- 1Division of Geriatric Medicine, Department of Medicine, University of Verona, Verona, Italy
- 2Division of Geriatric Medicine, Department of Surgery, Dentistry, Pediatric and Gynecology, University of Verona, Verona, Italy
Across aging, adipose tissue (AT) changes its quantity and distribution: AT becomes dysfunctional with an increase in production of inflammatory peptides, a decline of those with anti-inflammatory activity and infiltration of macrophages. Adipose organ dysfunction may lead to age-related metabolic alterations. Aging is characterized by an increase in adiposity and a decline in brown adipose tissue (BAT) depots and activity, and UCP1 expression. There are many possible links to age-associated involution of BAT, including the loss of mitochondrial function, impairment of the sympathetic nervous system, age-induced alteration of brown adipogenic stem/progenitor cell function and changes in endocrine signals. Aging is also associated with a reduction in beige adipocyte formation. Beige adipocytes are known to differentiate from a sub-population of progenitors resident in white adipose tissue (WAT); a defective ability of progenitor cells to proliferate and differentiate has been hypothesized with aging. The loss of beige adipocytes with age may be caused by changes in trophic factors in the adipose tissue microenvironment, which regulate progenitor cell proliferation and differentiation. This review focuses on possible mechanisms involved in the reduction of BAT and beige activity with aging, along with possible targets for age-related metabolic disease therapy.
Introduction
Aging is considered a common and a well-established risk factor for several chronic diseases, as well as for decline in physical function and frailty (1–3). Moreover, aging is associated with increasing prevalence in obesity, dyslipidaemia, type 2 diabetes, glucose intolerance and other comorbidities.
In recent years, the adipose organ has assumed considerable importance in aging and age-related metabolic dysfunction. Important and profound changes in the adipose organ occur with aging in terms of adipose tissue distribution and composition, and it has been suggested that progressive dysfunction of AT may represent an important hallmark of the aging process. AT dysfunction represents a process responsible for the metabolic alterations, the multi-organ damage and the systemic pro-inflammatory state (“inflammaging”) typical of aging itself (4). Data in the literature support the idea that adipose tissues are organized in a large adipose organ with discrete anatomy, vasculature and innervation, specific cytology and high plasticity (5). AT is distributed in several depots, localized into two main compartments: subcutaneous (SAT) and visceral (VAT) adipose tissue with different compositions and functions. The main cells of AT are represented by adipocytes, defined as white and brown adipocytes in relation to their different morphology, which reflects their different functions (5) (Table 1). Both types of cells are present within multiple sites of adipose organ in discrete depots and are named white and brown adipocytes.
At the morphological level, the main characteristic of white adipocytes is their single large intracellular lipid droplet (LD), while the brown adipocytes are characterized by the presence of multiple small cytoplasmic LDs (Table 1). White adipocytes have the function of storing excess lipids in the form of triglycerides (TG) and releasing free fatty acids (FFA) in periods of body energy demand; white adipocytes also synthesize and release adipokines which regulate metabolic homeostasis (Table 1). The main function of brown adipocytes is the dissipation of energy through uncoupled respiration so as to produce heat; this mechanism is mediated by a protein called the uncoupling protein-1 (UCP-1), present in the inner membrane of mitochondria (6) (Table 1).
A third type of adipocyte with an intermediate morphology between that of white and brown adipocytes, also referred to as beige, “brite” (brown-like-in-white) or “inducible brown” adipocytes, was firstly described in mouse WAT and then found in various human WAT depots (7, 8). Despite similarities to brown adipocytes, beige adipocytes can undergo a thermogenic or storage phenotype depending on environmental conditions (Table 1).
Across aging, AT undergoes changes in quantity and distribution, with an increase in total AT and VAT up to 65 years, as well as of ectopic fat deposition, and a decrease in SAT (9–11). Across aging, BAT declines, even if BAT activity may be identified in some rodents models and, under certain conditions, in human beings. However, the relevance and potential role of BAT decline with aging has still not been fully explored and determined.
Brown Adipose Tissue: Anatomical Decline With Aging
The main deposits of brown adipose tissue in the mouse are located around the spinal cord in the paravertebral area and in the mediastinum (12), especially in the para-aortic area and around the heart, at the apex. Infradiaphragmatic deposits have also been described, in particular in the perirenal area, which occur in smaller quantities than the supra-diaphragmatic deposits (13).
Most of available information on BAT has been obtained from rodents because a carcass evaluation can be performed. In mice, white, brown, and mixed areas are present in discrete depots; some subcutaneous and visceral depots are clearly partitioned into WAT and BAT (14, 15). Under physiological conditions, the exposure of mice to cold increases the amount of BAT depots. After cold exposure, UCP-1-dependent pathways are also activated in subcutaneous WAT (sWAT), and in brite adipocytes through the activation of the sympathetic nervous system (16–18).
In adult humans there are functionally active areas of BAT, more frequently in women than in men, in particular in the cervico-supraclavicular region; however, the study of human BAT is difficult (19). In humans, the 18F-fluorodeoxyglucose (18F-FDG) positron emission tomography (PET-CT) computed tomography is the most common method for the measurement of metabolically active BAT, by the identification of AT regions that have a high assimilation of 18F-FDG on the PET scan. It is important to point out that the BAT detected using 18F-FDG PET-CT has been demonstrated to correspond histologically to BAT (20). [18F] FDG PET reveals glucose metabolism in tissues; in detail, active BAT of mice and humans preferentially combusts fatty acids derived from plasma triglycerides after lipolysis, which is fueled by glucose. Indeed, [18F] FDG PET does not detect directly metabolically active BAT, but it is still a reliable indicator of activated BAT (21, 22).
In humans, BAT changes during the various stages of life (Figure 1). BAT begins to form during gestation, and it has a critical role in thermoregulation in the first phases of human life because newborns do not possess the ability to shiver yet. In infants, the large bilateral supraclavicular depot represents the most metabolically active form of BAT, which can be rapidly activated to heat production (Figure 1). During childhood, adolescent BAT is found mainly in the supraclavicular region but, although active BAT is present in every child, metabolically active BAT is detected only in about half of adolescents after cold exposure (23–25).
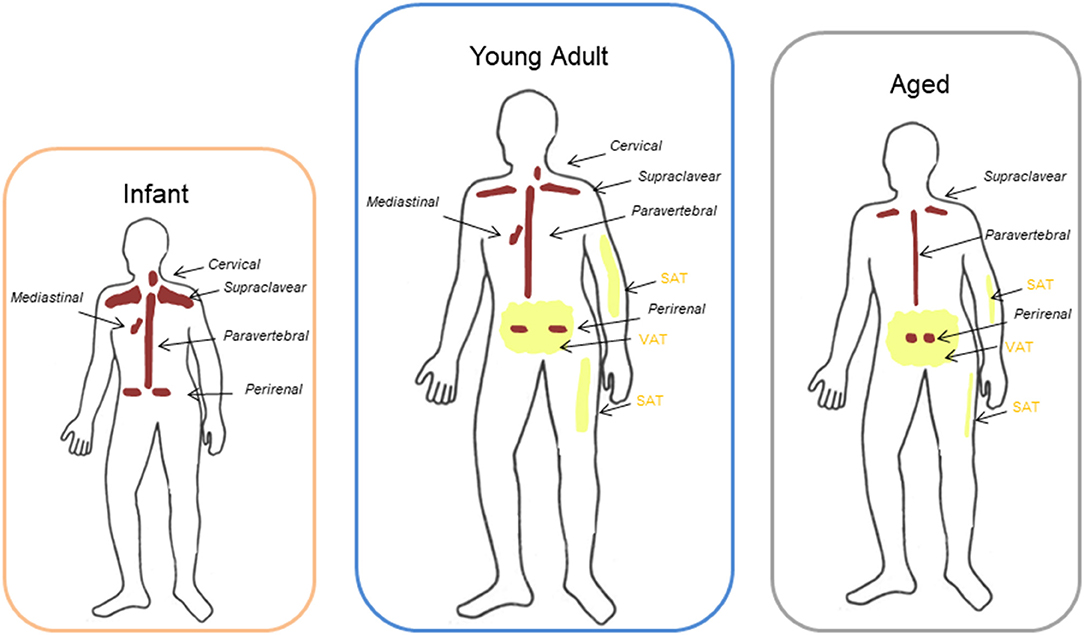
Figure 1. Different location of BAT and WAT at different ages. Brown adipose tissue (BAT) in infants and young adults had been described to be localized mainly in the cervical-supraclavicular region as well as in periaortic areas inside the thorax and the abdomen, and in particular in the perirenal fat. With aging the amount of detectable BAT decreases progressively and it remains represented mainly in the supraclavicular and perirenal sites. Peripheral depots (interscapular) are the first to loose BAT with increasing age, whereas deeper BAT depots, such as the perivascular or perirenal depots, decline in later stages of life. Aging is also characterized by a redistribution of white adipose tissue (WAT) with a progressive loss of subcutaneous adipose tissue (SAT) from limbs and an accumulation in trunk and abdomen of visceral adipose tissue (VAT) compared to adults.
Moreover, other evidence suggests that there is an increase in BAT activity during adolescence, especially during sexual maturation and musculoskeletal development. Studies with FDG-PET/TC scans indicate that there is a synchronized growth of BAT and skeletal muscle during puberty and the development of these tissues is related (24, 25).
BAT function and mass decline with aging. Anatomical distribution of BAT is similar between adolescents and adults: most of the depots are located in the cervical-supraclavicular region and other depots are in axillary, mediastinal, paravertebral, epicardial and abdominal regions (26, 27) (Figure 1). Peripheral depots, such as interscapular one, are the first to lose BAT with increasing age, whereas deeper BAT depots, in particular perivascular or perikidney ones, decline in later stages of life (28–30) (Figure 1).
Non-stimulated BAT can be identified in people under the age of 50 years at a rate of three times more than in individuals older than 64 years old (26–31). Through the use of FDG-PET/TC to visualize BAT in living subjects, it has been shown that cold-stimulated BAT activity decreases with age (26, 27, 29). Loss of BAT may plateau around the sixth decade of life and then decrease in later years, and cold-stimulated BAT activity is rarely detected in individuals over the age of sixty; this could explain why there is a decrease in the ability of the elderly to tolerate cold temperature and to control body temperature (29). However, FDG PET/TC studies measure only activated BAT, which may or may not reflect changes in mass (29). The decline in BAT activity with age in humans is consistently supported by findings from rodents: in fact, the interscapular BAT depot in rats becomes infiltrated by white adipocytes with age, with an important decline in UCP1 activity and function (30). A significant loss of UCP1 with age is also observed in rats subcutaneous WAT, confirming the findings of human autopsy studies (31). It therefore seems that many, if not all forms of BAT, may undergo a gradual transition toward WAT with increasing age both in human as in rodent studies (30, 31).
In summary, aging is one of the most relevant determinants of BAT activity and it is associated with a ubiquitous decline of BAT activity throughout life (32, 33).
Mechanisms Of Brown Adipose Tissue Decline With Aging
Several mechanisms have been shown to be related to BAT decline with aging, including loss of mitochondrial function, impairment in the sympathetic nervous system and age-induced alterations in endocrine signals and inflammation (Figure 2).
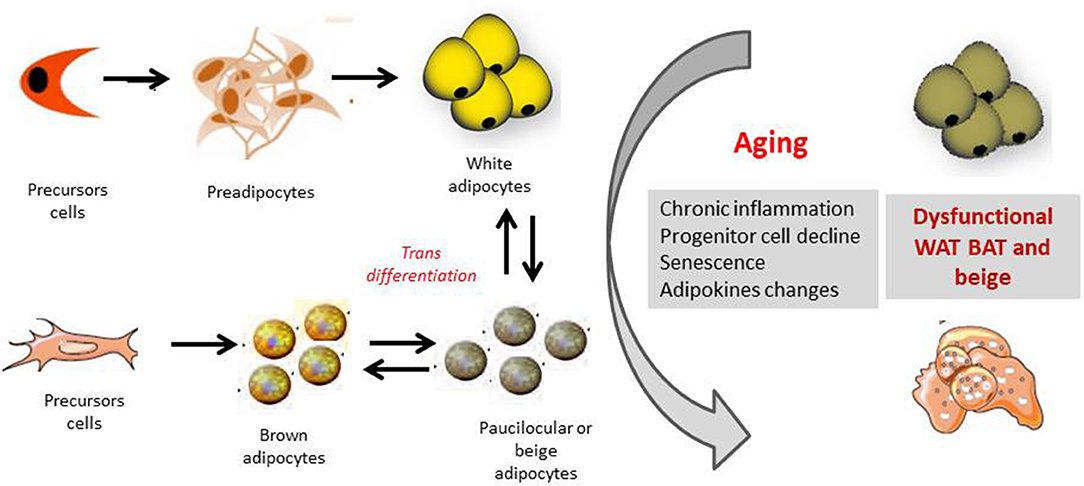
Figure 2. Orgin of white, brown and beige adipocytes and effect of aging. The appearance of beige cells among white adipose tissue (WAT) depots is referred to as “browning.” It has been hypothesized that these cells origin from the de novo differentiation of a distinct sub-population of WAT resident progenitors, which express the markers CD 137 and TMEM26 on their surface. Moreover beige-type cells may generate also from white-to-brown adipocyte transdifferentiation, from MYF5 positive cells. Several mechanisms as chronic inflammation, progenitor cell decline, senescence of the different adipose cell compartments as well as changes in adipokines productions, may together contribute to a dysfunctional adipose organ with aging.
Impairments in Mitochondrial Activity
During aging, there is a significant decline in UCP1 activity, a protein uniquely expressed in the inner mitochondrial membrane of brown adipocytes. Mitochondrial dysfunction has been recognized to have a relevant role in the pathogenesis of several age-related disorders, such as type 2 diabetes, obesity, heart failure, neurodegenerative diseases and tumorigenesis (34, 35).
In particular, mitochondrial dysfunction with aging is characterized by an increase in mitochondrial DNA mutations and a reduction in biogenesis and oxidative phosphorylation. For this reason, aging may be associated with an impairment in brown adipogenic stem/progenitor cell function and consequently with a reduction in the regenerative potential of BAT with storage of dysfunctional brown adipocytes (34, 36).
Decrease in the Sympathetic Nervous Stimulation and Sensitivity in BAT With Age
The sympathetic nervous system (SNS) plays a key role in regulation of BAT recruitment. Moreover, exposure to cold is a potent BAT stimulator through the SNS. Cathecolamines activate B3-adrenergic receptors located on the surface of brown adipocytes to promote UCP1 gene expression and activity related to thermogenesis and lipolysis (37, 38).
The sympathetic nervous system of BAT can be visualized by I-meta-iodobenzylguanidina SPECT (39). 123I-metaiodobenzylguanidine (123IMIBG), a radiolabeled norepinephrine analog, is commonly used for scintigraphic assessment of neuroendocrine tumors and cardiac sympathetic activity (39). 123I-MIBG scintigraphy, in particular, has already been used specifically to localize BAT in rats. Interestingly, a recent study in lean adult humans after cold exposure, measured BAT through the combination of the two imaging methods: the 123IMIBG SPECT/CT, as a measure of sympathetic stimulation and activation, and the 18F-FDG PET/CT, as an indicator of BAT metabolic activity (37–39). In older lean subjects, both sympathetic drive and BAT activity were lower compared to younger lean and obese men (37).
Therefore, it is possible that a lower absolute SNS signal and a possible decline in sensitivity of BAT for the SNS stimulation may result in a decreased ability to activate and recruit BAT in older men and may also explain why older humans have an inability to appropriately regulate their temperature when exposed to cold (37, 38).
Age-Related Hormonal Changes and BAT
BAT mass and/or function may decline during adulthood, as a consequence of changes particularly in the somatotropic and gonadotropic axes.
Human fetal BAT shows a high expression of estrogen receptors, suggesting that tissue-levels of estrogens may regulate BAT activity (40, 41). Recent evidence suggests that estrogens and androgens are positively related to BAT activity and function while glucocorticoids have negative effects. Glucocorticoids, such as dexamethasone, reduce the cathecholamine-induced expression of UCP1 (42). With aging, sex hormone levels decline, while glucocorticoid levels remain relatively stable; for this reason, this decrement in levels of gonadotropic hormones in late adulthood and relative increase in glucocorticoid activity may contribute to the loss of BAT activity with aging (41, 42).
Thyroid hormones are also known regulators of thermogenesis: UCP1 levels are related to triiodothyronine levels. Extensive evidence shows that T3 promotes UCP1 synthesis at transcriptional levels in BAT, as well as UCP1 activity by modulation of cAMP production. Aging is associated with a decrease in serum T3 and a reduced conversion of active T3 caused by an age-dependent loss of DIO2, as is demonstrated in murine WAT (43). Recent evidences demonstrated that UCP1 expression in WAT also correlates with circulating thyroxine serum levels (43, 44), suggesting that thyroid hormones may contribute to the browning of white adipose tissue and increasing thermogenesis (45).
A few recent papers suggest ghrelin signaling as an important thermogenic regulator in aging. The ablation of the ghrelin receptor, the growth hormone secretagogue receptor (GHS-R), decreases the risk of age-associated obesity and insulin resistance. Ghrelin and obestatin are derived from the same preproghrelin gene; however, in brown adipose tissue, ghrelin reduces the expression of UCP-1 but obestatin increases it. During aging, plasma ghrelin and GHS-R expression in BAT are increased, but plasma obestatin is stable; this may lead to an imbalance in thermogenic regulation, which may in turn exacerbate age-related thermogenic impairment. Moreover, GHS-R ablation activates thermogenic signaling, enhances insulin activation, mitochondrial biogenesis, and improves BAT mitochondrial function (46, 47).
In recent papers, it has been suggested that fibroblast growth factor 21 (FGF21), secreted by the liver and adipose tissue, may also play a role in the browning process of WAT depots. In fact, mice with a FGF21 deficiency display an impaired ability to adapt to chronic cold exposure, with reduced browning of WAT. In particular, it has been demonstrated that adipose-derived FGF21 increases the expression of UCP1 and other thermogenic genes in fat tissues in an autocrine/paracrine manner (48, 49). However, aging is characterized by a progressive increase in FGF21 levels from 5 to 80 years, independently of body composition (49). This discrepancy between the increase in FGF21 levels and decrease browning of WAT with aging could be at least in part reconciled, hypothesizing an FGF21-resistant state with age itself. In fact, evidences from studies conducted on rodents supported the existence of an age-related FGF21-resistant state (50). Similarly, in some metabolic states such as obesity and diabetes, FGF21 levels are elevated and an FGF21-resistant condition has been suggested to also accompany these diseases (51).
Inflammaging
The production of pro-inflammatory mediators and the infiltration of immune cells in AT, a process of chronic inflammation typical of obesity and metabolic conditions, also increases with age, so that has been referred to as “inflammaging” (52–54).
Several evidences have suggested that compared to WAT, brown and beige adipose tissue are less likely to undergo local inflammation due to immune cells infiltration, but present an increased production of inflammatory cytokines as TNF-alpha and MCP-1, in presence of dysregulated metabolism linked to obesity (53).
It has been hypothesized that inflammation with the production of these cytokines may indirectly impair the thermogenic activity in BAT because of altered insulin sensitivity and reduced glucose uptake (53).
Several pro-inflammatory cytokines are suggested to be involved in these mechanisms that globally reduce UCP-1 gene expression and browning phenomena (52, 54). In particular, TNF-a, one of the principal cytokines that increases during these inflammatory processes, via Toll-like receptor (TLR) activation induces apoptosis of brown adipocytes and inhibits the expression of UCP1 and b3-adrenergic receptor on adipocytes, ultimately decreasing thermogenesis in BAT (52). Other inflammatory mediators, such as pattern recognition receptors (PPR) and nucleotide-oligomerization domain containing proteins (NODs), have been suggested to have a critical role in modulating BAT activity during inflammation via activation of NF-kB and MAPK signaling pathways (53, 54).
Beige Adipocytes And Aging
Beige/brite adipocytes are likely to originate from both a white-to-brown transdifferentiation mechanism and de novo differentiation from specific precursor cells (Figure 2).
The phenomenon by which the appearance of beige cells among WAT depots is observed is referred to as “browning” (55); this mechanism is mainly based on adrenergic signals and cold exposure. The beige-type cells in white fat depots are genetically different from those in classic interscapular and perirenal BAT, which are derived from myogenic factor 5 (MYF-5) positive precursors (56). Moreover, recent evidence suggests that both BAT and WAT adipocytes derive from the vascular endothelial cells of adipose tissue, supporting a role for transdifferentiation (57). This induction of beige adipocytes depends on several mechanisms, including, in addition to the aforementioned environmental temperature, genetics factors, diet, developmental periods and anatomic location of the adipose tissues (58).
With regard to the de novo differentiation, adipose stem and/or progenitor cells reside within WAT, which can proliferate and differentiate into either white or beige/brite adipocytes. In particular, a distinct sub-population of WAT resident progenitors, which express the markers CD 137 and TMEM26 on their surface, show a greater ability to differentiate into beige cells (59).
As a consequence of this, the age-related dysfunctional regeneration and reduction of classical brown and beige tissues could be due to a defective ability to proliferate and differentiate from inducible WAT or due to a loss of CD137/TMEM 26+ progenitors.
Moreover, different molecular mechanisms have been described that underlie the loss of beige adipose tissue during aging. Interestingly, SIRT1, an important target in AT biology (60), drives the browning of adipose tissue by promoting the interaction between PPARgamma and PRDM16, a potent inducer of beige adipose-specific genes (61). A recent study demonstrated another role of SIRT1, via the regulation of the senescence pathway p53/p21 (62). By reducing the expression of p53, a transcription factor of p21, SIRT1, enhances beige adipocyte differentiation capability of elderly adipose tissue-derived mesenchymal stem cells (AT-MSCs). During aging there is a reduction in SIRT1 levels, but how its expression is regulated into these stem cells is still unclear. Recently, it has been demonstrated the microRNA 34a (miRNA 34a) is a direct regulator of SIRT1 (63). Interestingly, miRNA-34a suppresses the process of browning under conditions of obesity, in part via its regulation of SIRT1 and FGF-21. This evidence supports its role of being a candidate molecule for improving the differentiation ability of elderly AT-MSCs as a treatment of aging obesity (63).
Brown And Beige Aging: Potential Intervention Targets
White and brown AT has been suggested as a target for the prevention of type 2 diabetes, lipid disorders, as well as for delaying aging. Counteracting the age-associated loss of brown and beige adipose tissue could be an interesting and innovative therapeutic approach. Different types of strategies and molecular targets have been suggested for implementing possible interventions to slow age-related changes in brown and beige adipose tissue.
Physical Exercise
Well known and widely studied are the effects of physical exercise on adipose tissue physiology, since it is well known that regular physical activity improves glucose tolerance and reduces white adipose tissue mass. Several studies have shown that physical exercise is associated with changes in both subcutaneous and visceral fat, a reduction of adipocyte size and lipid content, enhanced expression of metabolic pathway genes, modified secretion of adipokines and increased mitochondrial activity (64). However, less well-known and studied are the effects of physical exercise in AT of elderly humans, and in particular on BAT.
Studies evaluating the effect of exercise on BAT in old rodents and humans have been published with conflicting results. In a recent study conducted in old rats, both strength and aerobic training determined an increase in BAT, in mitochondrial activity, thus reducing total body fat (65). These results were also confirmed in other rodent models, where the physical exercise-associated browning of subcutaneous WAT has been demonstrated (66).
However, these findings were not confirmed in elderly subjects as a decrease in mitochondrial activity and in glucose uptake in BAT after training has been shown (67).
More examination is mandatory to completely understand the impact of the complex relationships between different types of exercise and exercise-induced adaptations in WAT and BAT, as it is a field of study of considerable interest.
Nutritional Strategies
Since intermittent fasting was proved to optimize energy metabolism (68), in a recent study rodents were kept on an every-other-day fasting regimen: this approach favored the activation of beige fat thermogenesis and improved obesity-related metabolic diseases, probably via a microbiota-beige fat axis (69). Interestingly, in a human model, Orava and colleagues observed that insulin stimulated a 5-fold increase in FDG uptake in BAT, suggesting that BAT may contribute to postprandial energy metabolism in humans (70).
Recent researches have demonstrated that some food ingredients may be involved in promoting energy expenditure and fat oxidation in BAT. In fact, it has been shown that capsaicin and its analogs in hot peppers, as well as caffeine and catechins in green tea, could be related to an increased energy expenditure (71, 72). In particular, capsaicin may induce a browning program in WAT, stimulating UCP-1 and promoting SIRT1 expression and activation through TRPV1 (transient receptor potential channels) channels (73). Josse et al. have reported that an ingestion of meals supplemented with capsinoids may increase energy expenditure and lipid oxidation through an activity on Beta3-adrenergic receptors (74, 75).
It has also been shown that PUFAs (Long-chain omega-3 polyunsaturated fatty acid) and, in particular, eicosapentaenoic acid (EPA), known for their anti-inflammatory and cardio protective effects, reduce high-caloric diet related obesity and insulin resistance in mice. The mechanism underlying BAT activation seems to depend on FGF21 expression, also without cold exposure, suggesting that EPA supplementation alone may mimic the cold induced BAT activation (76).
All of this evidence may demonstrate future area of research for a non-pharmacological strategy to treat obesity and age-related metabolic disease, also in elderly individuals.
Cold Exposure
It is now widely known from studies on mice and humans that exposure to cold increases the activity of brown adipose tissue (27). Saito et al. found an unexpected high presence of cold-activated BAT by performing FDG-PET/CT scans in healthy adults under warm and cold conditions, which suggests an important role of temperature in the regulation of BAT activity and body fat content. More recently, gene expression profiles and metabolic pathways activated in BAT exposed to cold have been investigated; in an interesting study conducted in mice, it was shown that cold exposure highly influenced BAT metabolic activity. Exposure to cold is characterized by lower levels of glycolysis and gluconeogenesis intermediates, higher levels of tricarboxylic acid cycle metabolites, free fatty acids and acyl-carnitine metabolites, suggesting that glycolysis and β-oxidation of fatty acids in BAT are biological pathways that contribute to increased thermogenesis by cold exposure (77, 78).
Recent studies have evidenced that mitochondrial reactive oxygen species (ROS) play an important role in modulating thermogenesis and UCP1 activity. In fact, cold exposure is characterized by an increased mitochondrial superoxide and oxidation of lipids and proteins in BAT. Furthermore, acute cold exposure leads to enhanced oxidation and a decrease of reduced glutathione in BAT; this process is associated by increased protein thiol oxidation, which has been suggested as a vital signaling mechanism required for UCP1-induced thermogenic metabolism (79, 80).
Pharmacological Strategies
The PPARgamma agonist rosiglitazone exerts its thermogenic effects on adipocytes by increasing PRDM16 (regulator PR domain containing 16) protein half-life, a zinc-finger transcriptional factor that plays a key role in the differentiation of adipocytes (61). In fact, PRDM16 controls the bidirectional switch between brown adipocytes and myoblasts. PRDM16 determines the brown fat-like gene expression and thermogenesis in both BAT and WAT. Moreover, the expression of this transcriptional regulator is strongly correlated with beige cell-selective genes, in the so-called browning process (61). From a therapeutical point of view, some reports have supported extensive inhibition of adipokines production, including resistin, 1-acidglycoprotein, and haptoglobin, by treatment of white adipocytes with thiazolidinedione (TZD) and non-TZD synthetic PPARgamma ligands (81).
Moreover, Finlin et al. have suggested that mirabegron, a beta 3-agonist, induces the expression of UCP1 and beige adipocyte markers to a higher degree than 10 days of repeated cold exposure. This phenomenon may be exploited to increase beige adipose tissue in older, insulin-resistant, obese individuals (82).
Vitamin A metabolites or retinoids are other hormones required for BAT activation. Retinoic acid strongly induces UCP1 expression in adipocytes, indicating that body thermogenic capacity may also be related to the vitamin A status. Retinoic acid may also determine adipocyte differentiation and survival, with high doses inhibiting and low doses promoting adipogenesis of adipose cells precursors in vitro (83). The administration of retinoids in high-fat diet mice is associated with an increase in adipose UCP1 expression and a reduction in body weight. However, its role in humans is still controversial: UCP1 expression is differently affected by all-trans retinoic acid (ATRA) in mouse and human adipocytes (84).
Bone morphogenetic proteins (BMPs) are a family of secreted molecules that contribute to the differentiation of mesenchymal stem cells and drive the formation and thermogenic activation of BAT (85). BMP9 treatment has been shown to determine the browning of subcutaneous WAT, to improve glucose tolerance and reduce weight gain in in vivo experiments (86). A role for BMP8B in the regulation of thermogenesis has also been described; this protein regulates nutritional and thermogenic factors in mature BAT, improving the response to noradrenaline through enhanced p38MAPK/CREB signaling and increased lipase activity. Bmp8b−/− mice show impaired thermogenesis and decreased metabolic rate, determining weight gain despite hypophagia. BMP8B is also expressed in the hypothalamus, and Bmp8b−/− mice display altered neuropeptide levels and reduced phosphorylation of AMP-activated protein kinase (AMPK), indicating an anorexigenic state (87).
Fibrates exert their lipid-lowering activity via PPARalpha. The new compound, GW9578, was demonstrated to enhance insulin sensitivity and to decrease adiposity in vivo (88). Moreover, the PPARalpha agonist GW9578 stimulates expression of the thermogenic gene program in beige adipocytes and rescues the beige-to-white fat transition phenotype induced by loss of Lsd1 (89).
However, most of the research about transcriptional factors involved in the regulation of BAT development has been carried out in mice and the expression of these marker genes seems less consistent in the humans, with a lack of specific data for elderly subjects in particular.
Conclusions
Further human studies are needed to investigate the effectiveness of nutritional and pharmacological stimulation to maintain BAT and beige mass and sensitivity during aging.
However, any therapeutical targeting of BAT activity and/or mass will first require a clear understanding of the mechanisms involved in potentiating BAT activity.
Author Contributions
All authors listed have made a substantial, direct and intellectual contribution to the work, and approved it for publication.
Conflict of Interest Statement
The authors declare that the research was conducted in the absence of any commercial or financial relationships that could be construed as a potential conflict of interest.
Abbreviations
BAT, brown adipose tissue; WAT, white adipose tissue; SAT, subcutaneous adipose tissue; VAT, visceral adipose tissue.
References
1. López-Otín C, Blasco MA, Partridge L, Serrano M, Kroemer G. The hallmarks of aging. Cell. (2013) 153:1194–217. doi: 10.1016/j.cell.2013.05.039
2. Barzilai N, Huffman DM, Muzumdar RH, Bartke A. The critical role of metabolic pathways in aging. Diabetes. (2012) 61:1315–22. doi: 10.2337/db11-1300
4. Rosen ED, Spiegelman BM. What we talk when we talk about fat. Cell. (2014) 156:20–44. doi: 10.1016/j.cell.2013.12.012
5. Cinti S. The adipose organ at a glance. Dis Models Mech. (2012) 5:588–94. doi: 10.1242/dmm.009662
6. Cinti S. UCP1 protein: the molecular hub of adipose organ plasticità. Biochimie. (2017) 134:71–6. doi: 10.1016/j.biochi.2016.09.008
7. Young P, Arch JR, Ashwell M. Brown adipose tissue in the parametrial fat pad of the mouse. FEBS Lett. (1984) 167:10–4. doi: 10.1016/0014-5793(84)80822-4
8. Cousin B, Cinti S, Morroni M, Raimbaul S, Ricquier D, Pénicaud L, et al. Occurence of brown adipocytes in rat white adipose tissue:molecular and morphological characterization. J Cell Sci. (1992) 103(Pt 4):931–42.
9. Palmer AK, Kirkland JL. Aging and adipose tissue:potential interventions for diabetes and regenerative medicine. Exp Gerontol. (2016) 86:97–105. doi: 10.1016/j.exger.2016.02.013
10. Sepe A, Tchkonia T, Thomou T, Zamboni M, Kirkland JL. Aging and regional differences in fat cell progenitors-a mini-review. Gerontology. (2011) 57:66–75. doi: 10.1159/000279755
11. Zamboni M, Rossi AP, Fantin F, Zamboni G, Chirumbolo S, Zoico E, et al. Adipose tissue, diet and aging. Mech Ageing Dev. (2014) 136–137:129–37. doi: 10.1016/j.mad.2013.11.008
12. Truong MT, Erasmus JJ, Munden RF, Marom EM, Sabloff BS, Gladish GW, et al. Focal FDG uptake in mediastinal brown fat mimicking malignancy:a potential pitfall resolved on PET/CT. Am J Roentgenol. (2004) 83:1127–32. doi: 10.2214/ajr.183.4.1831127
13. Bar-Shalom R, Gaitini D, Keidar Z, Israel O. Non-malignant FDG uptake in infradiaphragmatic adipose tissue:a new site of physiological tracer biodistribution characterised by PET/CT. Eur J Nucl Med Mol Imaging. (2004) 31:1105–13. doi: 10.1007/s00259-004-1506-0
14. Cinti S. Adipocyte differentiation and transdifferentiation:plasticity of the adipose organ. J Endocrinol Invest. (2002) 25:823–35. doi: 10.1007/BF03344046
15. Cinti S. Transdifferentiation properties of adipocytes in the adipose organ. Am J Physiol Endocrinol Metab. (2009) 297:E977–86. doi: 10.1152/ajpendo.00183.2009
16. Petrovic N, Walden TB, Shabalina IG, Timmons JA, Cannon B, Nedergaard J.Chronic peroxisome proliferator-activated receptor γ (PPAR-γ) activation of epididymally derived white adipocyte cultures reveals a population of thermogenically competent, UCP1-containing adipocytes molecularly distinct from classic brown adipocytes. J. Biol. Chem. (2010) 285:7153–64. doi: 10.1074/jbc.M109.053942
17. Xue Y, Petrovic N, Cao R, Larsson O, Lim S, Chen S, et al. Hypoxia-independent angiogenesis in adipose tissues during cold acclimation. Cell Metab. (2009) 9:99–109. doi: 10.1016/j.cmet.2008.11.009
18. Feldmann HM, Golozoubova V, Cannon B, Nedergaard J. UCP1 ablation induces obesity and abolishes diet-induced thermogenesis in mice exempt from thermal stress by living at thermoneutrality. Cell Metab. (2009) 9:203–9. doi: 10.1016/j.cmet.2008.12.014
19. Heaton JM. The distribution of brown adipose tissue in the human. J Anat. (1972) 112(Pt 1):35–9.
20. Cypess AM, Lehman S, Williams G, Tal I, Rodman D, Goldfine AB, et al. Identification and importance of brown adipose tissue in adult humans. New Eng J Med. (2009) 360:1509–17. doi: 10.1056/NEJMoa0810780
21. Bakker LE, Boon MR, van der Linden RA, Arias-Bouda LP, van Klinken JB, Smit F, et al. Brown adipose tissue volume in healthy lean south Asian adults compared with white Caucasians:a prospective, case-controlled observational study. Lancet Diabetes Endocrinol. (2014) 2:210–7. doi: 10.1016/S2213-8587(13)70156-6
22. Berbée JF, Boon MR, Khedoe PP, Bartelt A, Schlein C, Worthmann A, et al. Brown fat activation reduces hypercholesterolaemia and protects from atherosclerosis development. Nat Commun. (2015) 6:6356. doi: 10.1038/ncomms7356
23. Symonds ME. Brown adipose tissue growth and development. Scientifica (Cairo). (2013) 2013:305763. doi: 10.1155/2013/305763
24. Gilsanz V, Chung SA, Jackson H, Dorey FJ, Hu HH. Functional brown adipose tissue is related to muscle volume in children and adolescents. J Pediatr. (2011) 158:722–6. doi: 10.1016/j.jpeds.2010.11.020
25. Ponrartana S, Hu HH, Gilsanz V. On the relevance of brown adipose tissue in children. Ann NY Acad Sci. (2013) 1302:24–29. doi: 10.1111/nyas.12195
26. Lee P, Swarbrick MM, Ho KK. Brown adipose tissue in adult humans: a metabolic renaissance. Endocr Rev. (2013) 34:413–438. doi: 10.1210/er.2012-1081
27. Saito M, Okamatsu-Ogura Y, Matsushita M, Watanabe K, Yoneshiro T, Nio-Kobayashi J, et al. High incidence of metabolically active brown adipose tissue in healthy adult humans:effects of cold exposure and adiposity. Diabetes. (2009) 58:1526–31. doi: 10.2337/db09-0530
28. Pfannenberg C, Werner MK, Ripkens S, Stef I, Deckert A, Schmadl M, et al. Impact of age on the relationships of brown adipose tissue with sex and adiposity in humans. Diabetes. (2010) 59:1789–93. doi: 10.2337/db10-0004
29. Persichetti A, Sciuto R, Rea S, Basciani S, Lubrano C, Mariani S, et al. Prevalence, mass, and glucose-uptake activity of (1)(8)F-FDG-detected brown adipose tissue in humans living in a temperate zone of Italy. PLoS ONE. (2013) 8:e63391. doi: 10.1371/journal.pone.0063391
30. Rogers NH. Brown adipose tissue during puberty and with aging. Ann Med. (2014) 47:142–9. doi: 10.3109/07853890.2014.914807
31. Lecoultre V, Ravussin E. Brown adipose tissue and aging. Curr Opin Clin Nutr Metab Care. (2011)14:1–6. doi: 10.1097/MCO.0b013e328341221e
32. Ravussin E, Galgani JE. The implication of brown adipose tissue for humans. Annu Rev Nutr. (2011) 31:33–47. doi: 10.1146/annurev-nutr-072610-145209
33. Tam CS, Lecoultre V, Ravussin E. Brown adipose tissue:mechanisms and potential therapeutic targets. Circulation. (2012) 125:2782–91. doi: 10.1161/CIRCULATIONAHA.111.042929
34. Lenaz G. Role of mitochondria in oxidative stress and ageing. Biochim Biophys Acta Bioenerget. (1998) 1366:53–67. doi: 10.1016/S0005-2728(98)00120-0
35. Poher A-L, Altirriba J, Veyrat-Durebex C, Rohner-Jeanrenaud F. Brown adipose tissue activity as a target for the treatment of obesity/insulin resistance. Front Physiol. (2015) 6:4. doi: 10.3389/fphys.2015.00004
36. Cedikova M, Kripnerová M, Dvorakova J, Pitule P, Grundmanova M, Babuska V, et al. Mitochondria in white, brown, and beige adipocytes. Stem Cells Int. (2016) 2016:6067349. doi: 10.1155/2016/6067349
37. Bahler L, Verberne HJ, Admiraal WM, Stok WJ, Soeters MR, Hoekstra JB, et al. Differences in sympathetic nervous stimulation of brown adipose tissue between the young and old, and the lean and obese. J Nuclear Med. (2015) 57:372–7. doi: 10.2967/jnumed.115.165829
38. Bahler L, Molenaars RJ, Verberne HJ, Holleman F. Role of the autonomic nervous system in activation of human brown adipose tissue: a review of the literature. Diabetes Metab. (2015) 41:437–45. doi: 10.1016/j.diabet.2015.08.005
39. Admiraal WM, Holleman F, Bahler L, Soeters MR, Hoekstra JB, Verberne HJ. Combining 123I-metaiodobenzylguanidine SPECT/CT and 18FFDG PET/CT for the assessment of brown adipose tissue activity in humans during cold exposure. J Nucl Med. (2013) 54:208–12. doi: 10.2967/jnumed.112.111849
40. Valle A, Santandreu FM, Garcia-Palmer FJ, Roca P, Oliver J. The serum levels of 17beta-estradiol, progesterone and triiodothyronine correlate with brown adipose tissue thermogenic parameters during aging. Cell Physiol Biochem. (2008) 22:337–46. doi: 10.1159/000149812
41. Velickovic K, Cvoro A, Srdic B, Stokic E, Markelic M, Golic I, et al. Expression and subcellular localization of estrogen receptors alpha and beta in human fetal brown adipose tissue. J Clin Endocrinol Metab. (2014) 99:151–9. doi: 10.1210/jc.2013-2017
42. Soumano K, Desbiens S, Rabelo R, Bakopanos E, Camirand A, Silva JE. Glucocorticoids inhibit the transcriptional response of the uncoupling protein-1 gene to adrenergic stimulation in a brown adipose cell line. Mol Cell Endocrinol. (2000) 165:7–15. doi: 10.1016/S0303-7207(00)00276-8
43. Lee JY, Takahashi N, Yasubuchi M, Kim Y-I, Hashizaki H, Kim M-J, et al. Triiodothyronine induces UCP-1 expression and mitochondrial biogenesis in human adipocytes. Am J Physiol Cell Physiol. (2012) 302:C463–72. doi: 10.1152/ajpcell.00010.2011
44. Martínez-Sánchez N, Moreno-Navarrete JM, Contreras C, Rial-Pensado E, Fernø J, Nogueiras R, et al. Thyroid hormones induce browning of white fat. J Endocrinol. (2016) 232:335–62. doi: 10.1530/JOE-16-0425
45. Weiner J, Hankir M, Heiker JT, Fenske W, Krause K. Thyroid hormones and browning of adipose tissue. Mol Cell Endocrino. (2017) 458:156–9. doi: 10.1016/j.mce.2017.01.011
46. Sun Y. Ghrelin receptor controls obesity by fat burning. Oncotarget. (2015) 6:6470–64. doi: 10.18632/oncotarget.3668
47. Lin L, Lee JH, Bongmba OY, Ma X, Zhu X, Sheikh-Hamad D, et al. The suppression of ghrelin signaling mitigates age-associated thermogenic impairment. Aging (Albany NY). (2014) 6:1019–32. doi: 10.18632/aging.100706
48. Fisher FM, Kleiner S, Douris N, Fox EC, Mepani RJ, Verdeguer F, et al. FGF21 regulates PGC-1a and browning of white adipose tissues in adaptive thermogenesis. Genes Dev. (2012) 26:271–81. doi: 10.1101/gad.177857.111
49. Villarroya J, Gallego-Escuredo JM, Delgado-Anglés A, Cairó M, Moure R, Gracia Mateo M, et al. Aging is associated with increased FGF21 levels but unaltered FGF21 responsiveness in adipose tissue. Aging Cell. (2018) 17:e12822. doi: 10.1111/acel.12822
50. Salminen A, Kaarniranta K, Kauppinen A. Regulation of longevity by FGF21:Interaction between energy metabolism and stress responses. Ageing Res Rev. (2017) 37:79–93. doi: 10.1016/j.arr.2017.05.004
51. Fisher FM, Chui PC, Antonellis PJ, Bina HA, Kharitonenkov A, Flier JS, et al. Obesity is a fibroblast growth factor 21 (FGF21)-resistant state. Diabetes. (2010) 59:2781–9. doi: 10.2337/db10-0193
52. Lorenzo M, Fernández-Veledo S, Vila-Bedmar R, Garcia-Guerra L, De Alvaro C, Nieto-Vazquez I. Insulin resistance induced by tumor necrosis factor-α in myocytes and brown adipocytes12. J Anim Sci. (2008) 86:E94–104. doi: 10.2527/jas.2007-0462
53. Villarroya F, Cereijo R, Gavaldà-Navarro A, Villarroya J, Giralt MJ. Inflammation of brown/beige adipose tissues in obesity and metabolic disease. Intern Med. (2018) 284:492–504. doi: 10.1111/joim.12803
54. Bae J, Ricciardi CJ, Esposito D, Komarnytsky S, Hu P, Curry BJ, et al. Activation of pattern recognition receptors in brown adipocytes induces inflammation and suppresses uncoupling protein 1 expression and mitochondrial respiration. Am J Physiol Cell Physiol. (2014) 306:C918–30. doi: 10.1152/ajpcell.00249.2013
55. Timmons JA, Wennmalm K, Larsson O, Walden TB, Lassmann T, Petrovic N, et al. Myogenic gene expression signature establishes that brown and white adipocytes originate from distinct cell lineages. Proc Natl Acad Sci USA. (2007) 104:4401–6. doi: 10.1073/pnas.0610615104
56. Seale P, Bjork B, Yang W, Kajimura S, Chin S, Kuang S, et al. PRDM16 controls a brown fat/skeletal muscle switch. Nature. (2008) 454:961–7. doi: 10.1038/nature07182
57. Tran KV, Gealekman O, Frontini A, Zingaretti MC, Morroni M, Giordano A, et al. The vascular endothelium of the adipose tissue gives rise to both white and brown fat cells. Cell Metab. (2012) 15:222–9. doi: 10.1016/j.cmet.2012.01.008
58. Xue B, Rim J-S, Hogan JC, Coulter AA, Koza RA, Kozak LP. Genetic variability affects the development of brown adipocytes in white fat but not in interscapular brown fat. J Lipid Res. (2012) 48:41–51. doi: 10.1194/jlr.M600287-JLR200
59. Wu J, Boström P, Sparks LM, Ye L, Choi JH, Giang AH, et al. Beige adipocytes are a distinct type of thermogenic fat cell in mouse and human. Cel. (2012) 150:366–76. doi: 10.1016/j.cell.2012.05.016
60. Qiang L, Wang L, Kon N, Zhao W, Lee S, Zhang Y, et al. Brown remodeling of white adipose tissue by SirT1-dependent deacetylation of Pparγ. Cell. (2012) 150:620–32. doi: 10.1016/j.cell.2012.06.027
61. Becerril S, Gómez-Ambrosi J, Martín M, Moncada R, Sesma P, Burrell MA, et al. Role of PRDM16 in the activation of brown fat programming. Relevance to the development of obesity. Histol Histopathol. (2013) 28:1411–25. doi: 10.14670/HH-28.1411
62. Khanh VC, Zulkifli AF, Tokunaga C, Yamashita T, Hiramatsu Y, Ohneda O. Aging impairs beige adipocyte differentiation of mesenchymal stem cells via the reduced expression of Sirtuin1. Biochem Biophys Res Commun. (2018). 500:682–90. doi: 10.1016/j.bbrc.2018.04.136
63. Yamakuchi M. MicroRNA Regulation of SIRT1. Front Physiol. (2012) 3:68. doi: 10.3389/fphys.2012.00068
64. Gollisch KSC, Brandauer J, Jessen N, Toyoda T, Nayer A, Hirshman MF, et al. Effects of exercise training on subcutaneous and visceral adipose tissue in normal- and high-fat diet-fed rats. AJP Endocrinol. Metab. (2009) 297:E495–504. doi: 10.1152/ajpendo.90424.2008
65. Thiripathi A, da Silva Pieri BL, Queiroz JAMP, Rodrigues MS, de Bem Silveira G, de Souza DR, et al. Strength training and aerobic exercise alter mitochondrial parameters in brown adipose tissue and equally reduce body adiposity in aged rats. J Physiol Biochem. (2019) 75:101–8. doi: 10.1007/s13105-019-00663-x
66. Ignacio D, Fortunato R, Neto RA, Da Silva Silvestre D, Nigro M, Frankenfeld TG, et al. Blunted response of pituitary type 1 and brown adipose tissue type 2 deiodinases to swimming training in ovariectomized rats. Horm Metab Res. (2012) 44:797–803. doi: 10.1055/s-0032-1314875
67. Vosselman MJ, Hoeks J, Brans B, Pallubinsky H, Nascimento EB, van der Lans AA, et al. Low brown adipose tissue activity in endurance trained compared to lean sedentary men. Int J Obes. (2015) 39:1–7. doi: 10.1038/ijo.2015.130
68. Fontana L, Partridge L. Promoting health and longevity through diet:from model organisms to humans. Cell. (2015) 161:106–18. doi: 10.1016/j.cell.2015.02.020
69. Li G, Xie C, Lu S, Nichols RG, Tian Y, Li L, et al. Intermittent fasting promotes white adipose browning and decreases obesity by shaping the gut microbiota. Cell Metabol. (2017) 26:672–85. doi: 10.1016/j.cmet.2017.08.019
70. Orava J, Nuutila P, Lidell ME, Oikonen V, Noponen T, Viljanen T, et al. Different metabolic responses of human brown adipose tissue to activation by cold and insulin. Cell Metab. (2011) 14:272–9. doi: 10.1016/j.cmet.2011.06.012
71. Dulloo AG. The search for coumpounds that stimulate thermogenesis in obesity management:from pharmaceuticals to functional food ingredients. Obes Rev. (2011) 12:866–83. doi: 10.1111/j.1467-789X.2011.00909.x
72. Janssens PLHR, Hursel R, Martens EAP, Westerterp–plantenga MS. Acute effects of capsaicin on energy expenditure and fat oxidation in negative energy balance. PLoS ONE. (2013) 8:e67786. doi: 10.1371/journal.pone.0067786
73. Baskaran P, Krishnan V, Ren J, Thyagarajan B. Capsaicin induces browning of white adipose tissue and counters obesity by activating TRPV1 channel-dependent mechanism. Br J Pharmacol. (2016) 173:2369–89. doi: 10.1111/bph.13514
74. Josse AR, Sherriffs SS, Holwerda AM, Andrews R, Staples AW, Phillips SM. Effects of capsinoids ingestion on energy expenditure and lipid oxidation at rest and during exercise. Nutr Metabol. (2010) 7:65. doi: 10.1186/1743-7075-7-65
75. Saito M. Capsaicin and related food ingredients reducing body fat through the activation of TRP and brown fat thermogenesis. Adv Food Nutr Res. (2015) 76:1–28. doi: 10.1016/bs.afnr.2015.07.002
76. Pahlavani M, Razafimanjato F, Ramalingam L, Kalupahana NS, Moussa H, Scoggin S, et al. Eicosapentaenoic acid regulates brown adipose tissue metabolism in high-fat-fed mice and in clonal brown adipocytes. J Nutr Biochem. (2017) 39:101–9. doi: 10.1016/j.jnutbio.2016.08.012
77. Hiroshima Y, Yamamoto T, Watanabe M, Baba Y, Shinohara Y. Effects of cold exposure on metabolites in brown adipose tissue of rats. Mol Genet Metabol Rep. (2018) 15:36–42. doi: 10.1016/j.ymgmr.2018.01.005
78. Yamamoto T, Yamamoto A, Watanabe M, Kataoka M, Terada H, Shinohara Y. Quantitative evaluation of the effects of cold exposure of rats on the expression levels of ten FABP isoforms in brown adipose tissue. Biotechnol Lett. (2011) 33:237–42. doi: 10.1007/s10529-010-0444-0
79. Chouchani ET, Kazak L, Jedrychiwski MP, Lu GZ, Erickson BK, Szpyt J, et al. Mitochondrial ROS regulate thermogenic energy expenditure and sulfenylation of UCP1. Nature. (2016) 532:112–6. doi: 10.1038/nature17399
80. Barbato DL, Tatulli G, Cannata SM, Bernardini S, Aquilano K, Ciriolo MR. Glutathione decrement drives thermogenic program in adipose cells. Sci Rep. (2015) 5:13091. doi: 10.1038/srep13091
81. Castriota G, Thompson GM, Lin Y, Scherer PE, Moller DE, Berger JP. Peroxisome proliferator-activated receptor gamma agonists inhibit adipocyte expression of alpha1-acid glycoprotein. Cell Biol Int. (2007) 31:586–91. doi: 10.1016/j.cellbi.2006.11.033
82. Finlin BS, Memetimin H, Confides AL, Kasza I, Zhu B, Vekaria HJ, et al. Human adipose beiging in response to cold and mirabegron. JCI Insight. (2018) 9:121510. doi: 10.1172/jci.insight.121510
83. Bonet ML, Ribot J, Felipe F, Palou A. Vitamin A and the regulation of fat reserves. Cell Mol Life Sci. (2003) 60:1311–21. doi: 10.1007/s00018-003-2290-x
84. Murholm M1, Isidor MS, Basse AL, Winther S, Sørensen C, Skovgaard-Petersen J, et al. Retinoic acid has different effects on UCP1 expression in mouse and human adipocytes. BMC Cell Biol. (2013) 14:41. doi: 10.1186/1471-2121-14-41
85. Schulz TJ, Tseng YH. Emerging role of bone morphogenetic proteins in adipogenesis and energy metabolism. Cytokine Growth Factor Rev. (2009) 20:523–31. doi: 10.1016/j.cytogfr.2009.10.019
86. Kim S, Choe S, Lee DK. BMP-9 enhances fibroblast growth factor 21 expression and suppresses obesity. Biochim Biophys Acta. (2006) 1862:1237–46. doi: 10.1016/j.bbadis.2016.04.006
87. Whittle AJ, Carobbio S, Martins L, Slawik M, Hondares E, Vázquez MJ, et al. BMP8B increases brown adipose tissue thermogenesis through both central and peripheral actions. Cell. (2012) 149:871–85. doi: 10.1016/j.cell.2012.02.066
88. Guerre-Millo M, Gervois P, Raspé E, Madsen L, Poulain P, Derudas B, et al. Peroxisome proliferator-activated receptor alpha activatorsimprove insulin sensitivity and reduce adiposity. J Biol Chem. (2002) 275:16638–42 doi: 10.1074/jbc.275.22.16638
Keywords: brown adipose tissue (BAT), beige adipose tissue, aging, inflammaging, metabolic disease
Citation: Zoico E, Rubele S, De Caro A, Nori N, Mazzali G, Fantin F, Rossi A and Zamboni M (2019) Brown and Beige Adipose Tissue and Aging. Front. Endocrinol. 10:368. doi: 10.3389/fendo.2019.00368
Received: 20 December 2018; Accepted: 23 May 2019;
Published: 20 June 2019.
Edited by:
Antonello Lorenzini, University of Bologna, ItalyReviewed by:
Joan Villarroya, Hospital de la Santa Creu i Sant Pau, SpainEz-Zoubir Amri, University of Nice Sophia Antipolis, France
Copyright © 2019 Zoico, Rubele, De Caro, Nori, Mazzali, Fantin, Rossi and Zamboni. This is an open-access article distributed under the terms of the Creative Commons Attribution License (CC BY). The use, distribution or reproduction in other forums is permitted, provided the original author(s) and the copyright owner(s) are credited and that the original publication in this journal is cited, in accordance with accepted academic practice. No use, distribution or reproduction is permitted which does not comply with these terms.
*Correspondence: Elena Zoico, elena.zoico@univr.it