- 1Endocrinology Department, Hospital de Pediatría “Prof. Dr. Juan P. Garrahan”, Buenos Aires, Argentina
- 2Research Institute Garrahan-CONICET, Hospital de Pediatría “Prof. Dr. Juan P. Garrahan”, Buenos Aires, Argentina
- 3Facultad de Medicina, Department of Cellular Biology and Histology, Universidad de Buenos Aires, Buenos Aires, Argentina
Several reports in humans as well as transgenic mouse models have shown that estrogens play an important role in male reproduction and fertility. Estrogen receptor alpha (ERα) and beta (ERβ) are expressed in different male tissues including the brain. The estradiol-binding protein GPER1 also mediates estrogen action in target tissues. In human testes a minimal ERα expression during prepuberty along with a marked pubertal up-regulation in germ cells has been reported. ERβ expression was detected mostly in spermatogonia, primary spermatocytes, and immature spermatids. In Sertoli cells ERβ expression increases with age. The aromatase enzyme (cP450arom), which converts androgens to estrogens, is widely expressed in human tissues (including gonads and hypothalamus), even during fetal life, suggesting that estrogens are also involved in human fetal physiology. Moreover, cP450arom is expressed in the early postnatal testicular Leydig cells and spermatogonia. Even though the aromatase complex is required for estrogen synthesis, its biological relevance is also related to the regulation of the balance between androgens and estrogens in different tissues. Knockout mouse models of aromatase (ArKO) and estrogen receptors (ERKOα, ERKOβ, and ERKOαβ) provide an important tool to study the effects of estrogens on the male reproductive physiology including the gonadal axis. High basal serum FSH levels were reported in adult aromatase-deficient men, suggesting that estrogens are involved in the negative regulatory gonadotropin feedback. However, normal serum gonadotropin levels were observed in an aromatase-deficient boy, suggesting a maturational pattern role of estrogen in the regulation of gonadotropin secretion. Nevertheless, the role of estrogens in primate testis development and function is controversial and poorly understood. This review addresses the role of estrogens in gonadotropin secretion and testicular physiology in male humans especially during childhood and puberty.
Introduction
The role of the hypothalamic-pituitary-gonadal (HPG) axis and the endocrine system in the control of both spermatogenesis and testosterone production in males is well-known. The reproductive system is coordinated by the HPG axis. Hypothalamic gonadotropin-releasing hormone (GnRH) neurons represent the final common pathway for neuronally derived endogenous as well as exogenous stimuli (1). The anterior pituitary gland secretes gonadotropins, follicle-stimulating hormone (FSH), and luteinizing hormone (LH) in response to the hypothalamic GnRH signal. Gonadotropins stimulate the gonads to produce sex steroids and gametes. GnRH and gonadotropin secretion are modulated by sex steroids acting through their receptors -estrogen (ER) and androgen (AR) receptors- on the hypothalamus and the pituitary gland by way of feedback-regulating mechanisms (2–4). The role of the estradiol-binding protein (GPER) at the hypothalamic and pituitary level remains to be elucidated (5). The aromatase enzyme (cP450arom), which converts androgens to estrogens, is widely expressed in human tissues (including gonads and hypothalamus) even during fetal life, suggesting that estrogens are also involved in human fetal physiology. During pregnancy, androgen precursors are produced by the fetal adrenal glands (6–8).
Knockout (KO) mouse models for aromatase (ArKO) and estrogen receptors (αERKO and βERKO) are useful tools for understanding the role of estrogens in the regulation of the HPG axis. In addition, patients with aromatase deficiency and deleterious ER variants represent a model of nature that may be helpful to explain how estrogens act in the human reproductive system and gonadal physiology from infancy through adulthood.
The role of estrogens in primate testis development and function is still poorly understood. Therefore, in this chapter we discuss the role of estrogens in gonadotropin secretion and testicular physiology.
Estrogen Synthesis. Cytochrome P450 Aromatase
Estrogens control many physiological processes in mammals, including reproduction, spermatogenesis, ovulation, granulosa-cell proliferation, reproductive tract development, breast development, cardiovascular health, and bone integrity. Biosynthesis of estrogens from androgens is catalyzed by cP450arom, an enzyme located in the endoplasmic reticulum of estrogen-producing cells. This enzyme, a member of the cytochrome P450 superfamily, is expressed in different human tissues, such as the central nervous system, gonads, adipose and bone tissues, among others, and fetal tissues, such as liver, skin, intestine, testis, and ovary, as well as the syncytiotrophoblast layer of the placenta (9).
Human cP450aromis encoded by a single gene, the CYP19A1, that maps to chromosome 15q21.1 and contains nine coding exons (E2-E10) spanning ~35 kb (10). There is a tissue-specific regulation of the aromatase gene expression by promoter regions related to several different first exons; however, a unique aromatase protein sequence is expressed in every tissue as these exons are not translated (Figure 1A).
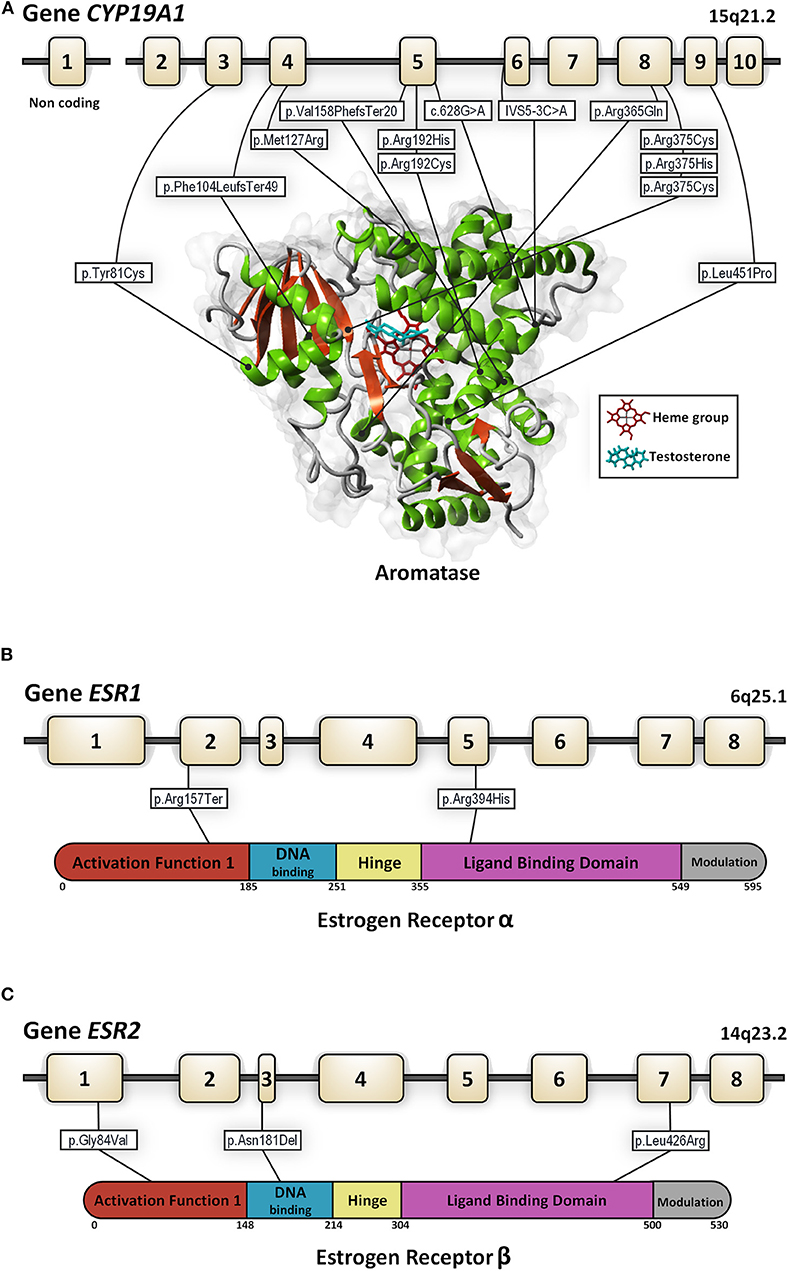
Figure 1. Genomic organization for the CYP19A1 (A), ESR1 (B), and ESR2 (C) genes. In each panel structure of the gene and the protein with its functional domains is presented as well as all the mutations described to date in 46, XY subjects (in white rectangles). Numbered boxes represent exons. Lines represent introns. For the aromatase enzyme the in-silico 3D model was created using the structure factor file containing the X-ray crystallographic structure of human placental aromatase cytochrome P450 (CYP19A1) complexed with testosterone (PDB ID: 5JKW) (doi: 10.2210/pdb5JKW/pdb). The 3D models were created and viewed using YASARA software (©1993–2018 by Elmar Krieger, www.yasara.org) with FoldX Suite plugin.
Estrogen Actions. Human Estrogen Receptors
Estrogen actions are mainly mediated through ERs, alpha (ERα), and beta (ERβ), which belong to the steroid receptor superfamily (11, 12) and are encoded by the ESR1 and ESR2 genes, respectively (Figures 1B,C). Both receptors contain six functional domains: the activation function (AF1) domain that contains the N-terminal transactivation domain, a target for phosphorylation, a DNA binding domain, a hinge region, a ligand-binding domain, and a modulation region (13). Both receptors are highly homologous in DNA and ligand-binding domains and divergent in other regions (14). The ESR1 gene consists of eight exons spanning more than 140 kb. The ESR2 gene contains eight exons as well. Both the ESR1 and ESR2 genes are subject to alternative splicing with the use different start sites and although their exon and functional domain organization is similar, the splice variant isoforms identified are distinct and have been implicated in tissue-specific patterns of gene expression.
These nuclear receptors act as transcriptional regulators through their direct interaction with specific co-regulators (15). The recruitment of different co-regulators in large multifunctional protein complexes is intrinsic to estrogen signaling which is involved in chromatin remodeling, histone modifications, transcription initiation and elongation, splicing, and proteasomal degradation. Therefore, co-regulators might be associated with either activation or repression of transcription. However, there are completely distinct cellular responses by ER subtype-specific and non-specific agonists. In this line, it has been reported that ERβ might exert an inhibitory effect on ERα-mediated signaling or an opposite effect in target tissues (16). Moreover, it has been reported that ERα and ERβ differentially mediated the regulation of miRNA expression (17–19). The AF1 domain in ERα is very active while the activity of the AF1 domain of ERβ is negligible. Interestingly, different responses of both receptors to synthetic antiestrogens have been reported. In this context, a better clinical outcome was proposed in tamoxifen-resistant ER-expressing breast tumors when high expression of ERβis found (20). A study by Lindberg et al. (21) showed that the expression of ERβ in breast cancer tissue reduced AKT activation and upregulated PTEN expression increasing sensitivity to tamoxifen therapy.
ERα is expressed in both the nucleus and the plasma membrane. The non-genomic or membrane action of estrogens exerts rapid membrane-cell signaling effects influencing gene transcription through membrane ERα and/or GPER1 (22–24). Moreover, some studies have shown a role of ERα in the mitochondria (25). The localization of estrogen receptors in the mitochondrial is tissue specific. However, its effects are not restricted to the presence inside the mitochondria. The major role of estrogens via ERs, mainly ERβ, is related to the control of mitochondrial biogenesis and quality control as well as antioxidant defenses (26, 27). In addition, it has been shown that the alteration of ER and GPR30 signaling induces mitochondrial dysfunction affecting testicular steroidogenesis and spermatogenesis (28, 29).
Tissue-specific expression of ERs has been reported. Specifically, ERα is highly expressed in the uterus and pituitary tissues with lesser expression in bone, liver, hypothalamus, mammary gland, vagina, and adult human testes (13, 22, 30) while ERβ expression is high in granulosa cells, prostate (31), and immature and adult human testes (32, 33).
Knockout Mouse Models for Aromatase and Estrogen Receptors
The Aromatase-Knockout Mouse
Murine aromatase is expressed in Sertoli cells of immature and in Leydig cells of adult rodents, as well as in spermatocytes and in round and elongated spermatids (34). An aromatase knockout (ArKO) model was presented by Fisher et al. (35). The newborn mice homozygous for the disrupted aromatase gene were described as phenotypically normal. At ~12–14 weeks of age, the internal anatomy of the male ArKO mice showed increased weight of the seminal vesicles (caused by increased volume of secretions) and of combined urinary bladder/prostate but no difference in testicular weight was found. Testosterone (T) levels varied among male mice; however, there was a trend toward overall higher levels compared to wild-type (WT) mice. Androstenedione concentration was also variable among animals, but several of them had values at least twice as high as values reported for the WT males. In the ArKO males, serum LH levels were elevated while serum FSH levels were normal. Additionally, Leydig-cell hyperplasia/hypertrophy was observed in the male ArKO mice, presumably because of high circulating LH levels. The ArKO mice showed normal fertility when young, although with advancing age they developed progressive infertility, as a decreased rate of litter siring became evident. Between 4.5 months and 1 year, these mice exhibited disruptions of spermatogenesis with increased frequency of germ-cell apoptosis and defects in spermiogenesis with the epididymis showing reduced or complete absence of sperm (36). These findings suggest that estrogen plays a role in the neuroendocrine regulation of LH secretion and in spermatid differentiation and spermatogenesis.
Recent studies in ArKO as well as αERKO mice have suggested that estrogens play a role in the normal development of the penis. The male ArKOmice, that completely lack estrogen production, presented with a mild hypospadias phenotype similar to that reported in the male αERKO mice (37).
Estrogen Receptor Knockout Mouse
Both estrogen receptor ERα and ERβ are expressed in the efferent ducts and epididymis, while ERα is found in Leydig cells and ERβ in spermatocytes in the developing mouse testis (30, 38).
The first αERKO mouse was developed by disruption of the mouse ER gene in 1993 (39). In these αERKO mice, decreased fertility was observed. However, subsequently the male offspring were found to be heterozygous αERKO mice. A new study in confirmed homozygous male αERKO mice revealed that these mice were completely infertile (40). They showed complete disruption of the seminiferous tubules and a significant reduction of testis weight. In adulthood, the lumen of all the seminiferous tubules were considerably enlarged and sperm quantity and quality was affected. The disrupted phenotype seemed to be more profound in older males. These findings suggest a progressive degenerative process. Fluid collection within the lumen of the seminiferous tubules that increased intratesticular pressure affecting the testicular blood flow was described. Serum testosterone levels were moderately but significantly higher, and serum LH and serum FSH were slightly higher than in the WT mice. In the male αERKO mice, behavioral aspects of reproduction were also affected reflected in decreased intromissions and weak ejaculation as well as significantly reduced aggressive behavior (41). Additionally, sperm from αERKO mice was ineffective at in vitro fertilization, suggesting that not only production but also function of spermatozoa was affected. Nevertheless, male WT mouse depleted of germ cells and transplanted with germ cells from male αERKO mouse produced fertile sperm, demonstrating that spermatogenic stem cells were not affected (42).
Another αERKO male mouse was generated with the deletion of exon 3 (a region encoding the DNA-binding domain), ExαERKO (43), presenting with the same phenotype as that seen in male αERKO mice (44). However, neither serum LH nor serum FSH levels were different from those in WT mice. Therefore, it could be proposed that estrogen receptor activation via a non-genomic pathway might be involved in the neuroendocrine regulation of gonadotropin synthesis and/or secretion.
The generation of the first βERKO mice (45) produced a completely different male phenotype compared to that of αERKO mice. Male βERKO mice were fully fertile, with some age-related abnormalities in the prostate and bladder. Later on, a true ERβ-null mouse was generated, in which exon 3 was deleted through a Cre/LoxP-system and no transcript of ERβ could be detected (46). In this ERβ-null mouse, testis as well as epididymis histology were normal, and the motility of their spermatozoa resemble normal sperm; however, mating defects were reported. It was concluded that both female and male ERβ-null mutants are sterile; however, the origin of the sterility in males is unknown.
Male mice lacking ERα and ERβ, αβERKO, showed a phenotype similar to that of male αERKO mice (31). Male αβERKO mice are infertile but have a generally normal reproductive tract. The number and motility of epididymal sperm was reduced. The most remarkable characteristic of αβERKO mice is seen in female adult mice, in which the ovaries show follicular differentiation to structures resembling seminiferous tubules of the testis, including Sertoli-like cells and expression of Müllerian inhibiting substance (MIS), sulfated glycoprotein-2, and Sox9. Elevated Sox9 mRNA levels were also detected in the testes of adult αβERKO and αERKO males (31, 47). It is well-known that very early in gestation, the expression of the transcription factor SOX9 in the bipotential gonad of the 46,XY fetus is required to induce testicular cell-fate differentiation (48). This finding raises the question of whether lack of expression of both ERα and ERβ in the bipotential gonad in 46,XY fetus is mandatory to allow for the expression of SOX9.
Membrane and Nuclear αERKO Mouse Models
ERs are predominantly nuclear and cytoplasmic; however, around 5–10% of ERα is found in the cell membranes. In order to define the role of membrane ERα in mouse physiology two mouse models were developed. One with membrane-only ERαexpression (MOER) and another with nuclear-only ERα expression (NOER) (49, 50). Both transgenic mice presented with male infertility and sperm abnormalities (51). However, no description about the reproductive tract of the male MOER mouse has been published.
Male NOER mice present with many abnormalities that are similar to those of male αERKO mice, such as decreased sperm production with impaired sperm motility and viability. Testis weight of NOER mice became greater than that of WT mice at 4 months of age when many of the seminiferous tubules were degenerated. Nevertheless, at 8 months of age, when profound histological changes in testis, rete testis, and efferent ducts were found in NOER mice, testis weight was not different from that of WT mice. At that time, NOER males showed great structural abnormalities in cauda epididymal sperm, similar to male αERKO mice. Serum T levels in NOER mice were significantly higher than in WT mice, while serum LH, FSH, and E2 levels were not different. Similar to male αERKO mice, NOER males become infertile with advancing age; however, juvenile NOER males are transiently fertile before tubule abnormalities become evident. The phenotype of male NOER mice may be considered less severe as it develops more slowly than that of male αERKO mice (52).
A comparison of the significant clinical phenotypes and hormonal studies in all KO-mice models is shown in Table 1.
Aromatase and Estrogen Receptor Expression in Human Testis From Infancy to Adulthood
Aromatase
In the human testis, aromatase is mainly expressed in interstitial, in fetal and adult Leydig, and germ cells. Nevertheless, the strongest aromatase immunostaining has been reported in neonatal and infant testes (Table 2) (30, 53, 54). Therefore, in human testes, in order to maintain an intratesticular balance between androgens and estrogens, it could be proposed that a short loop regulation of aromatase expression might occur mainly when postnatal testicular activation takes place. In addition, peritubular, and Sertoli cells showed weak and persistent aromatase expression without changes throughout the testicular maturation (33). In adult testes, aromatase is expressed in immature germ cells and in ejaculated spermatozoa, suggesting that aromatase might be involved in sperm motility. In this line, the fact that in 46, XY aromatase-deficient patients an alteration in the spermatogenesis related to sperm count and motility was reported, it could be speculated that estrogen action is required for immature germ-cell maturation and fertilizing capacity (33, 55, 56).
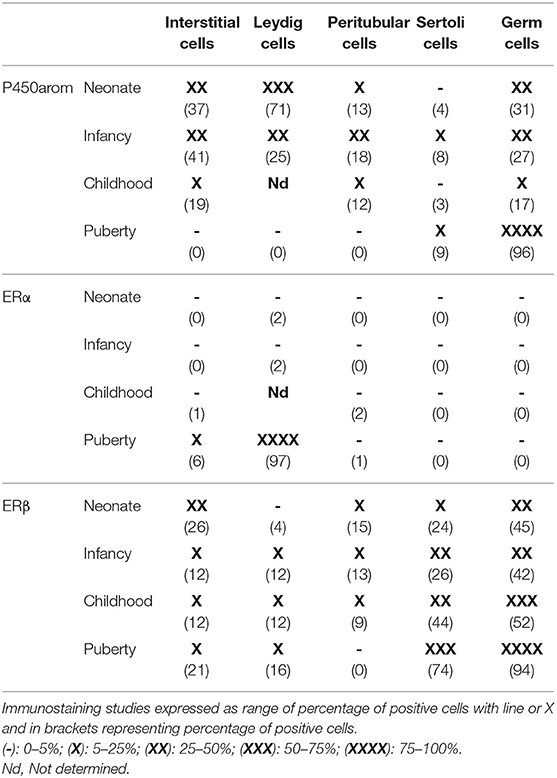
Table 2. Expression of P450arom, ERα, and ERβ during postnatal maturation in human testis (33).
ERα and ERβ in Primate Testes
We found that ERβ (Table 2) is the predominant form of ER expressed in human testes of neonates and infants (33). Different variants of ERβ have been reported, e.g., ERβcx, also known as ERβ2, a truncated variant with the loss of 61 aminoacids at the C-terminus. Expression of ERβ1 and ERβ2 is described in the human testis: ERβ1 was expressed in somatic cells, in pachytene spermatocytes and in round spermatids, while ERβ2 was expressed in fetal gonocytes (22, 57). In gonocytes and spermatogonia, ERβ was strongly immunoexpressed, while no expression of ERα was detected in germ cells. The spermatogonia might be a target of estrogens in prepuberty and adulthood. Positive expression of ERα in postmeiotic germ cells was found in puberty (33). Although the expression of ERβ in human testes increases during puberty, it is already high during childhood, when serum gonadotropins are very low or absent (33). Interestingly, even though there is no information about the role of ERβ target genes in human neonatal testis, it has been reported that in a mouse model with homozygous or heterozygous inactivation of ERβ, very soon after birth the number of gonocytes increased secondary to decreasing cell apoptosis. Nevertheless, the number of Sertoli and Leydig cells was not modified. It has been proposed that gonocytes are very sensitive to estrogens via the ERβ pathway during the neonatal period. Exposure to environmental endocrine disruptors (xenoestrogens) during fetal and neonatal testicular development might therefore lead to fertility disorders in adulthood (58). In adult human testicular biopsies, Cavaco et al. (32) showed that ERα and ERβ are expressed in somatic and germinal testicular cells. Immunoexpression of ERα was present in Leydig cells and Sertoli cells, as well as in immature and post-meiotic germ cells, while ERβ was expressed in the same cell types but not in spermatogonia and Sertoli cells, suggesting that both ER isoforms are involved in testicular function (32).
Lambard et al. (55) reported ERβ proteins (full-length and variant) in human male germ cells, but the possible mechanism of action of estrogens in these cells, in terms of both genomic and “non-genomic” pathways, remains to be elucidated (55).
As shown in our study (Table 2), in the immature human testes ERβ expression was higher in neonatal than in infant and juvenile testes. Interestingly, in newborns a high testicular growth rate mediated by decreased apoptosis was observed (59). In human and monkey testes, we have found minimal ERα expression in germ cells during prepuberty, but during puberty a marked upregulation of ERα was found in germ cells of both monkeys and humans (60).
A growing number ERβ splice variants have been reported. In haploid germ cells, high levels of novel ERβ deletion variants were found. The cell-specific distribution raises the question of whether the differential splicing of ERβ is regulated in a cell-specific manner and whether ERβ play a specific role in spermatogenesis (61).
Interstitial cells expressed ERβ, particularly in the neonatal and infant periods with a weaker expression during childhood, while ERα expression was <5% at all ages. Interstitial cells might be targets of estrogens during prepuberty and adulthood (33). During testicular development, three growth phases of Leydig cells have been reported in humans. In the first phase the cells are named “fetal Leydig cells” and they synthesize testosterone under placental βhCG stimulation, which is necessary for fetal masculinization, and insulin-like 3 factor for testicular descent. During infancy, testicular “infant Leydig cells” are involved in the production of testosterone under LH stimulation. This phase is known as the postnatal pituitary-testicular activation period (minipuberty). The last phase of “adult Leydig cells” coincides with pubertal development under LH regulation (33).
In neonatal and infant Leydig cells ERß staining was weak and during childhood it was even weaker. ERα staining was very weak in all cell compartments at any age in human prepubertal testicular tissue, but it was strong in the efferent ductules of the same samples (41) (Table 2). Similarly, no immuno expression of ERα was detected in juvenile primate testes, while strong expression was seen in the efferent ductules (22, 62).
Finally, although robust evidence is available on the role of estrogens in testis physiology during the life span, further studies, mainly in the human testes, are necessary.
Role of Estrogens in Gonadotropin and Testicular Physiology in Humans
Evidence suggests that in adult males, estrogens exert a negative feedback both at the hypothalamic and the pituitary level. The exogenous administration of estradiol to normal men and patients with isolated GnRH-deficiency was associated with significant decreases in serum gonadotropins as well as LH pulse amplitude (63) suggesting a direct pituitary effect of estrogens. Additionally, lack of gonadotropin suppression was reported when a non-aromatizable androgen was used (64–66).
The relevance of estrogen action in the hypothalamus is supported by the effect on serum gonadotropin levels with the use of the selective aromatase inhibitor anastrozole in normal men and men with idiopathic hypogonadotropic hypogonadism (IHH) after long-term pulsatile GnRH therapy (65, 66). Anastrozole led to an increase of mean serum LH and FSH levels in IHH and normal men. Moreover, greater increases in serum gonadotropin levels were observed in normal subjects, suggesting that an intact hypothalamus is needed for the full effect of the aromatase inhibitor.
Additionally to estradiol regulation, the product of Sertoli cells, inhibin B, participates in the negative feedback of FSH as shown mainly by animal studies (67). In normal men, however, the dynamics of the relationship between serum inhibin B and FSH levels varies according to pubertal development. At midpuberty, maturational changes in the negative feedback regulation of the HPG axis occur and persist into adulthood. In this process, estradiol is likely to play an important role (68). Therefore, it has been proposed that estrogen administration to boys during early and midpuberty would decrease serum LH concentration and LH pulse frequency but would have no effect on LH pulse amplitude (69). In the same line, it was reported that the use of letrozole in normal boys increased serum LH levels, LH pulse amplitude, and GnRH-induced LH during early and mid-puberty (70).
Human Models of Estrogen Deficiency and Resistance
The identification and characterization of human patients with mutations in ESR1 or aromatase allowed for a better understanding of the role of estrogen in the human HPG axis in men (71). Estrogen resistance due to an ESR1 (estrogen receptor α[ERα]) gene mutation is a rare condition, first described by Smith et al. (72). To date, only five patients with ESR1 mutations have been reported (46, XY n = 2 and 46, XX, n = 3 patients) (Figure 1B, Table 3).
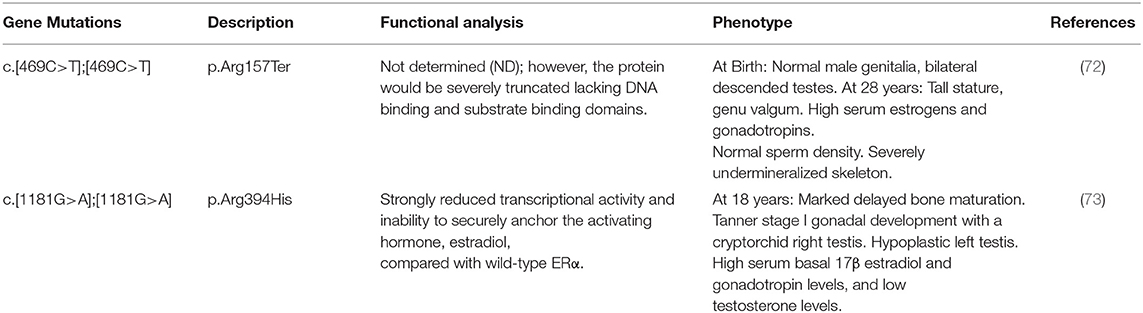
Table 3. Molecular defects in the ESR1 gene, in vitro activity of mutants, and clinical phenotype in published 46, XY subjects with estrogen resistance due to ESR1-mutations.
Two male patients from two consanguineous families with ESR1 mutations were reported (72, 73). The first patient described by Smith et al. presented at 28 years of age with normal male genitalia and bilateral 20–25 ml descended testes. Serum estrogens (estradiol and estrone) and gonadotropin concentration were high without changes after 6 months of estrogen therapy. Semen analysis revealed normal sperm density. The affected patient described by Bernard et al. (73) showed Tanner stage I pubertal development at 18 years of age with a cryptorchid right testis and a hypoplastic left testis (volume <1 mL). He also had high serum estrogen and gonadotropin levels, but AMH, inhibin B, and testosterone levels were low. In both cases, the variability in the clinical phenotype, hormonal profile, and testicular function was surprising, and it could not be explained by the genotype. Nevertheless, the contribution of the untreated cryptorchidism to the biochemical profile cannot be ruled out. In addition, in both cases bone age was delayed, confirming the role of estrogen in skeletal maturation.
Recently, biallelic and monoallelic ESR2 variants associated with a 46, XY disorder of sex development and in a 46, XX woman with early-onset complete ovarian failure were reported (74, 75) (Table 4). The 46, XY individuals had variable degree of gonadal dysgenesis. Interestingly, negative ERβ immunostaining was reported in a human 46, XY embryo at 8 weeks of gestational age around the time of sex determination (75). In this line, Kuiper et al. (12) proposed that the relationship between the two receptors is required to determine the different estrogen effects in target tissues. In addition, Baetens et al. (74) has proposed a role for ERβ in early gonadal development or in its differentiation. Nevertheless, as in male αβERKO mice a normal reproductive tract, but in female αβERKO mice sex reversal was observed (31), it could be speculated that in the undifferentiated gonad lack of ER expression is required to induce testicular cell fate. The two patients with more severe forms or complete gonadal dysgenesis presented with high serum FSH levels; however, a role forESR2 in the regulation of the HPG axis and gonadotropin secretion cannot be ruled out.
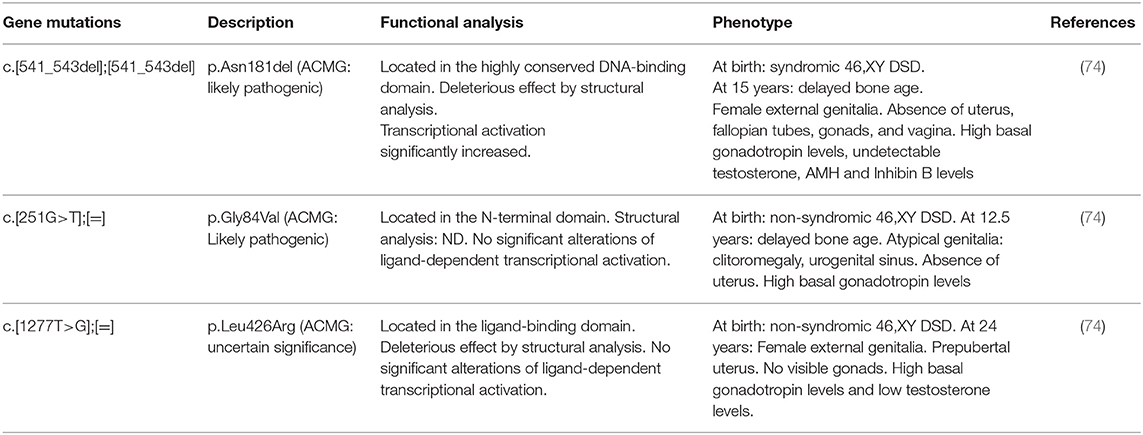
Table 4. Molecular defects in the ESR2 gene, in vitro activity of mutants, and clinical phenotype in published46,XY subjects with estrogen resistance due to ESR2 mutations.
Aromatase deficiency is an autosomal recessive disorder first described by Shozu et al. (76) (Table 5). Since then, 43 patients, 31 46, XX and 12 46, XY, have been reported (6).
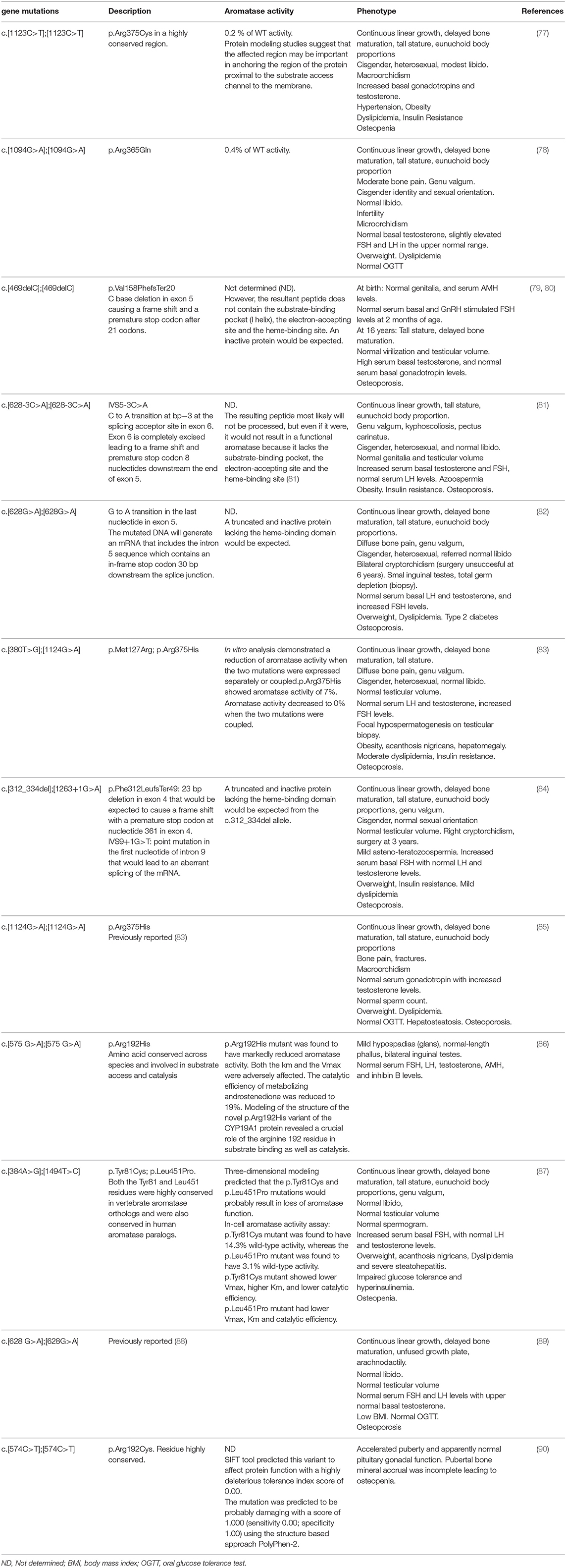
Table 5. Molecular defects in the CYP19A1 gene, in vitro aromatase activity of mutants, and clinical phenotype in published male aromatase-deficient subjects.
Different deleterious mutations in all exons and intron boundaries of the CYP19A1 gene were reported in individuals with aromatase deficiency. The mutations described to date in 46, XY patients are shown in Figure 1A.
In aromatase-deficient patients of both sexes, lack of suppression of serum gonadotropin levels into the normal range, along with high serum androgen levels support a major role for estrogens in the mechanism of gonadotropic regulation (6). In most affected men, basal serum LH levels are normal or slightly increased (77, 85); however, dynamic studies showed that LH pulsatility and pulse amplitude are increased (65, 91). Unlike serum LH, serum FSH levels are consistently increased (77, 78, 81–85, 87). Normal serum FSH levels were only reported in two affected males. A direct effect of high serum testosterone levels or residual aromatase activity might explain gonadotropin regulation in these cases (79, 89, 90). In two previously reported adults with aromatase deficiency and severely impaired spermatogenesis, Rochira et al. (91) described the presence of normal-to-low and low serum inhibin B levels along with increased serum FSH concentrations that did not normalize after estrogen replacement. The authors suggested that increments of serum FSH levels were not only related to the lack of estrogen negative feedback, but also to the spermatogenic damage in aromatase-deficient adult men. Nonetheless, in some other affected adult cases, serum FSH levels normalized after estrogen therapy even in the presence of low serum inhibin B levels and asthenozoospermia (84). The paucity of cases reported and the lack of systematic assessment of the HPG axis makes it difficult to validate conclusions regarding the relative contribution of estrogens and inhibin B to the gonadotropic negative feedback. In this line, it has been proposed that serum inhibin B, FSH, and the inhibin B/FSH ratio are useful biomarkers of impaired spermatogenesis (91).
The maturational changes in the dynamics of the regulation of the HGP axis during male pubertal development (see above), might explain the different findings regarding sex steroid-gonadotropin feedback found in adult and prepubertal aromatase-deficient patients. While serum estradiol levels were very low in an affected boy at 2 months of age, normal, and stimulated serum FSH levels were found at later ages. Moreover, serum androgen levels were high postnatally but decreased to the normal range during the first month of life, while serum levels of inhibin B were within the normal range for sex and age (80). The second aromatase-deficient boy identified was born with distal hypospadias and bilateral inguinal gonads (86). Serum FSH, LH, testosterone, AMH, and inhibin B levels were assessed at the age of 4 and 6 years and were within the normal reference range for age and sex. Since this is the only aromatase-deficient male reported with atypical genitalia, and a causative role of aromatase deficiency in the development of male external genitalia was not explored, a relationship between aromatase deficiency and genital phenotype has not been shown. A third boy was recently reported (90). At 8 years of age, the affected patient presented with normal basal and stimulated serum LH and FSH levels along with normal serum inhibin B and AMH concentrations. Taken together, these biochemical findings might suggest that, in contrast to adult men, estrogens do not seem to play a major role in the regulation of the gonadotropin negative feedback mechanism during infancy and prepubertal years in normal boys. Interestingly, the administration of an aromatase inhibitor in a prepubertal boy with idiopathic short stature was not associated with changes in serum gonadotropins (92).
On the other hand, an increase in serum gonadotropin levels along with a very high serum testosterone concentration were found when aromatase inhibitors were used during pubertal years. These findings raise concern regarding pubertal progression (92). In agreement with these observations, during male pubertal development, accelerated progression of secondary sexual characteristics was observed in an aromatase-deficient patient (90). This rapid progression of genital virilization was associated with a significant increase in basal serum testosterone levels and normal basal gonadotropin levels. LH pulsatility and pulse amplitude were not assessed and an increase in one or both parameters could not be ruled out as the underlying mechanism, considering that both effects have been previously demonstrated under estrogen suppression (65).
Conclusions
This review summarizes estrogen physiology, especially related to its role in the male reproductive system in different periods of maturation, from infancy to young adulthood.
The role of the HPG axis and the endocrine system in the control of both spermatogenesis and testosterone production in males is well-known. GnRH and gonadotropin secretion are modulated by sex steroids acting both on the hypothalamus and the pituitary gland through feedback-regulating mechanisms. Biosynthesis of estrogens from androgens is catalyzed bycP450arom, an enzyme located in the endoplasmic reticulum of estrogen-producing cells. This enzyme, a member of the cytochrome P450 superfamily, is expressed in a number of tissues, including the testes, placenta, and central nervous system.
Estrogen actions are mainly mediated through ERα and ERβ, which belong to the steroid receptor superfamily and are encoded by the ESR1 and ESR2 genes. These nuclear receptors act as transcriptional regulators through their direct interaction with specific co-regulators. Estrogens also exert rapid membrane-initiated effects that are known to impact on cell signaling and influence nuclear gene transcription. In addition, the estradiol-binding protein GPR30 also mediates multiple functions in various tissues. Moreover, a role in the mitochondria has been proposed.
ArKO, αERKO, and βERKO mouse models are useful tools for the understanding of the role of estrogens in the regulation of the HPG axis. In addition, patients with affected estrogen synthesis or action represent a model of nature to better understand the role of estrogens in the human reproductive system and gonadal physiology from infancy through adulthood. Male ArKO mouse model studies suggest that estrogen plays a role in the neuroendocrine regulation of LH secretion and in spermatid differentiation and spermatogenesis. Similar to the studies in male ArKO mice, studies in aromatase-deficient adult males but not in affected prepubertal boy simply that estrogen mainly plays a role in the neuroendocrine regulation of LH secretion. Nevertheless, inhibin B might be involved in the FSH negative feedback during childhood, puberty, and adulthood. In addition, a large variability in the number and quality of sperm was reported in the few affected cases studied. The αERKO mouse model showed slight increases in serum gonadotropin and testosterone levels, degeneration of the seminiferous tubules affecting spermatozoa production, including fertilizing capacity. However, in the βERKO null mice model testis and epididymis histology was normal but fertility was affected. The cause of the infertility is largely unknown. Interestingly, and in contrast to mice, ERβ but not ERα is expressed in the immature human testes. In adult human testes both receptors are expressed in somatic and germ cells.
In humans, only two 46, XY patients carrying an ERα gene mutation have been reported. Both patients presented with normal external genitalia but cryptorchidism was observed. Serum gonadotropin, LH, and FSH levels were high confirming the concept that estrogens are required for the regulation of normal negative feedback. Sperm count was normal in one and in the other delayed puberty was observed.
An ERβ gene mutation was reported in three 46, XY patients. Severe sex reversal was observed in all three. The cause of the alteration of sex determination in these 46, XY cases is poorly understood. Interestingly, at 8 weeks of gestational age, around the time of sex determination, negative ER-β immunostaining was reported in a human 46, XY embryo. Nevertheless, as in male αβERKO mice a normal reproductive tract and in female αβERKO mice sex reversal was observed, it could be speculated that in the undifferentiated gonad lack of ER expression is required to induce testicular cell fate.
Based on the current knowledge, several issues remain to be elucidated, such as: (1) The effect of peripheral and/or locally produced estrogen on gonadotropin synthesis and/or secretion and immature germ-cell apoptosis and maturation during the life span; (2) Genomic or non-genomic pathways of estrogen receptor activation involved in the regulation of HPG axis and testicular physiology; (3) The specific role of ERα and ERβ in testicular physiology from infancy to adulthood; (4) Variability in the clinical phenotype and hormonal profile in men with ER and P450arom gene mutations, and (5) The role of ER activation pathways in the bipotential gonad cell fate differentiation in early embryogenesis.
Finally, this review provides a new insight related to estrogen synthesis and estrogen action in human physiology, especially in the male reproductive system at different periods of maturation, from infancy to young adulthood.
Author Contributions
GG and NS contributed equally to the writing, review, and editing. MC, RM, and EB contributed to the writing and review. PR contributed to the figure design. AB conceived, wrote, reviewed, and determined the final version of the manuscript. All authors have contributed significantly, both related to their own scientific discipline and expertise, and to the overall manuscript and approved the final manuscript.
Funding
This work was supported by grants from National Scientific and Technical Research Council–Argentina (C.O.N.I.C.E.T.) and Fondo para la Investigación Científica y Tecnológica (FONCYT), Argentina.
Conflict of Interest
The authors declare that the research was conducted in the absence of any commercial or financial relationships that could be construed as a potential conflict of interest.
References
1. Herbison AE. Physiology of the adult gonadotropin-releasing hormone neuronal network. In: Plant T, editor. Knobil and Neill's Physiology of Reproduction. San Diego, CA: Academic Press (2006). p. 1415–82. doi: 10.1016/B978-012515400-0/50033-6
2. Belgorosky A, Pepe C, Marino R, Guercio G, Saraco N, Vaiani E, et al. Hypothalamic-pituitary-ovarian axis during infancy, early and late prepuberty in an aromatase-deficient girl who is a compound heterocygote for two new point mutations of the CYP19 gene. J Clin Endocrinol Metab. (2003) 88:5127–31. doi: 10.1210/jc.2003-030433
3. Belgorosky A, Guercio G, Pepe C, Saraco N, Rivarola MA. Genetic and clinical spectrum of aromatase deficiency in infancy, childhood and adolescence. Horm Res. (2009) 72:321–30. doi: 10.1159/000249159
4. Marino R, Perez Garrido N, Costanzo M, Guercio G, Juanes M, Rocco C, et al. Five new cases of 46,xx aromatase deficiency: clinical follow-up from birth to puberty, a novel mutation, and a founder effect. J Clin Endocrinol Metab. (2015) 100:301–7. doi: 10.1210/jc.2014-2967
5. Chimento A, Sirianni R, Casaburi I, Pezzi V. GPER signaling in spermatogenesis and testicular tumors. Front Endocrinol. (2014) 5:30. doi: 10.3389/fendo.2014.00030
6. Guercio G, Saraco N, Costanzo M, Marino R, Belgorosky A. Human aromatase deficiency. In: Huhtaniemi I, editor. Encyclopedia of Endocrine Diseases 2e. Cambrige, MA: Elsevier (2019). p. 532–49. doi: 10.1016/B978-0-12-801238-3.65212-1
7. Melau C, Nielsen JE, Frederiksen H, Kilcoyne K, Perlman S, Lundvall L, et al. Characterization of human adrenal steroidogenesis during fetal development. J Clin Endocrinol Metab. (2019). 104:1802–12. doi: 10.1210/jc.2018-01759
8. O'Shaughnessy PJ, Mitchell RT, Monteiro A, O'Hara L, Cruickshanks L, der Grinten HC, et al. Androgen receptor expression is required to ensure development of adult Leydig cells and to prevent development of steroidogenic cells with adrenal characteristics in the mouse testis. BMC Dev Biol. (2019) 19:8. doi: 10.1186/s12861-019-0189-5
9. Graham-Lorence S, Amarneh B, White RE, Peterson JA, Simpson ER. A three-dimensional model of aromatase cytochrome P450. Protein Sci. (1995) 4:1065–80. doi: 10.1002/pro.5560040605
10. Means GD, Mahendroo MS, Corbin CJ, Mathis JM, Powell FE, Mendelson CR, et al. Structural analysis of the gene encoding human aromatase cytochrome P-450, the enzyme responsible for estrogen biosynthesis. J Biol Chem. (1989) 264:19385–91.
11. Walter P, Green S, Greene G, Krust A, Bornert JM, Jeltsch JM, et al. Cloning of the human estrogen receptor cDNA. Proc Natl Acad Sci USA. (1985). 82:7889–93. doi: 10.1073/pnas.82.23.7889
12. Kuiper GG, Enmark E, Pelto-Huikko M, Nilsson S, Gustafsson JA. Cloning of a novel receptor expressed in rat prostate and ovary. Proc Natl Acad Sci USA. (1996) 93:5925–30. doi: 10.1073/pnas.93.12.5925
13. Hewitt SC, Korach KS. Estrogen receptors: new directions in the new millennium. Endocr Rev. (2018) 39:664–75. doi: 10.1210/er.2018-00087
14. Hamilton KJ, Hewitt SC, Arao Y, Korach KS. Estrogen hormone biology. Curr Top Dev Biol. (2017) 125:109–46. doi: 10.1016/bs.ctdb.2016.12.005
15. Dasgupta S, Lonard DM, O'Malley BW. Nuclear receptor coactivators: master regulators of human health and disease. Annu Rev Med. (2014) 65:279–92. doi: 10.1146/annurev-med-051812-145316
16. Nilsson S, Gustafsson J. Estrogen receptors: therapies targeted to receptor subtypes. Clin Pharmacol Ther. (2011) 89:44–55. doi: 10.1038/clpt.2010.226
17. Walton TJ, Li G, Seth R, McArdle SE, Bishop MC, Rees RC. DNA demethylation and histone deacetylation inhibition co-operate to re-express estrogen receptor beta and induce apoptosis in prostate cancer cell-lines. Prostate. (2008) 68:210–22. doi: 10.1002/pros.20673
18. Yap OW, Bhat G, Liu L, Tollefsbol TO. Epigenetic modifications of the Estrogen receptor beta gene in epithelial ovarian cancer cells. Anticancer Res. (2009) 29:139–44.
19. Tu Z, Li H, Ma Y, Tang B, Tian J, Akers W, et al. The enhanced antiproliferative response to combined treatment of trichostatin A with raloxifene in MCF-7 breast cancer cells and its relevance to estrogen receptor β expression. Mol Cell Biochem. (2012) 366:111–22. doi: 10.1007/s11010-012-1288-9
20. Fox EM, Davis RJ, Shupnik MA. ERβ in breast cancer: on looker, passive player, or active protector? Steroids. (2008) 73:1039–51. doi: 10.1016/j.steroids.2008.04.006
21. Lindberg K, Helguero LA, Omoto Y, Gustafsson JÅ, Haldosén LA. Estrogen receptor β represses Akt signaling in breast cancer cells via downregulation of HER2/HER3 and upregulation of PTEN: implications for tamoxifen sensitivity. Breast Cancer Res. (2011) 13:R43. doi: 10.1186/bcr2865
22. Saunders PT, Sharpe RM, Williams K, Macpherson Sh, Urquart H, Irvine DS, et al. Differential expression of estrogen receptor alpha and beta proteins in the testes and male reproductive system of human and non-human primates. Mol Hum Reprod. (2001) 7:227–36. doi: 10.1093/molehr/7.3.227
23. Xu Y, Traystman RJ, Hurn PD, Wang MM. Membrane restraint of estrogen receptor alpha enhances estrogen-dependent nuclear localization and genomic function. Mol Endocrinol. (2004). 18:86–96. doi: 10.1210/me.2003-0262
24. Zaitsu M, Narita S, Lambert KC, Grady JJ, Estes DM, Curran EM, et al. Quantitative measurement of estrogen-induced ERK 1 and 2 activation via multiple membrane-initiated signaling pathways. Steroids. (2004) 69:181–92. doi: 10.1016/j.steroids.2003.12.003
25. Yager JD, Chen JQ. Mitochondrial estrogen receptors–new insights into specific functions. Trends Endocrinol Metab. (2007) 18:89–91. doi: 10.1016/j.tem.2007.02.006
26. Simpkins JW, Yang SH, Sarkar SN, Pearce V. Estrogen actions on mitochondria–physiological and pathological implications. Mol Cell Endocrinol. (2008) 290:51–9. doi: 10.1016/j.mce.2008.04.013
27. Klinge CM. Estrogenic control of mitochondrial function and biogenesis. J Cell Biochem. (2008) 105:1342–51. doi: 10.1002/jcb.21936
28. Akingbemi BT. Estrogen regulation of testicular function. Reprod Biol Endocrinol. (2005) 3:51. doi: 10.1186/1477-7827-3-51
29. Yao Y, Chang X, Wang D, Ma H, Wang H, Zhang H, et al. Roles of ERK1/2 and PI3K/AKT signaling pathways in mitochondria-mediated apoptosis in testes of hypothyroid rats. Toxicol Res. (2018) 7:1214–24. doi: 10.1039/C8TX00122G
30. Hess RA, Cooke PS. Estrogen in the male: a historical perspective. Biol Reprod. (2018) 99:27–44. doi: 10.1093/biolre/ioy043
31. Couse JF, Hewitt SC, Bunch DO, Sar M, Walker VR, Davis BJ, et al. Postnatal sex reversal of the ovaries in mice lacking estrogen receptors alpha and beta. Science. (1999) 286:2328–31. doi: 10.1126/science.286.5448.2328
32. Cavaco JE, Laurentino SS, Barros A, Sousa M, Socorro S. Estrogen receptors alpha and beta in human testis: both isoforms are expressed. Syst Biol Reprod Med. (2009) 55:137–44. doi: 10.1080/19396360902855733
33. Berensztein E, Baquedano MS, Gonzalez CR, Saraco NI, Rodriguez J, Ponzio R, et al. Expression of aromatase, estrogen receptor alpha and beta, androgen receptor, and cytochrome P-450scc in the human early prepubertal testis. Pediatr Res. (2006) 60:740–4. doi: 10.1203/01.pdr.0000246072.04663.bb
34. O'Donnell L, Robertson KM, Jones ME, Simpson ER. Estrogen and spermatogenesis. Endocr Rev. (2001) 22:289–318. doi: 10.1210/er.22.3.289
35. Fisher CR, Graves KH, Parlow AF, Simpson ER. Characterization of mice deficient in aromatase (ArKO) because of targeted disruption of the cyp19 gene. Proc Natl Acad Sci USA. (1998) 95:6965–70. doi: 10.1073/pnas.95.12.6965
36. Robertson KM, O'Donnell L, Jones ME, Meachem SJ, Boon WC, Fisher CR, et al. Impairment of spermatogenesis in mice lacking a functional aromatase (cyp 19) gene. Proc Natl Acad Sci USA. (1999) 96:7986–91. doi: 10.1073/pnas.96.14.7986
37. Cripps SM, Mattiske DM, Black JR, Risbridger GP, Govers LC, Phillips TR, et al. A loss of estrogen signaling in the aromatase deficient mouse penis results in mild hypospadias. Differentiation. (2019) 109:42–52. doi: 10.1016/j.diff.2019.09.001
38. Jefferson WN, Couse JF, Banks EP, Korach KS, Newbold RR. Expression of estrogen receptor beta is developmentally regulated in reproductive tissues of male and female mice. Biol Reprod. (2000) 62:310–7. doi: 10.1095/biolreprod62.2.310
39. Lubahn DB, Moyer JS, Golding TS, Couse JF, Korach KS, Smithies O. Alteration of reproductive function but not prenatal sexual development after insertional disruption of the mouse estrogen receptor gene. Proc Natl Acad Sci USA. (1993) 90:11162–6. doi: 10.1073/pnas.90.23.11162
40. Eddy EM, Washburn TF, Bunch DO, Goulding EH, Gladen BC, Lubahn DB, et al. Targeted disruption of the estrogen receptor gene in male mice causes alteration of spermatogenesis and infertility. Endocrinology. (1996) 137:4796–805. doi: 10.1210/endo.137.11.8895349
41. Walker VR, Korach KS. Estrogen receptor knockout mice as a model for endocrine research. ILAR J. (2004) 45:455–61. doi: 10.1093/ilar.45.4.455
42. Mahato D, Goulding EH, Korach KS, Eddy EM. Estrogen receptor-alpha is required by the supporting somatic cells for spermatogenesis. Mol Cell Endocrinol. (2001) 178:57–63 doi: 10.1016/S0303-7207(01)00410-5
43. Goulding EH, Hewitt SC, Nakamura N, Hamilton K, Korach KS, Eddy EM. Ex3 αERKO male infertility phenotype recapitulates the αERKO male phenotype. J Endocrinol. (2010) 207:281–8. doi: 10.1677/JOE-10-0290
44. Akingbemi BT, Ge R, Rosenfeld CS, Newton LG, Hardy DO, Catterall JF, et al. Estrogen receptor-alpha gene deficiency enhances androgen biosynthesis in the mouse Leydig cell. Endocrinology. (2003) 144:84–93. doi: 10.1210/en.2002-220292
45. Krege JH, Hodgin JB, Couse JF, Enmark E, Warner M, Mahler JF, et al. Generation and reproductive phenotypes of mice lacking estrogen receptor beta. Proc Natl Acad Sci USA. (1998) 95:15677–82. doi: 10.1073/pnas.95.26.15677
46. Antal MC, Krust A, Chambon P, Mark M. Sterility and absence of histopathological defects in nonreproductive organs of a mouse ERbeta-null mutant. Proc Natl Acad Sci USA. (2008) 105:2433–8. doi: 10.1073/pnas.0712029105
47. Dupont S, Krust A, Gansmuller A, Dierich A, Chambon P, Mark M. Effect of single and compound knockouts of estrogen receptors alpha (ERalpha) and beta (ERbeta) on mouse reproductive phenotypes. Development. (2000) 127:4277–91.
48. Sekido R, Lovell-Badge R. Sex determination involves synergistic action of SRY and SF1 on a specific Sox9 enhancer. Nature. (2008) 453:930–4. doi: 10.1038/nature06944
49. Pedram A, Razandi M, Kim JK, O'Mahony F, Lee EY, Luderer U, et al. Developmental phenotype of a membrane only estrogen receptor alpha (MOER) mouse. J Biol Chem. (2009) 284:3488–95. doi: 10.1074/jbc.M806249200
50. Pedram A, Razandi M, Lewis M, Hammes S, Levin ER. Membrane-localized estrogen receptor α is required for normal organ development and function. Dev Cell. (2014) 29:482–90. doi: 10.1016/j.devcel.2014.04.016
51. Levin ER, Hammes SR. Nuclear receptors outside the nucleus: extranuclear signalling by steroid receptors. Nat Rev Mol Cell Biol. (2016) 17:783–97. doi: 10.1038/nrm.2016.122
52. Nanjappa MK, Hess RA, Medrano TI, Locker SH, Levin ER, Cooke PS. (2016) Membrane-localized estrogen receptor 1 is required for normal male reproductive development and function in mice. Endocrinology. 157:2909–19. doi: 10.1210/en.2016-1085
53. Saraco N, Berensztein E, Dardis A, Rivarola MA, Belgorosky A. Expression of the aromatase gene in the human prepubertal testis. J Pediatr Endocrinol Metab. (2000) 13:483–8. doi: 10.1515/JPEM.2000.13.5.483
54. Carreau S, de Vienne C, Galeraud-Denis I. Aromatase and estrogens in man reproduction: a review and latest advances. Adv Med Sci. (2008) 53:139–44. doi: 10.2478/v10039-008-0022-z
55. Lambard S, Galeraud-Denis I, Saunders PT, Carreau S. Human immature germ cells and ejaculated spermatozoa contain aromatase and oestrogen receptors. J Mol Endocrinol. (2004) 32:279–89. doi: 10.1677/jme.0.0320279
56. Carreau S, Silandre D, Bourguiba S, Hamden K, Said L, Lambard S, et al. Estrogens and male reproduction: a new concept. Braz J Med Biol Res. (2007) 40:761–8. doi: 10.1590/S0100-879X2007000600003
57. Gaskell TL, Robinson LL, Groome NP, Anderson RA, Saunders PT. Differential expression of two estrogen receptor beta isoforms in the human fetal testis during the second trimester of pregnancy. J Clin Endocrinol Metab. (2003). 88:424–32. doi: 10.1210/jc.2002-020811
58. Delbès G, Duquenne C, Szenker J, Taccoen J, Habert R, Levacher C. Developmental changes in testicular sensitivity to estrogens throughout fetal and neonatal life. Toxicol Sci. (2007) 99:234–43. doi: 10.1093/toxsci/kfm160
59. Berensztein E, Sciara M, Rivarola MA, Belgorosky A. Apoptosis and proliferation of human testicular somatic and germ cells during prepuberty: high rate of testicular growth in newborns mediated by decreased apoptosis. J Clin Endocrinol Metab. (2002) 87:5113–8. doi: 10.1210/jc.2002-020032
60. Berensztein E, Plant TM, Aliberti P, Baquedano MS, Chirico D, Rivarola M, et al. Comparative study of expression of estrogen receptor beta in testes of rhesus monkey and man. ENDO2014 Meeting, Endocrine Society. Endocr Rev. (2014) 35:4.
61. Aschim EL, Saether T, Wiger R, Grotmol T, Haugen TB. Differential distribution of splice variants of estrogen receptor beta in human testicular cells suggests specific functions in spermatogenesis. J Steroid Biochem Mol Biol. (2004) 92:97–106. doi: 10.1016/j.jsbmb.2004.05.008
62. Fisher JS, Millar MR, Majdic G, Saunders PT, Fraser HM, Sharpe RM. Immunolocalisation of oestrogen receptor-alpha within the testis and excurrent ducts of the rat and marmoset monkey from perinatal life to adulthood. J Endocrinol. (1997) 153:485–95. doi: 10.1677/joe.0.1530485
63. Finkelstein JS, O'Dea LS, Whitcomb RW, Crowley WF Jr. Sex steroid control of gonadotropin secretion in the human male. II. Effects of estradiol administration in normal and gonadotropin-releasing hormone-deficient men. J Clin Endocrinol Metab. (1991) 73:621–8. doi: 10.1210/jcem-73-3-621
64. Bagatell CJ, Dahl KD, Bremner WJ. The direct pituitary effect of testosterone to inhibit gonadotropin secretion in men is partially mediated by aromatization to estradiol. J Androl. (1994) 15:15–21.
65. Hayes FJ, Seminara SB, Decruz S, Boepple PA, Crowley WF Jr. Aromatase inhibition in the human male reveals a hypothalamic site of estrogen feedback. J Clin Endocrinol Metab. (2000) 85:3027–35. doi: 10.1210/jcem.85.9.6795
66. Pitteloud N, Dwyer AA, DeCruz S, Lee H, Boepple PA, Crowley WF Jr, et al. The relative role of gonadal sex steroids and gonadotropin-releasing hormone pulse frequency in the regulation of follicle-stimulating hormone secretion in men. J Clin Endocrinol Metab. (2008) 93:2686–92. doi: 10.1210/jc.2007-2548
67. Medhamurthy R, Culler MD, Gay VL, Negro-Vilar A, Plant TM. Evidence that inhibin plays a major role in the regulation of follicle-stimulating hormone secretion in the fully adult male rhesus monkey (Macacamulatta). Endocrinology. (1991) 129:389–95. doi: 10.1210/endo-129-1-389
68. Andersson AM, Juul A, Petersen JH, Müller J, Groome NP, Skakkebaek NE. Serum inhibin B in healthy pubertal and adolescent boys: relation to age, stage of puberty, and follicle-stimulating hormone, luteinizing hormone, testosterone, and estradiol levels. J Clin Endocrinol Metab. (1997) 82:3976–81. doi: 10.1210/jcem.82.12.4449
69. Kletter GB, Padmanabhan V, Beitins IZ, Marshall JC, Kelch RP, Foster CM. Acute effects of estradiol infusion and naloxone on luteinizing hormone secretion in pubertal boys. J Clin Endocrinol Metab. (1997) 82:4010–4. doi: 10.1210/jc.82.12.4010
70. Wickman S, Sipilä I, Ankarberg-Lindgren C, Norjavaara E, Dunkel L. A specific aromatase inhibitor and potential increase in adult height in boys with delayed puberty: a randomized controlled trial. Lancet. (2001) 357:1743–8. doi: 10.1016/S0140-6736(00)04895-9
71. Cooke PS, Nanjappa MK, Ko C, Prins GS, Hess RA. Estrogens in male physiology. Physiol Rev. (2017) 97:995–1043. doi: 10.1152/physrev.00018.2016
72. Smith EP, Boyd J, Frank GR, Takahashi H, Cohen RM, Specker B, et al. Estrogen resistance caused by a mutation in the estrogen-receptor gene in a man. N Engl J Med. (1994) 331:1056–61. doi: 10.1056/NEJM199410203311604
73. Bernard V, Kherra S, Francou B, Fagart J, Viengchareun S, Guéchot J, et al. Familial multiplicity of estrogen insensitivity associated with a loss-of-function ESR1mutation. J Clin Endocrinol Metab. (2017) 102:93–9. doi: 10.1210/jc.2016-2749
74. Baetens D, Güran T, Mendonca BB, Gomes NL, De Cauwer L, Peelman F, et al. Biallelic and monoallelic ESR2 variants associated with 46,XY disorders of sex development. Genet Med. (2018) 20:717–27. doi: 10.1038/gim.2017.163
75. Lang-Muritano M, Sproll P, Wyss S, Kolly A, Hürlimann R, Konrad D, et al. Early-onset complete ovarian failure and lack of puberty in a woman with mutated estrogen receptor β (ESR2). J Clin Endocrinol Metab. (2018) 103:3748–56. doi: 10.1210/jc.2018-00769
76. Shozu M, Akasofu K, Harada T, Kubota Y. A new cause of female pseudohermaphroditism: placental aromatase deficiency. J Clin Endocrinol Metab. (1991) 72:560–6. doi: 10.1210/jcem-72-3-560
77. Morishima A, Grumbach MM, Simpson ER, Fisher C, Qin K. Aromatase deficiency in male and female siblings caused by a novel mutation and the physiological role of estrogens. J Clin Endocrinol Metab. (1995) 80:3689–98. doi: 10.1210/jcem.80.12.8530621
78. Carani C, Qin K, Simoni M, Faustini-Fustini M, Serpente S, Boyd J, et al. Effect of testosterone and estradiol in a man with aromatase deficiency. N Engl J Med. (1997) 337:91–5. doi: 10.1056/NEJM199707103370204
79. Bouillon R, Bex M, Vanderschueren D, Boonen S. Estrogens are essential for male pubertal periosteal bone expansion. J Clin Endocrinol Metab. (2004) 89:6025–9. doi: 10.1210/jc.2004-0602
80. Deladoëy J, Flück C, Bex M, Yoshimura N, Harada N, Mullis PE. Aromatase deficiency caused by a novel P450arom gene mutation: impact of absent estrogen production on serumgonadotropin concentration in a boy. J Clin Endocrinol Metab. (1999) 84:4050–4. doi: 10.1210/jc.84.11.4050
81. Herrmann BL, Saller B, Janssen OE, Gocke P, Bockisch A, Sperling H, et al. Impact of estrogen replacement therapy in a male with congenital aromatase deficiency caused by a novel mutation in the CYP19 gene. J Clin Endocrinol Metab. (2002) 87:5476–84. doi: 10.1210/jc.2002-020498
82. Maffei L, Murata Y, Rochira V, Tubert G, Aranda C, Vazquez M, et al. Dysmetabolic syndrome in a man with a novel mutation of the aromatase gene: effects of testosterone, alendronate, and estradiol treatment. J Clin Endocrinol Metab. (2004) 89:61–70. doi: 10.1210/jc.2003-030313
83. Maffei L, Rochira V, Zirilli L, Antunez P, Aranda C, Fabre B, et al. A novel compound heterozygous mutation of the aromatase gene in an adult man: reinforced evidence on the relationship between congenital oestrogen deficiency, adiposity and the metabolic syndrome. Clin Endocrinol. (2007) 67:218–24. doi: 10.1111/j.1365-2265.2007.02864.x
84. Lanfranco F, Zirilli L, Baldi M, Pignatti E, Corneli G, Ghigo E, et al. A novel mutation inthe human aromatase gene: insights on the relationship among serum estradiol, longitudinal growth and bone mineral density in an adult man under estrogen replacement treatment. Bone. (2008) 43:628–35. doi: 10.1016/j.bone.2008.05.011
85. Baykan EK, Erdogan M, Özen S, Darcan S, Saygili LF. Aromatase deficiency, a rare syndrome: case report. J Clin Res Pediatr Endocrinol. (2013) 5:129–32. doi: 10.4274/Jcrpe.970
86. Bouchoucha N, Samara-Boustani D, Pandey AV, Bony-Trifunovic H, Hofer G, Aigrain Y, et al. Characterization of a novel CYP19A1 (aromatase) R192H mutation causing virilization of a 46,XX newborn, under virilization of the 46,XY brother, but no virilization of the mother during pregnancies. Mol Cell Endocrinol. (2014) 390:8–17. doi: 10.1016/j.mce.2014.03.008
87. Chen Z, Wang O, Nie M, Elison K, Zhou D, Li M, et al. Aromatase deficiency in a Chinese adult man caused by novel compound heterozygous CYP19A1 mutations: effects of estrogen replacement therapy on the bone, lipid, liver and glucose metabolism. Mol Cell Endocrinol. (2015) 399:32–42. doi: 10.1016/j.mce.2014.09.016
88. Pepe CM, Saraco NI, Baquedano MS, Guercio G, Vaiani E, Marino R, et al. The cytochrome P450 aromatase lacking exon 5 is associated with a phenotype of nonclassic aromatase deficiency and is also present in normal human steroidogenic tissues. Clin Endocrinol (Oxf). (2007) 67:698–705. doi: 10.1111/j.1365-2265.2007.02948.x
89. Miedlich SU, Karamooz N, Hammes SR. Aromatase deficiency in a male patient - case reportand review of the literature. Bone. (2016) 93:181–6. doi: 10.1016/j.bone.2016.09.024
90. Costanzo M, Garcia-Feyling J, Saraco N, Marino R, Pérez Garrido N, Touzon MS, et al. Accelerated pubertal tempo in a 46, XY aromatase-deficient patient. Horm Res Paediatr. (2018) 90:275–82. doi: 10.1159/000492128
91. Rochira V, Zirilli L, Genazzani AD, Balestrieri A, Aranda C, Fabre B, et al. Hypothalamic-pituitary-gonadal axis in two men with aromatase deficiency: evidence that circulating estrogens are required at the hypothalamic level for the integrity of gonadotropin negative feedback. Eur J Endocrinol. (2006) 155:513–22. doi: 10.1530/eje.1.02254
Keywords: cP450arom, ArKO, ERα, ERβ, ERKO, human male reproduction
Citation: Guercio G, Saraco N, Costanzo M, Marino R, Ramirez P, Berensztein E, Rivarola MA and Belgorosky A (2020) Estrogens in Human Male Gonadotropin Secretion and Testicular Physiology From Infancy to Late Puberty. Front. Endocrinol. 11:72. doi: 10.3389/fendo.2020.00072
Received: 28 August 2019; Accepted: 03 February 2020;
Published: 25 February 2020.
Edited by:
Rodolfo A. Rey, Hospital de Niños Ricardo Gutiérrez, ArgentinaReviewed by:
Dana Manuela Savulescu, National Institute of Communicable Diseases (NICD), South AfricaWinnie Shum, ShanghaiTech University, China
Copyright © 2020 Guercio, Saraco, Costanzo, Marino, Ramirez, Berensztein, Rivarola and Belgorosky. This is an open-access article distributed under the terms of the Creative Commons Attribution License (CC BY). The use, distribution or reproduction in other forums is permitted, provided the original author(s) and the copyright owner(s) are credited and that the original publication in this journal is cited, in accordance with accepted academic practice. No use, distribution or reproduction is permitted which does not comply with these terms.
*Correspondence: Alicia Belgorosky, YWJlbGdvMTIzNDVAZ21haWwuY29t
†These authors have contributed equally to this work
‡ORCID: Alicia Belgorosky orcid.org/0000-0002-4234-400X