- 1U.O. Endocrinologia, ASL Nord Ovest Toscana, Livorno, Italy
- 2Medical Affairs Department, Lo.Li. Pharma, Rome, Italy
- 3Department of Experimental Medicine, Sapienza University, Rome, Italy
Despite universal salt iodization programmes implemented over the last decades, iodine deficiency remains a major public health problem in many countries worldwide. Endeavors are still required to ensure sufficient iodine intake in the populations at risk in order to eliminate deficiency. Iodine is crucial for the synthesis of thyroid hormones triiodothyronine (T3) and thyroxine (T4), as well as for the thyroid health. When iodine levels are insufficient, T4 attests toward the lower limit of the physiological range, causing subtle fluctuations in the T3:T4 ratio. Monitoring these variations may be an accurate way to assess patient's iodine status. Recently, a number of published clinical studies documented a growing interest toward the use of myo-inositol in thyroid diseases. Myo-inositol, a carbocyclic polyol, regulates the generation of hydrogen peroxide (H2O2) in thyrocytes, crucial for iodine organification and thyroid hormone biosynthesis. Thus, combined supplementation of iodine and myo-inositol may promote higher iodine availability in thyrocytes improving thyroid functionality. This review presents novel strategies for the diagnosis and the management of iodine deficiency, focusing on the potential role of myo-inositol combined with iodine.
Introduction
Iodine deficiency (ID) is a major health problem in many countries worldwide. Even though the salt iodization programme has been operational for decades, 1.9 billion people are estimated at risk for ID (1). In case of severe ID, endemic goiter occurs, leading to cretinism as its most serious manifestation. Less severe forms have a broader spectrum of clinical manifestations. A higher incidence of ID is found among preschool children and gestating women in low-income countries. The condition exposes to several risks such as stillbirth, miscarriage, poor growth, and cognitive impairment (2). Several studies reported that even mild-to-moderate ID during pregnancy may have long-term adverse effects on child cognition (3). Goiter is the earliest sign of ID disorders (4) and incidence rate exceeds higher than 20% amongst children and women (5). Since iodine is needed to produce thyroid hormones, diminished levels can lead to hypothyroidism at any stage of life (6). Hence, the primary need is to drive the global elimination of ID (7).
Until the 1990s, the main indicator for the assessment of ID was the incidence of total goiter prevalence (TGP). Nowadays, urinary iodine (UI) concentration is used as a reliable indicator of iodine nutrition in the population. Interventions are planned accordingly. Surveillance is primarily done in preschool- and school-aged children to classify the national iodine status and the UI concentration distribution (8). According to the International Council for Control of Iodine Deficiency Disorders median UI concentrations below 100 μg/L for non-pregnant women and children define iodine deficient populations, while normal values for pregnant women should be between 150 and 249 μg/L. Regional and worldwide estimates of iodine status were collected between 1993 and 2003 from 126 countries (9). Only 75 of these have nationally representative surveys reporting data on UI that cover 45.7% of the school-age children population. These surveys have estimated in 2003 a global TGP incidence of 15.8%. Universal salt iodization is the most widely used strategy to manage ID, and globally, 68% of households worldwide now have access to iodized salt (10). The iodization program was previously restricted to the areas with severe endemic ID or with no access to iodized salt (2, 11). However, the presence of severe risks correlated with ID has extended the urgency of iodine supplementation to children and pregnant women worldwide (12). The survey in 2003 estimated that the iodine intake in school-aged children worldwide is insufficient (UI < 100 μg/L), covering ~285 million children (36.5%) (9) (Table 1). Iodine intake is insufficient (UI < 100 μg/L) in 35.2% of the general population. Among the World Health Organization (WHO) regions, the European general population has the highest proportion of insufficient iodine intake (56.9%) (9) (Table 1), while only 66% of school-aged children having adequate intakes in 2015 (13). Salt iodization programme has now started in many countries worldwide, and 71% of the population is covered by iodized salt (10). As recommended by WHO a low salt intake is advisable, and it should be iodized or “fortified” with iodine. According to the WHO guidelines, 150 μg per day of iodine are recommended for adults and 90–120 μg for children. During pregnancy a higher iodine intake is recommended (250 μg per day) because of additional thyroid hormones required to cover both maternal and fetal needs (2). In fact, fetal thyroid begins to work around the 12th week of gestation and, in early pregnancy, the fetus relies exclusively on maternal thyroid hormones supplied through the placenta. Therefore, an adequate nutritional intake of iodine guarantees maternal euthyroidism and fetus health. The Italian guidelines LARN (Reference Intake of nutrients and energy for Italian Population) (14), suggest the supplementation with 220 μg of iodine per day during pregnancy and with 290 μg during breastfeeding. Nevertheless, a survey study reported inadequate iodine intakes in two thirds of pregnant women in Europe (15). In Italy, the National Observatory for Monitoring the Iodine prophylaxis (OSNAMI) carries out routine surveillance activities. An interesting topic recently highlighted by a meta-analysis is the link between different levels of iodine intake and thyroid disease. Indeed, a higher incidence of thyroid nodules was observed in areas with medium and low iodine concentrations, compared to those with higher concentrations (16). This note was largely addressed also in the Medical Guidelines of the American Association of Clinical Endocrinologists (AACE), the American College of Endocrinology (ACE), and the Associazione Medici Endocrinologi (AME), where iodine supplementation is recommended in iodine-deficient geographic regions for the treatment of nodules (6). The importance of adequate nutritional intakes of iodine lies in the fact that it is an essential constituent of the thyroid hormones, triiodothyronine (T3) and thyroxine (T4). Such hormones play a critical role in cell differentiation, in the development of the central nervous system at the early stages of life and contribute to maintain the metabolic homeostasis during adult life. Recently, a number of published clinical trials documented a growing interest toward the use of myo-inositol for restoring proper functioning of the thyroid. Since the use of myo-inositol is gaining a positive opinion in the endocrinological field, exploring its potential role in the ID management is very appealing.
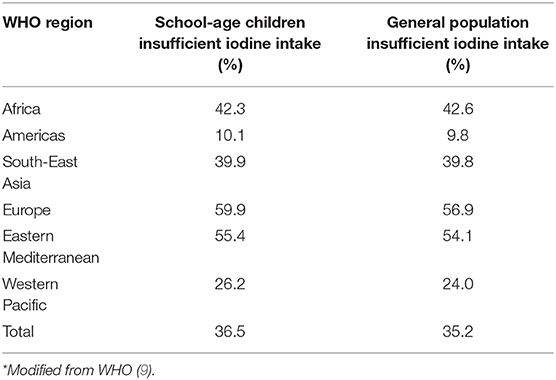
Table 1. Percentage of population (school-age children, 6–12 years and general population, all age groups) with insufficient iodine intake (UI < 100 μg/L) by WHO region: surveys carried out in 2003*.
Thyroid Hormones
Thyroid hormones T3 and T4 are produced and released through four important processes: uptake and oxidation of iodide, iodination of tyrosine, synthesis, resorption, and proteolysis of thyroglobulin (Tg). These processes depend on the availability of thyroid stimulating hormone (TSH) and iodine. Physiologically, a daily amount of 75 μg of iodine is required to produce 85 μg of T4. The thyroid actively incorporates the iodide through the basolateral plasma membrane of thyrocytes by the sodium/iodide symporter (NIS). Afterward the intracellular iodide is deposited in the lumen of thyroid follicles, storage site of Tg—a 660 kDa glycoprotein whose tyrosyl residues serve as substrate for iodination and hormone formation. The enzyme thyroid Peroxidase (TPO), sitting at the apical plasma membrane, uses hydrogen peroxide (H2O2) to oxidize and incorporate iodide in the tyrosyl groups of Tg. Dual oxidase (DUOX), a NADPH enzyme, endogenously generates H2O2 necessary for the process of the apex of the thyrocyte. Initial iodination of tyrosyl residues of Tg produces monoiodotyrosine (MIT) and diiodotyrosine (DIT). Homocoupling of DIT produces T4, while the coupling of DIT and MIT produces T3; both reactions are TPO-mediated. When thyroid hormones are required, Tg is transferred to the apical pole of thyrocytes, where it is then digested by proteases, namely endopeptidases cathepsins B, L, D, and exopeptidases. Following Tg digestion, hormones T3 and T4 are released. Approximately 70% of iodine from Tg (non-hormonal iodine) is recaptured intra-thyroidally by DEHAL1, an iodotyrosine deiodinase, and recycled within the gland. TSH is the stimulator that affects virtually every stage of thyroid hormone synthesis and release. The thyroid gland produces mainly T4, which reaches the organs where it is converted into T3, the most active form of thyroid hormones (17). The conversion of T4 into T3 is mediated by 5′-iodothyronine deiodinase either type I or II. Physiologically, a small amount of T4 is converted to reverse T3 by a 5-deiodinase (type III deiodinase): 80% of circulating T3 derives from deiodinase type I in liver and kidneys, while 20% is secreted directly from the thyroid gland (18, 19). In some diseases of the thyroid gland, the ratio of serum free T3 (FT3) to free T4 (FT4) can be altered. In case of inadequate intake of iodine, the T3:T4 ratio increases, presumably because the synthesis of T4 required 25% more iodine compared to T3 (20). Altered T3:T4 ratio was found in rats with iodine shortage (21) and in humans undergoing treatment with levothyroxine (L-T4). Arntzenius et al. demonstrated that the autoregulatory response to a lower iodine intake leads to decreased levels of circulating T4 (22), while serum T3 remains stable, or might even increase (23, 24). Altered T3:T4 ratios during endocrinological examination might be exploited as a novel tool for identifying iodine insufficiency.
Iodine
Iodine, a trace element found in soil and seawater, is relatively abundant in seafood, fruit and vegetables. It is ingested in several chemical forms that are reduced to iodide (25), which is absorbed in the stomach and in the duodenum. About 90% is excreted in the urine within 24–48 h (3, 7, 25, 26). Iodine is also released into the mammary gland in the breast milk to provide for the newborn (11, 27). The only physiological role known for iodine is the synthesis of thyroid hormones, comprising 58% of T3's weight, and 65% of T4's (2). Once T4 and T3 are degraded peripherally, iodide re-enters the plasma iodine pool and can be taken up again by the thyroid gland or excreted by the kidneys (10). When the thyroid works normally, plasma iodine has a half-life of about 10 h, shorter when the thyroid becomes overactive (25). Dietary iodine naturally occurs in the ion form, as potassium or sodium iodide, which is the preeminent substrate of the specific metabolism of thyrocytes, controlling thyroid functions. Either in vitro and in vivo iodide main roles are: (1) decreasing the response of TSH; (2) inhibiting its own oxidation (the Wolff-Chaikoff effect); (3) reducing its trapping after a delay; (4) at high levels, inhibiting thyroid hormone secretion (28). The Wolff–Chaikoff effect is an autoregulatory phenomenon that prevents the thyroid gland from synthesizing and releasing large quantities of thyroid hormones (19, 29) through the inhibition of organification when inorganic iodide levels in thyrocytes are too high (30, 31). The mechanism responsible for such effect is still unknown, but it may be ascribable to the inhibitory effect of iodide on TPO or other enzymes (19). Indeed, iodide is able to block the cyclic adenosine monophosphate cascade and the Ca2+-phosphatidylinositol 4, 5-bisphosphate (PIP2) cascade in thyrocytes and to induce H2O2 generation (28). H2O2 is crucial for the TPO-catalyzed thyroid hormone formation (32). In 1993, Chen at al. demonstrated in vitro that H2O2 specifically regulates iodide transport and organification in a dose-dependent manner (33). High H2O2 concentrations inhibit these functions and can be detrimental to the thyroid, although protection mechanisms prevents damages to the thyrocytes under physiological conditions (34). Intrathyroidal H2O2 generation, first reported about 50 years ago (35), and iodination are both stimulated by TSH. H2O2 can regulate iodination either directly, as a substrate of TPO, or indirectly by regulating the activity of TPO when iodide and Tg concentrations are kept constant.
Myo-Inositol
Myo-inositol, a carbocyclic polyol, belongs to the inositol family. Of nine possible structural isomers, it is the most widely distributed in nature, being present in fresh fruits, vegetables, cereals, legumes, and nuts. Myo-inositol is a fundamental component of structural lipids in cell membranes (36), such as phosphatidylinositol (PI) and the various phosphatidylinositol phosphates (PIPs). Myo-inositol is endogenously synthesized from glucose-6-phosphate and represents, in some tissues, about 99% of intracellular inositol. Initially incorporated at the level of cell membranes as phosphatidyl-myo-inositol, it is a precursor for many inositol-containing compounds involved in membrane biogenesis, signal transduction, vesicle trafficking and chromatin remodeling. It exerts important physiological functions, such as cell and tissue development, lipid synthesis, cell growth, and metabolism regulation. Myo-inositol is one of the precursors for the synthesis of PIPs, which are a source of second messengers like diacylglycerol. The latter regulates numerous species: members of the protein kinase C family; phosphatidylinositol-3,4,5-phosphate; inositol-1,4,5-triphosphate, which modulates intracellular calcium levels. As precursor also of the second messenger phosphoinositide, myo-inositol is involved in cell signaling and regulates the activities of hormones such as TSH, follicle-stimulating hormone (FSH) and insulin (37–39). It recently proved to be a very efficacious and safe treatment for subclinical hypothyroid patients with Autoimmune Thyroiditis (40–46). In 2013, Nordio and Pajalich demonstrated that treatment with myo-inositol plus selenium for 6 months in patients with subclinical hypothyroidism reduced significantly TSH concentrations by 31% (4.4 ± 0.9 mIU/mL vs. 3.1 ± 0.6 mIU/mL), compared to the control group treated only with selenium. These results were later corroborated by the same authors in a further clinical trial. Another study monitored TSH levels in Hashimoto patients with subclinical hypothyroidism receiving myo-inositol plus selenium for 6 months: significant decrease after only 3 months of treatment (from 4.38 ± 0.31 mIU/L at baseline to 3.42 ± 0.3 mIU/L) was observed, and even a greater reduction after 6 months (4.38 ± 0.31 mIU/L at baseline to 3.11 ± 0.2 mIU/L) was reported. No significant changes in the TSH levels in the control group, treated with only selenium, were observed (45). These data demonstrated that myo-inositol plus selenium effectively reduce TSH levels after a short-term supplementation, with even better results achieved after longer periods of treatment. Ferrari et al. confirmed that TSH levels significantly decreased in Hashimoto patients with subclinical hypothyroidism receiving myo-inositol plus selenium for 6 months. Interestingly, among these patients 46% of cases reached the normal TSH range (46). Furthermore, the treatment improved the levels of hormones and antibodies. In different studies, the action of myo-inositol was reported to balance the normal production of T3 and T4 hormones in patients with autoimmune thyroiditis (40, 43–45), as well as to reduce the blood TPO and Tg antibodies (47). A clinical trial reported a reduction in the antibody titer of 44 and 48%, respectively for anti-TPO and anti-Tg (40). A very recent study confirmed the immunomodulatory effect of myo-inositol combined with selenium in patients with autoimmune thyroiditis. In particular, the treatment maintained the euthyroid state, reducing the risk of developing either subclinical or over hypothyroidism (42).
The studies evaluating fT3 and fT4 reported a significant increase of serum fT4 levels after 6-month treatment with myo-inositol plus selenium, although remaining in the normal reference range (43, 45). Both studies reported significantly higher fT4 in patients treated for 6 months (from 1.05 ± 0.02 ng/dL at baseline to 1.142 ± 0.03 ng/dL, and from 0.93 ± 0.15 ng/dL at baseline to 1.06 ± 0.14 ng/dL, respectively). Interestingly, myo-inositol helps thyroid-hormone-producing cells to become more efficient and faster at building T4 (32, 41). This might be ascribable to a higher availability of iodine, whose organification is boosted by myo-inositol action. Indeed, myo-inositol is involved in one of the first steps of thyroid hormone production and modulates the H2O2-mediated iodination through the phospholipase C-dependent inositol phosphate Ca2+/diacylglycerol pathway, resulting in increased H2O2 generation (Figure 1). Differently, the cAMP cascade, induced by the TSH activity (through the TSH receptor activation), is more involved in cell growth and differentiation, and in thyroid hormones secretion. As myo-inositol plays a crucial role in the regulation of iodine organification, supplementation may promote faster recovery from ID. Indeed, H2O2 generated under the stimulus of myo-inositol is available for iodine incorporation inside the thyroid (33, 48). Such activity makes myo-inositol very appealing as a novel molecule to increase iodine availability.
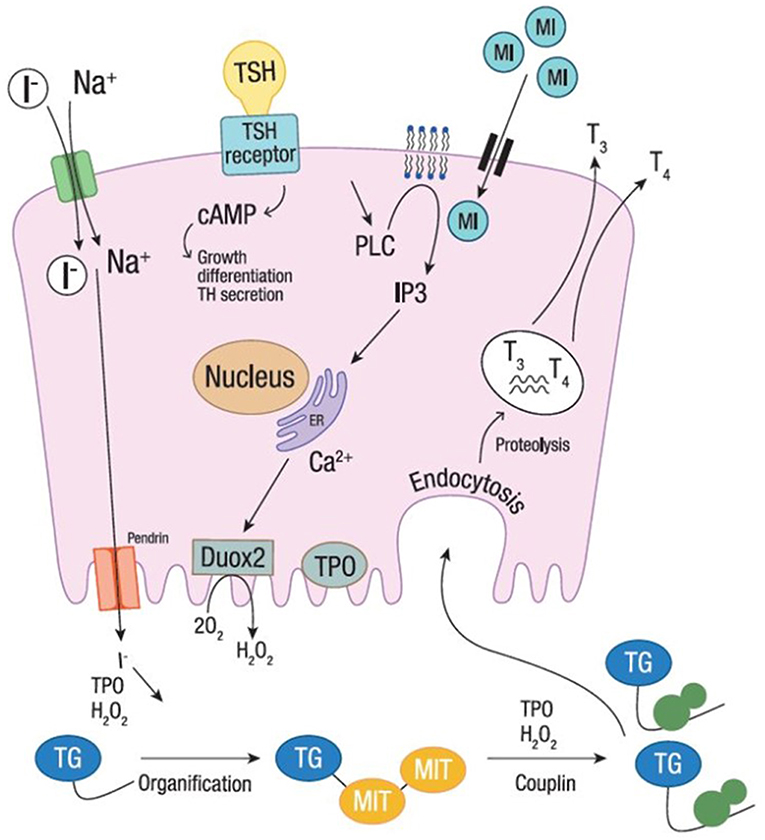
Figure 1. Iodine organification. Iodine organification into thyroid follicular cells. Iodide uptake is mediated by the sodium-iodide symporter, through the gradient generated by Na+. TPO, using H2O2 produced by DUOX2 system, mediates the oxidation, organification and coupling. The secretion of thyroid hormones is shown at the basolateral membrane. Myo-inositol regulates H2O2-mediated iodination through the phospholipase C-dependent inositol phosphate Ca2+/diacylglycerol pathway, resulting in a boost of H2O2 generation. The cAMP cascade, induced by the TSH activity (through the TSH receptor activation), is also shown. I−, Iodine; MI, Myo-inositol; TSH, thyroid stimulating hormone; T3, triiodothyronine; T4, thyroxine; TPO, thyroid peroxidase; Tg, thyroglobulin; H2O2, hydrogen peroxide; MIT, monoiodotyrosine; TH, thyroid hormone.
Conclusion
The use of myo-inositol has gained a positive opinion in the endocrinological field for the restoration of proper thyroid functioning. To date, endocrinologists and gynecologists recommend taking myo-inositol for several benefits in different pathologies, from thyroid diseases to polycystic ovary syndrome and gestational diabetes. Due to its action in regulating iodine organification and thyroid hormone biosynthesis, myo-inositol supplementation along with iodine may improve thyroid functionality and possibly lead to a faster recovery from ID. Under normal conditions, the thyroid gland preferentially synthesizes T4 whereas, in pathologies such as ID, T4 biosynthesis is closer to the lower cut-off limit of normality. When iodine intake is insufficient, the T3:T4 ratio increases along with the decrease of T4 production, which however remains within the reference range. These subtle fluctuations might describe the patient's iodine status, representing a new simple approach to diagnose ID. Under certain circumstances the supplementation of myo-inositol, along with iodine can be used to restore the normal balance of T3:T4. Clinical studies to investigate the combined effect of myo-inositol and iodine in counteracting ID are warranted. They might open new scenarios and pose novel challenges for clinical experts and researchers to prevent and treat ID.
Author Contributions
DB and BO wrote the first draft of the manuscript. VU wrote sections of the manuscript. All authors contributed to manuscript revision, read, and approved the submitted version.
Conflict of Interest Statement
VU and BO are employees at Lo.Li. Pharma, Rome, Italy.
The remaining author declares that the research was conducted in the absence of any commercial or financial relationships that could be construed as a potential conflict of interest.
References
1. Zimmermann MB, Andersson M. Update on iodine status worldwide. Curr Opin Endocrinol Diabetes Obes. (2012) 19:382–7. doi: 10.1097/MED.0b013e328357271a
2. International Council for Control of Iodine Deficiency Disorder. Assessment of Iodine Deficiency Disorders and Monitoring Their Elimination: A Guide for Programme Managers. Geneva: World Health Organization (2007).
3. Bath SC, Steer CD, Golding J, Emmett P, Rayman MP. Effect of inadequate iodine status in UK pregnant women on cognitive outcomes in their children: results from the Avon Longitudinal Study of Parents and Children (ALSPAC). Lancet. (2013) 382:331–7. doi: 10.1016/S0140-6736(13)60436-5
5. Oberlin O, Plantin-Carrenard E, Rigal O, Wilkinson C. Goitre and iodine deficiency in Afghanistan: a case-control study. Br J Nutr. (2006) 95:196–203. doi: 10.1079/BJN20051581
6. Gharib H, Papini E, Garber JR, Duick DS, Harrell RM, Hegedus L, et al. American Association Of Clinical Endocrinologists, American College Of Endocrinology, And Associazione Medici Endocrinologi Medical Guidelines For Clinical Practice For The Diagnosis And Management Of Thyroid Nodules−2016 Update. Endocr Pract. (2016) 22:622–39. doi: 10.4158/ep161208.gl
7. De Benoist B, McLean E, Andersson M, Rogers L. Iodine deficiency in 2007: global progress since 2003. Food Nutr Bull. (2008) 29:195–202. doi: 10.1177/156482650802900305
8. Andersson M, Karumbunathan V, Zimmermann MB. Global iodine status in 2011 and trends over the past decade−3. J Nutr. (2012) 142:744–50. doi: 10.3945/jn.111.149393
9. World Health Organization. Iodine Status Worldwide: WHO Global Database on Iodine Deficiency (2004).
10. Unicef. The State of the World's Children 2011: Adolescence-an Age of Opportunity. Unicef (2011).
11. Benmiloud M, Delange F, Pittman CS, Yaffe S, Thilly C, Voumard C, et al. Safe use of iodized oil to prevent iodine deficiency in pregnant women. Bull World Health Organ. (1996) 74:1–3.
12. World Health Organization Secretariat, Andersson M, De Benoist B, Delange F, Zupan J. Prevention and control of iodine deficiency in pregnant and lactating women and in children less than 2-years-old: conclusions and recommendations of the Technical Consultation. Public Health Nutr. (2007) 10:1606–11. doi: 10.1017/S1368980007361004
13. Iodine-Global-Network (2015). Available online at: http://www.ign.org/ (accessed June 2, 2015).
14. Società Italiana di Nutrizione Umana. LARN: Livelli di Assunzione di Riferimento di Nutrienti ed energia per la Popolazione Italiana, IV Revisione. Milan: Società Italiana di Nutrizione Umana (2014).
15. Zimmermann MB, Gizak M, Abbott K, Andersson M, Lazarus JH. Iodine deficiency in pregnant women in Europe. Lancet Diabetes Endocrinol. (2015) 3:672–4. doi: 10.1016/S2213-8587(15)00263-6
16. Weng W., Dong, M., Zhan, J., Yang, J., Zhang, B., and Zhao, X. (2017). A PRISMA-compliant systematic review and meta-analysis of the relationship between thyroid disease and different levels of iodine intake in mainland China. Medicine. 96:e7279. doi: 10.1097/MD.0000000000007279
17. Luongo C, Trivisano L, Alfano F, Salvatore D. Type 3 deiodinase and consumptive hypothyroidism: a common mechanism for a rare disease. Front Endocrinol. (2013) 4:115. doi: 10.3389/fendo.2013.00115
18. Morton ME, Chaikoff IL, Rosenfeld S. Inhibiting effect of inorganic iodide on the formation in vitro of thyroxine and diiodotyrosine by surviving thyroid tissue. J Biol Chem. (1944) 154:381–7.
19. Wolff J, Chaikoff IL. Plasma inorganic iodide as a homeostatic regulator of thyroid function. J Biol Chem. (1948) 174:555–64.
20. Abuid J, Larsen PR. Triiodothyronine and thyroxine in hyperthyroidism. Comparison of the acute changes during therapy with antithyroid agents. J Clin Invest. (1974) 54:201–8. doi: 10.1172/jci107744
21. Bizhanova A, Kopp P. Minireview: the sodium-iodide symporter NIS and pendrin in iodide homeostasis of the thyroid. Endocrinology. (2009) 150:1084–90. doi: 10.1210/en.2008-1437
22. Arntzenius AB, Smit LJ, Schipper J, van der Heide D, Meinders AE. Inverse relation between iodine intake and thyroid blood flow: color Doppler flow imaging in euthyroid humans. J Clin Endocrinol Metab. (1991) 73:1051–5. doi: 10.1210/jcem-73-5-1051
23. Patel YC, Pharoah PO, Hornabrook RW, Hetzel BS. Serum triiodothyronine, thyroxine and thyroid-stimulating hormone in endemic goiter: a comparison of goitrous and nongoitrous subjects in New Guinea. J Clin Endocrinol Metab. (1973) 37:783–9. doi: 10.1210/jcem-37-5-783
24. Vagenakis AG, Koutras DA, Burger A, Malamos B, Ingbar SH, Braverman LE. STudies of serum triiodothyronine, thyroxine and thyrotropin concentrations in endemic goiter in Greece. J Clin Endocrinol Metab. (1973) 37:485–8. doi: 10.1210/jcem-37-3-485.
25. Chung HR. Iodine and thyroid function. Ann Pediatr Endocrinol Metab. (2014) 19:8–12. doi: 10.6065/apem.2014.19.1.8
26. Jahreis G, Hausmann W, Kiessling G, Franke K, Leiterer M. Bioavailability of iodine from normal diets rich in dairy products-results of balance studies in women. Exp Clin Endocrinol Diabetes. (2001) 109:163–7. doi: 10.1055/s-2001-14840
27. Hampel D, Dror DK, Allen LH. Micronutrients in human milk: analytical methods. Adv Nutr. (2018) 9(Suppl. 1): 313S−31S. doi: 10.1093/advances/nmy017
28. De Groot LJ, Jameson JL. Endocrinology Adult and Pediatric: The Thyroid Gland E-Book. Phyladelphia: Elsevier Health Sciences (2013).
29. Markou K, Georgopoulos N, Kyriazopoulou V, Vagenakis AG. Iodine-Induced hypothyroidism. Thyroid. (2001) 11:501–10. doi: 10.1089/105072501300176462
30. Bianco AC, Salvatore D, Gereben B, Berry MJ, Larsen PR. Biochemistry, cellular and molecular biology, and physiological roles of the iodothyronine selenodeiodinases. Endocr Rev. (2002) 23:38–89. doi: 10.1210/edrv.23.1.0455
31. Galton VA, de Waard E, Parlow AF, St Germain DL, Hernandez A. Life without the iodothyronine deiodinases. Endocrinology. (2014) 155:4081–7. doi: 10.1210/en.2014-1184
32. Ohye H, Sugawara M. Dual oxidase, hydrogen peroxide and thyroid diseases. Exp Biol Med. (2010) 235:424–33. doi: 10.1258/ebm.2009.009241
33. Chen G, Pekary AE, Sugawara M, Hershman JM. Effect of exogenous hydrogen peroxide on iodide transport and iodine organification in FRTL-5 rat thyroid cells. Acta Endocrinol. (1993) 129:89–96.
34. Dunn JT, Dunn AD. Thyroglobulin: chemistry, biosynthesis, and proteolysis. In: Braverman LE, Utiger RD, editors. The Thyroid: A Fundamental and Clinical Text. 8th Edn. Philadelphia, PA: Lippincott Williams and Wilkinsp. 91. (2000).
35. Ahn CS, Rosenberg IN. Oxidation of 14C-Formate in Thyroid Slices: Effects of TSH, Dibutyric Cyclic 30, 50-AMP (dbc-AMP) and Prostaglandin E1 (PGE1). Vienna: Verlag Der Wiener Medizinischen Akademie(1971). p. 825–37.
36. Clements JRS, Darnell B. Myo-inositol content of common foods: development of a high-myo-inositol diet. Am J Clin Nutr. (1980) 33:1954–67. doi: 10.1093/ajcn/33.9.1954
37. Berridge MJ, Irvine RF. Inositol phosphates and cell signalling. Nature. (1989) 341:197–205. doi: 10.1038/341197a0
39. Bizzarri M, Fuso A, Dinicola S, Cucina A, Bevilacqua A. Pharmacodynamics and pharmacokinetics of inositol(s) in health and disease. Expert Opin Drug Metab Toxicol. (2016) 12:1181–96. doi: 10.1080/17425255.2016.1206887
40. Nordio M, Pajalich R. Combined treatment with Myo-inositol and selenium ensures euthyroidism in subclinical hypothyroidism patients with autoimmune thyroiditis. J Thyroid Res. (2013) 2013:424163. doi: 10.1155/2013/424163
41. Benvenga S, Antonelli A. Inositol(s) in thyroid function, growth and autoimmunity. Rev Endocr Metab Disord. (2016) 17:471–84. doi: 10.1007/s11154-016-9370-3
42. Ferrari SM, Fallahi P, Di Bari F, Vita R, Benvenga S, Antonelli A. Myo-inositol and selenium reduce the risk of developing overt hypothyroidism in patients with autoimmune thyroiditis. Eur Rev Med Pharmacol Sci. (2017) 21:36–42.
43. Nordio M, Basciani S. Treatment with myo-inositol and selenium ensures euthyroidism in patients with autoimmune thyroiditis. Int J Endocrinol. (2017) 2017:2549491. doi: 10.1155/2017/2549491
44. Nordio M, Basciani S. Myo-inositol plus selenium supplementation restores euthyroid state in Hashimoto's patients with subclinical hypothyroidism. Eur Rev Med Pharmacol Sci. (2017) 21(2Suppl.):51–9.
45. Briguglia G. Time-dependent efficacy of myo-inositol plus selenium in subclinical hypothyroidism. Int J Med Device Adjuv Treat. (2018) 1:e108.
46. Ferrari SM, Elia G, Ragusa F, Ruffilli I, Paparo SR, Caruso C, et al. Myo-inositol and selenium in subclinical hypothyroidism. Int J Med Device Adjuv Treat. (2018) 1:e166.
47. Benvenga S, Vicchio T, Di Bari F, Vita R, Fallahi P, Ferrari SM, et al. Favorable effects of myo-inositol, selenomethionine or their combination on the hydrogen peroxide-induced oxidative stress of peripheral mononuclear cells from patients with Hashimoto's thyroiditis: preliminary in vitro studies. Eur Rev Med Pharmacol Sci. (2017) 21:89–101.
Keywords: iodine deficiency, iodine, myo-inositol, thyroid, goiter, organification
Citation: Barbaro D, Orrù B and Unfer V (2019) Iodine and Myo-Inositol: A Novel Promising Combination for Iodine Deficiency. Front. Endocrinol. 10:457. doi: 10.3389/fendo.2019.00457
Received: 19 April 2019; Accepted: 24 June 2019;
Published: 16 July 2019.
Edited by:
Salman Razvi, Newcastle University, United KingdomReviewed by:
Angela Leung, University of California, Los Angeles, United StatesMihaly Mezei, Icahn School of Medicine at Mount Sinai, United States
Copyright © 2019 Barbaro, Orrù and Unfer. This is an open-access article distributed under the terms of the Creative Commons Attribution License (CC BY). The use, distribution or reproduction in other forums is permitted, provided the original author(s) and the copyright owner(s) are credited and that the original publication in this journal is cited, in accordance with accepted academic practice. No use, distribution or reproduction is permitted which does not comply with these terms.
*Correspondence: Daniele Barbaro, danielebarbaro1970@libero.it