- 1Department of Experimental Medicine, University of Perugia, Perugia, Italy
- 2Research Unit on Human Reproduction, International Scientific Institute Paul VI, Rome, Italy
- 3Division of Endocrinology, Fondazione Policlinico Universitario “Agostino Gemelli”, Rome, Italy
- 4Molecular Biology of Reproduction and Development Research Group, Institut d'Investigacions Biomèdiques August Pi i Sunyer (IDIBAPS), Department of Biomedical Sciences, Faculty of Medicine and Health Sciences, University of Barcelona, Barcelona, Spain
- 5Biochemistry and Molecular Genetics Service, Hospital Clínic, Barcelona, Spain
- 6Department of Medicine, University of Perugia, Perugia, Italy
- 7Biochemistry and Clinical Biochemistry Institute, School of Medicine, Catholic University of Rome, Rome, Italy
- 8Department of Laboratory Diagnostic and Infectious Diseases, Fondazione Policlinico “A. Gemelli” IRCCS, Rome, Italy
- 9Division of Medical Andrology and Endocrinology of Reproduction, University of Perugia and Saint Mary Hospital, Terni, Italy
Follicle-stimulating hormone (FSH), a major regulator of spermatogenesis, has a crucial function in the development and function of the testis and it is extensively given as a fertility treatment to stimulate spermatogenesis. We analyzed the effects of different FSH preparations (α-follitropin, β-follitropin, and urofollitropin) in combination with testosterone on porcine pre-pubertal Sertoli cells. To study the effect of the different FSH treatments in the Sertoli cell function we performed Real Time PCR analysis of AMH, inhibin B, and FSH-r, an ELISA assay for AMH and inhibin B, and a high-throughput comparative proteomic analysis. We verified that all three preparations induced a reduction of AMH in terms of mRNA and secreted proteins, and an increase of inhibin B in terms of mRNA in all the FSH formulations, while solely α-follitropin produced an increase of secreted inhibin B in the culture medium. Comparative proteomic analysis of the three FSH preparations identified 46 proteins, 11 up-regulated and 2 down-regulated. Surprisingly, the combination of testosterone with β-follitropin specifically induced an up-regulation of eight specific secreted proteins. Our study, showing that the three different FSH preparations induce different effects, could offer the opportunity to shed light inside new applications to a personalized reproductive medicine.
Introduction
Follicle-stimulating hormone (FSH), a glycoprotein hormone secreted by the anterior pituitary gland, plays a key function in the treatment of human infertility. In infertile women it is widely prescribed to stimulate follicular development, meanwhile, in males, it is used alone or in association with Human chorionic gonadotropin (hCG) to trigger off and maintain spermatogenesis both in hypogonadotropic hypogonadism (1), and in oligozoospermic subjects with normogonadotropic hypogonadism (2).
FSH comprises two subunits, α and β, which are both glycosylated and contain four N-linked carbohydrates. The different content in sialic acid at the C-terminal determines a family of glycoforms that explain the structural and functional heterogeneity of the different FSH formulations (3).
The preparations of FSH available in the market are derived by either recombinant DNA technology (rFSH such as α- and β-follitropin) or post-menopausal urines (urofollitropin). α- and β-follitropins are synthesized by the same recombinant technology, producing identical dimeric α-FSH and β-FSH subunits, but with differences in the further glycosylation and in the procedures of purification. In contrast, urofollitropin consists of FSH with a minimal LH activity, and it has low specific activity (~100–150 IU FSH/mg protein). The low specific activity of this preparation could be explained by the fact that more than 95% of the protein content correspond to non-specific co-purified urinary proteins (1).
Regarding the efficacy of the different FSH preparations in the female, many contradictory results have been published in the last 2 decades. The meta-analyses regarding the clinical efficacy of different FSH preparations demonstrated no significant differences in clinical or ongoing pregnancy and in the live-birth rate, in the miscarriage rate, or for the incidence of multiple pregnancy rate or ovarian hyperstimulation syndrome (OHSS) between rFSH and urofollitropin (4–8).
Up to now, in the male, no data exist regarding the efficacy of the treatment in relation to the FSH-therapy used. However, a meta-analysis reported a significant positive effect of the treatment with FSH both on sperm parameters and on pregnancy rate in oligozoospermic patients with normal FSH levels (9). Unfortunately, the studies included in the meta-analyses have an extremely heterogeneity in the selection criteria of the patients, in primary and secondary end-points, in the doses of FSH treatment and in time of treatment (10).
Sertoli cell (SC) is one of the principal actors in spermatogenesis as it provides nourishment, and structural and functional support to germ cells. Moreover, it protects germ cells by the blood-testis barrier (BTB) and by the production of immunomodulatory factors (11). In testis, FSH controls the function of SC through FSH receptors (FSH-r), which are only present in SC. In particular, FSH plays a pivotal role in the early stages of spermatogenesis, while testosterone has a major role in spermiogenesis (12).
In the prepubertal testis, SC is the most representative cell population. However, during this stage, there is a low activity of the hypothalamic–pituitary–gonadal axis reflected by the high levels of Anti-Müllerian Hormone (AMH) and inhibin B in serum (13). In contrast, during puberty, testosterone induces SC maturation and inhibits AMH production (13).
The aim of this research was to assess the effects of the different FSH formulation in an “in vitro” model of porcine pre-pubertal SC, in order to evaluate the SC responsiveness to pharmacological treatment of different FSH preparations, never assessed until now.
Materials and Methods
SC Culture, Characterization, and Stimulation
Pure porcine pre-pubertal SC were isolated and characterized according to previously reported methods (14–16).
Purified SC cultures, as previously stated (14–16), were treated for 48 h as follows, and stimulation was performed according to the previously described protocol (17):
• Stimulated with testosterone (0.2 μg/ml; SIT, Pavia, Italy) (control group);
• Stimulated with α-follitropin (α-FSH) 100 nM and testosterone (0.2 μg/ml);
• Stimulated with β-follitropin (β-FSH) 100 nM and testosterone (0.2 μg/ml);
• Stimulated with Urofollitropin (u-FSH) 100 nM and testosterone (0.2 μg/ml).
We used testosterone in addition to any of the FSH formulation to mimic a physiological condition in testis, considering that both FSH and testosterone are essential for the adequate spermatogenesis (12).
Quantitative, Real-Time PCR
Analyses for AMH, inhibin B, and FSH receptor (FSH-r) were performed as previously described (17) employing the primers listed in Table 1. Total RNA was extracted from SC monolayers obtained in the experimental groups using Trizol reagent (Sigma-Aldrich, Milan, Italy), and quantified by reading the optical density at 260 nm. In detail, 2.5 μg of total RNA was subjected to reverse transcription (RT, Thermo Scientific, Waltham, MA, USA) to a final volume of 20 μl. We performed the qPCR with the use of 25 ng of the cDNA obtained by RT and a SYBR Green Master Mix (Stratagene, Amsterdam, The Netherlands). This procedure was performed in an Mx3000P cycler (Stratagene), using FAM for detection and ROX as the reference dye. We normalized the mRNA level of each sample against β-actin mRNA and expressed it as fold changes vs. the levels in the control group.
Culture Media Isolation
Aliquots of the culture media (CM) of SC were collected after 48 h of stimulation, centrifuged at 1,500 g for 10 min, and the supernatant was saved at −20°C for proteomic analysis and for an ELISA assay for Inhibin B and AMH secretion performed as previously described (18).
Proteomic Analysis of the SC Secretome
The SC CM for each sample was thawed and centrifuged at 3,000 g for 20 min at 4°C. The resulting supernatants were filtered (0.45 μm pore size) to remove cell debris and other impurities if any. Afterward, to perform protein solubilization, sodium dodecyl sulfate (SDS) and phenylmethylsulfonyl fluoride (PMSF) were added to a final concentration of 2% (w/v) and 1 mM, respectively. After 30 min, all samples were centrifuged at 4°C at 16,000 g for 10 min, and the supernatants (soluble proteins) were kept, and proteins were precipitated overnight in 80% cold acetone (v/v) at −20°C. After centrifugation at 17,530 g for 15 min at 4°C, the protein precipitates were collected and resuspended in 1% SDS, 1 mM PMSF in phosphate-buffered saline (PBS) to perform protein quantification using the BCA protein Assay Kit (Pierce™ BCA protein Assay Kit, Thermo Fisher Scientific, Rockford, IL), according to manufacturer's recommendations.
The TMT labeling was performed as described previously (19, 20) and according to the manufacturer's instructions. Briefly, 50 μg of proteins from each sample were transferred to a new tube and adjusted to a final volume of 50 μl with 100 mM triethylammonium bicarbonate (TEAB) to obtain a 1 μg/μl concentration. Proteins were reduced in 9.5 mM tris (2-carboxyethyl) phosphine (TCEP), alkylated with 17 mM iodoacetamide (IAA), and precipitated by adding six volumes of 100% cold acetone. Then, samples were centrifuged at 17,500 g, and the acetone-precipitated protein pellets (containing 50 μg of proteins) were resuspended in 50 μl of 100 mM TEAB. Trypsin was added at a 1:22 protease-to-protein ratio and incubated overnight at 37°C. Prior to peptide labeling, an aliquot from each sample was taken and combined at equal amounts to form the internal control. After, 35 μg of peptides from each sample (including the internal control) were labeled with TMT isobaric tags (TMT 10-plex Mass Tag Labeling; Thermo Fisher Scientific, Rockford, IL). The technical reproducibility and analytical reliability of the approach were assessed by performing duplicate analyses on the internal control. Then, 15 μl of the TMT label reagents, previously resuspended in acetonitrile anhydrous (ACN), were added to the corresponding sample, followed by 1 h incubation at RT. Afterward, the reaction was stopped by adding 5% hydroxylamine. The TMT-labeled samples were combined at equal amounts constituting one multiplex pool, which was dried in a vacuum centrifuge and resuspended in 50 μl of 0.5% trifluoroacetic acid in 5% ACN. After, labeled peptides were cleaned up via reversed-phase C18 spin columns (Pierce C18 Spin Columns, Thermo Fisher Scientific, Rockford, IL), according to manufacturer's instructions. Then, the peptides were reconstituted in 0.1% formic acid (FA) to be processed by LC-MS/MS.
Our MS data was collected using a nano-LC Ultra 2D Eksigent (AB Sciex, Brugg, Switzerland) attached to an LTQ-Orbitrap Velos (Thermo scientific, San Jose, CA). Peptides were injected onto a C18 trap column (L 2 cm, 100 μm ID, 5 μm, 120 Å; NanoSeparations, Nieuwkoop, the Netherlands) and chromatographic analyses were performed using an analytical column (L 15 cm, 75 μm ID, 3 μm, 100 Å; Thermo scientific, San Jose, CA). The buffers used for the analysis were buffer A (97% H2O-3% ACN, 0.1% FA) and buffer B (3% H2O-97% ACN, 0.1% FA). A peptide mixture was loaded onto the analytical column with the following gradient: time 0–5 min, 0% of B; 5–180 min, 0–32.5% of B; 180–185 min, 32.5–100% of B at a flow rate of 400 ml/min; and 185–200 min, 100% of B at a flow rate of 400 nl/min to avoid carry-over. MS/MS analyses were performed using an LTQ-Orbitrap Velos (Thermo Fisher Scientific, Waltham, MA) with a nanoelectrospray ion source. The LTQ-Orbitrap Velos settings included one 30,000 resolution at 400 m/z MS1 scan for precursor ions followed by MS2 scans of the 15 most intense precursor ions, at 30,000 resolution at 400 m/z, in positive ion mode. The lock mass option was enabled, and mass calibration was performed on polysiloxane (m/z 445.12003). MS/MS data acquisition was completed using Xcalibur 2.1 (Thermo Fisher Scientific, Waltham, MA). MS2 experiments were performed using higher-energy collision dissociation (HCD) with a normalized collision energy of 42%.
LC-MS/MS data was analyzed using Proteome Discoverer 1.4.1.14 (Thermo Fisher Scientific, Waltham, MA, USA) based on SEQUEST HT cluster as search engine (University of Washington, licensed to Thermo Electron Corp., San Jose, CA) against UniProtKB/Swiss-Prot database with Sus scrofa (released September 2018; 3,339 sequences). Searches were run applying the following parameters: two maximum missed cleavage sites for trypsin, TMT-labeled lysine (+229.163 Da) and methionine oxidation (+15.995 Da) as dynamic modifications, cysteine carbamidomethylation (+57.021 Da) as a static modification, 20 ppm precursor mass tolerance, 0.6 Da fragment mass tolerance. Percolator was used for protein identification, applying the following identification criteria: at least one unique peptide per protein and a FDR of 1%. The reporter ion intensities were corrected according to the isotopic purities provided by the manufacturer.
For protein quantification purposes, only unique peptides were used, and protein ratios (i.e., TMT-127/TMT-126) were normalized to protein median. The cut-off for up-regulated proteins was ≥1.500, and for down-regulated proteins was ≤ 0.667 as previously reported (21).
Statistical Analysis
Values reported in the figures are the mean ± S.D. of three independent experiments, each one performed in triplicate. Statistical analysis was performed by the paired Student's t-test using SigmaStat 4.0 software (Systat Software Inc., CA, USA). All tests were performed in triplicate, and statistically significance was assigned for p < 0.05.
Results
Purification and Characterization of SC
The isolated SC culture was 95% pure as indicated by immunostaining for AMH (Figure 1a) with an extremely low percentage of non-SC cells (<5%) characterized by immunostaining for insulin-like 3-positive (Leydig) cells (INSL-3) (Figure 1b), alpha-smooth muscle actin positive (peritubular myoid) cells (ASMA) (Figure 1c) and protein gene product 9.5-positive (gonocytes and spermatogonial) cells (PGP9.5) (Figure 1d).
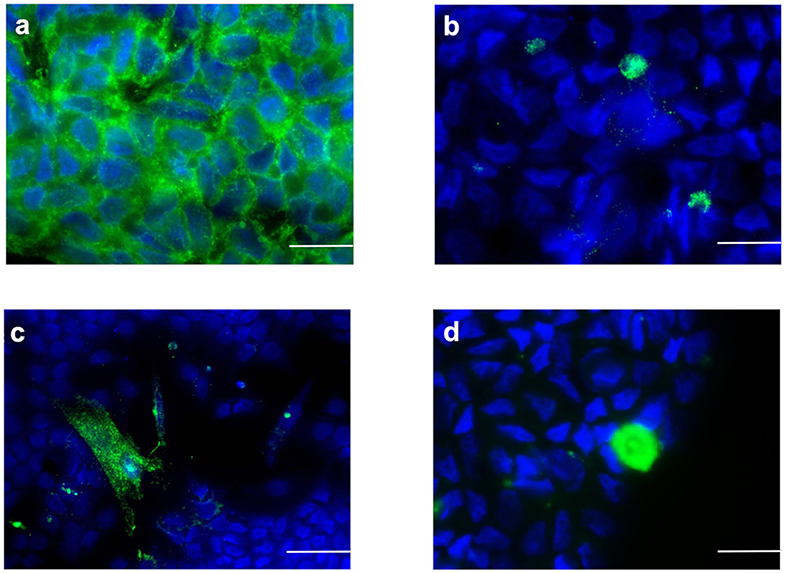
Figure 1. Sertoli cells characterization by immunofluorescence staining (green color). Sertoli cell monolayers were characterized by the expression of (a) AMH, (b) INSL3, (c) ASMA, and (d) (PGP9.5). Nuclei are labeled with DAPI in blue color. Bars = 20 μm.
Inhibin B, AMH, and FSH-r Gene Expression in SC
AMH gene expression in SC was significantly down-regulated by treatment with α-, β-follitropin, and urofollitropin in combination with testosterone treatment compared with testosterone alone (Figure 2A, p < 0.001).
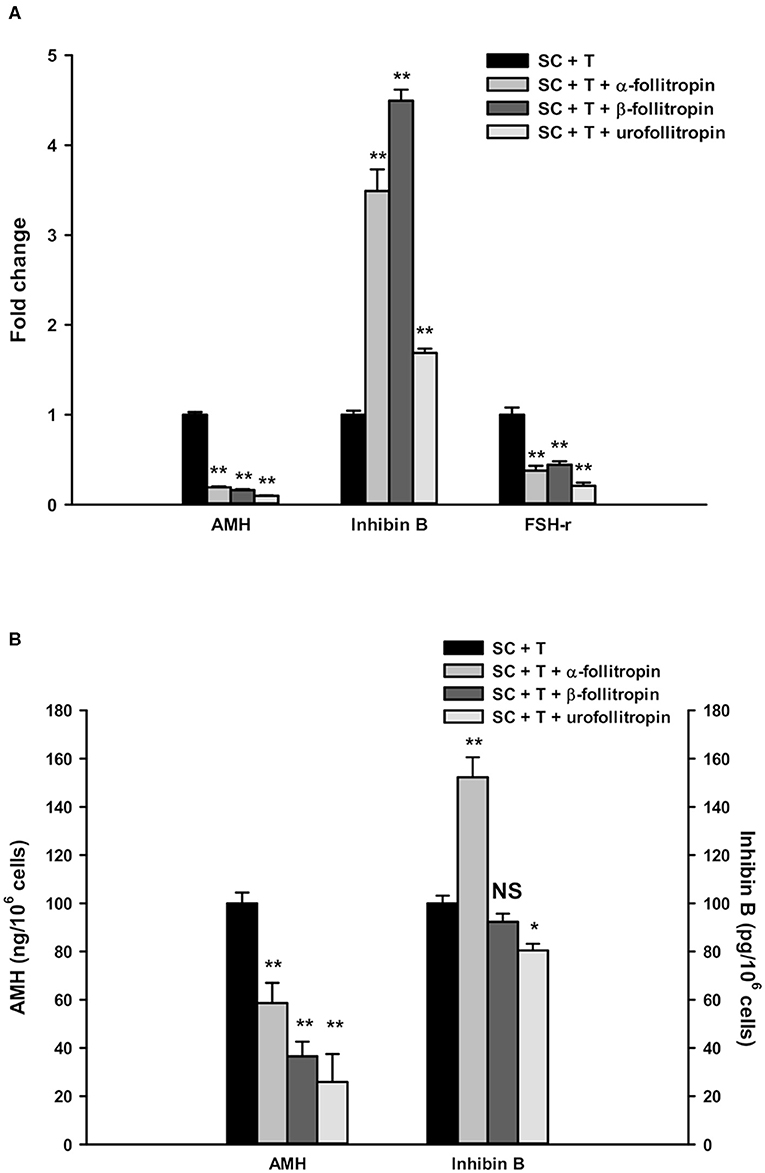
Figure 2. Real-time PCR analysis and ELISA assays. (A) Gene expression in SC of AMH, inhibin B, and FSH-r. (B) AMH and inhibin B secretion in SC culture medium. Data represent the mean ± S.D. (*p < 0.05, **p < 0.001) of three independent experiments performed in triplicate.
In contrast, inhibin B expression was significantly increased after treatment with α-, β-follitropin, and urofollitropin, each other combined with testosterone treatment compared with testosterone alone (Figure 2A, p < 0.001).
Moreover, we found a statistically significant reduction of FSH-r upon all three FSH preparations plus testosterone compared with testosterone alone (Figure 2A, p < 0.001).
Inhibin B and AMH Secretion Assay
The secretion of AMH was significantly down-regulated by α-, β-follitropin, and urofollitropin plus testosterone treatments compared with testosterone alone, consistent with the results of gene expression showed above (Figure 2B, p < 0.001).
Meanwhile, inhibin B was significantly increased in culture medium only after exposure to α-follitropin plus testosterone (Figure 2B, p < 0.001), while no changes were observed after β-follitropin plus testosterone stimulation. Interestingly, we observed a significant reduction of inhibin B after the stimulation with urofollitropin plus testosterone compared with testosterone alone (Figure 2B, p < 0.05).
Secretomic Protein Profiling
In order to evaluate the differences induced by the different FSH preparations on SC secretomic profiles, we performed a comparative proteomic analysis of the SC culture media in the groups treated with testosterone associated with α-, β-follitropin, and urofollitropin, and we compared it with the group of the testosterone treatment alone.
The proteomic analysis resulted in the identification of 46 TMT-labeled proteins in all the SC culture media proteomes (Table 2). Of those, 13 proteins were detected in a significantly altered abundance (Table 3). Specifically, 11 proteins were observed as up-regulated (Table 3; cut-off ≥ 1.500) and 2 proteins as down-regulated (Table 3; cut-off ≤ 0.667) by the different FSH preparations. All the different FSH preparations showed a down-regulation of the secreted SPARC protein and an up-regulation of 3 proteins (PLAT, INHBA, and TPI1, Table 3).
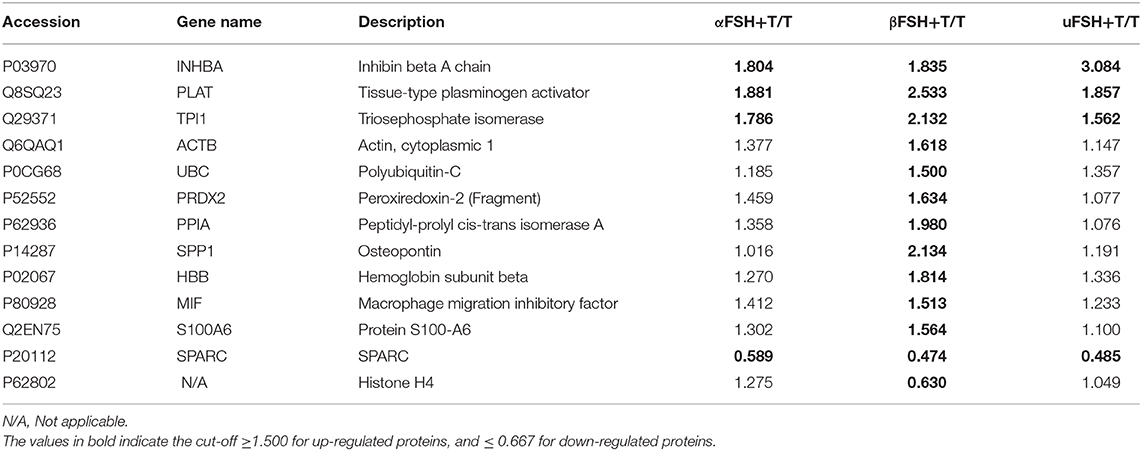
Table 3. List of the up- and down-regulated proteins in Sertoli cell medium after stimulation with the different FSH preparations plus testosterone, compared with testosterone treatment alone.
Interestingly, an up-regulation of 8 additional secreted proteins (ACTB, UBC, PRDX2, PPIA, SPP1, HBB, MIF, and S100A6) and a down-regulation of Histone H4 were specifically observed after the use of β-FSH with T, as reported in Table 3.
Discussion
In the present work, we have focused on the “in vitro” effect of different FSH preparations on pre-pubertal porcine SC culture, evaluating the modulation of specific markers determined through different approaches. All the FSH preparations assessed in the current study, α-, β-follitropin, and urofollitropin, induced a significant and similar response in terms of down-regulation of both AMH gene expression and AMH secretion, up-regulation of inhibin B gene expression and down-regulation of the expression of FSH-r gene expression. AMH is a glycoprotein dimeric hormone, a member of the transforming growth factor β (TGF-β) family, that plays a pivotal function in fetal sex differentiation, being involved in the regression of the Müllerian ducts (22). In the male, SC secretes high amounts of AMH from fetal life until the onset of puberty. AMH is exclusively secreted by SC and, for this reason, it is widely considered an important marker of the testicular function during the pre-pubertal life (22). We observed that testosterone alone and in combination with the three FSH preparations induced a significant down-regulation in AMH mRNA expression and secretion. As expected, we also demonstrated a statistically significant reduction in FSH-r expression independently of the FSH preparation. These data are in accordance with literature reporting how the interaction of the hormone with its receptor leads to the down-regulation of FSH-r mRNA expression by a cAMP-dependent post-transcriptional mechanism (23).
Another specific and important marker of SC functionality is inhibin B, which provides for a negative feedback on FSH secretion. In particular, serum inhibin B concentration is high during early postnatal life, and then gradually is reduced to a detectable plateau-level until its increase at the beginning of puberty (12). The assay of inhibin B is used in clinical practice to evaluate the presence and function of SC during childhood. Additionally, in adult life, the inhibin B levels depend on the presence of germ cells thus reflecting the efficiency of spermatogenesis (24). Our results demonstrated that all three FSH preparations plus testosterone significantly induced an up-regulation in the levels of inhibin B mRNA, confirming the role of FSH in inducing the transcription of inhibin B gene.
Our SC secretomic analysis uncovered a similar response after stimulation of SC with the different FSH preparations. On the one hand, we demonstrated the reduction levels of secreted SPARC protein after stimulation of SC with the different FSH preparations. SPARC, also known as osteonectin or BM-40, is a multifunctional protein that can modulate cell shape, proliferation, differentiation, and migration (25). SPARC has been found to interact with structural matrix proteins and may act to mediate their interactions with cells (24–28). In addition, SPARC can regulate the activity of several signaling molecules, either by direct interaction or by interfering with their signaling pathways (29–31). In our scenario, it is known that SPARC is produced by Leydig and Sertoli cells (32, 33), and that it is internalized in Sertoli, Leydig, and germ cells (34), playing a paracrine regulatory role during fetal testis development. Furthermore, the expression of SPARC in SC bearing late-stage elongate spermatids might suggest a role in the spermiation of elongated spermatids (33). Future studies will comprehensively define the function of SPARC in Sertoli-germ cell interaction and spermiogenesis. Here we provide for the first time information about the down-regulation of SPARC secretion by FSH.
On the other hand, the proteomic analysis also showed that tissue-type plasminogen activator (PLAT), triosephosphate isomerase (TPI1), and inhibin beta A chain (INHBA) proteins were up-regulated by the stimulation with α-, β-follitropin, and urofollitropin in presence of normal androgen milieu. Specifically, the inhibin beta A chain was observed as upregulated in proteomics by all the FSH preparations, and the higher increase was observed for urofollitropin. Despite this evidence, only α-follitropin stimulation induced a significative increase in the inhibin B levels in the medium, as documented by ELISA. INHBA is a subunit of both activin B and inhibin B (24). In the testis, it has been postulated that activin B acts as an autocrine and paracrine regulator of spermatogenesis (35, 36), modulating the proliferation in the testis of germ cells and SC (37). We might speculate that β-follitropin and, especially, urofollitropin, induce the activation of the INHB gene and the production of INHB, but do not increase the levels of INHB in the medium since they might increase the levels of activin B instead of inhibin B. Further studies are so needed to understand how the different FSH preparations modify the inhibin B and activin B balance.
The remaining two up-regulated proteins have been previously associated with SC. For example, previous studies demonstrated low levels of PLAT activity in cultured SC under basal conditions, whereas FSH stimulation induces PLAT (38, 39). Our results through a quantitative in vitro secretomic approach support the increased secretion of PLAT after FSH treatment independently of the FSH preparation. Interestingly, the plasminogen activator system acts in the process of spermiation (40), the detachment of residual bodies from the mature spermatids (41), and the residual body phagocytosis by SC (42). Triosephosphate isomerase has moreover been previously reported to be expressed in SC (43). An increased TPI1 expression in SC may influence the early activities of spermatogenesis, such as mitosis or initiation of meiosis, by spermatogonia or pre-leptotene spermatocytes, respectively (43).
Surprisingly, the combination of β-follitropin with testosterone revealed specific effects in the SC function besides the aforementioned similar effect of all tested FSH treatments. Specifically, the levels of eight additional proteins were up-regulated, which were: Actin (ACTB), Polyubiquitin-C (UBC), Peroxiredoxin-2 (PRDX2), Peptidyl-prolyl cis-trans isomerase A (PPIA), Osteopontin (SPP1), Hemoglobin subunit beta (HBB), Macrophage migration inhibitory factor (MIF), and Protein S100-A6 (S100A6); and just one protein, Histone H4, was down-regulated.
It is important to underline that some of these proteins might have a pivotal role both in the germ cell migration and in the cell-to-cell contact at the blood-testis barrier (BTB). For example, actin filament bundles have been described in specific Sertoli cell regions that are adjoining to tight junctions and to the sites of adhesion to spermatogenic cells (44). During spermatogenesis, these actin bundles undergo organizational changes, which might play a role in changing the interrelationship between SC and germ cells by facilitating the movement of spermatogenic cells (44). Similarly, SPP1, synthesized by SC and germ cells, is involved in cell adhesion and migration (45), and MIF, produced by SC under basal conditions, induces the migration of spermatogonial cells (46). Also, we found up-regulation of PPIA, a protein highly expressed in SC that has been recognized as a crucial factor in BTB integrity and maintenance (47), as well as S100A6, a protein that promotes cell migration and influences cell junction of SC (48) that could be implied in the spermatogenesis and modulation of BTB.
Interestingly, β-follitropin also increased PRDX2, an antioxidant protein preferentially expressed in SC that might play a role in removing or regulating the intracellular levels of peroxides produced during metabolism (49, 50). Additional up-regulated proteins were HBB, a metalloprotein that acts as a scavenger balancing the level of carbon monoxide (CO) in testis (51), and UBC, which is required for normal spermatogenesis development (52).
In conclusion, α-, β-follitropin, and urofollitropin induced a similar response as expressed by the down-regulation of AMH gene expression and AMH secretion, and an up-regulation and down-regulation of inhibin B and FSH-r gene expression, respectively, thus exerting an interesting effect in inducing maturation of SC from a pre-pubertal to an adult phenotype. This data confirms that the FSH in presence of an androgenic milieu regulates the proliferation and functional maturation of Sertoli cell type (53). Moreover, all three FSH preparations induced a down-regulation of a spermiation related protein, the SPARC, supporting the role of FSH in the regulation of spermatogenesis. Nevertheless, there are some specific effects of each FSH preparation, which need consideration before their prescription to infertile males.
For instance, only α-follitropin, in association with testosterone, induced the secretion in the media of inhibin B. Since inhibin B secreted by Sertoli cells could serve as negative feedback control on the hypothalamic-pituitary system to decrease FSH release (54), we might suppose that α-follitropin could have an inhibitory effect on the hypothalamic/pituitary axis in vivo. If these preliminary data obtained in our in vitro model would be confirmed by further in vitro and in vivo studies, we might conclude that male secondary hypogonadism would represent the best indication for this treatment since it induces a good response in terms of Sertoli activation, and the increase in Inhibin B secretion does not have any clinical relevance. In contrast, the increase of Inhibin B secretion as a response to α-follitropin treatment might represent a problem for normogonadotropic infertile patients since it might inhibit physiological pituitary FSH secretion.
In the case of urofollitropin, it induces a similar secretomic profile but also an increase in the release of INHBA without increasing the levels of secreted inhibin B, suggestive of an increasing release of activin B. This panel of action might be useful for treating oligozoospermic patients with normal FSH secretion. Therefore, we might speculate that—if these preliminary data would be confirmed—urofollitropin might represent the best treatment option for patients with normogonadotropic hypogonadism, since it seems to induce an increase in the secreted proteins, including the A chain of inhibin B and, consequentially, activin B, without increasing the levels of inhibin B, which might interfere with pituitary FSH secretion.
Finally, β-FSH stimulation exhibits additional effect up-regulating specific proteins mainly related to spermatogenic cell migration and BTB maintenance. In this context, the stimulation of SC with β-follitropin exerts his effect in the modulation of additional proteins implicated in the last stage of spermiation and in the related antioxidant activity. Further studies are needed to confirm that β-follitropin treatment is the best treatment option for patients with a spermatogenic arrest at the spermatid stage, as suggested by these preliminary in vitro data.
This molecular and proteomic approach demonstrated how some molecular effects seem to have a specific signature depending on each FSH preparation. The different molecular responses could help to choose which of the different FSH preparations could be used for infertility treatment according to the different present physio-pathological conditions. We can argue that α-follitropin can find a specific clinical use in hypospermatogenesis due to hypogonadotropic stimulus or in inducing spermatogenesis in puberty; β-follitropin could be specifically indicated to improve spermiation or in case of spermatidic arrest; and, finally, urofollitropin could be useful in idiopathic infertility in normogonadotropic patients. In conclusion, we performed for the first time a comparative study on the effects of different preparations of FSH on in vitro porcine pre-pubertal SC model. In the landscape of a personalized medicine, this study opens a window on the different use of the FSH formulations in relation to various clinical therapeutic targets.
Data Availability Statement
All datasets presented in this study are included in the article/supplementary material.
Ethics Statement
The animal study was reviewed and approved by Italian Approved Animal Welfare Assurance (A-3143-01).
Author Contributions
All authors had critically revised and approved the final version of the manuscript. IA, GG, and FB designed and drafted the manuscript. The experimental procedures and data analysis were performed by GG, FB, CB, CL, MJ, MA, FV, and FManci. AP, RC, and RO gave experimental guidance. GL, FMancu, and DM revised the manuscript.
Funding
This work was supported by grants to RO from the Spanish Ministry of Economy and Competitiveness (Ministerio de Economía y Competividad: fondos FEDER ‘una manera de hacer Europa' PI13/00699, PI16/00346). FB is granted by Spanish Ministry of Education, Culture, and Sports (Ministerio de Educación, Cultura y Deporte para la Formación de Profesorado Universitario, FPU15/02306). MJ is supported by the Government of Catalonia (Generalitat de Catalunya, pla estratègic de recerca i innovació en salut, PERIS 2016-2020, SLT002/16/00337).
Conflict of Interest
The authors declare that the research was conducted in the absence of any commercial or financial relationships that could be construed as a potential conflict of interest.
The handling Editor declared a past co-authorship with the authors IA, CL, GL, FM, and RC.
Acknowledgments
The authors would like to thank Josep Maria Estanyol and María José Fidalgo from the Proteomics Unit of the Scientific and Technological Centers, Universitat de Barcelona (CCiTUB).
References
1. Recombinant Human FSH Study Group. Clinical assessment of recombinant human follicle-stimulating hormone in stimulating ovarian follicular development before in vitro fertilization. Fertil Steril. (1995) 63:77–86. doi: 10.1016/S0015-0282(16)57300-2
2. Foresta C, Selice R, Ferlin A, Garolla A. Recombinant FSH in the treatment of oligozoospermia. Expert Opin Biol Ther. (2009) 9:659–66. doi: 10.1517/14712590902932673
3. Ulloa-Aguirre A, Timossi C. Structure-function relationship of follicle-stimulating hormone and its receptor. Hum Reprod Update. (1998) 4:260–83. doi: 10.1093/humupd/4.3.260
4. Al-Inany H, Aboulghar MA, Mansour RT, Serour GI. Ovulation induction in the new millennium: recombinant follicle-stimulating hormone versus human menopausal gonadotropin. Gynecol Endocrinol. (2005) 20:161–9. doi: 10.1080/09513590400027232
5. European and Israeli Study Group on Highly Purified Menotropin versus Recombinant Follicle-Stimulating Hormone (EISG). Efficacy and safety of highly purified menotropin versus recombinant follicle-stimulating hormone in in vitro fertilization/intracytoplasmic. Fertil Steril. (2002) 78:520–8. doi: 10.1016/S0015-0282(02)03250-8
6. Al-Inany H, Aboulghar M, Mansour R, Serour G. Meta-analysis of recombinant versus urinary-derived FSH: an update. Hum Reprod. (2003) 18:305–13. doi: 10.1093/humrep/deg088
7. Coomarasamy A, Afnan M, Cheema D, van der Veen F, Bossuyt PM, van Wely M. Urinary hMG versus recombinant FSH for controlled ovarian hyperstimulation following an agonist long down-regulation protocol in IVF or ICSI treatment: a systematic review and meta-analysis. Hum Reprod. (2008) 23:310–15. doi: 10.1093/humrep/dem305
8. van Wely M, Kwan I, Burt AL, Thomas J, Vail A, Van der Veen F, et al. Recombinant versus urinary gonadotrophin for ovarian stimulation in assisted reproductive technology cycles. Cochrane Database Syst Rev. (2011) 16:CD005354. doi: 10.1002/14651858.CD005354.pub2
9. Attia AM, Abou-Setta AM, Al-Inany HG. Gonadotrophins for idiopathic male factor subfertility. Cochrane Database Syst Rev. (2013) 23:CD005071. doi: 10.1002/14651858.CD005071.pub4
10. Barbonetti A, Calogero AE, Balercia G, Garolla A, Krausz C, La Vignera S, et al. The use of follicle stimulating hormone (FSH) for the treatment of the infertile man: position statement from the Italian Society of Andrology and Sexual Medicine (SIAMS). J Endocrinol Invest. (2018) 41:1107–22. doi: 10.1007/s40618-018-0843-y
11. Kaur G, Thompson LA, Dufour JM. SC-immunological sentinels of spermatogenesis. Semin Cell Dev Biol. (2014) 30:36–44. doi: 10.1016/j.semcdb.2014.02.011
12. Dimitriadis F, Tsiampali C, Chaliasos N, Tsounapi P, Takenaka A, Sofikitis N. The Sertoli cell as the orchestra conductor of spermatogenesis: spermatogenic cells dance to the tune of testosterone. Hormones (Athens). (2015) 14:479–503. doi: 10.14310/horm.2002.1633
13. Edelsztein NY, Grinspon RP, Schteingart HF, Rey RA. Anti-Müllerian hormone as a marker of steroid and gonadotropin action in the testis of children and adolescents with disorders of the gonadal axis. Int J Pediatr Endocrinol. (2016) 2016:20. doi: 10.1186/s13633-016-0038-2
14. Golat BT, Cameron DF. Sertoli cells enhance formation of capillary-like structures in vitro. Cell Transplant. (2008) 17:1135–44. doi: 10.3727/096368908787236512
15. Luca G, Fallarino F, Calvitti M, Mancuso F, Nastruzzi C, Arato I, et al. Xenograft of microencapsulated sertoli cells reverses T1DM in NOD mice by inducing neogenesis of beta-cells. Transplantation. (2010) 90:1352–57. doi: 10.1097/TP.0b013e3181ffb9d2
16. Luca G, Bellezza I, Arato I, Di Pardo A, Mancuso F, Calvitti M, et al. Terapeutic potential of microencapsulated sertoli cells in huntington disease. CNS Neurosci Ther. (2016) 22:686–90. doi: 10.1111/cns.12569
17. Arato I, Luca G, Mancuso F, Bellucci C, Lilli C, Calvitti M, et al. An in vitro prototype of a porcine biomimetic testis-like cell culture system: a novel tool for the study of reassembled Sertoli and Leydig cells. Asian J Androl. (2018) 20:160–5. doi: 10.4103/aja.ja_47_17
18. Mancuso F, Arato I, Lilli C, Bellucci C, Bodo M, Calvitti M, et al. Acute effects of lead on porcine neonatal Sertoli cells in vitro. Toxicol In Vitro. (2018) 48:45–52. doi: 10.1016/j.tiv.2017.12.013
19. Codina C, Estanyol JM, Fidalgo MJ, Ballescà JL, Oliva R. Advances in sperm proteomics: best-practise methodology and clinical potential. Expert Rev Proteomics. (2015) 12:255–77. doi: 10.1586/14789450.2015.1040769
20. Barrachina F, Jodar M, Delgado-Dueñas D, Soler-Ventura A, Estanyol JM, Mallofré C, et al. Stable-protein pair analysis as a novel strategy to identify proteomic signatures: application to seminal plasma from infertile patients. Mol Cell Proteomics. (2019) 18:S77–S90. doi: 10.1074/mcp.RA118.001248
21. Grande G, Vincenzoni F, Mancini F, Baroni S, Luca G, Calafiore R, et al. Semen Proteomics reveals the impact of enterococcus faecalis on male fertility. Protein Pept Lett. (2018) 25:472–77. doi: 10.2174/0929866525666180412161818
22. Josso N, Rey RA, Picard JY. Anti-müllerian hormone: a valuable addition to the toolbox of the pediatric endocrinologist. Int J Endocrinol. (2013) 2013:674105. doi: 10.1155/2013/674105
23. Themmen AP, Blok LJ, Post M, Baarends WM, Hoogerbrugge JW, Parmentier M, et al. Follitropin receptor down-regulation involves a cAMP-dependent post-transcriptional decrease of receptor mRNA expression. Mol Cell Endocrinol. (1991) 78:R7–R13. doi: 10.1016/0303-7207(91)90130-K
24. Meachem SJ, Nieschlag E, Simoni M. Inhibin B in male reproduction: pathophysiology and clinical relevance. Eur J Endocrinol. (2001) 145:561–71. doi: 10.1530/eje.0.1450561
25. Brekken RA, Sage EH. SPARC, a matricellular protein: at the crossroads of cell-matrix. Matrix Biol. (2000) 19:569–80. doi: 10.1016/S0945-053X(00)00105-0
26. Sage H, Vernon RB, Funk SE, Everitt EA, Angello J. SPARC, a secreted protein associated with cellular proliferation, inhibits cell spreading in vitro and exhibits Ca+2-dependent binding to the extracellular matrix. J Cell Biol. (1989) 109:341–56. doi: 10.1083/jcb.109.1.341
27. Mayer U, Aumailley M, Mann K, Timpl R, Engel J. Calcium-dependent binding of basement membrane protein BM-40 (osteonectin, SPARC) to basement membrane collagen type IV. Eur J Biochem. (1991) 198:141–50. doi: 10.1111/j.1432-1033.1991.tb15996.x
28. Lane TF, Sage EH. The biology of SPARC, a protein that modulates cell-matrix interactions. FASEB J. (1994) 8:163–73. doi: 10.1096/fasebj.8.2.8119487
29. Raines EW, Lane TF, Iruela-Arispe ML, Ross R, Sage EH. The extracellular glycoprotein SPARC interacts with platelet-derived growth factor (PDGF)-AB and -BB and inhibits the binding of PDGF to its receptors. Proc Natl Acad Sci U S A. (1992) 89:1281–5. doi: 10.1073/pnas.89.4.1281
30. Kupprion C, Motamed K, Sage EH. SPARC (BM-40, osteonectin) inhibits the mitogenic effect of vascular endothelial growth factor on microvascular endothelial cells. J Biol Chem. (1998) 273:29635–40. doi: 10.1074/jbc.273.45.29635
31. Motamed K, Blake DJ, Angello JC, Allen BL, Rapraeger AC, Hauschka SD, et al. Fibroblast growth factor receptor-1 mediates the inhibition of endothelial cell proliferation and the promotion of skeletal myoblast differentiation by SPARC: a role for protein kinase A. J Cell Biochem. (2003) 90:408–23. doi: 10.1002/jcb.10645
32. Howe CC, Overton GC, Sawicki J, Solter D, Stein P, Strickland S. Expression of SPARC/osteonectin transcript in murine embryos and gonads. Differentiation. (1988) 37:20–5. doi: 10.1111/j.1432-0436.1988.tb00792.x
33. Vernon RB, Sage H. The calcium-binding protein SPARC is secreted by Leydig and Sertoli cells of the adult mouse testis. Biol Reprod. (1989) 40:1329–40. doi: 10.1095/biolreprod40.6.1329
34. Wilson MJ, Bowles J, Koopman P. The matricellular protein SPARC is internalized in Sertoli, Leydig, and germ cells during testis differentiation. Mol Reprod Dev. (2006) 73:531–9. doi: 10.1002/mrd.20394
35. Mather JP, Woodruff TK, Krummen LA. Paracrine regulation of reproductive function by inhibin and activin. Proc Soc Exp Biol Med. (1992) 201:1–15. doi: 10.3181/00379727-201-43473
36. Bläuer M, Husgafvel S, Syvälä H, Tuohimaa P, Ylikomi T. Identification of nuclear localization signal in activin/inhibin betaA subunit; intranuclear βA in rat spermatogenic cells. Biol Reprod. (1999) 60:588–93. doi: 10.1095/biolreprod60.3.588
37. Ying SY, Zhang Z, Furst B, Batres Y, Huang G, Li G. Activin and activin receptors in cell growth. Proc Soc Exp Biol Med. (1997) 214:114–22. doi: 10.3181/00379727-214-44077
38. Liu YX, Du Q, Liu K, Fu GQ. Hormonal regulation of plasminogen activator in rat and mouse seminiferous epithelium. Biol Signals. (1995) 4:232–40. doi: 10.1159/000109447
39. Liu YX, Liu K, Zhou HM, Du Q, Hu ZY, Zou RJ. Hormonal regulation of tissue-type plasminogen activator and plasminogen activator inhibitor type-1 in cultured monkey Sertoli cells. Hum Reprod. (1995) 10:719–27. doi: 10.1093/oxfordjournals.humrep.a136022
40. Leblond CP, Clermont Y. Definition of the stages of the cycle of the seminiferous epithelium in the rat. Ann N Y Acad Sci. (1952) 55:548–73. doi: 10.1111/j.1749-6632.1952.tb26576.x
41. Morales C, Clermont Y, Nadler NJ. Cyclic endocytic activity and kinetics of lysosomes in Sertoli cells of the rat: a morphometric analysis. Biol Reprod. (1986) 34:207–18. doi: 10.1095/biolreprod34.1.207
42. Sigillo F, Pernod G, Kolodie L, Benahmed M, Le Magueresse-Battistoni B. Residual bodies stimulate rat Sertoli cell plasminogen activator activity. Biochem Biophys Res Commun. (1998) 250:59–62. doi: 10.1006/bbrc.1998.9264
43. Russell DL, Kim KH. Expression of triosephosphate isomerase transcripts in rat testis: evidence for retinol regulation and a novel germ cell transcript. Biol Reprod. (1996) 55:11–8. doi: 10.1095/biolreprod55.1.11
44. Vogl AW, Soucy LJ. Arrangement and possible function of actin filament bundles in ectoplasmic specializations of ground squirrel Sertoli cells. J Cell Biol. (1985) 100:814–25. doi: 10.1083/jcb.100.3.814
45. Moura AA. Seminal plasma proteins and fertility indexes in the bull: the case for osteopontin. Anim Reprod. (2005) 2:3–10.
46. Huleihel M, Abofoul-Azab M, Abarbanel Y, Einav I, Levitas E, Lunenfeld E. Production of macrophage inhibitory factor (MIF) by primary Sertoli cells; its possible involvement in migration of spermatogonial. Cells J Cell Physiol. (2017) 232:2869–77. doi: 10.1002/jcp.25718
47. Islam R, Yoon H, Kim BS, Bae HS, Shin HR, Kim WJ, et al. Blood-testis barrier integrity depends on Pin1 expression in Sertoli cells. Sci Rep. (2017) 7:6977. doi: 10.1038/s41598-017-07229-1
48. Ge LC, Chen ZJ, Liu H, Zhang KS, Sue Q, Ma XY, et al. Signaling related with biphasic effects of bisphenol A (BPA) on Sertoli cell proliferation: a comparative proteomic analysis. Biochim Biophys Acta. (2014) 1840:2663–73. doi: 10.1016/j.bbagen.2014.05.018
49. Kang SW, Chae HZ, Seo MS, Kim K, Baines IC, Rhee SG. Mammalian peroxiredoxin isoforms can reduce hydrogen peroxide generated in response to growth factors and tumor necrosis factor-alpha. J Biol Chem. (1998) 273:6297–302. doi: 10.1074/jbc.273.11.6297
50. Lee K, Park JS, Kim YJ, Lee YS, Hwang TS, Kim DJ, et al. Differential expression of Prx I and II in mouse testis and their up-regulation by radiation. Biochem Biophys Res Commun. (2002) 296:337–42. doi: 10.1016/S0006-291X(02)00801-X
51. Middendorff R, Kumm M, Davidoff MS, Holstein AF, Müller D. Generation of cyclic guanosine monophosphate by heme oxygenases in the human testis-A regulatory role for carbon monoxide in sertoli cells? Biol Reprod. (2000) 63:651–7. doi: 10.1095/biolreprod63.2.651
52. Kwon J, Mochida K, Wang YL, Sekiguchi S, Sankai T, Aoki S, et al. Ubiquitin C-terminal hydrolase L-1 is essential for the early apoptotic wave of germinal cells and for sperm quality control during spermatogenesis. Biol Reprod. (2005) 73:29–35. doi: 10.1095/biolreprod.104.037077
53. Griswold MD, Solari A, Tung PS, Fritz IB. Stimulation by follicle-stimulating hormone of DNA synthesis and of mitosis in cultured Sertoli cells prepared from testes of immature rats. Mol Cell Endocrinol. (1997) 7:151–65. doi: 10.1016/0303-7207(77)90064-8
Keywords: Sertoli cells, alpha follitropin, beta follitropin, urofollitropin, proteomic analysis
Citation: Arato I, Grande G, Barrachina F, Bellucci C, Lilli C, Jodar M, Aglietti MC, Mancini F, Vincenzoni F, Pontecorvi A, Calafiore R, Oliva R, Luca G, Mancuso F and Milardi D (2020) “In vitro” Effect of Different Follicle—Stimulating Hormone Preparations on Sertoli Cells: Toward a Personalized Treatment for Male Infertility. Front. Endocrinol. 11:401. doi: 10.3389/fendo.2020.00401
Received: 23 March 2020; Accepted: 19 May 2020;
Published: 18 June 2020.
Edited by:
Rossella Cannarella, University of Catania, ItalyReviewed by:
Paolo Magni, University of Milan, ItalyPascale Crepieux, Centre National de la Recherche Scientifique (CNRS), France
Copyright © 2020 Arato, Grande, Barrachina, Bellucci, Lilli, Jodar, Aglietti, Mancini, Vincenzoni, Pontecorvi, Calafiore, Oliva, Luca, Mancuso and Milardi. This is an open-access article distributed under the terms of the Creative Commons Attribution License (CC BY). The use, distribution or reproduction in other forums is permitted, provided the original author(s) and the copyright owner(s) are credited and that the original publication in this journal is cited, in accordance with accepted academic practice. No use, distribution or reproduction is permitted which does not comply with these terms.
*Correspondence: Giovanni Luca, giovanni.luca@unipg.it
†These authors share first authorship
‡These authors share senior authorship