- 1Laboratory of Photobiology, Department of Ophthalmology, Keio University School of Medicine, Tokyo, Japan
- 2Laboratory of Integrative Brain Sciences, Department of Biology and Center for Medical Life Science, Waseda University, Tokyo, Japan
Since gonadotropin-inhibitory hormone (GnIH) was discovered in 2000 as the first hypothalamic neuropeptide that actively inhibits gonadotropin release, researches conducted for the last 18 years have demonstrated that GnIH acts as a pronounced negative regulator of reproduction. Inhibitory effect of GnIH on reproduction is mainly accomplished at hypothalamic-pituitary levels; gonadotropin-releasing hormone (GnRH) neurons and gonadotropes are major targets of GnIH action based on the morphological interaction with GnIH neuronal fibers and the distribution of GnIH receptor. Here, we review molecular studies mainly focusing on the signal transduction pathway of GnIH in target cells, GnRH neurons, and gonadotropes. The use of well-defined cellular model systems allows the mechanistic study of signaling pathway occurring in target cells by demonstrating the direct cause-and-effect relationship. The insights gained through studying molecular mechanism of GnIH action contribute to deeper understanding of the mechanism of how GnIH communicates with other neuronal signaling systems to control our reproductive function. Reproductive axis closely interacts with other endocrine systems, thus GnIH expression levels would be changed by adrenal and thyroid status. We also briefly review molecular studies investigating the regulatory mechanisms of GnIH expression to understand the role of GnIH as a mediator between adrenal, thyroid and gonadal axes.
Introduction
Gonadotropin-inhibitory hormone (GnIH) was initially isolated from the Japanese quail hypothalamus that inhibited gonadotropin release from the cultured quail anterior pituitary; this was the first demonstration of a hypothalamic neuropeptide directly inhibiting gonadotropin release in any vertebrate (1). GnIH peptides have since been identified in all vertebrate classes, and these share an LPXRFamide (X = L or Q) motif at their C-termini (2–4), thus also known as RFamide-related peptides (RFRPs). In mammals, GnIH precursor gene is translated and cleaved into at least two peptides, RFRP1 and 3 (2–4). Not only the presence of GnIH/RFRP peptides, but their function to inhibit gonadotropin secretion is also conserved across mammals, including mice, rat and humans (2, 3, 5–8).
Two G protein-coupled receptors, GPR147 and GPR74 have been identified as GnIH receptors (GnIH-Rs) (9–12). Yin et al. identified that membrane fraction of COS-7 cells transfected with quail GPR147 binds specifically to GnIH (12). Ikemoto and Park cloned GnIH-Rs in the chicken; GPR147 cDNA was only expressed in the brain and pituitary, whereas GPR74 cDNA was ubiquitously expressed in various tissues (11). In mammals, Hinuma et al. identified a specific receptor for RFRP and named it OT7T022, which was identical to GPR147 (10). Bonini et al. reported two GPCRs for neuropeptide FF (NPFF), which has PQRFamide motif at its C-terminal, NPFF1 (identical to GPR147) and NPFF2 (identical to GPR74) (9). From the higher GnIH binding affinity for GPR147 than GPR74, GPR147 is thought to be the principal receptor for GnIH (9, 11). GnIH-R couples to Gαi, which inhibits the activity of adenylate cyclase (AC), thus reducing intracellular cAMP levels and protein kinase A (PKA) activity (10, 13–15). Cell bodies of GnIH neurons are located in the paraventricular nucleus (PVN) in birds (1, 16, 17) and in the dorsomedial hypothalamic area (DMH) in most mammals (10, 18–21). The projection of GnIH neurons to gonadotropin-releasing hormone (GnRH) neurons is the most conserved property of GnIH neurons. GnIH neuronal axon terminals contact with GnRH neurons in axo-somatic as well as axo-dendritic contacts, that express GnIH-R in the preoptic area (POA) (18, 21–25). GnIH neuronal fibers are also observed in the median eminence to control anterior pituitary function via GnIH-R expressed in gonadotropes (1, 6, 7, 17, 22, 26, 27).
As reviewed elsewhere (2, 3, 8, 15, 28–31), much evidence now supports the notion of GnIH as a key neurohormone to inhibit reproduction by regulating the hypothalamic-pituitary function. Recent studies for deeper understanding of the detailed molecular mechanisms of GnIH action have reinforced the physiological significance of GnIH in reproductive regulation. Here, we address selective studies demonstrating the GnIH action mechanism uncovered by using cellular and molecular model systems.
Potential Signaling Pathways That Convey the Inhibitory Action of GnIH in GnRH Neurons
Regulators of GnRH Neuronal Function
GnRH is the final output of the brain that regulates reproduction by stimulating gonadotropin secretion, thus GnRH neuronal functions are finely tuned by various stimulatory and inhibitory signals. There is strong evidence supporting a direct suppressive effect of GnIH on GnRH neuronal activities. Direct application of GnIH to hypothalamic brain slices decreases the firing rate of a subpopulation of GnRH neurons (32) and a direct postsynaptic inhibition of GnRH neuronal firing may occur via GnIH-mediated hyperpolarization of K+ channels in vGluT2-GnRH neurons (33). Similarly, intracerebroventricular administration of GnIH suppresses c-Fos immunoreactivity in GnRH neurons (34).
Following the discovery of GnIH, kisspeptin, encoded by the kiss1 gene (35), was demonstrated to play an important role in the up-regulation of the reproductive system in mammals (36–38). In contrast to GnIH actions, kisspeptin treatment potently activates electrical firing of GnRH neurons in hypothalamic slices (39, 40). Kisspeptin neurons make close contact with GnRH neurons acting at both the cell body and the nerve terminals (41, 42). The majority of GnRH neurons express the receptor for kisspeptin, GPR54 (43), which couples to Gαq/11 to activate phospholipase C and Ca2+ mobilization (44). Numerous studies have shown that kisspeptin acts as a key stimulatory regulator of the GnRH system (45).
Neurons synthesizing vasoactive intestinal polypeptide (VIP) are located in the suprachiasmatic nucleus (SCN) core sub-region and have monosynaptic connections with GnRH neurons (46, 47). GnRH neurons express the VIP/PACAP receptor subtype 2 (VPAC2) (48), which is preferentially coupled to the Gαs signal transduction pathway that leads to accumulation of cAMP (49). VIP-targeted GnRH neurons preferentially express c-Fos during the afternoon of the luteinizing hormone (LH) surge on the day of proestrus (50, 51), and blocking VIP signaling via in vivo antisense antagonism abolishes GnRH/c-Fos activation in ovariectomized, estradiol-treated female rats (52, 53). Additionally, electrical responses of GnRH neurons to exogenous VIP exhibited peak activity around the predicted onset of the LH surge (54). Together, these lines of evidence suggest that VIP may facilitate GnRH release that leads to the preovulatory LH surge.
Possible Interaction Between GnIH and Kisspeptin Signalings
From the opposite effects of kisspeptin and GnIH on the GnRH neuronal system, the interaction of their signal transduction pathways is expected to finetune the GnRH neuronal activity. The use of well-defined in vitro GnRH neuronal model system allows to examine their possible interaction occurring in GnRH neurons. GT1-7 is a clonal line of mature GnRH neurons of mouse hypothalamus (55). GT1-7 cells exhibit neuronal morphology with synapse formation and secrete mature GnRH in a pulsatile fashion, similar to GnRH neurons in vivo (55, 56). GT1-7 cells express GnIH-Rs, GPR147 and GPR74, as well as GPR54 (57–60), and the stimulatory effect of kisspeptin on GnRH system in GT1-7 cells has been demonstrated (57, 61–63). However, there was yet no evidence for direct inhibitory effect of GnIH on kisspeptin-induced signaling pathway in GnRH neurons. As the major downstream signaling events induced by kisspeptin/GPR54 in GT1-7 cells, Ca2+ mobilization-related nuclear factor of activated T-cells response element (NFAT-RE) activity and protein kinase C (PKC)-mediated extracellular-signal-regulated kinase (ERK)/mitogen activated protein kinase (MAPK) activity has been identified. However, it has been shown that GnIH has no inhibitory effect on these activities, even if GPR147 is overexpressed (60) (Figure 1). Although GnIH does not directly inhibit Gαq/11-mediated activities induced by kisspeptin in GT1-7 cells, there is strong evidence showing that GnIH may be involved in Ca2+ or PKC-related signaling pathway. Clarke et al. have found that ovine GnIH (RFRP3) potently blocks the generation of intracellular free Ca2+ in the pituitary elicited by GnRH, although they did not directly investigate the site of ovine GnIH action within the Ca2+ system (26). Reversely, Nichols et al. have shown that PKC inhibitor blocks human RFRP1 activity in cardiomyocytes, suggesting that RFRP1 activates PKC pathway to modulate cardiac contractile performance (64). Nevertheless, the effect of GnIH on kisspeptin-induced Ca2+ or PKC pathway has not yet been verified.
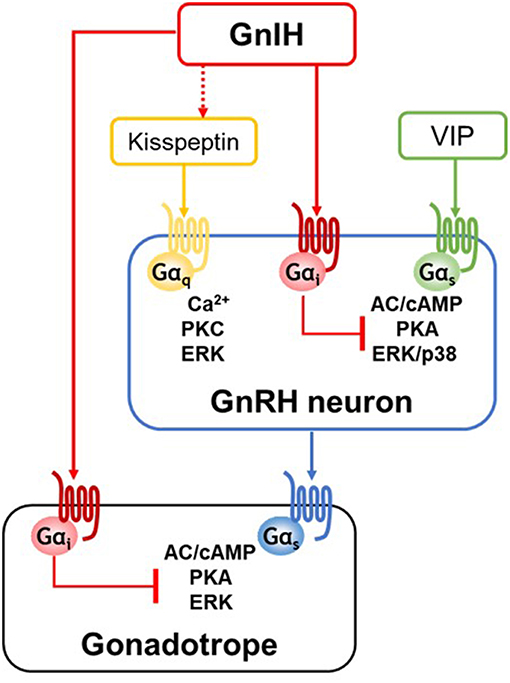
Figure 1. A schematic representation of GnIH action at the hypothalamic-pituitary levels. GnIH neurons project to hypothalamic GnRH neurons and pituitary gonadotropes, and GnIH directly acts via Gαi-coupled GPR147 or GPR74 expressed in its target cells. GnRH neurons are activated by kisspeptin or vasoactive intestine peptide (VIP) stimulation. GnIH has no direct inhibitory effect on kisspeptin/Gαq-coupled GPR54-induced Ca2+ or PKC pathway. Although kisspeptin/GPR54 pathway is not the direct target of GnIH action, GnIH may regulate kisspeptin neuronal activity via direct fiber contact and GnIH receptor expressed in kisspeptin neurons. On the other hands, GnIH effectively inhibits VIP/Gαs-coupled VPAC2-induced pathway by specifically acting on adenylate cyclase (AC)/cAMP/protein kinase A (PKA)-dependent pathway. In gonadotropes, GnIH exerts its inhibitory effect via AC/cAMP/PKA pathway, thus Gαs-coupled GnRH receptor signaling is specifically inhibited by GnIH.
A study using another GnRH neuronal cell model, mHypoA-GnRH/GFP, generated from adult-derived GnRH-GFP neurons, shows the interaction of GnIH and kisspeptin on GnRH transcriptional regulation (65). In mHypoA-GnRH/GFP cells, treatment of GnIH attenuates basal GnRH mRNA expression, whereas kisspeptin induces GnRH levels. Co-treatment of GnIH and kisspeptin suppresses GnRH mRNA expression, suggesting the inhibitory effect of GnIH may override the stimulatory effect of kisspeptin on GnRH mRNA expression. By using transcriptional inhibitors (actinomycin D and DRB), Gojska et al. further show that GnIH-mediated repression is involved in new RNA synthesis rather than affecting the stability of pre-existing GnRH mRNA in mHypoA-GnRH/GFP cells (65). Although they present a novel action mechanism of GnIH on GnRH transcriptional regulation, these results do not indicate the direct inhibitory effect of GnIH on kisspeptin-induced signaling pathway.
Interestingly, it was shown that GnIH effectively suppresses kisspeptin-induced GnRH release in hypothalamic culture of adult mice (60). This phenomenon may be a result from the inhibition of GnIH effects on exocytosis of GnRH, not on kisspeptin/GPR54 signaling pathway in GnRH neurons. It would be also explained by the action of GnIH on kisspeptin neurons. Compared with GnRH neuronal cell models, there exist several neuronal networks for the actions of GnIH and kisspeptin controlling GnRH release in hypothalamic culture, similar to the in vivo environment. In this respect, GnIH may not directly interfere with the stimulatory effect of kisspeptin on GnRH neurons; but rather regulate the kisspeptin neuronal activity leading to GnRH release. It has been shown that ~25% of kisspeptin neurons in the arcuate nucleus express GPR147 or GPR74, where ~35% of arcuate kisspeptin cells received GnIH fiber contacts (66), suggesting a regulatory role of GnIH-mediated signaling in arcuate kisspeptin neurons.
Inhibitory Action of GnIH on VIP/VPAC2 Signaling and its Physiological Significance
GT1-7 cells specifically express VPAC2 but not VPAC1 likewise GnRH neurons in situ and well respond to VIP stimulation (60, 61, 67, 68). Our recent study using GT1-7 cells clearly shows that GnIH suppresses the stimulatory effect of VIP at multiple levels, cAMP-response element (CRE) activity, ERK and p38 MAPK pathways, and c-Fos expression (60). In this study, the use of pharmacological inhibitor H89, which is specific to PKA pathway, but not the PKC inhibitor GF-109203X, results in an inhibition of VIP-induced pathways. Furthermore, it has been shown that ERK and p38 pathways activated by forskolin, which raise cAMP level, are effectively inhibited by GnIH, but GnIH has no inhibitory effect on PKC activator PMA (phorbol 12-myristate 13-acetate)-induced pathways (60), demonstrating the specific inhibitory action of GnIH on the cAMP/PKA pathway in GnRH neurons as in gonadotropes (Figure 1 and see also the section Specific inhibition of GnRH-induced signaling via cAMP pathway in gonadotropes by GnIH). Supporting this specific inhibitory role of GnIH on VIP-induced pathway shown in GT1-7 cells, GnIH eliminates the stimulated effect of VIP on GnRH release in female mouse hypothalamic explants (60).
It is hypothesized that VIP input is required for appropriate LH pulse frequencies and induction of an appropriately timed LH surge (52, 69, 70). The SCN of female rats, compared to males, have significantly greater VIP innervation of GnRH neurons (47), suggestive of a specific role for VIP in the regulation of estrous cycle. The necessity of VIP in triggering the afternoon GnRH surge has been also suggested (51, 52). These findings suggest that direct VIP projections from the SCN to GnRH system positively drive the GnRH/LH surge. Given the pronounced inhibitory actions of GnIH on VIP/VPAC2 signaling in GT1-7 cells and VIP-induced GnRH release (60), it seems probable that GnIH may regulate LH surge by suppressing VIP action on GnRH neurons. The inhibitory effect of GnIH on LH surge has been reported. Treatment of female rats with GnIH results in marked inhibition of GnRH neuronal activity at the time of LH surge (34) and intravenous infusion of GnIH blocks estrogen-induced LH surge in ewes (71). Henningsen et al. also showed that acute intracerebroventricular injection of GnIH just before the LH surge reduces the LH surge amplitude in female Syrian hamster (72). These findings have demonstrated the inhibitory role of GnIH on the amplitude of GnRH/LH surge, although the direct relationship between GnIH and VIP has not been investigated. Notably, SCN-derived VIP fibers project to GnIH neurons in female Syrian hamster, and central administration of VIP reduced c-Fos immunoreactivity in GnIH neurons in a time-dependent manner (73), indicating the possible SCN regulation of GnIH activity by VIP to control the timing of LH surge. Future studies are required to fully demonstrate the physiological relevance of interaction between GnIH and VIP on the timing and amplitude of GnRH/LH surge.
Inhibitory Mechanism of GnIH Action in Gonadotropes
Regulators of Pituitary Gonadotrope Activity
In addition to the role of GnIH at the hypothalamic level, GnIH neurons also project to the median eminence to control anterior pituitary function via GnIH-R expressed in gonadotropes (6, 7, 26, 27). On the other hand, there are relatively few or no GnIH fibers in some birds (74) and rodents (18, 21, 75), and GnIH has no direct inhibitory effect on LH secretion by the pituitary gonadotropes (34, 75, 76). Although there is some debate whether GnIH can directly act on the pituitary in some species, GnIH decreases the synthesis and/or release of pituitary gonadotropins, LH and follicle-stimulating hormone (FSH) in many species (24, 26, 77–80).
GnRH is the major activator of gonadotropes. Upon binding to its receptor (GnRH-R) on gonadotropes, GnRH stimulates the synthesis and release of LH and FSH (81). GnRH-R is a member of GPCR family (82). Most of the biological actions of GnRH are mediated by Gαq/11-coupled pathway (83). However, GnRH signaling may not be exclusively linked to Gαq/11-pathway, but also involve other pathways depending on the cell context (84). In fact, GnRH-R was shown to be coupled to Gαs (85, 86). In primary pituitary culture, rat pituitary-derived G-GH3 cells and mouse gonadotrope LβT2 cells, GnRH-R couples to Gαs as well as Gαq/11, whereas in αT3-1 pituitary precursor cells, CHO-K1 and COS-7 cells, GnRH-R seems to couple exclusively to Gαq/11 (87–89). Several studies have also suggested a physiological role of cAMP as a mediator of GnRH actions via Gαs-coupled pathway in the pituitary gland. A cell-permeable peptide that uncouples Gαs from receptors is able to inhibit ERK and c-Fos activation, and LHβ expression in LβT2 cells, indicating that Gαs is involved in GnRH-R signaling (86).
Specific Inhibition of GnRH-Induced Signaling via cAMP Pathway in Gonadotropes by GnIH
It was shown that GnIH inhibits gonadotropin synthesis and/or release from cultured pituitaries in birds (1, 90) and mammals (79, 91, 92). Based on the characteristic of Gαi-coupled GnIH-Rs, it is expected that GnIH inhibits cAMP-related signaling pathways triggered by GnRH in gonadotropes. Using the LβT2 gonadotrope model system, the detailed mechanisms underlying the inhibitory effect of GnIH on gonadotropin synthesis has been investigated (93). LβT2 cells exhibit the characteristics of fully differentiated gonadotropes, including the expression of LH, FSH, and GnRH-R as well as displaying the appropriate responses to GnRH with dose-dependent increase in LH secretion (94–96). Furthermore, LβT2 cells express both GPR147 and GPR74 (59, 93), indicating that LβT2 is an appropriate cellular model system to investigate GnIH action occurring in gonadotropes.
In this study using LβT2 cells, GnRH treatment activates CRE activities, and GnIH effectively suppresses GnRH-induced CRE activities in a dose-dependent manner as well as cAMP production (93). GnIH also inhibits the downstream ERK phosphorylation via AC/PKA-dependent manner (Figure 1). The AC/cAMP/PKA-dependent inhibitory effect of GnIH are also demonstrated in GnRH-stimulated transcriptions of gonadotropin subunit genes, LHβ, FSHβ, and common α subunit. The inhibitory effect of GnIH on GnRH-induced CRE activity, ERK phosphorylation, and gonadotropin expression leads to reduction in LH levels in LβT2 cells. This study suggests that as in GnRH neurons (described in section Inhibitory action of GnIH on VIP/VPAC2 signaling and its physiological significance), GnIH specifically acts via cAMP pathway in its target cells (93).
Common GnIH Inhibitory Mechanism in its Target Cells
From the identification of GPR147 and GPR74 as GnIH-Rs (9–12), suppression of cAMP production by GnIH has been shown in several studies (10, 13, 14). The precise mechanism of GnIH action in hypothalamic GnRH neurons as well as pituitary gonadotropes has been investigated in the cellular model systems through the molecular approaches on GPCR-related second messenger activity, downstream MAPK cascade, and the effect of pharmacological modulators. The results obtained by analysis of signaling pathway suggest that GnIH may play as a brake by preventing the excessive action of stimulatory inputs to maintain the balance in reproductive system. As a conserved mechanism of GnIH action, the AC/cAMP/PKA-specific inhibitory pathway has been demonstrated in hypothalamus-pituitary levels (60, 93) (Figure 1). Therefore, GnIH may govern the hypothalamic neuronal activities of GnRH by inhibiting the action of VIP and kisspeptin directly or indirectly, thus eventually reduce pituitary gonadotropin secretion. However, there are several exceptions of the GnIH effect on GnRH/LH release. There is a report showing that acute central injection of GnIH induces c-Fos expression in GnRH neurons and increases LH, FSH, and testosterone secretion in Syrian hamster (76). Similarly, GnIH has shown the dose-dependent stimulatory effect on LH secretion in adult male mice (97). In male Siberian hamsters, central administration of GnIH inhibits LH release in long day photoperiods, whereas stimulates LH release in short day, indicating that GnIH peptides finely tune LH levels in an opposite fashion across the seasons (21). Based on the complex regulation of GnIH action depending on the species/sexes, seasons and reproductive stages, future research is needed to determine when and how GnIH exerts its inhibitory or stimulatory effect in target cells.
Regulatory Mechanism of GnIH Expression by Glucocorticoid and Thyroid Hormone
Considering the role of GnIH as an upstream regulator of the hypothalamic-pituitary-gonadal (HPG) axis, abnormal GnIH expression levels may cause reproductive dysfunctions. Therefore, we discuss some endocrine regulators leading to GnIH expressional changes. See the recent review (31) for the regulatory mechanism of GnIH expression by melatonin and photoperiod (19, 21, 98).
Molecular Mechanism of Glucocorticoid-Mediated GnIH Activation
Reproductive function is suppressed under stress (99–101), suggesting the interaction between hypothalamic-pituitary-adrenal (HPA) and HPG axes. From the inhibitory role of GnIH in reproduction, the GnIH system could be a good candidate mediating stress-induced reproductive dysfunction. Supporting this, there have been several studies showing that stress activates the GnIH system in birds and mammals. In adult house sparrows, capture-handling stress shows a significant increase in the number of GnIH neurons (102). In rat, immobilization stress leads to an up-regulation of GnIH expression (103) and stressful stimuli increase the expression of c-Fos protein in GnIH neurons of the DMH (104). These results suggest that suppressive effects of stress upon reproductive functions are mediated by the hypothalamic GnIH system. The inhibitory effect of stress on reproductive function is potentially mediated by high concentrations of circulating glucocorticoids (GC) acting via the GC receptor (GR) (105, 106). In adult rat, approximately half of hypothalamic GnIH neurons express GR (103). In quail, most GnIH-positive cells express GR mRNA and 24 h treatment with corticosterone (CORT) increases GnIH mRNA expression (107).
Using a GnIH-expressing neuronal cell line, rHypoE-23 derived from rat hypothalamus (108), the detailed molecular mechanism of GC-mediated GnIH transcriptional activation has been investigated (107). rHypoE-23 cells express GR mRNA and GnIH mRNA expression is activated by 24 h CORT treatment. There exist several glucocorticoid response elements (GREs) in the upstream of rat GnIH precursor coding region. Through promoter analysis, it has been identified that −1,530 bp GRE is critical for the CORT-stimulated GR recruitment and its transcriptional activity (107) (Figure 2). This study provides a putative molecular basis for transcriptional activation of GnIH under stress by demonstrating that CORT directly induces GnIH transcription by recruitment of GR to its promoter. Another study using the rHypoE-23 GnIH neuronal cells has shown that the GC agonist, dexamethasone (DEX), which directly acts on GR, increases GnIH and GPR147 mRNA levels (109). The effect of neonatal DEX exposure on reproductive maturation has been also investigated in female mice (110). DEX-treated females have exhibited delayed pubertal onset and irregular estrus cycles with decreased GnRH mRNA expression in the POA and increased GnIH cell numbers in the DMH, suggesting that DEX-mediated activation of GnIH system may lead to inhibition of GnRH expression.
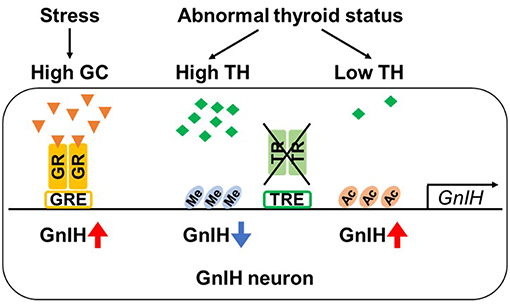
Figure 2. Regulation of GnIH promoter activity by glucocorticoid and thyroid hormone. GnIH expression is regulated by glucocorticoid (GC). GnIH neurons express GC receptor (GR) and GC-response element (GRE) is present in GnIH promoter region. Stress increases GC levels, and GC acts by binding to GR. When GC-bound GR is recruited to GRE, GnIH expression is up-regulated. GnIH expression is also actively changed by concentration of thyroid hormone (TH). Although GnIH neurons express TH receptors (TRα and β) and putative TH-response elements (TREs) exist in GnIH promoter region, TRs do not directly bind to GnIH promoter. However, thyroid status highly regulates the chromatin modification of GnIH promoter. Hypothyroidism exhibits increased GnIH expression with hyperacetylation of H3 (Ac) in promoter region. On the other hand, hyperthyroidism decreases GnIH expression associated with H3K9tri-methylation (Me).
Thyroid Hormone-Mediated GnIH Regulation by Chromatin Modification
Recently, thyroid hormones (THs; thyroxine, T4 and triiodothyronine, T3) have been suggested as a novel hormonal regulator of GnIH expression (111, 112). THs play an important role in proper development and function of the reproductive system, particularly in pubertal onset (113, 114), indicating interactions between the hypothalamic-pituitary-thyroid (HPT) and HPG axes. Therefore, thyroid disorders such as hypothyroidism and hyperthyroidism, cause abnormal puberty (115–117). Kiyohara et al. showed that hypothyroidism induced by long-term administration of propylthiouracil (PTU) in juvenile female mice leads to delayed pubertal onset with increased GnIH expression and reduced pituitary-gonadal activity, and knockout of GnIH prevents the effect of hypothyroidism to delay the pubertal onset. In contrast, hyperthyroidism induced by T4 leads to a decrease in GnIH expression, although pubertal onset was normal. Further, T3 treatment suppresses GnIH mRNA expression in hypothalamic explants. Although GnIH neurons express TH receptors, TRα and TRβ, and putative TH-response elements (TREs) are present in mouse GnIH promoter, TRs do not directly bind to GnIH promoter (111). As the molecular mechanism by which different TH concentration results in GnIH expressional changes, Kiyohara et al. have also demonstrated that the thyroid status highly regulates the chromatin modifications of GnIH promoter to activate and repress GnIH expression by H3acetylation and H3K9tri-methylation, respectively (Figure 2). Although to date limited information is available for the TH-mediated GnIH regulation, this study indicates a novel function of GnIH to mediate HPT-HPG interactions that contribute to proper pubertal development.
Conclusion
The endocrine systems, HPA, HPG and HPT axes are closely connected, thus hormonal imbalance leads to reproductive dysfunctions. As a key hypothalamic inhibitor, GnIH may act on the most upstream level of the HPG axis by regulating the hypothalamic GnRH and kisspeptin neurons as well as pituitary gonadotrope activity. The significance of GnIH system on reproduction has been emphasized by identifying the novel function of GnIH system and its interaction with other endocrine systems of HPA and HPT via GC and TH, respectively. Changes in GnIH expression levels by these endocrine modulators will alter GnRH neuronal activity and gonadotropin release by specifically acting on AC/cAMP/PKA pathway. Based on the complex regulatory system of endocrine interactions, it is also expected to uncover a novel involvement of GnIH system in reproductive regulation. The precise molecular mechanism for GnIH action and identification of molecular target for GnIH regulation may contribute to the development of new pharmaceuticals.
Author Contributions
YLS wrote the manuscript. TU and KT edited the manuscript.
Funding
This work was supported in part by Grants-in-Aid for Scientific Research 22132004 (KT) and 22227002 (KT) and Grants-in-Aid for JSPS Fellows 2402082 (YLS) and 15F15909 (YLS) from the Ministry of Education, Science, and Culture, Japan.
Conflict of Interest Statement
The authors declare that the research was conducted in the absence of any commercial or financial relationships that could be construed as a potential conflict of interest.
References
1. Tsutsui K, Saigoh E, Ukena K, Teranishi H, Fujisawa Y, Kikuchi M, et al. A novel avian hypothalamic peptide inhibiting gonadotropin release. Biochem Biophys Res Commun. (2000) 275:661–7. doi: 10.1006/bbrc.2000.3350
2. Tsutsui K. A new key neurohormone controlling reproduction, gonadotropin-inhibitory hormone (GnIH): Biosynthesis, mode of action and functional significance. Prog Neurobiol. (2009) 88:76–88. doi: 10.1016/j.pneurobio.2009.02.003
3. Tsutsui K, Bentley GE, Bedecarrats G, Osugi T, Ubuka T, Kriegsfeld LJ. Gonadotropin-inhibitory hormone (GnIH) and its control of central and peripheral reproductive function. Front Neuroendocrinol. (2010) 31:284–95. doi: 10.1016/j.yfrne.2010.03.001
4. Tsutsui K, Ubuka T, Bentley GE, Kriegsfeld LJ. Review: regulatory mechanisms of gonadotropin-inhibitory hormone (GnIH) synthesis and release in photoperiodic animals. Front Neurosci. (2013) 7:60. doi: 10.3389/fnins.2013.00060
5. Ukena K, Tsutsui K. Distribution of novel RFamide-related peptide-like immunoreactivity in the mouse central nervous system. Neurosci Lett. (2001) 300:153–6. doi: 10.1016/S0304-3940(01)01583-X
6. Ubuka T, Lai H, Kitani M, Suzuuchi A, Pham V, Cadigan PA, et al. Gonadotropin-inhibitory hormone identification, cDNA cloning, and distribution in rhesus macaque brain. J Comp Neurol. (2009) 517:841–55. doi: 10.1002/cne.22191
7. Ubuka T, Morgan K, Pawson AJ, Osugi T, Chowdhury VS, Minakata H, et al. Identification of human GnIH homologs, RFRP-1 and RFRP-3, and the cognate receptor, GPR147 in the human hypothalamic pituitary axis. PLoS ONE (2009) 4:e8400. doi: 10.1371/journal.pone.0008400
8. Ubuka T, Son YL, Tsutsui K. Molecular, cellular, morphological, physiological and behavioral aspects of gonadotropin-inhibitory hormone. Gen Comp Endocrinol. (2016) 227:27–50. doi: 10.1016/j.ygcen.2015.09.009
9. Bonini JA, Jones KA, Adham N, Forray C, Artymyshyn R, Durkin MM, et al. Identification and characterization of two G protein-coupled receptors for neuropeptide FF. J Biol Chem. (2000) 275:39324–31. doi: 10.1074/jbc.M004385200
10. Hinuma S, Shintani Y, Fukusumi S, Iijima N, Matsumoto Y, Hosoya M, et al. New neuropeptides containing carboxy-terminal RFamide and their receptor in mammals. Nat Cell Biol. (2000) 2:703–8. doi: 10.1038/35036326
11. Ikemoto T, Park MK. Chicken RFamide-related peptide (GnIH) and two distinct receptor subtypes: identification, molecular characterization, and evolutionary considerations. J Reprod Dev. (2005) 51:359–77. doi: 10.1262/jrd.16087
12. Yin H, Ukena K, Ubuka T, Tsutsui K. A novel G protein-coupled receptor for gonadotropin-inhibitory hormone in the Japanese quail (Coturnix japonica): identification, expression and binding activity. J Endocrinol. (2005) 184:257–66. doi: 10.1677/joe.1.05926
13. Mollereau C, Mazarguil H, Marcus D, Quelven I, Kotani M, Lannoy V, et al. Pharmacological characterization of human NPFF(1) and NPFF(2) receptors expressed in CHO cells by using NPY Y(1) receptor antagonists. Eur J Pharmacol. (2002) 451:245–56. doi: 10.1016/S0014-2999(02)02224-0
14. Gouarderes C, Mazarguil H, Mollereau C, Chartrel N, Leprince J, Vaudry H, et al. Functional differences between NPFF1 and NPFF2 receptor coupling: high intrinsic activities of RFamide-related peptides on stimulation of [35S]GTPgammaS binding. Neuropharmacology (2007) 52:376–86. doi: 10.1016/j.neuropharm.2006.07.034
15. Ubuka T, Son YL, Bentley GE, Millar RP, Tsutsui K. Gonadotropin-inhibitory hormone (GnIH), GnIH receptor and cell signaling. Gen Comp Endocrinol. (2013) 190:10–7. doi: 10.1016/j.ygcen.2013.02.030
16. Ubuka T, Ueno M, Ukena K, Tsutsui K. Developmental changes in gonadotropin-inhibitory hormone in the Japanese quail (Coturnix japonica) hypothalamo-hypophysial system. J Endocrinol. (2003) 178:311–8. doi: 10.1677/joe.0.1780311
17. Ukena K, Ubuka T, Tsutsui K. Distribution of a novel avian gonadotropin-inhibitory hormone in the quail brain. Cell Tissue Res. (2003) 312:73–9. doi: 10.1007/s00441-003-0700-x
18. Kriegsfeld LJ, Mei DF, Bentley GE, Ubuka T, Mason AO, Inoue K, et al. Identification and characterization of a gonadotropin-inhibitory system in the brains of mammals. Proc Natl Acad Sci USA. (2006) 103:2410–5. doi: 10.1073/pnas.0511003103
19. Revel FG, Saboureau M, Pevet P, Simonneaux V, Mikkelsen JD. RFamide-related peptide gene is a melatonin-driven photoperiodic gene. Endocrinology (2008) 149:902–12. doi: 10.1210/en.2007-0848
20. Legagneux K, Bernard-Franchi G, Poncet F, La Roche A, Colard C, Fellmann D, et al. Distribution and genesis of the RFRP-producing neurons in the rat brain: comparison with melanin-concentrating hormone- and hypocretin-containing neurons. Neuropeptides (2009) 43:13–9. doi: 10.1016/j.npep.2008.11.001
21. Ubuka T, Inoue K, Fukuda Y, Mizuno T, Ukena K, Kriegsfeld LJ, et al. Identification, expression, and physiological functions of Siberian hamster gonadotropin-inhibitory hormone. Endocrinology (2012) 153:373–85. doi: 10.1210/en.2011-1110
22. Bentley GE, Perfito N, Ukena K, Tsutsui K, Wingfield JC. Gonadotropin-inhibitory peptide in song sparrows (Melospiza melodia) in different reproductive conditions, and in house sparrows (Passer domesticus) relative to chicken-gonadotropin-releasing hormone. J Neuroendocrinol. (2003) 15:794–802. doi: 10.1046/j.1365-2826.2003.01062.x
23. Smith JT, Coolen LM, Kriegsfeld LJ, Sari IP, Jaafarzadehshirazi MR, Maltby M, et al. Variation in kisspeptin and RFamide-related peptide (RFRP) expression and terminal connections to gonadotropin-releasing hormone neurons in the brain: a novel medium for seasonal breeding in the sheep. Endocrinology (2008) 149:5770–82. doi: 10.1210/en.2008-0581
24. Kriegsfeld LJ, Gibson EM, Williams WP III, Zhao S, Mason AO, Bentley GE, et al. The roles of RFamide-related peptide-3 in mammalian reproductive function and behaviour. J Neuroendocrinol. (2010) 22:692–700. doi: 10.1111/j.1365-2826.2010.02031.x
25. Rizwan MZ, Poling MC, Corr M, Cornes PA, Augustine RA, Quennell JH, et al. RFamide-related peptide-3 receptor gene expression in GnRH and kisspeptin neurons and GnRH-dependent mechanism of action. Endocrinology (2012) 153:3770–9. doi: 10.1210/en.2012-1133
26. Clarke IJ, Sari IP, Qi Y, Smith JT, Parkington HC, Ubuka T, et al. Potent action of RFamide-related peptide-3 on pituitary gonadotropes indicative of a hypophysiotropic role in the negative regulation of gonadotropin secretion. Endocrinology (2008) 149:5811–21. doi: 10.1210/en.2008-0575
27. Maddineni S, Ocon-Grove OM, Krzysik-Walker SM, Hendricks GL III, Proudman JA, Ramachandran R. Gonadotrophin-inhibitory hormone receptor expression in the chicken pituitary gland: potential influence of sexual maturation and ovarian steroids. J Neuroendocrinol. (2008) 20:1078–88. doi: 10.1111/j.1365-2826.2008.01765.x
28. Ubuka T, Son YL, Tobari Y, Tsutsui K. Gonadotropin-inhibitory hormone action in the brain and pituitary. Front Endocrinol. (2012) 3:148. doi: 10.3389/fendo.2012.00148
29. Ubuka T, Son YL, Tobari Y, Narihiro M, Bentley GE, Kriegsfeld LJ, et al. Central and direct regulation of testicular activity by gonadotropin-inhibitory hormone and its receptor. Front Endocrinol. (2014) 5:8. doi: 10.3389/fendo.2014.00008
30. Tsutsui K, Ubuka T, Son YL, Bentley GE, Kriegsfeld LJ. Contribution of GnIH research to the progress of reproductive neuroendocrinology. Front Endocrinol. (2015) 6:179. doi: 10.3389/fendo.2015.00179
31. Tsutsui K, Ubuka T. How to contribute to the progress of neuroendocrinology: discovery of gnih and progress of GnIH research Front Endocrinol. (2018) 6:179. doi: 10.3389/fendo.2018.00662
32. Ducret E, Anderson GM, Herbison AE. RFamide-related peptide-3, a mammalian gonadotropin-inhibitory hormone ortholog, regulates gonadotropin-releasing hormone neuron firing in the mouse. Endocrinology (2009) 150:2799–804. doi: 10.1210/en.2008-1623
33. Wu M, Dumalska I, Morozova E, van den Pol AN, Alreja M. Gonadotropin inhibitory hormone inhibits basal forebrain vGluT2-gonadotropin-releasing hormone neurons via a direct postsynaptic mechanism. J Physiol. (2009) 587(Pt 7):1401–11. doi: 10.1113/jphysiol.2008.166447
34. Anderson GM, Relf HL, Rizwan MZ, Evans JJ. Central and peripheral effects of RFamide-related peptide-3 on luteinizing hormone and prolactin secretion in rats. Endocrinology (2009) 150:1834–40. doi: 10.1210/en.2008-1359
35. Lee JH, Miele ME, Hicks DJ, Phillips KK, Trent JM, Weissman BE, et al. KiSS-1, a novel human malignant melanoma metastasis-suppressor gene. J Natl Cancer Inst. (1996) 88:1731–7. doi: 10.1093/jnci/88.23.1731
36. de Roux N, Genin E, Carel JC, Matsuda F, Chaussain JL, Milgrom E. Hypogonadotropic hypogonadism due to loss of function of the KiSS1-derived peptide receptor GPR54. Proc Natl Acad Sci USA. (2003) 100:10972–6. doi: 10.1073/pnas.1834399100
37. Funes S, Hedrick JA, Vassileva G, Markowitz L, Abbondanzo S, Golovko A, et al. The KiSS-1 receptor GPR54 is essential for the development of the murine reproductive system. Biochem Biophys Res Commun. (2003) 312:1357–63. doi: 10.1016/j.bbrc.2003.11.066
38. Seminara SB, Messager S, Chatzidaki EE, Thresher RR, Acierno JS Jr Shagoury J.K, et al. The GPR54 gene as a regulator of puberty. N Engl J Med. (2003) 349:1614–27. doi: 10.1056/NEJMoa035322
39. Han SK, Gottsch ML, Lee KJ, Popa SM, Smith JT, Jakawich SK, et al. Activation of gonadotropin-releasing hormone neurons by kisspeptin as a neuroendocrine switch for the onset of puberty. J Neurosci. (2005) 25:11349–56. doi: 10.1523/JNEUROSCI.3328-05.2005
40. Dumalska I, Wu M, Morozova E, Liu R, van den Pol A, Alreja M. Excitatory effects of the puberty-initiating peptide kisspeptin and group I metabotropic glutamate receptor agonists differentiate two distinct subpopulations of gonadotropin-releasing hormone neurons. J Neurosci. (2008) 28:8003–13. doi: 10.1523/JNEUROSCI.1225-08.2008
41. Kinoshita M, Tsukamura H, Adachi S, Matsui H, Uenoyama Y, Iwata K, et al. Involvement of central metastin in the regulation of preovulatory luteinizing hormone surge and estrous cyclicity in female rats. Endocrinology (2005) 146:4431–6. doi: 10.1210/en.2005-0195
42. Clarkson J, Herbison AE. Postnatal development of kisspeptin neurons in mouse hypothalamus; sexual dimorphism and projections to gonadotropin-releasing hormone neurons. Endocrinology (2006) 147:5817–25. doi: 10.1210/en.2006-0787
43. Messager S, Chatzidaki EE, Ma D, Hendrick AG, Zahn D, Dixon J, et al. Kisspeptin directly stimulates gonadotropin-releasing hormone release via G protein-coupled receptor 54. Proc Natl Acad Sci USA. (2005) 102:1761–6. doi: 10.1073/pnas.0409330102
44. Kotani M, Detheux M, Vandenbogaerde A, Communi D, Vanderwinden JM, Le Poul E, et al. The metastasis suppressor gene KiSS-1 encodes kisspeptins, the natural ligands of the orphan G protein-coupled receptor GPR54. J Biol Chem. (2001) 276:34631–6. doi: 10.1074/jbc.M104847200
45. Colledge WH. Kisspeptins and GnRH neuronal signalling. Trends Endocrinol Metab. (2009) 20:115–21. doi: 10.1016/j.tem.2008.10.005
46. van der Beek EM, Horvath TL, Wiegant VM, Van den Hurk R, Buijs RM. Evidence for a direct neuronal pathway from the suprachiasmatic nucleus to the gonadotropin-releasing hormone system: combined tracing and light and electron microscopic immunocytochemical studies. J Comp Neurol. (1997) 384:569–79. doi: 10.1002/(SICI)1096-9861(19970811)384:4<569::AID-CNE6>3.0.CO;2-0
47. Horvath TL, Cela V, van der Beek EM. Gender-specific apposition between vasoactive intestinal peptide-containing axons and gonadotrophin-releasing hormone-producing neurons in the rat. Brain Res. (1998) 795:277–81. doi: 10.1016/S0006-8993(98)00208-X
48. Smith MJ, Jiennes L, Wise PM. Localization of the VIP2 receptor protein on GnRH neurons in the female rat. Endocrinology (2000) 141:4317–20. doi: 10.1210/endo.141.11.7876
49. McCulloch DA, MacKenzie CJ, Johnson MS, Robertson DN, Holland PJ, Ronaldson E, et al. Additional signals from VPAC/PAC family receptors. Biochem Soc Trans. (2002) 30:441–6. doi: 10.1042/bst0300441
50. Lee WS, Smith MS, Hoffman GE. Luteinizing hormone-releasing hormone neurons express Fos protein during the proestrous surge of luteinizing hormone. Proc Natl Acad Sci USA. (1990) 87:5163–7. doi: 10.1073/pnas.87.13.5163
51. van der Beek EM, van Oudheusden HJ, Buijs RM, van der Donk HA, van den Hurk R, Wiegant VM. Preferential induction of c-fos immunoreactivity in vasoactive intestinal polypeptide-innervated gonadotropin-releasing hormone neurons during a steroid-induced luteinizing hormone surge in the female rat. Endocrinology (1994) 134:2636–44. doi: 10.1210/endo.134.6.8194489
52. Harney JP, Scarbrough K, Rosewell KL, Wise PM. In vivo antisense antagonism of vasoactive intestinal peptide in the suprachiasmatic nuclei causes aging-like changes in the estradiol-induced luteinizing hormone and prolactin surges. Endocrinology (1996) 137:3696–701. doi: 10.1210/endo.137.9.8756535
53. Gerhold LM, Rosewell KL, Wise PM. Suppression of vasoactive intestinal polypeptide in the suprachiasmatic nucleus leads to aging-like alterations in cAMP rhythms and activation of gonadotropin-releasing hormone neurons. J Neurosci. (2005) 25:62–7. doi: 10.1523/JNEUROSCI.3598-04.2005
54. Christian CA, Moenter SM. Vasoactive intestinal polypeptide can excite gonadotropin-releasing hormone neurons in a manner dependent on estradiol and gated by time of day. Endocrinology (2008) 149:3130–6. doi: 10.1210/en.2007-1098
55. Mellon PL, Windle JJ, Goldsmith PC, Padula CA, Roberts JL, Weiner RI. Immortalization of hypothalamic GnRH neurons by genetically targeted tumorigenesis. Neuron (1990) 5:1–10. doi: 10.1016/0896-6273(90)90028-E
56. Mellon PL, Wetsel WC, Windle JJ, Valenca MM, Goldsmith PC, Whyte DB, et al. Immortalized hypothalamic gonadotropin-releasing hormone neurons. Ciba Found Symp. (1992) 168:104–17. Discussion 117–26.
57. Novaira HJ, Ng Y, Wolfe A, Radovick S. Kisspeptin increases GnRH mRNA expression and secretion in GnRH secreting neuronal cell lines. Mol Cell Endocrinol. (2009) 311:126–34. doi: 10.1016/j.mce.2009.06.011
58. Tonsfeldt KJ, Goodall CP, Latham KL, Chappell PE. Oestrogen induces rhythmic expression of the Kisspeptin-1 receptor GPR54 in hypothalamic gonadotrophin-releasing hormone-secreting GT1-7 cells. J Neuroendocrinol. (2011) 23:823–30. doi: 10.1111/j.1365-2826.2011.02188.x
59. Sukhbaatar U, Kanasaki H, Mijiddorj T, Oride A, Miyazaki K. Expression of gonadotropin-inhibitory hormone receptors in mouse pituitary gonadotroph LbetaT2 cells and hypothalamic gonadotropin-releasing hormone-producing GT1-7 cells. Endocr J. (2014) 61:25–34. doi: 10.1507/endocrj.EJ13-0238
60. Son YL, Ubuka T, Soga T, Yamamoto K, Bentley GE, Tsutsui K. Inhibitory action of gonadotropin-inhibitory hormone on the signaling pathways induced by kisspeptin and vasoactive intestinal polypeptide in GnRH neuronal cell line, GT1-7. Faseb J. (2016) 30:2198–210. doi: 10.1096/fj.201500055
61. Zhao S, Kriegsfeld LJ. Daily changes in GT1-7 cell sensitivity to GnRH secretagogues that trigger ovulation. Neuroendocrinology (2009) 89:448–57. doi: 10.1159/000192370
62. Novaira HJ, Fadoju D, Diaczok D, Radovick S. Genetic mechanisms mediating kisspeptin regulation of GnRH gene expression. J Neurosci. (2012) 32:17391–400. doi: 10.1523/JNEUROSCI.2438-12.2012
63. Sukhbaatar U, Kanasaki H, Mijiddorj T, Oride A, Miyazaki K. Kisspeptin induces expression of gonadotropin-releasing hormone receptor in GnRH-producing GT1-7 cells overexpressing G protein-coupled receptor 54. Gen Comp Endocrinol. (2013) 194:94–101. doi: 10.1016/j.ygcen.2013.09.002
64. Nichols R, Demers LA, Larsen BM, Robinson D, Converso K, Russell MW, et al. Human RFamide-related peptide-1 diminishes cellular and integrated cardiac contractile performance. Peptides (2013) 31:2067–74. doi: 10.1016/j.peptides.2010.07.012
65. Gojska NM, Friedman Z, Belsham DD. Direct regulation of gonadotrophin-releasing hormone (GnRH) transcription by RF-amide-related peptide-3 and kisspeptin in a novel GnRH-secreting cell line, mHypoA-GnRH/GFP. J Neuroendocrinol. (2014) 26:888–97. doi: 10.1111/jne.12225
66. Poling MC, Quennell JH, Anderson GM, Kauffman AS. Kisspeptin neurones do not directly signal to RFRP-3 neurones but RFRP-3 may directly modulate a subset of hypothalamic kisspeptin cells in mice. J Neuroendocrinol. (2013) 25:876–86. doi: 10.1111/jne.12084
67. Olcese J, McArdle CA, Middendorff R, Greenland K. Pituitary adenylate cyclase-activating peptide and vasoactive intestinal peptide receptor expression in immortalized LHRH neurons. J Neuroendocrinol. (1997) 9:937–43. doi: 10.1046/j.1365-2826.1997.00663.x
68. Mahata SK, Mahata M, Livsey CV, Gerdes HH, Huttner WB, O'Connor DT. Neuroendocrine cell type-specific and inducible expression of the secretogranin II gene: crucial role of cyclic adenosine monophosphate and serum response elements. Endocrinology (1999) 140:739–49. doi: 10.1210/endo.140.2.6476
69. Krajnak K, Kashon ML, Rosewell KL, Wise PM. Aging alters the rhythmic expression of vasoactive intestinal polypeptide mRNA but not arginine vasopressin mRNA in the suprachiasmatic nuclei of female rats. J Neurosci. (1998) 18:4767–74. doi: 10.1523/JNEUROSCI.18-12-04767.1998
70. Colwell CS, Michel S, Itri J, Rodriguez W, Tam J, Lelievre V, et al. Disrupted circadian rhythms in VIP- and PHI-deficient mice. Am J Physiol Regul Integr Comp Physiol. (2003) 285:R939–949. doi: 10.1152/ajpregu.00200.2003
71. Clarke IJ, Smith JT, Henry BA, Oldfield BJ, Stefanidis A, Millar RP, et al. Gonadotropin-inhibitory hormone is a hypothalamic peptide that provides a molecular switch between reproduction and feeding. Neuroendocrinology (2012) 95:305–16. doi: 10.1159/000332822
72. Henningsen JB, Ancel C, Mikkelsen JD, Gauer F, Simonneaux V. Roles of RFRP-3 in the daily and seasonal regulation of reproductive activity in female syrian hamsters. Endocrinology (2017) 158:652–63. doi: 10.1210/en.2016-1689
73. Russo KA, La JL, Stephens SB, Poling MC, Padgaonkar NA, Jennings KJ, et al. Circadian control of the female reproductive axis through gated responsiveness of the RFRP-3 system to VIP signaling. Endocrinology (2015) 156:2608–18. doi: 10.1210/en.2014-1762
74. Small TW, Sharp PJ, Bentley GE, Deviche P. Relative photorefractoriness, prolactin, and reproductive regression in a flexibly breeding sonoran desert passerine, the rufous-winged sparrow, Aimophila carpalis. J Biol Rhythms (2008) 23:69–80. doi: 10.1177/0748730407310790
75. Rizwan MZ, Porteous R, Herbison AE, Anderson GM. Cells expressing RFamide-related peptide-1/3, the mammalian gonadotropin-inhibitory hormone orthologs, are not hypophysiotropic neuroendocrine neurons in the rat. Endocrinology (2009) 150:1413–20. doi: 10.1210/en.2008-1287
76. Ancel C, Bentsen AH, Sebert ME, Tena-Sempere M, Mikkelsen JD, Simonneaux V. Stimulatory effect of RFRP-3 on the gonadotrophic axis in the male Syrian hamster: the exception proves the rule. Endocrinology (2012) 153:1352–63. doi: 10.1210/en.2011-1622
77. Johnson MA, Tsutsui K, Fraley GS. Rat RFamide-related peptide-3 stimulates GH secretion, inhibits LH secretion, and has variable effects on sex behavior in the adult male rat. Horm Behav. (2007) 51:171–80. doi: 10.1016/j.yhbeh.2006.09.009
78. Murakami M, Matsuzaki T, Iwasa T, Yasui T, Irahara M, Osugi T, et al. Hypophysiotropic role of RFamide-related peptide-3 in the inhibition of LH secretion in female rats. J Endocrinol. (2008) 199:105–12. doi: 10.1677/JOE-08-0197
79. Kadokawa H, Shibata M, Tanaka Y, Kojima T, Matsumoto K, Oshima K, et al. Bovine C-terminal octapeptide of RFamide-related peptide-3 suppresses luteinizing hormone (LH) secretion from the pituitary as well as pulsatile LH secretion in bovines. Domest Anim Endocrinol. (2009) 36:219–24. doi: 10.1016/j.domaniend.2009.02.001
80. Smith JT, Young IR, Veldhuis JD, Clarke IJ. Gonadotropin-inhibitory hormone (GnIH) secretion into the ovine hypophyseal portal system. Endocrinology (2012) 153:3368–75. doi: 10.1210/en.2012-1088
81. Seeburg PH, Mason AJ, Stewart TA, Nikolics K. The mammalian GnRH gene and its pivotal role in reproduction. Recent Prog Horm Res. (1987) 43:69–98. doi: 10.1016/B978-0-12-571143-2.50008-3
82. Millar RP, Lu ZL, Pawson AJ, Flanagan CA, Morgan K, Maudsley SR. Gonadotropin-releasing hormone receptors. Endocr Rev. (2004) 25:235–75. doi: 10.1210/er.2003-0002
83. Naor Z. Signaling by G-protein-coupled receptor (GPCR): studies on the GnRH receptor. Front Neuroendocrinol. (2009) 30:10–29. doi: 10.1016/j.yfrne.2008.07.001
84. Dobkin-Bekman M, Naidich M, Pawson AJ, Millar RP, Seger R, Naor Z. Activation of mitogen-activated protein kinase (MAPK) by GnRH is cell-context dependent. Mol Cell Endocrinol. (2006) 252:184–90. doi: 10.1016/j.mce.2006.03.035
85. Stanislaus D, Ponder S, Ji TH, Conn PM. Gonadotropin-releasing hormone receptor couples to multiple G proteins in rat gonadotrophs and in GGH3 cells: evidence from palmitoylation and overexpression of G proteins. Biol Reprod. (1998) 59:579–86. doi: 10.1095/biolreprod59.3.579
86. Liu F, Usui I, Evans LG, Austin DA, Mellon PL, Olefsky JM, et al. Involvement of both G(q/11) and G(s) proteins in gonadotropin-releasing hormone receptor-mediated signaling in L beta T2 cells. J Biol Chem. (2002) 277:32099–108. doi: 10.1074/jbc.M203639200
87. Han XB, Conn PM. The role of protein kinases A and C pathways in the regulation of mitogen-activated protein kinase activation in response to gonadotropin-releasing hormone receptor activation. Endocrinology (1999) 140:2241–51. doi: 10.1210/endo.140.5.6707
88. Grosse R, Schmid A, Schoneberg T, Herrlich A, Muhn P, Schultz G, et al. Gonadotropin-releasing hormone receptor initiates multiple signaling pathways by exclusively coupling to G(q/11) proteins. J Biol Chem. (2000) 275:9193–200. doi: 10.1074/jbc.275.13.9193
89. Tsutsumi R, Mistry D, Webster NJ. Signaling responses to pulsatile gonadotropin-releasing hormone in LbetaT2 gonadotrope cells. J Biol Chem. (2010) 285:20262–72. doi: 10.1074/jbc.M110.132662
90. Ciccone NA, Dunn IC, Boswell T, Tsutsui K, Ubuka T, Ukena K, et al. Gonadotrophin inhibitory hormone depresses gonadotrophin alpha and follicle-stimulating hormone beta subunit expression in the pituitary of the domestic chicken. J Neuroendocrinol. (2004) 16:999–1006. doi: 10.1111/j.1365-2826.2005.01260.x
91. Sari IP, Rao A, Smith JT, Tilbrook AJ, Clarke IJ. Effect of RF-amide-related peptide-3 on luteinizing hormone and follicle-stimulating hormone synthesis and secretion in ovine pituitary gonadotropes. Endocrinology (2009) 150:5549–56. doi: 10.1210/en.2009-0775
92. Pineda R, Garcia-Galiano D, Sanchez-Garrido MA, Romero M, Ruiz-Pino F, Aguilar E, et al. Characterization of the inhibitory roles of RFRP3, the mammalian ortholog of GnIH, in the control of gonadotropin secretion in the rat: In vivo and in vitro studies. Am J Physiol Endocrinol Metab. (2010) 299:E39–46. doi: 10.1152/ajpendo.00108.2010
93. Son YL, Ubuka T, Millar RP, Kanasaki H, Tsutsui K. Gonadotropin-inhibitory hormone inhibits GnRH-induced gonadotropin subunit gene transcriptions by inhibiting AC/cAMP/PKA-dependent ERK pathway in LbetaT2 cells. Endocrinology (2012) 153:2332–43. doi: 10.1210/en.2011-1904
94. Alarid ET, Windle JJ, Whyte DB, Mellon PL. Immortalization of pituitary cells at discrete stages of development by directed oncogenesis in transgenic mice. Development (1996) 122:3319–29.
95. Turgeon JL, Kimura Y, Waring DW, Mellon PL. Steroid and pulsatile gonadotropin-releasing hormone (GnRH) regulation of luteinizing hormone and GnRH receptor in a novel gonadotrope cell line. Mol Endocrinol. (1996) 10:439–50.
96. Liu F, Austin DA, Mellon PL, Olefsky JM, Webster NJ. GnRH activates ERK1/2 leading to the induction of c-fos and LHbeta protein expression in LbetaT2 cells. Mol Endocrinol. (2002) 16:419–34. doi: 10.1210/mend.16.3.0791
97. Ancel C, Inglis MA, Anderson GM. Central RFRP-3 Stimulates LH Secretion in Male Mice and Has Cycle Stage-Dependent Inhibitory Effects in Females. Endocrinology (2017) 158:2873–83. doi: 10.1210/en.2016-1902
98. Ubuka T, Bentley GE, Ukena K, Wingfield JC, Tsutsui K. Melatonin induces the expression of gonadotropin-inhibitory hormone in the avian brain. Proc Natl Acad Sci USA. (2005) 102:3052–7. doi: 10.1073/pnas.0403840102
99. Collu R, Gibb W, Ducharme JR. Effects of stress on the gonadal function. J Endocrinol Invest. (1984) 7:529–37. doi: 10.1007/BF03348463
100. Rabin D, Gold PW, Margioris AN, Chrousos GP. Stress and reproduction: physiologic and pathophysiologic interactions between the stress and reproductive axes. Adv Exp Med Biol. (1988) 245:377–87. doi: 10.1007/978-1-4899-2064-5_29
101. Rivest S, Rivier C. The role of corticotropin-releasing factor and interleukin-1 in the regulation of neurons controlling reproductive functions. Endocr Rev. (1995) 16:177–99.
102. Calisi RM, Rizzo NO, Bentley GE. Seasonal differences in hypothalamic EGR-1 and GnIH expression following capture-handling stress in house sparrows (Passer domesticus). Gen Comp Endocrinol. (2008) 157:283–7. doi: 10.1016/j.ygcen.2008.05.010
103. Kirby ED, Geraghty AC, Ubuka T, Bentley GE, Kaufer D. Stress increases putative gonadotropin inhibitory hormone and decreases luteinizing hormone in male rats. Proc Natl Acad Sci USA. (2009) 106:11324–9. doi: 10.1073/pnas.0901176106
104. Kaewwongse M, Takayanagi Y, Onaka T. Effects of RFamide-related peptide (RFRP)-1 and RFRP-3 on oxytocin release and anxiety-related behaviour in rats. J Neuroendocrinol. (2010) 23:20–7. doi: 10.1111/j.1365-2826.2010.02077.x
105. Evans RM. The steroid and thyroid hormone receptor superfamily. Science (1988) 240:889–95. doi: 10.1126/science.3283939
106. Ramamoorthy S, Cidlowski JA. Exploring the molecular mechanisms of glucocorticoid receptor action from sensitivity to resistance. Endocr Dev. (2013) 24:41–56. doi: 10.1159/000342502
107. Son YL, Ubuka T, Narihiro M, Fukuda Y, Hasunuma I, Yamamoto K, et al. Molecular basis for the activation of gonadotropin-inhibitory hormone gene transcription by corticosterone. Endocrinology (2014) 155:1817–26. doi: 10.1210/en.2013-2076
108. Gingerich S, Wang X, Lee PK, Dhillon SS, Chalmers JA, Koletar MM, et al. The generation of an array of clonal, immortalized cell models from the rat hypothalamus: analysis of melatonin effects on kisspeptin and gonadotropin-inhibitory hormone neurons. Neuroscience (2009) 162:1134–40. doi: 10.1016/j.neuroscience.2009.05.026
109. Gojska NM, Belsham DD. Glucocorticoid receptor-mediated regulation of Rfrp (GnIH) and Gpr147 (GnIH-R) synthesis in immortalized hypothalamic neurons. Mol Cell Endocrinol. (2014) 384:23–31. doi: 10.1016/j.mce.2013.12.015
110. Soga T, Dalpatadu SL, Wong DW, Parhar IS. Neonatal dexamethasone exposure down-regulates GnRH expression through the GnIH pathway in female mice. Neuroscience (2012) 218:56–64. doi: 10.1016/j.neuroscience.2012.05.023
111. Kiyohara M, Son YL, Tsutsui K. Involvement of gonadotropin-inhibitory hormone in pubertal disorders induced by thyroid status. Sci Rep. (2017) 7:1042. doi: 10.1038/s41598-017-01183-8
112. Tsutsui K, Son YL, Kiyohara M, Miyata I. Discovery of GnIH and Its Role in Hypothyroidism-Induced Delayed Puberty. Endocrinology (2018) 159:62–8. doi: 10.1210/en.2017-00300
113. Rivkees SA, Bode HH, Crawford JD. Long-term growth in juvenile acquired hypothyroidism: the failure to achieve normal adult stature. N Engl J Med. (1988) 318:599–602. doi: 10.1056/NEJM198803103181003
114. Doufas AG, Mastorakos G. The hypothalamic-pituitary-thyroid axis and the female reproductive system. Ann N Y Acad Sci. (2000) 900:65–76. doi: 10.1111/j.1749-6632.2000.tb06217.x
116. Weber G, Vigone MC, Stroppa L, Chiumello G. Thyroid function and puberty. J Pediatr Endocrinol Metab. (2003) 16(Suppl. 2):253–7.
Keywords: gonadotropin-inhibitory hormone/RFamide-related peptides (GnIH/RFRPs), GnIH receptor (GnIH-R), gonadotropin-releasing hormone (GnRH), kisspeptin, vasoactive intestinal polypeptide (VIP), gonadotropes, glucocorticoid (GC), thyroid hormone (TH)
Citation: Son YL, Ubuka T and Tsutsui K (2019) Molecular Mechanisms of Gonadotropin-Inhibitory Hormone (GnIH) Actions in Target Cells and Regulation of GnIH Expression. Front. Endocrinol. 10:110. doi: 10.3389/fendo.2019.00110
Received: 22 November 2018; Accepted: 06 February 2019;
Published: 25 February 2019.
Edited by:
Honoo Satake, Suntory Foundation for Life Sciences, JapanReviewed by:
Valerie Simonneaux, Centre National de la Recherche Scientifique (CNRS), FranceKataaki Okubo, The University of Tokyo, Japan
Copyright © 2019 Son, Ubuka and Tsutsui. This is an open-access article distributed under the terms of the Creative Commons Attribution License (CC BY). The use, distribution or reproduction in other forums is permitted, provided the original author(s) and the copyright owner(s) are credited and that the original publication in this journal is cited, in accordance with accepted academic practice. No use, distribution or reproduction is permitted which does not comply with these terms.
*Correspondence: You Lee Son, youleeson@keio.jp