- 1Endocrinology and Nutrition Department, Institut de Recerca Biomèdica de Lleida (IRBLleida), Hospital Universitari Arnau de Vilanova, Obesity, Diabetes and Metabolism Research Group (ODIM), Universitat de Lleida (UdL), Lleida, Spain
- 2Respiratory Department, Institut de Recerca Biomèdica de Lleida (IRBLleida), Hospital Universitari Arnau de Vilanova-Santa María, Translational Research in Respiratory Medicine, Universitat de Lleida (UdL), Lleida, Spain
- 3Centro de Investigación Biomédica en Red de Enfermedades Respiratorias (CIBERES), Instituto de Salud Carlos III (ISCIII), Madrid, Spain
- 4Section of Hormones, Clinic Laboratory, Hospital Universitari Arnau de Vilanova, Universitat de Lleida (UdL), Lleida, Spain
- 5Clinic Laboratory, Hospital Universitari Arnau de Vilanova, Universitat de Lleida (UdL), Lleida, Spain
- 6Clinic Laboratory, Department of Laboratory Medicine, Hospital Universitari Arnau de Vilanova, Universitat de Lleida (UdL), Lleida, Spain
- 7Diabetes and Metabolism Research Unit, Endocrinology and Nutrition Department, Vall d'Hebron Institut de Recerca (VHIR), Hospital Universitari Vall d'Hebron, Universitat Autònoma de Barcelona, Barcelona, Spain
- 8Centro de Investigación en Red en Diabetes y Enfermedades Metabólicas Asociadas (CIBERDEM), Instituto de Salud Carlos III (ISCIII), Madrid, Spain
Introduction: Many studies on the impact of type 2 diabetes mellitus (T2DM) on sleep breathing have shown a higher prevalence and severity of sleep apnea-hypopnea syndrome (SAHS) in those with T2DM. Moreover, an increased activity of the sympathetic nervous system has been described in both pathologies. This cross-sectional study aimed to assess sympathetic activity in patients with T2DM, and to investigate the relationship between sympathetic activity and polysomnographic parameters.
Materials and Methods: Thirty-six patients with T2DM without known clinical macrovascular nor pulmonary disease and 11 controls underwent respiratory polygraphy, and their cardiac variability and 24-h urine total metanephrines were measured.
Results: SAHS was highly prevalent with a mean apnea-hypopnea index (AHI) in the range of moderate SAHS. In patients with T2DM, the nocturnal concentration of total metanephrines in urine were higher than diurnal levels [247.0 (120.0–1375.0) vs. 210.0 (92.0–670.0), p = 0.039]. The nocturnal total metanephrine concentration was positively and significantly associatedwith the percentage of sleeping time spent with oxygen saturation <90%(CT90). In the entire population and in subjects with T2DM, the multivariate regression analysis showed a direct interaction between the nocturnal concentration of urine metanephrines and the CT90.
Conclusion: These findings suggest that the increase in sympathetic activity previously described in patients with T2DM could be mediated through nocturnal breathing disturbances. The diagnosis and treatment of SAHS may influence sympathetic activity disorders and may contribute to an improvement in T2DM and cardiovascular risk.
Introduction
A wealth of clinical and cross-sectional studies showed a higher prevalence of sleep apnoea-hypopnea syndrome (SAHS) in patients with type 2 diabetes (T2DM). The data vary according to the population studied, but reach a prevalence of up to 85%, with 70% for the range of moderate SAHS (1–5). Despite the lack of prospective studies to demonstrate the SAHS impact in patients with T2D, it is well known that intermittent nocturnal hypoxia is associated with poorer glycaemic control and an increase in cardiovascular risk (6–13). Alternatively, patients with T2D, in comparison with the general population have a negative impact on breathing during sleep, creating greater sleep fragmentation, an increase in micro-awakenings and a higher prevalence of nocturnal hypoxia episodes (14–18). However, there is little evidence and some controversy on whether these alterations are responsible for hyperactivation of the sympathetic nervous system described in patients with T2DM.
Usually, the autonomic nervous system shows episodic oscillations of broad-spectrum activity during sleep with dynamic fluctuation of vital factors such as heart rate, blood pressure and ventilation (19, 20). Moreover, changes in sleep architecture are associated with increased sympathetic activity, leading to endothelial dysfunction and decreased nitric oxide concentration (21–23). In this way, episodes of nocturnal hypoxia also activate the sympathetic system, promoting alterations in cardiac variability and probably endothelial damage, which contributes to increased cardiovascular risk (24–27). Sympathetic overactivity has also been associated with metabolic dysfunction such as insulin resistance, obesity, hypertension and dyslipidaemia (28–30). Even genetic polymorphisms have been identified the sympathetic nervous system of humans and animals and seem to contribute to the development of obesity and T2DM in certain populations (31–33).
Since the origin of sympathetic hyperactivation in patients with T2DM is not precisely known, we propose to analyse the relation between the presence of breathing disorders that characterize their sleep with data related with sympathetic activity. We aimed to deepen this hypothesis by studying the polysomnographic record of 36 subjects with T2DM and its relationship with measurement of urine metanephrine concentration, heart rate variability (HRV) and other serum markers of sympathetic activity.
Materials and Methods
Ethic Statement
The study was approved by the human ethics committee from the Arnau de Vilanova University Hospital and was conducted according to the ethical guidelines of the Helsinki Declaration. Informed written consent was obtained from all participants included in the study.
Description of the Study Population
In this cross-sectional study, a total of 36 patients of Caucasian origin with T2DM were prospectively recruited between September 2017 and July 2018 at the outpatient clinic in the Endocrinology Department of the Arnau de Vilanova University Hospital. Willing participants were given detailed information about the objectives and procedures of the study.
Participants fulfilled the following inclusion criteria: T2DM with more than 5 years of known evolution, men and women aged 40 and 70 years without any history of vascular disease or lung disease and a BMI of less than 40 kg/m2. The exclusion criteria were presence of type 1 diabetes, chronic kidney disease, active neoplasia, neuromuscular diseases, active smoking or former smokers, history of alcohol abuse and treatment with continuous positive airway pressure (CPAP). Patients who were treated with drugs with activity on the central nervous system (e.g., hypnotics, antidepressants, sedatives, psycholeptics) were also excluded from the study. No pregnant women were included. A control group of 11 healthy subjects without T2DM or lung disease and well matched by age, body mass index (BMI), neck and waist circumferences and blood pressure was also recruited during the same period among the relatives of patients with diabetes, as well as the employees of our institution.
Measurement of Sleep-Disordered Breathing
The 36 recruited patients and the 11 controls underwent a non-attended respiratory polygraphic record with a portable Sleep Diagnostic Alice PDx (Philips Respironics, Spain) which records nasal airflow (nasal cannula), respiratory effort (chest and abdominal bands), snoring, body position and finger pulse oximetry. The same technician manually checked all sleep studies to reduce variability. Records with less than 5 h of correct signal were repeated. An apnea was defined as cessation of airflow for more than 10 s. Hypopnea was defined as a reduction in respiratory signals for at least 10 s associated with oxygen desaturation of 4% or more. The apnea-hypopnea index (AHI) was calculated as the total number of respiratory events (apneas plus hypopneas) divided by the recording time in bed (per hour of sleep). On this basis, SAHS was defined as an AHI >10 events/hour (e/h). The cumulative percentage of time spent with oxygen saturations below 90% (CT90) was also assessed (34–36).
Laboratory Assessment
After patients fasted overnight for 12 h, venous blood was collected from the antecubital vein. Samples were separated by centrifugation (2,000 g at 4°C for 20 min) and analyzed in the clinical laboratory of our hospital using standard methods to obtain biochemical parameters. The measurement of urine metanephrines was realized by high performance liquid chromatography. In order to compare the concentrations of urine metanephrines between day and night, urine collection was carried out in two 12-h time periods [from 8:00 to 20:00 (day) and from 20:00 to 8:00 (night)]. The serum concentration of hormones related with glucose metabolism [cortisol, insulin, glucagon, insulin-like growth factor-1 (IGF-1) and growth hormone (GH)] were also determined through chemiluminescence enzyme immunoassay. All samples were collected in the absence of clinical evidence of active infection or inflammatory diseases.
Cardiac Variability Study: Heart Rate Variability Parameters
Heart rate variability (HRV), defined as the measurement of the variation in milliseconds between heart beats, was studied in its R-R intervals, through a QHRV device with DT-HW6c hardware (QHRV, Santa Bárbara, CA, United States). The results were analyzed after measuring in four different stages: after a 15-min rest, breathing deeply, in Valsalva and in a standing position. In the time domain, we analyzed RR intervals, standard deviations of RR intervals (SDNN), the square root of the mean squared difference of successive RR intervals (RMSSD), and the percentage of adjacent NN intervals differing by more than 50 ms (pNN50). This last measurement was referred to as “short-term” HRV and reflects the parasympathetic influence on the heart rate. In the frequency domain, we analyzed the low-frequency band (LF, an index of both sympathetic and parasympathetic activity), high-frequency band (HF) and very low-frequency (VLF, that reflects thermoregulatory mechanisms, fluctuation in activity of the renin–angiotensin system, and the function of peripheral chemoreceptors) (37). Patients were advised not to consume food, coffee or nicotine 3 h before the test and to not receive treatment with anticholinergics, fludrocortisone, antihistamines, alpha or beta antagonists, or anxiolytics during 48 h before the test.
Statistical Analysis
Normal distribution of the variables was assessed using the Kolmogorov-Smirnov test. Data were expressed as the mean ± standard deviation or percentage. Given their skewed distribution, AHI, CT90 and serum triglycerides are shown as median (range) and were logarithmically transformed to achieve a normal distribution. The relationship between the continuous variables was examined by the Pearson linear correlation test. Univariate and multivariate logistic regression models were computed. A stepwise multivariate regression analysis was performed to explore the variables independently related to nocturnal urine metanephrines. Variables significantly associated with changes in urine metanephrines in the bivariate analysis (i.e., CT90 and serum glucagon concentration), together with heart rate variability parameters and clinically relevant variables (i.e., gender, BMI, age, smoking habit, blood pressure, AHI, neck circumference and HbA1c) were included in the analysis. All p-values were based on a two-sided test of statistical significance. Significance was accepted at the level of p < 0.05. Statistical analyses were performed using the SPSS statistical package (IBM SPSS, Statistics for Windows, Version 20.0. Armonk, NY, USA).
Results
The main clinical features and metabolic data of the study population are presented in Table 1. Briefly, patients with T2DM were 56.3 ± 8.7 years old, mainly female (27.7%) and obese (BMI 31.9 ± 4.8 kg/m2) and showed a poor metabolic control (HbA1c 8.0 ± 1.3%). Regarding measurements from the non-attended polygraphy, they exhibited a median AHI of 23.5 (3.0–78.0) e/h, with a median CT90 of 7.5 (0–64)%. Finally, hormone values and HRV parameters were within the normal range (Table 2).
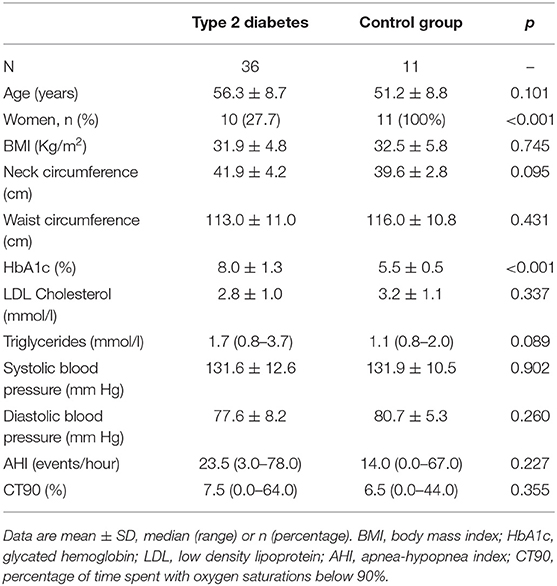
Table 1. Baseline main clinical, metabolic and sleep-breathing characteristics of participants in the study.
Urine concentrations of total metanephrines were statistically higher during the night compared with the concentrations during the day period in the group of subjects with T2DM [247.0 (120.0.1375.0) vs. 210.0 (92.0–670.0), p = 0.039]. However, no differences between day and night were observed in the control group [243.0 (104.0–560.0) vs. 250.5 (94.0–485.0), p = 0.674).
Linear correlations between day and night metanephrines and serum hormones and heart rate variability parameters are shown in Table 3. Whereas the nocturnal concentration of urine metanephrines were positively associated with values of CT90 (r = 0.617, p < 0.001), this association disappeared with the metanephrines collected during the day (r = 0.093, p = 0.644) (Figure 1). In addition, nocturnal and daily urine metanephrines were negatively associated with serum glucagon concentrations (r = −0.427, p = 0.008). In the multivariate regression analysis including the entire population we found a significant direct interaction between the concentration of urine metanephrines at night and the percentage of time spent with oxygen saturation <90% (p = 0.001) [together with serum glucagon concentration (p = 0.001), LFa/RFa resting (p = 0.009) and diastolic blood pressure (p = 0.047), R2 = 0.741]. This indicated that the increase in the secretion of metanephrines at night could be related with the severity of nocturnal hypoxia (Table 4). When subjects with T2DM were assessed alone, serum glucagon concentrations (p = 0.011) and CT90 (p = 0.016) still independently predicted nocturnal urine metanephrines (R2 = 0.549). However, in the control group, only diastolic blood pressure and serum glucagon concentrations but not CT90 were significantly related with nocturnal total metanephrines.
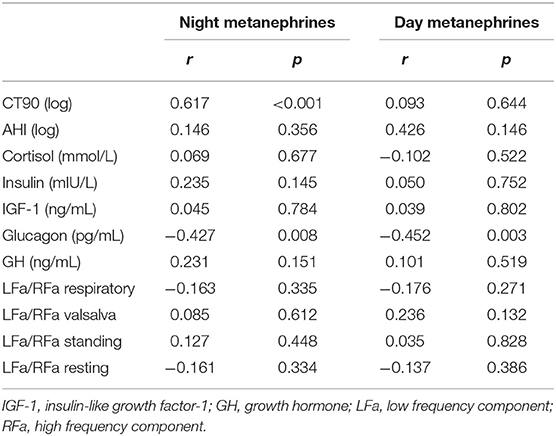
Table 3. Linear correlations between day and night metanephrines and serum hormones and heart rate variability parameters.
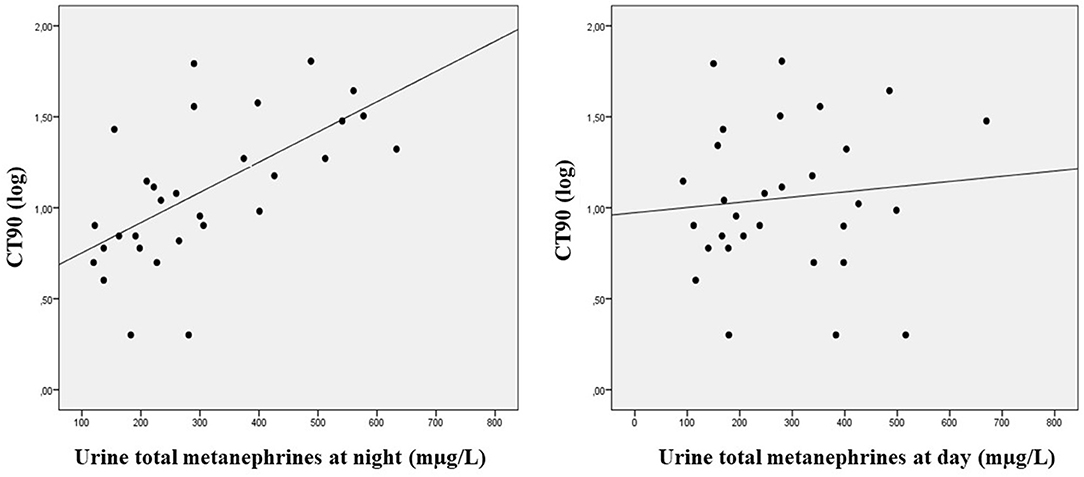
Figure 1. Linear correlation between concentration of urine metanephrines and percentage of time with oxygen saturations below 90%.
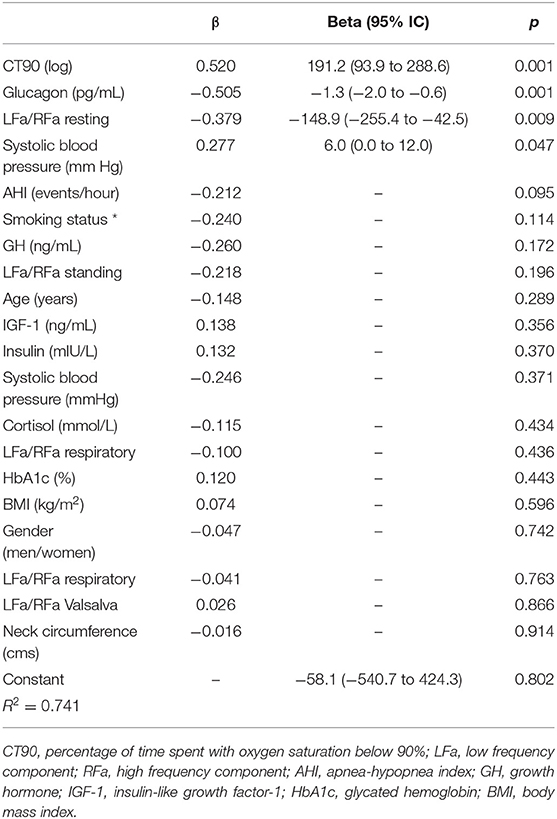
Table 4. Variables independently related to nocturnal urine total metanephrines in the multiple regression analysis (stepwise method) in the entire population.
Discussion
The main findings in this study were that a subgroup of patients with T2DM exhibited an increase in urine metanephrines at night associated with nocturnal hypoxia and with a decrease in serum glucagon concentrations, specifically for the parasympathetic tone. This autonomic disturbance can be explained by the deleterious effects of diabetes during night-time sleep, leading to an important nocturnal hypoxia.
It is well known that T2DM patients exhibit a hyperactivation of the sympathetic nervous system (SNS) that amplifies the effect of hyperglycaemia, increasing both cardiovascular risk and microangiopathic diabetic complications (38). The origin of this sympathetic hyperactivation has not been precisely described. We propose that, both intermittent hypoxia and the greater number of micro-awakenings that characterize the sleep of T2DM patients could explain the increase in SNS activity.
While the evidence is controversial, previous studies have demonstrated that apnea and hypopnea events are accompanied by concomitant cyclic variations in heart rate and changes in both sympathetic and parasympathetic activity without differences in subjects with diabetes (39, 40). As far as we know, hyperactivation of the autonomic system has been described in patients with T2DM but with involvement of both patterns (sympathetic and parasympathetic) and it was related to male sex and the presence of neuropathy (41–43). None of our patients had clinical neuropathy, and the test group was predominantly female.
Although we cannot elucidate whether the effects of hypoxia and diabetes overlap with the effects of obesity, some recent studies have shown an increased SAHS prevalence in non-overweight patients with Type 1 diabetes, suggesting that nocturnal hypoxia might be more related to autonomic disturbances rather than obesity (44, 45).
Our study combined data from men and women in the analyses, but differences regarding sex and menopausal status have been previously described. In this way, some findings suggest that men have a higher sympathetic nerve activity than women after the development of essential hypertension, and that this could have implications for gender-specific management of hypertension (46, 47). Similarly, muscle sympathetic nerve activity has been described to be greater in postmenopausal women in comparison with premenopausal women (48). In our study, the LFa/RFa respiratory parameter was the only parameter related with heart rate variability that was significantly decreased in women when compared to men [0.7 (0.2–1.7) vs. 0.4 (0.2–0.8), p = 0.002], and it was also the only parameter that was positively associated with age in the bivariate analysis (r = 0.318, p = 0.038). The other heart rate variability parameters and serum hormone concentrations showed no differences between genders nor correlations with age. In addition, gender and age were not independently associated with total nocturnal urine metanephrines in the multivariate analysis.
The present study does not have sufficient power to exhibit endothelial damage or cardiovascular risk caused by this hyperactivity, but some previous studies have described the relationship between variability in autonomic function and an increase in insulin resistance, oxidative stress and inflammatory response that cause endothelial celldamage, development of atherosclerosis and increased cardiovascular risk (49–51). Along the same lines, it has been described that cardiovascular autonomic neuropathy contributes to a poorer prognosis in ischemic heart disease and heart failure (52). Also, the decrease in HRV can predict the progression of atherosclerosis in T2DM patients (53).
Our study has some limitations. First, this is a cross sectional whereby causality cannot be determined. The size of the study might have been inadequate, and a prospective study with a higher number of patients could yield better results. In addition, it should be noted that the control group in our study was composed only of women. As previously mentioned, women have a lower sympathetic nerve activity than men, so this data could influence our results. However, in the multivariate regression analysis, gender was not related to the concentration of urine metanephrines at night. Second, the HRV study might have been influenced by differences in baseline physical condition or cardiac status. We did not perform any cardiac evaluation although all participants were asked for cardiovascular diseases and arrhythmias and neither of them were athletic. Third, the effects of the antidiabetics (insulin, SGLT2, incretins) could not be excluded.
Conclusion
We suggest that the increased sympathetic activity previously described in patients with T2DM is mediated through the deleterious effect of diabetes in nocturnal breathing. In addition, sympathetic activity is associated with disorders of autonomic tone at resting, suggesting a new pathological pathway between T2DM and cardiovascular risk. More studies are needed to clarify whether treatment of SAHS will improve sympathetic hyperactivity, T2DM and cardiovascular outcomes in these patients.
Data Availability Statement
The datasets generated for this study are available on request to the corresponding author.
Author Contributions
CL-C, LG-C, and ES recruited patients, collected and analyzed data, wrote the first draft of the manuscript, and had final approval of the version for publication. FB supervised the research, interpreted data, and critically reviewed the draft of the article. JG, SB, ES, MR, and RM collected and analyzed data, and critically reviewed the draft of the article. LG-C, MH, and XG recruited patients, collected data, and contributed to the discussion. GC, MR, and AY collected and analyzed data, and contributed to the discussion. CH and RS designed the study, supervised the statistical analysis, interpreted data, critically revised draft of the article, and had final approval of the version for publication. CL-C and AL designed the study, supervised the research, analyzed and interpreted data, and wrote the manuscript.
Funding
This study was supported by grants from the Instituto de Salud Carlos III (Fondo de Investigación Sanitaria, PI12/00803 and PI15/00260), European Union (European Regional Development Fund, Fondo Europeo de Desarrollo Regional, Una manera de hacer Europa), the Fundación Sociedad Española Endocrinología y Nutrición, and Menarini Spain S.A. The funders had no role in the study design, data collection and analysis, decision to publish, or preparation of the manuscript.
Conflict of Interest
The authors declare that the research was conducted in the absence of any commercial or financial relationships that could be construed as a potential conflict of interest.
References
1. Foster GD, Sanders MH, Millman R, Zammit G, Borradaile KE, Newman AB, et al. Obstructive sleep apnea among obese patients with type 2 diabetes. Diabetes Care. (2009) 32:1017–19. doi: 10.2337/dc08-1776
2. West SD, Nicoll DJ, Stradling JR. Prevalence of obstructive sleep apnoea in men with type 2 diabetes. Thorax. (2006) 61:945–50. doi: 10.1136/thx.2005.057745
3. Heffner JE, Rozenfeld Y, Kai M, Stephens EA, Brown LK. Prevalence of diagnosed sleep apnea among patients with type 2 diabetes in primary care. Chest. (2012) 141:1414–21. doi: 10.1378/chest.11-1945
4. Lecomte P, Criniere L, Fagot-Campagna A, Druet C, Fuhrman C. Underdiagnosis of obstructive sleep apnoea syndrome in patients with type 2 diabetes in France: ENTRED 2007. Diabetes Metab. (2013) 39:139–47. doi: 10.1016/j.diabet.2012.10.004
5. Hermans MP, Ahn SA, Rousseau MF. Cardiometabolic phenotype and UKPDS risk in male type 2 diabetic patients with obstructive sleep apnoea. Diabetes Metab Synd Clin Res Rev. (2009) 3:50–4. doi: 10.1016/j.dsx.2008.10.011
6. Aronsohn RS, Whitmore H, Van Cauter E, Tasali E. Impact of untreated obstructive sleep apnea on glucose control in type 2 diabetes. Am J Respir Crit Care Med. (2010) 181:507–13. doi: 10.1164/rccm.200909-1423OC
7. Pamidi S, Tasali E. Obstructive sleep apnea and type 2 diabetes: is there a link? Front Neurol. (2012) 3:126. doi: 10.3389/fneur.2012.00126
8. Drager LF, Lopes HF, Maki-Nunes C, Trombetta IC, Toschi-Dias E, Alves MJ, et al. The impact of obstructive sleep apnea on metabolic and inflammatory markers in consecutive patients with metabolic syndrome. PLoS ONE. (2010) 5:e12065. doi: 10.1371/journal.pone.0012065
9. Brady EM, Davies MJ, Hall AP, Talbot DC, Dick JL, Khunti K. An investigation into the relationship between sleep-disordered breathing, the metabolic syndrome, cardiovascular risk profiles, and inflammation between South Asians and Caucasians residing in the United Kingdom. Metab Syndr Relat Disord. (2012) 10:152–8. doi: 10.1089/met.2011.0073
10. Tancredi M, Rosengren A, Svensson A-M, Kosiborod M, Pivodic A, GudbjÖrnsdottir S, et al. excess mortality among persons with type 2 diabetes. N Engl J Med. (2015) 373:1720–32. doi: 10.1056/NEJMoa1504347
11. Newman AB, Nieto FJ, Guidry U, Lind BK, Redline S, Pickering TG, et al. Relation of sleep-disordered breathing to cardiovascular disease risk factors: the sleep heart health study. Am J Epidemiol. (2001) 154:50–9. doi: 10.1093/aje/154.1.50
12. Tobaldini E, Costantino G, Solbiati M, Cogliati C, Kara T, Nobili L, et al. Sleep, sleep deprivation, autonomic nervous system and cardiovascular diseases. Neurosci Biobehav Rev. (2017) 74(Pt B):321–9. doi: 10.1016/j.neubiorev.2016.07.004
13. Parish JM, Somers VK. Obstructive sleep apnea and cardiovascular disease. Mayo Clin Proc. (2004) 79:1036–46. doi: 10.4065/79.8.1036
14. Engeda J, Mezuk B, Ratliff S, Ning Y. Association between duration and quality of sleep and the risk of pre-diabetes: evidence from NHANES. Diabet Med. (2013) 30:676–80. doi: 10.1111/dme.12165
15. Grandner MA, Seixas A, Shetty S, Shenoy S. Sleep duration and diabetes risk: population trends and potential mechanisms. Curr Diab Rep. (2016) 16:106. doi: 10.1007/s11892-016-0805-8
16. Lee SWH, Ng KY, Chin WK. The impact of sleep amount and sleep quality on glycemic control in type 2 diabetes: a systematic review and meta-analysis. Sleep Med Rev. (2017) 31:91–101. doi: 10.1016/j.smrv.2016.02.001
17. Resnick HE, Redline S, Shahar E, Gilpin A, Newman A, Walter R, et al. Diabetes and sleep disturbances: findings from the Sleep Heart Health Study. Diabetes Care. (2003) 26:702–9. doi: 10.2337/diacare.26.3.702
18. Lecube A, Sampol G, Lloberes P, Romero O, Mesa J, Hernández C, et al. Diabetes is an independent risk factor for severe nocturnal hypoxemia in obese patients. A case control study. PLoS ONE. (2009) 4:e4692. doi: 10.1371/journal.pone.0004692
19. Chokroverty S. Neurophysiological and neurochemical mechanisms of wakefulness and sleep. In: Clinical Companion to Sleep Disorders. Medicine, 2nd ed. Butterworth Heinemann (2000).
20. Verrier RL, Harper RM, Hobson JA. Cardiovascular physiology: central and autonomic regulation. In: Kryger MH, Roth T, Dement WC, Editors. Principles and Practice of Sleep Medicine. Philadelphia: Saunders (2000).
21. Meerlo P, Sgoifo A, Suchecki D. Restricted and disrupted sleep: effects on autonomic function, neuroendocrine stress systems and stress responsivity. Sleep Med Rev. (2008) 12:197–210. doi: 10.1016/j.smrv.2007.07.007
22. Tasali E, Leproult R, Ehrmann DA, Van Cauter E. Slow-wave sleep and the risk of type 2 diabetes in humans. Proc Natl Acad Sci USA. (2008) 105:1044–9. doi: 10.1073/pnas.0706446105
23. Stamatakis KA, Punjabi NM. Effects of sleep fragmentation on glucose metabolism in normal subjects. Chest. (2010) 137:95–101. doi: 10.1378/chest.09-0791
24. Smith ML, Niedermaier ON, Hardy SM, Decker MJ, Strohl KP. Role of hypoxemia in sleep apnea-induced sympathoexcitation. J Auton Nerv Syst. (1996) 56:184–90. doi: 10.1016/0165-1838(95)00062-3
25. Narkiewicz K, Somers VK. Sympathetic nerve activity in obstructive sleep apnoea. Acta Physiol Scand. (2003) 177:385–90. doi: 10.1046/j.1365-201X.2003.01091.x
26. Imadojemu VA, Mawji Z, Kunselman A, Gray KS, Hogeman CS, Leuenberger UA. Sympathetic chemoreflex responses in obstructive sleep apnea and effects of continuous positive airway pressure therapy. Chest. (2007) 131:1406–13. doi: 10.1378/chest.06-2580
27. Xie A, Skatrud JB, Puleo DS, Morgan BJ. Exposure to hypoxia produces long-lasting sympathetic activation in humans. J Appl Physiol. (2001) 91:1555–62. doi: 10.1152/jappl.2001.91.4.1555
28. Conde S, Sacramento JF, Guarino MP, Gonzalez C, Obeso A, Diogo LN. Carotid body, insulin, and metabolic diseases: unraveling the links. Front Physiol. (2014) 5:418. doi: 10.3389/fphys.2014.00418
29. Fletcher EC. Sympathetic over activity in the etiology of hypertension of obstructive sleep apnea. Sleep. (2003) 26:15–9. doi: 10.1093/sleep/26.1.15
30. Tsioufis C, Kordalis A, Flessas D, Anastasopoulos I, Tsiachris D, Papademetriou V, et al. Pathophysiology of resistant hypertension: the role of sympathetic nervous system. Int J Hypertens. (2011) 2011:642416. doi: 10.4061/2011/642416
31. Wang H, Wu M, Zhu W, Shen J, Shi X, Yang J, et al. Evaluation of the association between the AC3 genetic polymorphisms and obesity in a Chinese Han population. PLoS ONE. (2010) 5:e1385. doi: 10.1371/journal.pone.0013851
32. Jenkinson CP, Cray K, Walder K, Herzog H, Hanson, Ravussin E. Novel polymorphisms in the neuropeptide-Y Y5 receptor associated with obesity in Pima Indians. Int J Obes Relat Metab Disord. (2000) 24:580–4. doi: 10.1038/sj.ijo.0801200
33. Nonogaki, K. New insights into sympathetic regulation of glucose and fat metabolism. Diabetologia. (2000) 43:533e549. doi: 10.1007/s001250051341
34. Esnaola S, Duran J, Infante-Rivard C, Rubio R, Fernández A. Diagnostic accuracy of a portable device (Mesam IV) in suspected sleep apnoea. Eur Respir J. (1996) 9:2597–605. doi: 10.1183/09031936.96.09122597
35. Lloberes P, Sampol G, Levy G, Aristizabal D, Sagales T, et al. Influence of setting on unattended respiratory monitoring in the sleep apnoea-hypopnoea syndrome. Eur Respir J. (2001) 16:530–4. doi: 10.1183/09031936.01.00072401
36. Mbata G, Chukwuka J. Obstructive sleep apnea hypopnea syndrome. Ann Med Health Sci Res. (2012) 2:74–7. doi: 10.4103/2141-9248.96943
37. Otzenberger H, Gronfier C, Simon C, Charloux A, Ehrhart J, Piquard F, et al. Dynamic heart rate vari-ability: a tool for exploring sympathovagal balance continuously during sleep in men. Am J Physiol. (1998) 275:H946–950. doi: 10.1152/ajpheart.1998.275.3.H946
38. Benichou T, Pereira B, Mermillod M, Tauveron I, Pfabigan D, Maqdasy S, et al. Heart rate variability in type 2 diabetes mellitus: a systematic review and meta-analysis. PLoS ONE. (2018) 13:e0195166. doi: 10.1371/journal.pone.0195166
39. Guilleminault C, Connolly S, Winkle R, Melvin K, Tilkian A. Cyclical variation of heart rate in sleep apnea syndrome. Lancet. (1984) 1:126e31. doi: 10.1016/S0140-6736(84)90062-X
40. Jo JA, Blasi A, Valladares E, Juarez R, Baydur A, Khoo MC. Determinants of heart rate variability in obstructive sleep apnea syndrome during wakefulness and sleep. Am J Physiol Heart Circ Physiol. (2005) 288:H1103e12. doi: 10.1152/ajpheart.01065.2003
41. Laitinen T, Lindström J, Eriksson J, Ilanne-Parikka P, Aunola, Keinänen-Kiukaanniemi S, et al. Cardiovascular autonomic dysfunction is associated with central obesity in persons with impaired glucose tolerance. Diabet Med. (2011) 28:699–704. doi: 10.1111/j.1464-5491.2011.03278.x
42. Subbalakshmi NK, Adhikari PM, Rajeev A, Asha K, Jeganathan PS. Independent predictors of cardiac parasympathetic dysfunction in type 2 diabetes mellitus. Singapore Med J. (2008) 49:121–8.
43. Jyotsna VP, Ambekar S, Singla R, Joshi A, Dhawan A, Kumar N, et al. Cardiac autonomic function in patients with diabetes improves with practice of comprehensive yogic breathing program. Indian J Endocrinol Metab. (2013) 17:480–5. doi: 10.4103/2230-8210.111645
44. Banghoej AM, Nerild HH, Kristensen PL, Pedersen-Bjergaard U, Fleischer J, Jensen AEK, et al. Obstructive sleep apnoea is frequent in patients with type 1 diabetes. J Diab Complicat. (2017) 31:156–61. doi: 10.1016/j.jdiacomp.2016.10.006
45. Janovsky CCPS, Rolim LCdSP, Sá JRd, Poyares D, Tufik S, Silva AB, et al. Cardiovascular autonomic neuropathy contributes to sleep apnea in young and lean type 1 diabetes mellitus patients. Front Endocrinol. (2014) 5:119. doi: 10.3389/fendo.2014.00119
46. Matsukawa T, Sugiyama Y, Watanabe T, Kobayashi F, Mano T. Gender difference in age-related changes in muscle sympathetic nerve activity in healthy subjects. Am J Physiol. (1998) 275:R1600–4. doi: 10.1152/ajpregu.1998.275.5.R1600
47. Hogarth AJ, Burns J, Mackintosh AF, Mary DA. Sympathetic nerve hyperactivity of essential hypertension is lower in postmenopausal women than men. J Hum Hypertens. (2008) 22:544–9. doi: 10.1038/jhh.2008.31
48. Hogarth AJ, Graham LN, Corrigan JH, Deuchars J, Mary DA, Greenwood JP. Sympathetic nerve hyperactivity and its effect in postmenopausal women. J Hypertens. (2011) 29: 2167–75 doi: 10.1097/HJH.0b013e32834b8014
49. Thiyagarajan R, Subramanian SK, Sampath N, Madanmohan Trakroo, Pal P, Bobby Z, et al. Association between cardiac autonomic function, oxidative stress and inflammatory response in impaired fasting glucose subjects: cross-sectional study. PLoS ONE. (2012) 7:e41889. doi: 10.1371/journal.pone.0041889
50. Kratnov AE, Lysenkova NO. Parameters of heart rhythm variability and QT-interval in patients with ischemic heart disease and type 2 diabetes. Kardiologiia. (2010) 50:27–31.
51. Piwonska A, Piotrowski W, Broda G, Drygas W, Głuszek J, Zdrojewski T, et al. The relationship between resting heart rate and atherosclerosis risk factors. Kardiol Pol. (2008) 66:1069–75. discussion: 1076–8.
52. Vinik AI, Casellini C, Parson HK, Colberg SR, Nevoret ML. Cardiac autonomic neuropathy in diabetes: a predictor of cardiometabolic events. Front Neurosci. (2018) 12:591. doi: 10.3389/fnins.2018.00591
Keywords: type 2 diabetes, sympathetic hyperactivity, sleep apnea-hypopnea syndrome, sleep breathing, cardiovascular risk
Citation: López-Cano C, Gutiérrez-Carrasquilla L, Sánchez E, González J, Yeramian A, Martí R, Hernández M, Cao G, Ribelles M, Gómez X, Barril S, Barbé F, Hernández C, Simó R and Lecube A (2019) Sympathetic Hyperactivity and Sleep Disorders in Individuals With Type 2 Diabetes. Front. Endocrinol. 10:752. doi: 10.3389/fendo.2019.00752
Received: 05 August 2019; Accepted: 17 October 2019;
Published: 01 November 2019.
Edited by:
Gaetano Santulli, Columbia University, United StatesReviewed by:
Jan Brož, Charles University, CzechiaNirav Dhanesha, The University of Iowa, United States
Copyright © 2019 López-Cano, Gutiérrez-Carrasquilla, Sánchez, González, Yeramian, Martí, Hernández, Cao, Ribelles, Gómez, Barril, Barbé, Hernández, Simó and Lecube. This is an open-access article distributed under the terms of the Creative Commons Attribution License (CC BY). The use, distribution or reproduction in other forums is permitted, provided the original author(s) and the copyright owner(s) are credited and that the original publication in this journal is cited, in accordance with accepted academic practice. No use, distribution or reproduction is permitted which does not comply with these terms.
*Correspondence: Albert Lecube, YWxlY3ViZUBnbWFpbC5jb20=