- 1Department of Child and Adolescent Psychiatry, Psychotherapy and Psychosomatics, University Hospital, RWTH University Aachen, Aachen, Germany
- 2Institute for Experimental Medicine, Kiel University and Max Planck Institute for Evolutionary Biology, Plön, Germany
- 3Institute of Medical Informatics and Statistics, Kiel University, Kiel, Germany
Interactions between the gut microbiome and the brain are of increasing interest to both researchers and clinicians. Evidence is mounting on the causal role of an altered gut microbiome in inflammatory diseases such as arthritis, inflammatory bowel disease, obesity and diabetes, and psychiatric diseases like anxiety and depression. Mechanisms include altered energy harvest from food, hormonal changes, increased gut permeability, inflammation, immune response, and a direct influence on the brain and behavior. Anorexia nervosa (AN) is the third most common disease in adolescence and exacts a high burden on patients and caregivers. It often becomes chronic and has the highest mortality of all psychiatric diseases. As AN is characterized by nutritional restrictions, weight loss, and severe behavioral symptoms including weight phobia, comorbid anxiety and depression, accompanied by endocrine alterations, increased inflammation, and immune response, exploring the role of the gut microbiome is crucial. Here, we present an overview of the potential mechanisms of interaction between the gut microbiome, the host and particularly the brain in AN and summarize the initial findings of microbiome research on AN. We conclude by identifying future research directions and potential therapeutic approaches, including nutritional interventions, probiotics, prebiotics and food supplements, that could become important additions to current AN therapy.
Introduction
Anorexia nervosa (AN) is one of the most common chronic illnesses in adolescence, with a lifetime prevalence of 1–4% in 12–18-year-old girls in Europe (1). Recent studies report incidence rates of 100–200/100,000 person-years in 15–19-year-old females, which is similar to that of type 1 diabetes (2, 3). In Germany and the UK, the number of hospitalized children and adolescents has risen substantially during the last decade (4), and the age of onset has decreased (5). After a relatively brief window of treatment opportunity, the starvation process in AN is often self-perpetuating (6). AN has a high risk of becoming chronic and has the highest mortality of all psychiatric disorders (7, 8). However, in the early stages of the disorder, no indicator currently exists that enables predicting the course and outcome of the disorder. In an 18-year follow-up study, 25% of the subjects with previous adolescent AN were unemployed because of a mental disorder (9). Psychiatric and somatic comorbidity, including depression, anxiety and starvation-induced somatic changes such as endocrine dysfunctions and osteoporosis is high, and therefore further impedes a favorable outcome. A multimodal treatment approach involving weight rehabilitation and psychotherapy is the current state-of-the-art approach, but underlying pathophysiology and proliferating factors are not well understood.
Evidence is now growing that AN-induced starvation is associated with profound alterations of the gut microbiome (10–13), which is of critical interest given its important interactions with the host metabolism in terms of weight regulation, hormonal, immunologic and inflammatory processes, along with a direct influence on the brain and behavior (“gut-brain axis”) (14–18) (see Figure 1). AN is one of the most “exemplary” disorders for studying gut-brain interactions because no other mental disease exists in which nutrition and its changes, which are important factors influencing microbial growth in the intestine, play such a crucial role (19). Based on the DSM-5, we differentiate between two subtypes of AN, restrictive and binge/purging. While the restrictive subtype is characterized by fasting and often, but not always, physical hyperactivity, patients classified as the binge/purging subtype may engage in binging and purging (e.g., laxative abuse or self-induced vomiting), only binging (with intermittent periods of fasting or excessive exercising) or only purging. In an important study by Mack et al. (10) significant differences in the microbiome were found between the restrictive and the binge/purging subtypes. Moreover, we may speculate that iatrogenic changes in the diet implicated in the treatment of AN, and thus of the microbiome, might also impact the course of the disorder.
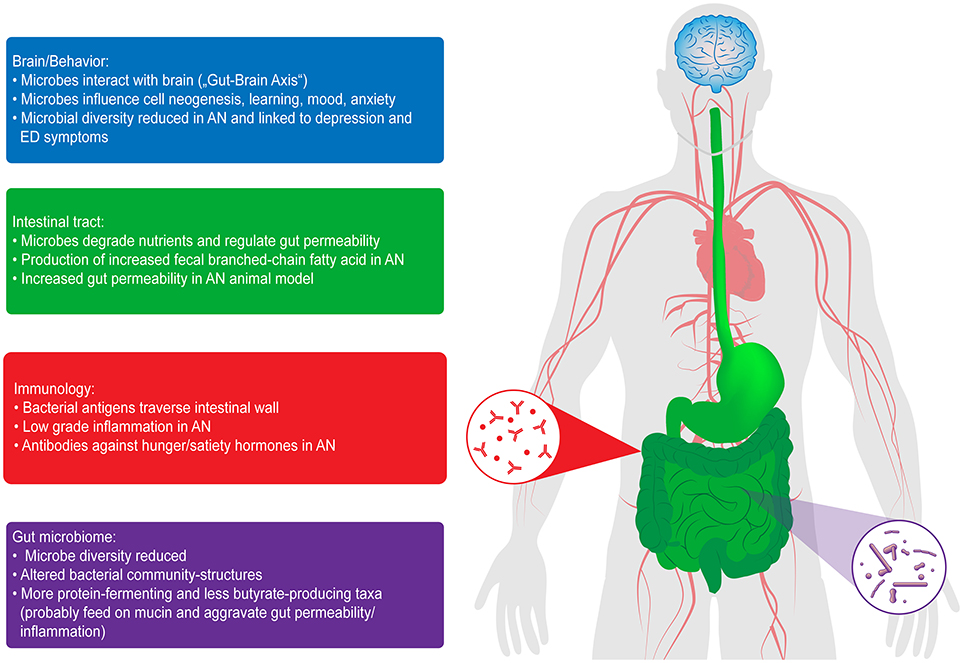
Figure 1. Interactions of the gut microbiome with the brain and behavior, in the intestinal tract and with immunology in patients with AN. ED, eating disorder [from Seitz et al. (18)].
Each human individual has close to 500 out of approximately 1,000 different gut microbial species (20), yielding a highly personal composition. Many digestive functions are thought to have been “delegated” to the gut microbiome over the course of evolution. In this symbiotic relationship, certain gut microbes break down food to supply the host with essential vitamins, fatty acids and other nutrients that the host would otherwise be unable to extract. However, the role of the gut microbiome extends well beyond digestion: the community of microbes is in continuous exchange with the cells of the gut wall and beyond, informing our immune system and affecting intestinal permeability, hormones and inflammation (15). Mechanisms involved in the gut-brain axis that directly influence the brain and behavior are not well understood, but seem to include direct vagal nerve signaling, immune cell migration to the brain, antibodies, cytokines and hormones (15, 21, 22). Causal roles of microbiome alterations have been found in anxiety disorder, depression and reactions to stress (21), all of which are important symptoms or comorbidities of AN (1). In this review, we summarize the pertinent findings regarding these mechanisms of interaction between the microbiome and the host and how they can influence the pathophysiology and symptoms of AN.
Hormones
AN is characterized by profound endocrine alterations (23). Gut microbes are influenced by hormones and neurotransmitters and in turn affect hormonal and neurotransmitter secretion. Consistent evidence of multiple pathways of bidirectional interaction [for a review, see Neuman et al. (15)], including microbes producing dopamine (24), serotonin (24), norepinephrine (25) and GABA (25), as well as insulin and blood sugar regulation (26), has been reported. Germ free (GF) mice, born and raised in completely sterile environments, have proven to be valuable sources of information. Compared to normally raised control animals, they exhibited a 25% increased TSH level (27), but decreased plasma catecholamine (25), and serotonin (28) levels. The satiety hormone leptin was associated with an increased abundance of Bifidobacterium and Lactobacillus and a decreased abundance of Bacteroides and Prevotella, while the hunger hormone ghrelin showed inverse relationships (29). Gut bacteria are thought to help regulate orexin release from neuroendocrine cells which in turn influences local neural communication in the gut as well as central nervous functions of hunger signaling (30). Patients with AN have increased ghrelin and orexin and reduced leptin and thyroxin levels, so hormonal interaction with the microbiome seems likely. Amenorrhea induced by a chronic estrogen deficit is a core symptom of AN. Estrogen has been shown to decrease bacterial virulence but enhance bacterial culture growth rates (24), so further interactions with the gut microbiome can be expected. Lastly, starvation in AN is characterized by increased serum, urinary and salivary cortisol levels (23). GF-mice also showed elevated corticosterone levels, indicating a stress modulating and potentially stress reducing role of the microbiome (31). Notably, the administration of Lactobacillus and Bifidobacteria decreased circulating corticoid hormone levels in both humans and rats (32), further emphasizing the causal role of the microbiome in stress regulation, which might suggest a useful therapeutic option.
Energy Harvest/Body Weight
The microbiome plays a central role in the amount of energy harvested from a specific quantity of food, with important implications for body weight regulation. In 2005, it was discovered that compared with normal weight controls, overweight patients have an altered gut microbiome, which appears able to extract more energy from the same food (33). Furthermore, a causal role of the microbiome was demonstrated when stool transplanted from obese mice into GF-mice resulted in greater weight gain than stool from lean rodents (34). This finding was confirmed in humans when stool transferred before bariatric surgery caused rats to increase in fat mass, whereas stool transferred after surgery did not (35). Stool transfer from the malnourished child of twin children discordant for kwashiorkor in Malawi into GF-mice induced weight loss and malnutrition (36). Additionally, oral antibiotics measurably ameliorated the nutritional state of children from Malawi (37). The proportion of Bacteroidetes was found to be a potentially important factor, as their abundance was associated with body mass index in normal-, under-, and overweight patients (12). Notably, Bacteroidetes is decreased in acutely ill patients with AN and increased upon weight recovery (10, 11, 38). Mack et al. also showed a significant difference of the microbiome between the binge-purging and the restrictive subtypes of AN (10). Differences in the microbial species that extract energy from the same quantity of food could help explain why patients with the restrictive subtype require dramatically more calories to gain weight compared to patients with the binge-purging subtype (39).
Intestinal Permeability
Elevated stress and cortisol levels increase gut permeability, increasing the number of digestion components traversing the gut wall barrier and entering intra- and extra-cellular spaces in the host (40). However, whether higher cortisol levels in patients with AN also cause increased gut permeability is currently being researched. Mörkl et al. (41) could not provide evidence of an increased intestinal permeability in an initial study in patients with AN using blood zonulin levels, and Monteleone et al. (42) found reduced permeability in the small intestine by studying lactulose/mannitol absorption. However, Jesus et al. (43) showed a “leaky gut” in the colon, including fewer tight junction proteins, reduced gastric wall thickness and increased colonic permeability using the Activity-Based Anorexia mouse model. Achamrah et al. (44) also found increased colonic permeability using FITC-Dextran in the same AN animal model, and our own animal research may further support these initial findings, showing reduced cryptal depth and decreased tight junction proteins in the colon of rats, but not in the small intestine (manuscript in preparation). The gut microbiome interacts with the intestinal wall and strongly influences its permeability and barrier function (45). The microbiome in patients with AN appears to shift toward an increase of mucin-degrading Firmicutes and Verrucomicrobia and away from the carbohydrate-degrading species Bacteroidetes (10, 11) (see below). This shift could increase digestion of the protective intestinal wall mucus and further weaken the intestinal wall barrier in the colon, allowing greater translocation of bacterial products and components (10, 43), which might trigger immune and inflammatory responses (46–48).
AN—An Autoimmune Disorder?
In two recent meta-analyses, patients with AN showed a low-grade inflammatory state with increased TNF-alpha, IL-6, and IL-1-beta (49, 50). As certain Lactobacilli can reduce TNF-alpha, IL-6, and IL-8 (51), this approach may offer an interesting treatment option for reducing intestinal permeability and inflammation in patients with AN. Fetissov et al. (52) found that the translocation of bacteria and their subcomponents across the intestinal wall can also cause cross-reactive antibodies to form, which can bind to hunger and satiety hormones such as ghrelin or alpha-melanocyte stimulating hormone (alpha-MSH) (53), thus, altering food intake and weight regulation (54, 55). Moreover, increased levels of antibodies for a large number of hunger and satiety hormones including leptin, ghrelin, orexin, and alpha-MSH were found in patients with eating disorders, including patients with AN, and some of the antibodies even correlated with eating disorder symptom severity (54, 56). A potential mechanism could be that these antibodies protect these hormones from degradation, as could be evidenced for anti-ghrelin antibodies (55, 57). Auto-antibodies could thus be an important underlying phenomenon in AN and one mechanism by which the gut microbiome influences the brain and complex behaviors such as hunger and satiety (18). Notably, patients with AN also showed a generally increased proneness to autoimmune diseases in large Finnish and Swedish cohorts (58, 59). Specifically, the prevalence of gastrointestinal (OR 1.8) and endocrine autoimmune diseases (OR 2.4) was higher, whereas Crohn's disease showed the highest rate with an OR of 3.9. Patients with autoimmune diseases also had an increased risk of AN (60). Anecdotally, a Crohn's disease patient also affected by AN reported a significant improvement in AN symptoms after anti-TNF-alpha treatment, suggesting another link between autoimmune diseases and AN (61).
Gut-Brain Axis
The gut microbiome has a significant effect on our development and the brain, starting at birth. Contact with different bacteria, depending on our mode of birth, i.e., vaginally or via C-section, and whether we were breastfed strongly influence which bacteria inhabit our gastrointestinal tract, with probably lifelong consequences for our immune system (62). The number of metabolic and neurologic diseases with altered gut microbiome and evidence for gut-brain interactions is constantly rising (63): In type 2 diabetes the microbiome appears to disturb the enteric nervous system via inflammatory processes, impairing its role in informing the hypothalamus of the nutritional state to properly control glucose entry in tissues (64, 65). In Parkinson's disease, α-Synuclein aggregates, the major neuropathologic marker of the disease, are detectable in the enteric nervous system prior to the brain, with a possible gut to brain prion-like transmission (66); abundance of Prevotellaceae is reduced by 80%, and abundance of Enterobacteriaceae was highly correlated with postural instability and gait difficulty (67). Stool from depressive patients, who often show increased inflammatory markers, was able to evoke depressive-like symptoms when introduced into antibiotics-treated rodents - but not the stool of healthy controls with no mood disorder (68). In 18 children with autism, who had a heavily altered gut microbiome, continued stool transplants from healthy subjects normalized the microbiome, reduced gastrointestinal symptoms by 80% and improved autistic symptoms for at least 8 weeks after treatment ended (69). Again, causal inferences can be made by studying GF-mice: they exhibit deviant morphologic and functional brain development (70) and different brain-derived neurotropic factor (BDNF) concentrations in the blood and the hippocampus (71), which influence neuron growth and protection as well as synapse formation and connectivity. Notably, patients with AN also showed reduced BDNF serum levels during semi-starvation, which were alleviated upon weight restoration (72). Antibiotics were shown to decrease BDNF levels in the hippocampus in GF-animals increasing levels of anxiety and thus indicating a direct link between the microbiome, behavior and the brain (73). This finding was further corroborated in a study with mice in which antibiotics were used to eradicate the gut microbiome. The result was decreased cell neurogenesis in the hippocampus with ensuing learning deficits, mediated by certain monocytes that could cross the blood-brain barrier. Probiotics, the application of potentially beneficial live bacteria (here, Bifidobacteria and Lactobacillus), were able to reverse both deficits (22). Patients with AN have been shown to suffer from significant brain volume loss, both in gray and white matter (74), associated with neuropsychological deficits and negative clinical prognosis (75). Here, our own group could also identify underlying impaired cell neogenesis in the AN animal model that was most strongly affecting astrocytes (76). We also found functional memory impairments (77). The neurotransmitter serotonin seems to be another important mediator between the microbiome and the brain. GF-mice showed a significantly higher level of serotonin metabolite 5-hydroxyindoleacetic acid [5-HIAA] in the hippocampus (78). The same metabolite was found to be reduced in the CSF of patients with AN during the acute phase, but it increased after weight recovery (79). The exact link between microbiota and brain changes in AN, however, remains to be elucidated.
Initial Findings of AN
Initial studies analyzing altered microbiomes in patients with AN have reported heterogeneous results (14, 16, 18, 80). Cross-sectional studies involving between nine and 20 patients (12, 13, 38, 41, 81, 82), a longitudinal study with three patients (83) and combined cross-sectional studies with a longitudinal follow-up of up to 44 patients (10, 11) have been reported. Two studies found reduced alpha diversity (measuring the number of different species in the sample) (11, 41), whereas two others found changes in the same direction that were not significant (10, 82). Lower alpha diversity is commonly interpreted as disequilibrium of the microbial community, potentially decreasing its genetic pool and ability to react to disturbances. Reduced alpha diversity was also observed in patients with obesity and inflammatory bowel disease and has been correlated with heightened inflammatory levels and intestinal permeability (84). Mack et al. (10) showed a significant increase in alpha diversity during weight restoration, and Kleiman et al. (11) found a trend in the same direction. In Kleiman et al.'s cohort, alpha diversity in the starved state was correlated with eating disorders and depressive and anxious symptoms (11), highlighting another functional relevance of this finding. Increased beta diversity (measuring the dissimilarity of the microbiome between different individuals in the group) was found for two groups (10, 11), showing an increased variability in the AN sample. This diversity decreased after weight rehabilitation, but importantly, the microbiome in the patients with AN nevertheless more closely resembled their own microbiome before weight gain than that of the healthy controls (10). Firmicutes increased in abundance whereas Bacteroidetes decreased in two samples, as noted above (10, 11). The archaea Methanobrevibacter smithii increased in three samples (10, 81, 82), while the Roseburia species decreased in two samples (10, 82). M. smithii was inversely correlated with body mass index in one study (81). Its presence is typically interpreted as an adaptive mechanism to increase energy exploitation (10). Increased protein-degrading Firmicutes, as mentioned, could further induce mucin reduction and intestinal permeability. Decreased Roseburia, which feeds on carbohydrates and normally produces butyrate, could additionally increase intestinal permeability (85) and inflammation (86) due to a lack of the protective impact of this non-branched-chain fatty acid. Two studies showed heightened levels of branched-chain fatty acids. These digestive products of protein fermentation were previously found to increase PYY-production, a gastric peptide known to decrease appetite and increase depressive symptoms (87).
Consequences for Future Research and Therapeutic Outlook
Research on the interaction of the human microbiome with its host is rapidly increasing for a variety of somatic and mental disorders (14, 21, 46, 84). In patients with AN, we require longitudinal observation studies that identify the role of the gut microbiome in energy utilization, appetite, gastrointestinal symptoms, neurocognition and learning, depression, anxiety, and clinical outcome. To clarify the pathophysiologic mechanisms, human and animal intervention studies that pay careful attention to determining causality are required. Furthermore, we must critically analyze current refeeding practices for patients with AN. As nutrition is a crucial influencing factor of the gut microbiome, we must question the provision of standard hospital food to patients without considering the patients' prior diets. The rapid change from typically low amounts of a fiber-rich, low-fat diet prior to admission to a high caloric, high fat and carbohydrate diet will affect the composition of their gut microbiome. The change from a non-animal- to an animal-based diet can tremendously impact the microbiome (19). However, patients who have often been on a vegan or vegetarian diet and who require oral supplements or nasogastric feeding typically receive cow milk-based products, with unclear consequences on their microbiome (14, 17). Most doctors, furthermore, do not know that 25% of all drugs (not only antibiotics) impact the gut microbiome (88). Thus, research on refeeding therapy for AN is urgently required to prevent causing iatrogenic harm to our patients (14).
Future additions to traditional AN therapy aiming to positively influence the gut microbiome could include nutritional interventions, food supplements, probiotics and prebiotics (dietary fibers favoring the growth of certain bacteria). The goals could be to increase the amount of energy harvested from the same quantity of food and to decrease gut permeability, inflammation and antibody formation, with the potential consequence of reducing depressive and anxious symptoms. Preclinical research has demonstrated promising results (45), and healthy controls have shown improved mood and cognitive function in a recent study (89, 90). A recent systematic review of 10 RCTs on the use of probiotics for anxiety and depression in patients found, despite methodological limitations because of the different bacterial strains applied, “preliminary evidence of psychological benefits” (91).
Author Contributions
JS, BH-D, and JB: conception and design; JS, NS, and BH-D: literature search; MB, JB, and AD: critical evaluation of literature search results; JS and BH-D: draft; MB, JB, AD, NS, BH-D, and JS: revisions.
Funding
This study was supported by grants from the German Research Foundation (FOR 2107, Grant No. DE 1614/3-1 and DE 1614/3-2).
Conflict of Interest Statement
The authors declare that the research was conducted in the absence of any commercial or financial relationships that could be construed as a potential conflict of interest.
References
1. Herpertz-Dahlmann B. Adolescent eating disorders: update on definitions, symptomatology, epidemiology, and comorbidity. Child Adolesc Psychiatr Clin N Am. (2015) 24:177–96. doi: 10.1016/j.chc.2014.08.003
2. Javaras KN, Runfola CD, Thornton LM, Agerbo E, Birgegård A, Norring C. Sex- and age-specific incidence of healthcare-register-recorded eating disorders in the complete swedish 1979–2001 birth cohort. Int J Eat Disord. (2018) 48:1070–81. doi: 10.1002/eat.22467
3. Smink FRE, van Hoeken D, Donker GA, Susser ES, Oldehinkel AJ, Hoek HW. Three decades of eating disorders in Dutch primary care: decreasing incidence of bulimia nervosa but not of anorexia nervosa. Psychol Med. (2016) 46:1189–96. doi: 10.1017/S003329171500272X
4. Holland J, Hall N, Yeates DGR, Goldacre M. Trends in hospital admission rates for anorexia nervosa in Oxford (1968-2011) and England (1990-2011): database studies. J R Soc Med. (2016) 109:59–66. doi: 10.1177/0141076815617651
5. Steinhausen H-C, Jensen CM. Time trends in lifetime incidence rates of first-time diagnosed anorexia nervosa and bulimia nervosa across 16 years in a Danish nationwide psychiatric registry study. Int J Eat Disord. (2015) 48:845–50. doi: 10.1002/eat.22402
6. Treasure J, Stein D, Maguire S. Has the time come for a staging model to map the course of eating disorders from high risk to severe enduring illness? An examination of the evidence. Early Interv Psychiatry (2015) 9:173–84. doi: 10.1111/eip.12170
7. Hoang U, Goldacre M, James A. Mortality following hospital discharge with a diagnosis of eating disorder: national record linkage study, England, 2001–2009. Int J Eat Disord. (2014) 47:507–15. doi: 10.1002/eat.22249
8. Schmidt U, Adan R, Böhm I, Campbell IC, Dingemans A, Ehrlich S. Eating disorders: the big issue. Lancet Psychiatry (2016) 3:313–5. doi: 10.1016/S2215-0366(16)00081-X
9. Wentz E, Gillberg IC, Anckarsäter H, Gillberg C, Råstam M. Reproduction and offspring status 18 years after teenage-onset anorexia nervosa—A controlled community-based study. Int J Eat Disord. (2009) 42:483–91. doi: 10.1002/eat.20664
10. Mack I, Cuntz U, Grämer C, Niedermaier S, Pohl C, Schwiertz A. Weight gain in anorexia nervosa does not ameliorate the faecal microbiota, branched chain fatty acid profiles, and gastrointestinal complaints. Sci Rep. (2016) 6:26752. doi: 10.1038/srep26752
11. Kleiman SC, Watson HJ, Bulik-Sullivan EC, Huh EY, Tarantino LM, Bulik CM. The intestinal microbiota in acute anorexia nervosa and during renourishment: relationship to depression, anxiety, and eating disorder psychopathology. Psychosom Med. (2015) 77:969–81. doi: 10.1097/PSY.0000000000000247
12. Mörkl S, Lackner S, Müller W, Gorkiewicz G, Kashofer K, Oberascher A. Gut microbiota and body composition in anorexia nervosa inpatients in comparison to athletes, overweight, obese, and normal weight controls. Int J Eat Disord. (2017) 50:1421–31. doi: 10.1002/eat.22801
13. Morita C, Tsuji H, Hata T, Gondo M, Takakura S, Kawai K. Gut dysbiosis in patients with anorexia nervosa. PLoS ONE (2015) 10:e0145274. doi: 10.1371/journal.pone.0145274
14. Herpertz-Dahlmann B, Seitz J, Baines J. Food matters: how the microbiome and gut–brain interaction might impact the development and course of anorexia nervosa. Eur Child Adolesc Psychiatry (2017) 26:1031–41. doi: 10.1007/s00787-017-0945-7
15. Neuman H, Debelius JW, Knight R, Koren O. Microbial endocrinology: the interplay between the microbiota and the endocrine system. FEMS Microbiol Rev. (2015) 39:509–21. doi: 10.1093/femsre/fuu010
16. Mack I, Penders J, Cook J, Dugmore J, Mazurak N, Enck P. Is the impact of starvation on the gut microbiota specific or unspecific to anorexia nervosa? A narrative review based on a systematic literature search. Curr Neuropharmacol. (2018) 16:1131–49. doi: 10.2174/1570159X16666180118101354
17. Seitz J, Baines J, Herpertz-Dahlmann B. Chapter 14: Microbiome and inflammation in eating disorders. In: Hebebrand J and Herpertz-Dahlmann B, editors. Eating Disorders and Obesity in Childhood and Adolescence. Philadelphia, PA: Elsevier (2019). p. 87–92. doi: 10.1016/B978-0-323-54852-6.00014-8
18. Seitz J, Trinh S, Herpertz-Dahlmann B. The microbiome and eating disorders. Psychiatr Clin North Am. (2019) 42:93–103. doi: 10.1016/j.psc.2018.10.004
19. David LA, Maurice CF, Carmody RN, Gootenberg DB, Button JE, Wolfe BE. Diet rapidly and reproducibly alters the human gut microbiome. Nature (2014) 505:559–63. doi: 10.1038/nature12820
20. Clavel T, Lagkouvardos I, Hiergeist A. Microbiome sequencing: challenges and opportunities for molecular medicine. Expert Rev Mol Diagn. (2016) 16:795–805. doi: 10.1080/14737159.2016.1184574
21. Cryan JF, Dinan TG. Mind-altering microorganisms: the impact of the gut microbiota on brain and behaviour. Nat Rev Neurosci. (2012) 13:701–12. doi: 10.1038/nrn3346
22. Möhle L, Mattei D, Heimesaat MM, Bereswill S, Fischer A, Alutis M. Ly6Chi monocytes provide a link between antibiotic-induced changes in gut microbiota and adult hippocampal neurogenesis. Cell Rep. (2016) 15:1945–56. doi: 10.1016/j.celrep.2016.04.074
23. Schorr M, Miller KK. The endocrine manifestations of anorexia nervosa: mechanisms and management. Nat Rev Endocrinol. (2017) 13:174–86. doi: 10.1038/nrendo.2016.175
24. Roshchina V. Evolutionary considerations of neurotransmitters in microbial, plant, and animal cells. In: Lyte M and Fitzgerald P, editors. Microbial Endocrinology: Interkingdom Signaling in Infectious Disease and Health. New York, NY: Springer (2010). p. 17–52. doi: 10.1007/978-1-4419-5576-0_2
25. Asano Y, Hiramoto T, Nishino R, Aiba Y, Kimura T, Yoshihara K. Critical role of gut microbiota in the production of biologically active, free catecholamines in the gut lumen of mice. Am J Physiol Gastrointest Liver Physiol. (2012) 303:G1288–95. doi: 10.1152/ajpgi.00341.2012
26. Vrieze A, Van Nood E, Holleman F, Salojärvi J, Kootte RS, Bartelsman JFWM. Transfer of intestinal microbiota from lean donors increases insulin sensitivity in individuals with metabolic syndrome. Gastroenterology (2012) 143:913–6.e7. doi: 10.1053/j.gastro.2012.06.031
27. Wostmann B. Morphology and physiology, endocrinology and biochemistry. In: Wostmann BS, editor. Germfree and Gnotobiotic Animal Models. Boca Raton, FL: CRC Press (1996). p. 43–71.
28. Wikoff WR, Anfora AT, Liu J, Schultz PG, Lesley SA, Peters EC. Metabolomics analysis reveals large effects of gut microflora on mammalian blood metabolites. Proc Natl Acad Sci USA. (2009) 106:3698–703. doi: 10.1073/pnas.0812874106
29. Queipo-Ortuño MI, Seoane LM, Murri M, Pardo M, Gomez-Zumaquero JM, Cardona F. Gut microbiota composition in male rat models under different nutritional status and physical activity and its association with serum leptin and ghrelin levels. PLoS ONE (2013) 8:e65465. doi: 10.1371/journal.pone.0065465
30. Rea K, Dinan TG, Cryan JF. The microbiome: A key regulator of stress and neuroinflammation. Neurobiol Stress. (2016) 4:23–33. doi: 10.1016/j.ynstr.2016.03.001
31. Grenham S, Clarke G, Cryan JF, Dinan TG. Brain-gut-microbe communication in health and disease. Front Physiol. (2011) 2:94. doi: 10.3389/fphys.2011.00094
32. Messaoudi M, Lalonde R, Violle N, Javelot H, Desor D, Nejdi A. Assessment of psychotropic-like properties of a probiotic formulation (Lactobacillus helveticus R0052 and Bifidobacterium longum R0175) in rats and human subjects. Br J Nutr. (2011) 105:755–64. doi: 10.1017/S0007114510004319
33. Ley RE, Bäckhed F, Turnbaugh P, Lozupone CA, Knight RD, Gordon JI. Obesity alters gut microbial ecology. Proc Natl Acad Sci USA. (2005) 102:11070–5. doi: 10.1073/pnas.0504978102
34. Ridaura VK, Faith JJ, Rey FE, Cheng J, Duncan AE, Kau AL. Gut microbiota from twins discordant for obesity modulate metabolism in mice. Science (2013) 341:1241214. doi: 10.1126/science.1241214
35. Tremaroli V, Karlsson F, Werling M, Ståhlman M, Kovatcheva-Datchary P, Olbers T, et al. Roux-en-Y gastric bypass and vertical banded gastroplasty induce long-term changes on the human gut microbiome contributing to fat mass regulation. Cell Metab. (2015) 22:228–38. doi: 10.1016/j.cmet.2015.07.009
36. Smith MI, Yatsunenko T, Manary MJ, Trehan I, Mkakosya R, Cheng J. Gut microbiomes of Malawian twin pairs discordant for kwashiorkor. Science (2013) 339:548–54. doi: 10.1126/science.1229000
37. Trehan I, Goldbach HS, LaGrone LN, Meuli GJ, Wang RJ, Maleta KM. Antibiotics as part of the management of severe acute malnutrition. N Engl J Med. (2013) 368:425–35. doi: 10.1056/NEJMoa1202851
38. Million M, Angelakis E, Maraninchi M, Henry M, Giorgi R, Valero R. Correlation between body mass index and gut concentrations of Lactobacillus reuteri, Bifidobacterium animalis, Methanobrevibacter smithii and Escherichia coli. Int J Obes. (2005) 37:1460–6. doi: 10.1038/ijo.2013.20
39. Marzola E, Nasser JA, Hashim SA, Shih P-AB, Kaye WH. Nutritional rehabilitation in anorexia nervosa: review of the literature and implications for treatment. BMC Psychiatry (2013) 13:290. doi: 10.1186/1471-244X-13-290
40. Petra AI, Panagiotidou S, Stewart JM, Conti P, Theoharides TC. Spectrum of mast cell activation disorders. Expert Rev Clin Immunol. (2014) 10:729–39. doi: 10.1586/1744666X.2014.906302
41. Mörkl S, Lackner S, Meinitzer A, Gorkiewicz G, Kashofer K, Painold A. [Pilot study: Gut microbiome and intestinal barrier in anorexia nervosa]. Fortschr Neurol Psychiatr. (2018). doi: 10.1055/s-0043-123826. [Epub ahead of print].
42. Monteleone P, Carratù R, Cartenì M, Generoso M, Lamberti M, Magistris LD. Intestinal permeability is decreased in anorexia nervosa. Mol Psychiatry. (2004) 9:76–80. doi: 10.1038/sj.mp.4001374
43. Jesus P, Ouelaa W, Francois M, Riachy L, Guerin C, Aziz M. Alteration of intestinal barrier function during activity-based anorexia in mice. Clin Nutr Edinb Scotl. (2014) 33:1046–53. doi: 10.1016/j.clnu.2013.11.006
44. Achamrah N, Nobis S, Breton J, Jésus P, Belmonte L, Maurer B. Maintaining physical activity during refeeding improves body composition, intestinal hyperpermeability and behavior in anorectic mice. Sci Rep. (2016) 6:21887. doi: 10.1038/srep21887
45. Kelly JR, Kennedy PJ, Cryan JF, Dinan TG, Clarke G, Hyland NP. Breaking down the barriers: the gut microbiome, intestinal permeability and stress-related psychiatric disorders. Front Cell Neurosci. (2015) 9:392. doi: 10.3389/fncel.2015.00392
46. Dinan TG, Stanton C, Cryan JF. Psychobiotics: a novel class of psychotropic. Biol Psychiatry (2013) 74:720–6. doi: 10.1016/j.biopsych.2013.05.001
47. Kelly JR, Minuto C, Cryan JF, Clarke G, Dinan TG. Cross talk: the microbiota and neurodevelopmental disorders. Front Neurosci. (2017) 11:490. doi: 10.3389/fnins.2017.00490
48. Bambury A, Sandhu K, Cryan JF, Dinan TG. Finding the needle in the haystack: systematic identification of psychobiotics. Br J Pharmacol. (2017) 175:4430–38. doi: 10.1111/bph.14127
49. Solmi M, Veronese N, Favaro A, Santonastaso P, Manzato E, Sergi G. Inflammatory cytokines and anorexia nervosa: a meta-analysis of cross-sectional and longitudinal studies. Psychoneuroendocrinology (2015) 51:237–52. doi: 10.1016/j.psyneuen.2014.09.031
50. Dalton B, Bartholdy S, Robinson L, Solmi M, Ibrahim MA, Breen G. A meta-analysis of cytokine concentrations in eating disorders. J Psychiatr Res. (2018) 103:252–64. doi: 10.1016/j.jpsychires.2018.06.002
51. Dai C, Zheng C-Q, Meng F-J, Zhou Z, Sang L-X, Jiang M. VSL#3 probiotics exerts the anti-inflammatory activity via PI3k/Akt and NF-κB pathway in rat model of DSS-induced colitis. Mol Cell Biochem. (2013) 374:1–11. doi: 10.1007/s11010-012-1488-3
52. Fetissov SO. Role of the gut microbiota in host appetite control: bacterial growth to animal feeding behaviour. Nat Rev Endocrinol. (2017) 13:11. doi: 10.1038/nrendo.2016.150
53. Fetissov SO, Lucas N, Legrand R. Ghrelin-reactive immunoglobulins in conditions of altered appetite and energy balance. Front Endocrinol. (2017) 8:10. doi: 10.3389/fendo.2017.00010
54. Tennoune N, Chan P, Breton J, Legrand R, Chabane YN, Akkermann K. Bacterial ClpB heat-shock protein, an antigen-mimetic of the anorexigenic peptide alpha-MSH, at the origin of eating disorders. Transl Psychiatry (2014) 4:e458. doi: 10.1038/tp.2014.98
55. François M, Takagi K, Legrand R, Lucas N, Beutheu S, Bôle-Feysot C. Increased ghrelin but low ghrelin-reactive immunoglobulins in a rat model of methotrexate chemotherapy-induced anorexia. Front Nutr. (2016) 3:23. doi: 10.3389/fnut.2016.00023
56. Breton J, Legrand R, Akkermann K, Järv A, Harro J, Déchelotte P. Elevated plasma concentrations of bacterial ClpB protein in patients with eating disorders. Int J Eat Disord. (2016) 49:805–8. doi: 10.1002/eat.22531
57. Takagi K, Legrand R, Asakawa A, Amitani H, François M, Tennoune N. Anti-ghrelin immunoglobulins modulate ghrelin stability and its orexigenic effect in obese mice and humans. Nat Commun. (2013) 4:2685. doi: 10.1038/ncomms3685
58. Raevuori A, Haukka J, Vaarala O, Suvisaari JM, Gissler M, Grainger M. The increased risk for autoimmune diseases in patients with eating disorders. PLoS ONE (2014) 9:e104845. doi: 10.1371/journal.pone.0104845
59. Hedman A, Breithaupt L, Hübel C, Thornton LM, Tillander A, Norring C. Bidirectional relationship between eating disorders and autoimmune diseases. J Child Psychol Psychiatry (2018) doi: 10.1111/jcpp.12958. [Epub ahead of print].
60. Zerwas S, Larsen JT, Petersen L, Thornton LM, Quaranta M, Koch SV. Eating disorders, autoimmune, and autoinflammatory disease. Pediatrics (2017) 140:e20162089. doi: 10.1542/peds.2016-2089
61. Solmi M, Santonastaso P, Caccaro R, Favaro A. A case of anorexia nervosa with comorbid nervosa with comorbid Crohn's disease: beneficial effects of anti-TNF-α therapy? Int J Eat Disord. (2013) 46:639–41. doi: 10.1002/eat.22153
62. Santoro A, Ostan R, Candela M, Biagi E, Brigidi P, Capri M. Gut microbiota changes in the extreme decades of human life: a focus on centenarians. Cell Mol Life Sci CMLS (2018) 75:129–48. doi: 10.1007/s00018-017-2674-y
63. Dinan TG, Cryan JF. The microbiome-gut-brain axis in health and disease. Gastroenterol Clin North Am. (2017) 46:77–89. doi: 10.1016/j.gtc.2016.09.007
64. Cani PD. Human gut microbiome: hopes, threats and promises. Gut (2018) 67:1716–25. doi: 10.1136/gutjnl-2018-316723
65. Bessac A, Cani PD, Meunier E, Dietrich G, Knauf C. Inflammation and gut-brain axis during type 2 diabetes: focus on the crosstalk between intestinal immune cells and enteric nervous system. Front Neurosci. (2018) 12:725. doi: 10.3389/fnins.2018.00725
66. Felice VD, Quigley EM, Sullivan AM, O'Keeffe GW, O'Mahony SM. Microbiota-gut-brain signalling in Parkinson's disease: implications for non-motor symptoms. Parkinsonism Relat Disord. (2016) 27:1–8. doi: 10.1016/j.parkreldis.2016.03.012
67. Scheperjans F, Aho V, Pereira PAB, Koskinen K, Paulin L, Pekkonen E. Gut microbiota are related to Parkinson's disease and clinical phenotype: gut microbiota in Parkinson's disease. Mov Disord. (2015) 30:350–8. doi: 10.1002/mds.26069
68. Kelly JR, Borre Y, O'Brien C, Patterson E, El Aidy S, Deane J. Transferring the blues: depression-associated gut microbiota induces neurobehavioural changes in the rat. J Psychiatr Res. (2016) 82:109–18. doi: 10.1016/j.jpsychires.2016.07.019
69. Kang D-W, Adams JB, Gregory AC, Borody T, Chittick L, Fasano A. Microbiota Transfer Therapy alters gut ecosystem and improves gastrointestinal and autism symptoms: an open-label study. Microbiome (2017) 5:10. doi: 10.1186/s40168-016-0225-7
70. Luczynski P, McVey Neufeld K-A, Oriach CS, Clarke G, Dinan TG, Cryan JF. Growing up in a bubble: using germ-free animals to assess the influence of the gut microbiota on brain and behavior. Int J Neuropsychopharmacol. (2016) 19:pyw020. doi: 10.1093/ijnp/pyw020
71. Sherwin E, Rea K, Dinan TG, Cryan JF. A gut (microbiome) feeling about the brain. Curr Opin Gastroenterol. (2016) 32:96–102. doi: 10.1097/MOG.0000000000000244
72. Zwipp J, Hass J, Schober I, Geisler D, Ritschel F, Seidel M. Serum brain-derived neurotrophic factor and cognitive functioning in underweight, weight-recovered and partially weight-recovered females with anorexia nervosa. Prog Neuropsychopharmacol Biol Psychiatry. (2014) 54:163–9. doi: 10.1016/j.pnpbp.2014.05.006
73. Desbonnet L, Clarke G, Traplin A, O'Sullivan O, Crispie F, Moloney RD. Gut microbiota depletion from early adolescence in mice: implications for brain and behaviour. Brain Behav Immun. (2015) 48:165–73. doi: 10.1016/j.bbi.2015.04.004
74. Seitz J, Herpertz-Dahlmann B, Konrad K. Brain morphological changes in adolescent and adult patients with anorexia nervosa. J Neural Transm. (2016) 123:949–59. doi: 10.1007/s00702-016-1567-9
75. Seitz J, Walter M, Mainz V, Herpertz-Dahlmann B, Konrad K, Polier G von. Brain volume reduction predicts weight development in adolescent patients with anorexia nervosa. J Psychiatr Res. (2015) 68:228–37. doi: 10.1016/j.jpsychires.2015.06.019
76. Frintrop L, Liesbrock J, Paulukat L, Johann S, Kas MJ, Tolba R. Reduced astrocyte density underlying brain volume reduction in activity-based anorexia rats. World J Biol Psychiatry (2018) 19:225–35. doi: 10.1080/15622975.2016.1273552
77. Paulukat L, Frintrop L, Liesbrock J, Heussen N, Johann S, Exner C. Memory impairment is associated with the loss of regular oestrous cycle and plasma oestradiol levels in an activity-based anorexia animal model. World J Biol Psychiatry (2016) 17:274–284. doi: 10.3109/15622975.2016.1173725
78. Clarke G, Grenham S, Scully P, Fitzgerald P, Moloney RD, Shanahan F. The microbiome-gut-brain axis during early life regulates the hippocampal serotonergic system in a sex-dependent manner. Mol Psychiatry (2013) 18:666–73. doi: 10.1038/mp.2012.77
79. Kaye WH, Wierenga CE, Bailer UF, Simmons AN, Wagner A, Bischoff-Grethe A. Does a shared neurobiology for foods and drugs of abuse contribute to extremes of food ingestion in anorexia and bulimia nervosa? Biol Psychiatry (2013) 73:836–42. doi: 10.1016/j.biopsych.2013.01.002
80. Carr J, Kleiman SC, Bulik CM, Bulik-Sullivan EC, Carroll IM. Can attention to the intestinal microbiota improve understanding and treatment of anorexia nervosa? Expert Rev Gastroenterol Hepatol. (2016) 10:565–9. doi: 10.1586/17474124.2016.1166953
81. Armougom F, Henry M, Vialettes B, Raccah D, Raoult D. Monitoring bacterial community of human gut microbiota reveals an increase in Lactobacillus in obese patients and Methanogens in anorexic patients. PLoS ONE (2009) 4:e7125. doi: 10.1371/journal.pone.0007125
82. Borgo F, Riva A, Benetti A, Casiraghi MC, Bertelli S, Garbossa S. Microbiota in anorexia nervosa: The triangle between bacterial species, metabolites and psychological tests. PLoS ONE (2017) 12:e0179739. doi: 10.1371/journal.pone.0179739
83. Kleiman SC, Glenny EM, Bulik-Sullivan EC, Huh EY, Tsilimigras MCB, Fodor AA. Daily changes in composition and diversity of the intestinal microbiota in patients with anorexia nervosa: a series of three cases. Eur Eat Disord Rev J Eat Disord Assoc. (2017) 25:423–7. doi: 10.1002/erv.2524
84. Kostic AD, Xavier RJ, Gevers D. The microbiome in inflammatory bowel disease: current status and the future ahead. Gastroenterology (2014) 146:1489–99. doi: 10.1053/j.gastro.2014.02.009
85. Geirnaert A, Calatayud M, Grootaert C, Laukens D, Devriese S, Smagghe G. Butyrate-producing bacteria supplemented in vitro to Crohn's disease patient microbiota increased butyrate production and enhanced intestinal epithelial barrier integrity. Sci Rep. (2017) 7:11450. doi: 10.1038/s41598-017-11734-8
86. Vieira AT, Fukumori C, Ferreira CM. New insights into therapeutic strategies for gut microbiota modulation in inflammatory diseases. Clin Transl Immunol. (2016) 5:e87. doi: 10.1038/cti.2016.38
87. Holzer P, Farzi A. Neuropeptides and the microbiota-gut-brain axis. Adv Exp Med Biol. (2014) 817:195–219. doi: 10.1007/978-1-4939-0897-4_9
88. Maier L, Pruteanu M, Kuhn M, Zeller G, Telzerow A, Anderson EE. Extensive impact of non-antibiotic drugs on human gut bacteria. Nature (2018) 555:623. doi: 10.1038/nature25979
89. Bagga D, Reichert JL, Koschutnig K, Aigner CS, Holzer P, Koskinen K. Probiotics drive gut microbiome triggering emotional brain signatures. Gut Microbes (2018) 9:486–96. doi: 10.1080/19490976.2018.1460015
90. Bagga D, Aigner CS, Reichert JL, Cecchetto C, Fischmeister FPS, Holzer P. Influence of 4-week multi-strain probiotic administration on resting-state functional connectivity in healthy volunteers. Eur J Nutr. (2018) doi: 10.1007/s00394-018-1732-z. [Epub ahead of print].
Keywords: anorexia nervosa, microbiome, inflammation, autoimmune disease, gut-brain axis, nutrition, probiotics, prebiotics
Citation: Seitz J, Belheouane M, Schulz N, Dempfle A, Baines JF and Herpertz-Dahlmann B (2019) The Impact of Starvation on the Microbiome and Gut-Brain Interaction in Anorexia Nervosa. Front. Endocrinol. 10:41. doi: 10.3389/fendo.2019.00041
Received: 30 September 2018; Accepted: 17 January 2019;
Published: 12 February 2019.
Edited by:
Paul Forsythe, McMaster University, CanadaReviewed by:
Yobana Perez-Cervera, Benito Juárez Autonomous University of Oaxaca, MexicoAlain Couvineau, Institut National de la Santé et de la Recherche Médicale (INSERM), France
Copyright © 2019 Seitz, Belheouane, Schulz, Dempfle, Baines and Herpertz-Dahlmann. This is an open-access article distributed under the terms of the Creative Commons Attribution License (CC BY). The use, distribution or reproduction in other forums is permitted, provided the original author(s) and the copyright owner(s) are credited and that the original publication in this journal is cited, in accordance with accepted academic practice. No use, distribution or reproduction is permitted which does not comply with these terms.
*Correspondence: Jochen Seitz, jseitz@ukaachen.de
†These authors share first authorship
‡These authors share last authorship