- 1Virta Health Corp, San Francisco, CA, United States
- 2Indiana University Health Arnett, Lafayette, IN, United States
- 3Department of Nutrition Science, Purdue University, West Lafayette, IN, United States
- 4Department of Human Sciences, The Ohio State University, Columbus, OH, United States
- 5Department of Genetics, Washington University School of Medicine, St. Louis, MO, United States
Purpose: Studies on long-term sustainability of low-carbohydrate approaches to treat diabetes are limited. We previously reported the effectiveness of a novel digitally-monitored continuous care intervention (CCI) including nutritional ketosis in improving weight, glycemic outcomes, lipid, and liver marker changes at 1 year. Here, we assess the effects of the CCI at 2 years.
Materials and methods: An open label, non-randomized, controlled study with 262 and 87 participants with T2D were enrolled in the CCI and usual care (UC) groups, respectively. Primary outcomes were retention, glycemic control, and weight changes at 2 years. Secondary outcomes included changes in body composition, liver, cardiovascular, kidney, thyroid and inflammatory markers, diabetes medication use and disease status.
Results: Reductions from baseline to 2 years in the CCI group resulting from intent-to-treat analyses included: HbA1c, fasting glucose, fasting insulin, weight, systolic blood pressure, diastolic blood pressure, triglycerides, and liver alanine transaminase, and HDL-C increased. Spine bone mineral density in the CCI group was unchanged. Use of any glycemic control medication (excluding metformin) among CCI participants declined (from 55.7 to 26.8%) including insulin (-62%) and sulfonylureas (-100%). The UC group had no changes in these parameters (except uric acid and anion gap) or diabetes medication use. There was also resolution of diabetes (reversal, 53.5%; remission, 17.6%) in the CCI group but not in UC. All the reported improvements had p < 0.00012.
Conclusion: The CCI group sustained long-term beneficial effects on multiple clinical markers of diabetes and cardiometabolic health at 2 years while utilizing less medication. The intervention was also effective in the resolution of diabetes and visceral obesity with no adverse effect on bone health.
Clinical Trial Registration: Clinicaltrials.gov NCT02519309
Introduction
Type 2 diabetes (T2D), obesity, and metabolic disease impact over one billion people and present a challenge to public health and economic growth (1, 2). In the United States, over 30 million people have diabetes and it is recognized among the leading causes of morbidity and mortality, especially through increased cardiovascular disease (CVD) (3). The remission rate under usual care is 0.5–2% (4) while an intensive lifestyle intervention resulted in remission rates (both partial and complete) of 11.5 and 9.2% at 1 and 2 years (5). When lifestyle interventions are insufficient, medications are indicated to manage the disease and slow progression (6, 7).
When T2D care directed at disease reversal is successful, this includes achievement of restored metabolic health, glycemic control with reduced dependence on medication, and in some cases disease remission. Three non-pharmaceutical approaches have demonstrated high rates of at least temporary T2D diabetes reversal or remission: bariatric surgery, very low calorie diets (VLCD), and nutritional ketosis achieved through carbohydrate restriction (8–10). In controlled clinical trials, each approach has demonstrated improved glycemic control and CVD risk factors, reduced pharmaceutical dependence, and weight loss. The three approaches show a similar time-course with glycemic control preceding weight loss by weeks or months, suggesting potential overlap of mechanisms (11, 12).
With bariatric surgery, up to 60% of patients demonstrate T2D remission at 1 year (13). Outcomes at 2 years and beyond indicate ~50% of patients can achieve ongoing diabetes remission (13, 14). The second Diabetes Surgery Summit recommended using bariatric surgery to treat T2D with support from worldwide medical and scientific societies (15), but both complications associated with surgery and cost limit its widespread use (16, 17). VLCDs providing <900 kcal/day allow rapid discontinuation of most medications, improved glycemic control, and weight loss. This approach is necessarily temporary, however, with weight regain and impaired glucose control typically occurring within 3–6 months of reintroduction of substantial proportions of dietary carbohydrates (9, 18–20).
A third approach to diabetes reversal is sustained dietary carbohydrate restriction. Low-carbohydrate diets have consistently elicited improvements in T2D, metabolic disease, and obesity up to one year (21, 22), however, longer-term studies and studies including patients prescribed insulin are limited. A low-carbohydrate Mediterranean diet caused remission in 14.7% of newly diagnosed diabetes patients at 1 year vs. 4.1% with a low-fat diet (23), and a small randomized trial utilizing a ketogenic diet demonstrated improved weight and diabetes control at 1 year (24). Systematic reviews also corroborate the effectiveness of a low-carbohydrate diet for T2D (25, 26) and it has recently become a consensus recommended dietary option (27–29). Nonetheless, sustained adherence to carbohydrate restriction is considered challenging (27, 28) and an LDL-C increase is sometimes observed (30–33). Given that total LDL-particles (LDL-P), small LDL-P, and ApoB tend to improve or remain unchanged, the impact of an increase in LDL-C on CVD risk in the context of this dietary pattern is unknown.
We have previously reported 1 year outcomes of an open-label, non-randomized, controlled, longitudinal study with 262 continuous care intervention (CCI), and 87 usual care (UC) participants with T2D (10). The CCI included individualized support with telemedicine, health coaching, and guidance in nutritional ketosis using an individualized low-carbohydrate diet. Nutritional guidance encouraged sustained nutritional ketosis; patients were counseled on preparation of a low-carbohydrate diet adapted to meet their life circumstances. Eighty-three percent of CCI participants remained enrolled at 1 year and 60% of completers achieved an HbA1c <6.5% while prescribed metformin or no diabetes medication. Weight was reduced and most CVD risk factors improved (33).
Long-term studies of low-carbohydrate dietary approaches to treat type 2 diabetes and obesity are limited, particularly among those that are delivered and supported remotely. Here we assess longer-term outcomes in CCI participants with T2D at 2 years, as well as the effects on body composition and related comorbidities. The primary aims were to investigate the effect of the CCI on retention, glycemic control, diabetes status, and weight. Secondary aims included: (1) investigating the effect of the CCI on bone mineral density, visceral fat composition, cardiovascular risk factors, liver, kidney, thyroid and inflammatory markers, and related disease outcomes (e.g., metabolic syndrome); and (2) comparing 2-year outcomes between the CCI and UC groups.
Materials and Methods
Study Design and Participants
The comprehensive study design was previously published with the 1 year outcomes (10, 33), and the results presented here are the follow-up 2-year results from the same ongoing five-year clinical trial (Clinical trials.gov identifier: NCT02519309). This is an open-label, non-randomized, outpatient study, and results presented here are based on data from the first 2 years of the trial collected from August, 2015 to May, 2018. Participants aged 21 to 65 years with a confirmed diagnosis of T2D and a body mass index (BMI) >25 kg/m2 self-selected to receive either the CCI or usual care (UC). Major exclusion criteria are listed in the previous publication (10, 33). All study participants provided written informed consent and the study was approved by the Franciscan Health Lafayette Institutional Review Board, Lafayette, IN, USA.
Study Interventions
Continuous Care Intervention (CCI)
For the intervention group, participants were advised to achieve and sustain nutritional ketosis (blood BHB level of 0.5–3.0 mmol L−1) through sufficient carbohydrate restriction (initially <30 g day−1 but gradually increased based on personal carbohydrate tolerance and health goals). Participants' daily protein intake was initially targeted at a level of 1.5 g kg−1 of a medium-frame ideal weight body and further individualized based on biomarkers. Participants were instructed to include sufficient dietary fat in meals to achieve satiety without tracking energy intake. Nutrition education directed consumption of monounsaturated and saturated fat with sufficient intake of omega-3 and omega-6 polyunsaturated fats. The participants were also encouraged to consume sufficient fluid, vitamins and minerals including sodium and magnesium, especially if signs of mineral deficiency were encountered (e.g., decreased circulating volume) (10).
The CCI participants were provided access to a web-based software application (app), which was used to provide telemedicine communication, online resources and biomarker tracking tools. The participants used the app to upload and monitor their reportable biomarkers including body weight, blood glucose and beta-hydroxybutyrate (BHB). Biomarkers allowed for daily feedback to the care team and individualization of patient instruction. Frequency of reporting was personalized over time based on care needs. The web-based app was also used by participants to communicate with their remote care team consisting of a health coach and a medical provider. The remote care team provided education and support regarding dietary changes, behavior modification techniques for maintenance of lifestyle changes, and directed medication changes for diabetes and antihypertensive medications. Education modules covered core concepts related to the dietary changes for achieving nutritional ketosis, and adaptation to and maintenance of the diet (10). Participants selected their preferred education mode (CCI-virtual, n = 126 or CCI-onsite, n = 136) during recruitment. The CCI-virtual group received care and education primarily via app-based communication. The CCI-onsite group also received care and education via clinic-based group meetings (weekly for 12 weeks, bi-weekly for 12 weeks, monthly for 6 months, and then quarterly in the second year). All participants had access to the app for communication with their care team, online resources, biomarker tracking and the opportunity to participate in an online peer community for social support.
Usual Care (UC)
The participants recruited for usual care (UC) received care from their primary care physician or endocrinologist and were counseled by a registered dietician as part of a diabetes education program. These participants received the American Diabetes Association (ADA) recommendations on nutrition, lifestyle and diabetes management. No modification of their care was made for the study and routine biomarkers (weight, glucose and ketones) were not collected from these participants. This group was used as a reference control to study the effect of disease progression over 2 years in a cohort of participants prospectively recruited from the same geography and healthcare system.
Figure 1 depicts the study flow from recruitment to 2 years post-enrollment.
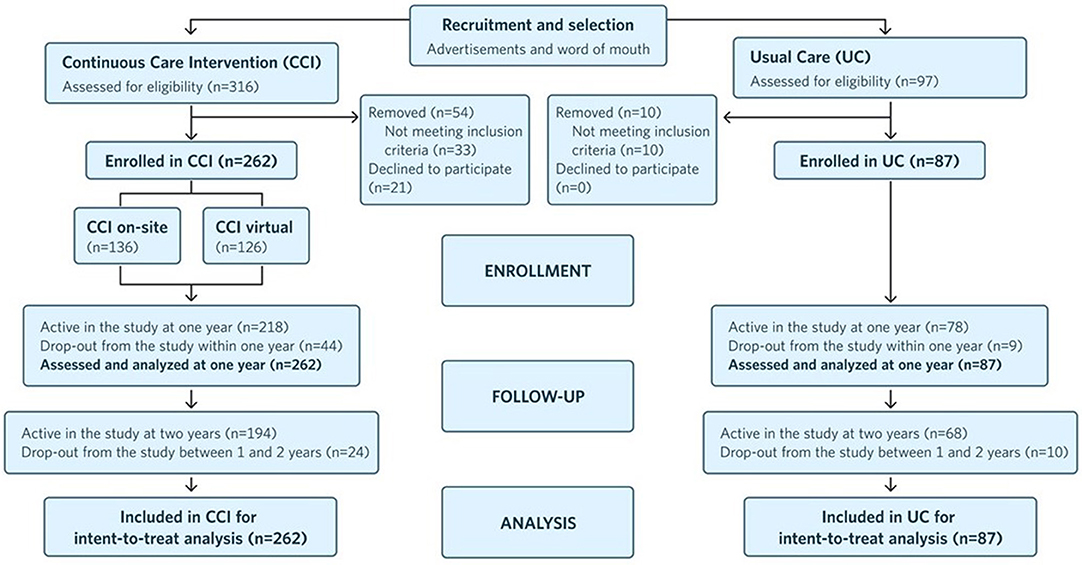
Figure 1. Flow chart of participants in each stage of the study from recruitment to 2 years post-enrollment and analysis.
Outcomes
Primary Outcomes
The primary outcomes were retention, HbA1c, HOMA-IR derived from insulin or c-peptide (formulas listed in Supplementary Table 1), fasting glucose, fasting insulin, c-peptide and weight.
Secondary Outcomes
Long-term body composition changes assessed in CCI participants included bone mineral density (BMD), abdominal fat content (CAF and A/G ratioC), and lower extremity lean mass (LELM). Body composition was not assessed in UC participants. Cardiovascular-related markers included resting blood pressure (systolic and diastolic), triglycerides, total cholesterol, HDL-C and calculated LDL-C (Friedewald equation, Supplementary Table 1). Liver-related markers included the liver enzymes alanine aminotransferase (ALT), aspartate aminotransferase (AST), and alkaline phosphatase (ALP), bilirubin, and two calculated liver scores: non-alcoholic liver fat score (NLF) and non-alcoholic liver fibrosis score (NFS) (formulas in Supplementary Table 1). Kidney-related markers included serum creatinine, uric acid, anion gap, blood urea nitrogen (BUN), and estimated glomerular filtration rate (eGFR). Thyroid-related markers included thyroid stimulating hormone (TSH) and free T4. Inflammatory markers included high sensitive C-reactive protein (hsCRP) and white blood cell count (WBC). Changes in overall diabetes medication use, use by class, and insulin dose were tracked over the 2 years of the trial.
The prevalence and resolution of T2D (diabetes reversal, partial and complete remission), metabolic syndrome, liver steatosis, and fibrosis were evaluated at baseline and 2 years using the criteria provided in Supplementary Table 2. Assignment of metabolic syndrome was based on the presence of three of the five defined criteria according to measured laboratory and anthropometric variables (34, 35) and pharmacological treatment for any of the conditions was not considered in the assignment (Supplementary Table 2).
Adverse events encountered in the study were reported to the Principal Investigator and reviewed by the Institutional Review Board (IRB).
Laboratory Measures
Clinical anthropometrics and laboratory blood analyte measurements were obtained at baseline, 1 year, and 2 years from the CCI and UC participants. Details of the methods were previously published (10). All blood analytes were measured at a Clinical Laboratory Improvement Amendments (CLIA) certified laboratory.
Body Composition Measures
The CCI participants' total body composition was measured at baseline, 1 year and 2 years using dual-energy X-ray absorptiometry (DXA) (Lunar GE Prodigy, Madison, WI). Participants were scanned while wearing light clothing using standard clinical imaging procedures. The scans obtained were analyzed using GE Encore software (v11.10, Madison, WI). In many obese patients, full body scans were not obtained due to the scanner not accommodating the patient's complete body resulting in issues such as cropping of the arms and/or overlapping of arms with the chest (36, 37). To address these limitations, changes in bone density and fat and lean mass were assessed using subregions rather than the full body scan. We assessed changes in the bone mass by evaluating total spine bone mineral density (BMD) from baseline to 2 years (38). For assessment of fat mass, we manually selected the central abdominal fat (CAF) region using the software and evaluated the changes in CAF over time, as previously suggested for overweight individuals (36, 39). Furthermore, we assessed changes in the android:gynoid (A/G) ratio by time. Due to lack of proper arm lean mass measurement, we analyzed the lower extremity lean mass (LELM) to assess weight-related changes in lean mass over time (40, 41).
Statistical Analyses
All analyses were conducted using SPSS statistical software (Version 25.0, Armonk, NY). First, we examined the assumptions of normality and linearity. According to Kline's (2011) guidelines (42), 14 outcomes (i.e., fasting insulin, insulin and C-peptide-derived HOMA-IR scores, triglycerides, ALT, AST, bilirubin, N-LFS, BUN, serum creatinine, TSH, Free T4, hsCRP, and BHB) were positively skewed. We explored two approaches to handling the skewed variables: natural log-transformations and removing the top 1% of values. For N-LFS, which includes both positive and negative values, a modulus log-transformation was performed instead of a natural log-transformation (43). For most variables, both approaches resulted in new skew and kurtosis values within the acceptable range. One variable (triglycerides) was only corrected via log-transformation, whereas two variables (C-peptide-derived HOMA-IR and TSH) were only corrected by removing the top 1% of values. For the other variables, we conducted sensitivity analyses to compare the two approaches. Because the results did not differ between the approaches and because interpretation of outcomes is more difficult with transformed variables, we report results from the approach of removing the top 1% of values for all variables except triglycerides. For triglycerides, analyses were performed and p-values reported on the log-transformed variable but the means and standard errors reported were computed from the untransformed variable. Next, we ran independent sample t-tests to examine differences in baseline characteristics between CCI and UC, and completers and dropouts.
We performed linear mixed-effects models (LMMs) to assess (1) within-group changes in the continuous study outcomes from baseline to 2 years and (2) between-group differences (CCI vs. UC) in the study outcomes at 2 years. The LMMs included fixed effects for time, group (CCI vs. UC), and a time by group interaction. Covariates included baseline age, sex, race (African American vs. other), BMI, and insulin use. This maximum likelihood-based approach uses all available repeated data, resulting in an intent-to-treat analysis. An unstructured covariance structure was specified for all models to account for correlations between repeated measures.
Within-group changes and between-group differences in dichotomous disease outcome variables [i.e., diabetes reversal, diabetes remission (partial or complete) and complete remission (44), metabolic syndrome (34, 35), steatosis (45), fibrosis (46)] were assessed, controlling for baseline age, sex, race, time since diagnosis, BMI, and insulin use. For this set of analyses, multiple imputation was used to replace missing values from baseline and 2 years with a set of plausible values, facilitating an intent-to-treat analysis (all ns = 262). Missing values were estimated from 40 imputations (47) from logistic regression. Within-group changes from baseline to 2 years and between-group differences at 2 years were assessed using generalized estimating equations with binary logistic models and unstructured covariance matrices.
We also examined changes in participants' diabetes medication use. First, we compared the rates of diabetes medication use within groups from baseline to 2 years using McNemar's test with continuity correction when appropriate. Next, we calculated the proportion of participants in each group with each diabetes medication class eliminated, reduced, not changed, increased, or added. Paired t-tests were used to assess within-group changes in insulin dosages from baseline to 2 years among participants taking insulin at baseline and among participants taking insulin at both baseline and 2 years.
We conducted a second set of analyses with 2-year completers only. Results of the completers-only analyses appear in Supplementary Table 3. Given that 2 different modes (virtual and onsite) were utilized for delivery of the CCI group educational content, we also conducted another set of analyses to assess whether differences existed between the groups on all analyses of primary outcomes. As in our prior time points (10, 48), no group differences were found; thus, the data from the two CCI educational groups were combined for this report. For all study analyses, nominal significance levels (P) are presented in the tables. A significance level of P < 0.0012 ensures overall simultaneous significance of P < 0.05 over the 43 variables using Bonferroni correction.
Results
Participant Characteristics
Table 1 presents baseline characteristics of the 262 CCI and 87 UC participants. Participants did not differ between groups in demographic characteristics, except the proportion of African Americans was higher in the CCI group. Baseline characteristics were well-matched between the groups, except for mean weight and BMI, which were higher in the CCI group. There were no differences between completers and dropouts on baseline characteristics for either group.
Retention and Long-Term Dietary Adherence
One hundred ninety four participants (74% of 262) remained enrolled in the CCI at 2 years (Figure 1), as did 68 UC group participants (78% of 87). CCI-participant-reported reasons for dropout included: intervening life events (e.g., family emergencies), difficulty attending or completing laboratory and clinic visits associated with the trial, and insufficient motivation for participation in the intervention. At both 1 and 2 years, laboratory-measured blood BHB was 0.27 ± 0.02 mmol L−1, 50% higher than the baseline value (0.18 ± 0.01 mmol L−1). The mean laboratory BHB level was stable from 1 to 2 years, and 61.5% (n = 161) of participants uploaded a blood BHB measurement >0.5 mmol L−1 in the app at least once between 1 and 2 years.
Glycemic Control
HbA1c improved at 2 years (0.9% unit decrease, P = 1.8 × 10−17; Figure 2A) among CCI participants and was lower than the UC group. Related markers including C-peptide, fasting glucose, fasting insulin (Figure 2B), insulin-derived HOMA-IR excluding exogenous insulin users, and c-peptide-derived HOMA-IR also significantly decreased after correction for multiple comparisons in the CCI group at 2 years and were lower than the UC group (except C-peptide); no changes from baseline to 2 years were observed in the UC group (Supplementary Figures 1A,B; Table 2).
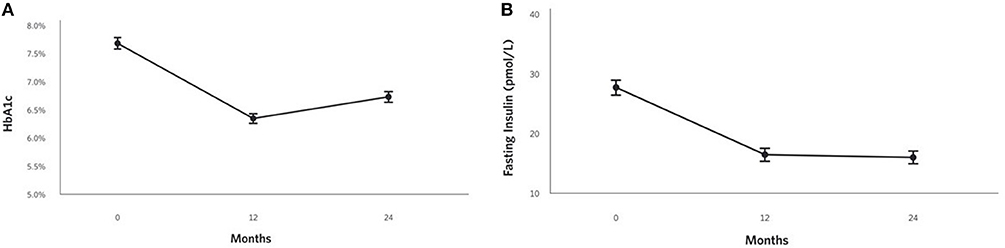
Figure 2. Adjusted mean changes from baseline to 2 years in the CCI group for (A) HbA1c (−12% relative to baseline, P = 1.8 × 10−17), (B) Fasting insulin (−42% relative to baseline, P = 2.2 × 10−18).
Within the CCI, reduction in glycemia occurred concurrently with reduced medication use (Supplementary Table 3). The proportion of CCI completers taking any diabetes medication (excluding metformin) decreased at 2 years (Figure 3A). The mean dose among CCI participants prescribed insulin at baseline decreased by 81% at 2 years (from 81.9 to 15.5 U/day), but not among UC participants (+13%; from 96.6 to 109.3 U/day) (Figure 3B). For participants who remained insulin-users at 2 years, mean dose also decreased in the CCI by 61% (from 104.3 to 40.2 U/day, P = 9.2 × 10−5) but not in UC participants (+19% from 103.8 to 123.5 U/day, P = 0.29). Among completers prescribed each diabetes medication class, the proportion with each dosage change (eliminated, reduced, unchanged, increased or newly added) at 2 years in each group appears in Figure 3C.
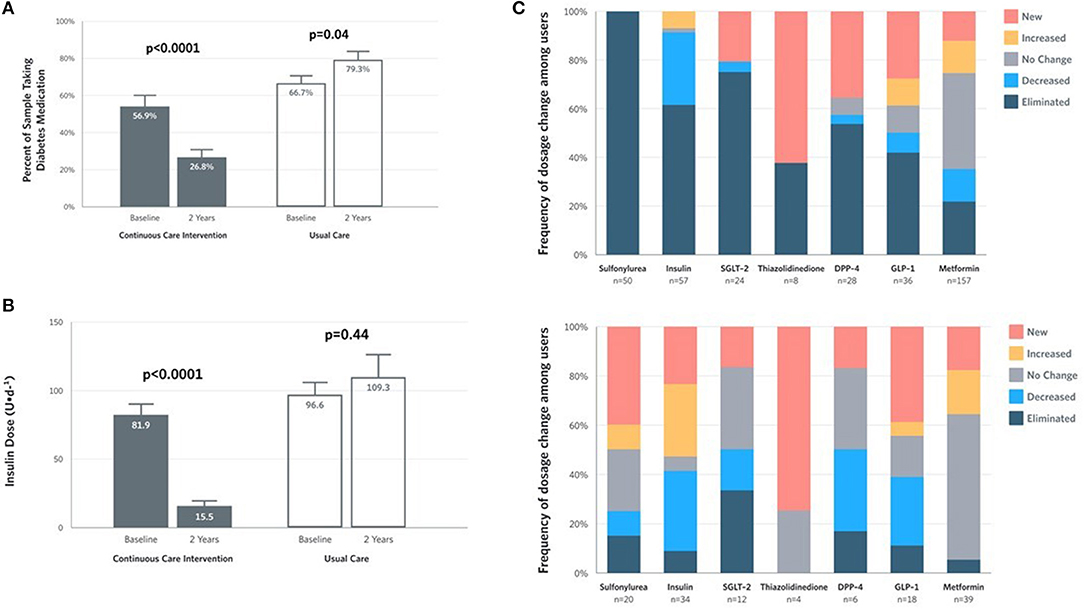
Figure 3. Medication and insulin dose changes from baseline to 2 years for CCI and UC group completers. (A) Percent of completers taking diabetes medications, excluding metformin. (B) Mean ± SE prescribed insulin dose among baseline users. (C) Frequency of medication dosage and use change among prescribed users by diabetes medication class.
Diabetes Status
All within-group changes and between-group differences in diabetes status among the CCI and UC group participants appear in Supplementary Table 4 (intent-to-treat analyses were conducted, all below ns = 262). The proportion of participants meeting the defined criteria for diabetes reversal at 2 years increased to 53.5% from baseline in the CCI group, whereas no change was observed in the UC group. Diabetes remission (partial or complete) was observed in 46 (17.6%) participants in the CCI group and two (2.4%) of the UC participants at 2 years. Complete remission was observed in 17 (6.7%) CCI participants and none (0%) of the UC participants at 2 years.
Weight and Body Composition Outcomes
At 2 years, the mean weight reduction from baseline was −10% (Figure 4A) in the CCI group, whereas no change was observed in the UC group (Supplementary Figure 1C). Among CCI patients, 74% had ≥5% weight loss compared to only 14% of UC patients (Supplementary Figure 2; completers analysis, n = 193). Consistent with the weight loss observed, the CCI group had reductions in abdominal fat content with decreases in CAF (Figure 4B) and the A/G ratio from baseline to 2 years (Table 2). Total spine BMD within the CCI remained unchanged from baseline to 2 years after correction for multiple comparisons, whereas the average LELM was reduced from baseline to 2 years (Table 2).
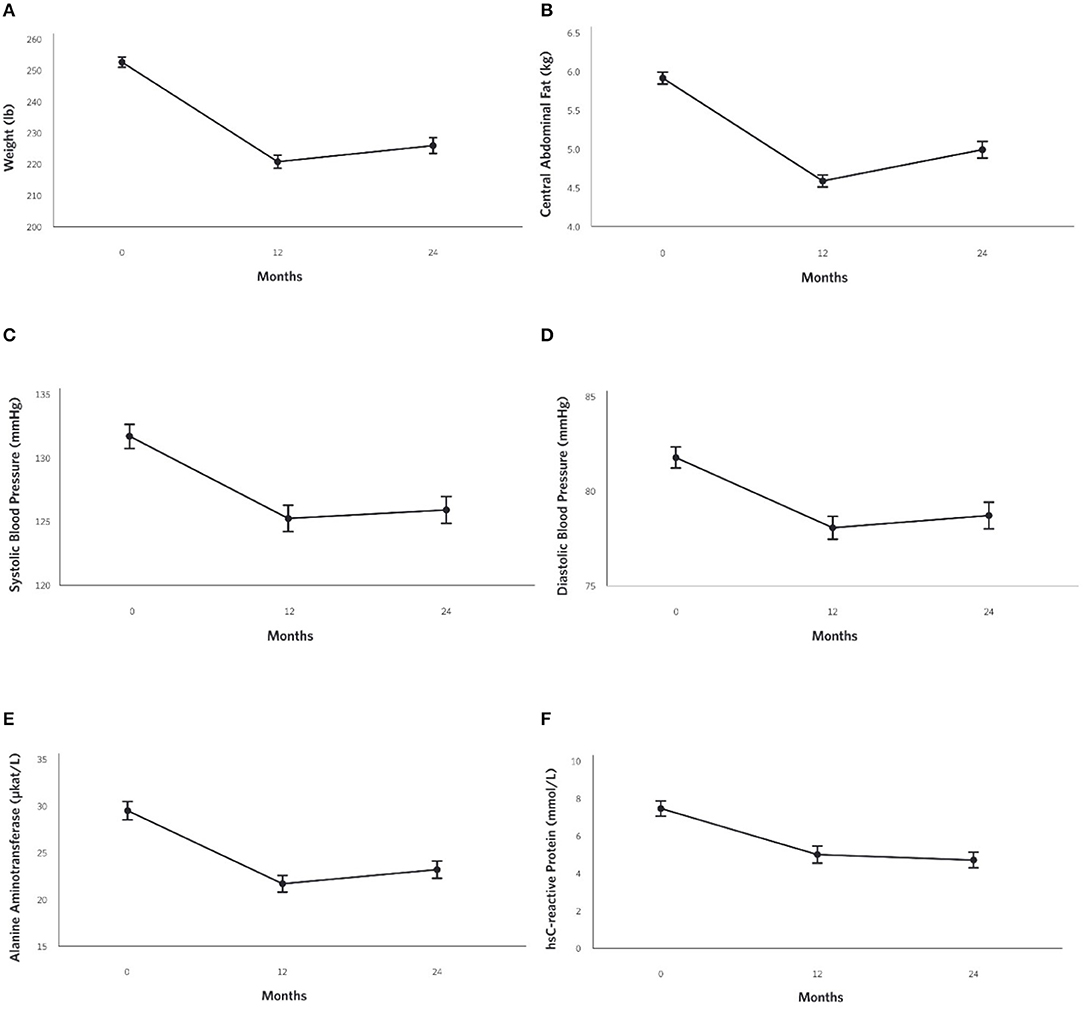
Figure 4. Adjusted mean changes from baseline to 2-years in the CCI group for (A) Weight (−10% relative to baseline, P = 8.8 × 10−28), (B) Central Abdominal Fat [CAF] (−15% relative to baseline, P = 1.6 × 10−21), (C) Systolic Blood Pressure (−4% relative to baseline, P = 2.4 × 10−6), (D) Diastolic Blood Pressure (−4% relative to baseline, P = 3.3 × 10−5) (E) Alanine aminotransferase [ALT] (−21% relative to baseline, P = 4.0 × 10−10), and (F) High sensitive C-reactive protein [hsCRP](−37% relative to baseline, P = 6.9 × 10−13).
Cardiovascular Risk Factor Outcomes
Decreases in systolic (Figure 4C) and diastolic (Figure 4D) blood pressures and triglycerides were observed in the CCI but not UC group at 2 years (Table 2; Supplementary Figures 3A,B). The CCI group's HDL-cholesterol and LDL-cholesterol both increased from baseline to 2 years, whereas no changes were observed in the UC group (Table 2). No changes in total cholesterol were observed in either the CCI or UC group. At 2 years, the CCI group had higher HDL-cholesterol, higher LDL-cholesterol, and lower triglycerides than UC. No between-group differences were observed at 2 years in systolic or diastolic blood pressure or total cholesterol (Table 2).
Liver-Related Outcomes
Reductions were observed in liver-related outcomes including ALT (Figure 4E), AST, ALP, NLF and NFS in the CCI group, whereas no changes were observed in the UC group (Table 2, e.g., ALT; Supplementary Figure 3C). No Bonferroni-corrected group differences were observed for bilirubin, ALT, or AST at 2 years (Table 2).
Kidney, Thyroid, and Inflammation Outcomes
The eGFR increased in the CCI but not UC group at 2 years (Table 2). The UC but not CCI group had increased anion gap and decreased uric acid. No bonferroni-corrected within-group changes in BUN, serum creatinine, TSH, or Free T4 were observed in either the CCI or UC group from baseline to 2 years. No between-group differences were observed for any thyroid- or kidney-related markers at 2 years (Table 2).
From baseline to 2 years, decreases in the CCI group's hsCRP (Figure 4F) and white blood cells were observed. No changes were observed in the UC group (Supplementary Figure 3D). At 2 years, both markers of inflammation were lower in the CCI group compared to the UC group (Table 2).
Related Comorbidities
All within-group changes and between-group differences in comorbidities status among the CCI and UC group participants appear in Supplementary Table 4 (intent-to-treat analyses were conducted, all below ns = 262) and Supplementary Table 5 (per-protocol analyses). At 2 years, 27.2% of CCI participants (P = 4.9 × 10−15) and 6.5% of UC patients showed resolution of metabolic syndrome. The proportion of CCI patients with suspected steatosis was reduced from 95.8 to 67.4% (P < 0.0 × 10−36), whereas no change occurred in UC at 2 years. The proportion of patients without suspected fibrosis increased from 18.3 to 30.8% (P = 1.4 × 10−5) in the CCI, but did not change in the UC at 2 years.
Adverse Events
In the CCI group, there were no reported adverse events between 1 and 2 years related to the intervention or that resulted in discontinuation, including no reported episodes of ketoacidosis or severe hypoglycemia requiring assistance. Limited or no change in kidney and thyroid functions were seen in the CCI at 2 years. Adverse events occurring in the first year of intervention (n = 6) were previously reported (11); during the second year of intervention, nine adverse events were reported including: one breast cancer diagnosis, one mycosis fungoides, one onset of atrial fibrillation (Afib) with heart failure, one onset of migraine, two cases of chest pain (one resulting in stent placement), one pulmonary effusion, and two pulmonary embolisms (one following orthopedic surgery and one with benign ovarian mass/Afib) in the CCI group. In the UC group, adverse events occurring in the first year (n = 6) were previously reported (11), and in the second year, adverse events occurred in six participants: one death from liver cancer, one hospitalization from recurrent seizure, one ureteropelvic junction obstruction from kidney stone, one cerebrovascular accident with left side weakness and sensory disturbances, one chest pain requiring percutaneous coronary intervention, and one deep vein thrombosis.
Discussion
Following 2 years of a remote continuous care intervention supporting medical and lifestyle changes, the CCI participants demonstrated improved HbA1c, fasting glucose and insulin, and HOMA-IR. Pharmaceutical interventions of 1.5 to 3 years duration report HbA1c reductions of 0.2 to 1.0% with DPP-4 inhibitors, SGLT-2 inhibitors and GLP-1 agonists (6, 7, 49). The HbA1c reduction of 0.9% with this CCI is comparable to that observed in pharmaceutical trials, but is achieved while discontinuing 67.0% of diabetes-specific prescriptions including most insulins and all sulfonylureas that engender risks for weight gain and hypoglycemia (50, 51). Comparable improvements in glycemic control and reduced medication were not observed in UC participants recruited from the same healthcare system, suggesting that the CCI improves diabetes management relative to usual care. Other interventions using carbohydrate restriction reported variable long-term glycemic improvement outcomes (52–57). The 0.9% absolute (12% relative) HbA1c reduction observed at 2 years is consistent with low carbohydrate studies reporting HbA1c reductions of 8–15% at 2 to 3.5 years (52, 55–57) with medication reduction. Two other studies reported no changes in HbA1c from baseline to 2 years, even though the low-carbohydrate arm reduced HbA1c in the first 6 months (53, 54).
Criticisms of low-carbohydrate diets relate to poor adherence and long-term sustainability (25, 26, 28). In this CCI, self-monitoring combined with continuous remote-monitoring and feedback from the care team, including behavioral support and nutrition advice via the app, may have improved accountability and engagement (58). In addition to glucose and weight tracking, dietary adherence was monitored by blood ketones. The 2 year BHB increase above baseline demonstrates sustained dietary modification. While laboratory BHB levels were increased from baseline, the encouraged range of nutritional ketosis (≥0.5 mM) was observed in only a minority (14.1%) of participants at 2 years. On average, patient-measured BHB was ≥0.5 mM for 32.8% of measurements over the 2 years (Supplementary Figure 4). This reveals an opportunity to increase adherence to nutritional ketosis for patients not achieving their desired health outcomes while prompting future research investigating the association between dietary adherence and health improvements.
A majority of the CCI participants (53.5%) met criteria for diabetes reversal at 2 years while 17.6% achieved diabetes remission (i.e., glycemic control without medication use) based on intent-to-treat with multiple imputation. The percentage of all CCI enrollees (N = 262) with verified reversal and remission requiring both completion of 2 years of the trial and an obtained laboratory value for HbA1c were 37.8 and 14.9%, respectively. CCI diabetes reversal exceeds remission as prescriptions for metformin were usually continued given its role in preventing disease progression (10, 59), preserving β-cell function (59) and in the treatment of pre-diabetes per guidelines (28). Partial and complete remission rates of 2.4 and 0.2% per year, respectively, were reported in 122,781 T2D patients receiving standard diabetes care (4). The 2 year remission rate (both partial and complete) in the CCI (17.6%) is higher than that achieved through intensive lifestyle intervention (ILI) in the Look AHEAD trial (9.2%) (5). Greater diabetes remission in the CCI vs. Look AHEAD ILI could result from differences in the dietary intervention (23), patients' ability to self-select their lifestyle change or effectiveness of continuous remote care. Length of time with a T2D diagnosis is a factor in remission, with longer time since diagnosis resulting in lower remission (4, 5, 9). Despite a mean and median of 8.4 and 7 years since diagnosis among CCI participants, the remission rate was higher than the Look AHEAD trial where its participants had a median of 5 years (4) since diabetes diagnosis.
Participants in the CCI achieved 10% mean weight loss (−11.9 kg) at 2 years. CCI weight loss was comparable to observed weight loss following surgical gastric banding (−10.7 kg) at 2 years (59). Previous studies consistently report that weight loss increases the likelihood of T2D remission (4, 5, 9). CCI participants also improved blood pressure, triglycerides, and HDL-cholesterol. Total cholesterol was unchanged and LDL-cholesterol was increased at 2 years, but was not different from the LDL-cholesterol level observed at 1 year (+0.51 mg/dL, P = 0.85). Despite the rise in LDL-cholesterol, the CCI cohort improved in 22 out of 26 CVD markers at 1 year (33). These changes included a decrease in small LDL-particles and large VLDL-P and an increase in LDL-particle size partitioning with no changes in ApoB (33), a marker considered a better predictor of CVD risk than LDL-cholesterol (33, 60). Non-elevated LDL cholesterol values together with higher triglycerides and lower HDL-cholesterol are common in patients with abdominal obesity, T2D, and metabolic syndrome (61, 62); these individuals often still have elevated atherogenic lipoproteins such as non-HDL (63), small LDL particles (62, 64), and VLDL (62, 64). In the CCI group, non-HDL cholesterol did not change from baseline to 2 years (141.7 ± 2.6 at baseline to 143.7 ± 3.1 mg/dl, P = 0.51) and several cardiovascular risk factors improved, suggesting that the rise in LDL-cholesterol may not be associated with increased atherogenic risk (65).
The CCI group had a reduction in visceral fat content, CAF and A/G ratio. This is consistent with other low-carbohydrate interventions reporting visceral fat reduction as a component of weight loss (30, 56, 66–68). Anatomical distribution of fat around the abdominal area (“android” obesity) is associated with T2D (69) and other comorbidities such as metabolic syndrome (70) and NAFLD (71). The alleviation of visceral fat in the CCI group was concurrent with resolution of metabolic syndrome at 2 years, while sustaining 1 year improvements of liver enzymes (10), steatosis, and fibrosis (72). The comprehensive effect of reduced visceral fat and improvement in associated comorbidities was previously reported (68, 73, 74). Rat studies have shown that removal of visceral adipose tissue increases insulin sensitivity while delaying T2D (75), and prevents metabolic syndrome and NAFLD (76). Resolution of liver steatosis and fibrosis may protect against other T2D macrovascular and microvascular complications such as cardiovascular disease and nephropathy (77). Furthermore, abdominal adiposity and NAFLD are frequently associated with altered inflammatory pathways in T2D patients (71). Excess free fatty acids from visceral adipose tissue may initiate chronic low-grade inflammation and activate nuclear factor kappa B signaling (71, 77). CCI participants also improved inflammatory status (hsCRP and WBC) at 1 year (10) and 2 years.
While some studies in animal models (78, 79) and children treated with ketogenic diets (80, 81) have suggested retardation in skeletal development and reduction in BMD, in this study of adults with T2D the CCI group had no change in total spine BMD over 2 years. Our results are consistent with other adult ketogenic dietary studies that reported no bone mass loss in short-term (66) or long-term follow-up of 2 (67, 82) and 5 (83) years. The differing findings of ketogenic diet on bone mass between adults and children could be due to differential effects on developed and mineralized vs. developing bones (84). In this study, the CCI group had a reduction (7.0%, 1.3 kg) in the calculated LELM. Most lean mass loss was encountered in the first year without further reduction in year 2. Studies have reported that obese adults have about 20% higher thigh muscle mass than those with normal weight (85). The reduced upper body load burden achieved through weight loss might explain the reduction of LELM. This reflects an appropriate weight loss-related reduction in muscle mass rather than muscle deficiency (86, 87). Weight loss (~10%) induced by energy restriction resulted in slightly higher lean mass loss than the CCI (8.4% appendicular lean mass and 7.6% total lean mass loss at 20 weeks) (88). Total lean mass loss from 10% weight reduction by bariatric surgery is reported in the range of 7.3 to 15.9% from baseline (89, 90). Greater weight is associated with more lean mass loss (91, 92). Approximately 25% of diet-induced weight loss (without exercise) often arises from lean mass (93). In the present intervention, lean mass loss contributed an estimated 14% to the lower extremity weight loss. The lower proportion of lean mass loss in the CCI group, despite higher percentage of weight loss, may be due to the adequate dietary protein recommendations (94, 95). Since ~73% of lean mass is water, the observed reduction of LELM in the first year of intervention may have arisen from natriuresis and water loss that occurs during keto-adaptation (96, 97).
Strengths and Limitations
This study's strengths include its size and prospective, longitudinal data collection from two participant groups (CCI and UC) which allowed statistical analysis by linear mixed effects model to investigate intervention time and treatment effects. The UC group was prospectively recruited from the same healthcare system. While not randomized, the participants' self-selection of intervention may contribute to the observed high retention and predicts real-life clinical management of chronic disease. The study also included patients prescribed insulin and with long-standing disease, groups often excluded from prior studies. The multi-component aspect of the intervention involving regular biomarker monitoring and access to a remote care team may have improved the long-term dietary adherence and engagement. The dietary advice including encouraging participants to restrict carbohydrates, moderate protein intake, and eat to satiety may also help in maintaining long-term effectiveness. Weaknesses of this study include the lack of randomization and racial diversity limiting generalization of the results to all T2D patients. Interpretation of DXA body composition was limited to subregion analyses due to the scanner not accommodating the patients' complete body.
Conclusions
At 2 years, the CCI, including remote medical management with instruction in nutritional ketosis, was associated with improvements in blood glucose, insulin, HbA1c, weight, blood pressure, triglyceride, liver function, and inflammation and reduced dependence upon medication. These long-term benefits were achieved concurrent with reduced prevalence of metabolic syndrome, and visceral adiposity. The CCI had no adverse effect on bone mineral density. The CCI group also had a higher prevalence of diabetes reversal and remission compared to the UC group following a standard diabetes care program. These results provide evidence that sustained improvement in diabetes status can be achieved through the continuous remote monitoring and accountability mechanisms provided by this multi-component CCI including recommendations for low-carbohydrate nutrition.
Ethics Statement
This study was carried out in accordance with the recommendations of Franciscan Health Lafayette Institutional Review Board with written informed consent from all subjects. All subjects gave written informed consent in accordance with the Declaration of Helsinki. The protocol was approved by the Franciscan Health Lafayette Institutional Review Board.
Author Contributions
SA, RA, and JM drafted the manuscript. SA, RA, AM, NB, and SH participated in data acquisition and compiling. RA and SA analyzed the data. JM, AM, NB, WC, RA, SA, SH, SP, and JV edited the manuscript. All authors approved the final version of the manuscript.
Funding
Virta Health Corp. was the study sponsor.
Conflict of Interest Statement
SA, RA, SH, AM, NB, SP, and JM are employed by Virta Health Corp and were offered stock options. SP and JV are founders of Virta Health Corp.
The remaining author declares that the research was conducted in the absence of any commercial or financial relationships that could be construed as a potential conflict of interest.
Acknowledgments
We thank the participants for their involvement and commitment to be part of the study and help advance scientific knowledge. Particular thanks to all IUH staff, especially Sue Huff in helping with the DXA data analyses as well as Sydney Rivera who helped with other study related logistics. Finally, we appreciate the efforts of all the health coaches that were involved in working one-on-one with participants to achieve their health and dietary goals.
Supplementary Material
The Supplementary Material for this article can be found online at: https://www.frontiersin.org/articles/10.3389/fendo.2019.00348/full#supplementary-material
Abbreviations
CCI, continuous care intervention; UC, usual care; T2D, type 2 diabetes; HbA1c, hemoglobin A1c; CVD, cardiovascular disease; VLCD, very low calorie diet; BMI, body mass index; BHB, beta-hydroxybutryrate; BMD, bone mineral density; CAF, central abdominal fat; A/G, android:gynoid ratio; LELM, lower extremity lean mass; HDL, high density lipoprotein; LDL, low density lipoprotein; LDL-C, LDL cholesterol; LDL-P, LDL particle; ALT, alanine aminotransferase; AST, aspartate aminotransferase; ALP, alkaline phosphatase; NAFLD, nonalcoholic fatty liver disease; NLF, NAFLD liver fat score; NFS, NAFLD fibrosis score; TSH, thyroid stimulating hormone; BUN, blood urea nitrogen; eGFR, estimated glomerular filtration rate; hsCRP, high sensitive C-reactive protein; WBC, white blood cells; HOMA-IR, Homeostatic Model Assessment of Insulin Resistance; SGLT-2, sodium-glucose cotransporter-2 inhibitors; DPP-4, dipeptidyl peptidase-4 inhibitors; GLP-1, glucagon-like-peptide 1 receptor agonists; FFM, fat-free mass; VAT, visceral adipose tissue; GLM, generalized linear model; LMM, linear mixed-effect model; ADA, American Diabetes Association; CLIA, Clinical Laboratory Improvement Amendments; IRB, Institutional Review Board; DXA, dual-energy X-ray absorptiometry.
References
1. World Health Organization. Global Report on Diabetes. (2016). Available online at: http://www.who.int/iris/handle/10665/204871
2. Ng M, Fleming T, Robinson M, Thomson B, Graetz N, Margono C, et al. Global, regional, and national prevalence of overweight and obesity in children and adults during 1980-2013: a systemic analysis for the Global Burden of Disease Study 2013. Lancet. (2014) 384:766–81. doi: 10.1016/S0140-6736(14)60460-8
3. Centers for Disease Control and Prevention. National Diabetes Statistics Report, 2017. Atlanta, GA: Centers for Disease Control and Prevention, US. Dept of Health and Human Services (2017).
4. Karter AJ, Nundy S, Parker MM, Moffet HH, Huang ES. Incidence of remission in adults with type 2 diabetes: the diabetes & aging study. Diabetes Care. (2014) 37:3188–95. doi: 10.2337/dc14-0874
5. Gregg EW, Chen H, Wagenknecht LE, Clark JM, Delahanty LM, Bantle J, et al. Association of an intensive lifestyle intervention with remission of type 2 diabetes. JAMA. (2012) 308:2489–96. doi: 10.1001/jama.2012.67929
6. Bethel MA, Patel RA, Merrill P, Lokhnygina Y, Buse JB, Mentz RJ, et al. Cardiovascular outcomes with glucagon-like peptide-1 receptor agonists in patients with type 2 diabetes: a meta-analysis. Lancet Diabetes Endocrinol. (2018) 6:105–13. doi: 10.1016/S2213-8587(17)30412-6
7. Zinman B, Wanner C, Lachin JM, Loknygina Y, Buse JB, Mentz RJ, et al. Empagliflozin, cardiovascular outcomes, and mortality in type 2 diabetes. N Engl J Med. (2015) 373:2117–8. doi: 10.1056/NEJMoa1504720
8. Sjostrom L, Peltonen M, Jacobson P, Ahlin S, Andersson-Assarsson J, Anveden A, et al. Association of bariatric surgery with long-term remission of type 2 diabetes and with microvascular and macrovascular complications. JAMA. (2014) 311:2297–304. doi: 10.1001/jama.2014.5988
9. Lean MEJ, Leslie WS, Barnes AC, Brosnahan N, Thom G, McCombie L, et al. Primary care-led weight management for remission of type 2 diabetes (DiRECT): an open-label, cluster-randomised trial. The Lancet. (2018) 391:541–51. doi: 10.1016/S0140-6736(17)33102-1
10. Hallberg SJ, McKenzie AL, Williams PT, Bhanpuri NH, Peters AL, Campbell WW, et al. Effectiveness and safety of a novel care model for the management of type 2 diabetes at 1 year: an open-label, non-randomized, controlled study. Diabetes Ther. (2018) 9:583–612. doi: 10.1007/s13300-018-0373-9
11. Knop FK, Taylor R. Mechanism of metabolic advantages after bariatric surgery: its all gastrointestinal factors versus it's all food restriction. Diabetes Care. (2013) 36(Suppl. 2): S287–91. doi: 10.2337/dcS13-2032
12. Taylor R. Type 2 diabetes: etiology and reversibility. Diabetes Care. (2013) 36:1047–55. doi: 10.2337/dc12-1805
13. Dicker D, Yahalom R, Comaneshter DS, Comaneshter DS, Vinker S. Long-term outcomes of three types of bariatric surgery on obesity and type 2 diabetes control and remission. Obes Surg. (2016) 26:1814–20. doi: 10.1007/s11695-015-2025-8
14. Arterburn DE, Bogart A, Sherwood NE, Sidney S, Coleman KJ, Haneuse S, et al. A multisite study of long-term remission and relapse of type 2 diabetes mellitus following gastric bypass. Obes Surg. (2013) 23:93–102. doi: 10.1007/s11695-012-0802-1
15. Rubino F, Nathan DM, Eckel RH, Schauer PR, Alberti KG, Zimmet PZ, et al. Metabolic surgery in the treatment algorithm for type 2 diabetes: a joint statement by International Diabetes Organizations. Diabetes Care. (2016) 39:861–7. doi: 10.2337/dc16-0236
16. Hamdan K, Somers S, Chand M. Management of late postoperative complications of bariatric surgery. Br J Surgery. (2011) 98:1345–55. doi: 10.1002/bjs.7568
17. Berger ER, Huffman KM, Fraker T, Petrick AT, Brethauer SA, Hall BL, et al. Prevalence and risk factors for bariatric surgery readmissions: findings from 130,007 admissions in the metabolic and bariatric surgery accreditation and quality improvement program. Ann Surg. (2018) 67:122–31. doi: 10.1097/SLA.0000000000002079
18. Snel M, Jonker JT, Hammer S, Kerpershoek G, Lamb HJ, Meinders AE, et al. Long-term beneficial effect of a 16-week very low calorie diet on pericardial fat in obese type 2 diabetes mellitus patients. Obesity. (2012) 20:1572–6. doi: 10.1038/oby.2011.390
19. Goday A, Bellido D, Sajoux I, Crujeiras AB, Burguera B, Garcia-Luna PP, et al. Short-term safety, tolerability and efficacy of a very low- calorie-ketogenic diet interventional weight loss program versus hypocaloric diet in patients with type 2 diabetes mellitus. Nutr Diabetes. (2016) 6:e230. doi: 10.1038/nutd.2016.36
20. Wing RR, Blair E, Marcus M, Epstein LH, Harvey J. Year-long weight loss treatment for obese patients with type II diabetes: does including intermittent very-low calorie diet improve outcome? Am J Med. (1994) 97:354–62. doi: 10.1016/0002-9343(94)90302-6
21. Yamada Y, Uchida J, Izumi H, Tsukamoto Y, Inoue G, Watanabe Y, et al. A non-calorie-restricted low-carbohydrate diet is effective as an alternative therapy for patients with type 2 diabetes. Intern Med. (2014) 53:13–9. doi: 10.2169/internalmedicine.53.0861
22. Westman EC, Yancy WS, Mavropoulos JC, Marquart M, McDuffie JR. The effect of a low-carbohydrate, ketogenic diet versus a low-glycemic index diet on glycemic control in type 2 diabetes mellitus. Nutr Metab. (2008) 2008:5. doi: 10.1186/1743-7075-5-36
23. Esposito K, Maiorino MI, Petrizzo M, Bellastella G, Giugliano D. The effects of a mediterranean diet on the need for diabetes drugs and remission of newly diagnosed type 2 diabetes: follow-up of a randomized trial. Diabetes Care. (2014) 37:1824–30. doi: 10.2337/dc13-2899
24. Saslow LR, Daubenmier JJ, Moskowitz JT, Kim S, Murphy EJ, Phinney SD, et al. Twelve-month outcomes of a randomized trial of a moderate-carbohydrate versus very low-carbohydrate diet in overweight adults with type 2 diabetes mellitus or prediabetes. Nutr Diabetes. (2017) 2017:304. doi: 10.1038/s41387-017-0006-9
25. Kirk JK, Graves DE, Craven TE, Lipkin EW, Austin M, Margolis KL. Restricted-carbohydrate diets in patients with type 2 diabetes: a meta-analysis. J Am Diet Assoc. (2008) 108:91–100. doi: 10.1016/j.jada.2007.10.003
26. Wheeler ML, Dunbar SA, Jaacks LM, Karmally W, Mayer-Davis EJ, Wylie-Rosett J, et al. Macronutrients, food groups, and eating patterns in the management of diabetes: a systematic review of the literature, 2010. Diabetes Care. (2012) 35: 434–55. doi: 10.2337/dc11-2216
27. Davies MJ, D'Alessio DA, Fradkin J, Kernan WN, Mathieu C, Mingrone C, et al. Management of hyperglycemia in type 2 diabetes, 2018. A consensus report by the American Diabetes Association (ADA) and the European Association for the Study of Diabetes (EASD). Diabetes Care. (2018) 41:2669–701. doi: 10.2337/dci18-0033
28. American Diabetes Association (ADA). Standards of Medical Care in Diabetes-2019. Diabetes Care. (2019) 42(Suppl. 1). doi: 10.2337/dc19-Sdis01
29. Department of Veteran Affairs and Department of Defense. VA/DoD Clinical Practice Guideline for the Management of Type 2 Diabetes Mellitus in Primary Care. Version 5.0. (2017). doi: 10.131410/RG.2.214188.67209
30. Volek JS, Phinney SD, Forsythe CE, Quann EE, Wood RJ, Puglisi MJ, et al. Carbohydrate restriction has a more favorable impact on the metabolic syndrome than a low fat diet. Lipids. (2009) 44:297–309. doi: 10.1007/s11745-008-3274-2
31. Seshadri P, Iqbal N, Stern L, Williams M, Chicano KL, Daily DA, et al. A randomized study comparing the effects of a low-carbohydrate diet and a conventional diet on lipoprotein subfractions and C-reactive protein levels in patients with severe obesity. Am J Med. (2004) 117:398–405. doi: 10.1016/j.amjmed.2004.04.009
32. Volek JS, Sharman MJ, Gomez AL, DiPasquale C, Roti M, Pumerantz A, et al. Comparison of a very low-carbohydrate and low-fat diet on fasting lipids, LDL subclasses, insulin resistance, and postprandial lipemic responses in overweight women. J Am Coll Nutr. (2004) 23:177–84. doi: 10.1080/07315724.2004.10719359
33. Bhanpuri NH, Hallberg SJ, Williams PT, McKenzie AL, Ballard KD, Campbell WW, et al. Cardiovascular disease risk factor responses to a type 2 diabetes care model including nutritional ketosis induced by sustained carbohydrate restriction at 1 year: an open label, non-randomized, controlled study. Cardiovasc Diabetol. (2018) 17:56. doi: 10.1186/s12933-018-0698-8
34. International Diabetes Federation (IDF). The IDF consensus worldwide definition of the metabolic syndrome. IDF Commun. (2006) 2006:1–24. Available online at: https://www.idf.org/e-library/consensus-statements/60-idfconsensusworldwide-definitionof-the-metabolic-syndrome
35. Huang PL. A comprehensive definition for metabolic syndrome. Dis Model Mech. (2009) 2:231–7. doi: 10.1242/dmm.001180
36. Brownbill RA, Ilich JZ. Measuring body composition in overweight individuals by dual energy x-ray absorptiometry. BMC Med Imaging. (2005) 5:1. doi: 10.1186/1471-2342/5/I.
37. Rothney MP, Brychta RJ, Schaefer EV, Chen KY, Skarulis MC. Body composition measured by dual-energy X-ray absorptiometry half-body scans in obese adults. Obesity. (2009) 17:1281–6. doi: 10.1038/oby.2009.14
38. Chun KJ. Bone densitometry. Semin Nucl Med. (2011) 41:220–8. doi: 10.1053/j.semnuclmed.2010.12.002
39. Kamel EG, McNeill G, Van Wijk CWV. Usefulness of anthropometry and DXA in predicting intra-abdominal fat in obese men and women. Obes Res. (2000) 8:36–42. doi: 10.1038/oby.2000.6
40. Reid KF, Naumova EN, Carabello RJ, Phillips EM, Fielding RA. Lower extremity muscle mass predicts functional performance in mobility-limited elders. J Nutr Health Aging. (2008) 12:493–8. doi: 10.1007/BF02982711
41. Moon JJ, Park SG, Ryu SM, Park CH. New skeletal muscle mass index in diagnosis of sacropenia. J Bone Metab. (2018) 25:15–21. doi: 10.11005/jbm.2018.25.1.15
42. Kline RB. Principles and Practice of Structural Equation Modeling. 3rd ed. New York: The Guilford Press (2011).
43. John JA, Draper NR. An alternative family of transformations. Appl Statist. (1980) 29:190–7. doi: 10.2307/2986305
44. Buse JB, Caprio S, Cefalu WT, Ceriello A, Del Prato S, Inzucchi SE, et al. How do we define cure of diabetes? ADA consensus statement. Diabetes Care. (2009) 32:2133–5. doi: 10.2337/dc09-9036
45. Kotronen A, Peltonen M, Hakkarainen A, Sevastianova K, Bergholm R, Johansson LM, et al. Prediction of non-alcoholic fatty liver disease and liver fat using metabolic and genetic factors. Gastroenterology. (2009) 137:865–72. doi: 10.1053/j.gastro.2009.06.005
46. Angulo P, Hui JM, Marchesini G, Bugianesi E, George J, Farrell GC, et al. The NAFLD fibrosis score: a noninvasive system that identifies liver fibrosis in patients with NAFLD. Hepatology. (2007) 45:846–54. doi: 10.1002/hep.21496
47. Graham JW, Olchowski AE, Gilreath TD. How many imputations are really needed? Some practical clarifications of multiple imputation theory. Prevention Sci. (2007) 8:206–13. doi: 10.1007/s11121-007-0070-9
48. McKenzie A, Hallberg S, Creighton BC, Volk BM, Link TM, Abner MK, et al. A novel intervention including nutritional recommendations reduces hemoglobin A1c level, medication use, and weight in type 2 diabetes. JMIR Diabetes. (2017) 2:e5. doi: 10.2196/diabetes.6981
49. White WB, Cannon CP, Heller CR Nissen SE, Bergenstal RM, Bakris GL, et al. Alogliptin after acute coronary syndrome in patients with type 2 diabetes. N Engl J Med. (2013) 369:1327–35. doi: 10.1056/NEJMoa1305889
50. Action to Control Cardiovascular Risk in Diabetes Study Group, Gerstein HC, Miller ME, Byington RP, Goff DC Jr, Bigger JT, et al. Effects of intensive glucose lowering in type 2 diabetes. N Engl J Med. (2008) 358: 2545–59. doi: 10.1056/NEJMoa0802743
51. Henry RR, Gumbiner B, Ditzler T, Wallace P, Lyon R, Glauber HS. Intensive conventional insulin therapy for type II diabetes. Metabolic effects during a 6-mo outpatient trial. Diabetes Care. (1993) 16:21–31. doi: 10.2337/diacare.16.1.21
52. Shai I, Schwarzfuchs D, Henkin Y, Shahar DR, Witkow S, Greenberg I, et al. Weight loss with a low-carbohydrate, mediterranean, or low-fat diet. N Engl J Med. (2008) 359:229–41. doi: 10.1056/NEJMoa0708681
53. Iqbal N, Vetter ML, Moore RH, Chittams JL, Dalton-Bakes CV, Dowd M, et al. Effects of a low-intensity intervention that prescribed a low-carbohydrate vs. a low fat diet in obese, diabetic participants. Obesity. (2010) 18:1733–8. doi: 10.1038/oby.2009.460
54. Guldbrand H, Dizdar B, Bunjaku B, Lindstrom T, Bachrach-Lindstrom M, Fredrikson M, et al. In type 2 diabetes, randomisation to advice to follow a low-carbohydrate diet transiently improves glycaemic control compared with advice to follow a low-fat diet producing a similar weight loss. Diabetologia. (2012) 55:2118–27. doi: 10.1007/s00125-012-2567-4
55. Nielsen JV, Joensson EA. Low carbohydrate diet in type 2 diabetes: stable improvement of bodyweight and glycemic control during 44 months follow-up. Nutr Metab. (2008) 5:14. doi: 10.1186/1743-7075-5-14
56. Haimoto H, Iwata M, Wakai K, Umegaki H. Long-term effects of a diet loosely restricting carbohydrates on HbA1c levels, BMI and tapering of sulfonylureas in type 2 diabetes: a 2-year follow-up study. Diab Res Clin Prac. (2008) 79:350–6. doi: 10.1016/j.diabres.2007.09.009
57. Tay J, Thompson CH, Luscombe-Marsh ND, Wycherley TP, Noakes M, Buckley JD, et al. Effects of an energy-restricted low-carbohydrate, high unsaturated fat/low saturated fat diet versus a high-carbohydrate, low-fat diet in type 2 diabetes: a 2-year randomized clinical trial. Diabetes Obes Metab. (2018) 20:858–71. doi: 10.1111/dom.13164
58. Steinberg DM, Tate DF, Bennett GG, Ennett S, Samuel-Hodge V, Ward DS. The efficacy of a daily self-weighing weight loss intervention using smart scales and email. Obesity. (2013) 21:1789–97. doi: 10.1002/oby.20396
59. Xiang AH, Trigo E, Martinez M, Katkhouda N, Beale E, Wang X, et al. Impact of gastric banding versus metformin on β-cell function in adults with impaired glucose tolerance or mild type 2 diabetes. Diabetes Care. (2018) 41:2544–51. doi: 10.2337/dc18-1662
60. Sniderman AD, Toth PP, Thanassoulis G, Furberg CD. An evidence-based analysis of the National Lipid Association recommendations concerning non-HDL-C and apoB. J Clin Lipidol. (2016) 10:248–58. doi: 10.1016/j.jacl.2016.07.008
61. Verges B. Lipid modification in type 2 diabetes: the role of LDL and HDL. Fundam Clin Pharmacol. (2009) 23:681–5. doi: 10.1111/j.1472-8206.2009.00739.x
62. Welthy FK. How do elevated triglycerides and low HDL-cholesterol affect inflammation and atherothrombosis? Curr Cardiol Rep. (2013) 15:400. doi: 10.1007/s11886-013-0400-4
63. Lu W, Resnick HE, Jablonski KA, Jones KL, Howard WJ, Robbins DC, et al. Non-HDL cholesterol as a predictor of cardiovascular disease in type 2 diabetes: the strong heart disease. Diabetes Care. (2003) 26:16–23. doi: 10.2337/diacare.26.1.16
64. Hirano T. Pathophysiology of diabetic dyslipidemia. J Atheroscler Thromb. (2018) 25:771–85. doi: 10.5551/jat.RV17023
65. Creighton BC, Hyde PN, Maresh CM, Kraemer WJ, Phinney SD, Volek JS. Paradox of hypercholesterolaemia in highly trained, keto-adapted athletes. BMJ Open Sport Exerc Med. (2008) 4:e000429. doi: 10.1136/bmjsem-2018-000429
66. Gomez-Arbelaez D, Bellido D, Castro AI, Ordonez- Mayan L, Carreira J, Galban C, et al. Body composition changes after very low-calorie-ketogenic diet in obesity evaluated by three standardized methods. J Clin Endocrinol Metab. (2017) 102:488–98. doi: 10.1210/jc.2016-2385
67. Moreno B, Crujeiras AB, Bellido D, Sajoux I, Casanueva FF. Obesity treatment by very low-calorie-ketogenic diet at two years: reduction in visceral fat and on the burden of disease. Endocrine. (2016) 54:681–90. doi: 10.1007/s12020-016-1050-2
68. Gross BA, Goss AM. A lower-carbohydrate, higher-fat diet reduces abdominal and intermuscular fat and increases insulin sensitivity in adults at risk of type 2 diabetes. J Nutr. (2015) 145:177S−83S. doi: 10.3945/jn.114.195065
69. Levelt E, Pavlides M, Banerjee R, Mahmod M, Kelly C, Sellwood J, et al. Ectopic and visceral fat deposition in lean and obese patients with type 2 diabetes. J Am Coll Cardiol. (2016) 68:53–63. doi: 10.1016/j.jacc.2016.03.597
70. Shah RV, Murthy VL, Abbasi SA, Blankstein R, Kwong RY, Goldfine AB, et al. Visceral adiposity and the risk of metabolic syndrome across of body mass index: the MESA Study. JACC Cardiovasc Imaging. (2014) 7:1221–35. doi: 10.1016/j.jcmg.2014.07.017
71. Mirza MS. Obesity, visceral fat, and NAFLD: querying the role of adipokines in the progression of nonalcoholic fatty liver disease. ISRN Gastroenterol. (2011) 2011:592404. doi: 10.5402/2011/592404
72. Vilar-Gomez E, Athinarayanan SJ, Adams RN, Hallberg SJ, Bhanpuri NH, McKenzie AL, et al. Post-hoc analyses of surrogate markers of non-alcoholic fatty liver disease (NAFLD) and liver fibrosis in patients with type 2 diabetes in a digitally-supported continuous care intervention: an open-label, non-randomized, controlled study. BMJ Open. (2019) 9:e023597. doi: 10.1136/bmjopen-2018-023597
73. Bouchi R, Nakano Y, Fukuda T, Takeuchi T, Murakami M, Minami I, et al. Reduction of visceral fat by liraglutide is associated with ameliorations of hepatic steatosis, albuminuria, and micro-inflammation in type 2 diabetic patients with insulin treatment: a randomized control trial. Endocr J. (2017) 64: 269–81. doi: 10.1507/endocrj.EJ16-0449
74. Gabriely I, Ma XH, Yang XM, Atzmon G, Rajala MW, Berg AH, et al. Removal of visceral fat prevents insulin resistance and glucose intolerance of aging: an adipokine-mediated process? Diabetes. (2002) 51:2951–8. doi: 10.2337/diabetes.51.10.2951
75. Garcia-Ruiz I, Solis-Munoz P, Fernandez-Moreira D, Grau M, Munoz-Yague MT, Solis-Herruzo JA. Omentectomy prevents metabolic syndrome by reducing appetite and body weight in a diet induced obesity rat model. Sci Rep. (2018) 8:1540. doi: 10.1038/s41598-018-19973-z
76. Bril F, Cusi K. Management of nonalcoholic fatty liver disease in patients with type 2 diabetes: a call to action. Diabetes Care. (2017) 40:419–30. doi: 10.2337/dc16-1787
77. Verrijen A, Francque S, Van Gaal L. The role of visceral adipose tissue in the pathogenesis of non-alcoholic fatty liver disease. Eur Endocrinol. (2011) 7:96–103. doi: 10.17925/EE.2011.07.02.96
78. Bielohuby M, Matsuura M, Herbach N, Kienzle E, Slawik M, Hoeflich A, et al. Short-term exposure to low-carbohydrate, high-fat diets induces low bone mineral density and reduces bone formation in rats. J Bone Miner Res. (2010) 25:275–84. doi: 10.1359/jbmr.090813
79. Wu X, Huang Z, Wang X. Fu Z, Liu J, Huang Z, et al. Ketogenic diet compromises both cancellous and corticol bone mass in mice. Calcif Tissue Int. (2017) 101:412–21. doi: 10.1007/s00223-017-0292-1
80. Simm PJ, Bicknell-Royle J, Lawrie J, Nation J, Draffin K, Stewart KG, et al. The effect of the ketogenic diet on the developing skeleton. Epilepsy Res. (2017) 136: 62–6. doi: 10.1016/j.eplepsyres.2017.07.014
81. Willi SM, Oexmann MJ, Wright NM, Collop NA, Key LL Jr. The effects of a high-protein, low-fat, ketogenic diet on adolescents with morbid obesity: body composition, blood chemistries and sleep abnormalities. Pediatric. (1998) 101:61–7. doi: 10.1542/peds.101.1.61
82. Moreno B, Bellido D, Sajoux I, Goday A, Saavdra D, Crujeiras AB, et al. Comparison of a very low-calorie-ketogenic diet with a standard low-calorie diet in the treatment of obesity. Endocrine. (2014) 47:793–805. doi: 10.1007/s12020-014-0192-3
83. Bertoli S, Trentani C, Ferraris C, De Giorgis V, Veggiotti P, Tagliabue A. Long-term effects of a ketogenic diet on body composition and bone mineralization in GLUT-1 deficiency syndrome: a case series. Nutrition. (2014) 30: 726–8. doi: 10.1016/j.nut.2014.01.005
84. Stagi S, Cavalli L, Iurato C, Seminara S, Brandi M, de Martino M. Bone metabolism in children and adolescents: main characteristics of the determinants of peak bone mass. Clin Cases Miner Bone Metab. (2013) 10:172–9.
85. Choi SJ, Files DC, Zhang T, Wang ZM, Messi ML, Gregory H, et al. Intramyocellular lipid and impaired myofiber contraction in normal weight and obese older adults. J Gerontol A Biol Sci Med Sci. (2016) 71:557–64. doi: 10.1093/gerona/glv169
86. Mingrone G, Marino S, DeGaetano A, Capristo E, Heymsfield SB, Gasbarrini G, et al. Different limit to the body's ability of increasing fat-free mass. Metabolism. (2001) 50:1004–7. doi: 10.1053/meta.2001.25650
88. Bopp MJ, Houston DK, Lenchik L, Easter L, Kritchevsky SB, Nicklas BJ. Lean mass loss is associated with low protein intake during dietary-induced weight loss in postmenopausal women. J Am Diet Assoc. (2008) 108:1216–20. doi: 10.1016/j.jada.2008.04.017
89. Ciangura C, Bouillot JL, Lloret-Linares C, Poitou C, Veyrie N, Basdevant A, et al. Dynamics of change in total and regional body composition after gastric bypass in obese patients. Obesity. (2010) 18: 760–65. doi: 10.1038/oby.2009.348
90. Varma S, Brown T, Clark J, Maruthur N, Schweitzer M, Magnuson T, et al. Comparative effects of medical vs. surgical weight loss on body composition in a randomized trial. Diabetes. (2018) 67:S1. doi: 10.2337/db18-2460-PUB
91. Zalesin KC, Franklin BA, Lillystone MA, Shamoun T, Krause KR, Chengelis DL, et al. Differential loss of fat and lean mass in the morbidly obese after bariatric surgery. Met Syndrome Related Dis. (2010) 8:15–20. doi: 10.1089/met.2009.0012
92. Redmon JB, Reck KP, Raatz SK, Swanson JE, Kwong CA, Ji H, et al. Two year outcome of a combination of weight loss therapies for type 2 diabetes. Diabetes Care. (2005) 28:1311–5. doi: 10.2337/diacare.28.6.1311
93. Heymsfield SB, Gonzalez MCC, Shen W, Redman L, Thomas D. Weight loss composition is one-fourth fat-free mass: a critical review and critique of this widely cited rule. Obes Rev. (2014) 15:310–21. doi: 10.1111/obr.12143
94. Davis PG, Phinney SD. Differential effects of two very low calorie diets on aerobic and anaerobic performance. Int J Obes. (1990) 14:779–87.
95. Kim JE, O'Connor LE, Sands LP, Slebodnik MB, Campbell WW. Effects of dietary protein intake on body composition changes after weight loss in older adults: a systematic review and meta-analysis. Nutr Rev. (2016) 74:210–24. doi: 10.1093/nutrit/nuv065
96. Frigolet ME, Ramos Barragan VE, Tamez Gonzalez M. Low-carbohydrate diets: a matter of love or hate. Ann Nutr Metab. (2011) 28: 320–34. doi: 10.1159/000331994
Keywords: type 2 diabetes, nutritional ketosis, HbA1c, body composition, reversal and remission
Citation: Athinarayanan SJ, Adams RN, Hallberg SJ, McKenzie AL, Bhanpuri NH, Campbell WW, Volek JS, Phinney SD and McCarter JP (2019) Long-Term Effects of a Novel Continuous Remote Care Intervention Including Nutritional Ketosis for the Management of Type 2 Diabetes: A 2-Year Non-randomized Clinical Trial. Front. Endocrinol. 10:348. doi: 10.3389/fendo.2019.00348
Received: 04 February 2019; Accepted: 14 May 2019;
Published: 05 June 2019.
Edited by:
Undurti Narasimha Das, UND Life Sciences LLC, United StatesReviewed by:
Joseph Aloi, Wake Forest Baptist Medical Center, United StatesDilek Gogas Yavuz, Marmara University, Turkey
Copyright © 2019 Athinarayanan, Adams, Hallberg, McKenzie, Bhanpuri, Campbell, Volek, Phinney and McCarter. This is an open-access article distributed under the terms of the Creative Commons Attribution License (CC BY). The use, distribution or reproduction in other forums is permitted, provided the original author(s) and the copyright owner(s) are credited and that the original publication in this journal is cited, in accordance with accepted academic practice. No use, distribution or reproduction is permitted which does not comply with these terms.
*Correspondence: James P. McCarter, amFtZXNwbWNjYXJ0ZXJAZ21haWwuY29t