- 1Steno Diabetes Center Copenhagen, Gentofte, Denmark
- 2Department of Endocrinology, Hvidovre Hospital, University of Copenhagen, Hvidovre, Denmark
- 3Clinical Institute of Medicine, Aarhus University, Århus, Denmark
- 4Department of Biomedical Sciences, Faculty of Health and Medical Sciences, University of Copenhagen, Copenhagen, Denmark
- 5Faculty of Health and Medical Sciences, Novo Nordisk Foundation Center for Basic Metabolic Research, University of Copenhagen, Copenhagen, Denmark
- 6Nordsjællands Hospital Hillerød, University of Copenhagen, Hillerød, Denmark
- 7Clinical Metabolic Physiology, Steno Diabetes Center Copenhagen, Gentofte Hospital, University of Copenhagen, Hellerup, Denmark
- 8Department of Clinical Medicine, Faculty of Health and Medical Sciences, University of Copenhagen, Copenhagen, Denmark
The role of the autonomic nervous system in the efficacy of glucagon-like peptide-1 receptor agonists (GLP-1 RA) in patients with type 1 diabetes is unknown. We assessed the association between autonomic function and weight loss induced by the GLP-1 RA liraglutide.
Methods: Lira-1 was a randomized, double-blind, placebo-controlled trial assessing the efficacy and safety of 1.8 mg liraglutide once-daily for 24 weeks in overweight patients with type 1 diabetes. Autonomic function was assessed by heart rate response to deep breathing (E/I ratio), to standing (30/15 ratio), to the Valsalva maneuver and resting heart rate variability (HRV) indices. Associations between baseline the cardiovascular autonomic neuropathy (CAN) diagnosis (> 1 pathological non-resting test) and levels of test outcomes on liraglutide-induced weight loss was assessed by linear regression models.
Results: Ninety-nine patients with mean age 48 (SD 12) years, HbA1c 70 (IQR 66;75) mmol/mol and BMI of 30 (SD 3) kg/m2 were assigned to liraglutide (N = 50) or placebo (N = 49). The CAN diagnosis was not associated with weight loss. A 50% higher baseline level of the 30/15 ratio was associated with a larger weight reduction by liraglutide of −2.65 kg during the trial (95% CI: −4.60; −0.69; P = 0.009). Similar significant associations were found for several HRV indices.
Conclusions: The overall CAN diagnosis was not associated with liraglutide-induced weight loss in overweight patients with type 1 diabetes. Assessed separately, better outcomes for several CAN measures were associated with higher weight loss, indicating that autonomic involvement in liraglutide-induced weight loss may exist.
Introduction
Glucagon-like peptide-1 (GLP-1) is a gut-derived hormone with anorexigenic properties (1). The GLP-1 receptor agonist (GLP-1RA) liraglutide is known to induce weight loss in patients with type 2 diabetes as well as in patients with type 1 diabetes (2–5). However, the exact mechanisms by which GLP-1 exerts its anorectic effects are not fully clarified. Activation of GLP-1 receptors in peripheral vagal neurons (6, 7) seems to be involved, suggesting that dysfunction of the vagal nerve may affect the body weight-reducing effect of liraglutide in patients. If this is the case, a substantial subset of people with diabetes may experience a reduced effect of treatment as autonomic neuropathy is a common complication to diabetes. Prevalence rates of cardiovascular autonomic neuropathy (CAN) in people with type 1 diabetes and type 2 diabetes, respectively, range from 20% in unselected diabetes populations (8, 9) to 65% in patients with long-standing diabetes(10). We hypothesize that autonomic dysfunction might be expected to influence the efficacy of GLP-1RAs in type 1 patients. Here, we explored the possible association between CAN measures and liraglutide-induced weight loss, insulin requirements and gastric emptying rate in patients with type 1 diabetes.
Participants and Methods
Study Design
The present study is a secondary analysis of data from the Lira-1 study (3). Lira-1 was a single-center, parallel-group, double-blinded, randomized, placebo-controlled trial performed at Steno Diabetes Center (Gentofte, Denmark). In total, 100 overweight (BMI> 25 kg/m2) patients with type 1 diabetes and insufficient glycaemic control (HbA1c > 64 mmol/mol (8%)) were randomly allocated (1:1) to receive 24 weeks of identical liraglutide 1.8 mg once daily (QD) (Novo Nordisk, Måløv, Denmark) or placebo QD (saline injection), as an add-on to existing insulin treatment. Liraglutide dose was successively increased weekly by 0.6 mg from 0.6 mg QD to 1.8 mg QD. To reduce the risk of hypoglycaemia bolus and basal insulin doses were reduced by 33 and 25% at randomization. Insulin doses were adjusted throughout the trial aiming at preprandial glucose targets of 4–7 mmol/L and a postprandial glucose of <10 mmol/L.
The study included 5 visits at weeks 0, 3, 12, 23, and 24 at which information about concomitant medication, basal and bolus insulin doses, bodyweight, blood pressure, and heart rate were collected. Blood and urine samples were collected at these visits. Details about the study design and results regarding main outcomes have been described previously (3). The study was approved by the Scientific Ethical Committee of the Capital Region of Denmark (H-1-2012-031), the Danish Medicines Authority (EudraCT: 2012-001150-26), and the Danish Data Protection Agency. The trial is registered with ClinicalTrials.gov, number NCT01612468.
Assessment of Autonomic Function
Autonomic function was assessed by measures of CAN; heart rate variability (HRV) and cardiovascular autonomic reflex tests (CARTs). Assessments were done at baseline (week 0) and at end of trial (week 24) by 5-min of passive supine recordings of HRV followed by three measures of CARTs.
HRV indices were analyzed using normal statistical description (time domain) and by estimating frequency-specific fluctuations in HRV (frequency domain) (11). HRV indices in the time domain were calculated by the root mean square of the sum of the squares of differences between consecutive R–R intervals (RMSSD) and as the standard deviation of normal-to-normal intervals (SDNN). In the frequency domain, the calculations of power were done in the low-frequency (LF) power (0.04–0.15 Hz) band, the high-frequency (HF) power (0.15–0.4 Hz) band and in the total frequency (≤ 0.4 Hz) spectrum autoregressive model. RMSSD and HF are measures of parasympathetic activity and SDNN, LF, total power and LF/HF-ratio are measures of both the parasympathetic and sympathetic activity (11).
The three standard CARTs recommended for diagnosing CAN (12) were performed: the lying-to-standing test (30/15) testing a mix of the sympathetic and the parasympathetic nervous system, the deep breathing test (E/I ratio) primarily testing parasympathetic nervous system and the Valsalva maneuver primarily testing sympathetic nervous system. CARTs were performed in the mentioned order and in accordance with procedures suggested by Ewing (13). CAN assessment was performed after 5 min of supine resting in a quiet room at 18–23 degrees Celsius. Patients were fasting and refrained from strenuous physical activity 24 h prior to the examination. Smoking was not allowed 3 h prior to testing.
All CARTs and HRV measures were analyzed as continuous variables. Age-dependent cut-off levels as recommended by Spallone et al. (14) were used to define pathological results of CARTs. Manifest CAN diagnosis was defined as having more than one pathological CARTs as recommended by the American Diabetes Association (15). Higher values of the all autonomic measures imply better autonomic function.
Resting HRV indices and CARTs were recorded by trained technicians using a Vagustm device (Medicus Engineering, Aarhus, Denmark). The Vagus device is a handheld device that enables the measurement of R-R intervals of a two-point electrocardiogram. Patients connects to the device by holding on to two electrodes at each end. Measure are obtained automatically. Instructions of breathing frequency, when to rise and when to blow though a mouthpiece at 40 mmHg are given on a display on the device (16).
Assessment of Gastric Emptying Rate
The first 40 patients included in the Lira-1 trial were subjected to a standardized liquid mixed meal test (Nutridrink Protein (Nutricia, Schiphol, Netherlands); 200 mL containing 300 kcal, 31,2 g carbohydrate, 20 g protein, and 10,6 g fat) at week 0, 3 and 24. Gastric emptying rate was assessed by the paracetamol absorption test (17).
Statistical Methods
Patient characteristics are presented as means with standard deviations (SD), as medians with interquartile range (IQR) for characteristics with a skewed distribution or as percentages.
Outcomes were repeated measurements of body weight in kilograms, daily insulin requirements (units/day) andgastric emptying assessed by the paracetamol absorption test. CAN measures as continuous variables and the CAN diagnosis as a binary variable were used as determinants. All analyses including HRV were adjusted for 5 min resting heart rate at the time of testing. Analyses of HRV were also performed without adjustments for heart rate to assess the effect of heart rate on estimates. The trapezoidal rule was used to calculate AUC0−240min during the meal test (3).
Associations were modeled by linear mixed-effect models with a patient-specific random intercept to account for the correlation of repeated measurements within patients. We tested for a modifying effect of having the CAN diagnosis at baseline or not on liraglutide-induced changes in outcomes. Also, the modifying effect of increasing (better) levels of CAN measures at baseline on the effect of liraglutide-induced changes in outcomes was tested. All analyses were performed as an intention to treat analysis. To fulfill the requirement of a normal distribution of the model residuals, all determinants were log1.5-transformed and the following outcomes were log-transformed: total insulin requirements per day and measures of gastric emptying. For log-transformed outcomes, estimates are presented as % change due to subsequent back transformation of results in log-scale. Estimates are therefore a function of a 50% higher level of determinants at baseline on outcomes, e.g. liraglutide-induced weight loss. An increase of 50% was chosen to assess a clinically relevant difference in autonomic measures. Where relevant, analyses of a 50% higher change in determinants during the trial period were assessed. Standardized regression coefficients for log1.5-transformed CARTs and HRV measures were further calculated to allow for direct comparison between the parameter estimates.
Group differences (liraglutide vs. placebo) in change in continuous CAN measures between baseline and end of trial were assessed by linear regression analyses adjusting for baseline values of the CAN measure analyzed.
Statistical significance was inferred at a two-tailed P-value < 0.05.
Analyses were performed using SAS version 9.4 (SAS Institute, Cary, NC) and R version 3.2.1 (The R Foundation for Statistical Computing, www.R-project.org).
Results
Of the 100 patients enrolled in the Lira-1 trial, one patient in the placebo group had no usable CAN measures, leaving 50 patients in the liraglutide and 49 patients in the placebo arm for analysis. During the trial four patients assigned to liraglutide treatment and six patients in the placebo arm discontinued the trial.
Patients were predominantly male (60% in the liraglutide group and 67.3% in the placebo group) with a mean age of 48 (SD 12) years, a median HbA1c of 70 (IQR 66;75) mmol/mol and a mean BMI of 30 (SD 3.5) kg/m2. Baseline demographic, anthropometric and cardio-metabolic markers were similar in the two groups except for diabetes duration which on average was 4 years longer in the placebo group. At baseline, 15 patients (30%) in the liraglutide group and 12 patients (25%) in the placebo group had the CAN diagnosis. CARTs and HRV measures were similar in the two groups (Table 1).
Weight Loss
In the liraglutide group, patients with and without CAN had similar weight change of −6.08 kg (95% CI −11.76;-0.38) vs. −5.77 kg (95% CI −13.51;1.98), respectively (P = 0.438 for between-group difference).
The effect of the CAN diagnosis at baseline on weight loss were similar in the liraglutide group and the placebo group (P = 0.513 for group difference).
In the liraglutide group a 50% higher baseline 30/15 ratio was associated with a larger weight change of −2.65 kg (95% CI −4.60; −0.69 P = 0.009). Similar numerical results were found for the E/I ratio and the Valsalva maneuver and liraglutide induced weight loss but did not reach statistical significance, no differences between groups were observed (Table 2).
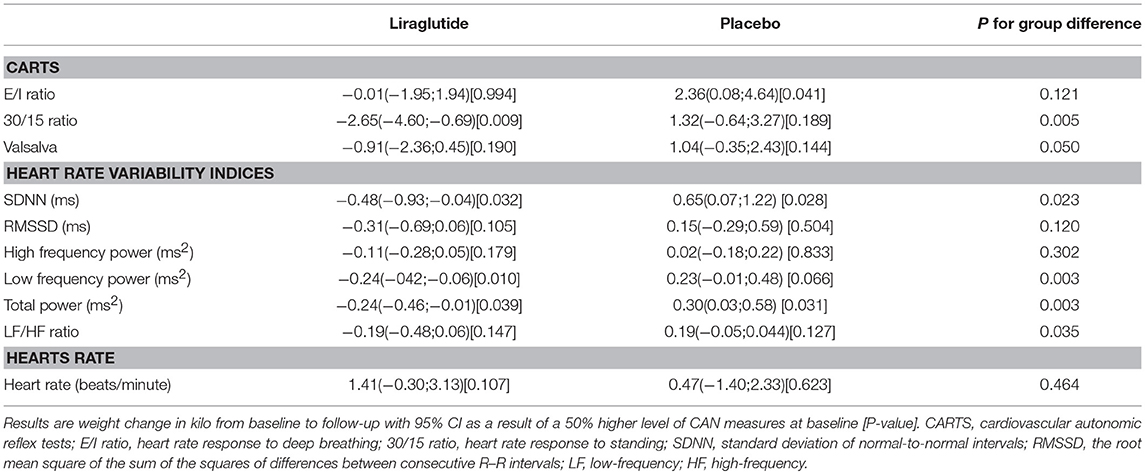
Table 2. The association between CAN measure and weight change (measured in kilo from baseline to follow-up).
In patients treated with liraglutide, higher baseline HRV indices SDNN, LF, and total power were significantly associated with larger weight loss during the trial. For the remaining HRV indices a similar but non-significant association to liraglutide-induced weights loss was seen. In the placebo group, higher baseline SDNN and total power were associated with body weight gain during trial. Resting heart rate was not associated with body weight change in any of the groups (Table 2). As illustrated in Figure 1, increasing levels of CAN measures associated with weight change had similar effects on weight change elicited by liraglutide when assessed in standardized regression models.
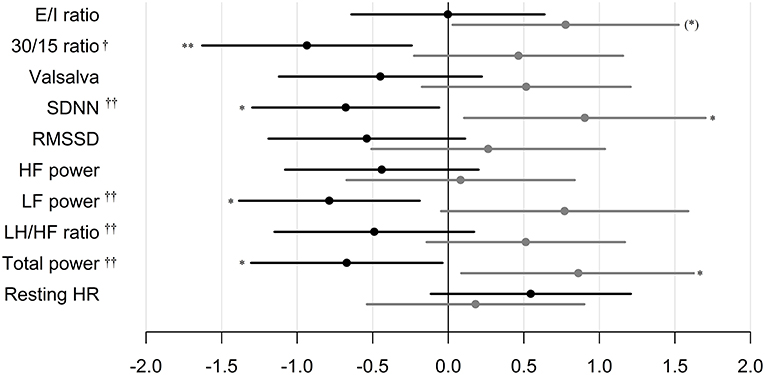
Figure 1. Standardized regression coefficients with 95% CL of the associations between CAN measures and weight change during trial. Estimates are in kilo on the log-scale by one SD increase in log1.5 of the determinant. Black: Liraglutide group. Gray: placebo group. E/I ratio, heart rate response to deep breathing; 30/15 ratio, heart rate response to standing; SDNN, standard deviation of normal-to-normal intervals; RMSSD, the root mean square of the sum of the squares of differences between consecutive R–R intervals; LF, low-frequency; HF, high-frequency. P-value for between group difference indicated by † = <0.01, †† = <0.005, P-value for change within group indicated by *P < 0.05, ** P < 0.01. * in brackets indicate no group difference.
In the placebo group, patients with and without CAN had similar weight change of −1.05 kg (95% CI −8.30; 6.20) vs. 0.67 kg (95% CI −4.10; 5.44), respectively (P = 0.0993 for between-group difference).
Insulin Requirements
CAN diagnosis was not associated with changes in insulin requirements in any of the groups. Neither, CARTs, HRV indices or resting heart rate were associated with changes in insulin requirements in any of the group (Table 3 and Figure 2).
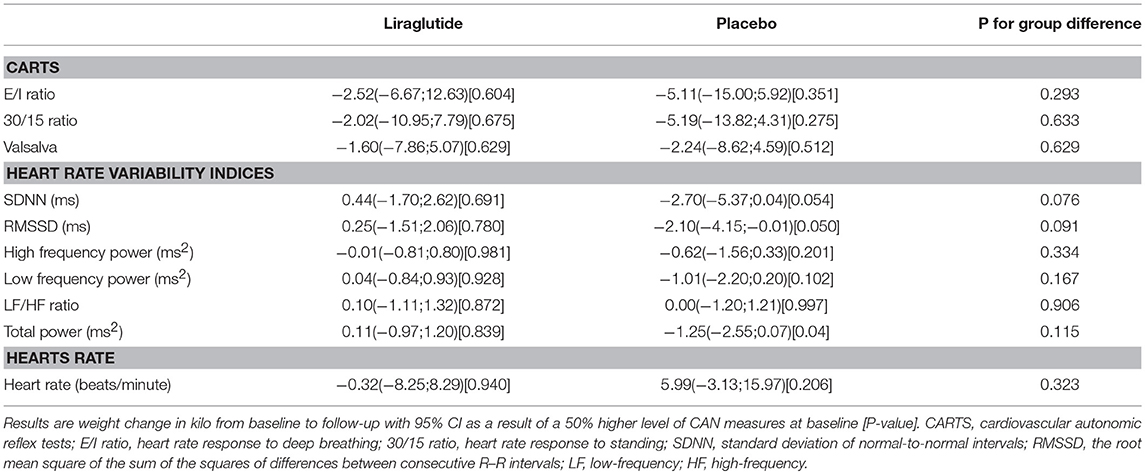
Table 3. The association between CAN measures and change in insulin use (in percentage) during trial.
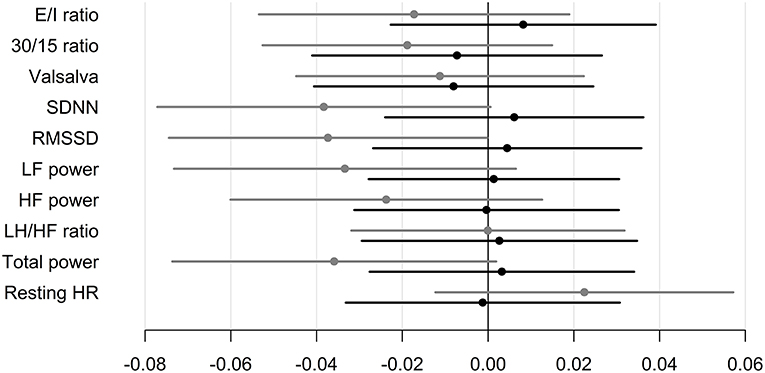
Figure 2. Standardized regression coefficients with 95% CL of the associations between CAN measures and change in insulin requirements during trial. Estimates are in IU per day on the log-scale by one SD increase in log1.5 of the determinant. Black: Liraglutide group. Gray: placebo group. E/I ratio, heart rate response to deep breathing; 30/15 ratio, heart rate response to standing; SDNN, standard deviation of normal-to-normal intervals; RMSSD, the root mean square of the sum of the squares of differences between consecutive R–R intervals; LF, low-frequency; HF, high-frequency.
Gastric Emptying
In the subset of 40 patients who underwent liquid mixed meal testing, liraglutide induced a delay in gastric emptying rate measured by paracetamol as reported earlier (3). The CAN diagnosis at baseline did not affect the change in gastric emptying rate induced by liraglutide when assessed by total AUC0−240min (P = 0.406).
In the liraglutide group a 50% higher level of E/I ratio at baseline was associated with lower effect of liraglutide on paracetamol AUC0−240min of −15.10 mmol/L x min (95% CI −26.78; −1.56 P = 0.034). No other baseline autonomic measures were associated with gastric emptying (Table 4).
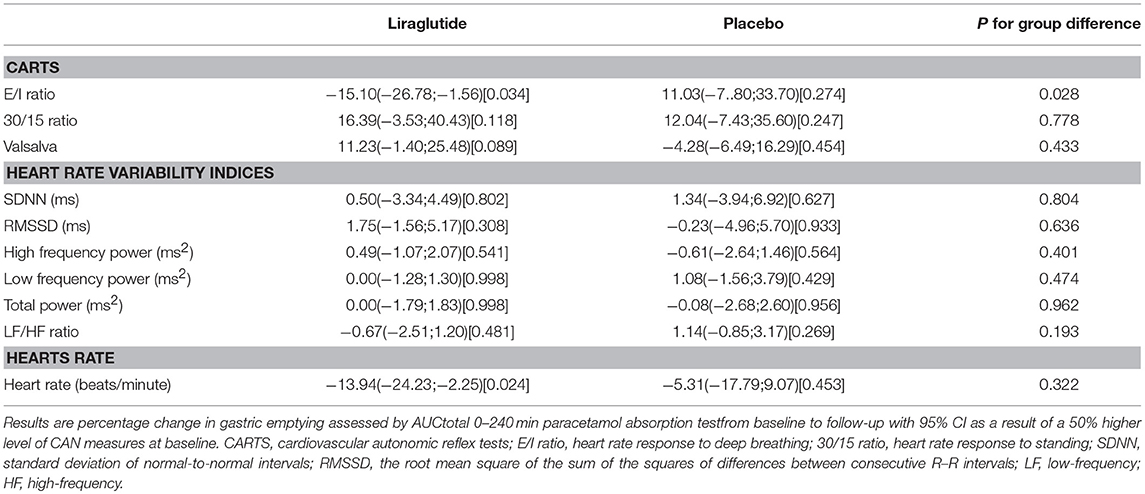
Table 4. CAN measures and association to gastric emptying assessed by AUC0−240min of serum paracetamol.
A 50% increase in paracetamol AUC0−240min during trial was associated with a decrease in body weight of 5.8% (95% CI 1.4; 10.0 P = 0.016) in the liraglutide group.
CAN Measures
Liraglutide treatment did not elicit changes in CAN measures from baseline to end of trial, but increased resting heart rate by 8 beats per min. compared to placebo as described previously (3). A study effect on the 30/15 ratio was seen as a decrease from baseline to follow-up of 0.06 (95% CI −0.11;0.02, P = 0.004 in the lira group and P = 0.007 in the placebo group) in both treatment groups, with no between-group difference (P = 0.900). All other measures of CAN and resting heart rate remained unchanged in the placebo group throughout the trial (Table 5).
Discussion
The CAN diagnosis per se was not associated with liraglutide induced weight loss. This could be attributed to the composite nature of the CAN diagnosis which is comprised by a diverse array of autonomic measures, limiting the ability to assess the individual components of the autonomic nervous system (18). On the other hand, our findings may suggest that autonomic function is associated with liraglutide-induced weight loss in overweight patients with type 1 diabetes and insufficient glycaemic control. We found that higher baseline values of mixed parasympathetic and sympathetic measures (the 30/15 ratio and the HRV indices SDNN, LF power and total power) were associated with increased liraglutide induced weight loss. Specific parasympathetic measures were not significantly associated with weight loss in the liraglutide group, which indicates that the modifying effect of autonomic function on liraglutide induced weight loss is not solely mediated by vagal function, but rather by a complex interplay between the sympathetic and parasympathetic nervous system.
The lack of association between the CAN diagnosis and liraglutide-induced weight loss may indicate the activation of peripheral autonomic nerves is not a prerequisite for GLP-1 RA-induced weight-loss. Direct stimulation of the central nervous system may be required for weight loss.
Native GLP-1 and liraglutide may not directly pass the blood brain barrier, but may access certain regions of the brain via the circumventricular organs (19–22), and it has been suggested that exogenous GLP-1 may in this way have a direct effect on receptors in the central nervous system and thereby induce weight loss by increased satiety (23, 24). In mice, liraglutide effects on food intake were abolished after genetic deletion of brain receptors, but remained after deletion of peripheral autonomic nerves (25). In summary, our results do not rule out that the peripheral autonomic nervous system plays a role in liraglutide induced weight loss.
A potential cause of liraglutide induced weight loss could be the reduction in insulin use seen in the liraglutide group (3). However, autonomic function was not associated with insulin requirements during trial.
Only baseline E/I ratio was associated with liraglutide induced deceleration of gastric emptying, indicating that vagal nerve function might be associated with the effect of liraglutide on gastric motility. Earlier studies have shown that GLP-1-induced deceleration of gastric emptying is lost after vagotomy in non-diabetic individuals (26) suggesting that the effect of GLP-1 on gastric emptying is mediated via the vagus nerve as indicated by our findings. Presently, however it remains controversial whether liraglutide's effect on gastric emptying contributes to liraglutide-induced weight loss (27, 28). As the baseline E/I ratio was not associated with weight loss, and as no other CAN measure were associated with gastric emptying, we conclude that the association between baseline CAN measures and liraglutide induced weight loss was not mediated through gastric emptying rate.
As CAN measures were not affected by liraglutide or placebo treatment in this trial it is unlikely that associations between baseline measures of CAN and study outcomes are a result of changes in autonomic nervous function. The E/I ratio did decline in both study arms at comparable magnitudes which could be attributed to an overall study effect. In contrast, a recent study in type 2 diabetes patients reported that 12 weeks of liraglutide treatment induced detrimental reductions in several HRV indices (29). However, 18 months of treatment with the short-acting GLP1-RA exenatide had no effect on CAN measures in patients with type 2 diabetes (30) which is in line with the findings of the present study.
As the study was not designed to assess the association between CAN and the efficacy of liraglutide it may be underpowered to show significant association on more CAN parameters than presented here.
Measures of autonomic function used in the present study are not purely associated to either branch of the autonomic nervous system, which hampers the ability to conclude on associations specifically related to either the sympathetic or the parasympathetic nervous system. Measures of blood pressure changes as a response to Valsalva maneuver and active standing could have enabled a more specific assessment of sympathetic nervous function.
The present study is a post-hoc analyses of data from a randomized controlled trial designed to estimate the efficacy of liraglutide in overweight patients with type 1 diabetes. This study was not designed or powered to assess differences in efficacy in patients with or without autonomic dysfunction. Hence, the lack of a modifying effect of the CAN diagnosis on the efficacy of liraglutide-induced weight change may be due to lack of power. Confirmatory studies where participants are stratified by CAN status must be performed before conclusions can be made on whether autonomic neuropathy affects the weight-lowering effect of GLP-1 RAs.
In conclusion, our results suggest that weight loss induced by liraglutide in type 1 diabetes patients is not associated with CAN diagnosis, but weight loss may in part be modulated by autonomic function not solely attributed to vagal function.
Ethics Statement
The study was approved by the Scientific Ethical Committee of the Capital Region of Denmark (H-1-2012-031), the Danish Medicines Authority (EudraCT: 2012-001150-26), and the Danish Data Protection Agency. The trial is registered with ClinicalTrials.gov, number NCT01612468.
Author Contributions
TD, LT, FK, and HA initiated and designed the trial. CH, JF TD, CF, LT, JH FK, SM, and HA participated in data collection and interpretation of data. CH, JF, and DV did the statistical analysis. CH wrote the first draft of the manuscript. All authors have revised the manuscript for crucial intellectual content.
Funding
The original study was funded by an unrestricted grant by Novo Nordisk a/s. This pot-hoc study was funded by internal research funds at Steno Diabetes Center Copenhangen.
Conflict of Interest Statement
CF has received research support and lecture fees from Novo Nordisk. JF is consulted and stock owner in Medicus Engineering and received lecture fees from Eli Lilly. DV and LT own stocks in Novo Nordisk, have received lecture fees from and consulted for Merck Sharp & Dome and Novo Nordisk. JH has consulted for Merck Sharp & Dome, Novo Nordisk and Roche. FK has received lecture fees and/or research support from, served on advisory boards of and/or consulted for AstraZeneca, Boehringer Ingelheim Pharmaceuticals, Bristol-Myers Squibb, Eli Lilly, and Company, Gilead Sciences, Merck Sharp & Dohme, Novo Nordisk, Ono Pharmaceuticals, Sanofi and Zealand Pharma. SM has served on advisory boards for Novartis Pharma, Novo Nordisk, Merck Sharp & Dome, Sanofi-Aventis, AstraZeneca, Johnson & Johnson, Roche, Mankind, Boehringer-Ingelheim, Zeeland, Eli Lilly and Intarcia Therapeutics, and has received lecture fees from Novo Nordisk, Merck Sharp & Dome, Astra-Zeneca, Johnson and Johnson, Roche, Shering-Ploug, Sanofi-Aventis, Novartis Pharma, Eli Lilly and Bristol-Meyer Squibb. HA owns stock in Novo Nordisk and has served on advisory boards for Abbott and Novo Nordisk. TD has received research support from Novo Nordisk and AstraZeneca and lecture fees from Novo Nordisk and Boehringer-Ingelheim.
The remaining author declares that the research was conducted in the absence of any commercial or financial relationships that could be construed as a potential conflict of interest.
Acknowledgments
The Lira-1 trial was funded by an unrestricted grant from Novo Nordisk A/S. We thank the staff in the Clinical Research Unit at Steno Diabetes Center Copenhagen for assistance with collecting data and laboratory work. We also thank Sofie P. Olesen (Novo Nordisk Foundation Center for Basic Metabolic Research, Faculty of Health and Medical Sciences, University of Copenhagen, Copenhagen, Denmark) for excellent technical assistance. Finally, we thank all the patients for their participation in this trial.
Abbreviations
30/15 ratio, Heart rate response to standing; ANS, Autonomic nervous system; CAN, Cardiovascular autonomic neuropathy; CARTS, Cardiovascular autonomic reflex tests; E/I ratio, Heart rate response to deep breathing; GLP-1 RA, Glucagon-like peptide-1 receptor agonists; HF, High frequency power; HRV, Heart rate variability; LF, Low frequency power; RMSSD, Root mean square of the sum of the squares of differences between consecutive R–R intervals; SDNN, Standard deviation of normal-to-normal intervals.
References
1. Holst JJ. Incretin hormones and the satiation signal. Int J Obesity (2005). (2013) 37:1161–8. doi: 10.1038/ijo.2012.208
2. Astrup A, Rossner S, Van Gaal L, Rissanen A, Niskanen L, Al Hakim M, et al. Effects of liraglutide in the treatment of obesity: a randomised, double-blind, placebo-controlled study. Lancet. (2009) 374:1606–16. doi: 10.1016/S0140-6736(09)61375-1
3. Dejgaard TF, Frandsen CS, Hansen TS, Almdal T, Urhammer S, Pedersen-Bjergaard U, et al. Efficacy and safety of liraglutide for overweight adult patients with type 1 diabetes and insufficient glycaemic control (Lira-1): a randomised, double-blind, placebo-controlled trial. Lancet Diabetes Endocrinol. (2016) 4:221–32. doi: 10.1016/S2213-8587(15)00436-2
4. Blonde L, Russell-Jones D. The safety and efficacy of liraglutide with or without oral antidiabetic drug therapy in type 2 diabetes: an overview of the LEAD 1-5 studies. Diabetes Obes Metab. (2009) 11(Suppl 3):26–34. doi: 10.1111/j.1463-1326.2009.01075.x
5. Mathieu C, Zinman B, Hemmingsson JU, Woo V, Colman P, Christiansen E, et al. Efficacy and safety of liraglutide added to insulin treatment in type 1 diabetes: The ADJUNCT ONE treat-to-target randomized trial. Diabetes Care. (2016) 39:1702–10. doi: 10.2337/dc16-0691
6. Nakagawa A, Satake H, Nakabayashi H, Nishizawa M, Furuya K, Nakano S, et al. Receptor gene expression of glucagon-like peptide-1, but not glucose-dependent insulinotropic polypeptide, in rat nodose ganglion cells. Auton Neurosci. (2004) 110:36–43. doi: 10.1016/j.autneu.2003.11.001
7. Vahl TP, Tauchi M, Durler TS, Elfers EE, Fernandes TM, Bitner RD, et al. Glucagon-like peptide-1 (GLP-1) receptors expressed on nerve terminals in the portal vein mediate the effects of endogenous GLP-1 on glucose tolerance in rats. Endocrinology. (2007) 148:4965–73. doi: 10.1210/en.2006-0153
8. Valensi P, Paries J, Attali JR. Cardiac autonomic neuropathy in diabetic patients: influence of diabetes duration, obesity, and microangiopathic complications–the French multicenter study. Metabolism. (2003) 52:815–20. doi: 10.1016/S0026-0495(03)00095-7
9. Ziegler D, Dannehl K, Muhlen H, Spüler M, Gries FA. Prevalence of cardiovascular autonomic dysfunction assessed by spectral analysis, vector analysis, and standard tests of heart rate variation and blood pressure responses at various stages of diabetic neuropathy. Diabet Med. (1992) 9:806–14.
10. Low PA, Benrud-Larson LM, Sletten DM, Opfer-Gehrking TL, Weigand SD, O'Brien PC, et al. Autonomic symptoms and diabetic neuropathy: a population-based study. Diabetes Care. (2004) 27:2942–7. doi: 10.2337/diacare.27.12.2942
11. Heart rate variability: standards of measurement physiological interpretation and clinical use. task force of the European society of cardiology and the North American society of pacing and electrophysiology. Circulation. (1996) 93:1043–65. doi: 10.1161/01.CIR.93.5.1043
12. Pop-Busui R, Boulton AJ, Feldman EL, Bril V, Freeman R, Malik RA, et al. Diabetic neuropathy: a position statement by the American diabetes association. Diabetes Care. (2017) 40:136–54. doi: 10.2337/dc16-2042
13. Hansen CS, Jensen TM, Jensen JS, Nawroth P, Fleming T, Witte DR, et al. The role of serum methylglyoxal on diabetic peripheral and cardiovascular autonomic neuropathy: the ADDITION Denmark study. Diabet Med. (2015) 32:778–85. doi: 10.1111/dme.12753
14. Spallone V, Bellavere F, Scionti L, Maule S, Quadri R, Bax G, et al. Recommendations for the use of cardiovascular tests in diagnosing diabetic autonomic neuropathy. Nutr Metab Cardiovasc Dis. (2011) 21:69–78. doi: 10.1016/j.numecd.2010.07.005
15. Boulton AJ, Vinik AI, Arezzo JC, Bril V, Feldman EL, Freeman R, et al. Diabetic neuropathies: a statement by the American diabetes association. Diabetes Care. (2005) 28:956–62. doi: 10.2337/diacare.28.4.956
16. Ejskjaer N, Fleischer J, Fleischer J, Jacobsen PE, Poulsen PL, Nygaard H. A pocket-size device to detect autonomic neuropathy. J Diabetes Sci Technol. (2008) 2:692–6. doi: 10.1177/193229680800200421
17. Jorgensen NB, Jacobsen SH, Dirksen C, Bojsen-Moller KN, Naver L, Hvolris L, et al. Acute and long-term effects of Roux-en-Y gastric bypass on glucose metabolism in subjects with Type 2 diabetes and normal glucose tolerance. Am J Physiol Endocrinol Metab. (2012) 303:E122–31. doi: 10.1152/ajpendo.00073.2012
18. Vinik AI, Maser RE, Mitchell BD, Freeman R. Diabetic autonomic neuropathy. Diabetes Care. (2003) 26:1553–79. doi: 10.2337/diacare.26.5.1553
19. Christensen M, Sparre-Ulrich AH, Hartmann B, Grevstad U, Rosenkilde MM, Holst JJ, et al. Transfer of liraglutide from blood to cerebrospinal fluid is minimal in patients with type 2 diabetes. Int J Obesity (2005). (2015) 39:1651–4. doi: 10.1038/ijo.2015.136
20. Orskov C, Poulsen SS, Moller M, Holst JJ. Glucagon-like peptide I receptors in the subfornical organ and the area postrema are accessible to circulating glucagon-like peptide I. Diabetes. (1996) 45:832–5. doi: 10.2337/diab.45.6.832
21. Knudsen LB, Secher A, Hecksher-Sorensen J, Pyke C. Long-acting glucagon-like peptide-1 receptor agonists have direct access to and effects on pro-opiomelanocortin/cocaine- and amphetamine-stimulated transcript neurons in the mouse hypothalamus. J Diabet Invest. (2016) 7(Suppl 1):56–63. doi: 10.1111/jdi.12463
22. Secher A, Jelsing J, Baquero AF, Hecksher-Sorensen J, Cowley MA, Dalboge LS, et al. The arcuate nucleus mediates GLP-1 receptor agonist liraglutide-dependent weight loss. J Clin Invest. (2014) 124:4473–88. doi: 10.1172/JCI75276
23. Pannacciulli N, Le DS, Salbe AD, Chen K, Reiman EM, Tataranni PA, et al. Postprandial glucagon-like peptide-1 (GLP-1) response is positively associated with changes in neuronal activity of brain areas implicated in satiety and food intake regulation in humans. NeuroImage. (2007) 35:511–7. doi: 10.1016/j.neuroimage.2006.12.035
24. van Dijk G, Thiele TE. Glucagon-like peptide-1 (7-36) amide: a central regulator of satiety and interoceptive stress. Neuropeptides. (1999) 33:406–14. doi: 10.1054/npep.1999.0053
25. Sisley S, Gutierrez-Aguilar R, Scott M, D'Alessio DA, Sandoval DA, Seeley RJ. Neuronal GLP1R mediates liraglutide's anorectic but not glucose-lowering effect. J Clin Invest. (2014) 124:2456–63. doi: 10.1172/JCI72434
26. Plamboeck A, Veedfald S, Deacon CF, Hartmann B, Wettergren A, Svendsen LB, et al. The effect of exogenous GLP-1 on food intake is lost in male truncally vagotomized subjects with pyloroplasty. Am J Physiol Gastrointest Liver Physiol. (2013) 304:G1117–27. doi: 10.1152/ajpgi.00035.2013
27. van Can J, Sloth B, Jensen CB, Flint A, Blaak EE, Saris WH. Effects of the once-daily GLP-1 analog liraglutide on gastric emptying, glycemic parameters, appetite and energy metabolism in obese, non-diabetic adults. Int J Obesity (2005). (2014) 38:784–93. doi: 10.1038/ijo.2013.162
28. Halawi H, Khemani D, Eckert D, O'Neill J, Kadouh H, Grothe K, et al. Effects of liraglutide on weight, satiation, and gastric functions in obesity: a randomised, placebo-controlled pilot trial. lancet Gastroenterol Hepatol. (2017) 2: 890–9. doi: 10.1016/S2468-1253(17)30285-6
29. Kumarathurai P, Anholm C, Larsen BS, Olsen RH, Madsbad S, Kristiansen O, et al. Effects of liraglutide on heart rate and heart rate variability: a randomized, double-blind, placebo-controlled crossover study. Diabetes Care. (2017) 40:117–24. doi: 10.2337/dc16-1580
30. Jaiswal M, Martin CL, Brown MB, Callaghan B, Albers JW, Feldman EL, et al. Effects of exenatide on measures of diabetic neuropathy in subjects with type 2 diabetes: results from an 18-month proof-of-concept open-label randomized study. J Diabet Complicat. (2015) 29:1287–94. doi: 10.1016/j.jdiacomp.2015.07.013
Keywords: liraglutide, autonomic neuropathy, weight loss, insulin requirements, type 1 diabetes
Citation: Hansen CS, Frandsen CS, Fleischer J, Vistisen D, Holst JJ, Tarnow L, Knop FK, Madsbad S, Andersen HU and Dejgaard TF (2019) Liraglutide-Induced Weight Loss May be Affected by Autonomic Regulation in Type 1 Diabetes. Front. Endocrinol. 10:242. doi: 10.3389/fendo.2019.00242
Received: 04 February 2019; Accepted: 27 March 2019;
Published: 12 April 2019.
Edited by:
Åke Sjöholm, Gävle Hospital, SwedenReviewed by:
Stefan Trapp, University College London, United KingdomMario Habek, University of Zagreb, Croatia
Copyright © 2019 Hansen, Frandsen, Fleischer, Vistisen, Holst, Tarnow, Knop, Madsbad, Andersen and Dejgaard. This is an open-access article distributed under the terms of the Creative Commons Attribution License (CC BY). The use, distribution or reproduction in other forums is permitted, provided the original author(s) and the copyright owner(s) are credited and that the original publication in this journal is cited, in accordance with accepted academic practice. No use, distribution or reproduction is permitted which does not comply with these terms.
*Correspondence: Christian Stevns Hansen, Y2hyaXN0aWFuLnN0ZXZucy5oYW5zZW5AcmVnaW9uaC5kaw==