- Department of Neurosurgery, Yale University School of Medicine, New Haven, CT, United States
Gonadotropin-releasing hormone (GnRH) neuron activity and GnRH secretion are essential for fertility in mammals. Here, I review findings from mouse studies on the direct modulation of GnRH neuron activity and GnRH secretion by non-peptide neurotransmitters (GABA, glutamate, dopamine, serotonin, norepinephrine, epinephrine, histamine, ATP, adenosine, and acetylcholine), gasotransmitters (nitric oxide and carbon monoxide), and gliotransmitters (prostaglandin E2 and possibly GABA, glutamate, and ATP). These neurotransmitters, gasotransmitters, and gliotransmitters have been shown to directly modulate activity and/or GnRH secretion in GnRH neurons in vivo or ex vivo (brain slices), from postnatal through adult mice, or in embryonic or immortalized mouse GnRH neurons. However, except for GABA, nitric oxide, and prostaglandin E2, which appear to be essential for normal GnRH neuron activity, GnRH secretion, and fertility in males and/or females, the biological significance of their direct modulation of GnRH neuron activity and/or GnRH secretion in the central regulation of reproduction remains largely unknown and requires further exploration.
Introduction
Gonadotropin-releasing hormone (GnRH) neurons, whose cell bodies (in rodents) reside mainly in the preoptic area (POA) of the hypothalamus as well as in the medial septum (MS) and diagonal band of Broca (DBB), provide the final output in the central regulation of mammalian fertility: pulsatile and, in females, surge GnRH secretion from axon terminals in the median eminence (ME). This is despite the fact that subpopulations of kisspeptin (KP) neurons in the arcuate nucleus (ARC) and rostral periventricular area of the third ventricle (RP3V), which innervate GnRH neurons, appear to be the “GnRH pulse generator” and “GnRH surge generator,” respectively, and together with KP neurons in the posterodorsal medial amygdala (MePD) integrate endocrine, metabolic, and environmental (including circadian, stress, pheromonal, and sexual behavior-related) signals in the hypothalamic-pituitary-gonadal axis (1–11). These signals are conveyed to GnRH neurons by KP neurons, other neurons, and glial cells that release one or more peptide neurotransmitters (including KP), non-peptide neurotransmitters (also called conventional, classical, or small-molecule neurotransmitters), gasotransmitters, and/or gliotransmitters onto GnRH neuron cell bodies, dendrites, or “dendrons” to modulate GnRH neuron activity (considered here to be changes in membrane potential, action potential firing, and/or cytoplasmic, free Ca2+ concentration ([Ca2+]i)) and GnRH secretion (12–28). The dendrons are processes (blended dendrites and axons), found so far only in GnRH neurons, that receive synaptic input like dendrites but also conduct action potentials like axons, and that project from GnRH cell bodies to the ME where they split into short axon terminals to enable GnRH secretion into the portal vasculature (21, 29). GnRH neurons receive their highest density of synaptic innervation along their proximal dendrons (up to ~500 μm from the cell body), which contain the action potential initiation site (30, 31). At their distal dendrons (~500 μm from the cell body), GnRH neurons appear to be interconnected through dendro-dendritic bundling and associated shared inputs from ARC KP neurons and possibly other neurons and glial cells, which may provide a mechanism for the synchronization of GnRH neuron activity necessary for pulsatile GnRH secretion, as well as an additional mechanism for its direct modulation (4, 32–36).
GnRH secreted from GnRH neuron axon terminals in the ME into the hypothalamo-hypophyseal portal circulation binds to GnRH receptors on pituitary gonadotrophs and stimulates the synthesis and secretion into the systemic circulation of luteinizing hormone (LH) and follicle-stimulating hormone (FSH). LH and FSH are required for gametogenesis and gonadal biosynthesis in both sexes, for secretion of estradiol (E, a.k.a. E2) and progesterone (P), as well as for ovulation, in females, and for secretion of testosterone (T) in males (37). E, P, and T feed back onto the brain to inhibit GnRH secretion (via inhibition of ARC KP or other neurons), and onto the anterior pituitary to inhibit LH/FSH secretion, except in the afternoon on the day of proestrus in females, when E and P stimulate a surge of GnRH secretion [via activation of RP3V KP neurons (6)]. The switch from E negative feedback to E positive feedback at proestrus is triggered by circadian input from vasopressin neurons in the suprachiasmatic nucleus (SCN) to RP3V KP neurons and GnRH neurons, and by increased levels of E. This switch, combined with E-mediated de novo synthesis of P within the hypothalamus, initiates the GnRH surge, which in turn triggers the LH surge that results in ovulation (6). The GnRH/LH surge is associated with increased activation of RP3V KP neurons, which (unlike ARC KP neurons) project to the soma and proximal dendrites of a subpopulation of GnRH neurons, along with increased GnRH neuron somatic and dendritic spine density, and critically depends on KP receptor signaling in GnRH neurons (1, 3, 33, 38). This suggests that an increase in excitatory synaptic input from RP3V KP neurons to a subpopulation of GnRH neurons is required for the GnRH/LH surge; however, as discussed below, the GnRH/LH surge may also depend on excitatory input from other cells (28, 39).
Owing to the relative ease with which mouse GnRH neurons can be genetically manipulated, electrophysiologically assayed, and imaged, mice represent the most tractable system for studying mammalian GnRH neurons. I recently reviewed the direct modulation of GnRH neuron activity and GnRH secretion in mice by neuropeptides, with direct modulation considered to be modulation that persists in the presence of the Na+ channel blocker tetrodotoxin (TTX) and amino acid transmitter receptor antagonists (40). Here, I review the direct modulation by non-peptide neurotransmitters, gasotransmitters, and gliotransmitters, including their cognate receptors and signaling mechanisms, of GnRH neuron activity and GnRH secretion in mice. The review is based on data obtained from green fluorescent protein (GFP)- or genetically encoded Ca2+ indicator (GECI)-expressing GnRH neurons in vivo or ex vivo (brain slices), from postnatal through adult mice, when data are available, or from alternatives such as embryonic mouse GnRH neurons or immortalized cell lines of mouse GnRH neurons (19, 25, 41–49).
Modulation of GnRH Neuron Activity and Secretion by Non-Peptide Neurotransmitters
Non-peptide neurotransmitters are defined here as endogenous chemicals (other than neuropeptides) that transmit signals across a synapse from presynaptic neurons, where they are released from secretory vesicles via Ca2+-dependent exocytosis, to postsynaptic neurons (e.g., GnRH neurons), where they bind to and activate ionotropic and/or metabotropic receptors. Activation of ionotropic receptors results in the opening of ion channels through which one or more types of ions such as Na+, K+, Cl−, and Ca2+ flow, whereas activation of metabotropic receptors results in second messenger activation or inhibition of ion channels via signal transduction mechanisms that often involve G proteins. This, in turn, results in a change, or modulation, of the activity (membrane potential and/or [Ca2+]i) and output (neurotransmitter or neuropeptide release) of the postsynaptic neurons.
Amino Acids
γ-aminobutyric Acid (GABA)
GABA released from non-GnRH neurons, and potentially from a subpopulation of GnRH neurons (50), binds to GABAA receptors (GABAARs), which are GABA-gated Cl− channels, and to GABAB receptors (GABABRs), which are G protein-coupled receptors (GPCRs) linked to K+ channels, in GnRH neurons. This results in modulation of GnRH neuron activity and GnRH/LH secretion, with net GABA effects determined by the ratio of GABAAR and GABABR-mediated effects (19, 26, 50–55).
Activation of GABAARs in GnRH neurons of pubertal and adult mice has complex effects on GnRH neurons but mainly depolarizes and excites them (i.e., increases their action potential firing frequency). Exogenous GABA, the GABAAR agonist muscimol, and the GABAAR antagonist bicuculline increase, decrease, or have mixed effects on action potential firing frequency that may depend on GnRH neuron resting membrane potential and/or intracellular Cl− concentration, which may in turn depend on ongoing GABAAR signaling by endogenous GABA (19, 56–58). However, the GABAAR antagonist picrotoxin, which prevents activation of GABAARs by endogenous GABA, consistently suppresses GnRH neuron firing (19, 59), and GABA increases [Ca2+]i in GnRH neurons (60, 61), suggesting that GABA predominantly depolarizes, or depolarizes and excites, GnRH neurons. GABA increases [Ca2+]i by first opening GABAAR channels, which results in depolarization followed by Ca2+ entry through voltage-gated Ca2+ channels (61, 62). Additional evidence for GABAergic excitation of adult GnRH neurons via GABAAR activation is that most adult GnRH neurons express the Cl− accumulator transporter NKCC1 and anion exchanger AE2, which contribute to depolarization after GABAAR activation, but not the primary Cl− extruder transporter KCC2, which contributes to hyperpolarization after GABAAR activation (63, 64).
Adult GnRH neurons appear to express α1, α2, α3, α5, β1, β2, β3, γ1, γ2, δ, ε, and ρ1 GABAAR subunits, with some differences between males and females (65–70). Spontaneous release of endogenous GABA onto GnRH neurons evokes phasic postsynaptic currents mediated by γ2 subunit-containing GABAARs as well as tonic extrasynaptic currents mediated by γ2 subunit- and δ subunit-containing GABAARs (52, 71). The amplitude and frequency of GABAergic postsynaptic currents (PSCs) are reduced by 70 and 77%, respectively, and the response to exogenous GABA is reduced by 90%, in GnRH neurons of mice in which the γ2 subunit is knocked out specifically in GnRH neurons (71). Taken together with the finding that the δ subunit appears to be expressed in only 44% of GnRH neurons (52), this indicates that the γ2 subunit is critical for normal GABAAR function in GnRH neurons. Although male and female GnRH neuron γ2 knockout mice exhibit normal fecundity, estrous cycles, and puberty onset, suggesting that GABAAR-mediated neurotransmission at the GnRH neuron is not essential for GnRH secretion or fertility, it is also possible that other GABAAR subunits, other transmitters, or an intrinsic conductance may have compensated for the loss of the γ2 subunit in those mice (71). A 4,5,6,7-tetrahydroisoxazolo[5,4-c]pyridin-3-ol (THIP)-sensitive tonic GABAergic current modulates GnRH neuron activity, suggesting a role for δ subunit-containing GABAARs in GnRH neurons, which confer neurosteroid sensitivity, as well as a potential role for stress-derived neurosteroid modulation in the regulation of fertility [(52), reviewed by (72)]. GABAARs are expressed in the cell bodies, dendrites, and dendrons of GnRH neurons (21, 44, 62).
GABA modulates GnRH neuron activity through GABABRs in addition to GABAARs. Exogenous GABA (in the presence of the GABAAR antagonist picrotoxin) and the GABABR agonist baclofen directly hyperpolarize GnRH neurons, by inducing a Ba2+-sensitive outward K+ current, and decrease GnRH neuron firing (51, 53, 54). Endogenous GABA inhibits GnRH neurons via GABABRs following excitation mediated by GABAARs or KP receptors (73). GABABRs in GnRH neurons are composed of GABAB1 and GABAB2 subunits as in other neurons and are expressed in 22% of GnRH neurons in male mice and 70% of GnRH neurons in female mice, with expression remaining relatively constant across the estrous cycle (51, 53, 69). Female GABAB1 knockout mice exhibit disrupted estrous cyclicity and reduced fertility, as well as increased expression levels of genes including Gad1 (which codes for glutamate decarboxylase, the enzyme that catalyzes the production of GABA from glutamate), Kiss1 (which codes for KP), and Gnrh1 (which codes for GnRH) that may affect the sexual differentiation of the brain and the proper wiring of the GnRH and KP systems (74, 75). However, the effects on GnRH neuron activity, GnRH secretion, or fertility of knocking out GABAB1 and/or GABAB2 subunits specifically in GnRH neurons have yet to be reported.
GABAergic wiring and transmission onto GnRH neurons appear to be important for fertility. GnRH neuron cell bodies and dendrites receive inputs from GABAergic neurons in the ARC and RP3V (26, 35, 76). GnRH neurons in the POA of prenatally androgenized (PNA) female mice receive an increased number of appositions and increased GABAergic transmission from GABAergic neurons in the ARC (35, 77). This results in increased GABAergic PSC amplitude and frequency, increased firing, persistently elevated GnRH/LH release frequency, and reduced progesterone (P) negative feedback prior to androgen (T) excess and reproductive impairments (including disrupted reproductive cycles) that mimic those of polycystic ovary syndrome (PCOS), the most common endocrinopathy in women of reproductive age and the leading cause of female infertility (35, 59, 77–80). This suggests that normal GABAergic wiring and transmission onto GnRH neurons is essential for normal GnRH neuron firing, GnRH/LH secretion, and reproductive function in females, and that increased GABAergic wiring and transmission onto GnRH neurons may play an important role in PCOS. However, it should be emphasized that, while PCOS is associated with increased cerebrospinal fluid concentrations of GABA, PCOS has a complex etiology which includes genetic, environmental, and metabolic factors along with neuroendocrine factors (78, 81).
GABA also appears to be important for activating the GnRH neurons that trigger ovulation. Low-frequency (2 Hz) in vitro optogenetic stimulation of GABAergic neurons in the RP3V, which co-express KP (82), generates an immediate and transient GABAAR-mediated increase in GnRH neuron firing, whereas higher frequencies (10 Hz) recruit the long-lasting activation observed following RP3V KP neuron stimulation (26). However, 2-Hz activation of RP3V GABAergic neurons in vivo does not alter LH secretion, whereas 10-Hz stimulation evokes a sustained large increase in LH secretion identical to RP3V KP neuron activation, suggesting that KP, rather than GABA, is the functionally dominant co-transmitter at the time of ovulation (26). Yet, KP may exert some of its effects on GnRH neurons via GABA. GABAergic PSC (and miniature PSC; mPSC) frequency in GnRH neurons increases (along with GnRH neuron firing rate and burst frequency) during the afternoon of proestrus in normally cycling female mice (83, 84), and KP increases the amplitude and frequency of GABAergic PSCs in GnRH neurons from ovariectomized mice treated with E (85), whose serum level rises during the afternoon of proestrus. E-dependent GABAergic transmission may be important for reproduction in females, as female mice lacking estrogen receptor α (ERα, also called ESR1) in their GABAergic neurons are infertile and have abnormal estrous cycles and abolished E positive feedback responsible for the proestrus GnRH/LH surge required for ovulation (39). In addition to acting indirectly on GnRH neurons via ERα expressed in GABAergic neurons (60), E acts directly on GnRH neurons via the estrogen receptor β (ERβ, also called ESR2)/Akt/neuronal nitric oxide synthase (nNOS) pathway to generate nitric oxide synthase (NOS) that retrogradely accelerates GABA and glutamate release from presynaptic terminals contacting GnRH neurons, which increases mPSC frequency and firing rate and thus may also contribute to the proestrus GnRH/LH surge (86).
GABA may also mediate the effects of leptin, an important regulator of food intake and energy expenditure, on GnRH neurons, which do not express leptin receptors. Mice lacking leptin receptors in their GABAergic neurons exhibit delayed puberty onset and decreased fertility in both sexes, as well as a suppressed proestrus GnRH/LH surge (87). Leptin-responsive GABAergic neurons in the ARC project to the POA (88), but it has not yet been reported whether they project to GnRH neurons in the POA and affect GnRH neuron activity or secretion.
Glutamate (Glu)
Glu increases GnRH neuron activity via α-amino-3-hydroxy-5-methyl-4-isoxazolepropionic acid (AMPA)/kainate (KA), N-methyl-D-aspartate (NMDA), and metabotropic glutamate (mGlu) receptors (AMPARs, NMDARs, and mGluRs) expressed in the cell bodies, dendrites, and dendrons of GnRH neurons (21, 44, 62, 89, 90). Glu may be released onto GnRH neurons from KP neurons in the ARC as well as from other neurons, possibly including GnRH neurons, since they express vesicular glutamate transporter 2 [vGluT2, (55, 70, 76, 82, 90, 91)].
AMPA increases GnRH neuron firing (19, 54), as well as Ca2+ entry through AMPARs, which are non-selective cation channels (61). GnRH neurons appear to express GluA1, GluA2, GluA3, and GluA4 AMPAR subunits (67, 69, 70).
NMDA increases [Ca2+]i in only about 20% of GnRH neurons (61), probably via Ca2+ entry through NMDAR channels, which are non-selective cation channels with higher Ca2+ permeability than AMPARs, as well as via membrane depolarization followed by Ca2+ entry through L-type Ca2+ channels, and subsequently via Ca2+-induced Ca2+ release involving internal Ca2+ stores and IP3 receptors. GnRH neurons appear to express GluN1, GluN2B, GluN2D, and GluN3A NMDAR subunits (44, 67, 69, 70, 92).
The mGluR1/mGluR5 agonist (S)-3,5-dihydroxyphenylglycine (DHPG) increases firing in a subpopulation of GnRH neurons (45% in whole-cell recordings and 23% in cell-attached recordings) in the MS/DBB (90) but not in the POA (93). The DHPG-induced increase in GnRH neuron firing in the MS/DBB may occur via Gq protein stimulation of phospholipase C (PLC) resulting in the generation of inositol 1,4,5-trisphosphate (IP3) and diacylglycerol (DAG), followed by DAG activation of transient receptor potential-canonical (TRPC) channels, which are non-selective cation channels. GnRH neurons appear to express mGluR1, mGluR5, and mGluR8 mGluRs (67, 70).
Glu may play an important role in the modulation of GnRH neuron activity and secretion required for fertility. Both the decrease in GnRH neuron firing during E negative feedback and the increase in GnRH neuron firing during E positive feedback depend on Glu neurotransmission (15). Moreover, female mice lacking ERα in their glutamatergic neurons, which include most ARC KP neurons but only a small percentage of RP3V KP neurons, exhibit advanced puberty onset and abnormal negative feedback, are infertile, and have abolished E positive feedback responsible for the proestrus GnRH/LH surge (39). Glu neurotransmission onto GnRH neurons, at least through GluA2-containing AMPARs or all NMDARs, does not appear to be essential for normal GnRH or fertility, as shown using mice lacking GluA2-containing AMPARs or all NMDARs in GnRH neurons and other mainly limbic system neurons in hypothalamic and septal areas (94). However, other neurotransmitter receptors may have compensated during development for the lack of GluA2-containing AMPARs or all NMDARs in GnRH neurons and other mainly limbic system neurons in those mice.
Monoamines
Dopamine (DA)
DA exerts complex pre- and postsynaptic actions on GnRH neurons. Exogenous DA decreases firing via direct postsynaptic actions, as well as indirectly via RP3V-evoked GABAergic and glutamatergic PSCs (presynaptic actions), in ~50% of GnRH neurons through D1-like and/or D2-like receptors (95). On the other hand, endogenous DA (from neurons in the RP3V and possibly elsewhere) increases firing in ~30% of GnRH neurons, whereas DA from RP3V neurons alone decreases firing in ~20% of GnRH neurons, also through D1-like and/or D2-like receptors (95). D1-like receptors are Gs-coupled receptors that stimulate adenylyl cyclase (AC) to produce cyclic adenosine monophosphate (cAMP), which activates protein kinase A (PKA) and may in turn inhibit K+ channels, while D2-like receptors, including D2, D3, and D4 receptors that appear to be expressed in GnRH neurons (67, 70), are Gi-coupled receptors that inhibit AC, which results in reduced cAMP levels, inhibition of PKA, and activation of K+ channels. Yet, it is unclear how activation of D1-like receptors decreases GnRH neuron firing, since they are coupled to Gs, and since forskolin, which also stimulates AC and increases cAMP, increases GnRH neuron firing (96). DA, or tyrosine hydroxylase (TH), the rate-limiting enzyme in DA synthesis, is co-expressed in ~90% of RP3V KP neurons, and axon terminals of TH neurons appose GnRH neurons (97, 98), but mice in which TH has been knocked out exhibit normal puberty, LH levels, and fertility, suggesting that DA from RP3V KP neurons is not required for puberty or reproduction (99). However, other neurotransmitters may have compensated for the loss of TH (and likely absence of DA) in RP3V KP neurons in those mice.
Serotonin (5-HT)
GnRH neurons in the POA receive direct projections from 5-HT neurons in the raphe nuclei [RN (100)]. Exogenous 5-HT, or the selective serotonin reuptake inhibitor (SSRI) fluoxetine, which increases endogenous 5-HT levels, directly inhibits (via 5-HT1A receptors, which are coupled to Gi) and then excites (via 5-HT2A receptors, which are coupled to Gq) GnRH neurons (96). The inhibition depends on K+ channel activation and AC inhibition, whereas the excitation depends on protein kinase C (PKC), and the balance of 5-HT-evoked inhibition vs. excitation varies according to age, sex, and estrous cycle stage (96). However, it should be noted that preventing or blocking AC activity has also been shown to have no effect on GnRH neuron activity (101, 102). GnRH neurons appear to express 5-HT1A, 5-HT2A, 5-HT1A, 5-HT3A, and 5-HT4 5-HT receptor subunits (67, 69, 70).
Norepinephrine (NE)
Brainstem A2 (solitary tract nucleus; NTS) and A6 (locus coeruleus; LC) NE-containing neurons project to GnRH neurons in the POA (100), and dopamine-β-hydroxylase (the rate-limiting enzyme for NE synthesis)-immunoreactive terminals form synapses on GnRH neuron dendrites (103). NE suppresses GnRH neuron firing directly, and the suppression is mimicked by the α1-adrenergic agonist phenylephrine and β-adrenergic agonist isoproterenol, but not by the α2-adrenergic receptor agonist guanabenz, suggesting that NE activates α1- and β-adrenergic, but not α2-adrenergic receptors, in GnRH neurons (104). Additional evidence for the participation of both α1- and β-adrenergic receptors is that the α1-adrenergic antagonist prazosin reduces the hyperpolarizing action of NE significantly but not completely (104). However, it is unclear how α1- and β-adrenergic receptors mediate the suppression since α1- and β-adrenergic receptors are coupled to the stimulatory G proteins Gq and Gs, respectively. GnRH neurons appear to express α1A-, α1B-, α2A-, α2B-, α2C-, and β1-adrenergic subunits (67, 69, 70).
Epinephrine (Epi)
Inhibition of central Epi synthesis blocks the GnRH/LH surge in E and P-treated ovariectomized rats (105); however, the effects of Epi, which like NE may bind to α1- and β-adrenergic receptors, on GnRH neuron activity or GnRH/LH secretion in mice have yet to be reported.
Histamine (H)
H increases [Ca2+]i and GnRH secretion in immortalized mouse GnRH neurons via H1 receptors coupled to phosphoinositide hydrolysis (106, 107). Also, axons of ERα-expressing histaminergic neurons in the tuberomammillary nucleus (TMN) in rats and humans exhibit axo-dendritic and axo-somatic appositions onto GnRH neurons, and intracerebroventricular administration of the H1 receptor antagonist, mepyramine, blocks the GnRH/LH surge in ovariectomized E-treated rats, suggesting that E-receptive histaminergic neurons in the TMN contribute to the positive feedback effect of E in the induction of the GnRH/LH surge (108). Although mouse GnRH neurons appear to express H1 and H2 receptors (67, 70), which are coupled to Gq and Gs, respectively, the effects of H on GnRH neuron activity and secretion in mice have yet to be reported.
Purines
Adenosine triphosphate (ATP)
Extracellular ATP increases [Ca2+]i and GnRH secretion via activation of P2X receptor channels, which are non-selective cation channels, followed by membrane depolarization and subsequent Ca2+ influx through L-type Ca2+ channels, in GnRH neurons cultured from the olfactory placode of monkey embryos (109). Mice express P2X2, P2X4, P2X5, P2X6, and P2X7 ATP receptor subunits in GnRH neurons (70, 110). While blockade of P2X receptor channels by the P2X receptor antagonist pyridoxal-phosphate-6-azophenyl-2′,4′-disulfonate (PPADS) has no effect on spontaneous [Ca2+]i oscillations in GnRH neurons cultured from nasal pit explants of mouse embryos (27), there are no reports yet on the effects of extracellular ATP, or of P2X receptor antagonists, on GnRH neuron electrical activity, [Ca2+]i, or secretion in GnRH neurons of postnatal mice.
Adenosine (Ado)
GnRH neurons appear to express Ado receptors 2A and 2B (67, 70), which are coupled to Gs. However, as with extracellular ATP, there are no reports yet on the effects of extracellular Ado on GnRH neuron electrical activity, [Ca2+]i, or secretion in mice.
Cholinergics
Acetylcholine (ACh)
ACh exerts stimulatory and inhibitory effects on GnRH secretion in immortalized GnRH neurons, which express α7 nicotinic ACh receptors (AChRs) and M1, M2, and M4 muscarinic AChRs (111–113). Activation of α7 nicotinic ACh AChRs, which are non-selective cation channels, transiently increases basal GnRH secretion from immortalized GnRH neurons via depolarization followed by Ca2+ influx through voltage-gated Ca2+ channels (111, 112). It also inhibits prostaglandin E2 (PGE2)- and high K+-induced GnRH secretion (113), possibly due to Ca2+-dependent Ca2+ channel inactivation. Activation of M1 muscarinic AChRs, which couple to Gq, with micromolar concentrations of ACh, increases basal GnRH secretion from immortalized GnRH neurons via IP3-mediated Ca2+ mobilization (111, 114), whereas activation of M2 and M4 muscarinic AChRs, which couple to Gi, with nanomolar concentrations of ACh, inhibits basal GnRH secretion from immortalized GnRH neurons (111, 112). Most likely, ACh first increases [Ca2+]i and GnRH secretion in immortalized GnRH neurons by activating α7 nicotinic AChRs, then decreases [Ca2+]i and GnRH secretion by activating M2/M4 AChRs, and subsequently induces a return of [Ca2+]i and GnRH secretion to basal levels by activating M1 muscarinic AChRs. Cholinergic axons appose, but do not make classical synapses onto, GnRH neurons in rats, suggesting a non-synaptic route of cholinergic communication to GnRH neurons (115). Mouse GnRH neurons appear to express β1, β2, β4, γ, and ε nicotinic AChRs as well as M1 and M4 muscarinic AChRs (67, 69, 70). However, the effects of ACh on GnRH neuron activity or secretion in mice, and whether cholinergic axons appose GnRH neurons in mice, have yet to be reported.
Modulation of GnRH Neuron Activity and Secretion by Gasotransmitters
Gasotransmitters are defined here as small molecules of gas that are produced in the cytoplasm of neurons and immediately diffuse through the cell membrane into the extracellular fluid and into nearby neurons (e.g., GnRH neurons) to stimulate production of second messengers (such as cGMP) that can affect neuronal activity and neurotransmitter/neuropeptide release.
Nitric Oxide (NO)
NO released from neuronal nitric oxide synthase (nNOS)-expressing neurons in the POA directly inhibits the firing of GnRH neurons in the POA by activating soluble guanylyl cyclase (sGC, the NO receptor) and a K+ conductance (16). The nNOS in the POA nNOS neurons (and thus, presumably, NO release from those neurons) is required for E-mediated feedback inhibition of pulsatile GnRH/LH release, as well as for the proestrus GnRH/LH surge, gonadal development, and fertility (28, 116). POA nNOS neurons are apposed by KP-immunoreactive fibers, express the kisspeptin receptor, G protein-coupled receptor 54 (GPR54), and exhibit increased nNOS phosphorylation/activation in response to both E and KP (28). However, the POA nNOS neurons, unlike GnRH neurons, appear not to be the key site of KP-GPR54 signaling for fertility, since GnRH neuron-specific deletion of GPR54 prevents gonadal/pubertal development and results in infertility while GnRH neuron-specific rescue of GPR54 in global GPR54 knockout mice results in normal pubertal development and fertility (3). In addition to nNOS expression in POA neurons, GnRH neurons themselves express nNOS and are capable of generating NO: the retrograde messenger acts on presynaptic GABA and Glu boutons that contain sGC and, as mentioned above, increases GABAergic and glutamatergic transmission to GnRH neurons in response to E and may contribute to the proestrous GnRH/LH surge (86, 117).
Carbon Monoxide (CO)
Although the heme oxygenase 1 (HO-1) activator/CO donor hematin was reported to have no effect on in vivo release of GnRH from rat hypothalamus (118), the HO-1 donor hemin was reported to increase GnRH release from immortalized GnRH neurons (119). However, there are no reports yet on the effect of CO on GnRH neuron activity or secretion in mice.
Modulation of GnRH Neuron Activity and Secretion by Gliotransmitters
Gliotransmitters are defined here as endogenous chemicals released from glial cells (primarily astrocytes), through plasma membrane channels, plasma membrane transporters, or Ca2+-dependent exocytosis onto neurons (e.g., GnRH neurons), where they bind to and activate specific receptors linked to ion channels or second messenger pathways. Similar to neurotransmission, gliotransmission results in a change or modulation of the activity (electrical activity and/or [Ca2+]i) and output (neurotransmitter or hormone secretion) of the neurons.
Prostaglandin E2 (PGE2)
PGE2 released from astrocytes in response to neuregulin-erbB signaling acts directly on GnRH neuron cell bodies in the POA, which morphologically interact with astrocytes, via the EP2 class of PGE2 receptors and PKA signaling to increase GnRH neuron firing and GnRH secretion (17, 120). It may mediate the stimulatory effects of Glu, oxytocin and/or blood-borne factors on GnRH neurons (17, 120) and is necessary for the normal timing of puberty and for adult reproductive function (121, 122).
Other Gliotransmitters?
Some of the non-peptide neurotransmitters, such as GABA, Glu, and ATP, may also function as gliotransmitters [reviewed by (123–125)]. Whether they do so to modulate GnRH neuron activity and secretion remains to be explored. In addition, microglia-derived cytokines, including interleukin-1 (IL-1), interleukin-10 (IL-10), and interleukin-18 (IL-18), which are proteins, as well as microglia-derived prostaglandins, may function as gliotransmitters and together with their receptors expressed in GnRH neurons appear to be important in modulating GnRH neuron activity, especially under inflammatory conditions (126–132).
Concluding Remarks
As described above, and indicated in Figure 1, various non-peptide neurotransmitters, gasotransmitters, and gliotransmitters modulate GnRH neuron activity and GnRH secretion controlling fertility in mice. Modulation by some transmitters has been demonstrated in immortalized mouse GnRH neurons, rats, or other mammalian species but not yet in mice. Further research is needed to address whether those transmitters modulate GnRH neuron activity or GnRH/LH secretion in mice. Research is also needed to determine which presynaptic neurons and glial cells release which transmitters onto GnRH neurons, and under which physiological conditions, as well as the roles of selected transmitter receptors of interest in GnRH neurons on GnRH neuron activity, GnRH/LH secretion, and fertility. Approaches such as rabies viral monosynaptic tracing [to identify the cells that make direct synaptic connections onto GnRH neurons (133)] and inducible GnRH neuron-specific deletion of selected transmitter receptor subunits [to avoid developmental compensation by other subunits or transmitters (134)], should help in this regard. In vivo or ex vivo recording, imaging, or photometry of GnRH neuron electrical activity or Ca2+ dynamics (5, 19, 25, 135), in combination with optogenetic or chemogenetic stimulation or inhibition of presynaptic cells [to evoke or inhibit the release of transmitters from presynaptic cells (4, 26, 135–138)], along with new or improved methods for measuring GnRH/LH secretion, and monitoring of fertility (22, 137, 139, 140), should also help. Finally, to better understand how endocrine, metabolic, and environmental signals integrate into the HPG axis to control fertility, in addition to elucidating the direct modulation of GnRH neuron activity and secretion by transmitters, it will be important to further delineate the upstream networks that connect to KP neurons, other neurons, and glial cells that release the transmitters that affect GnRH neuron [see for example (141)].
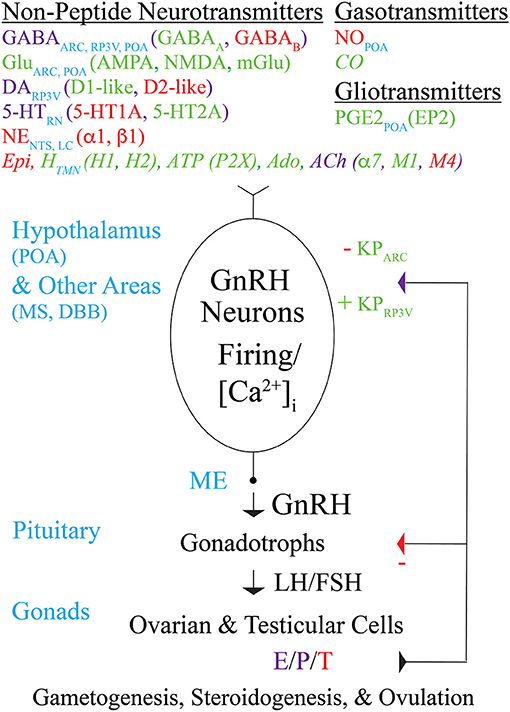
Figure 1. Modulation by non-peptide neurotransmitters, gasotransmitters, and gliotransmitters of GnRH neuron activity and GnRH secretion controlling fertility in mice. Schematic diagram showing non-peptide neurotransmitters (and their receptors), gasotransmitters, and gliotransmitters that act directly on the cell bodies, dendrites, axons, and/or “dendrons” of GnRH neurons to modulate their action potential firing, [Ca2+]i, and GnRH secretion. Transmitters that excite, inhibit, or both excite and inhibit GnRH neurons are indicated in green, red, or purple, respectively, and are listed along with the brain areas in which they are produced. Transmitters that modulate GnRH neuron activity and/or secretion in immortalized GnRH neurons, rat GnRH neurons, or monkey GnRH neurons but have not yet been reported to modulate GnRH neuron activity or secretion in mice are indicated in italics. GnRH secreted from GnRH neuron axon terminals in the ME into the hypothalamo-hypophyseal circulation binds to GnRH receptors on pituitary gonadotrophs to stimulate the synthesis and secretion of LH and FSH into the general circulation. LH and FSH, which are required for gametogenesis and ovulation, bind to receptors in the gonads to stimulate the synthesis and secretion of E, P, and T, which in turn exert negative or positive feedback effects on GnRH neurons (via KP neurons) and gonadotrophs depending on the sex and estrous cycle stage of the animal. Abbreviations are explained at their first occurrence in the main text.
Author Contributions
The author confirms being the sole contributor of this work and has approved it for publication.
Conflict of Interest Statement
The author declares that the research was conducted in the absence of any commercial or financial relationships that could be construed as a potential conflict of interest.
References
1. Clarkson J, d'Anglemont de Tassigny X, Moreno AS, Colledge WH, Herbison AE. Kisspeptin-GPR54 signaling is essential for preovulatory gonadotropin-releasing hormone neuron activation and the luteinizing hormone surge. J Neurosci. (2008) 28:8691–7. doi: 10.1523/JNEUROSCI.1775-08.2008
2. Navarro VM, Gottsch ML, Chavkin C, Okamura H, Clifton DK, Steiner RA. Regulation of gonadotropin-releasing hormone secretion by kisspeptin/dynorphin/neurokinin B neurons in the arcuate nucleus of the mouse. J Neurosci. (2009) 29:11859–66. doi: 10.1523/JNEUROSCI.1569-09.2009
3. Kirilov M, Clarkson J, Liu X, Roa J, Campos P, Porteous R, et al. Dependence of fertility on kisspeptin-Gpr54 signaling at the GnRH neuron. Nat Commun. (2013) 4:2492. doi: 10.1038/ncomms3492
4. Han SY, McLennan T, Czieselsky K, Herbison AE. Selective optogenetic activation of arcuate kisspeptin neurons generates pulsatile luteinizing hormone secretion. Proc Natl Acad Sci USA. (2015) 112:13109–14. doi: 10.1073/pnas.1512243112
5. Han SY, Kane G, Cheong I, Herbison AE. Characterization of GnRH pulse generator activity in male mice using GCaMP fiber photometry. Endocrinology. (2019) 160:557–67. doi: 10.1210/en.2018-01047
6. Herbison AE. Control of puberty onset and fertility by gonadotropin-releasing hormone neurons. Nat Rev Endocrinol. (2016) 12:452–66. doi: 10.1038/nrendo.2016.70
7. Herbison AE. The gonadotropin-releasing hormone pulse generator. Endocrinology. (2018) 159:3723–36. doi: 10.1210/en.2018-00653
8. Constantin S. Progress and challenges in the search for the mechanisms of pulsatile gonadotropin-releasing hormone secretion. Front Endocrinol (Lausanne). (2017) 8:180. doi: 10.3389/fendo.2017.00180
9. Fergani C, Leon S, Padilla SL, Verstegen AM, Palmiter RD, Navarro VM. NKB signaling in the posterodorsal medial amygdala stimulates gonadotropin release in a kisspeptin-independent manner in female mice. Elife. (2018) 19:e40476. doi: 10.7554/eLife.40476
10. Hellier V, Brock O, Candlish M, Desroziers E, Aoki M, Mayer C, et al. Female sexual behavior in mice is controlled by kisspeptin neurons. Nat Commun. (2018) 9:400. doi: 10.1038/s41467-017-02797-2
11. Yeo SH, Colledge WH. The role of Kiss1 neurons as integrators of endocrine, metabolic, and environmental factors in the hypothalamic-pituitary-gonadal axis. Front Endocrinol (Lausanne). (2018) 9:188. doi: 10.3389/fendo.2018.00188
12. Christian CA, Mobley JL, Moenter SM. Diurnal and estradiol-dependent changes in gonadotropin-releasing hormone neuron firing activity. Proc Natl Acad Sci USA. (2005) 102:15682–7. doi: 10.1073/pnas.0504270102
13. Pielecka J, Quaynor SD, Moenter SM. Androgens increase gonadotropin-releasing hormone neuron firing activity in females and interfere with progesterone negative feedback. Endocrinology. (2006) 147:1474–9. doi: 10.1210/en.2005-1029
14. Christian CA, Moenter SM. Estradiol induces diurnal shifts in GABA transmission to gonadotropin-releasing hormone neurons to provide a neural signal for ovulation. J Neurosci. (2007) 27:1913–21. doi: 10.1523/JNEUROSCI.4738-06.2007
15. Christian CA, Moenter SM. Critical roles for fast synaptic transmission in mediating estradiol negative and positive feedback in the neural control of ovulation. Endocrinology. (2008) 149:5500–8. doi: 10.1210/en.2008-0453
16. Clasadonte J, Poulain P, Beauvillain JC, Prevot V. Activation of neuronal nitric oxide release inhibits spontaneous firing in adult gonadotropin-releasing hormone neurons: a possible local synchronizing signal. Endocrinology. (2008) 149:587–96. doi: 10.1210/en.2007-1260
17. Clasadonte J, Poulain P, Hanchate NK, Corfas G, Ojeda SR, Prevot V. Prostaglandin E2 release from astrocytes triggers gonadotropin-releasing hormone (GnRH) neuron firing via EP2 receptor activation. Proc Natl Acad Sci USA. (2011) 108:16104–9. doi: 10.1073/pnas.1107533108
18. Farkas I, Kalló I, Deli L, Vida B, Hrabovszky E, Fekete C, et al. Retrograde endocannabinoid signaling reduces GABAergic synaptic transmission to gonadotropin-releasing hormone neurons. Endocrinology. (2010) 151:5818–29. doi: 10.1210/en.2010-0638
19. Constantin S, Iremonger KJ, Herbison AE. In vivo recordings of GnRH neuron firing reveal heterogeneity and dependence upon GABAA receptor signaling. J Neurosci. (2013) 33:9394–401. doi: 10.1523/JNEUROSCI.0533-13.2013
20. Roland AV, Moenter SM. Glucosensing by GnRH neurons: inhibition by androgens and involvement of AMP-activated protein kinase. Mol Endocrinol. (2011) 25:847–58. doi: 10.1210/me.2010-0508
21. Herde MK, Iremonger KJ, Constantin S, Herbison AE. GnRH neurons elaborate a long-range projection with shared axonal and dendritic functions. J Neurosci. (2013) 33:12689–97. doi: 10.1523/JNEUROSCI.0579-13.2013
22. Glanowska KM, Moenter SM. Differential regulation of GnRH secretion in the preoptic area (POA) and the median eminence (ME) in male mice. Endocrinology. (2015) 156:231–41. doi: 10.1210/en.2014-1458
23. Cimino I, Casoni F., Liu X, Messina A, Parkash J, Jamin SP, et al. Novel role for anti-Müllerian hormone in the regulation of GnRH neuron excitability and hormone secretion. Nat Commun. (2016) 7:10055. doi: 10.1038/ncomms10055
24. Klenke U, Constantin S, Wray S. BPA directly decreases GnRH neuronal activity via noncanonical pathway. Endocrinology. (2016) 157:1980–90. doi: 10.1210/en.2015-1924
25. Iremonger KJ, Porteous R, Herbison AE. Spike and neuropeptide-dependent mechanisms control GnRH neuron nerve terminal Ca2+ over diverse time scales. J Neurosci. (2017) 37:3342–51. doi: 10.1523/JNEUROSCI.2925-16.2017
26. Piet R, Kalil B, McLennan T, Porteous R, Czieselsky K, Herbison AE. Dominant neuropeptide cotransmission in kisspeptin-GABA regulation of GnRH neuron firing driving ovulation. J Neurosci. (2018) 38:6310–22. doi: 10.1523/JNEUROSCI.0658-18.2018
27. Constantin S, Klenke U, Wray S. The calcium oscillator of GnRH-1 neurons is developmentally regulated. Endocrinology. (2010) 151:3863–73. doi: 10.1210/en.2010-0118
28. Hanchate NK, Parkash J, Bellefontaine N, Mazur D, Colledge WH, d'Anglemont de Tassigny X, et al. Kisspeptin-GPR54 signaling in mouse NO-synthesizing neurons participates in the hypothalamic control of ovulation. J Neurosci. (2012) 32:932–45. doi: 10.1523/JNEUROSCI.4765-11.2012
29. Herde MK, Herbison AE. Morphological characterization of the action potential initiation segment in GnRH neuron dendrites and axons of male mice. Endocrinology. (2015) 156:4174–86. doi: 10.1210/en.2015-1284
30. Campbell RE, Han SK, Herbison AE. Biocytin filling of adult gonadotropin-releasing hormone neurons in situ reveals extensive, spiny, dendritic processes. Endocrinology. (2005) 146:1163–9. doi: 10.1210/en.2004-1369
31. Iremonger KJ, Herbison AE. Initiation and propagation of action potentials in gonadotropin-releasing hormone neuron dendrites. J Neurosci. (2012) 32:151–8. doi: 10.1523/JNEUROSCI.3739-11.2012
32. Campbell RE, Gaidamaka G, Han SK, Herbison AE. Dendro-dendritic bundling and shared synapses between gonadotropin-releasing hormone neurons. Proc Natl Acad Sci USA. (2009) 106:10835–40. doi: 10.1073/pnas.0903463106
33. Yip SH, Boehm U, Herbison AE, Campbell RE. Conditional viral tract tracing delineates the projections of the distinct kisspeptin neuron populations to gonadotropin-releasing hormone (GnRH) neurons in the mouse. Endocrinology. (2015) 156:2582–94. doi: 10.1210/en.2015-1131
34. Pinet-Charvet C, Geller S, Desroziers E, Ottogalli M, Lomet D, Georgelin C, et al. GnRH episodic secretion is altered by pharmacological blockade of gap junctions: possible involvement of glial cells. Endocrinology. (2016) 157:304–22. doi: 10.1210/en.2015-1437
35. Moore AM, Prescott M, Marshall CJ, Yip SH, Campbell RE. Enhancement of a robust arcuate GABAergic input to gonadotropin-releasing hormone neurons in a model of polycystic ovarian syndrome. Proc Natl Acad Sci USA. (2015) 112:596–601. doi: 10.1073/pnas.1415038112
36. Moore AM, Prescott M, Czieselsky K, Desroziers E, Yip SH, Campbell RE, et al. Synaptic innervation of the GnRH neuron distal dendron in female mice. Endocrinology. (2018) 159:3200–8. doi: 10.1210/en.2018-00505
37. Kaprara A, Huhtaniemi IT. The hypothalamus-pituitary-gonad axis: tales of mice and men. Metabolism. (2018) 86:3–17. doi: 10.1016/j.metabol.2017.11.018
38. Chan H, Prescott M, Ong Z, Herde MK, Herbison AE, Campbell RE. Dendritic spine plasticity in gonadotropin-releasing hormone (GnRH) neurons activated at the time of the preovulatory surge. Endocrinology. (2011) 152:4906–14. doi: 10.1210/en.2011-1522
39. Cheong RY, Czieselsky K, Porteous R, Herbison AE. Expression of ESR1 in glutamatergic and GABAergic neurons is essential for normal puberty onset, estrogen feedback, and fertility in female mice. J Neurosci. (2015) 35:14533–43. doi: 10.1523/JNEUROSCI.1776-15.2015
40. Spergel DJ. Neuropeptidergic modulation of GnRH neuronal activity and GnRH secretion controlling reproduction: insights from recent mouse studies. Cell Tissue Res. (2018) 375:179–91. doi: 10.1007/s00441-018-2893-z
41. Mellon PL, Windle JJ, Goldsmith PC, Padula CA, Roberts JL, Weiner RI. Immortalization of hypothalamic GnRH neurons by genetically targeted tumorigenesis. Neuron. (1990) 5:1–10. doi: 10.1016/0896-6273(90)90028-E
42. Radovick S, Wray S, Lee E, Nicols DK, Nakayama Y, Weintraub BD, et al. Migratory arrest of gonadotropin-releasing hormone neurons in transgenic mice. Proc Natl Acad Sci USA. (1991) 88:3402–6. doi: 10.1073/pnas.88.8.3402
43. Kusano K, Fueshko S, Gainer H, Wray S. Electrical and synaptic properties of embryonic luteinizing hormone-releasing hormone neurons in explant cultures. Proc Natl Acad Sci USA. (1995) 92:3918–22. doi: 10.1073/pnas.92.9.3918
44. Spergel DJ, Krüth U, Hanley DF, Sprengel R, Seeburg PH. GABA- and glutamate-activated channels in green fluorescent protein-tagged gonadotropin-releasing hormone neurons in transgenic mice. J Neurosci. (1999) 19:2037–50. doi: 10.1523/JNEUROSCI.19-06-02037.1999
45. Suter KJ, Song WJ, Sampson TL, Wuarin JP, Saunders JT, Dudek FE, et al. Genetic targeting of green fluorescent protein to gonadotropin-releasing hormone neurons: characterization of whole-cell electrophysiological properties and morphology. Endocrinology. (2000) 141:412–9. doi: 10.1210/endo.141.1.7279
46. Salvi R, Castillo E, Voirol MJ, Glauser M, Rey JP, Gaillard RC, et al. Gonadotropin-releasing hormone-expressing neurons immortalized conditionally are activated by insulin: implication of the mitogen-activated protein kinase pathway. Endocrinology. (2006) 147:816–26. doi: 10.1210/en.2005-0728
47. Jasoni CL, Todman MG, Strumia MM, Herbison AE. Cell type-specific expression of a genetically encoded calcium indicator reveals intrinsic calcium oscillations in adult gonadotropin-releasing hormone neurons. J Neurosci. (2007) 27:860–7 doi: 10.1523/JNEUROSCI.3579-06.2007
48. Wolfe A, Ng Y, Divall SA, Singh SP, Radovick S. Development of an immortalised, post-pubertal gonadotrophin-releasing hormone neuronal cell line. J Neuroendocrinol. (2008) 20:1029–37. doi: 10.1111/j.1365-2826.2008.01760.x
49. McFadden SA, Menchella JA, Chalmers JA, Centeno ML, Belsham DD. Glucose responsiveness in a novel adult-derived GnRH cell line, mHypoA-GnRH/GFP: involvement of AMP-activated protein kinase. Mol Cell Endocrinol. (2013) 377:65–74. doi: 10.1016/j.mce.2013.06.035
50. Zhu J, Xu XH, Knight GE, He C, Burnstock G, Xiang Z. A subpopulation of gonadotropin-releasing hormone neurons in the adult mouse forebrain is γ-aminobutyric acidergic. J Neurosci Res. (2015) 93:1611–21. doi: 10.1002/jnr.23610
51. Zhang C, Bosch MA, Rønnekleiv OK, Kelly MJ. Gamma-aminobutyric acid B receptor mediated inhibition of gonadotropin-releasing hormone neurons is suppressed by kisspeptin-G protein-coupled receptor 54 signaling. Endocrinology. (2009) 150:2388–94. doi: 10.1210/en.2008-1313
52. Bhattarai JP, Park SA, Park JB, Lee SY, Herbison AE, Ryu PD, et al. Tonic extrasynaptic GABA(A) receptor currents control gonadotropin-releasing hormone neuron excitability in the mouse. Endocrinology. (2011) 152:1551–61. doi: 10.1210/en.2010-1191
53. Liu X, Herbison AE. Estrous cycle- and sex-dependent changes in pre- and postsynaptic GABAB control of GnRH neuron excitability. Endocrinology. (2011) 152:4856–64. doi: 10.1210/en.2011-1369
54. Constantin S, Piet R, Iremonger K, Hwa Yeo S, Clarkson J, Porteous R, et al. GnRH neuron firing and response to GABA in vitro depend on acute brain slice thickness and orientation. Endocrinology. (2012) 153:3758–69. doi: 10.1210/en.2012-1126
55. Chachlaki K, Malone SA, Qualls-Creekmore E, Hrabovszky E, Münzberg H, Giacobini P, et al. Phenotyping of nNOS neurons in the postnatal and adult female mouse hypothalamus. J Comp Neurol. (2017) 525:3177–89. doi: 10.1002/cne.24257
56. DeFazio RA, Heger S, Ojeda SR, Moenter SM. Activation of A-type gamma-aminobutyric acid receptors excites gonadotropin-releasing hormone neurons. Mol Endocrinol. (2002) 16:2872–91. doi: 10.1210/me.2002-0163
57. Han SK, Abraham IM, Herbison AE. Effect of GABA on GnRH neurons switches from depolarization to hyperpolarization at puberty in the female mouse. Endocrinology. (2002) 143:1459–66. doi: 10.1210/endo.143.4.8724
58. Han SK, Todman MG, Herbison AE. Endogenous GABA release inhibits the firing of adult gonadotropin-releasing hormone neurons. Endocrinology. (2004) 145:495–9. doi: 10.1210/en.2003-1333
59. Moenter SM, DeFazio RA. Endogenous gamma-aminobutyric acid can excite gonadotropin-releasing hormone neurons. Endocrinology. (2005) 146:5374–9. doi: 10.1210/en.2005-0788
60. Romanò N, Lee K, Abrahám IM, Jasoni CL, Herbison AE. Nonclassical estrogen modulation of presynaptic GABA terminals modulates calcium dynamics in gonadotropin-releasing hormone neurons. Endocrinology. (2008) 149:5335–44. doi: 10.1210/en.2008-0424
61. Constantin S, Jasoni CL, Wadas B, Herbison AE. Gamma-aminobutyric acid and glutamate differentially regulate intracellular calcium concentrations in mouse gonadotropin-releasing hormone neurons. Endocrinology. (2010) 151:262–70. doi: 10.1210/en.2009-0817
62. Hemond PJ, O'Boyle MP, Roberts CB, Delgado-Reyes A, Hemond Z, Suter KJ. Simulated GABA synaptic input and L-type calcium channels form functional microdomains in hypothalamic gonadotropin-releasing hormone neurons. J Neurosci. (2012) 32:8756–66. doi: 10.1523/JNEUROSCI.4188-11.2012
63. Leupen SM, Tobet SA, Crowley WF Jr, Kaila K. Heterogeneous expression of the potassium-chloride cotransporter KCC2 in gonadotropin-releasing hormone neurons of the adult mouse. Endocrinology. (2003) 144:3031–6. doi: 10.1210/en.2002-220995
64. Taylor-Burds C, Cheng P, Wray S. Chloride accumulators NKCC1 and AE2 in mouse GnRH neurons: implications for GABAA mediated excitation. PLoS ONE. (2015) 10:e0131076. doi: 10.1371/journal.pone.0131076
65. Sim JA, Skynner MJ, Pape JR, Herbison AE. Late postnatal reorganization of GABA(A) receptor signalling in native GnRH neurons. Eur J Neurosci. (2000) 12:3497–504. doi: 10.1046/j.1460-9568.2000.00261.x
66. Pape JR, Skynner MJ, Sim JA, Herbison AE. Profiling gamma-aminobutyric acid (GABA(A)) receptor subunit mRNA expression in postnatal gonadotropin-releasing hormone (GnRH) neurons of the male mouse with single cell RT-PCR. Neuroendocrinology. (2001) 74:300–8. doi: 10.1159/000054697
67. Todman MG, Han SK, Herbison AE. Profiling neurotransmitter receptor expression in mouse gonadotropin-releasing hormone neurons using green fluorescent protein-promoter transgenics and microarrays. Neuroscience. (2005) 132:703–12. doi: 10.1016/j.neuroscience.2005.01.035
68. Penatti CA, Davis MC, Porter DM, Henderson LP. Altered GABAA receptor-mediated synaptic transmission disrupts the firing of gonadotropin-releasing hormone neurons in male mice under conditions that mimic steroid abuse. J Neurosci. (2010) 30:6497–506. doi: 10.1523/JNEUROSCI.5383-09.2010
69. Vastagh C, Rodolosse A, Solymosi N, Farkas I, Auer H, Sárvári M, et al. Differential gene expression in gonadotropin-releasing hormone neurons of male and metestrous female mice. Neuroendocrinology. (2015) 102:44–59. doi: 10.1159/000430818
70. Vastagh C, Rodolosse A, Solymosi N, Liposits Z. Altered expression of genes encoding neurotransmitter receptors in GnRH neurons of proestrous mice. Front Cell Neurosci. (2016) 10:230. doi: 10.3389/fncel.2016.00230
71. Lee K, Porteous R, Campbell RE, Lüscher B, Herbison AE. Knockdown of GABA(A) receptor signaling in GnRH neurons has minimal effects upon fertility. Endocrinology. (2010) 151:4428–36. doi: 10.1210/en.2010-0314
72. Camille Melón L, Maguire J. GABAergic regulation of the HPA and HPG axes and the impact of stress on reproductive function. J Steroid Biochem Mol Biol. (2016) 160:196–203. doi: 10.1016/j.jsbmb.2015.11.019
73. Liu X, Porteous R, d'Anglemont de Tassigny X, Colledge WH, Millar R, Petersen SL, et al. Frequency-dependent recruitment of fast amino acid and slow neuropeptide neurotransmitter release controls gonadotropin-releasing hormone neuron excitability. J Neurosci. (2011) 31:2421–30. doi: 10.1523/JNEUROSCI.5759-10.2011
74. Catalano PN, Bonaventura MM, Silveyra P, Bettler B, Libertun C, Lux-Lantos VA. GABA(B1) knockout mice reveal alterations in prolactin levels, gonadotropic axis, and reproductive function. Neuroendocrinology. (2005) 82:294–305. doi: 10.1159/000093128
75. Di Giorgio NP, Catalano PN, López PV, González B, Semaan SJ, López GC, et al. Lack of functional GABAB receptors alters Kiss1, Gnrh1 and Gad1 mRNA expression in the medial basal hypothalamus at postnatal day 4. Neuroendocrinology. (2013) 98:212–23. doi: 10.1159/000355631
76. Moore AM, Abbott G, Mair J, Prescott M, Campbell RE. Mapping GABA and glutamate inputs to gonadotrophin-releasing hormone neurones in male and female mice. J Neuroendocrinol. (2018) 30:e12657. doi: 10.1111/jne.12657
77. Sullivan SD, Moenter SM. Prenatal androgens alter GABAergic drive to gonadotropin-releasing hormone neurons: implications for a common fertility disorder. Proc Natl Acad Sci USA. (2004) 101:7129–34. doi: 10.1073/pnas.0308058101
78. Goodarzi MO, Dumesic DA, Chazenbalk G, Azziz R. Polycystic ovary syndrome: etiology, pathogenesis and diagnosis. Nat Rev Endocrinol. (2011) 7:219–31. doi: 10.1038/nrendo.2010.217
79. Berg T, Silveira MA, Moenter SM. Prepubertal development of GABAergic transmission to gonadotropin-releasing hormone (GnRH) neurons and postsynaptic response are altered by prenatal androgenization. J Neurosci. (2018) 38:2283–93. doi: 10.1523/JNEUROSCI.2304-17.2018
80. Silva MS, Prescott M, Campbell RE. Ontogeny and reversal of brain circuit abnormalities in a preclinical model of PCOS. JCI Insight. (2018) 3:99405. doi: 10.1172/jci.insight.99405
81. Kawwass JF, Sanders KM, Loucks TL, Rohan LC, Berga SL. Increased cerebrospinal fluid levels of GABA, testosterone and estradiol in women with polycystic ovary syndrome. Hum Reprod. (2017) 32:1450–6. doi: 10.1093/humrep/dex086
82. Cravo RM, Margatho LO, Osborne-Lawrence S, Donato J Jr, Atkin S, Bookout AL, et al. Characterization of Kiss1 neurons using transgenic mouse models. Neuroscience. (2011) 173:37–56. doi: 10.1016/j.neuroscience.2010.11.022
83. Farkas I, Vastagh C, Sárvári M, Liposits Z. Ghrelin decreases firing activity of gonadotropin-releasing hormone (GnRH) neurons in an estrous cycle and endocannabinoid signaling dependent manner. PLoS ONE. (2013) 8:e78178. doi: 10.1371/journal.pone.0078178
84. Adams C, Chen X, Moenter SM. Changes in GABAergic transmission to and intrinsic excitability of gonadotropin-releasing hormone (GnRH) neurons during the estrous cycle in mice. eNeuro. (2018) 5:ENEURO.0171-18.2018. doi: 10.1523/ENEURO.0171-18.2018
85. Pielecka-Fortuna J, Moenter SM. Kisspeptin increases gamma-aminobutyric acidergic and glutamatergic transmission directly to gonadotropin-releasing hormone neurons in an estradiol-dependent manner. Endocrinology. (2010) 151:291–300. doi: 10.1210/en.2009-0692
86. Farkas I, Bálint F, Farkas E, Vastagh C, Fekete C, Liposits Z. Estradiol increases glutamate and GABA neurotransmission into GnRH neurons via retrograde NO-signaling in proestrous mice during the positive estradiol feedback period. eNeuro. (2018) 5:ENEURO.0057-18.2018. doi: 10.1523/ENEURO.0057-18.2018
87. Zuure WA, Roberts AL, Quennell JH, Anderson GM. Leptin signaling in GABA neurons, but not glutamate neurons, is required for reproductive function. J Neurosci. (2013) 33:17874–83. doi: 10.1523/JNEUROSCI.2278-13.2013
88. Zuure WA, Quennell JH, Anderson GM. Leptin responsive and GABAergic projections to the rostral preoptic area in mice. J Neuroendocrinol. (2016) 28:12357. doi: 10.1111/jne.12357
89. Kuehl-Kovarik MC, Pouliot WA, Halterman GL, Handa RJ, Dudek FE, Partin KM. Episodic bursting activity and response to excitatory amino acids in acutely dissociated gonadotropin-releasing hormone neurons genetically targeted with green fluorescent protein. J Neurosci. (2002) 22:2313–22. doi: 10.1523/JNEUROSCI.22-06-02313.2002
90. Dumalska I, Wu M, Morozova E, Liu R, van den Pol A, Alreja M. Excitatory effects of the puberty-initiating peptide kisspeptin and group I metabotropic glutamate receptor agonists differentiate two distinct subpopulations of gonadotropin-releasing hormone neurons. J Neurosci. (2008) 28:8003–13. doi: 10.1523/JNEUROSCI.1225-08.2008
91. Hrabovszky E, Turi GF, Kalló I, Liposits Z. Expression of vesicular glutamate transporter-2 in gonadotropin-releasing hormone neurons of the adult male rat. Endocrinology. (2004) 145:4018–21. doi: 10.1210/en.2004-0589
92. Adjan V, Centers A, Jennes L. Expression and activation of N-methyl-D-aspartate receptor subunit-1 receptor subunits in gonadotrophin-releasing hormone neurones of young and middle-aged mice during the luteinising hormone surge. J Neuroendocrinol. (2008) 20:1147–54. doi: 10.1111/j.1365-2826.2008.01775.x
93. Chu Z, Moenter SM. Endogenous activation of metabotropic glutamate receptors modulates GABAergic transmission to gonadotropin-releasing hormone neurons and alters their firing rate: a possible local feedback circuit. J Neurosci. (2005) 25:5740–9. doi: 10.1523/JNEUROSCI.0913-05.2005
94. Shimshek DR, Bus T, Grinevich V, Single FN, Mack V, Sprengel R, et al. Impaired reproductive behavior by lack of GluR-B containing AMPA receptors but not of NMDA receptors in hypothalamic and septal neurons. Mol Endocrinol. (2006) 20:219–31. doi: 10.1210/me.2005-0262
95. Liu X, Herbison AE. Dopamine regulation of gonadotropin-releasing hormone neuron excitability in male and female mice. Endocrinology. (2013) 154:340–50. doi: 10.1210/en.2012-1602
96. Bhattarai JP, Roa J, Herbison AE, Han SK. Serotonin acts through 5-HT1 and 5-HT2 receptors to exert biphasic actions on GnRH neuron excitability in the mouse. Endocrinology. (2014) 155:513–24. doi: 10.1210/en.2013-1692
97. Hoffman GE. Organization of LHRH cells: differential apposition of neurotensin, substance P and catecholamine axons. Peptides. (1985) 6:439–61. doi: 10.1016/0196-9781(85)90110-X
98. Clarkson J, Herbison AE. Dual phenotype kisspeptin-dopamine neurones of the rostral periventricular area of the third ventricle project to gonadotrophin-releasing hormone neurones. J Neuroendocrinol. (2011) 23:293–301. doi: 10.1111/j.1365-2826.2011.02107.x
99. Stephens SBZ, Rouse ML, Tolson KP, Liaw RB, Parra RA, Chahal N, et al. Effects of selective deletion of tyrosine hydroxylase from kisspeptin cells on puberty and reproduction in male and female mice. eNeuro. (2017) 4:ENEURO.0150-17.2017. doi: 10.1523/ENEURO.0150-17.2017
100. Campbell RE, Herbison AE. Definition of brainstem afferents to gonadotropin-releasing hormone neurons in the mouse using conditional viral tract tracing. Endocrinology. (2007) 148:5884–90. doi: 10.1210/en.2007-0854
101. Constantin S, Wray S. Gonadotropin-releasing hormone-1 neuronal activity is independent of cyclic nucleotide-gated channels. Endocrinology. (2008) 149:279–90 doi: 10.1210/en.2007-0955
102. Constantin S, Wray S. Gonadotropin-releasing hormone-1 neuronal activity is independent of hyperpolarization-activated cyclic nucleotide-modulated channels but is sensitive to protein kinase A-dependent phosphorylation. Endocrinology. (2008) 149:3500–11. doi: 10.1210/en.2007-1508
103. Miller MM, Zhu L. Ovariectomy and age alter gonadotropin hormone releasing hormone-noradrenergic interactions. Neurobiol Aging. (1995) 16:613–25. doi: 10.1016/0197-4580(95)00044-F
104. Han SK, Herbison AE. Norepinephrine suppresses gonadotropin-releasing hormone neuron excitability in the adult mouse. Endocrinology. (2008) 149:1129–35. doi: 10.1210/en.2007-1241
105. Terry LC, Crowley WR, Lynch C, Longserre C, Johnson MD. Role of central epinephrine in regulation of anterior pituitary hormone secretion. Peptides. (1982) 3:311–8. doi: 10.1016/0196-9781(82)90092-4
106. Noris G, Hol D, Clapp C, Martínez de la Escalera G. Histamine directly stimulates gonadotropin-releasing hormone secretion from GT1-1 cells via H1 receptors coupled to phosphoinositide hydrolysis. Endocrinology. (1995) 136:2967–74. doi: 10.1210/endo.136.7.7789322
107. Zamani MR, Dupere JR, Bristow DR. Receptor-mediated desensitisation of histamine H1 receptor-stimulated inositol phosphate production and calcium mobilisation in GT1-7 neuronal cells is independent of protein kinase C. J Neurochem. (1995) 65:160–9. doi: 10.1046/j.1471-4159.1995.65010160.x
108. Fekete CS, Strutton PH, Cagampang FR, Hrabovszky E, Kalló I, Shughrue PJ, et al. Estrogen receptor immunoreactivity is present in the majority of central histaminergic neurons: evidence for a new neuroendocrine pathway associated with luteinizing hormone-releasing hormone-synthesizing neurons in rats and humans. Endocrinology. (1999) 140:4335–41. doi: 10.1210/endo.140.9.6968
109. Terasawa E, Keen KL, Grendell RL, Golos TG. Possible role of 5'-adenosine triphosphate in synchronization of Ca2+ oscillations in primate luteinizing hormone-releasing hormone neurons. Mol Endocrinol. (2005) 19:2736–47. doi: 10.1210/me.2005-0034
110. Fu J, Yu Q, Guo W, He C, Burnstock G, Xiang Z. P2X receptors are expressed on neurons containing luteinizing hormone-releasing hormone in the mouse hypothalamus. Neurosci Lett. (2009) 458:32–6. doi: 10.1016/j.neulet.2009.04.017
111. Krsmanovic LZ, Mores N, Navarro CE, Saeed SA, Arora KK, Catt KJ. Muscarinic regulation of intracellular signaling and neurosecretion in gonadotropin-releasing hormone neurons. Endocrinology. (1998) 139:4037–43. doi: 10.1210/endo.139.10.6267
112. Arai Y, Ishii H, Kobayashi M, Ozawa H. Subunit profiling and functional characteristics of acetylcholine receptors in GT1-7 cells. J Physiol Sci. (2017) 67:313–23. doi: 10.1007/s12576-016-0464-1
113. Messi E, Pimpinelli F, Andrè V, Rigobello C, Gotti C, Maggi R. The alpha-7 nicotinic acetylcholine receptor is involved in a direct inhibitory effect of nicotine on GnRH release: In vitro studies. Mol Cell Endocrinol. (2018) 460:209–18. doi: 10.1016/j.mce.2017.07.025
114. Morales A, Díaz M, Ropero AB, Nadal A, Alonso R. Estradiol modulates acetylcholine-induced Ca2+ signals in LHRH-releasing GT1-7 cells through a membrane binding site. Eur J Neurosci. (2003) 18:2505–14. doi: 10.1046/j.1460-9568.2003.02997.x
115. Turi GF, Liposits Z, Hrabovszky E. Cholinergic afferents to gonadotropin-releasing hormone neurons of the rat. Neurochem Int. (2008) 52:723–8. doi: 10.1016/j.neuint.2007.09.001
116. Gyurko R, Leupen S, Huang PL. Deletion of exon 6 of the neuronal nitric oxide synthase gene in mice results in hypogonadism and infertility. Endocrinology. (2002) 143:2767–74. doi: 10.1210/endo.143.7.8921
117. Farkas I, Vastagh C, Farkas E, Bálint F, Skrapits K, Hrabovszky E, et al. Glucagon-like peptide-1 excites firing and increases GABAergic miniature postsynaptic currents (mPSCs) in gonadotropin-releasing hormone (GnRH) neurons of the male mice via activation of nitric oxide (NO) and suppression of endocannabinoid signaling pathways. Front Cell Neurosci. (2016) 10:214. doi: 10.3389/fncel.2016.00214
118. Kohsaka A, Watanobe H, Kakizaki Y, Suda T. A comparative study of the effects of nitric oxide and carbon monoxide on the in vivo release of gonadotropin-releasing hormone and neuropeptide Y from rat hypothalamus during the estradiol-induced luteinizing hormone surge: estimation by push-pull perfusion. Neuroendocrinology. (1999) 69:245–53. doi: 10.1159/000054425
119. Errico S, Shohreh R, Barone E, Pusateri A, Mores N, Mancuso C. Heme oxygenase-derived carbon monoxide modulates gonadotropin-releasing hormone release in immortalized hypothalamic neurons. Neurosci Lett. (2010) 471:175–8. doi: 10.1016/j.neulet.2010.01.036
120. Sharif A, Baroncini M, Prevot V. Role of glia in the regulation of gonadotropin-releasing hormone neuronal activity and secretion. Neuroendocrinology. (2013) 98:1–15. doi: 10.1159/000351867
121. Prevot V, Rio C, Cho GJ, Lomniczi A, Heger S, Neville CM, et al. Normal female sexual development requires neuregulin-erbB receptor signaling in hypothalamic astrocytes. J Neurosci. (2003) 23:230–9. doi: 10.1523/JNEUROSCI.23-01-00230.2003
122. Prevot V, Lomniczi A, Corfas G, Ojeda SR. erbB-1 and erbB-4 receptors act in concert to facilitate female sexual development and mature reproductive function. Endocrinology. (2005) 146:1465–72. doi: 10.1210/en.2004-1146
123. Halassa MM, Haydon PG. Integrated brain circuits: astrocytic networks modulate neuronal activity and behavior. Annu Rev Physiol. (2010) 72:335–55. doi: 10.1146/annurev-physiol-021909-135843
124. Yoon BE, Lee CJ. GABA as a rising gliotransmitter. Front Neural Circuits. (2014) 8:141. doi: 10.3389/fncir.2014.00141
125. Harada K, Kamiya T, Tsuboi T. Gliotransmitter release from astrocytes: functional, developmental, and pathological implications in the brain. Front Neurosci. (2016) 9:499. doi: 10.3389/fnins.2015.00499
126. Jasoni CL, Todman MG, Han SK, Herbison AE. Expression of mRNAs encoding receptors that mediate stress signals in gonadotropin-releasing hormone neurons of the mouse. Neuroendocrinology. (2005) 82:320–8. doi: 10.1159/000093155
127. Spulber S, Schultzberg M. Connection between inflammatory processes and transmittor function-Modulatory effects of interleukin-1. Prog Neurobiol. (2010) 90:256–62. doi: 10.1016/j.pneurobio.2009.10.015
128. Fujioka H, Kakehashi C, Funabashi T, Akema T. Immunohistochemical evidence for the relationship between microglia and GnRH neurons in the preoptic area of ovariectomized rats with and without steroid replacement. Endocr J. (2013) 60:191–6. doi: 10.1507/endocrj.EJ12-0280
129. Kuwahara-Otani S, Maeda S, Kobayashi K, Minato Y, Tanaka K, Yamanishi K, et al. Interleukin-18 and its receptor are expressed in gonadotropin-releasing hormone neurons of mouse and rat forebrain. Neurosci Lett. (2017) 650:33–7. doi: 10.1016/j.neulet.2017.03.051
130. Barabás K, Barad Z, Dénes Á, Bhattarai JP, Han SK, Kiss E, et al. The role of interleukin-10 in mediating the effect of immune challenge on mouse gonadotropin-releasing hormone neurons in vivo. eNeuro. (2018) 5:ENEURO.0211-18.2018. doi: 10.1523/ENEURO.0211-18.2018
131. Burmeister AR, Marriott I. The interleukin-10 family of cytokines and their role in the CNS. Front Cell Neurosci. (2018) 12:458. doi: 10.3389/fncel.2018.00458
132. Lainez NM, Jonak CR, Nair MG, Ethell IM, Wilson EH, Carson MJ, et al. Diet-induced obesity elicits macrophage infiltration and reduction in spine density in the hypothalami of male but not female mice. Front Immunol. (2018) 9:1992. doi: 10.3389/fimmu.2018.01992
133. Kim EJ, Jacobs MW, Ito-Cole T, Callaway EM. Improved monosynaptic neural circuit tracing using engineered rabies virus glycoproteins. Cell Rep. (2016) 15:692–9. doi: 10.1016/j.celrep.2016.03.067
134. Raftogianni A, Roth LC, García-González D, Bus T, Kühne C, Monyer H, et al. Deciphering the contributions of CRH receptors in the brain and pituitary to stress-induced inhibition of the reproductive axis. Front Mol Neurosci. (2018) 11:305. doi: 10.3389/fnmol.2018.00305
135. Clarkson J, Han SY, Piet R, McLennan T, Kane GM, Ng J, et al. Definition of the hypothalamic GnRH pulse generator in mice. Proc Natl Acad Sci USA. (2017) 114:E10216–E10223. doi: 10.1073/pnas.1713897114
136. Qiu J, Nestor CC, Zhang C, Padilla SL, Palmiter RD, Kelly MJ, et al. High-frequency stimulation-induced peptide release synchronizes arcuate kisspeptin neurons and excites GnRH neurons. Elife. (2016) 23:e16246. doi: 10.7554/eLife.16246
137. Padilla SL, Qiu J, Nestor CC, Zhang C, Smith AW, Whiddon BB, et al. AgRP to Kiss1 neuron signaling links nutritional state and fertility. Proc. Natl. Acad. Sci. USA. (2017) 114:2413–8. doi: 10.1073/pnas.1621065114
138. Stamatakis AM, Schachter MJ, Gulati S, Zitelli KT, Malanowski S, Tajik A, et al. Simultaneous optogenetics and cellular resolution calcium imaging during active behavior using a miniaturized microscope. Front Neurosci. (2018) 12:496. doi: 10.3389/fnins.2018.00496
139. Glanowska KM, Venton BJ, Moenter SM. Fast scan cyclic voltammetry as a novel method for detection of real-time gonadotropin-releasing hormone release in mouse brain slices. J Neurosci. (2012) 32:14664–9. doi: 10.1523/JNEUROSCI.1303-12.2012
140. Czieselsky K, Prescott M, Porteous R, Campos P, Clarkson J, Steyn FJ, et al. Pulse and surge profiles of luteinizing hormone secretion in the mouse. Endocrinology. (2016) 157:4794–802. doi: 10.1210/en.2016-1351
Keywords: GnRH neurons, neurotransmitters, gasotransmitters, gliotransmitters, signaling pathways, electrophysiology, calcium, GnRH secretion
Citation: Spergel DJ (2019) Modulation of Gonadotropin-Releasing Hormone Neuron Activity and Secretion in Mice by Non-peptide Neurotransmitters, Gasotransmitters, and Gliotransmitters. Front. Endocrinol. 10:329. doi: 10.3389/fendo.2019.00329
Received: 09 March 2019; Accepted: 07 May 2019;
Published: 22 May 2019.
Edited by:
Pierrette Gaudreau, Université de Montréal, CanadaReviewed by:
Stephanie Constantin, National Institutes of Health (NIH), United StatesZsolt Liposits, Institute of Experimental Medicine (MTA), Hungary
Copyright © 2019 Spergel. This is an open-access article distributed under the terms of the Creative Commons Attribution License (CC BY). The use, distribution or reproduction in other forums is permitted, provided the original author(s) and the copyright owner(s) are credited and that the original publication in this journal is cited, in accordance with accepted academic practice. No use, distribution or reproduction is permitted which does not comply with these terms.
*Correspondence: Daniel J. Spergel, ZGFuaWVsLnNwZXJnZWwmI3gwMDA0MDt5YWxlLmVkdQ==