- Department of Physiology, Medical University of Bialystok, Bialystok, Poland
Currently, an increasing number of diseases related to insulin resistance and obesity is an alarming problem worldwide. It is well-known that the above states can lead to the development of type 2 diabetes, hypertension, and cardiovascular diseases. An excessive amount of triacylglycerols (TAGs) in a diet also evokes adipocyte hyperplasia and subsequent accumulation of lipids in peripheral organs (liver, cardiac muscle). Therefore, new therapeutic methods are constantly sought for the prevention, treatment and alleviation of symptoms of the above mentioned diseases. Currently, much attention is paid to Cannabis derivatives—phytocannabinoids, which interact with the endocannabinoid system (ECS) constituents. Δ9-tetrahydrocannabinol (Δ9-THC) and cannabidiol (CBD) are the most abundant compounds of Cannabis plants and their therapeutic application has been suggested. CBD is considered as a potential therapeutic agent due to its anti-inflammatory, anti-oxidant, anti-tumor, neuroprotective, and potential anti-obesity properties. Therefore, in this review, we especially highlight pharmacological properties of CBD as well as its impact on obesity in different tissues.
Introduction
A well-known ancient plant Cannabis sativa has been a subject of scientific interest for over 50 years (1). Moreover, it has been used for recreational and medical purposes for thousands of years. The plant comprises about 100 phytocannabinoids, which are C21 terpenophenolic constituents (2). Nowadays, the most-studied phytocannabinoids are: Δ9- tetrahydrocannabinol (Δ9-THC), Δ9-tetrahydrocannabivarin (Δ9-THCV), cannabinol (CBN), cannabidiol (CBD), cannabidivarin (CBDV), cannabigerol (CBG), and cannabichromene (CBC) (1). So far, many studies have shown therapeutic properties of the above mentioned Cannabis compounds. Therefore, the aim of the current review is to focus on the emerging potential of CBD and other phytocannabinoids, which act as novel therapeutic agents in obesity treatment.
The Expanded Endocannabinoid System (ECs)
The canonical endocannabinoid system consists of the endocannabinoids (ECs), enzymes responsible for their production and metabolism as well as specific receptors (3). The most studied ECs, i.e., anandamide (AEA) and 2-arachidonoylglycerol (2-AG), are bioactive lipid mediators derived from long-chain polyunsaturated fatty acids (4, 5). It is known that they are released on demand and act through cannabinoid CB1 and CB2 receptors, which are G-protein-coupled receptors (GPCRs) (4–7). Interestingly, in the past the CB1 receptor was thought to be present only in the brain structures, whereas the CB2 receptor expression was limited to immune cells (8). However, recent research revealed that both CB1 and CB2 receptors are expressed in the brain and peripheral tissues, including the liver, skeletal muscle, heart, gut, bones, and adipose tissue (9–13). ECS mediators, AEA and 2-AG, are degraded by two enzymes: fatty acid amide hydrolase (FAAH) and monoacylglycerol lipase (MAGL), respectively (14). In addition the existence of many fatty acid-derived mediators, such as N-palmitoyl-, N-oleoyl-ethanolamine (PEA, OEA), 2-oleoyl-, 2-linoleoyl-glycerol (2-OG, 2-LG), and 2-arachidonoyl glyceryl ether (2-AGE, noladin ether), has been discovered recently (more than 100) (15, 16). These several congeners often share common molecular targets and are inactivated by the same enzymes as ECs. Among these novel targets we include some orphan GPCRs such as GPR55, thermosensitive transient receptor potential (TRP) channels and peroxisome proliferator-activated receptors α and γ (PPARα and PPARγ) (17). The aforementioned discoveries have extended the concept of this inner signaling system from ECS itself to the expanded ECS or endocannabinoidome (eCBome). The expanded ECS is involved in controlling various processes, including appetite, energy balance, metabolism, thermogenesis, inflammation, nociception as well as regulation of stress, and emotions (18). Recent data have shown that different phytocannabinoids interact with several components of endocannabinoidome by affecting either cannabinoid receptors (to a lesser extent), non-cannabinoid receptor targets and/or enzymes involved in the metabolism of endogenous ligands (11, 15). Hence, the interest on their regulatory mechanisms and a potent therapeutic action has risen greatly in the last few years.
Δ9-Tetrahydrocannabinol (Δ9-THC)
Δ9-THC is a major psychoactive constituent of Cannabis sativa (1). It was shown that Δ9-THC acts as a partial agonist of both CB1 and CB2 receptors (19). Throughout such activation Δ9-THC is able to trigger many different physiological processes, i.e., regulation of gastrointestinal, liver and cardiovascular functions, pain perception along with modulation of neurotransmitters release in the nervous system (20, 21). Now, it is clearly established that, Δ9-THC exerts a well-known psychoactive effect, which is mediated by the activation of CB1 receptor in the central nervous system (CNS) (22). Additionally, Δ9-THC by activating CB1 receptors, located in the limbic (enhancing motivational properties of food) and hypothalamic (increasing appetite) structures, causes the orexigenic effects (Figure 1) (23). Apart from the above implications, Δ9-THC has also the ability to bind to eCBome receptors including GPR55 (G protein-coupled receptor 55), 5- HT3A (serotonin receptor subunit), TRPV2, 3, 4 receptors (transient receptor potential channels of vanilloid type 2, 3, 4), which produce some of its pharmacological effects (22). Lauckner et al. revealed that Δ9-THC activates the GPR55 receptor in HEK293 and CHO cells, which resulted in increased intracellular calcium level (24). Another study conducted on HEK293 cells showed that Δ9-THC acts as a 5- HT3A receptor antagonist, whereas several reports demonstrated that Δ9-THC has agonistic effects on the TRPV2, 3, 4 channels (25–28). Furthermore, Δ9-THC was reported to exhibit a beneficial impact on the regulation of insulin sensitivity in the insulin resistant adipocytes. The authors have demonstrated that natural extract containing Δ9-THC decreased the TAGs content and improved the glucose uptake in the insulin resistant 3T3-L1 cells in a concentration-dependent manner (29). In the same study it was shown that the level of tumor necrosis factor alpha (TNF-α) in the 3T3-L1 cells was substantially decreased in the presence of Δ9-THC, which also improved sensitivity of the cells to insulin (29). The above mentioned studies also revealed that in a differentiated 3T3-L1 cells Δ9-THC treatment enhanced glucose transporter type 4 (GLUT4) and insulin receptor substrate 1 and 2 (IRS-2) gene expressions, which play key roles in the insulin signaling pathway (29). However, we have to keep in mind that in the above experimental models natural extract were applied and no information upon their purity was provided, therefore, it can be considered as the limitation of such studies in the face of stating pharmacological properties of examined substances.
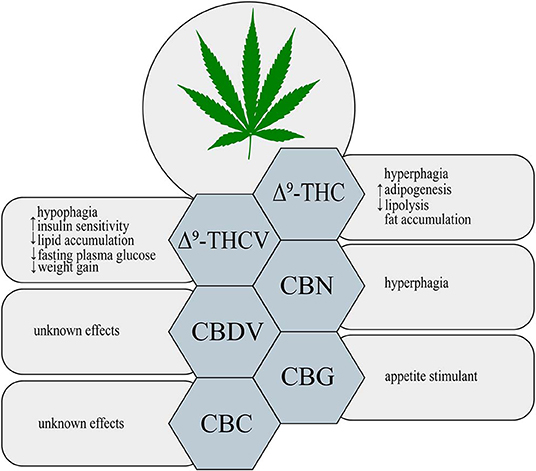
Figure 1. Metabolic effects of different phytocannabinoids. ↑, increase; ↓, decrease; Δ9-THC, Δ9- tetrahydrocannabinol; Δ9-THCV, Δ9-tetrahydrocannabivarin; CBN, cannabinol; CBDV, cannabidivarin; CBG, cannabigerol; CBC, cannabichromene.
On the other hand, in comparison to the well-documented orexigenic effect of Δ9-THC, many studies have also shown a potential anti-obesity effect of this phytocannabinoid derivative. Cluny et al. (30) using the in vivo model have demonstrated that chronic administration of Δ9-THC to diet-induced obesity (DIO) mice for 3 and 4 weeks treatment (intraperitoneal injections of Δ9-THC in a dose of 2 or 4 mg/kg and vehicle) prevented weight gain due to reduced energy intake (30). This effect might have been associated with the fact that Δ9-THC is a partial agonist of CB1 and CB2 receptors. Since, Δ9-THC does not produce maximal stimulation of the above receptors, the background of such changes could result from blocking endogenous full agonist, i.e., anandamide from binding to CB1 receptor, especially, when the endocannabinoid tone is high (e.g., obesity) (30, 31). However, potential positive influence of Δ9-THC in the prevention and treatment of obesity requires further investigation.
Δ9-Tetrahydrocannabivarin (Δ9-THCV)
Δ9-THCV is a propyl Δ9-THC analog, which binds to the cannabinoid CB1 and CB2 receptors (32). So far, data indicate that Δ9-THCV is a neutral antagonist at low doses and agonist at high doses of CB1 and CB2 receptors (2, 32, 33). Little evidence exists upon the activation of various eCBome receptors by Δ9-THCV. Nevertheless, it has been established that Δ9-THCV acts as an agonist of human TRPV1, rat TRP channels of ankyrin type-1 (TRPA1) and TRPV2, 3, 4 channels as well as an antagonist of rat's TRP channel of melastatin type-8 (TRPM8) (26). In one study it was demonstrated that Δ9-THCV activated 5-HT1A receptor in the rat brain and human 5-HT1A receptor-transfected CHO (Chinese hamster ovary) cells, and thereby exerted antipsychotic effects (34). Interestingly, recent research has shown that this non-psychotropic phytocannabinoid has a potential therapeutic effect in the treatment of diabetes associated with obesity. Wargent et al. (35) revealed that Δ9-THCV increased insulin sensitivity and improved glucose tolerance in DIO mice as well as genetically obese ob/ob mice (Figure 1). However, it did not substantially affect food intake and body weight gain in the above models, whereas body fat content was decreased (35). In the same experiment, the authors investigated the impact of Δ9-THCV on the insulin resistant C2C12 myotubes and HL-5 hepatocytes showing that Δ9-THCV restored intracellular insulin signaling pathway (35). Other studies have also displayed that Δ9-THCV (1–10 μM) decreased lipid accumulation in the in vitro model of hepatosteatosis in HHL-5 (Human Hepatocyte Line 5) cells and adipocytes (3T3-L1 cells) (36). According to the above results, a pilot study in patients with type 2 diabetes showed that Δ9-THCV reduced fasting plasma glucose with parallel improvement in β-cell function as well as increased Apo A (apolipoprotein A) and adiponectin concentrations compared with placebo, which was well-tolerated in patients (37). Nonetheless, further research is necessary to confirm the potential therapeutic properties of Δ9-THCV in the treatment of obesity, metabolic syndrome and type 2 diabetes.
Cannabinol (CBN)
An oxidized metabolite of Δ9-THC, cannabinol, was isolated in 1896 by Wood and colleagues (38). CBN is a non-enzymatic oxidative breakdown product of Δ9-THC due to aging or light exposure (32). It was shown that CBN binds to cannabinoid receptors CB1 and CB2 in the peripheral organs and central nervous system (CNS), and therefore, it exerts a weak psychoactive activity (39). CBN also displays the ability to bind to expanded ECS receptors including agonistic and antagonistic effects on TRPA1 and TRPM8 channels, respectively (26). So far, studies focusing on the impact of CBN on obesity and feeding behavior have been limited. Only in one of the recent studies it has been demonstrated that CBN increased food intake in rats in a dose-dependent manner (Figure 1) (40). Probably, the hyperphagic CBN's effect was induced by its interaction with CB1 receptor in CNS (40) but it should be confirmed by additional experiments including for example gene silencing or knockout animals. Nevertheless, further experiments are required to completely characterize the role of CBN in food consumption and body weight control.
Cannabidivarin (CBDV)
Among phytocannabinoids we can find a propyl analog of CBD, cannabidivarin, which exerts non-psychotropic properties (32). CBDV has a weak affinity for cannabinoid receptors CB1 and CB2 with simultaneous stronger activation of other molecular targets related with the ECS (41). Predominantly, anticonvulsant and other therapeutic CBDV's properties are associated with activation of TRP channels (TRPV1, 2 and TRPA1) and TRPM8 inhibition (26, 42). Another pharmacological target for CBDV is GPR55 receptor, where observed effect is inhibitory (43). However, currently there is insufficient research on the ground of CBDV's effects on obesity, insulin resistance and other metabolic disturbances (Figure 1).
Cannabigerol (CBG)
Another phytocannabinoid, which lacks psychotropic properties and occurs only in trace amounts in Cannabis, is cannabigerol (41). Cannabis plants produce cannabigerolic acid (CBGA), which is a direct precursor of cannabinoids: Δ9-THC, CBC and CBD (44, 45). Preliminary studies, using plasma membranes isolated from the CHO and HEK-293 cells expressing human CB1 and CB2 receptors, have shown that CBG acts as a partial agonist of both cannabinoid receptors (46). Similarly to other phytocannabinoids CBG has an ability to interact with endocannabinoidome receptors, such as TRPV1, 2, TRPA1, and TRPM8 channels (26). Moreover, one study has provided for the first time evidence upon activation of α2-adrenoreceptors and 5-HT1A receptor blockage by CBG in the vas deferens and brain, respectively (47). Furthermore, recent investigation has revealed that CBG serves as a novel appetite stimulant in rats and importantly, no side effects were observed during its administration (Figure 1) (48). However, extensive research is necessary to determine a more detailed mechanisms of CBG action with respect to metabolic diseases.
Cannabichromene (CBC)
The discovery of cannabichromene was reported by Izzo et al. (32). It is a minor component of Cannabis plant, which presents low affinity for CB1 and CB2 receptors (49, 50). Nonetheless, researchers discovered another molecular target of CBC, i.e., TRP channels. De Petrocellis et al. revealed that CBC is the most potent agonist of the TRPA1 channels (26). However, it has also the ability to activate in a lower potency, TRPV3 and TRPV4 channels, and additionally inhibits TRPM8 receptors, but to a much lesser extent (26). Although CBC exhibits different medical properties, its influence on metabolism and obesity has not been found, yet (Figure 1).
Cannabidiol (CBD)
Cannabidiol (CBD) is one of the most common non-psychotropic constituent of Cannabis plant (this depends on the cannabis strain). It was revealed that CBD induces a wide-range of pharmacological effects through different mechanisms. CBD functions as a negative allosteric modulator of CB1 receptor (51). Therefore, it has therapeutic potential in the treatment of the central nervous system diseases (neurodegenerative diseases, epilepsy, anxiety, and depression) without concurrent psychotic side effects (2, 52, 53). Furthermore, it was indicated that CBD is able to block CB1 receptor, thereby producing anti-obesity effects. Otherwise, CBD unexpectedly exhibited a high affinity for CB2 receptor as an agonist or inverse agonist depending on the research model (in vitro or in vivo) (54, 55). An interesting result of the latest studies was the fact that CBD has greater affinity for various eCBome receptors, including GPR55, α1-adrenoreceptors, 5-HT1A, TRPV channels and PPARγ (Figure 2) (19, 26, 42, 56, 57). Hegde et al. as well as Esposito et al. reported that CBD significantly induced the transcriptional activity of PPARγ (58, 59). It is known that PPARγ is an interesting therapeutic target due to its crucial role in regulating glucose homeostasis, lipoprotein metabolism, and inflammation (60, 61).
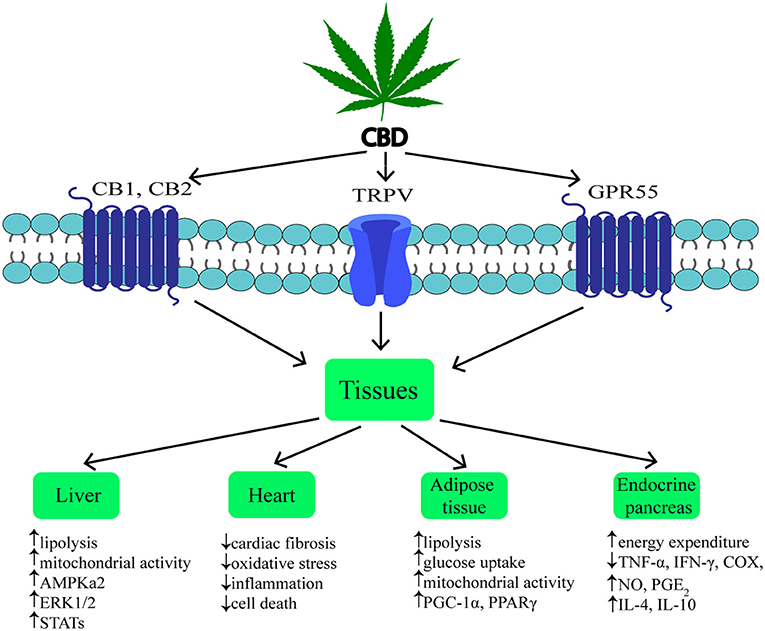
Figure 2. Mechanism and effects of CBD action on various tissues during obesity. ↑, increase; ↓, decrease; CBD, cannabidiol; CB1, cannabinoid receptor 1; CB2, cannabinoid receptor 2; TRPV1, transient receptor potential channel of vanilloid type 1; GPR55, G protein coupled receptor 55; AMPKa2, catalytic subunit of AMP-activated protein kinase; ERK1/2, extracellular signal-regulated kinase; STATs, signal transducers and activators of transcription; PGC-1α, peroxisome proliferator-activated receptor gamma coactivator 1-alpha; PPARγ, peroxisome proliferator-activated receptor gamma; TNF-α, tumor necrosis factor alpha; IFN-γ, interferon gamma; COX, cyclooxygenase; NO, nitrogen oxide; PGE2, prostaglandin E2; IL-4, interleukin 4; IL-10, interleukin 10.
Moreover, recent research has focused on the therapeutic properties of CBD, including anti-inflammatory, anti-oxidant, anti-tumor, anti-convulsant and neuroprotective effects (62, 63). In the light of the above mentioned properties, CBD emerges as a potential therapeutic agent, which can be used in the treatment of diabetes and its complications, obesity, ischemia, neurodegenerative diseases as well as pain relieving and depression. Although CBD's anti-inflammatory and neuroprotective properties are well-confirmed, there are few studies that have investigated anti-obesity effects of this compound. Wierucka-Rybak et al. have examined the effect of CBD on food intake, food preferences and weight gain in rats (64). The authors administered CBD (3 mg/kg) for 3 days to rats maintained on a standard diet (SD), high fat diet (HFD), or free choice diet (FC; high sucrose). The authors revealed that CBD injections in the case of rats being on the HFD resulted in an increase in body weight despite significantly reduced food intake. On the other hand, in rats fed FC diet CBD did not cause significant change in food consumption and body weight (64). Previous studies investigating the impact of CBD on food intake showed contradictory results. One study demonstrated CBD-induced (2.5 and 5 mg/kg) decrease in body weight gain in rats (54), while other studies have shown no significant impact on food intake and body weight in mice and rats (65, 66). Importantly, pilot studies were conducted to investigate the effect of CBD on glycemic and lipid parameters in patients with type 2 diabetes (37). The findings from these studies demonstrated that CBD did not produce any improvement in glycemic and lipid control despite producing eligible changes in gut hormones (GIP—glucose dependent insulinotropic peptide) and adipokines (resistin) concentrations (37). Probably, the reason for the lack of therapeutic effects seen during CBD administration could have been too low dosage used in the study. The anti-epileptic properties of CBD were investigated in many preclinical studies and in randomized trials (67–71). Data obtained from these studies confirmed CBD's anti-seizure properties along with a good tolerance profile. Currently, the first cannabis based medicine containing CBD/Δ9-THC combination for the multiple sclerosis related spasticity treatment (Sativex/Nabiximols; GW Pharmaceuticals) has been approved in numerous countries, including Australia, Canada, New Zealand and in most European Union countries (72). Furthermore, a drug composed solely of CBD (Epidiolex; GW Pharmaceuticals) has been approved in June 2018 in the United States for the treatment of Dravet and Lennox-Gastaut syndromes in children (52, 73, 74).
Overactivation of the ECS in Obesity
Obesity and coexisting disorders such as insulin resistance, hypertension, and hypertriglyceridemia lead to the development of metabolic syndrome and type 2 diabetes (75). Whenever, the excessive fatty acids (FAs) consumption takes place, simultaneously we can observe an increased differentiation of pre-adipocytes to mature adipocytes with subsequent stimulation of their growth as well (76, 77). Over time, at a later stage of obesity development, adipocytes are overloaded, which results in the accumulation of lipids in other tissues such as liver, skeletal, and cardiac muscles (60, 78). Paralell excessive fat accumulation can be observed in the liver or cardiac muscle, which contributes to the development of liver steatosis and cardiomyopathy, respectively (78). Many scientists are looking for new therapeutic strategies, including expanded ECS, which can be a useful tool in preventing and treating the above mentioned diseases. Therefore, the components of eCBome are emerging as potent therapeutic targets due to their well-established role in the regulation of food consumption and energy balance as well as lipid and glucose metabolism (79, 80). Literature data indicated, that ECS is upregulated during obesity and associated diseases (81–84). It is well-confirmed that the level of endogenous cannabinoids in the above mentioned conditions is increased, i.e., in CNS, adipose tissue, pancreas, skeletal muscle, kidney, liver, and blood of obese rodents and humans (76, 84–87). The cause of such ECS overactivation may be due to enhanced synthesis of ECs or their reduced degradation as well as overexpression of the cannabinoid receptors (88, 89). Various studies have shown upregulated levels of 2-AG in both different organs and serum during obesity and hyperglycemia, which was correlated with body fat content, visceral fat mass and fasting plasma triacylglyceride and insulin concentrations (84, 86, 87, 90). On the other hand, the reverse situation was described for ECs in the liver of DIO mice, where the hepatic levels of AEA were increased in animals fed HFD, while no significant difference in 2-AG liver levels was observed (91). Accordingly, Kimberly et al. revealed substantial association between AEA level and body mass index (BMI) value, which was an argument for making it a biomarker of NASH (non-alcoholic steatohepatitis) (92). In contrast with the above results, other studies have shown that patients with NAFLD (non-alcoholic fatty liver disease) had significantly increased levels of 2-AG without any change in AEA levels (93). Hence, it has been proposed to attenuate overactivation of ECS as a new approach for the treatment of obesity and its coexisting disorders. Such mechanism was used by researchers to create an anti-obesity drug (rimonabant; SR141716A), which was the first selective antagonist of CB1 receptors expressed in the brain and different peripheral organs/tissues controlling energetic homeostasis of the body (liver, muscle, adipose tissue, etc.) (94–96). Several studies have confirmed the beneficial effect of rimonabant on cardiometabolic risk markers, body weight as well as lipid and glucose parameters (97–99). However, rimonabant (Acomplia® Sanofi-Aventis) treatment turned out to be harmful so that it was forbidden in 2009 due to its adverse psychotropic side effects (100). This contributed to the invention of peripherally restricted CB1 receptor antagonists with limited brain penetration. Many studies investigating the effects of these antagonists (inverse agonists), such as AM6545, JD5037, have shown their positive effects against obesity in preclinical studies (101–103). For instance, AM6545 (10 mg/kg per day) treatment in DIO and genetically obese (ob/ob) mice attenuated obesity-related glucose intolerance, insulin resistance, dyslipidemia and reversed hepatic steatosis (104). Accordingly, Tam et al. demonstrated the hypophagic and weight-reducing effects of JD5037 (3 mg/kg per day, 7 days) in DIO mice but not in ob/ob and db/db mice, indicating leptin-dependent action (102). Importantly, JD5037 treatment resulted in the attenuation of hyperglycemia, hyperinsulinemia, insulin resistance, and reduction of hepatic triacylglycerols in all the above mentioned strains (102). We can conclude that peripherally restricted CB1 receptor antagonists have great therapeutic potential in the treatment of obesity.
Specific Sites of CBD Actions Related to Obesity
Liver
In view of the increasing prevalence of obesity, type 2 diabetes and metabolic syndrome, the development of liver diseases occurs more often. The above mentioned diseases are associated with excessive fat accumulation resulting from upregulated FA influx and de novo lipid synthesis in different cells, e.g., hepatocytes (105). Alterations in the hepatic fatty acid oxidation are highly related with the aforementioned metabolic disturbances, which were shown in many experimental models together with discrepancies in this field. Studies conducted on NASH and liver steatosis patients showed an increase (106, 107), decrease (108), or no change (109) in the fatty acid oxidation status. On the other hand, the expected consequence of lipid accumulation in the liver would be simultaneous enhancement in hepatic oxidation of fatty acids, thus, it seems that certain factors can also influence this process as well.
In previous studies it was shown that, hepatocytes produce endocannabinoids, AEA and 2-AG, and possess CB1 and CB2 receptors (80, 91). A study carried out by Liu et al. revealed that liver specific CB1 receptor knockout mice were protected from the development of both insulin resistance and hepatosteatosis but not obesity (55). Silvestri et al. revealed positive influence of CBD on the liver, namely the reduction of intracellular lipid content in the in vitro hepatosteatosis model, possibly by enhancing lipolysis and mitochondrial activity through increased fatty acids oxidation (36). The authors have shown that CBD increases the expression of selected proteins involved in upregulating lipid metabolism, i.e., catalytic subunit of 5′AMP-activated protein kinase (AMPKa2), extracellular signal-regulated kinase (ERK1/2) along with signal transducers and transcription activators (STATs) in hepatocytes (Figure 2, Table 1) (36). Concomitantly, the authors observed that CBD enhanced the level of glutathione (GSH), adenosine triphosphate (ATP), and nicotinamide adenine dinucleotide (NAD), which supported the assumption that CBD increases intracellular lipolysis and mitochondrial activity (36). Additionally, the study carried out by Wang's research group (119) indicated, in a murine model, an impact of CBD on alcohol-induced liver injury. The obtained results displayed that CBD attenuates liver steatosis (decreased triacylglyceride and fat droplet accumulation), inflammatory response [reduced the alcohol-feeding induced mRNA expressions of interleukin 1beta (IL1β), TNF-α and monocyte chemoattractant protein 1 (MCP1)], oxidative/nitrative stress (reduced lipid peroxidation, expression of reactive oxygen species generating enzyme—NADPH oxidase 2 (NOX2) and 3-nitrotyrosine production) and neutrophil infiltration in the liver (Table 1). This also confirmed the well-known, whole body anti-inflammatory and antioxidant properties of CBD (119).
Adipose Tissue
Obesity is associated with chronic low-grade inflammatory state and excessive fat accumulation (120). The expression of CB1 and CB2 receptors, other eCBome molecular targets (i.e., TRPV1,GPR55) and ECs enzymes level in the visceral and subcutaneous adipose tissue has been found (121, 122). These additional receptors are the most promising target for major non-psychogenic constituent of Cannabis plant, which has well-established anti-inflammatory effects and potential anti-obesity properties (123). Silvestri et al. (36) pointed out that CBD, depending on the time and dose, reduced triacylglycerols accumulation in 3T3-L1 adipocytes treated with oleic acid (OA). These results emphasize a potential role of CBD on lipolysis induction (Table 1). However, more research is necessary to investigate the exact mechanism of this phenomenon (36). Similarly, Ramlugon et al. (124) revealed that CBD treatment, in a time-dependent manner, induced the mitochondrial activation and increased oxygen consumption, which may be an explanation for the reduced fat accumulation in adipocytes despite of increased glucose uptake (Figure 2) (124). Additionally, recent research has shown that CBD inhibited weight gain in rats subjected to high fat diet (HFD) for 14 days and this effect was probably mediated by CB2 receptor (54). The authors confirmed the above effect by using a selective CB2 antagonist-AM630, which prevented the reduction in weight gain due to CBD treatment (54). However, additional studies are required to uncover the mechanisms by which CBD induces the above metabolic changes in adipocytes.
Endocrine Pancreas
The pancreas plays a pivotal role in the blood glucose homeostasis and whole body metabolism through insulin and glucagon secretion (125). In obesity, secretion of the above hormones is dysregulated. To date, the expression of cannabinoid receptors within the endocrine pancreas has been shown (114, 121). However, inconsistent data have been obtained regarding the expression of CB1 and CB2 receptors in the pancreas islet cells. Starowicz et al. (121) demonstrated the expression of CB1 receptor in glucagon-secreting α-cells of mice, while CB2 receptor was found inside mouse α-cells and insulin-secreting β-cells. Similarly, in the study conducted on human samples it was determined that CB1 receptor was present in glucagon-secreting α-cells and in a lesser extent in insulin-secreting β-cells, whereas CB2 was only located in somatostatin-secreting delta cells (114). On the other hand, other studies indicated that β-cells exhibited the expression of both cannabinoid receptors or only CB2 receptor (84, 115). Interestingly, Levendal et al. (126) investigated the effect of CBD on β-cell function in obese rats. The authors found that in a rat model of diet-induced obesity (DIO), cannabis extract treatment reduced the weight gain (mainly fat depots) and increased energy expenditure through upregulation of protein kinase B (PKB), mitochondrial uncoupling protein 2 (UCP2), and glucose transporter 2 (GLUT2) expression in pancreatic β-cells (rats were injected subcutaneously every second day for 28 days; the first five treatments containing an equivalence of 5 mg THC/kg body weight and the remaining treatments an equivalence of 2,5 mg THC/kg body weight) (126). Moreover, studies conducted by Weiss et al. have shown that CBD treatment (mice were administered 10–20 intraperitoneal injections at a dose of 5 mg/kg CBD) decreased the frequency of type 1 diabetes incidence and development in a model of non-obese diabetic (NOD) mice (116). Furthermore, the authors reported that CBD reduced plasma level of the pro-inflammatory cytokines, i.e., TNF-α and interferon gamma (IFN-γ) together with inflammation mediators, such as nitric oxide (NO), cyclooxygenase (COX), and prostaglandin E2 (PGE2). Whereas, anti-inflammatory cytokines—interleukins IL-4, IL-10 levels were increased (Figure 2, Table 1). Hence, reduced pancreas islets inflammation was observed in the histological examination as well as lower β-cell destruction (116). Concluding, it is likely that CBD has the ability to prevent and reduce the pancreatic damage associated with obesity and insulin resistance.
Cardiac Muscle
Chronic inflammatory state associated with obesity reduces the ability of the adipose tissue to store incoming plasma-borne fatty acids and leads to their accumulation in other organs, including cardiac muscle (127). Then, the local inflammatory state, oxidative stress and hyperinsulinemia can develop and progress induction of insulin resistance in the heart muscle (128). Currently, many studies are focused on the effect of CBD on the lipid and glucose metabolism in the heart, which is dysregulated during obesity and subsequently can lead to the cardiomyopathy and cardiac contractile dysfunction development (117). The impact of CBD on myocardial dysfunction, oxidative/nitrative stress, inflammation, and cell death has been recently investigated by Rajesh and coworkers (117). They used a primary human cardiomyocytes subjected to a high glucose concentration and a mouse model of type 1 diabetic cardiomyopathy. It was demonstrated that CBD reduced cardiac fibrosis, myocardial oxidative/ nitrative stress, inflammation and cell death in diabetic hearts (in the first set of experiments, 1 week diabetic mice were treated with CBD 1, 10 or 20 mg/kg intraperitoneal injections for 11 weeks; in the second set of experiments 8 weeks diabetic mice were treated with CBD for 4 weeks) (Figrue 2, Table 1) (117). These effects were mediated by decreased intracellular adhesion molecule 1 (ICAM-1), vascular cell adhesion molecule 1 (VCAM-1), and TNF-α expression as well as reduced mitogen-activated protein kinase (MAPK) and nuclear factor-κB (NFκB) activation. Moreover, CBD attenuated the high glucose-induced enhanced ROS (reactive oxygen species) generation and cell death in human cardiomyocytes (117). These findings highlight the role of CBD in the prevention or treatment of diabetic complications.
Conclusions
Overweight, insulin resistance and obesity emerged as leading health concerns all over the world. The above mentioned disturbances are characterized by excessive or abnormal fat accumulation, and are major risk factors for a number of chronic diseases, such as cardiovascular diseases, diabetes, and cancer. Currently, the non-psychotropic component of Cannabis sativa—CBD is in the center of interest, due to its well-established anti-inflammatory, anti-oxidant, anti-convulsant, anti-psychotic and potential anti-obesity properties. Many studies indicated that CBD affects both lipid and glucose metabolism through the action on various receptors as well as several metabolites. From the existing data, we can conclude that CBD has the promising potential as a therapeutic agent and might be effective in alleviating the symptoms of insulin resistance, type 2 diabetes and metabolic syndrome.
Author Contributions
PB participated in the design of the work, drafted the manuscript, prepared figures and tables, and approved final version submitted. EH-S helped to draft the manuscript and approved final version submitted. AC participated in the design of the study, revised manuscript, and approved final version submitted. All authors agreed to be accountable for all aspects of the work.
Funding
Publication financed under the Project No. POWR.03.02.00-00-I051/16 from European Union funds, PO WER 2014-2020 (Grant No. 01/IMSD/G/2019) and supported by the National Science Centre of Poland (Grant No. 2017/26/D/NZ3/01119) and Medical University of Bialystok (Grant No. N/ST/ZB/18/004/1118).
Conflict of Interest
The authors declare that the research was conducted in the absence of any commercial or financial relationships that could be construed as a potential conflict of interest.
References
1. Pertwee RG. Cannabinoid pharmacology: the first 66 years. Br J Pharmacol. (2006) 147(Suppl. 1):S163–71. doi: 10.1038/sj.bjp.0706406
2. Hill AJ, Williams CM, Whalley BJ, Stephens GJ. Phytocannabinoids as novel therapeutic agents in CNS disorders. Pharmacol Ther. (2012) 133:79–97. doi: 10.1016/j.pharmthera.2011.09.002
3. Silvestri C, Di Marzo V. The endocannabinoid system in energy homeostasis and the etiopathology of metabolic disorders. Cell Metab. (2013) 17:475–90. doi: 10.1016/j.cmet.2013.03.001
4. Lu HC, MacKie K. An introduction to the endogenous cannabinoid system. Biol Psychiatry. (2016) 79:516–25. doi: 10.1016/j.biopsych.2015.07.028
5. Di Marzo V. Endocannabinoids: synthesis and degradation. Rev Physiol Biochem Pharmacol. (2006) 160:1–24. doi: 10.1007/112_0505
6. Di Marzo V, Piscitelli F. The endocannabinoid system and its modulation by phytocannabinoids. Neurotherapeutics. (2015) 12:692–8. doi: 10.1007/s13311-015-0374-6
7. Wang J, Ueda N. Biology of endocannabinoid synthesis system. Prostaglandins Other Lipid Mediat. (2009) 89:112–9. doi: 10.1016/j.prostaglandins.2008.12.002
8. Mackie K, Stella N. Cannabinoid receptors and endocannabinoids: evidence for new players. AAPS J. (2006) 8:E298. doi: 10.1007/BF02854900
9. Mazier W, Saucisse N, Gatta-Cherifi B, Cota D. The endocannabinoid system: pivotal orchestrator of obesity and metabolic disease. Trends Endocrinol Metab. (2015) 26:524–537. doi: 10.1016/j.tem.2015.07.007
10. Engeli S, Jordan J. The endocannabinoid system: body weight and metabolic regulation. Clin Cornerstone. (2007) 8(Suppl 4):S24–35. doi: 10.1016/S1098-3597(06)80041-4
11. Pacher P, Bátkai S, Kunos G. The endocannabinoid system as an emerging target of pharmacotherapy. Pharmacol Rev. (2006) 58:389–462. doi: 10.1124/pr.58.3.2
12. Brusco A, Tagliaferro PA, Saez T, Onaivi ES. Ultrastructural localization of neuronal brain CB2 cannabinoid receptors. Ann N Y Acad Sci. (2008) 1139:450–7. doi: 10.1196/annals.1432.037
13. Gong JP, Onaivi ES, Ishiguro H, Liu QR, Tagliaferro PA, Brusco A, et al. Cannabinoid CB2 receptors: Immunohistochemical localization in rat brain. Brain Res. (2006) 1071:10–23. doi: 10.1016/j.brainres.2005.11.035
14. Clark TM, Jones JM, Hall AG, Tabner SA, Kmiec RL. Theoretical explanation for reduced body mass index and obesity rates in cannabis users . Cannabis Cannabinoid Res. (2018) 3:259–71. doi: 10.1089/can.2018.0045
15. Di Marzo V, Silvestri C. Lifestyle and metabolic syndrome: contribution of the endocannabinoidome. Nutrients. (2019) 11:1–24. doi: 10.3390/nu11081956
16. Ramer R, Schwarz R, Hinz B. Modulation of the endocannabinoid system as a potential anticancer strategy. Front Pharmacol. (2019) 10:430. doi: 10.3389/fphar.2019.00430
17. Veilleux A, Di Marzo V, Silvestri C. The expanded endocannabinoid system/endocannabinoidome as a potential target for treating diabetes mellitus. Curr Diab Rep. (2019) 19:117. doi: 10.1007/s11892-019-1248-9
18. Aizpurua-Olaizola O, Elezgarai I, Rico-Barrio I, Zarandona I, Etxebarria N, Usobiaga A. Targeting the endocannabinoid system: future therapeutic strategies. Drug Discov Today. (2017) 22:105–110. doi: 10.1016/j.drudis.2016.08.005
19. Pertwee RG. The diverse CB1 and CB2 receptor pharmacology of three plant cannabinoids: Δ9-tetrahydrocannabinol, cannabidiol and Δ9-tetrahydrocannabivarin. Br J Pharmacol. (2008) 153:199–215. doi: 10.1038/sj.bjp.0707442
20. Pisanti S, Malfitano AM, Ciaglia E, Lamberti A, Ranieri R, Cuomo G, et al. Cannabidiol: state of the art and new challenges for therapeutic applications. Pharmacol Ther. (2017) 175:133–50. doi: 10.1016/j.pharmthera.2017.02.041
21. Schlicker E, Kathmann M. Modulation of transmitter release via presynaptic cannabinoid receptors. Trends Pharmacol Sci. (2001) 22:565–72. doi: 10.1016/S0165-6147(00)01805-8
22. Morales P, Reggio PH. An update on non-CB 1, non-CB 2 cannabinoid related G-protein-coupled receptors. Cannabis Cannabinoid Res. (2017) 2:265–73. doi: 10.1089/can.2017.0036
23. Thorens B. Sensing of glucose in the brain. Handb Exp Pharmacol. (2012). 209:277–94. doi: 10.1007/978-3-642-24716-3_12
24. Lauckner JE, Jensen JB, Chen H-Y, Lu H-C, Hille B, Mackie K. GPR55 is a cannabinoid receptor that increases intracellular calcium and inhibits M current. Proc Natl Acad Sci USA. (2008) 105:2699–704. doi: 10.1073/pnas.0711278105
25. Barann M, Molderings G, Brüss M, Bönisch H, Urban BW, Göthert M. Direct inhibition by cannabinoids of human 5-HT3A receptors: probable involvement of an allosteric modulatory site. Br J Pharmacol. (2002) 137:589–96. doi: 10.1038/sj.bjp.0704829
26. De Petrocellis L, Ligresti A, Moriello AS, Allarà M, Bisogno T, Petrosino S, et al. Effects of cannabinoids and cannabinoid-enriched cannabis extracts on TRP channels and endocannabinoid metabolic enzymes. Br J Pharmacol. (2011) 163:1479–94. doi: 10.1111/j.1476-5381.2010.01166.x
27. De Petrocellis L, Orlando P, Moriello AS, Aviello G, Stott C, Izzo AA, et al. Cannabinoid actions at TRPV channels: effects on TRPV3 and TRPV4 and their potential relevance to gastrointestinal inflammation. Acta Physiol. (2012) 204:255–66. doi: 10.1111/j.1748-1716.2011.02338.x
28. Muller C, Morales P, Reggio PH. Cannabinoid ligands targeting TRP channels. Front Mol Neurosci. (2019) 11:1–15. doi: 10.3389/fnmol.2018.00487
29. Gallant M, Odei-Addo F, Frost CL, Levendal R-A. Biological effects of THC and a lipophilic cannabis extract on normal and insulin resistant 3T3-L1 adipocytes. Phytomedicine. (2009) 16:942–9. doi: 10.1016/j.phymed.2009.02.013
30. Cluny NL, Keenan CM, Reimer RA, Le Foll B, Sharkey KA. Prevention of diet-induced obesity effects on body weight and gut microbiota in mice treated chronically with Δ9-tetrahydrocannabinol. PLoS ONE. (2015) 10:e0144270. doi: 10.1371/journal.pone.0144270
31. Di Marzo V. The endocannabinoid system in obesity and type 2 diabetes. Diabetologia. (2008) 51:1356–67. doi: 10.1007/s00125-008-1048-2
32. Izzo AA, Borrelli F, Capasso R, Di Marzo V, Mechoulam R. Non-psychotropic plant cannabinoids: new therapeutic opportunities from an ancient herb. Trends Pharmacol Sci. (2009) 30:515–27. doi: 10.1016/j.tips.2009.07.006
33. McPartland JM, Duncan M, Di Marzo V, Pertwee RG. Are cannabidiol and Δ(9) -tetrahydrocannabivarin negative modulators of the endocannabinoid system? a systematic review. Br J Pharmacol. (2015) 172:737–53. doi: 10.1111/bph.12944
34. Cascio MG, Zamberletti E, Marini P, Parolaro D, Pertwee RG. The phytocannabinoid, δ9-tetrahydrocannabivarin, can act through 5-HT1A receptors to produce antipsychotic effects. Br J Pharmacol. (2015) 172:1305–18. doi: 10.1111/bph.13000
35. Wargent ET, Zaibi MS, Silvestri C, Hislop DC, Stocker CJ, Stott CG, et al. The cannabinoid Δ9-tetrahydrocannabivarin (THCV) ameliorates insulin sensitivity in two mouse models of obesity. Nutr Diabetes. (2013) 3:e68. doi: 10.1038/nutd.2013.9
36. Silvestri C, Paris D, Martella A, Melck D, Guadagnino I, Cawthorne M, et al. Two non-psychoactive cannabinoids reduce intracellular lipid levels and inhibit hepatosteatosis. J Hepatol. (2015) 62:1382–90. doi: 10.1016/j.jhep.2015.01.001
37. Jadoon KA, Ratcliffe SH, Barrett DA, Thomas EL, Stott C, Bell JD, et al. Efficacy and safety of cannabidiol and tetrahydrocannabivarin on glycemic and lipid parameters in patients with type 2 diabetes: a randomized, double-blind, placebo-controlled, parallel group pilot study. Diabetes Care. (2016) 39:1777–86. doi: 10.2337/dc16-0650
38. Wood TB, Spivey WTN, Easterfield TH. XL.—Charas. the resin of Indian hemp. J Chem Soc Trans. (1896) 69:539–46. doi: 10.1039/CT8966900539
39. Andre CM, Hausman JF, Guerriero G. Cannabis sativa: the plant of the thousand and one molecules. Front Plant Sci. (2016) 7:19. doi: 10.3389/fpls.2016.00019
40. Farrimond JA, Whalley BJ, Williams CM. Cannabinol and cannabidiol exert opposing effects on rat feeding patterns. Psychopharmacology. (2012) 223:117–29. doi: 10.1007/s00213-012-2697-x
41. Morales P, Reggio PH, Jagerovic N. An overview on medicinal chemistry of synthetic and natural derivatives of cannabidiol. Front Pharmacol. (2017) 8:422. doi: 10.3389/fphar.2017.00422
42. Hill TDM, Cascio M-G, Romano B, Duncan M, Pertwee RG, Williams CM, et al. Cannabidivarin-rich cannabis extracts are anticonvulsant in mouse and rat via a CB1 receptor-independent mechanism. Br J Pharmacol. (2013) 170:679–92. doi: 10.1111/bph.12321
43. Anavi-Goffer S, Baillie G, Irving AJ, Gertsch J, Greig IR, Pertwee RG, et al. Modulation of l-α-Lysophosphatidylinositol/GPR55 Mitogen-activated Protein Kinase (MAPK) signaling by cannabinoids. J Biol Chem. (2012) 287:91–104. doi: 10.1074/jbc.M111.296020
44. De Meijer EPM, Hammond KM. The inheritance of chemical phenotype in cannabis sativa L. (II): cannabigerol predominant plants. Euphytica. (2005) 145:189–98. doi: 10.1007/s10681-005-1164-8
45. Aizpurua-Olaizola O, Soydaner U, Öztürk E, Schibano D, Simsir Y, Navarro P, et al. Evolution of the cannabinoid and terpene content during the growth of cannabis sativa plants from different chemotypes. J Nat Prod. (2016) 79:324–31. doi: 10.1021/acs.jnatprod.5b00949
46. Navarro G, Varani K, Reyes-Resina I, Sánchez de Medina V, Rivas-Santisteban R, Sánchez-Carnerero Callado C, et al. Cannabigerol action at cannabinoid CB1 and CB2 receptors and at CB1–CB2 heteroreceptor complexes. Front Pharmacol. (2018) 9:632. doi: 10.3389/fphar.2018.00632
47. Cascio MG, Gauson LA, Stevenson LA, Ross RA, Pertwee RG. Evidence that the plant cannabinoid cannabigerol is a highly potent alpha2-adrenoceptor agonist and moderately potent 5HT1A receptor antagonist. Br J Pharmacol. (2010) 159:129–41. doi: 10.1111/j.1476-5381.2009.00515.x
48. Brierley DI, Samuels J, Duncan M, Whalley BJ, Williams CM. Cannabigerol is a novel, well-tolerated appetite stimulant in pre-satiated rats. Psychopharmacology. (2016) 233:3603–13. doi: 10.1007/s00213-016-4397-4
49. Rosenthaler S, Pöhn B, Kolmanz C, Nguyen Huu C, Krewenka C, Huber A, et al. Differences in receptor binding affinity of several phytocannabinoids do not explain their effects on neural cell cultures. Neurotoxicol Teratol. (2014) 46:49–56. doi: 10.1016/j.ntt.2014.09.003
50. De Meijer EPM, Hammond KM, Micheler M. The inheritance of chemical phenotype in cannabis sativa L. (III): variation in cannabichromene proportion. Euphytica. (2009) 165:293–311. doi: 10.1007/s10681-008-9787-1
51. Laprairie RB, Bagher AM, Kelly MEM, Denovan-Wright EM. Cannabidiol is a negative allosteric modulator of the cannabinoid CB1 receptor. Br J Pharmacol. (2015) 172:4790–805. doi: 10.1111/bph.13250
52. Silvestro S, Mammana S, Cavalli E, Bramanti P, Mazzon E. Use of cannabidiol in the treatment of epilepsy: efficacy and security in clinical trials. Molecules. (2019) 24:E1459. doi: 10.3390/molecules24081459
53. Iuvone T, Esposito G, De Filippis D, Scuderi C, Steardo L. Cannabidiol: a promising drug for neurodegenerative disorders? CNS Neurosci Ther. (2009) 15:65–75. doi: 10.1111/j.1755-5949.2008.00065.x
54. Ignatowska-Jankowska B, Jankowski MM, Swiergiel AH. Cannabidiol decreases body weight gain in rats: involvement of CB2 receptors. Neurosci Lett. (2011) 490:82–4. doi: 10.1016/j.neulet.2010.12.031
55. Liu J, Zhou L, Xiong K, Godlewski G, Mukhopadhyay B, Tam J, et al. Hepatic cannabinoid receptor-1 mediates diet-induced insulin resistance via inhibition of insulin signaling and clearance in mice. Gastroenterology. (2012) 142:1218–28.e1. doi: 10.1053/j.gastro.2012.01.032
56. Russo EB, Burnett A, Hall B, Parker KK. Agonistic properties of cannabidiol at 5-HT1a receptors. Neurochem Res. (2005) 30:1037–43. doi: 10.1007/s11064-005-6978-1
57. O'Sullivan SE. An update on PPAR activation by cannabinoids. Br J Pharmacol. (2016) 173:1899–910. doi: 10.1111/bph.13497
58. Esposito G, Scuderi C, Valenza M, Togna GI, Latina V, de Filippis D, et al. Cannabidiol reduces Aβ-induced neuroinflammation and promotes hippocampal neurogenesis through PPARγ involvement. PLoS ONE. (2011) 6:e28668. doi: 10.1371/journal.pone.0028668
59. Hegde VL, Singh UP, Nagarkatti PS, Nagarkatti M. Critical role of mast cells and peroxisome proliferator–activated receptor γ in the induction of myeloid-derived suppressor cells by marijuana cannabidiol in vivo. J Immunol. (2015) 194:5211–22. doi: 10.4049/jimmunol.1401844
60. Stienstra R, Duval C, Müller M, Kersten S. PPARs, obesity, and inflammation. PPAR Res. (2007) 2007:95974. doi: 10.1155/2007/95974
61. Blaschke F, Takata Y, Caglayan E, Law RE, Hsueh WA. Obesity, peroxisome proliferator-activated receptor, and atherosclerosis in type 2 diabetes. Arterioscler Thromb Vasc Biol. (2006) 26:28–40. doi: 10.1161/01.ATV.0000191663.12164.77
62. Izzo AA, Piscitelli F, Capasso R, Aviello G, Romano B, Borrelli F, et al. Peripheral endocannabinoid dysregulation in obesity: relation to intestinal motility and energy processing induced by food deprivation and re-feeding. Br J Pharmacol. (2009) 158:451–61. doi: 10.1111/j.1476-5381.2009.00183.x
63. Mechoulam R, Peters M, Murillo-Rodriguez E, Hanuš LO. Cannabidiol - recent advances. Chem Biodivers. (2007) 4:1678–92. doi: 10.1002/cbdv.200790147
64. Wierucka-Rybak M, Wolak M, Bojanowska E. The effects of leptin in combination with a cannabinoid receptor 1 antagonist, AM 251, or cannabidiol on food intake and bodyweight in rats fed a high-fat or a free-choice high sugar diet. J Physiol Pharmacol. (2014) 65:487–96.
65. Riedel G, Fadda P, McKillop-Smith S, Pertwee RG, Platt B, Robinson L. Synthetic and plant-derived cannabinoid receptor antagonists show hypophagic properties in fasted and non-fasted mice. Br J Pharmacol. (2009) 156:1154–66. doi: 10.1111/j.1476-5381.2008.00107.x
66. Wiley JL, Burston JJ, Leggett DC, Alekseeva OO, Razdan RK, Mahadevan A, et al. CB 1 cannabinoid receptor-mediated modulation of food intake in mice. Br J Pharmacol. (2005) 145:293–300. doi: 10.1038/sj.bjp.0706157
67. Stockings E, Zagic D, Campbell G, Weier M, Hall WD, Nielsen S, et al. Evidence for cannabis and cannabinoids for epilepsy: a systematic review of controlled and observational evidence. J Neurol Neurosurg Psychiatry. (2018) 89:741–53. doi: 10.1136/jnnp-2017-317168
68. Pickrell WO, Robertson NP. Cannabidiol as a treatment for epilepsy. J Neurol. (2017) 264:2506–8. doi: 10.1007/s00415-017-8663-0
69. White CM. A review of human studies assessing cannabidiol's (CBD) therapeutic actions and potential. J Clin Pharmacol. (2019) 59:923–34. doi: 10.1002/jcph.1387
70. Devinsky O, Marsh E, Friedman D, Thiele E, Laux L, Sullivan J, et al. Cannabidiol in patients with treatment-resistant epilepsy: an open-label interventional trial. Lancet Neurol. (2016) 15:270–8. doi: 10.1016/S1474-4422(15)00379-8
71. Jones NA, Glyn SE, Akiyama S, Hill TDM, Hill AJ, Weston SE, et al. Cannabidiol exerts anti-convulsant effects in animal models of temporal lobe and partial seizures. Seizure. (2012) 21:344–52. doi: 10.1016/j.seizure.2012.03.001
72. Akgün K, Essner U, Seydel C, Ziemssen T. Daily practice managing resistant multiple sclerosis spasticity with delta-9-tetrahydrocannabinol: cannabidiol oromucosal spray: a systematic review of observational studies. J Cent Nerv Syst Dis. (2019) 11:117957351983199. doi: 10.1177/1179573519831997
73. Thiele E, Marsh E, Mazurkiewicz-Beldzinska M, Halford JJ, Gunning B, Devinsky O, et al. Cannabidiol in patients with lennox-gastaut syndrome: interim analysis of an open-label extension study. Epilepsia. (2019) 60:419–28. doi: 10.1111/epi.14670
74. Devinsky O, Patel AD, Thiele EA, Wong MH, Appleton R, Harden CL, et al. Randomized, dose-ranging safety trial of cannabidiol in dravet syndrome. Neurology. (2018) 90:e1204–11. doi: 10.1212/WNL.0000000000005254
75. Adela Hruby, Frank B. Hu M. The epidemiology of obesity: a big picture. Pharmacoeconomics. (2015) 33:673–89. doi: 10.1007/s40273-014-0243-x
76. Matias I, Belluomo I, Cota D. The fat side of the endocannabinoid system: role of endocannabinoids in the adipocyte. Cannabis Cannabinoid Res. (2016) 1:176–85. doi: 10.1089/can.2016.0014
77. Tripathi YB, Pandey V. Obesity and endoplasmic reticulum (ER) stresses. Front Immunol. (2012) 3:240. doi: 10.3389/fimmu.2012.00240
78. Aldrich CK. Mechanisms and management of obesity. Med Clin North Am. (1963) 47:77–84. doi: 10.1016/S0025-7125(16)33621-5
79. Vettor R, Pagano C. The role of the endocannabinoid system in lipogenesis and fatty acid metabolism. Best Pract Res Clin Endocrinol Metab. (2009) 23:51–63. doi: 10.1016/j.beem.2008.10.002
80. Shrestha N, Cuffe JSM, Hutchinson DS, Headrick JP, Perkins A V, McAinch AJ, et al. Peripheral modulation of the endocannabinoid system in metabolic disease. Drug Discov Today. (2018) 23:592–604. doi: 10.1016/j.drudis.2018.01.029
81. Gruden G, Barutta F, Kunos G, Pacher P. Role of the endocannabinoid system in diabetes and diabetic complications. Br J Pharmacol. (2016) 173:1116–27. doi: 10.1111/bph.13226
82. Rosenson RS. Role of the endocannabinoid system in abdominal obesity and the implications for cardiovascular risk. Cardiology. (2009) 114:212–25. doi: 10.1159/000230691
83. Silvestri C, Ligresti A, Di Marzo V. Peripheral effects of the endocannabinoid system in energy homeostasis: adipose tissue, liver and skeletal muscle. Rev Endocr Metab Disord. (2011) 12:153–62. doi: 10.1007/s11154-011-9167-3
84. Matias I, Gonthier M-P, Orlando P, Martiadis V, De Petrocellis L, Cervino C, et al. Regulation, function, and dysregulation of endocannabinoids in models of adipose and beta-pancreatic cells and in obesity and hyperglycemia. J Clin Endocrinol Metab. (2006) 91:3171–80. doi: 10.1210/jc.2005-2679
85. Osei-Hyiaman D, Liu J, Zhou L, Godlewski G, Harvey-White J, Jeong W, et al. Hepatic CB1 receptor is required for development of diet-induced steatosis, dyslipidemia, and insulin and leptin resistance in mice. J Clin Invest. (2008) 118:3160–9. doi: 10.1172/JCI34827
86. Blüher M, Engeli S, Klöting N, Berndt J, Fasshauer M, Bátkai S, et al. Dysregulation of the peripheral and adipose tissue endocannabinoid system in human abdominal obesity. Diabetes. (2006) 55:3053–60. doi: 10.2337/db06-0812
87. Côté M, Matias I, Lemieux I, Petrosino S, Alméras N, Després JP, et al. Circulating endocannabinoid levels, abdominal adiposity and related cardiometabolic risk factors in obese men. Int J Obes. (2007) 31:692–9. doi: 10.1038/sj.ijo.0803539
88. Lu D, Dopart R, Kendall DA. Controlled downregulation of the cannabinoid CB1 receptor provides a promising approach for the treatment of obesity and obesity-derived type 2 diabetes. Cell Stress Chaperones. (2016) 21:1–7. doi: 10.1007/s12192-015-0653-5
89. Sarzani R, Bordicchia M, Marcucci P, Bedetta S, Santini S, Giovagnoli A, et al. Altered pattern of cannabinoid type 1 receptor expression in adipose tissue of dysmetabolic and overweight patients. Metabolism. (2009) 58:361–7. doi: 10.1016/j.metabol.2008.10.009
90. Azar S, Sherf-Dagan S, Nemirovski A, Webb M, Raziel A, Keidar A, et al. Circulating endocannabinoids are reduced following bariatric surgery and associated with improved metabolic homeostasis in humans. Obes Surg. (2019) 29:268–76. doi: 10.1007/s11695-018-3517-0
91. Osei-Hyiaman D, DePetrillo M, Pacher P, Liu J, Radaeva S, Bátkai S, et al. Endocannabinoid activation at hepatic CB1 receptors stimulates fatty acid synthesis and contributes to diet-induced obesity. J Clin Invest. (2005) 115:1298–305. doi: 10.1172/JCI200523057
92. Kimberly WT, O'Sullivan JF, Nath AK, Keyes M, Shi X, Larson MG, et al. Metabolite profiling identifies anandamide as a biomarker of nonalcoholic steatohepatitis. JCI Insight. (2017) 2:e92989. doi: 10.1172/jci.insight.92989
93. Zelber-Sagi S, Azar S, Nemirovski A, Webb M, Halpern Z, Shibolet O, et al. Serum levels of endocannabinoids are independently associated with nonalcoholic fatty liver disease. Obesity. (2017) 25:94–101. doi: 10.1002/oby.21687
94. Rinaldi-Carmona M, Barth F, Héaulme M, Shire D, Calandra B, Congy C, et al. SR141716A, a potent and selective antagonist of the brain cannabinoid receptor. FEBS Lett. (1994) 350:240–4. doi: 10.1016/0014-5793(94)00773-X
95. Tucci SA, Rogers EK, Korbonits M, Kirkham TC. The cannabinoid CB 1 receptor antagonist SR141716 blocks the orexigenic effects of intrahypothalamic ghrelin. Br J Pharmacol. (2004) 143:520–3. doi: 10.1038/sj.bjp.0705968
96. Trillou CR, Arnone M, Delgorge C, Gonalons N, Keane P, Maffrand JP, et al. Anti-obesity effect of SR141716, a CB1 receptor antagonist, in diet-induced obese mice. Am J Physiol Regul Integr Comp Physiol. (2003) 284:345–53. doi: 10.1152/ajpregu.00545.2002
97. Després JP, Ross R, Boka G, Alméras N, Lemieux I. Effect of rimonabant on the high-triglyceride/low-HDL-cholesterol dyslipidemia, intraabdominal adiposity, and liver fat the ADAGIO-lipids trial. Arterioscler Thromb Vasc Biol. (2009) 29:416–23. doi: 10.1161/ATVBAHA.108.176362
98. van Gaal LF, Rissanen AM, Scheen AJ, Ziegler O, Rössner S. Effects of the cannabinoid-1 receptor blocker rimonabant on weight reduction and cardiovascular risk factors in overweight patients: 1-Year experience from the RIO-Europe study. Lancet. (2005) 365:1389–97. doi: 10.1016/S0140-6736(05)66374-X
99. Hollander PA, Amod A, Litwak LE, Chaudhari U. Effect of rimonabant on glycemic control in insulin-treated type 2 diabetes: the ARPEGGIO trial. Diabetes Care. (2010) 33:605–7. doi: 10.2337/dc09-0455
100. Bermudez-Silva FJ, Viveros MP, McPartland JM, Rodriguez de Fonseca F. The endocannabinoid system, eating behavior and energy homeostasis: the end or a new beginning? Pharmacol Biochem Behav. (2010) 95:375–82. doi: 10.1016/j.pbb.2010.03.012
101. Cluny NL, Vemuri VK, Chambers AP, Limebeer CL, Bedard H, Wood JT, et al. A novel peripherally restricted cannabinoid receptor antagonist, AM6545, reduces food intake and body weight, but does not cause malaise, in rodents. Br J Pharmacol. (2010) 161:629–42. doi: 10.1111/j.1476-5381.2010.00908.x
102. Tam J, Cinar R, Liu J, Godlewski G, Wesley D, Szanda G, et al. Peripheral cannabinoid-1 receptor inverse agonism reduces obesity by reversing leptin resistance. Cell Metab. (2013) 16: 167–79. doi: 10.1016/j.cmet.2012.07.002
103. Knani I, Earley BJ, Udi S, Nemirovski A, Hadar R, Gammal A, et al. Targeting the endocannabinoid/CB1 receptor system for treating obesity in Prader–Willi syndrome. Mol Metab. (2016) 5:1187–99. doi: 10.1016/j.molmet.2016.10.004
104. Tam J, Vemuri VK, Liu J, Bátkai S, Mukhopadhyay B, Godlewski G, et al. Peripheral CB1 cannabinoid receptor blockade improves cardiometabolic risk in mouse models of obesity. J Clin Invest. (2010) 120:2953–66. doi: 10.1172/JCI42551
105. Vanni E, Bugianesi E, Kotronen A, De Minicis S, Yki-Järvinen H, Svegliati-Baroni G. From the metabolic syndrome to NAFLD or vice versa? Dig Liver Dis. (2010) 42:320–30. doi: 10.1016/j.dld.2010.01.016
106. Dasarathy S, Yang Y, McCullought AJ, Marczewski S, Bennet S. Elevated hepatic fatty acid oxidation, high plasma fibroblast growth factor 21, and fasting bile acids in nonalcoholic steatohepatitis. Eur J Gastroenterol Hepatol. (2013) 23:382–8. doi: 10.1097/MEG.0b013e328345c8c7
107. Sanyal AJ, Campbell-Sargent C, Mirshahi F, Rizzo WB, Contos MJ, Sterling RK, et al. Nonalcoholic steatohepatitis: association of insulin resistance and mitochondrial abnormalities. Gastroenterology. (2001) 120:1183–92. doi: 10.1053/gast.2001.23256
108. Croci I, Byrne NM, Choquette S, Hills AP, Chachay VS, Clouston AD, et al. Whole-body substrate metabolism is associated with disease severity in patients with non-alcoholic fatty liver disease. Gut. (2013) 62:1625–33. doi: 10.1136/gutjnl-2012-302789
109. Kotronen A, Seppälä-Lindroos A, Vehkavaara S, Bergholm R, Frayn KN, Fielding BA, et al. Liver fat and lipid oxidation in humans. Liver Int. (2009) 29:1439–46. doi: 10.1111/j.1478-3231.2009.02076.x
110. André A, Gonthier MP. The endocannabinoid system: its roles in energy balance and potential as a target for obesity treatment. Int J Biochem Cell Biol. (2010) 42:1788–801. doi: 10.1016/j.biocel.2010.06.002
111. Maccarrone M, Bab I, Bíró T, Cabral GA, Dey SK, Di Marzo V, et al. Endocannabinoid signaling at the periphery: 50 years after THC. Trends Pharmacol Sci. (2015) 36:277–96. doi: 10.1016/j.tips.2015.02.008
112. Mukhopadhyay P, Rajesh M, Horváth B, Bátkai S, Park O, Tanchian G, et al. Cannabidiol protects against hepatic ischemia/reperfusion injury by attenuating inflammatory signaling and response, oxidative/nitrative stress, and cell death. Free Radic Biol Med. (2011) 50:1368–81. doi: 10.1016/j.freeradbiomed.2011.02.021
113. Parray HA, Yun JW. Cannabidiol promotes browning in 3T3-L1 adipocytes. Mol Cell Biochem. (2016) 416:131–9. doi: 10.1007/s11010-016-2702-5
114. Bermúdez-Silva FJ, Suárez J, Baixeras E, Cobo N, Bautista D, Cuesta-Muñoz AL, et al. Presence of functional cannabinoid receptors in human endocrine pancreas. Diabetologia. (2008) 51:476–87. doi: 10.1007/s00125-007-0890-y
115. Juan-Picó P, Nadal A, Javier Díaz-Molina F, Ripoll C, Javier Bermúdez-Silva F, Fuentes E, et al. Cannabinoid receptors regulate Ca(2+) signals and insulin secretion in pancreatic beta-cell. Cell Calcium. (2005) 39:155–62. doi: 10.1016/j.ceca.2005.10.005
116. Weiss L, Zeira M, Reich S, Har-Noy M, Mechoulam R, Slavin S, et al. Cannabidiol lowers incidence of diabetes in non-obese diabetic mice. Autoimmunity. (2006) 39:143–51. doi: 10.1080/08916930500356674
117. Rajesh M, Mukhopadhyay P, Bátkai S, Patel V, Saito K, Matsumoto S, et al. Cannabidiol attenuates cardiac dysfunction, oxidative stress, fibrosis, and inflammatory and cell death signaling pathways in diabetic cardiomyopathy. J Am Coll Cardiol. (2010) 56:2115–25. doi: 10.1016/j.jacc.2010.07.033
118. Durst R, Danenberg H, Gallily R, Mechoulam R, Meir K, Grad E, et al. Cannabidiol, a nonpsychoactive cannabis constituent, protects against myocardial ischemic reperfusion injury. Am J Physiol Circ Physiol. (2007) 293:H3602–7. doi: 10.1152/ajpheart.00098.2007
119. Wang Y, Mukhopadhyay P, Cao Z, Wang H, Feng D, Haskó G, et al. Cannabidiol attenuates alcohol-induced liver steatosis, metabolic dysregulation, inflammation and neutrophil-mediated injury. Sci Rep. (2017) 7:12064. doi: 10.1038/s41598-017-10924-8
120. Mehrpouya-Bahrami P, Chitrala KN, Ganewatta MS, Tang C, Murphy EA, Enos RT, et al. Blockade of CB1 cannabinoid receptor alters gut microbiota and attenuates inflammation and diet-induced obesity. Sci Rep. (2017) 7:15645. doi: 10.1038/s41598-017-15154-6
121. Starowicz KM, Cristino L, Matias I, Capasso R, Racioppi A, Izzo AA, et al. Endocannabinoid dysregulation in the pancreas and adipose tissue of mice fed with a high-fat diet. Obesity. (2008) 16:553–65. doi: 10.1038/oby.2007.106
122. Zhang LL, Liu DY, Ma LQ, Luo ZD, Cao TB, Zhong J, et al. Activation of transient receptor potential vanilloid type-1 channel prevents adipogenesis and obesity. Circ Res. (2007) 100:1063–70. doi: 10.1161/01.RES.0000262653.84850.8b
123. Burstein S. Cannabidiol (CBD) and its analogs: a review of their effects on inflammation. Bioorganic Med Chem. (2015) 23:1377–85. doi: 10.1016/j.bmc.2015.01.059
124. Ramlugon S, Levendal R-A, Frost CL. Time-dependent effect of phytocannabinoid treatments in fat cells. Phyther Res. (2018) 32:1080–9. doi: 10.1002/ptr.6047
125. Chandra R, Liddle RA. Neural and hormonal regulation of pancreatic secretion. Curr Opin Gastroenterol. (2009) 25:441–6. doi: 10.1097/MOG.0b013e32832e9c41
126. Levendal R-A, Schumann D, Donath M, Frost CL. Cannabis exposure associated with weight reduction and β-cell protection in an obese rat model. Phytomedicine. (2012) 19:575–82. doi: 10.1016/j.phymed.2012.02.001
127. Harasim E, Stȩpek T, Konstantynowicz-Nowicka K, Baranowski M, Górski J, Chabowski A. Myocardial lipid profiling during time course of high fat diet and its relationship to the expression of fatty acid transporters. Cell Physiol Biochem. (2015) 37:1147–58. doi: 10.1159/000430238
Keywords: cannabidiol, diabetes, drugs, glucose metabolism, obesity, phytocannabinoids
Citation: Bielawiec P, Harasim-Symbor E and Chabowski A (2020) Phytocannabinoids: Useful Drugs for the Treatment of Obesity? Special Focus on Cannabidiol. Front. Endocrinol. 11:114. doi: 10.3389/fendo.2020.00114
Received: 04 December 2019; Accepted: 21 February 2020;
Published: 04 March 2020.
Edited by:
Claire Joanne Stocker, University of Buckingham, United KingdomReviewed by:
Joseph Tam, Hebrew University of Jerusalem, IsraelVincenzo Di Marzo, Italian National Research Council, Italy
Jon Arch, University of Buckingham, United Kingdom
Copyright © 2020 Bielawiec, Harasim-Symbor and Chabowski. This is an open-access article distributed under the terms of the Creative Commons Attribution License (CC BY). The use, distribution or reproduction in other forums is permitted, provided the original author(s) and the copyright owner(s) are credited and that the original publication in this journal is cited, in accordance with accepted academic practice. No use, distribution or reproduction is permitted which does not comply with these terms.
*Correspondence: Patrycja Bielawiec, cGF0cnljamEuYmllbGF3aWVjJiN4MDAwNDA7dW1iLmVkdS5wbA==