (4). In total, 51.1% (n = 68) were diagnosed with subclinical DSPN. One patient (0.8%) had confirmed DSPN, and two (1.5%) possible DSPN. None met the criteria for probable DSPN. Prevalence estimates of symmetric abnormal SNAP and SNCV were 20.3% (n = 26) and 34.4% (n = 44), respectively. Prevalence of the composite measure of SNAP/SNCV, SNC was 48.4% (n = 62). Abnormal ESC results on feet were found in 4.5% (n = 6) and 3% (n = 4) on hands. Symmetrically abnormal VPT was detected in 0.8% (n = 1) and likewise for symmetrical neuropathy diagnosed by the BPI questionnaire. No participants had abnormal results when light touch, pain perception or MNSI questionnaire were used.
Definite CAN was diagnosed in 6.1% (n = 8) and early CAN in 26.9% (n = 35).
Distribution and prevalence estimate of the outcomes are presented in Table 2.
Glucose Variability
Mean (SD) CV was 40% (10) and median (IQR) SD was 3.9 mmol/l (3.2;4.7). Distribution of GV measures are presented in Table 2.
Association Between Glucose Variability and Diabetic Neuropathy
Coefficient of Variation (CV)
Greater CV was associated with a decrease in incidents of symmetric abnormalities in SNAP, SNC, and definite CAN unadjusted and when adjusted for age and gender in model 2. Only for SNC significance remained in fully adjusted models. In addition, higher CV was inversely associated to incidents of subclinical DSPN in fully adjusted models (Table 3, Figure 1). However, both associations lost significance after applying the Benjamini-Hochberg procedure (40) to account for multiple testing.
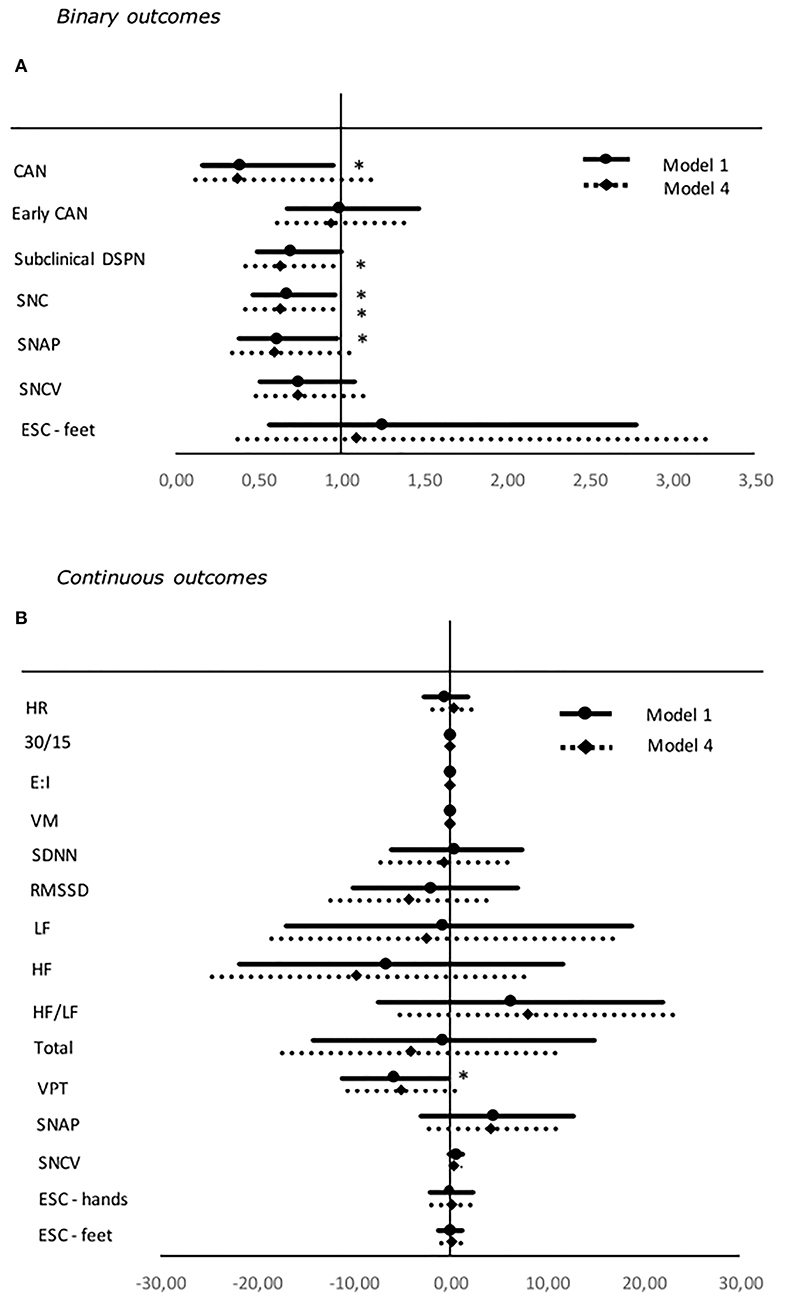
Figure 1. Forest plot of the associations between standardized values of CV and both binary (A) and continuous (B) neuropathy endpoints. For binary outcomes results are presented as odds ratio and 95% confidence intervals. Odds ratio shows the change in odds for an increase of one deviation in the HbA1c. For continuous outcomes results are presented as estimates and 95% confidence intervals. Estimates show the percentage change in the outcomes for an increase of one standard deviation in the SD. Studies with confidence interval crossing the vertical line are inconclusive. Model 1: unadjusted. Model 4: adjusted for age, gender, diabetes duration, BMI, exercise, systolic blood pressure, triglycerides, LDL cholesterol, and current smoking. SDNN, RMSSD, LF, HF, LF/HF ratio, and total are adjusted for HR in every model. Outcomes of DSPN are define as presence of symmetric abnormal results. Binary outcomes were only included in the analyses if presence of five or more abnormal events. CV, coefficient of variation; CAN, Cardiovascular autonomic neuropathy; SNC, sural nerve conduction; SNAP, sural nerve amplitude potential; SNCV, sural nerve conduction velocity; ESC, Electrochemical skin conduction; RMSSD, root mean square of the sum of the squares of differences between consecutive R–R intervals; SDNN, standard deviation of normal-to-normal intervals; LF/HF ratio, low-frequency power/high-frequency power ratio; VPT, vibration perception threshold. *P < 0.05.
Standard Deviation (SD)
Higher SD was associated to a decreased risk of subclinical DSPN and increasing levels of the continuous outcome SNCV when adjusted for diabetes duration, BMI, and exercise and HbA1c in model 3. Again, no significance persisted after correcting for multiple tests (Table 4, Figure 2).
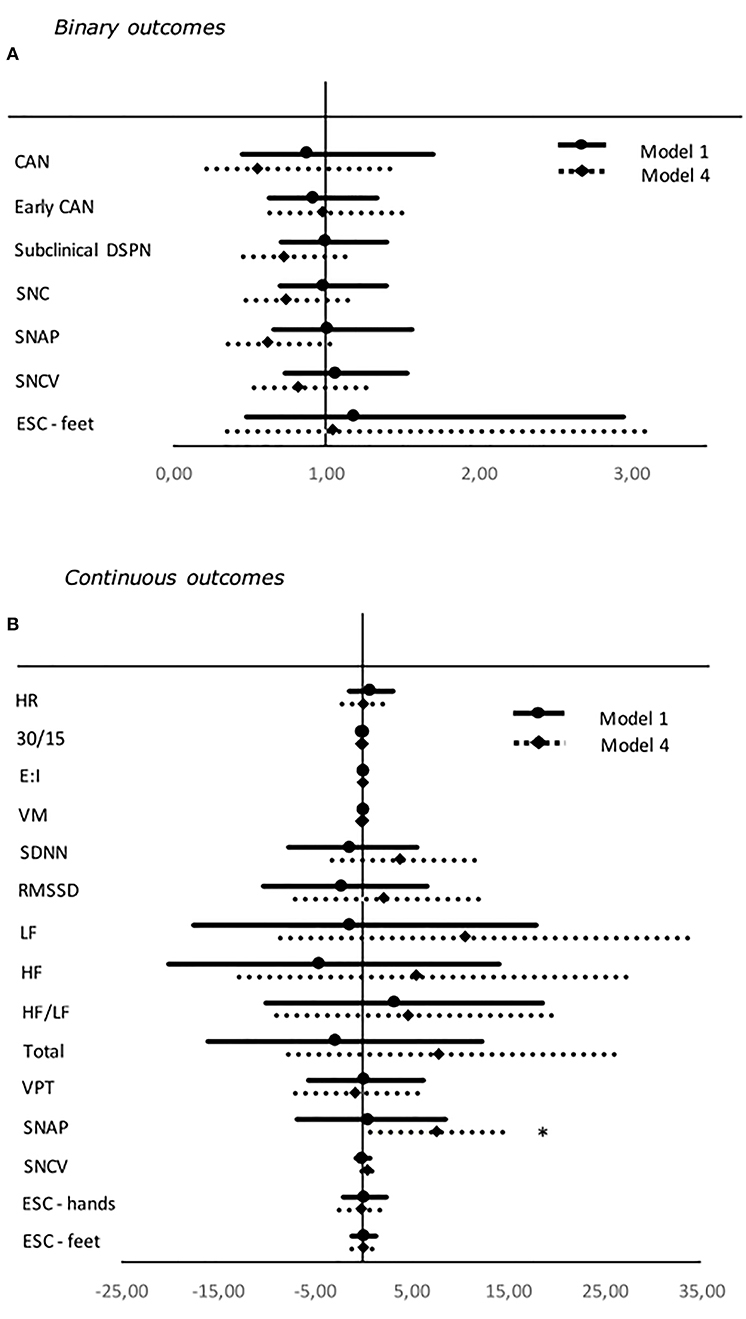
Figure 2. Forest plot of the associations between standardized values of SD and both binary (A) and continuous (B) neuropathy endpoints. For binary outcomes results are presented as odds ratio and 95% confidence intervals. Odds ratio shows the change in odds for an increase of one deviation in the HbA1c. For continuous outcomes results are presented as estimates and 95% confidence intervals. Estimates show the percentage change in the outcomes for an increase of one standard deviation in the SD. Studies with confidence interval crossing the vertical line are inconclusive. Model 1: unadjusted. Model 4: adjusted for age, gender, diabetes duration, BMI, exercise, systolic blood pressure, triglycerides, LDL cholesterol, and current smoking. SDNN, RMSSD, LF, HF, LF/HF ratio, and total are adjusted for HR in every model. Outcomes of DSPN are define as presence of symmetric abnormal results. Binary outcomes were only included in the analyses if presence of five or more abnormal events. CAN, Cardiovascular autonomic neuropathy; SNC, sural nerve conduction; SNAP, sural nerve amplitude potential; SNCV, sural nerve conduction velocity; ESC, Electrochemical skin conduction; RMSSD, root mean square of the sum of the squares of differences between consecutive R–R intervals; SDNN, standard deviation of normal-to-normal intervals; LF/HF ratio, low-frequency power/high-frequency power ratio; VPT, vibration perception threshold. *P < 0.05.
Continuous Overall Net Glycemic Action (CONGA)
In the unadjusted model 1 higher CONGA was significantly associated to increasing incidents of subclinical DSPN, symmetric abnormalities SNAP, SNCV, and the composite measure SNC. Moreover, higher CONGA was associated to decreasing levels of the continuous measure of SNCV. When adjusted for gender and age in model 2 significance was kept for every outcome and in addition higher CONGA was associated with an increased risk of definite CAN. However, for every estimate significance was lost when adjusted for diabetes duration, BMI, and exercise and HbA1c in model 3 (Table A1).
Mean Amplitude of Glucose Excursions (MAGE)
Greater MAGE became significantly associated with higher continuous measures of HRV only in the fully adjusted model 4 (Table A2) but when correcting for multiple tests significance was lost.
Time Spent in Hypo-, Eu-, and Hyperglycemia
None of the determinants “time spent in hypo-, eu-, and hyperglycemia” were significantly associated with diabetic neuropathy (Tables A3–A5).
Association Between HbA1c and Diabetic Neuropathy
An increase in HbA1c was associated with higher odds of subclinical DSPN when adjusted for diabetes duration, BMI, and exercise in model 3. Higher HbA1c was significantly associated with increasing incidents of symmetric abnormalities in SNAP, SNCV, and the composite measure SNC in the fully adjusted model 4. Congruently, higher HbA1c was associated to decreasing continuous values of SNAP and SNCV in model 4. Also, increasing levels of HbA1c were associated with an increase in heart rate (HR) in model 3 and decreasing measures of HRV in model 4 (Table 5, Figure 3).
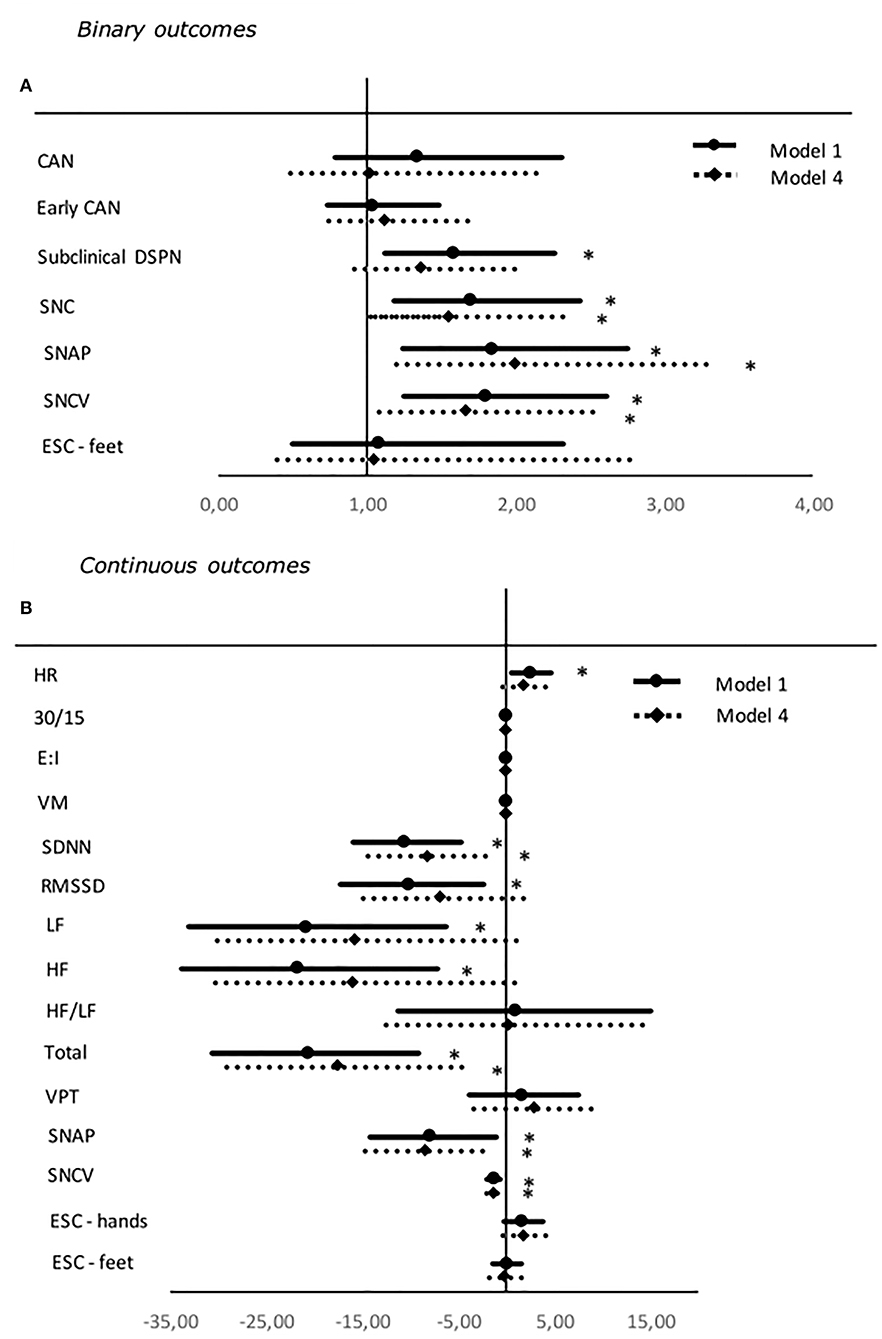
Figure 3. Forest plot of the associations between standardized values of HbA1c and both binary (A) and continuous (B) neuropathy endpoints. For binary outcomes results are presented as odds ratio and 95% confidence intervals. Odds ratio shows the change in odds for an increase of one deviation in the HbA1c. For continuous outcomes results are presented as estimates and 95% confidence intervals. Estimates show the percentage change in the outcomes for an increase of one standard deviation in the SD. Studies with confidence interval crossing the vertical line are inconclusive. Model 1: unadjusted. Model 4: adjusted for age, gender, diabetes duration, BMI, exercise, systolic blood pressure, triglycerides, LDL cholesterol, and current smoking. SDNN, RMSSD, LF, HF, LF/HF ratio, and total are adjusted for HR in every model. Outcomes of DSPN are define as presence of symmetric abnormal results. Binary outcomes were only included in the analyses if presence of five or more abnormal events. CAN, Cardiovascular autonomic neuropathy; SNC, sural nerve conduction; SNAP, sural nerve amplitude potential; SNCV, sural nerve conduction velocity; ESC, Electrochemical skin conduction; RMSSD, root mean square of the sum of the squares of differences between consecutive R–R intervals; SDNN, standard deviation of normal-to-normal intervals; LF/HF ratio, low-frequency power/high-frequency power ratio; VPT, vibration perception threshold. *P < 0.05.
Discussion
In this cross-sectional study 133 young adults with type 1 diabetes were identified with modest GV (38) with a mean coefficient of variation (CV) of 40% (38).
Modest associations between GV and measures of peripheral and autonomic diabetic neuropathy were found in the study. Higher CV was, against expectations, associated with decreased risk of DSPN and CAN, although not statistically significant when adjusting for relevant risk factors and multiple tests. Higher CONGA was associated with increasing incidents of both peripheral and autonomic neuropathy, but findings were confounded by relevant risk factors for diabetic neuropathy. After adjusting for risk factors higher MAGE was significantly associated with a slight increase in measures of HRV indicating an improvement of CAN. This may just be spurious findings—notably when the significant findings were attenuated after correcting for multiple tests. Overall only modest associations were found between GV and DSPN and CAN. Associations were confounded by known risk factors.
However, significant associations were found between higher levels of HbA1c and increased risks of both peripheral and autonomic neuropathy in fully adjusted models. This only supports earlier findings of high levels of HbA1c being an established and essential risk factor of diabetic neuropathy in type 1 diabetes (41).
Previous studies have revealed conflicting conclusions when examining the association between GV and diabetic neuropathy. Lachin et al. (17) evaluated 1,441 participants with type 1 diabetes and a mean age of 27 years from the DCCT. CARTs were performed every 2 years to evaluate CAN. GV was assessed by SD, MAGE, and M-value (a hybrid of glucose exposure and glycemic variability) (42). No significant associations were found between GV and CAN after correcting for within-day and longitudinal mean blood glucose and multiple tests. However, in the DCCT the GV parameters were calculated from 7 fingerstick glucose levels per day and this may have been an inadequate metrics of GV to detect GV's association with CAN.
Jaiswal et al. (16) found modest associations between GV and CAN. The study included 44 participants with type 1 diabetes and a mean age of 34 years. CAN was assessed by CARTs and HRV. Five days CGM was used to compute GV measures: low blood glucose index (LBGI) and area under the curve (AUC) for hypoglycemia. Significant inverse associations were found between both LBGI and AUC and the HF and LF power which implicates impaired autonomic function when longer and more severe hypoglycemia. However, when adjusting for relevant risk factors significance was attenuated. No relationship was identified between GV and any of the CARTs. Moreover, Kwai et al. (14) found significant associations between MAGE, assessed from 6-days CGM, and median motor and sensory excitability assessment in a study comprising 17 participants with type 1 diabetes and a mean age of 28.6 years. The findings of the study point at impairment of peripheral neuropathy induced by higher GV.
Nyiraty et al. (18) investigated the association between GV and autonomic neuropathy (AN) in 20 participants with type 1 diabetes and a mean age of 39.5 years. Fifty percent of the participants were diagnosed with CAN. AN was evaluated by CARTs and orthostatic blood pressure assessment. GV was assessed by 6 days of CGM and calculation of SD, MAGE, CONGA and mean absolute glucose (MAG). Only a correlation between SD and orthostatic hypotension was significant after adjusting for relevant risk factors. Again, the study does not provide a clear conclusion on the relationship between diabetic neuropathy and GV.
Studies investigating the association between GV and diabetic neuropathy in type 2 diabetes do not present uniform results. The number of studies investigating the association is limited and in particular studies assessing GV by CGM. However, CV in CGM was previously found to increase the risk of CAN in type 2 diabetes (23). Moreover, MAGE was significantly associated to DPN (24). Other studies have identified significant relations between variability in HbA1c and both DSPN and CAN (21–23). This may indicate an association between GV and diabetic neuropathy in type 2 diabetes however, like for studies concerning type 1 diabetes, there is a need for more studies with comparable measures of GV.
The results of our study are pointing at GV not being a risk factor for developing CAN and DSPN. However, the study has its limitations making causal conclusions difficult. We did find a significant association between higher levels of HbA1c and CAN and DSPN which to some extent shows that poor glycemic control is indeed a risk factor for diabetic neuropathy.
Strengths and Limitations
The cross-sectional design of the study is not ideal when examining the relationship between GV and diabetic neuropathy in a causal manner. A prospective observational study design would have been more appropriate.
As the study comprised 133 young adults identified with modest GV and prevalence of diabetic neuropathy there may not be enough power to extrapolate the results to the overall young population with type 1 diabetes. A larger sample size would have been beneficial in order to draw a more valid conclusion.
The aim of the study was to investigate the association between GV and early possible reversible neuropathy in a young cohort. Thus, conclusions on associations are limited to type 1 diabetes patients in the age-rage of the study cohort.
Novel and established methods of detecting DSPN and CAN (2, 35) were used which is a considerable strength in our study and may give a more detailed description of the nerve function.
CGM was used to assess GV as recommended (38) in order to detect periods of acute hypo- and hyperglycemia. However, the participants were only asked to wear the sensors for 5 days which may not be a sufficient duration to present a representable picture of daily fluctuations of blood glucose. Longer measuring durations may have given a more nuanced understanding.
It may be possible that more resourceful young adults chose to participate in the study after receiving the written invitation which could have caused selections bias.
It is recommended that participants avoid test confounders as smoking, use of several drugs, meals, and caffeine-containing liquids before testing for CAN (26). The participants were advised not to drink caffeine-containing liquids on the day before testing but the other recommendations were not met and may have affected CAN measures (4).
Conclusion
After adjusting for relevant risk factors and multiple tests, no significant associations were found between GV and diabetic neuropathy in a cohort of young adults with type 1 diabetes. This finding is in line with some of the previous studies which have failed to provide consistent evidence that GV is a risk factor of development of CAN and DSPN.
This suggests that GV may not be a risk factor for early diabetic neuropathy in young adults with type 1 diabetes. However, the cross-sectional study approach including a relatively small sample size of young participants with modest GV and diabetic neuropathy make a strong conclusion difficult. Moreover, long-term effects of GV excursions may still play a role in the pathogenic mechanisms leading to neuropathy in later life. Increasing levels of HbA1c were significantly associated with both measures of DSPN and CAN which support earlier findings of high levels of HbA1c being an established and essential risk factor of diabetic neuropathy.
Previous studies addressing the aim of the present project have assessed GV and DSPN and CAN by heterogenic measuring modalities hampering comparability. To improve comparability there is a need for studies using recommended measures of GV and diabetic neuropathy. Furthermore, more studies on young adults with type 1 diabetes are needed to confirm our findings.
Data Availability Statement
The raw data supporting the conclusions of this article will be made available by the authors, without undue reservation.
Ethics Statement
The studies involving human participants were reviewed and approved by The Danish Research Ethics Committee (project id.: H-15006967). The patients/participants provided their written informed consent to participate in this study.
Author Contributions
MC has contributed to the design of the study, acquired, analyzed, interpreted data, drafted the article, and approved the final version to be published. EH, MJ, and JF has contributed to the design of the study, analyzed, interpreted data, revised the article critically, and approved the final version to be published. CH has contributed to the design of the study, acquisition, analysis, interpretation of data, revised the article critically, and approved the final version to be published. All authors contributed to the article and approved the submitted version.
Funding
Funding for this manuscript was provided by: The Augustinus Foundation, 15-2274 and The Toyota Foundation, BG 8687. The foundations were not involved in the conducted research or preparation of the article.
Conflict of Interest
JF holds stocks in Medicus Engineering.
The remaining authors declare that the research was conducted in the absence of any commercial or financial relationships that could be construed as a potential conflict of interest.
Acknowledgments
MC is the guarantor of this work and, as such had full access to all the data in the study and takes responsibility for the integrity of the data and the accuracy of the data analysis.
Supplementary Material
The Supplementary Material for this article can be found online at: https://www.frontiersin.org/articles/10.3389/fendo.2020.00644/full#supplementary-material
Abbreviations
BPI, Brief Pain Inventory; CAN, cardiovascular autonomic neuropathy; CARTs, cardiovascular autonomic reflex tests; CONGA, continuous overall net glycemic action; CSII, continuous subcutaneous insulin infusion; CV, coefficient of variation; DSPN, diabetic symmetric polyneuropathy; E:I, deep breathing test; ESC, electrochemical skin conductance; HF, high frequency; HRV, heart rate variability; IQR, interquartile range; LF, low frequency; LF/HF, ratio low-frequency power/high-frequency power ratio; MAGE, mean amplitude of glucose excursions; MDI, multiple dose injections; MNSI, Michigan Neuropathy Screening Instrument; RMSSD, root mean square of the sum of the squares of differences between consecutive R–R intervals; SDNN, standard deviation of normal-to-normal intervals; SNAP, sural nerve amplitude potential; SNCV, sural nerve conduction velocity; VM, Valsalva Maneuver; VPT, vibration perception threshold; 30:15, lying-to-standing test.
References
1. Kuehl M, Stevens MJ. Cardiovascular autonomic neuropathies as complications of diabetes mellitus. Nat Rev Endocrinol. (2012) 8:405–16. doi: 10.1038/nrendo.2012.21
2. Tesfaye S, Boulton AJ, Dyck PJ, Freeman R, Horowitz M, Kempler P, et al. Diabetic neuropathies: update on definitions, diagnostic criteria, estimation of severity, and treatments. Diabetes Care. (2010) 33:2285–93. doi: 10.2337/dc10-1303
3. Trotta D VA, Salladini C, Chiarelli F. Diabetic neuropathy in children and adolescents. Pediatric Diabetes. (2004) 5:44–57. doi: 10.1111/j.1399-543X.2004.00041.x
4. Christensen MMB, Hommel EE, Jorgensen ME, von Scholten BJ, Fleischer J, Hansen CS. Prevalence of diabetic neuropathy in young adults with type 1 diabetes and the association with insulin pump therapy. Diabetes Technol Ther. (2018) 20:787–96. doi: 10.1089/dia.2018.0249
5. Rojas J, Chávez-Castillo M, Torres W, Chávez C, Apruzzese V, Cabrera M, et al. Peripheral and autonomic neuropathy in an adolescent with type 1 diabetes mellitus: evidence of symptom reversibility after successful correction of hyperglycemia. J Res Diabetes. (2014) 2014:1–22. doi: 10.5171/2014.899900
6. Group TDCaCTR. The effect of intensive diabetes therapy on measures of autonomic nervous system function in the Diabetes Control and Complications Trial (DCCT). Diabetologia. (1998) 41:416–23. doi: 10.1007/s001250050924
7. Martin CL, Albers JW, Pop-Busui R, Group DER. Neuropathy and related findings in the diabetes control and complications trial/epidemiology of diabetes interventions and complications study. Diabetes Care. (2014) 37:31–8. doi: 10.2337/dc13-2114
8. Gibbons CH, Freeman R. Treatment-induced diabetic neuropathy: a reversible painful autonomic neuropathy. Ann Neurol. (2010) 67:534–41. doi: 10.1002/ana.21952
9. Burger AJ, Weinrauch LA, D'Elia JA, Aronson D. Effect of glycemic control on heart rate variability in type I diabetic patients with cardiac autonomic neuropathy. Am J Cardiol. (1999) 84:687–91. doi: 10.1016/S0002-9149(99)00417-8
10. Diabetes Control Complications Trial Research Group ND, Genuth S, Lachin J, Cleary P, Crofford O, Davis M, et al. The effect of intensive treatment of diabetes on the development and progression of long-term complications in insulin-dependent diabetes mellitus. N Engl J Med. (1993) 329:977–86. doi: 10.1056/NEJM199309303291401
11. American Diabetes A. Standards of medical care in diabetes−2014. Diabetes Care. (2014) 37(Suppl.1):S14–80. doi: 10.2337/dc14-S014
12. American Diabetes A. 6. Glycemic targets: standards of medical care in diabetes-2018. Diabetes Care. (2018) 41(Suppl.1):S55–64. doi: 10.2337/dc18-S006
13. Monnier LME, Ginet C, Michel F, Villon L, Cristol JP, Colette C. Activation of oxidative stress by acute glucose fluctuations compared with sustained chronic hyperglycemia in patients with type 2 diabetes. JAMA. (2006) 295:1681–7. doi: 10.1001/jama.295.14.1681
14. Kwai NC, Arnold R, Poynten AM, Krishnan AV. Association between glycemic variability and peripheral nerve dysfunction in type 1 diabetes. Muscle Nerve. (2016) 54:967–9. doi: 10.1002/mus.25274
15. Virk SA, Donaghue KC, Cho YH, Benitez-Aguirre P, Hing S, Pryke A, et al. Association between HbA1c variability and risk of microvascular complications in adolescents with type 1 diabetes. J Clin Endocrinol Metab. (2016) 101:3257–63. doi: 10.1210/jc.2015-3604
16. Jaiswal M, McKeon K, Comment N, Henderson J, Swanson S, Plunkett C, et al. Association between impaired cardiovascular autonomic function and hypoglycemia in patients with type 1 diabetes. Diabetes Care. (2014) 37:2616–21. doi: 10.2337/dc14-0445
17. Lachin JM BI, Bergenstal RM, Pop-Busui R, Service FJ, Zinman B, Nathan DM. Association of glycemic variability in type 1 diabetes with progression of microvascular outcomes in the diabetes control and complications trial. Diabetes Care. (2017) 40:777–83. doi: 10.2337/dc16-2426
18. Nyiraty S, Pesei F, Orosz A, Coluzzi S, Vagi OE, Lengyel C, et al. Cardiovascular autonomic neuropathy and glucose variability in patients with type 1 diabetes: is there an association? Front Endocrinol. (2018) 9:174. doi: 10.3389/fendo.2018.00174
19. Akaza M, Akaza I, Kanouchi T, Sasano T, Sumi Y, Yokota T. Nerve conduction study of the association between glycemic variability and diabetes neuropathy. Diabetol Metab Syndr. (2018) 10:69. doi: 10.1186/s13098-018-0371-0
20. Di Flaviani A, Picconi F, Di Stefano P, Giordani I, Malandrucco I, Maggio P, et al. Impact of glycemic and blood pressure variability on surrogate measures of cardiovascular outcomes in type 2 diabetic patients. Diabetes Care. (2011) 34:1605–9. doi: 10.2337/dc11-0034
21. Su JB, Zhao LH, Zhang XL, Cai HL, Huang HY, Xu F, et al. HbA1c variability and diabetic peripheral neuropathy in type 2 diabetic patients. Cardiovasc Diabetol. (2018) 17:47. doi: 10.1186/s12933-018-0693-0
22. Lai YR, Chiu WC, Huang CC, Tsai NW, Wang HC, Lin WC, et al. HbA1C variability is strongly associated with the severity of peripheral neuropathy in patients with type 2 diabetes. Front Neurosci. (2019) 13:90. doi: 10.3389/fnins.2019.00090
23. Jun JE, Jin SM, Baek J, Oh S, Hur KY, Lee MS, et al. The association between glycemic variability and diabetic cardiovascular autonomic neuropathy in patients with type 2 diabetes. Cardiovasc Diabetol. (2015) 14:70. doi: 10.1186/s12933-015-0233-0
24. Xu F SJ, Chen T, Wang XQ, Chen J, Wu G, Jin Y, et al. The relationship between glycemic variability and diabetic peripheral neuropathy in type 2 diabetes with well-controlled HbA1c. Diabetol Metabolic Syndrome. (2014) 6:139. doi: 10.1186/1758-5996-6-139
25. Cardoso CRL, Leite NC, Moram CBM, Salles GF. Long-term visit-to-visit glycemic variability as predictor of micro- and macrovascular complications in patients with type 2 diabetes: The Rio de Janeiro type 2 diabetes cohort study. Cardiovasc Diabetol. (2018) 17:33. doi: 10.1186/s12933-018-0677-0
26. Spallone V, Ziegler D, Freeman R, Bernardi L, Frontoni S, Pop-Busui R, et al. Cardiovascular autonomic neuropathy in diabetes: clinical impact, assessment, diagnosis, and management. Diabetes Metab Res Rev. (2011) 27:639–53. doi: 10.1002/dmrr.1239
27. Boulton AJ, Armstrong DG, Albert SF, Frykberg RG, Hellman R, Kirkman MS, et al. Comprehensive foot examination and risk assessment: a report of the task force of the foot care interest group of the American Diabetes Association, with endorsement by the American Association of Clinical Endocrinologists. Diabetes Care. (2008) 31:1679–85. doi: 10.2337/dc08-9021
28. Pop-Busui R, Boulton AJ, Feldman EL, Bril V, Freeman R, Malik RA, et al. Diabetic neuropathy: a position statement by the american diabetes association. Diabetes Care. (2017) 40:136–54. doi: 10.2337/dc16-2042
29. Donaghue KC, Bonney M, Simpson JM, Schwingshandl J, Fung ATW, Howard NJ. Autonomic and peripheral nerve function in adolescents with and without diabetes. Diabet Med. (1993) 10:664–71. doi: 10.1111/j.1464-5491.1993.tb00142.x
30. Selvarajah D, Cash T, Davies J, Sankar A, Rao G, Grieg M, et al. SUDOSCAN: a simple, rapid, and objective method with potential for screening for diabetic peripheral neuropathy. PLoS ONE. (2015) 10:e0138224. doi: 10.1371/journal.pone.0138224
31. Vinik AI, Smith AG, Singleton JR, Callaghan B, Freedman BI, Tuomilehto J, et al. Normative values for electrochemical skin conductances and impact of ethnicity on quantitative assessment of sudomotor function. Diabetes Technol Ther. (2016) 18:391–8. doi: 10.1089/dia.2015.0396
32. Lee JA, Halpern EM, Lovblom LE, Yeung E, Bril V, Perkins BA. Reliability and validity of a point-of-care sural nerve conduction device for identification of diabetic neuropathy. PLoS ONE. (2014) 9:e86515. doi: 10.1371/journal.pone.0086515
33. Neurometrix. NC-stat DPNcheck Normative Database: Collection, Analysis and Recommended Normal Limits. (2013).
34. Electrophysiology TFotESoCatNASoPa. Heart rate variability: standards of measurement, physiological interpretation and clinical use. Eur Heart J. (1996) 17:354–81.
35. Spallone V, Bellavere F, Scionti L, Maule S, Quadri R, Bax G, et al. Recommendations for the use of cardiovascular tests in diagnosing diabetic autonomic neuropathy. Nutr Metab Cardiovasc Dis. (2011) 21:69–78. doi: 10.1016/j.numecd.2010.07.005
36. Hansen CS, Jensen TM, Jensen JS, Nawroth P, Fleming T, Witte DR, et al. The role of serum methylglyoxal on diabetic peripheral and cardiovascular autonomic neuropathy: the ADDITION Denmark study. Diabet Med. (2015) 32:778–85. doi: 10.1111/dme.12753
37. Feldman EL, Stevens MJ, Thomas PK, Brown MB, Canal N, Greene DA. A practical two-step quantitative clinical and electrophysiological assessment for the diagnosis and staging of diabetic neuropathy. Diabetes Care. (1994) 17:1281–9. doi: 10.2337/diacare.17.11.1281
38. Danne TNR, Battelino T, Bergenstal RM, Close KL, DeVries JH, Garg S, et al. International consensus on use of continuous glucose monitoring. Diabetes Care. (2017) 40:1631–40. doi: 10.2337/dc17-1600
39. Levey AS, Stevens LA, Schmid CH, Zhang YL, Castro AF, Feldman HI, et al. A new equation to estimate glomerular filtration rate. Ann Intern Med. (2011) 155:408. doi: 10.7326/0003-4819-155-6-201109200-00024
40. Benjamini YHY. Controlling the false discovery rate: a practical and powerful approach to multiple testing. J Royal Statist Soc Ser B. (1995) 57:289–300. doi: 10.1111/j.2517-6161.1995.tb02031.x
41. Group TDCaCTR. The relationship of glycemic exposure (HbA1c) to the risk of development and progression of retinopathy in the diabetes control and complications trial. Diabetes. (1995) 44:968–83. doi: 10.2337/diabetes.44.8.968
Keywords: type 1 diabetes, glycemic variability, young adults, cardiovascular autonomic neuropathy, distal symmetric polyneuropathy, continuous glucose monitoring
Citation: Christensen MMB, Hommel EE, Jørgensen ME, Fleischer J and Hansen CS (2020) Glycemic Variability and Diabetic Neuropathy in Young Adults With Type 1 Diabetes. Front. Endocrinol. 11:644. doi: 10.3389/fendo.2020.00644
Received: 21 April 2020; Accepted: 07 August 2020;
Published: 23 September 2020.
Edited by:
Erwin Dieter Schleicher, University of Tübingen, GermanyReviewed by:
Abdurezak Ahmed Abdela, Addis Ababa University, EthiopiaJean-Henri Calvet, Impeto Medical, Inc., United States
Copyright © 2020 Christensen, Hommel, Jørgensen, Fleischer and Hansen. This is an open-access article distributed under the terms of the Creative Commons Attribution License (CC BY). The use, distribution or reproduction in other forums is permitted, provided the original author(s) and the copyright owner(s) are credited and that the original publication in this journal is cited, in accordance with accepted academic practice. No use, distribution or reproduction is permitted which does not comply with these terms.
*Correspondence: Marie Mathilde Bjerg Christensen, marie.mathilde.bjerg.christensen.01@regionh.dk
†ORCID: Marie Mathilde Bjerg Christensen orcid.org/0000-0002-7055-6835