- 1Department of Pharmacy Practice, College of Pharmacy, University of Illinois at Chicago, Chicago, IL, United States
- 2Department of Epidemiology, Erasmus Medical Centre Rotterdam, Rotterdam, Netherlands
- 3Department of Psychiatry, College of Medicine, University of Illinois at Chicago, Chicago, IL, United States
- 4The Psychiatric Institute, Department of Psychiatry, College of Medicine, University of Illinois at Chicago, Chicago, IL, United States
Although results of animal research show that interactions between stress and sex hormones are implicated in the development of affective disorders in women, translation of these findings to patients has been scarce. As a basic step toward advancing this field of research, we analyzed findings of studies which reported circulating cortisol levels in healthy women in the follicular vs. luteal phase of the menstrual cycle. We deemed this analysis critical not only to advance our understanding of basic physiology, but also as an important contrast to the findings of future studies evaluating stress and sex hormones in women with affective disorders. We hypothesized that cortisol levels would be lower in the follicular phase based on the proposition that changes in levels of potent GABAergic neurosteroids, including allopregnanolone, during the menstrual cycle dynamically change in the opposite direction relative to cortisol levels. Implementing strict inclusion criteria, we compiled results of high-quality studies involving 778 study participants to derive a standardized mean difference between circulating cortisol levels in the follicular vs. luteal phase of the menstrual cycle. In line with our hypothesis, our meta-analysis found that women in the follicular phase had higher cortisol levels than women in the luteal phase, with an overall Hedges' g of 0.13 (p < 0.01) for the random effects model. No significant between-study difference was detected, with the level of heterogeneity in the small range. Furthermore, there was no evidence of publication bias. As cortisol regulation is a delicate process, we review some of the basic mechanisms by which progesterone, its potent metabolites, and estradiol regulate cortisol output and circulation to contribute to the net effect of higher cortisol in the follicular phase.
Introduction
Women exhibit high prevalence of stress-related disorders, such as major depressive disorder (MDD) and anxiety spectrum disorder (1–9). Importantly, the increase in prevalence of these disorders is observed during periods of drastic hormonal changes, such as puberty, the pre-menstrual period, pregnancy, postpartum and menopause (10–12). These observations suggest that interactions between sex hormones, regulated by the hypothalamic-pituitary-gonadal (HPG) axis, and cortisol, a stress hormone under the control of the hypothalamic-pituitary-adrenal (HPA) axis, may be critical determinants of stress-related disorder development and progression.
Research evaluating stress effects in MDD and anxiety disorders demonstrates a blunted cortisol response to psychosocial stress in female patients compared to their respective controls [for a meta-analysis, see (13)]. However, although these research studies provide valuable information, they only examine function of the HPA axis, without evaluating how sex hormones influence it.
The number of studies evaluating interactions between the provoked HPA and the HPG axes is limited in both diseased as well as healthy participants. Results of studies comparing reactivity to psychosocial stress in healthy women suggest that cortisol output is higher in the luteal vs. follicular phase of the menstrual cycle (14, 15). However, their small sample size and opposite findings from other studies (16–19) indicate that more research needs to be completed before a conclusion can be drawn. Additional studies, implementing strict verification of menstrual cycle phase, stress manipulation and participants' healthy status, are needed.
An even more fundamental question, though, is related to the physiological relationship between the HPA and HPG axes under unprovoked, tonic conditions. However, results from human laboratory and observational studies in healthy volunteers evaluating basal cortisol levels across the menstrual cycle range broadly. Thus, we focused on the function of the HPA axis with the hypothesis that there would be a higher physiological output of cortisol during the follicular compared to the luteal phase of the menstrual cycle. Our hypothesis was based on the finding that the progesterone metabolite allopregnanolone positively modulates gamma-aminobutyric acid (GABA)A receptors via an allosteric binding site to potentiate inhibitory signaling (20) and enhance the negative feedback on the HPA axis (21, 22). Therefore, during the luteal phase, when allopregnanolone levels are high, cortisol levels would be expected to decrease relative to the follicular phase, when allopregnanolone levels are low.
Tonic levels of cortisol across the menstrual cycle have been reported as higher for example, (23, 24) or unchanged (25, 26) in the follicular vs. luteal phase. These discrepancies are rooted in marked methodological differences across studies. Hence, our analysis only included high-quality research studies implementing strict criteria and phase identification. Based on mechanistic considerations of basic research studies (reviewed in the Discussion section) that have reported the effects of neuroactive steroids on the HPA axis function, we predicted a surge in cortisol during the early/mid-follicular phase. Our meta-analysis, indeed, shows that circulating cortisol levels change dynamically as a function of menstrual cycle phase, suggesting cortisol is specifically required during the early/mid follicular phase to mediate adaptive physiological processes in response to environmental stimuli, when both estradiol and progesterone are low.
Methods
Search Strategy
We conducted a literature search in PubMed, Web of Knowledge and PsychInfo, and included eligible studies published through December 5th, 2019. Two authors (AH and KK) completed their search independently according to the Preferred Reporting Items for Systematic Reviews and Meta-Analyses (PRISMA) guidelines (27). Any discrepancies were reconciled by reviewing the literature jointly for specific points of difference. We used the following search string: [(“Cortisol”) AND (“Menstrual” OR “Luteal” OR “Follicular”) for (DOCUMENT TYPE: (Article); LANGUAGE: English; SUBJECTS: Human)]. We compiled the results in EndNote X8.
Inclusion/Exclusion Criteria
This meta-analysis evaluated tonic peripheral cortisol levels of healthy menstruating female study participants across follicular vs. luteal phases of the menstrual cycle. Studies were considered eligible if a baseline value was provided prior to a laboratory intervention (for example, psychosocial stress procedure or exercise), if samples were collected longitudinally in a naturalistic (or a laboratory) setting across the menstrual cycle, if an experimental design evaluating a disease state included a healthy control or if an intervention included a placebo control. The exclusionary criteria implementation was carried out in a two-step approach.
In the first step, study abstracts (N = 2,225) were excluded if they were: (1) abstracts, review papers or case studies, (2) animal studies, or evaluation of cell lines, (3) male-only evaluations, (4) abstracts which only mentioned one menstrual phase (luteal or follicular) as a means of controlling for menstrual cycle phase (i.e., not as a comparison of the two phases), (5) studies evaluating a diseased population (including smokers or other substance use disorder population) or implementing a menstrual phase-specific intervention (without a placebo control). This category also included abstracts describing pregnant as well as women in the peri- or post-menstrual phases, as well as women who were on oral contraceptives. Finally, abstracts describing athletes or women who experienced early life trauma were also coded in this category. The remaining abstracts were excluded if they: (6) did not mention cortisol (blood, salivary or urinary), (7) were overlapping study participants with an already published study, and (8) if they described a procedure (such as IV fertilization, for example) which could cause changes in circulating cortisol due to anticipation.
In the second round of exclusion criteria implementation, we evaluated full articles. We excluded papers which did not evaluate groups according to menstrual cycle phases, measure cortisol, have a healthy, non-athletic control group, or report mean and/or variance and were published more than 25 years ago. Furthermore, we excluded papers which re-administered stress (as this can distort baseline cortisol levels), performed non-linear cortisol modeling, were non-original, written in languages other than English, or had overlapping participants. Given the rapid decline of cortisol in the morning, studies which collected cortisol during morning times that varied ≥2 h or did not mention what time of the day cortisol sample collection -took place were excluded, as well as studies which determined cycle phase using self-report.
Data Extraction
Information on the following variables was collected: (1) age, (2) BMI, (3) day of follicular and luteal phase of cortisol collection, (4) phase estimation method, (5) time of day of cortisol collection, and (6) physiological source of cortisol level. Regarding the length of phase variable, whereas some studies reported a range of days, others reported multiple, exact days of the cycle on which cortisol was reported. In the event that a study reported cortisol values across multiple days of the menstrual cycle, data from the day closest to the beginning of the cycle (day 1) for follicular phase, and day 21 of the luteal phase were extracted to reflect the greatest contrast of estradiol and progesterone levels across the cycle. In the event that cortisol was reported on multiple “sub-phases” (for example, early-follicular, mid-follicular), again, data from the day closest to the beginning of the cycle (day 1) for follicular phase, and day 21 of the luteal phase were extracted. If cortisol was collected across multiple menstrual cycles (for example, two menstrual cycles), values from the last cycle were reported. In the event that studies reported cortisol values at multiple times of the day (for example, morning and evening), or multiple sources (for example, salivary and plasma), the most frequently reported time (morning) and source (blood) in the remaining studies were used to extract cortisol values.
Data Analysis
Analyses of were carried out by first calculating the Cohen's d effect size (28). In the event mean and variance values were provided for sub-groups of women in follicular and luteal phases, those were combined using the following formulas:
where, N1 = sample size group 1, N2 = sample size group 2, M1 = mean group 1, M2 = mean group 2, SD1 = standard deviation group 1, SD2 = standard deviation group 2.
We divided the mean difference between the two groups by the pooled standard deviation. Next, we used the J-correction factor to obtain the Hedges' g effect size, which corrects for small samples (29) and is considered small, medium, and large for values 0.2, 0.5, and 0.8, respectively. Within- and between-subject study designs were combined as described in Morris et al. (30). We used a random effects model to calculate the pooled effect size with an associated 95% CI and a p-value (31). We assessed source-study heterogeneity using the χ2-based Q test with its associated p-value. A statistically significant Q statistic suggests different effect sizes across studies, implying that methodological or population sample differences may be introducing variance across individual studies. We quantified heterogeneity using I2 with values 25, 50, and 75% suggestive of small, medium and large heterogeneity and calculated potential publication bias using the Classical Tests (32). We completed sub-analyses according to source (saliva vs. plasma) and time of day (morning vs. afternoon). The meta-analysis was performed using the “escalc” function, and publication bias was assessed using the “ranktest” function in “metafor” package (33) in R.
Results
Characteristics of Individual Studies
After removal of duplicate studies, literature search identified 2, 226 individual abstracts as shown in the PRISMA figure (Figure 1). Those abstracts were screened for relevance and coded for exclusion reasons. The greatest number of abstracts excluded was based on absence of menstrual cycle groups, followed by cell line/animal research studies. A total of 256 full-text articles were reviewed for relevance, of which 221 were excluded. Of the 221 studies, 44 were excluded based on the self-report nature of menstrual cycle phase determination, and 40 were excluded because they either didn't mention cortisol collection time, or the morning sample was collected at times which varied by two or more hours. The analysis included data from 35 final studies.
As shown in Table 1, most of the studies included participants in their 20s, with a BMI below 25. Whereas, some studies incorporated fine-grained sub-phases of follicular and luteal phases (see Table 2), others defined the phases as day 1–14 and 15–28. Phase estimation was determined via progesterone level acquisition, LH surge measurement, basal body temperature measurement, or pelvic ultrasound. Times of cortisol collection were in the morning, afternoon/evening or over several hours, and the source was saliva, plasma or urine. Whereas, Supplementary Table 1 shows all the days/phases of menstrual cycle, time of collection and sources of cortisol across all the studies, Table 2 only shows the actual day (or range of days), time and source of cortisol which were included in the analysis. These two tables are provided in order to increase transparency of reporting, as several studies reported several values, for which we implemented the rules as specified in the Methods Section Data Extraction.
Evaluation of Standardized Mean Difference in Cortisol Levels Across Menstrual Cycle
Women in the follicular phase had higher cortisol levels than women in the luteal phase, with an overall Hedges' g of 0.13 (p < 0.01) for the random effects model (Figure 2). The confidence interval range was between 0.05 and 0.20. No significant between-study difference was detected (tau2 =0; H = 1.0), with the level of heterogeneity in the small range (I2 = 0%, Q = 30.69; p = 0.63). The Rank Correlation Test for Funnel Plot Asymmetry resulted in Kendall's tau = 0.14 (p = 0.23), indicating absence of small study effects.
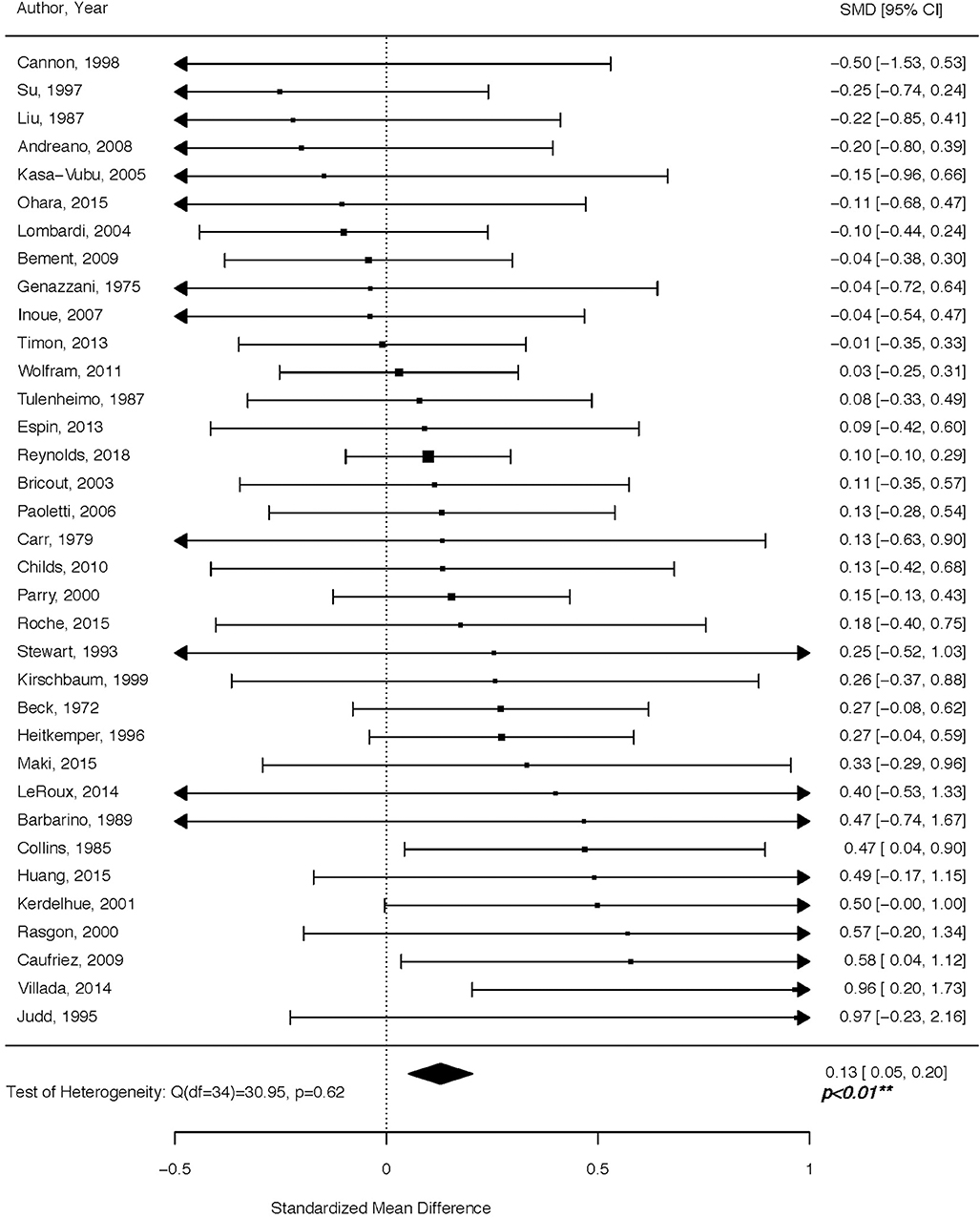
Figure 2. Forest plot of cortisol levels across the menstrual cycle. Positive standardized mean difference (SMD) means that cortisol levels were higher in the follicular vs. luteal phase.
Sub-analysis of Cortisol Levels According to Source and Time of Day
Sub-analysis according to the biospecimen source showed a significant effect of plasma (p = 0.036) and marginally significant effect of saliva (p = 0.055). The time of day sub-analysis showed a significant effect of morning (p = 0.005), but not afternoon (p = 0.177). Table 3 displays all relevant sub-analyses statistics, including standardized mean difference, standard error, z and p-values, confidence intervals, degrees of freedom, Q statistic and its associated p-value.
Discussion
For decades, literature on cortisol has yielded mixed results with respect to its concentration in the follicular vs. luteal phase of the menstrual cycle. Implementing a comprehensive search of high-quality studies spanning a period of almost 50 years of research, we show that circulating cortisol levels are higher in the follicular vs. luteal phase. Cortisol regulation is a delicate process of extensive physiological processes working in concert to adjust responses to environmental stimuli. Below, we review mechanisms driving circulating cortisol levels to both increase and decrease across various menstrual cycle time-points, while noting that the net effect of these, or other, still unidentified processes, is a higher circulating cortisol during the follicular compared to the luteal phase, as reported in our analysis (Figure 2).
The paraventricular nucleus (PVN) of the hypothalamus integrates numerous circadian and environmental inputs to funnel information through neurons expressing corticotropin-releasing hormone (CRH). The release of CRH into the hypophyseal portal vasostructure enhances the synthesis and release of adrenocorticotropic hormone (ACTH) from the anterior pituitary, which, in turn, stimulates adrenal glands to synthesize and release cortisol to adapt metabolic processes and behavioral responses.
Intriguingly, PVN neurons express high levels of estrogen receptor beta (ER-β) and low levels of estrogen receptor alpha (ER-α) (64–66). Several research studies have demonstrated that estradiol, through its near equivalent affinity for the two estrogen receptor subtypes, can selectively decrease or increase HPA axis function. A high number of ER-β-expressing cells in the PVN are oxytocin and vasopressin immunoreactive (67–69), which complement the CRH neurons that also express ER-β (64). Stimulation of ER-β in the PVN results in a reduction of cortisol levels. In accord, both centrally- and peripherally-delivered ER-β selective agonists inhibit the HPA function (70). ER-α occupancy, on the other hand, has an indirect, trans-synaptic activation in the PVN. The peri-PVN region contains ER-α neurons, and their activation can impair glucocorticoid-mediated negative feedback regulation of the HPA axis (71). These opposing actions of estradiol—with ER-α amplifying, and ER-β reducing HPA function—are in agreement with laboratory observations that estradiol both enhances (72, 73) and inhibits (74, 75) HPA function. Hence, in the luteal phase, when estradiol levels are higher compared to the early/mid follicular phase, theoretically, depending on the extent of ER-β or ER-α expression and activation in or near the PVN, estradiol can either decrease or increase circulating cortisol levels.
The activity of CRH neurons in the PVN is tightly regulated by inhibitory GABAergic interneuron populations (76). Allopregnanolone, a progesterone derivative resulting from conversion by 5α-reductase type I and 3α-hydroxysteroid dehydrogenase, is an endogenous neurosteroid and a potent, positive, allosteric modulator of the action of the inhibitory neurotransmitter GABA at GABAA receptor. Studies in rodents show an inhibitory effect of allopregnanolone on the function of the HPA axis (77–79). This effect of allopregnanolone seems to be exerted through its action at GABAA receptors, and subsequent inhibition on PVN neurons (80) under both basal and stressful conditions (81). In support of these findings, in addition to allopregnanolone, another potent GABAA receptor modulator and deoxycorticosterone-derived steroid, tetrahydrodeoxycorticosterone (TH-DOC), also attenuates the HPA axis function (77, 82, 83). Therefore, in the luteal phase, under the physiological milieu of higher circulating progesterone levels and, most importantly, of its potent GABAA receptor-modulating metabolite, allopregnanolone, lower circulating cortisol levels can be expected relative to the follicular phase. Not surprisingly, our meta-analysis has confirmed this expectation (Figure 3).
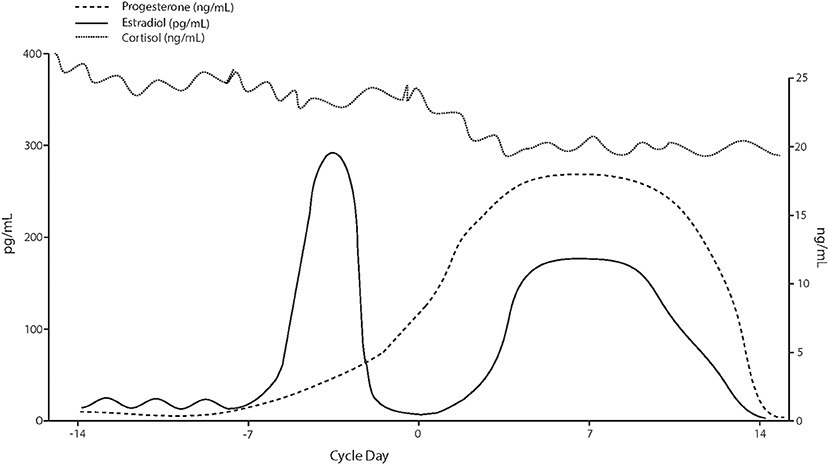
Figure 3. Hypothetical cortisol levels across the menstrual cycle based on values reported in the analyzed studies.
Once released in the blood flow, ~80% of circulatory cortisol is bound to corticosteroid-binding globulin (CBG), leaving ~5% of cortisol in the free form (84). CBG is primarily synthesized by the liver and secreted into the bloodstream, where it binds and provides a pool of circulating cortisol. It enhances the availability of cortisol to be released on demand both systemically and at a tissue level. Whereas plasma cortisol reflects total cortisol (i.e., bound and unbound), the salivary levels only reflect unbound/free cortisol. Changes in circulating CBG have a significant impact on total, but not free, cortisol concentrations (85, 86). The CBG is under a tight regulation by estradiol, with women expressing greater CBG basal concentrations than men (87). Interestingly, ethinyl estradiol, found in oral contraceptives (OC), dose-dependently increases CBG serum levels (88). Ethynyl estradiol is also a potent ER modulator, with 194 and 151% of the affinity of estradiol for the ER-α and ER-β, respectively (89). It is, however, unclear whether changes in estradiol across the menstrual cycles can alter CBG. Nenke et al. (90) report that total CBG concentration reaches ~1,000 nmol/L in pregnancy as well as during the active phase of pill cycle containing ethinyl estradiol. These expression levels are substantially higher than the CBG levels of ~500 nmol/L determined in non-pregnant, non-OC taking individuals (90). Unfortunately, this study did not account for menstrual cycle phase, which would have provided valuable information regarding CBG changes, if any, across the menstrual cycle. The question of potential CBG changes across the menstrual cycle due to changes in estradiol concentrations should be evaluated in future studies by carefully examining the potential anti-estrogenic and blunting effect of luteal progesterone on estradiol-induced increase in CBG (91).
There are several limitations to consider in the present meta-analysis. First, our sub-analyses may have been underpowered to detect effects of time variations. For example, the “time of day” sub-analysis showed a significant effect of morning, but not afternoon menstrual phase cortisol (Table 3). However, degrees of freedom for morning vs. afternoon sub-analyses were 21 and 7, respectively, with the afternoon sample possibly underpowered to detect a significant effect. Importantly, our sub-analysis of “source” (plasma vs. serum) was consistent with our main study results, showing higher follicular cortisol in both the free and total form. Furthermore, given that the overall sample was fairly homogenous, with most women in the same age range (20–29 years old) and a normal BMI (Table 1), we were unable to perform a meta-regression, which would have provided meaningful information related to the direction of future mechanistic studies.
As the research evaluating interacting effects between HPA and HPG axes unfolds, there are several issues to consider. Perhaps the greatest pitfall of menstrual cycle research is the inadequate assessment of the menstrual cycle phase. In the full-article evaluation step of our meta-analysis, we excluded findings from 44 studies because menstrual cycle phase was estimated based on self-report. Retrospective reports of menstrual cycle “start” and “duration” are plagued by profound phase misinformation (92) and prospective measures confirming both ovulation and luteal phase status are essential. Menstrual cycle phase determination was also based on self-report in approximately half of the studies evaluating the HPA reactivity in the follicular vs. luteal phase (16, 18, 19), contributing to the inability to make meaningful conclusions regarding the direction of effect.
HPA axis dysfunction is strongly implicated in the etiopathology of affective disorders (93–95) with women at an increased risk (96, 97). Yet, basic questions related to the function of the HPA axis throughout stages of the menstrual cycle under acute or prolonged stress conditions remain largely unanswered. Whereas, our meta-analysis reflects a single time point in the cortisol diurnal cycle, it is still unknown whether there are phase-specific effects on the shape of the diurnal curve. This assessment could be easily implemented given the availability of the salivary (unbound) cortisol assay, and would provide a comprehensive picture of daily cortisol trajectory and its potential mean, amplitude and/or phase shift as the hormonal milieu changes across the menstrual cycle. In this case, the awakening and morning cortisol should be taken more frequently than the late afternoon/evening samples.
It is strongly recommended that future studies employ a fine-grained approach (i.e., evaluating early/mid follicular, ovulatory, early, mid and late luteal phases) to advance women's mental health research, rather than broadly defining phases as “follicular” vs. “luteal.”
In summary, the aim of our meta-analysis was to summarize findings of a period spanning over 50 years of research and test whether circulating cortisol levels change as a function of menstrual phase. With respect to this objective, we showed higher cortisol levels in the follicular vs. luteal phase of the menstrual cycle. By completing this aim, our hope is to simultaneously increase awareness of the poor state of women's neuroendocrine science research. Experimental protocols designed to study effects that influence the HPA and HGA axis function need to be specifically designed to account for women's physiological requirements. The joint protocols previously designed for both sexes rarely apply to studies involving women. Whereas, participant recruitment, evaluation and analysis are more rapid in many research scenarios involving men, given the dynamic nature of the menstrual cycle and the need for prospective data collection, the same parameters should not be applied to research involving women. Resources and completion expectations need to be adjusted as such. Once implemented, these changes will contribute meaningful information to progress our understanding of rhythmic hormonal changes, which are crucial for understanding the now well-established sex difference in affective disorder development and progression.
Data Availability Statement
The datasets generated for this study are available on request to the corresponding author.
Author Contributions
AH, KK, SC, and TE-M conceptualized the question, completed literature search and data gathering. AH and FS completed statistical analysis. AH and GP wrote the manuscript.
Funding
Research reported in this publication was supported by the National Institute of Mental Health of the National Institutes of Health under Award Number R21MH119642.
Disclaimer
The content is solely the responsibility of the authors and does not necessarily represent the official views of the National Institutes of Health.
Conflict of Interest
The authors declare that the research was conducted in the absence of any commercial or financial relationships that could be construed as a potential conflict of interest.
Acknowledgments
The authors would like to thank Dr. Ann Caufriez (Laboratoire de Physiologie et Pharmacologie, Université libre de Bruxelles, Brussels, Belgium) for sending a complete dataset and Dr. Scott Morris (Department of Psychology Illinois Institute of Technology. Chicago, Illinois, USA) for statistical consultation.
Supplementary Material
The Supplementary Material for this article can be found online at: https://www.frontiersin.org/articles/10.3389/fendo.2020.00311/full#supplementary-material
References
1. Albert PR. Why is depression more prevalent in women? J Psychiatry Neurosci. (2015) 40:219–21. doi: 10.1503/jpn.150205
2. Angst J, Gamma A, Gastpar M, Lepine JP, Mendlewicz J, Tylee A, et al. Gender differences in depression. Epidemiological findings from the European DEPRES I and II studies. Eur Arch Psychiatry Clin Neurosci. (2002) 252:201–9. doi: 10.1007/s00406-002-0381-6
3. Barnes VA, Treiber FA, Ludwig DA. African-American adolescents' stress responses after the 9/11/01 terrorist attacks. J Adolesc Health. (2005) 36:201–7. doi: 10.1016/j.jadohealth.2004.02.035
4. Breslau N, Schultz L, Peterson E. Sex differences in depression: a role for preexisting anxiety. Psychiatr Res. (1995) 58:1–12. doi: 10.1016/0165-1781(95)02765-O
5. Cahill L. Why sex matters for neuroscience. Nat Rev Neurosci. (2006) 7:477–84. doi: 10.1038/nrn1909
6. Kessler RC, McGonagle KA, Nelson CB, Hughes M, Swartz M, Blazer DG. Sex and depression in the National Comorbidity Survey. II: Cohort effects. J Affect Disord. (1994) 30:15–26. doi: 10.1016/0165-0327(94)90147-3
7. McLean CP, Asnaani A, Litz BT, Hofmann SG. Gender differences in anxiety disorders: prevalence, course of illness, comorbidity and burden of illness. J Psychiatr Res. (2011) 45:1027–35. doi: 10.1016/j.jpsychires.2011.03.006
8. Olff M. Sex and gender differences in post-traumatic stress disorder: an update. Eur J Psychotraumatol. (2017) 8:1351204. doi: 10.1080/20008198.2017.1351204
9. Seeman MV. Psychopathology in women and men: focus on female hormones. Am J Psychiatry. (1997) 154:1641–7. doi: 10.1176/ajp.154.12.1641
10. Beddig T, Kuhner C. Current aspects of premenstrual dysphoric disorder - a review. Psychother Psychosom Med Psychol. (2017) 67:504–13. doi: 10.1055/s-0043-113816
11. Biaggi A, Conroy S, Pawlby S, Pariante CM. Identifying the women at risk of antenatal anxiety and depression: A systematic review. J Affect Disord. (2016) 191:62–77. doi: 10.1016/j.jad.2015.11.014
12. Birkhauser M. Depression, menopause and estrogens: is there a correlation? Maturitas. (2002) 41:S3–8. doi: 10.1016/S0378-5122(02)00009-9
13. Zorn JV, Schur RR, Boks MP, Kahn RS, Joels M, Vinkers CH. Cortisol stress reactivity across psychiatric disorders: a systematic review and meta-analysis. Psychoneuroendocrinology. (2017) 77:25–36. doi: 10.1016/j.psyneuen.2016.11.036
14. Espin L, Almela M, Hidalgo V, Villada C, Salvador A, Gomez-Amor J. Acute pre-learning stress and declarative memory: impact of sex, cortisol response and menstrual cycle phase. Horm Behav. (2013) 63:759–65. doi: 10.1016/j.yhbeh.2013.03.013
15. Villada C, Hidalgo V, Almela M, Salvador A. Assessing performance on an evaluated speaking task. J Psychophysiol. (2018) 32:64–74. doi: 10.1027/0269-8803/a000185
16. Montero-Lopez E, Santos-Ruiz A, Garcia-Rios MC, Rodriguez-Blazquez M, Rogers HL, Peralta-Ramirez MI. The relationship between the menstrual cycle and cortisol secretion: Daily and stress-invoked cortisol patterns. Int J Psychophysiol. (2018) 131:67–72. doi: 10.1016/j.ijpsycho.2018.03.021
17. Kirschbaum C, Kudielka BM, Gaab J, Schommer NC, Hellhammer DH. Impact of gender, menstrual cycle phase, and oral contraceptives on the activity of the hypothalamus-pituitary-adrenal axis. Psychosom Med. (1999) 61:154–62. doi: 10.1097/00006842-199903000-00006
18. Duchesne A, Tessera E, Dedovic K, Engert V, Pruessner JC. Effects of panel sex composition on the physiological stress responses to psychosocial stress in healthy young men and women. Biol Psychol. (2012) 89:99–106. doi: 10.1016/j.biopsycho.2011.09.009
19. Duchesne A, Pruessner JC. Association between subjective and cortisol stress response depends on the menstrual cycle phase. Psychoneuroendocrinology. (2013) 38:3155–9. doi: 10.1016/j.psyneuen.2013.08.009
20. Girdler SS, Klatzkin R. Neurosteroids in the context of stress: implications for depressive disorders. Pharmacol Ther. (2007) 116:125–39. doi: 10.1016/j.pharmthera.2007.05.006
21. Brunton PJ. Neuroactive steroids and stress axis regulation: Pregnancy and beyond. J Steroid Biochem Mol Biol. (2016) 160:160–8. doi: 10.1016/j.jsbmb.2015.08.003
22. Gunn BG, Cunningham SG, Mitchell JD, Swinny JJ, Lambert D, Belellie D. GABAA receptor-acting neurosteroids: a role in the development and regulation of the stress response. Front Neuroendocrinol. (2015) 35:28–48. doi: 10.1016/j.yfrne.2014.06.001
23. Kerdelhué B, Brown S, Lenoir V, Queenan JT, Jones GS, Scholler R, et al. Timing of Initiation of the preovulatory luteinizing hormone surge and its relationship with the circadian cortisol rhythm in the human. Neuroendocrinology. (2002) 75:158–63. doi: 10.1159/000048233
24. Villada C, Hidalgo V, Almela M, Mastorci F, Sgoifo A, Salvador A. Coping with an acute psychosocial challenge: behavioral and physiological responses in young women. PLoS ONE. (2014) 9:e114640. doi: 10.1371/journal.pone.0114640
25. Timon R, Corvillo M, Brazo J, Robles MC, Maynar M. Strength training effects on urinary steroid profile across the menstrual cycle in healthy women. Eur J Appl Physiol. (2013) 113:1469–75. doi: 10.1007/s00421-012-2575-6
26. Inoue Y, Terao T, Iwata N, Okamoto K, Kojima H, Okamoto T, et al. Fluctuating serotonergic function in premenstrual dysphoric disorder and premenstrual syndrome: findings from neuroendocrine challenge tests. Psychopharmacology. (2007) 190:213–9. doi: 10.1007/s00213-006-0607-9
27. Liberati A, Altman DG, Tetzlaff J, Mulrow C, Gotzsche PC, Ioannidis JP, et al. The PRISMA statement for reporting systematic reviews and meta-analyses of studies that evaluate health care interventions: explanation and elaboration. PLoS Med. (2009) 6:e1000100. doi: 10.1371/journal.pmed.1000100
28. Cohen J. Statistical Power Analysis for the Behavioral Sciences. New York, NY: Routledge Academic (1988).
30. Morris SB, DeShon RP. Combining effect size estimates in meta-analysis with repeated measures and independent-groups designs. Psychol Methods. (2002) 7:105–25. doi: 10.1037/1082-989x.7.1.105
31. Borenstein M. Effect sizes for continuous data. In: Cooper H, Hedges L, Valentine J, editors. The Handbook of Research Synthesis and Meta Analysis. New York, NY: Russell Sage Foundation (2009).
32. Egger M, Smith G, Schneider M, Minder C. Bias in meta-analysis detected by a simple, graphical test. Br Med J. (1997) 315:629–34. doi: 10.1136/bmj.315.7109.629
33. Schwarzer G, Antes G, Schumacher M. A test for publication bias in meta-analysis with sparse binary data. Stat Med. (2007) 26:721–33. doi: 10.1002/sim.2588
34. Andreano JM, Arjomandi H, Cahill L. Menstrual cycle modulation of the relationship between cortisol and long-term memory. Psychoneuroendocrinology. (2008) 33:874–82. doi: 10.1016/j.psyneuen.2008.03.009
35. Barbarino A, De Marinis L, Folli G, Tofani A, Della Casa S, D'Amico C, et al. Corticotropin-releasing hormone inhibition of gonadotropin secretion during the menstrual cycle. Metabolism. (1989) 38:504–6. doi: 10.1016/0026-0495(89)90208-4
36. Beck RP, Morcos F, Fawcett D, Watanabe M. Adrenocortical function studies during the normal menstrual cycle and in women receiving norethindrone with and without mestranol. Am J Obstet Gynecol. (1972) 112:364–8. doi: 10.1016/0002-9378(72)90478-4
37. Hoeger Bement MK, Rasiarmos RL, DiCapo JM, Lewis A, Keller ML, Harkins AL, et al. The role of the menstrual cycle phase in pain perception before and after an isometric fatiguing contraction. Eur J Appl Physiol. (2009) 106:105–12. doi: 10.1007/s00421-009-0995-8
38. Bricout VA, Wright F, Lagoguey M. Urinary profile of androgen metabolites in a population of sportswomen during the menstrual cycle. Int J Sports Med. (2003) 24:197–202. doi: 10.1055/s-2003-39090
39. Cannon JG, Angel JB, Abad LW, O'Grady J, Lundgren N, Fagioli L, et al. Hormonal influences on stress-induced neutrophil mobilization in health and chronic fatigue syndrome. J Clin Immunol. (1998) 18:291–8. doi: 10.1023/A:1027389907780
40. Carr BR, Parker CR, Madden JD, MacDonald PC, Porter JC. Plasma levels of adrenocorticotropin and cortisol in women receiving oral contraceptive steroid treatment. J Clin Endocrinol Metab. (1979). 49:346–9. doi: 10.1210/jcem-49-3-346
41. Caufriez A, Leproult R, L'Hermite-Baleriaux M, Moreno-Reyes R, Copinschi G. A potential role of endogenous progesterone in modulation of GH, prolactin and thyrotrophin secretion during normal menstrual cycle. Clin Endocrinol. (2009) 71:535–42. doi: 10.1111/j.1365-2265.2009.03539.x
42. Childs E, Dlugos A, De Wit H. Cardiovascular, hormonal, and emotional responses to the TSST in relation to sex and menstrual cycle phase. Psychophysiology. (2010) 47:550–9. doi: 10.1111/j.1469-8986.2009.00961.x
43. Collins A, Eneroth P, Landgren BM. Psychoneuroendocrine stress responses and mood as related to the menstrual cycle. Psychosom Med. (1985) 47:512–27. doi: 10.1097/00006842-198511000-00002
44. Genazzani AR, Lemarchand-Béraud T, Aubert ML, Felber JP. Pattern of plasma ACTH, hGH, and cortisol during menstrual cycle. J Clin Endocrinol Metab. (1975) 41:431–7. doi: 10.1210/jcem-41-3-431
45. Heitkemper M, Jarrett M, Cain K, Shaver J, Bond E, Woods NF, et al. Increased urine catecholamines and cortisol in women with irritable bowel syndrome. Am J Gastroenterol. (1996) 91:906–13.
46. Huang Y, Zhou R, Wu M, Wang Q, Zhao Y. Premenstrual syndrome is associated with blunted cortisol reactivity to the TSST. Stress. (2015) 18:160–8. doi: 10.3109/10253890.2014.999234
47. Judd SJ, Wong J, Saloniklis S, Maiden M, Yeap B, Filmer S, et al. The effect of alprazolam on serum cortisol and luteinizing hormone pulsatility in normal women and in women with stress-related anovulation. J Clin Endocrinol Metab. (1995) 80:818–23. doi: 10.1210/jcem.80.3.7883836
48. Kasa-Vubu JZ, Dimaraki EV, Young EA. The pattern of growth hormone secretion during the menstrual cycle in normal and depressed women. Clin Endocrinol. (2005) 62:656–60. doi: 10.1111/j.1365-2265.2005.02274.x
49. LeRoux A, Wright L, Perrot T, Rusak B. Impact of menstrual cycle phase on endocrine effects of partial sleep restriction in healthy women. Psychoneuroendocrinology. (2014) 49:34–46. doi: 10.1016/j.psyneuen.2014.06.002
50. Liu JH, Rasmussen DD, Rivier J, Vale W, Yen SS. Pituitary responses to synthetic corticotropin-releasing hormone: absence of modulatory effects by estrogen and progestin. Am J Obstet Gynecol. (1987) 157:1387–91. doi: 10.1016/S0002-9378(87)80229-6
51. Lombardi I, Luisi S, Quirici B, Monteleone P, Bernardi F, Liut M, et al. Adrenal response to adrenocorticotropic hormone stimulation in patients with premenstrual syndrome. Gynecol Endocrinol. (2004) 18:79–87. doi: 10.1080/09513590310001652955
52. Maki PM, Mordecai KL, Rubin LH, Sundermann E, Savarese A, Eatough E, et al. Menstrual cycle effects on cortisol responsivity and emotional retrieval following a psychosocial stressor. Horm Behav. (2015) 74:201–8. doi: 10.1016/j.yhbeh.2015.06.023
53. Ohara K, Okita Y, Kouda K, Mase T, Miyawaki C, Nakamura H. Cardiovascular response to short-term fasting in menstrual phases in young women: an observational study. BMC Womens Health. (2015) 15:67. doi: 10.1186/s12905-015-0224-z
54. Paoletti AM, Romagnino S, Contu R, Orru MM, Marotto MF, Zedda P, et al. Observational study on the stability of the psychological status during normal pregnancy and increased blood levels of neuroactive steroids with GABA-A receptor agonist activity. Psychoneuroendocrinology. (2006) 31:485–92. doi: 10.1016/j.psyneuen.2005.11.006
55. Parry BL, Javeed S, Laughlin GA, Hauger R, Clopton P. Cortisol circadian rhythms during the menstrual cycle and with sleep deprivation in premenstrual dysphoric disorder and normal control subjects. Biol Psychiatry. (2000) 48:920–31. doi: 10.1016/S0006-3223(00)00876-3
56. Rasgon N, McGuire M, Tanavoli S, Fairbanks L, Rapkin A. Neuroendocrine response to an intravenous L-tryptophan challenge in women with premenstrual syndrome. Fertil Steril. (2000) 73:144–9. doi: 10.1016/S0015-0282(99)00452-5
57. Reynolds TA, Makhanova A, Marcinkowska UM, Jasienska G, McNulty JK, Eckel LA, et al. Progesterone and women's anxiety across the menstrual cycle. Horm Behav. (2018) 102:34–40. doi: 10.1016/j.yhbeh.2018.04.008
58. Roche DJ, King AC. Sex differences in acute hormonal and subjective response to naltrexone: the impact of menstrual cycle phase. Psychoneuroendocrinology. (2015) 52:59–71. doi: 10.1016/j.psyneuen.2014.10.013
59. Stewart PM, Penn R, Holder R, Parton A, Ratcliffe JG, London DR. The hypothalamo-pituitary-adrenal axis across the normal menstrual cycle and in polycystic ovary syndrome. Clin Endocrinol. (1993) 38:387–91. doi: 10.1111/j.1365-2265.1993.tb00519.x
60. Su TP, Schmidt PJ, Danaceau M, Murphy DL, Rubinow DR. Effect of menstrual cycle phase on neuroendocrine and behavioral responses to the serotonin agonist M-chlorophenylpiperazine in women with premenstrual syndrome and controls. J Clin Endocrinol Metab. (1997) 82:1220–8. doi: 10.1210/jc.82.4.1220
61. Tulenheimo A, Laatikainen T, Salminen K. Plasma Beta-Endorphin Immunoreactivity in Premenstrual Tension. Br J Obstet Gynaecol. (1987) 94:26–9. doi: 10.1111/j.1471-0528.1987.tb02247.x
62. Villada C, Espin L, Hidalgo V, Rubagotti S, Sgoifo A, Salvador A. The influence of coping strategies and behavior on the physiological response to social stress in women: the role of age and menstrual cycle phase. Physiol Behav. (2017) 170:37–46. doi: 10.1016/j.physbeh.2016.12.011
63. Wolfram M, Bellingrath S, Kudielka BM. The cortisol awakening response (CAR) across the female menstrual cycle. Psychoneuroendocrinology. (2011) 36:905–12. doi: 10.1016/j.psyneuen.2010.12.006
64. Suzuki S, Handa RJ. Estrogen receptor-beta, but not estrogen receptor-alpha, is expressed in prolactin neurons of the female rat paraventricular and supraoptic nuclei: comparison with other neuropeptides. J Comp Neurol. (2005) 484:28–42. doi: 10.1002/cne.20457
65. Simerly RB, Chang C, Muramatsu M, Swanson LW. Distribution of androgen and estrogen receptor mRNA-containing cells in the rat brain: an in situ hybridization study. J Comp Neurol. (1990) 294:76–95. doi: 10.1002/cne.902940107
66. Estacio MA, Tsukamura H, Yamada S, Tsukahara S, Hirunagi K, Maeda K. Vagus nerve mediates the increase in estrogen receptors in the hypothalamic paraventricular nucleus and nucleus of the solitary tract during fasting in ovariectomized rats. Neurosci Lett. (1996) 208:25–8. doi: 10.1016/0304-3940(96)12534-9
67. Somponpun SJ, Sladek CD. Osmotic regulation of estrogen receptor-beta in rat vasopressin and oxytocin neurons. J Neurosci. (2003) 23:4261–9. doi: 10.1523/JNEUROSCI.23-10-04261.2003
68. Hrabovszky E, Kalló I, Hajszán T, Shughrue PJ, Merchenthaler I, Liposits Z. Expression of estrogen receptor-beta messenger ribonucleic acid in oxytocin and vasopressin neurons of the rat supraoptic and paraventricular nuclei. Endocrinology. (1998) 139:2600–4. doi: 10.1210/endo.139.5.6024
69. Alves SE, Lopez V, McEweb BS, Weiland NG. Differential colocalization of estrogen receptor b (ER b) with oxytocin and vasopressin in the paraventricular and supraoptic nuclei of the female rat brain: An immunocytochemical study. Proc Natl Acad Sci USA. (1998) 95:3281–6. doi: 10.1073/pnas.95.6.3281
70. Lund TD, Hinds LR, Handa RJ. The androgen 5alpha-dihydrotestosterone and its metabolite 5alpha-androstan-3beta, 17beta-diol inhibit the hypothalamo-pituitary-adrenal response to stress by acting through estrogen receptor beta-expressing neurons in the hypothalamus. J Neurosci. (2006) 26:1448–56. doi: 10.1523/JNEUROSCI.3777-05.2006
71. Weiser MJ, Handa RJ. Estrogen impairs glucocorticoid dependent negative feedback on the hypothalamic-pituitary-adrenal axis via estrogen receptor alpha within the hypothalamus. Neuroscience. (2009) 159:883–95. doi: 10.1016/j.neuroscience.2008.12.058
72. Gaskin JH, Kitay JI. Adrenocortical function in the hamster. Sex differences and effects of gonadal hormones. Endocrinology. (1970) 87:779–86. doi: 10.1210/endo-87-4-779
73. Burgess LH, Handa RJ. Chronic estrogen-induced alterations in adrenocorticotropin and corticosterone secretion, and glucocorticoid receptor-mediated functions in female rats. Endocrinology. (1992) 131:1261–9. doi: 10.1210/endo.131.3.1324155
74. Ochedalski T, Subburaju S, Wynn PC, Aguilera G. Interaction between oestrogen and oxytocin on hypothalamic-pituitary-adrenal axis activity. J Neuroendocrinol. (2007) 19:189–97. doi: 10.1111/j.1365-2826.2006.01525.x
75. Ter Horst GJ, Wichmann R, Gerrits M, Westenbroek C, Lin Y. Sex differences in stress responses: focus on ovarian hormones. Physiol Behav. (2009) 97:239–49. doi: 10.1016/j.physbeh.2009.02.036
76. Boudaba C, Szabo K, Tasker JG. Physiological mapping of local inhibitory inputs to the hypothalamic paraventricular nucleus. J Neurosci. (1996) 16:7151–60. doi: 10.1523/JNEUROSCI.16-22-07151.1996
77. Brunton PJ, McKay AJ, Ochedalski T, Piastowska A, Rebas E, Lachowicz A, et al. Central opioid inhibition of neuroendocrine stress responses in pregnancy in the rat is induced by the neurosteroid allopregnanolone. J Neurosci. (2009) 29:6449–60. doi: 10.1523/JNEUROSCI.0708-09.2009
78. Gunn BG, Cunningham L, Cooper MA, Corteen NL, Seifi M, Swinny JD, et al. Dysfunctional astrocytic and synaptic regulation of hypothalamic glutamatergic transmission in a mouse model of early-life adversity: relevance to neurosteroids and programming of the stress response. J Neurosci. (2013) 33:19534–54. doi: 10.1523/JNEUROSCI.1337-13.2013
79. Biggio G, Pisu MG, Biggio F, Serra M. Allopregnanolone modulation of HPA axis function in the adult rat. Psychopharmacology. (2014) 231:3437–44. doi: 10.1007/s00213-014-3521-6
80. Sarkar J, Wakefield S, MacKenzie G, Moss SJ, Maguire J. Neurosteroidogenesis is required for the physiological response to stress: role of neurosteroid-sensitive GABAA receptors. J Neurosci. (2011) 31:18198–210. doi: 10.1523/JNEUROSCI.2560-11.2011
81. Misztal T, Mlotkowska P, Marciniak E, Misztal A. Allopregnanolone reduces neuroendocrine response to acute stressful stimuli in sheep. J Endocrinol. (2020) 244:201–11. doi: 10.1530/JOE-19-0376
82. Owens MJ, Ritchie JC, Nemeroff CB. 5a-Pregnane-3a,21-diol-20-one (THDOC) attenuates mild stress-induced increases in plasma corticosterone via a non-glucocorticoid mechanism: comparison with alprazolam. Brain Res. (1992) 573:353–5. doi: 10.1016/0006-8993(92)90788-B
83. Patchev VK, Hassan AH, Holsboer DF, Almeida OF. The neurosteroid tetrahydroprogesterone attenuates the endocrine response to stress and exerts glucocorticoid-like effects on vasopressin gene transcription in the rat hypothalamus. Neuropsychopharmacology. (1996) 15:533–40. doi: 10.1016/S0893-133X(96)00096-6
84. Siiteri PK, Murai JT, Hammond GL, Nisker JA, Raymoure WJ, Kuhn RW. The serum transport of steroid hormones. Recent Prog Horm Res. (1982) 38:457–510. doi: 10.1016/B978-0-12-571138-8.50016-0
85. Coolens JL, Van Baelen H, Heyns W. Clinical use of unbound plasma cortisol as calculated from total cortisol and corticosteroid-binding globulin. J Steroid Biochem. (1987) 26:197–202. doi: 10.1016/0022-4731(87)90071-9
86. Vinning RF, McGinley RA, Maksvytis J, Ho KY. Salivary cortisol: a better measure ofadrenal cortical function than serum cortisol. Ann Clin Biochem. (1983) 20:329–35. doi: 10.1177/000456328302000601
87. Westphal U. Steroid-protein interactions. Monogr Endocrinol. (1971) 4:1–567. doi: 10.1007/978-3-642-46262-7_1
88. Moore DE, Kawagoe S, Davajan V, Nakamura RM, Mishell DR. An in vivo system in man for quantitation of estrogenicity. II Pharmacologic changes in binding capacity of serum corticosteroid-binding globulin induced by conjugated estrogens, mestranol, and ethinyl estradiol. Am J Obstet Gynecol. (1978) 130:482–6. doi: 10.1016/0002-9378(78)90294-6
89. Jeyakumar M, Carlson KE, Gunther JR, Katzenellenbogen JA. Exploration of dimensions of estrogen potency: parsing ligand binding and coactivator binding affinities. J Biol Chem. (2011) 286:12971–82. doi: 10.1074/jbc.M110.205112
90. Nenke MA, Zeng A, Meyer EJ, Lewis JG, Rankin W, Johnston J, et al. Differential effects of estrogen on corticosteroid-binding globulin forms suggests reduced cleavage in pregnancy. J Endocr Soc. (2017) 1:202–10. doi: 10.1210/js.2016-1094
91. Herrera AY, Faude S, Nielsen SE, Locke M, Mather M. Effects of hormonal contraceptive phase and progestin generation on stress-induced cortisol and progesterone release. Neurobiol Stress. (2019) 10:100151. doi: 10.1016/j.ynstr.2019.100151
92. Hampson E. A brief guide to the menstrual cycle and oral contraceptive use for researchers in behavioral endocrinology. Horm Behav. (2019) 119:104655. doi: 10.1016/j.yhbeh.2019.104655
93. Daskalakis NP, Cohen H, Nievergelt CM, Baker DG, Buxbaum JD, Russo SJ, et al. New translational perspectives for blood-based biomarkers of PTSD: From glucocorticoid to immune mediators of stress susceptibility. Exp Neurol. (2016) 284:133–40. doi: 10.1016/j.expneurol.2016.07.024
94. Plotsky PM, Owens MJ, Nemeroff CB. Psychoneuroendocrinology of depression. Hypothalamic-pituitary-adrenal axis. Psychiatr Clin North Am. (1998) 21:293–307. doi: 10.1016/S0193-953X(05)70006-X
95. Sze Y, Brunton PJ. Sex, stress and steroids. Eur J Neurosci. (2019). doi: 10.1111/ejn.14615. [Epub ahead of print].
96. Altemus M, Sarvaiya N, Neill Epperson C. Sex differences in anxiety and depression clinical perspectives. Front Neuroendocrinol. (2014) 35:320–30. doi: 10.1016/j.yfrne.2014.05.004
Keywords: cortisol, hypothalamic-pituitary-gonadal (HPG) axis, hypothalamic-pituitary-adrenal (HPA) axis, menstrual cycle, follicular, luteal
Citation: Hamidovic A, Karapetyan K, Serdarevic F, Choi SH, Eisenlohr-Moul T and Pinna G (2020) Higher Circulating Cortisol in the Follicular vs. Luteal Phase of the Menstrual Cycle: A Meta-Analysis. Front. Endocrinol. 11:311. doi: 10.3389/fendo.2020.00311
Received: 10 February 2020; Accepted: 24 April 2020;
Published: 02 June 2020.
Edited by:
Deborah Suchecki, Federal University of São Paulo, BrazilReviewed by:
William R. Lovallo, Independent Researcher, Oklahoma City, United StatesRichard G. Hunter, University of Massachusetts Boston, United States
Copyright © 2020 Hamidovic, Karapetyan, Serdarevic, Choi, Eisenlohr-Moul and Pinna. This is an open-access article distributed under the terms of the Creative Commons Attribution License (CC BY). The use, distribution or reproduction in other forums is permitted, provided the original author(s) and the copyright owner(s) are credited and that the original publication in this journal is cited, in accordance with accepted academic practice. No use, distribution or reproduction is permitted which does not comply with these terms.
*Correspondence: Ajna Hamidovic, ahamidov@uic.edu