Corrigendum: Molecular Network Basis of Invasive Pituitary Adenoma: A Review
- Department of Neurosurgery, Xiangya Hospital, Central South University, Changsha, China
Cases with pituitary adenoma comprise 10–25% of intracranial neoplasm, being the third most common intracranial tumor, most of the adenomas are considered to be benign. About 35% of pituitary adenomas are invasive. This review summarized the known molecular basis of the invasiveness of pituitary adenomas. The study pointed out that hypoxia-inducible factor-1α, pituitary tumor transforming gene, vascular endothelial growth factor, fibroblast growth factor-2, and matrix metalloproteinases (MMPs, mainly MMP-2, and MMP-9) are core molecules responsible for the invasiveness of pituitary adenomas. The reason is that these molecules have the ability to directly or indirectly induce cell proliferation, epithelial-to-mesenchymal transition, angiogenesis, degradation, and remodeling of extracellular matrix. HIF-1α induced by hypoxia or apoplexy inside the adenoma might be the initiating factor of invasive transformation, followed with angiogenesis for overexpressed VEGF, EMT for overexpressed PTTG, degradation of ECM for overexpressed MMPs, creating a suitable microenvironment within the tumor. Together, they form a complex interactive network. More investigations are required to further elucidate the mechanisms underlying the invasiveness of pituitary adenomas.
Introduction
Cases with pituitary adenoma comprise 10–25% of intracranial neoplasm (1) and has a prevalence rate of about 17% in the general population (2). Most of the adenomas are considered to be benign. The symptoms of pituitary adenomas contain two major aspects, endocrine related and tumor occupying symptoms, the former differs according to the various hormones that get involved, the later one includes vision loss and headache. Some adenomas found accidentally on an MRI scan also show no clinical symptoms at all. The diagnosis of pituitary adenoma requires both imaging evidence and serum hormone level. About 35% of pituitary adenomas are invasive (3), which are defined and graded by the extent of tumor invading the adjacent sphenoid sinus and cavernous sinus. Invasive pituitary adenomas not only are more difficult to achieve total resection, but also have a higher recurrent rate after standard surgery compared to benign ones.
A few classification systems are available for evaluating invasive pituitary adenomas to aid surgical planning, including Hardy classification, Wilson–Hardy classification, and Knosp classification. Invasive pituitary adenomas are more difficult to surgically remove, and most of the time, they require surgical resection for the relatively more severe symptoms. An invasive pituitary adenoma was considered synonymous with an aggressive adenoma in a number of studies, moreover, aggressive ones are usually macroadenoma (4). However, some scholars (4, 5) preferred to regard an aggressive adenoma as a separated type that displays more aggressive clinical progression despite of the tumor size and should be diagnosed based on the elevated immunoreactivity of Ki-67 and P53 over more “benign” types of pituitary adenoma using tissue immunohistochemistry. Ki-67 is a biomarker widely used to evaluate cell proliferation. P53 is also a biomarker that indicates malignancy and invasiveness when found strongly positive on tumor tissue immunohistochemistry. The 4th edition of WHO classification of pituitary tumors has removed the term atypical pituitary adenoma (APA) for the difficulty and inconsistency in determining proper cutoff of the diagnostic criteria being used before (4, 6–9), in the previous 3rd version, APA is defined as tumors that display invasive growth, Ki-67 index >3%, extensive nuclear staining for p53 and elevated mitotic activity (10), which is vague. So the 4th edition of WHO classification of pituitary tumors has also suggested that the grading of aggressive pituitary adenoma should be evaluated on an individual case basis with criteria mentioned above (7). In some ways, aggressive, and invasive pituitary adenomas can be different in clinical behavior, but they largely share the same molecular basis in terms of malignancy and invasiveness. P53 protein and Ki-67 protein are common biomarkers shared between kinds of tumors, the difficulty (i.e., inconsistent criteria) of using them in grading of pituitary adenoma means that we need some more accurate biomarkers to better distinguish them from benign pituitary adenomas.
Compared with non-invasive pituitary adenomas, those with invasive behavior are difficult to tackle with. Therefore, it is necessary to identify their causes. This review summarized the known molecular basis of the invasiveness of invasive pituitary adenomas, providing insights for further exploration in this field.
Vascular Endothelial Growth Factor and Related Factors in an Invasive Pituitary Adenoma
Increased angiogenesis is found to be essential for the invasiveness and spread of many types of tumors including invasive pituitary adenoma. Invasive macroprolactinomas and non-functional adenomas were more vascular compared with non-invasive ones (11) on surgically removed human pituitary adenomas. As shown in Figure 1, angiogenesis in the tumor is a complex and dynamic process involving the endothelial matrix degradation, proliferation, and migration of endothelial cells, and remodeling of the vascular basement membrane. In general, whether pituitary adenomas are more vascular is still controversial (12–14), but when it comes to invasive or aggressive pituitary adenomas and carcinomas, it is safe to say that angiogenesis is essential (15).
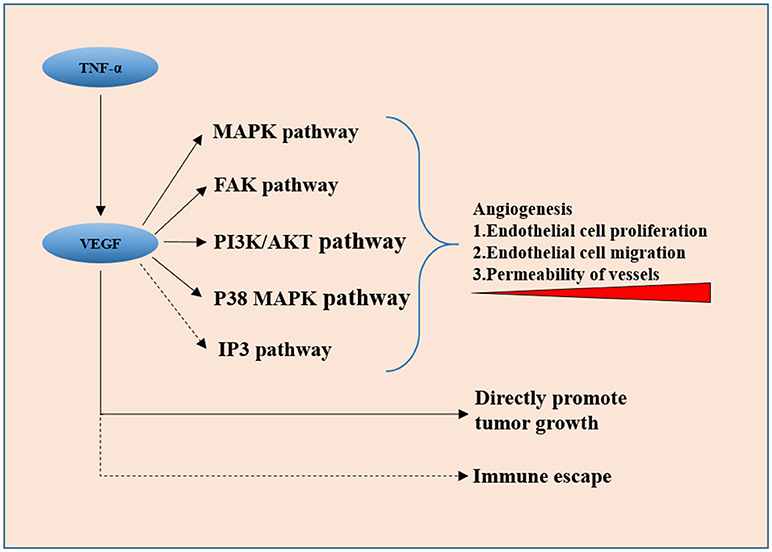
Figure 1. VEGF secreted by tumor cells promotes neovascularization via downstream pathways including the MAPK signaling pathway, FAK pathway, PI3K/Akt pathway, and p38 MAP kinase pathway, it also directly promotes tumor cell proliferation. IP3 pathway and VEGF-induced immune escape might be involved in invasiveness of the pituitary adenoma.
Vascular Endothelial Growth Factor
Vascular endothelial growth factor (VEGF) is proved to be the key factor in angiogenesis in many human tumors. Overexpression of VEGF in clinical samples of invasive pituitary adenomas is observed by many scholars (16). Meaning that VEGF could also be used as an independent prognosis-predicting factor except for Ki-67 and P53. There is a significant relationship between the expression of VEGF and apoplexy of pituitary adenoma (17), which, from another point of view, is an indicator of rapid tumor vascular growth. VEGF secreted by tumor cells promotes neovascularization via downstream pathways including the MAPK signaling pathway (18), FAK pathway (19), PI3K/Akt pathway (20), and p38 MAP kinase pathway (21), directly stimulating tumor cell proliferation (22). The function and the role of IP3 signaling pathway, which is also a classic downstream pathway of VEGF, in the invasiveness of pituitary adenoma are not clear yet. This could be a new entry point for the investigation of VEGF and its peripheral signaling pathways. The activation of these pathways promotes angiogenesis in many ways, including vascular endothelial cell proliferation, migration, and increase in the permeability of newly formed vessels. The controversial results on the microvascular density of pituitary adenomas indicate that VEGF has a more direct and pivotal role in tumorigenesis and invasiveness rather than just in angiogenesis because VEGF-related pathways have been found to directly promote tumor cell proliferation in other cancers recently (23). The immune escape modulated by VEGF was reported (24) and left untested in pituitary adenomas.
Tumor Necrosis Factor α
Some other molecules involving angiogenesis have shown a regulatory effect on the expression of VEGF. Tumor necrosis factor- α (TNF-α) has been reported to upregulate the expression of VEGF and matrix metalloproteinase-9 (MMP-9) in rodent cell line MMQ. In human pituitary adenoma surgical specimens, higher expression levels of TNF-α, VEGF, and MMP-9 were found in hemorrhagic adenomas than in non-hemorrhagic ones. Also, the expression levels of both VEGF and MMP-9 were positively correlated with TNF-α (25). Pituitary apoplexy can cause secondary hypoxia of the tumor tissue, so the elevation of TNF-α and VEGF expression might just be the self-saving struggle under extreme conditions.
Hypoxia-Inducible Factor-1α
Hypoxia-inducible factor-1α (HIF-1α) is able to regulate VEGF in pituitary adenomas and other human tumors. More than a decade ago, the apoptotic protective function of HIF-1α in human pituitary adenoma cell line HP75 under hypoxic conditions via a knockdown experiment was observed (26). VEGF was then confirmed to be activated by HIF-1α (27), but the HIF-1α overexpression model of rodent MMQ cell culture showed a higher apoptotic rate compared with the control, which contradicted the results of knockdown experiments in the human cell line. RWD-containing sumoylation enhancer (RSUME), a stabilizer of HIF-1α under hypoxia (28), is reported to upregulate VEGF in vitro, substantiating the interaction between HIF-1α and VEGF (29). There is overexpression of RSUME, HIF-1α, and VEGF-A in invasive pituitary adenoma surgical specimens compared with non-invasive ones, confirming RSUME as an upstream regulator of expression after the HIF-1α knockdown. Furthermore, the RSUME knockdown rodent AtT-20 cell line demonstrated a more invasive behavior (30). All these experiments displayed the whole picture of an invasiveness-inducing pathway of RSUME–HIF-1α-VEGF.
Notably, the von Hippel-Lindau gene-related protein (pVHL) is a known negative regulator of HIF-1α. Its low expression with a high expression level of VEGF leads to a higher recurrence rate and more aggressive behavior (31). A study reported an aggressive GH-PRL pituitary adenoma in a young patient with a VHL gene missense mutation (32). All these further confirm the importance of VEGF-related pathways in the invasiveness and aggressiveness of pituitary adenomas.
Fibroblast Growth Factor-2
It has been shown that fibroblast growth factor-2 (FGF-2), a growth factor, also promotes vascular endothelial cell proliferation and differentiation similar to VEGF. It is an upstream upregulator of VEGF in human vascular endothelial cells (33) and rodent GH3 pituitary cell line (34). However, FGF-2 had no significant modulatory effect on VEGF at the transcription level in human pituitary cell line HP75 (35). The overexpression of fibroblast growth factor receptor 1 (FGFR1), a receptor of FGF-2, is also closely related to the invasiveness of pituitary adenomas (36). Additionally, FGF-2 had significantly reduced expression levels in male and female prolactinoma patients (37), together with the study showing that FGF-2 had no regulatory effect on cell proliferation (38) and no significant modulating effect on VEGF at the transcription level in human gonadotrophic cell line HP75 (35), indicating FGF-2 might only be effective only in early stages of the development of pituitary adenoma, moreover, only in human prolactinoma.
Exploiting VEGF-Related Pathways in Treating Pituitary Adenoma
Advances in molecular biology have provided a better understanding of invasive pituitary adenomas, improving clinical prognosis. Many successful attempts had been made to exploit VEGF-related pathways in treating pituitary adenomas, from directly targeting VEGF (39–42) to its upstream pathways (43, 44), and to other related molecules (45).
Pituitary Tumor Transforming Gene
First cloned in 1997 (46), the pituitary tumor transforming gene (PTTG) is a known oncogene and upregulator of VEGF (47). The relationship between PTTG and VEGF was later elucidated with the findings that the PTTG upregulate and co-locate with VEGF, thus indirectly promoting angiogenesis in pituitary adenomas.
The PTTG1 is also called securin protein, which counters the function of separin. The degradation of PTTG1 triggers the anaphase of mitosis. Separin then promotes chromosome segregation (48). In human pituitary surgical specimens, invasive pituitary adenomas had the highest level of PTTG followed by non-invasive ones. And the PTTG doesn't express in a normal pituitary tissue (36, 49). Other researchers also reported PTTG as an indicator of both invasiveness and aggressiveness of pituitary adenomas in clinical studies (50–52). A meta-analysis on 15 cohorts of a total 752 patients with a pituitary adenoma further corroborated the relationship between PTTG and invasiveness in pituitary adenomas (53). The elevated PTTG expression level is expected to directly increase cell proliferation and chromosomal instability (54), implying enhanced tumor invasiveness.
Apart from the relationship with VEGF, the PTTG has a wide interaction spectrum with many genes and molecules related to survival, mitogenesis, tumor growth, and invasion. Estrogen receptor α (ERα), a nucleus-located receptor and the mediator of estrogen, is positively related to the invasiveness of human pituitary adenomas (55, 56) and has a significantly higher expression in male patients with prolactinoma than in normal pituitary tissue (37). In the present study, male patients had much higher serum PRL level and much larger tumor volume compared with female patients. The expression of ERα was also elevated in female patients, but not significantly. Estrogen is the first discovered inducer of PTTG, the transcription and translation levels of the PTTG both increased within 48 h after estrogen administration in a “synced” fashion to the estrogen administration in a study using prolactinoma rat model, and under the administration of estrogen, PTTG, FGF-2, VEGF showed the same expression pattern, showing that estrogen is an inducer of PTTG. In the same study, microscopic observation of the pituitary tumor showed progressive neovascularization and remodeling of the extracellular matrix (ECM) (57). All these findings elucidated that PTTG, FGF-2, and VEGF might act in synergy from the early development to increase the invasiveness and angiogenesis of pituitary adenomas, especially prolactinoma (58) and growth hormone–secreting adenomas (59). Connexins (Cx) is a protein family forming the gap connections of cells, expression changes of which between tumor and normal tissue have been reported in many types of cancers (60). Among them, Cx43 is ubiquitously expressed in vertebrates and is considered a tumor suppressor, in most cancer types like testis cancer (61, 62), breast cancer (63, 64), and colorectal cancer (65) tumor cells tend to have lower expression of it, but that is not the case in prolactinoma. Experiments on rat prolactinoma model has shown us that estrogen can induce increasing of gap junctions and of course Cx43, and the silencing of Cx43 could attenuate estrogen-induced up-regulation of PTTG (66). Cx43 might play an import part in tumorigenesis of prolactinoma, the relationship between Cx43 and VEGF, HIF-1α in pituitary adenomas requires future investigation. It is worth mentioning that the PTTG can upregulate the expression and secretion of MMP-2 in HEK293 cells (67), MMP-2 is capable of inducing invasiveness in pituitary adenomas. It could be the same for the PTTG in pituitary adenomas. The clarification of this would be worthwhile because both PTTG and MMPs are potentially valuable therapeutic targets.
Degradation and Remodeling of ECM by Matrix Metalloproteinases Family
Matrix metalloproteinases (MMPs) are a group of calcium-dependent zinc-containing endopeptidases with the ability to degrade basement membrane and ECM. Together with the tissue inhibitor of metalloproteinases (TIMPs), they are the essential elements in the stability and remodeling of ECM (11). A dynamic balance is maintained between MMPs and TIMPs. The major types of MMPs involved in pituitary adenoma invasion can be classified into collagenases (MMP-1), gelatinases (MMP-2 and MMP-9), stromelysins (MMP-3), and membrane type (MMP-14) according to their function and location. TIMPs (TIMP-1, TIMP-2, TIMP-3, and TIMP-4) and reversion-inducing cysteine-rich protein with Kazal motifs (RECK) act as inhibitors, and extracellular matrix metalloproteinase inducer (EMMPRIN) acts as the inducer of MMPs.
MMP-9 is the first matrix metalloproteinase found to have a significantly higher expression level in pituitary adenomas invaded to cavernous sinus (68). However, TIMP-1 was undetectable by immunochemistry staining in all samples (69). The correlation between MMP-9 overexpression and invasiveness of pituitary adenomas has been verified by many researchers in human pituitary adenoma specimens (70–75) as well as cell lines (76). Later studies showed that high expression levels of EMMPRIN (77, 78), MMP-2 (71, 75, 79), and MMP-14 (80, 81) and low expression levels of TIMP-2 (82, 83), TIMP-3 (82, 84), and RECK (85) were also correlated with invasiveness. There is a report that found TIMP-2 have higher expression in more patients of invasive prolactinomas then non-invasive ones (74), most of the aforementioned studies were performed on patients with prolactinoma or mixed patients of all secreting types, the contradicting results of TIMP-2 indicating that different types of pituitary adenoma might have distinct signaling pathways regarding to invasiveness. However, no statistical difference in the MMP-9 expression level between invasive and non-invasive non-functioning pituitary adenomas could be found (86).
MMP-9 plays an important role in promoting invasiveness in many type of pituitary adenomas. A transcriptome analysis on somatotroph pituitary adenomas (87) identified genes having a differential expression pattern between the two groups depending on the invasiveness. Hepatocellular carcinoma, downregulated 1 (HEPN1) was found to be less expressed in invasive somatotroph pituitary adenoma. First found to be downregulated in hepatocellular carcinoma. HEPN1 can induce apoptosis when overexpressed in HepG2 (88). In rodent cell lines GH3 and GT1-1, it inhibited the expression of MMP-2 and MMP-9, resulting in reduced invasiveness (87). A transcriptome and proteome analyses on pituitary null cell adenomas, a subtype of non-functioning pituitary adenomas, by the same research team (89), identified that upregulated IL-6R/JAK2/STAT3 promoted invasiveness via MMP-9.
MMPs not only promote invasiveness by the degradation of ECM and the consequential release of various ECM-anchored growth factors (90, 91), other functions are also observed. Interfering with the expression of MMP-14 using shRNA could result in the reduced expression of PTTG, VEGF, and TGFβ in rodent AtT-20 cells (80), implying that MMPs would also directly promote tumor growth and angiogenesis. IL-17 and IL-17 receptors were positively related to MMP-19 in terms of expression levels. The levels were all elevated in invasive pituitary adenomas compared with non-invasive ones (92).
More recent studies have demonstrated the difference in genotyping of patients with pituitary adenoma; polymorphisms of MMP-9 (93) and promoter of MMP-1 (94) could affect invasive phenotype. Other proteases were also demonstrated to promote the invasiveness of human pituitary adenomas, including a disintegrin and metalloproteinase 12 (ADAM12) (81) and serine proteases urokinase-type plasminogen activator (uPA) (83). Interestingly, the same research team reported the involvement of ADAM12 in invasiveness. They later demonstrated that ADAM12 was also involved in epithelial-to-mesenchymal transition (EMT) (95).
Enhancer of zeste homolog 2 (EZH2) is widely involved in many cancers. It is a key catalytic component in polycomb repressive complex 2 (PRC2), which is responsible for the methylation modification of many development- and differentiation-related genes. It is therefore important in tumorigenesis of many human cancers (96). The overexpression of EZH2 in pituitary tumors was found to be related to invasiveness possibly via the upregulation of MMP-14 (97).
Potentials of MMP Inhibitors in Treating Pituitary Adenoma
Efforts were made to evaluate the potential of MMP inhibitor in treating invasive and chemotherapy-refractory pituitary adenomas. Batimastat showed inhibitory effect on the rat prolactinoma model (98) by reducing the cell proliferation rate and promoting the apoptosis.
EMT and Invasive Pituitary Adenoma
EMT is a process that increases the invasiveness of tumor characterized by the loss of epithelial-cadherin (E-cadherin) and the enhanced expression of transcription factor snail family transcriptional repressor 1 (SNAI1) gene (also referred to as Snail), transcription factor snail family transcriptional repressor 2 (SNAI2) gene (also referred to as Slug), forkhead box C1 (FOXC1), twist-related protein 1(TWIST1), neural cadherin (N-cadherin), and Vimentin.
In an early study employing immunohistochemistry on 30 pituitary adenomas, the semi-quantified immunoreactivity of E-cadherin level was not correlated with cavernous sinus invasion (99). A follow-up study with larger sample size and better methodology, using quantitative real-time polymerase chain reaction on cadherin 13 (CDH13) and immunohistochemistry on E-cadherin and β-catenin, demonstrated the expected significantly lower expression levels of E-cadherin (100–102), CHD13 (101), and β-catenin (100, 102) in invasive pituitary adenomas, resulting from a more frequently methylated CDH13 and E-cadherin genes (101). Also, a study reported the nuclear accumulation and translocation of E-cadherin (103), suggesting another possible mechanism for the less expressed E-cadherin in invasive pituitary adenomas.
In a microarray analysis of human somatotroph adenomas, epithelial splicing regulatory protein 1(ESRP1) was differentially expressed in two groups with relatively low or high transcription levels of E-cadherin; the results were validated with RT-PCR and in vivo experiment in GH3 cells. A gene set comprising ESRP1, PKP2, TP53, PERP, IRF6, ROBO1, BICC1, SPINT1, and of course, CDH1 (E-cadherin) was also found to have reduced expression levels (104). With the same sample set, they demonstrated that it was possible to accurately discriminate invasive pituitary adenomas from non-invasive ones using the binary tree analysis on a group of genes including ESRP1, CDH1, and CTNNb1. Therefore, the potential EMT and invasiveness promoting function of genes in this set makes them valuable targets worth further investigation. Among them, the expression level of ESRP1 was confirmed related to the invasiveness of prolactinoma and GH-secreting adenoma later (105).
A number of miRNAs were reported to regulate EMT. The overexpressed miR-133 could upregulate the expression of E-cadherin and downregulate the expression of N-cadherin and Snail (106). The overexpression of miR-132, miR-15a, and miR-16 could downregulate the expression of N-cadherin and TWIST1 genes (107). The expression of Slug was positively correlated with ERα and invasiveness in clinical pituitary adenoma specimens (56), showing that the ERα-Slug–E-cadherin pathway was vital in the invasiveness of pituitary adenomas. The overexpression of miR-133 suppressed invasion by downregulating the expression of transcription factor forkhead box C1 (FOXC1) in HP75 cells (106), implying the involvement of miR-133 in EMT; FOXC1 is a known promoter of EMT (108).
PTTG-induced EMT is an important mechanism of tumor invasiveness and metastasis in lung cancer (109) and ovarian cancer (110). However, its involvement in pituitary adenomas has not been elucidated yet.
MiRNAs and Invasive Pituitary Adenoma
Available evidence shows that the levels of miR-24, miR-34a, miR-93 (111), miR-148-3p, miR-152 (112), miR-132, miR-15a, and miR-16 (107) are significantly lower in invasive pituitary adenomas (111) compared with non-invasive ones. The overexpression of miR-148-3p and miR-152 suppressed invasion by downregulating activated leukocyte cell adhesion molecule (ALCAM) in rodent GH3 cells (112). Also, the overexpression of miR-132, miR-15a, and miR-16 suppressed invasion by downregulating sex-determining region Y-box protein 5 (Sox5) gene in rodent GH3 cells (107). Some miRNAs are reported to have elevated expression levels in invasive pituitary adenomas. MiR-93-5p was overexpressed in invasive (113) corticotroph pituitary adenomas. The expression of miR-106b-5p, miR-93-5p, miR-93-3p, and miR-25-3p, as a cluster, is also positively correlated with invasiveness. The enhanced expression of miR-106b can induce invasiveness via PI3K/PTEN/Akt pathway and sequential overexpression of MMP-9 in HP75 cells (114). Using a miRNA microarray, many differentially expressed miRNAs in non-functioning pituitary adenomas was identified in a single study. The expression levels of miR-181b-5p, miR-181d, miR-191-3p, and miR-598 were upregulated, and the expression levels of miR-3676-5p and miR-383 were downregulated (115). Caveolin-1 (Cav-1) was reported to promote invasiveness via the EGR1/KLF5 pathway in GH3 cells. Its knockdown resulted in a cytoplasmic enrichment of EGR1, which then induced miR-145, miR-124, and miR-183 targeting FSCN1, PTTG1IP, and EZR, respectively (116). MiRNAs are critical in prompting invasiveness in pituitary adenoma, many molecules and pathways are involved, miRNA sequencing would be a proper method comprehensively identifying differentiatly expressed miRNAs, after which targets of these miRNAs can be predicted with bioinformatics tools, functions of them would then be validated with pertinence.
Other Genes Involved in the Invasiveness of Pituitary Adenoma
In a prospective study on 94 patients with prolactinoma, A Disintegrin And Metalloproteinase With Thrombospondin Motifs 6 (ADAMTS6) and Collapsin Response Mediator Protein 1 (CRMP1) were found to be positively related to invasiveness, while the overexpression of PTTG, Cyclin B1(CCNB1), Aurora Kinase B(AURKB), and Centromere Protein E(CENPE) indicated both invasive and aggressive behavior (51), these genes are all mitosis or development related, they might be the key biological processes that transduce invasiveness transformation. A causative CDH23 gene mutation was identified in a family of familial pituitary adenoma and sporadic patients with this mutation have non-invasive phenotype (117). Secreted frizzled-related protein 1(sFRP1) and Wnt inhibitory factor 1(WIF-1) genes were found to be less expressed in invasive non-functioning pituitary adenomas (118). Also, transforming growth factor, beta receptor II(TGFβII), is less expressed in invasive non-functioning pituitary adenomas (119).
Epidermal growth factor-like domain multiple 7(EGFL7) has a higher level of cytoplasmic expression in invasive growth hormone–secreting pituitary adenomas (120), and is positively correlated with Notch2 and Dll3 in knockdown experiments on GH3 cells. Later the invasiveness reduction phenomenon was reported after the knockdown of EGFL7 in GH3 and GT1-1 cells in vitro (121), confirming EGFL7 as a valuable therapeutic target.
Recent reports implied that long non-coding RNAs (lncRNAs) were involved in invasiveness. The expression of lncRNA C5orf66-AS1 was downregulated and inversely related to invasiveness in pituitary null cell adenoma compared with normal pituitary and non-invasive ones (122). However, lncRNA H19 was upregulated in invasive growth hormone–secreting pituitary adenomas (123).
Epigenetic modification of certain genes has been proved to induce invasiveness in pituitary adenomas including P16, DAPK, and Rb1 (124–129), these genes could be new targets of therapy (130). High throughput sequencing of methylation status (i.e., ChIP-sequencing) in the future will hopefully provide us with the global view of the epigenome of pituitary adenomas.
Next-generation sequencing (NGS) is a powerful tool discovering new disease-related genes at a relatively low cost, especially RNA sequencing and whole-exome sequencing. Using NGS on invasive pituitary adenomas and 6 non-invasive pituitary adenomas, 15 genes with pathogenic mutations were identified (131), including EGFL7, LRP1B, MGAM, MAST4, DSPP, PRDM2, PRDM8, ZNF717, LRRC50, TRIOBP, MX2, DPCR1, PRB3, SPANXN2, and KIAA0226. They also reported that CAT, CLU, CHGA, EZR, KRT8, LIMA1, SH3GLB2, and SLC2A1 were invasion-related genes in non-functioning pituitary adenomas (132). And data mining on existing pituitary adenoma RNA sequencing data from the National Center for Biotechnology Information Gene Expression Omnibus identified invasion-associated genes (133), including CLDN7, CNTNAP2, ITGA6, JAM3, PTPRC, and CTNNA1. All these genes could be the critical cause for invasiveness, which needs further exploration.
Conclusions and Perspective
Efforts were made to elucidate the molecular mechanisms of the invasiveness in pituitary adenomas, from the observation of possible biomarkers on tumor specimens to function verification experiments in vitro, and to recent application of multi-omics analysis. Yet, the whole picture is unclear because the molecular basis of invasiveness is highly complex involving multiple genes, proteins, and pathways. What makes it even more difficult is the fact that subtypes of pituitary adenomas are different in many ways rather than just the hormone they secrete.
Luckily, studies in the last two decades provide some clues in this regard. The key nodes of the invasiveness molecular network are easily spotted out in the review of literature. As shown in Figure 2, HIF-1α, PTTG, VEGF, FGF-2, and MMPs (mainly MMP-2 and MMP-9) are core molecules responsible for invasiveness owing to their ability to directly or indirectly induce cell proliferation, EMT, angiogenesis, degradation, and remodeling of ECM. HIF-1α induced by hypoxia or apoplexy inside the adenoma might be the initiating factor of invasive transformation, followed with angiogenesis for overexpressed VEGF, EMT for overexpressed PTTG, degradation of ECM for overexpressed MMPs, creating a suitable microenvironment within the tumor. Next generation sequencing could be the next point of breakthrough for the investigation of pituitary adenomas, high throughput genomic data on methylation status, expression, copy number variance $$et al. on bulk samples and single cell level would push our understanding of the invasive pituitary adenomas to a much higher level.
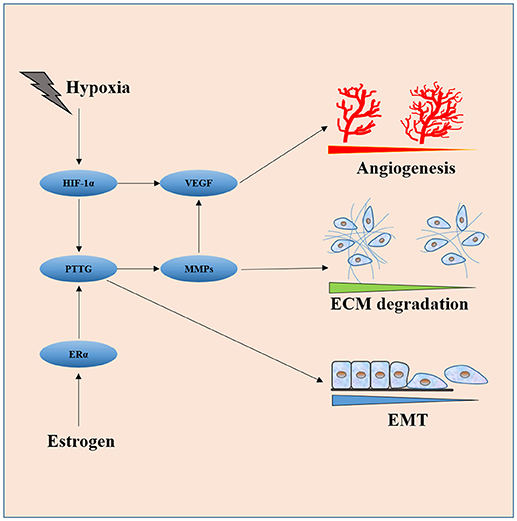
Figure 2. Interaction of core molecules with each other and their relationship with EMT, angiogenesis, and ECM degradation. HIF-1α induced by hypoxia or apoplexy inside the adenoma might be the initiating factor of invasive transformation, followed with angiogenesis for overexpressed VEGF, EMT for overexpressed PTTG, degradation of ECM for overexpressed MMPs.
Many of the studies demonstrated interactions between these molecules both in vivo and in vitro. Most of them focusing on the invasiveness of pituitary adenomas finally came down to the conclusion that a molecule or part of the network was involved. This network is far from complete, and the factors inducing the aforementioned changes are still not known.
More systemic investigations are required to fully understand the mechanisms of the invasiveness of different subtypes of pituitary adenomas. Attempts can still be made targeting one or many molecules at a time, to the invention of new drugs or testing existing chemicals on certain molecules.
Author Contributions
XL designed the study. QY performed the research and wrote the paper. All authors listed have made a substantial, direct and intellectual contribution to the work, and approved it for publication.
Funding
This study was supported by the National Natural Science Foundation of China (Grant No. 81770781).
Conflict of Interest Statement
The authors declare that the research was conducted in the absence of any commercial or financial relationships that could be construed as a potential conflict of interest.
References
1. Landman RE, Horwith M, Peterson RE, Khandji AG, Wardlaw SL. Long-term survival with ACTH-secreting carcinoma of the pituitary: a case report and review of the literature. J Clin Endocrinol Metab. (2002) 87:3084–9. doi: 10.1210/jcem.87.7.8667
2. Ezzat S, Asa SL, Couldwell WT, Barr CE, Dodge WE, Vance ML, et al. The prevalence of pituitary adenomas: a systematic review. Cancer (2004) 101:613–9. doi: 10.1002/cncr.20412
3. Scheithauer BW, Kovacs KT, Laws ER Jr Randall RV. Pathology of invasive pituitary tumors with special reference to functional classification. J Neurosurg. (1986) 65:733–44. doi: 10.3171/jns.1986.65.6.0733
4. Raverot G, Burman P, McCormack A, Heaney A, Petersenn S, Popovic V, et al. European society of endocrinology clinical practice guidelines for the management of aggressive pituitary tumours and carcinomas. Eur J Endocrinol. (2018) 178:G1–24. doi: 10.1530/EJE-17-0796
5. Di Ieva A, Rotondo F, Syro LV, Cusimano MD, Kovacs K. Aggressive pituitary adenomas–diagnosis and emerging treatments. Nat Rev Endocrinol. (2014) 10:423–35. doi: 10.1038/nrendo.2014.64
6. Miermeister CP, Petersenn S, Buchfelder M, Fahlbusch R, Ludecke DK, Holsken A, et al. Histological criteria for atypical pituitary adenomas - data from the German pituitary adenoma registry suggests modifications. Acta Neuropathol Commun. (2015) 3:50. doi: 10.1186/s40478-015-0229-8
7. Lopes MBS. The 2017 World Health Organization classification of tumors of the pituitary gland: a summary. Acta Neuropathol. (2017) 134:521–35. doi: 10.1007/s00401-017-1769-8
8. Mete O, Lopes MB. Overview of the 2017 WHO classification of pituitary tumors. Endocr Pathol. (2017) 28:228–43. doi: 10.1007/s12022-017-9498-z
9. Dworakowska D, Grossman AB. Aggressive and malignant pituitary tumours: state-of-the-art. Endocr Relat Cancer (2018) 25:R559–75. doi: 10.1530/ERC-18-0228
10. Al-Shraim M, Asa SL. The 2004 World Health Organization classification of pituitary tumors: what is new? Acta Neuropathol. (2006) 111:1–7. doi: 10.1007/s00401-005-1093-6
11. Turner HE, Nagy Z, Gatter KC, Esiri MM, Harris AL, Wass JA. Angiogenesis in pituitary adenomas-relationship to endocrine function, treatment and outcome. J Endocrinol. (2000) 165:475–81. doi: 10.1677/joe.0.1650475
12. Viacava P, Gasperi M, Acerbi G, Manetti L, Cecconi E, Bonadio AG, et al. Microvascular density and vascular endothelial growth factor expression in normal pituitary tissue and pituitary adenomas. J Endocrinol Invest. (2003) 26:23–8. doi: 10.1007/BF03345118
13. Takada K, Yamada S, Teramoto A. Correlation between tumor vascularity and clinical findings in patients with pituitary adenomas. Endocr Pathol. (2004) 15:131–9. doi: 10.1385/EP:15:2:131
14. Takano S, Akutsu H, Hara T, Yamamoto T, Matsumura A. Correlations of vascular architecture and angiogenesis with pituitary adenoma histotype. Int J Endocrinol. (2014) 2014:989574. doi: 10.1155/2014/989574
15. Cristina C, Luque GM, Demarchi G, Lopez Vicchi F, Zubeldia-Brenner L, Perez Millan MI, et al. Angiogenesis in pituitary adenomas: human studies and new mutant mouse models. Int J Endocrinol. (2014) 2014:608497. doi: 10.1155/2014/608497
16. Sanchez-Ortiga R, Sanchez-Tejada L, Moreno-Perez O, Riesgo P, Niveiro M, Pico Alfonso AM. Over-expression of vascular endothelial growth factor in pituitary adenomas is associated with extrasellar growth and recurrence. Pituitary (2013) 16:370–7. doi: 10.1007/s11102-012-0434-4
17. Jin Kim Y, Hyun Kim C, Hwan Cheong J, Min Kim J. Relationship between expression of vascular endothelial growth factor and intratumoral hemorrhage in human pituitary adenomas. Tumori (2011) 97:639–46. doi: 10.1177/030089161109700517
18. Zhan X, Desiderio DM. Signaling pathway networks mined from human pituitary adenoma proteomics data. BMC Med Genomics (2010) 3:13. doi: 10.1186/1755-8794-3-13
19. Wang F, Shu K, Lei T, Xue D. The expression of integrinbeta1 and FAK in pituitary adenomas and their correlation with invasiveness. J Huazhong Univ Sci Technol Med Sci. (2008) 28:572–5. doi: 10.1007/s11596-008-0518-6
20. Zhao C, Zhang M, Liu W, Wang C, Zhang Q, Li W. beta-Catenin knockdown inhibits pituitary adenoma cell proliferation and invasion via interfering with AKT and gelatinases expression. Int J Oncol. (2015) 46:1643–50. doi: 10.3892/ijo.2015.2862
21. Kanasaki H, Fukunaga K, Takahashi K, Miyazaki K, Miyamoto E. Involvement of p38 mitogen-activated protein kinase activation in bromocriptine-induced apoptosis in rat pituitary GH3 cells. Biol Reprod. (2000) 62:1486–94. doi: 10.1095/biolreprod62.6.1486
22. Onofri C, Theodoropoulou M, Losa M, Uhl E, Lange M, Arzt E, et al. Localization of vascular endothelial growth factor (VEGF) receptors in normal and adenomatous pituitaries: detection of a non-endothelial function of VEGF in pituitary tumours. J Endocrinol. (2006) 191:249–61. doi: 10.1677/joe.1.06992
23. Elaimy AL, Guru S, Chang C, Ou J, Amante JJ, Zhu LJ, et al. VEGF-neuropilin-2 signaling promotes stem-like traits in breast cancer cells by TAZ-mediated repression of the Rac GAP beta2-chimaerin. Sci Signal. (2018) 11:eaao6897. doi: 10.1126/scisignal.aao6897
24. Voron T, Colussi O, Marcheteau E, Pernot S, Nizard M, Pointet AL, et al. VEGF-A modulates expression of inhibitory checkpoints on CD8+ T cells in tumors. J Exp Med. (2015) 212:139–48. doi: 10.1084/jem.20140559
25. Xiao Z, Liu Q, Mao F, Wu J, Lei T. TNF-alpha-induced VEGF and MMP-9 expression promotes hemorrhagic transformation in pituitary adenomas. Int J Mol Sci. (2011) 12:4165–79. doi: 10.3390/ijms12064165
26. Yoshida D, Kim K, Noha M, Teramoto A. Anti-apoptotic action by hypoxia inducible factor 1-alpha in human pituitary adenoma cell line, HP-75 in hypoxic condition. J Neurooncol. (2006) 78:217–25. doi: 10.1007/s11060-005-9017-9
27. Xiao Z, Liu Q, Zhao B, Wu J, Lei T. Hypoxia induces hemorrhagic transformation in pituitary adenomas via the HIF-1alpha signaling pathway. Oncol Rep. (2011) 26:1457–64. doi: 10.3892/or.2011.1416
28. Carbia-Nagashima A, Gerez J, Perez-Castro C, Paez-Pereda M, Silberstein S, Stalla G, et al. RSUME, a small RWD-containing protein, enhances SUMO conjugation and stabilizes HIF-1alpha during hypoxia. Cell (2007) 131:309–23. doi: 10.1016/j.cell.2007.07.044
29. Shan B, Gerez J, Haedo M, Fuertes M, Theodoropoulou M, Buchfelder M, et al. RSUME is implicated in HIF-1-induced VEGF-A production in pituitary tumour cells. Endocr Relat Cancer (2012) 19:13–27. doi: 10.1530/ERC-11-0211
30. He W, Huang L, Shen X, Yang Y, Wang D, Yang Y, et al. Relationship between RSUME and HIF-1alpha/VEGF-A with invasion of pituitary adenoma. Gene (2017) 603:54–60. doi: 10.1016/j.gene.2016.12.012
31. Shimoda Y, Ogawa Y, Watanabe M, Tominaga T. Clinicopathological investigation of vascular endothelial growth factor and von Hippel-Lindau gene-related protein expression in immunohistochemically negative pituitary adenoma–possible involvement in tumor aggressiveness. Endocr Res. (2013) 38:242–50. doi: 10.3109/07435800.2013.774411
32. Tudorancea A, Francois P, Trouillas J, Cottier JP, Girard JJ, Jan M, et al. Von Hippel-Lindau disease and aggressive GH-PRL pituitary adenoma in a young boy. Ann Endocrinol. (2012) 73:37–42. doi: 10.1016/j.ando.2011.12.001
33. Seghezzi G, Patel S, Ren CJ, Gualandris A, Pintucci G, Robbins ES, et al. Fibroblast growth factor-2 (FGF-2) induces vascular endothelial growth factor (VEGF) expression in the endothelial cells of forming capillaries: an autocrine mechanism contributing to angiogenesis. J Cell Biol. (1998) 141:1659–73. doi: 10.1083/jcb.141.7.1659
34. Lombardero M, Vidal S, Hurta R, Roman A, Kovacs K, Lloyd RV, et al. Modulation of VEGF/Flk-1 receptor expression in the rat pituitary GH3 cell line by growth factors. Pituitary (2006) 9:137–43. doi: 10.1007/s11102-006-9989-2
35. Horiguchi H, Jin L, Ruebel KH, Scheithauer BW, Lloyd RV. Regulation of VEGF-A, VEGFR-I, thrombospondin-1,−2, and−3 expression in a human pituitary cell line (HP75) by TGFbeta1, bFGF, and EGF. Endocrine (2004) 24:141–6. doi: 10.1385/ENDO:24:2:141
36. McCabe CJ, Khaira JS, Boelaert K, Heaney AP, Tannahill LA, Hussain S, et al. Expression of pituitary tumour transforming gene (PTTG) and fibroblast growth factor-2 (FGF-2) in human pituitary adenomas: relationships to clinical tumour behaviour. Clin Endocrinol. (2003) 58:141–50. doi: 10.1046/j.1365-2265.2003.01598.x
37. Lv H, Li C, Gui S, Zhang Y. Expression of estrogen receptor alpha and growth factors in human prolactinoma and its correlation with clinical features and gender. J Endocrinol Invest. (2012) 35:174–80. doi: 10.3275/7607
38. Atkin SL, Landolt AM, Jeffreys RV, Diver M, Radcliffe J, White MC. Basic fibroblastic growth factor stimulates prolactin secretion from human anterior pituitary adenomas without affecting adenoma cell proliferation. J Clin Endocrinol Metab. (1993) 77:831–7.
39. Onofri C, Carbia Nagashima A, Schaaf L, Feirer M, Lohrer P, Stummer W, et al. Estradiol stimulates vascular endothelial growth factor and interleukin-6 in human lactotroph and lactosomatotroph pituitary adenomas. Exp Clin Endocrinol Diabetes (2004) 112:18–23. doi: 10.1055/s-2004-815722
40. Schaaf C, Shan B, Onofri C, Stalla GK, Arzt E, Schilling T, et al. Curcumin inhibits the growth, induces apoptosis and modulates the function of pituitary folliculostellate cells. Neuroendocrinology (2010) 91:200–10. doi: 10.1159/000287236
41. Luque GM, Perez-Millan MI, Ornstein AM, Cristina C, Becu-Villalobos D. Inhibitory effects of antivascular endothelial growth factor strategies in experimental dopamine-resistant prolactinomas. J Pharmacol Exp Ther. (2011) 337:766–74. doi: 10.1124/jpet.110.177790
42. Gagliano T, Filieri C, Minoia M, Buratto M, Tagliati F, Ambrosio MR, et al. Cabergoline reduces cell viability in non-functioning pituitary adenomas by inhibiting vascular endothelial growth factor secretion. Pituitary (2013) 16:91–100. doi: 10.1007/s11102-012-0380-1
43. Shan B, Schaaf C, Schmidt A, Lucia K, Buchfelder M, Losa M, et al. Curcumin suppresses HIF1A synthesis and VEGFA release in pituitary adenomas. J Endocrinol. (2012) 214:389–98. doi: 10.1530/JOE-12-0207
44. Kun Z, Yuling Y, Dongchun W, Bingbing X, Xiaoli L, Bin X. HIF-1alpha inhibition sensitized pituitary adenoma cells to temozolomide by regulating presenilin 1 expression and autophagy. Technol Cancer Res Treat (2016) 15:NP95–104. doi: 10.1177/1533034615618834
45. Zhao Y, Xiao Z, Chen W, Yang J, Li T, Fan B. Disulfiram sensitizes pituitary adenoma cells to temozolomide by regulating O6-methylguanine-DNA methyltransferase expression. Mol Med Rep. (2015) 12:2313–22. doi: 10.3892/mmr.2015.3664
46. Pei L, Melmed S. Isolation and characterization of a pituitary tumor-transforming gene (PTTG). Mol Endocrinol. (1997) 11:433–41. doi: 10.1210/mend.11.4.9911
47. McCabe CJ, Boelaert K, Tannahill LA, Heaney AP, Stratford AL, Khaira JS, et al. Vascular endothelial growth factor, its receptor KDR/Flk-1, and pituitary tumor transforming gene in pituitary tumors. J Clin Endocrinol Metab. (2002) 87:4238–44. doi: 10.1210/jc.2002-020309
48. Zou H, McGarry TJ, Bernal T, Kirschner MW. Identification of a vertebrate sister-chromatid separation inhibitor involved in transformation and tumorigenesis. Science (1999) 285:418–22. doi: 10.1126/science.285.5426.418
49. Zhang X, Horwitz GA, Heaney AP, Nakashima M, Prezant TR, Bronstein MD, et al. Pituitary tumor transforming gene (PTTG) expression in pituitary adenomas. J Clin Endocrinol Metab. (1999) 84:761–7. doi: 10.1210/jcem.84.2.5432
50. Filippella M, Galland F, Kujas M, Young J, Faggiano A, Lombardi G, et al. Pituitary tumour transforming gene (PTTG) expression correlates with the proliferative activity and recurrence status of pituitary adenomas: a clinical and immunohistochemical study. Clin Endocrinol. (2006) 65:536–43. doi: 10.1111/j.1365-2265.2006.02630.x
51. Raverot G, Wierinckx A, Dantony E, Auger C, Chapas G, Villeneuve L, et al. Prognostic factors in prolactin pituitary tumors: clinical, histological, and molecular data from a series of 94 patients with a long postoperative follow-up. J Clin Endocrinol Metab. (2010) 95:1708–16. doi: 10.1210/jc.2009-1191
52. Robertson AM, Heaney AP. Molecular markers in pituitary tumors. Curr Opin Endocrinol Diabetes Obes. (2016) 23:324–30. doi: 10.1097/MED.0000000000000266
53. Li Y, Zhou LP, Ma P, Sui CG, Meng FD, Tian X, et al. Relationship of PTTG expression with tumor invasiveness and microvessel density of pituitary adenomas: a meta-analysis. Genet Test Mol Biomarkers (2014) 18:279–85. doi: 10.1089/gtmb.2013.0447
54. Chesnokova V, Melmed S. Pituitary senescence: the evolving role of Pttg. Mol Cell Endocrinol. (2010) 326:55–9. doi: 10.1016/j.mce.2010.02.012
55. Pereira-Lima JF, Marroni CP, Pizarro CB, Barbosa-Coutinho LM, Ferreira NP, Oliveira MC. Immunohistochemical detection of estrogen receptor alpha in pituitary adenomas and its correlation with cellular replication. Neuroendocrinology (2004) 79:119–24. doi: 10.1159/000077269
56. Zhou W, Song Y, Xu H, Zhou K, Zhang W, Chen J, et al. In non-functional pituitary adenomas, estrogen receptors and slug contribute to development of invasiveness. J Clin Endocrinol Metab. (2011) 96:E1237–45. doi: 10.1210/jc.2010-3040
57. Heaney AP, Horwitz GA, Wang Z, Singson R, Melmed S. Early involvement of estrogen-induced pituitary tumor transforming gene and fibroblast growth factor expression in prolactinoma pathogenesis. Nat Med. (1999) 5:1317–21. doi: 10.1038/15275
58. Heaney AP, Fernando M, Melmed S. Functional role of estrogen in pituitary tumor pathogenesis. J Clin Invest. (2002) 109:277–83. doi: 10.1172/JCI0214264
59. Ozkaya HM, Comunoglu N, Keskin FE, Oz B, Haliloglu OA, Tanriover N, et al. Locally produced estrogen through aromatization might enhance tissue expression of pituitary tumor transforming gene and fibroblast growth factor 2 in growth hormone-secreting adenomas. Endocrine (2016) 52:632–40. doi: 10.1007/s12020-015-0802-8
60. Kandouz M, Batist G. Gap junctions and connexins as therapeutic targets in cancer. Expert Opin Ther Targets (2010) 14:681–92. doi: 10.1517/14728222.2010.487866
61. Chevallier D, Carette D, Gilleron J, Segretain D, Pointis G. The emerging role of connexin 43 in testis pathogenesis. Curr Mol Med. (2013) 13:1331–44. doi: 10.2174/15665240113139990066
62. Chevallier D, Carette D, Segretain D, Gilleron J, Pointis G. Connexin 43 a check-point component of cell proliferation implicated in a wide range of human testis diseases. Cell Mol Life Sci. (2013) 70:1207–20. doi: 10.1007/s00018-012-1121-3
63. McLachlan E, Shao Q, Laird DW. Connexins and gap junctions in mammary gland development and breast cancer progression. J Membr Biol. (2007) 218:107–21. doi: 10.1007/s00232-007-9052-x
64. Grek CL, Rhett JM, Bruce JS, Ghatnekar GS, Yeh ES. Connexin 43, breast cancer tumor suppressor: missed connections? Cancer Lett. (2016) 374:117–26. doi: 10.1016/j.canlet.2016.02.008
65. Kanczuga-Koda L, Koda M, Sulkowski S, Wincewicz A, Zalewski B, Sulkowska M. Gradual loss of functional gap junction within progression of colorectal cancer – a shift from membranous CX32 and CX43 expression to cytoplasmic pattern during colorectal carcinogenesis. In Vivo (2010) 24:101–7. Available online at: http://iv.iiarjournals.org/content/24/1/101.long
66. Wang H, Zhang Y, Zhou A, Zhang R, Meng Q. Effects of silencing connexin43 on expression of pituitary tumor-transforming gene in prolactinomas. Neurol Res. (2015) 37:153–8. doi: 10.1179/1743132814Y.0000000419
67. Malik MT, Kakar SS. Regulation of angiogenesis and invasion by human Pituitary tumor transforming gene (PTTG) through increased expression and secretion of matrix metalloproteinase-2 (MMP-2). Mol Cancer (2006) 5:61. doi: 10.1186/1476-4598-5-61
68. Kawamoto H, Uozumi T, Kawamoto K, Arita K, Yano T, Hirohata T. Type IV collagenase activity and cavernous sinus invasion in human pituitary adenomas. Acta Neurochir (1996) 138:390–5. doi: 10.1007/BF01420300
69. Kawamoto H, Kawamoto K, Mizoue T, Uozumi T, Arita K, Kurisu K. Matrix metalloproteinase-9 secretion by human pituitary adenomas detected by cell immunoblot analysis. Acta Neurochir (1996) 138:1442–8. doi: 10.1007/BF01411124
70. Turner HE, Nagy Z, Esiri MM, Harris AL, Wass JA. Role of matrix metalloproteinase 9 in pituitary tumor behavior. J Clin Endocrinol Metab. (2000) 85:2931–5. doi: 10.1210/jcem.85.8.6754
71. Liu W, Matsumoto Y, Okada M, Miyake K, Kunishio K, Kawai N, et al. Matrix metalloproteinase 2 and 9 expression correlated with cavernous sinus invasion of pituitary adenomas. J Med Invest. (2005) 52:151–8. doi: 10.2152/jmi.52.151
72. Pan LX, Chen ZP, Liu YS, Zhao JH. Magnetic resonance imaging and biological markers in pituitary adenomas with invasion of the cavernous sinus space. J Neurooncol. (2005) 74:71–6. doi: 10.1007/s11060-004-6150-9
73. Gong J, Zhao Y, Abdel-Fattah R, Amos S, Xiao A, Lopes MB, et al. Matrix metalloproteinase-9, a potential biological marker in invasive pituitary adenomas. Pituitary (2008) 11:37–48. doi: 10.1007/s11102-007-0066-2
74. Gultekin GD, Cabuk B, Vural C, Ceylan S. Matrix metalloproteinase-9 and tissue inhibitor of matrix metalloproteinase-2: prognostic biological markers in invasive prolactinomas. J Clin Neurosci. (2015) 22:1282–7. doi: 10.1016/j.jocn.2015.02.021
75. Liu HY, Gu WJ, Wang CZ, Ji XJ, Mu YM. Matrix metalloproteinase-9 and−2 and tissue inhibitor of matrix metalloproteinase-2 in invasive pituitary adenomas: a systematic review and meta-analysis of case-control trials. Medicine (2016) 95:e3904. doi: 10.1097/MD.0000000000003904
76. Hussaini IM, Trotter C, Zhao Y, Abdel-Fattah R, Amos S, Xiao A, et al. Matrix metalloproteinase-9 is differentially expressed in non-functioning invasive and non-invasive pituitary adenomas and increases invasion in human pituitary adenoma cell line. Am J Pathol. (2007) 170:356–65. doi: 10.2353/ajpath.2007.060736
77. Qu X, Yang W, Jiang M, Han T, Han L, Qu Y, et al. CD147 expression in pituitary adenomas and its significance for clinical outcome. Hum Pathol. (2010) 41:1165–71. doi: 10.1016/j.humpath.2009.10.023
78. Zhang Y, He N, Zhou J, Chen Y. The relationship between MRI invasive features and expression of EMMPRIN, galectin-3, and microvessel density in pituitary adenoma. Clin Imaging (2011) 35:165–73. doi: 10.1016/j.clinimag.2010.06.002
79. Liu W, Kunishio K, Matsumoto Y, Okada M, Nagao S. Matrix metalloproteinase-2 expression correlates with cavernous sinus invasion in pituitary adenomas. J Clin Neurosci. (2005) 12:791–4. doi: 10.1016/j.jocn.2005.03.010
80. Hui P, Xu X, Xu L, Hui G, Wu S, Lan Q. Expression of MMP14 in invasive pituitary adenomas: relationship to invasion and angiogenesis. Int J Clin Exp Pathol. (2015) 8:3556–67.
81. Wang J, Voellger B, Benzel J, Schlomann U, Nimsky C, Bartsch JW, et al. Metalloproteinases ADAM12 and MMP-14 are associated with cavernous sinus invasion in pituitary adenomas. Int J Cancer (2016) 139:1327–39. doi: 10.1002/ijc.30173
82. Beaulieu E, Kachra Z, Mousseau N, Delbecchi L, Hardy J, Beliveau R. Matrix metalloproteinases and their inhibitors in human pituitary tumors. Neurosurgery (1999) 45:1432–40; discussion 1440–31. doi: 10.1097/00006123-199912000-00033
83. Knappe UJ, Hagel C, Lisboa BW, Wilczak W, Ludecke DK. Expression of serine proteases and metalloproteinases in human pituitary adenomas and anterior pituitary lobe tissue. Acta Neuropathol. (2003) 106:471–8. doi: 10.1007/s00401-003-0747-5
84. Sun B, Liu X, Yang Y, Dai C, Li Y, Jiao Y, et al. The clinical utility of TIMP3 expression in ACTH-secreting pituitary tumor. J Mol Neurosci. (2016) 58:137–44. doi: 10.1007/s12031-015-0698-z
85. Yoshida D, Nomura R, Teramoto A. Regulation of cell invasion and signalling pathways in the pituitary adenoma cell line, HP-75, by reversion-inducing cysteine-rich protein with kazal motifs (RECK). J Neurooncol. (2008) 89:141–50. doi: 10.1007/s11060-008-9606-5
86. Yokoyama S, Hirano H, Moroki K, Goto M, Imamura S, Kuratsu JI. Are non-functioning pituitary adenomas extending into the cavernous sinus aggressive and/or invasive? Neurosurgery (2001) 49:857–62; discussion 862–53. doi: 10.1097/00006123-200110000-00014
87. Peng H, Fan J, Wu J, Lang J, Wang J, Liu H, et al. Silencing of HEPN1 is responsible for the aggressive biological behavior of pituitary somatotroph adenomas. Cell Physiol Biochem. (2013) 31:379–88. doi: 10.1159/000343375
88. Moh MC, Lee LH, Yang X, Shen S. HEPN1, a novel gene that is frequently down-regulated in hepatocellular carcinoma, suppresses cell growth and induces apoptosis in HepG2 cells. J Hepatol. (2003) 39:580–6. doi: 10.1016/S0168-8278(03)00359-3
89. Feng J, Yu SY, Li CZ, Li ZY, Zhang YZ. Integrative proteomics and transcriptomics revealed that activation of the IL-6R/JAK2/STAT3/MMP9 signaling pathway is correlated with invasion of pituitary null cell adenomas. Mol Cell Endocrinol. (2016) 436:195–203. doi: 10.1016/j.mce.2016.07.025
90. Whitelock JM, Murdoch AD, Iozzo RV, Underwood PA. The degradation of human endothelial cell-derived perlecan and release of bound basic fibroblast growth factor by stromelysin, collagenase, plasmin, and heparanases. J Biol Chem. (1996) 271:10079–86. doi: 10.1074/jbc.271.17.10079
91. Paez Pereda M, Ledda MF, Goldberg V, Chervin A, Carrizo G, Molina H, et al. High levels of matrix metalloproteinases regulate proliferation and hormone secretion in pituitary cells. J Clin Endocrinol Metab. (2000) 85:263–9. doi: 10.1210/jc.85.1.263
92. Qiu L, He D, Fan X, Li Z, Liao C, Zhu Y, et al. The expression of interleukin (IL)-17 and IL-17 receptor and MMP-9 in human pituitary adenomas. Pituitary (2011) 14:266–75. doi: 10.1007/s11102-011-0292-5
93. Glebauskiene B, Liutkeviciene R, Vilkeviciute A, Kriauciuniene L, Jakstiene S, Zlatkute E, et al. Does MMP-9 gene polymorphism play a role in pituitary adenoma development? Dis Markers (2017) 2017:5839528. doi: 10.1155/2017/5839528
94. Altas M, Bayrak OF, Ayan E, Bolukbasi F, Silav G, Coskun KK, et al. The effect of polymorphisms in the promoter region of the MMP-1 gene on the occurrence and invasiveness of hypophyseal adenoma. Acta Neurochir (2010) 152:1611–7; discussion 1617. doi: 10.1007/s00701-010-0671-0
95. Wang J, Zhang Z, Li R, Mao F, Sun W, Chen J, et al. ADAM12 induces EMT and promotes cell migration, invasion and proliferation in pituitary adenomas via EGFR/ERK signaling pathway. Biomed Pharmacother. (2018) 97:1066–77. doi: 10.1016/j.biopha.2017.11.034
96. Volkel P, Dupret B, Le Bourhis X, Angrand PO. Diverse involvement of EZH2 in cancer epigenetics. Am J Transl Res. (2015) 7:175–93.
97. Liu B, Pang B, Wang Q, Yang S, Gao T, Ding Q, et al. EZH2 upregulation correlates with tumor invasiveness, proliferation, and angiogenesis in human pituitary adenomas. Hum Pathol. (2017) 66:101–7. doi: 10.1016/j.humpath.2017.03.028
98. Mucha SA, Melen-Mucha G, Godlewski A, Stepien H. Inhibition of estrogen-induced pituitary tumor growth and angiogenesis in Fischer 344 rats by the matrix metalloproteinase inhibitor batimastat. Virchows Arch. (2007) 450:335–41. doi: 10.1007/s00428-006-0351-x
99. Kawamoto H, Mizoue T, Arita K, Tominaga A, Eguchi K, Kurisu K. Expression of epithelial cadherin and cavernous sinus invasion in human pituitary adenomas. J Neurooncol. (1997) 34:105–9. doi: 10.1023/A:1005709014239
100. Qian ZR, Li CC, Yamasaki H, Mizusawa N, Yoshimoto K, Yamada S, et al. Role of E-cadherin, alpha-, beta-, and gamma-catenins, and p120 (cell adhesion molecules) in prolactinoma behavior. Mod Pathol. (2002) 15:1357–65. doi: 10.1097/01.MP.0000039572.75188.1A
101. Qian ZR, Sano T, Yoshimoto K, Asa SL, Yamada S, Mizusawa N, et al. Tumor-specific downregulation and methylation of the CDH13 (H-cadherin) and CDH1 (E-cadherin) genes correlate with aggressiveness of human pituitary adenomas. Mod Pathol. (2007) 20:1269–77. doi: 10.1038/modpathol.3800965
102. Zhou K, Jin H, Luo Y. Expression and significance of E-cadherin and beta-catenins in pituitary adenoma. Int J Surg Pathol. (2013) 21:363–7. doi: 10.1177/1066896912471850
103. Elston MS, Gill AJ, Conaglen JV, Clarkson A, Cook RJ, Little NS, et al. Nuclear accumulation of e-cadherin correlates with loss of cytoplasmic membrane staining and invasion in pituitary adenomas. J Clin Endocrinol Metab. (2009) 94:1436–42. doi: 10.1210/jc.2008-2075
104. Lekva T, Berg JP, Fougner SL, Olstad OK, Ueland T, Bollerslev J. Gene expression profiling identifies ESRP1 as a potential regulator of epithelial mesenchymal transition in somatotroph adenomas from a large cohort of patients with acromegaly. J Clin Endocrinol Metab. (2012) 97:E1506–14. doi: 10.1210/jc.2012-1760
105. Chauvet N, Romano N, Meunier AC, Galibert E, Fontanaud P, Mathieu MN, et al. Combining cadherin expression with molecular markers discriminates invasiveness in growth hormone and prolactin pituitary adenomas. J Neuroendocrinol. (2016) 28:12352. doi: 10.1111/jne.12352
106. Wang DS, Zhang HQ, Zhang B, Yuan ZB, Yu ZK, Yang T, et al. miR-133 inhibits pituitary tumor cell migration and invasion via down-regulating FOXC1 expression. Genet Mol Res. (2016) 15:gmr7453. doi: 10.4238/gmr.15017453
107. Renjie W, Haiqian L. MiR-132, miR-15a and miR-16 synergistically inhibit pituitary tumor cell proliferation, invasion and migration by targeting Sox5. Cancer Lett. (2015) 356:568–78. doi: 10.1016/j.canlet.2014.10.003
108. Yu M, Bardia A, Wittner BS, Stott SL, Smas ME, Ting DT, et al. Circulating breast tumor cells exhibit dynamic changes in epithelial and mesenchymal composition. Science (2013) 339:580–4. doi: 10.1126/science.1228522
109. Shah PP, Fong MY, Kakar SS. PTTG induces EMT through integrin alphaVbeta3-focal adhesion kinase signaling in lung cancer cells. Oncogene (2012) 31:3124–35. doi: 10.1038/onc.2011.488
110. Shah PP, Kakar SS. Pituitary tumor transforming gene induces epithelial to mesenchymal transition by regulation of Twist, Snail, Slug, and E-cadherin. Cancer Lett. (2011) 311:66–76. doi: 10.1016/j.canlet.2011.06.033
111. Yu G, Wang H, Yu S, Li C, Bai J, Gui S, et al. Study on miRNAs' expression for the invasion of pituitary adenomas. Turk Neurosurg. (2017) 28:530–7. doi: 10.5137/1019-5149.JTN.20760-17.1
112. He W, Huang L, Li M, Yang Y, Chen Z, Shen X. MiR-148b, MiR-152/ALCAM axis regulates the proliferation and invasion of pituitary adenomas cells. Cell Physiol Biochem. (2017) 44:792–803. doi: 10.1159/000485342
113. Garbicz F, Mehlich D, Rak B, Sajjad E, Maksymowicz M, Paskal W, et al. Increased expression of the microRNA 106b~25 cluster and its host gene MCM7 in corticotroph pituitary adenomas is associated with tumor invasion and Crooke's cell morphology. Pituitary (2017) 20:450–63. doi: 10.1007/s11102-017-0805-y
114. Zheng Z, Zhang Y, Zhang Z, Yang Y, Song T. Effect of miR-106b on invasiveness of pituitary adenoma via PTEN-PI3K/AKT. Med Sci Monit. (2017) 23:1277–85. doi: 10.12659/MSM.900092
115. Wu S, Gu Y, Huang Y, Wong T, Ding H, Liu T, et al. Novel biomarkers for non-functioning invasive pituitary adenomas were identified by using analysis of microRNAs expression profile. Biochem Genet. (2017) 55:253–67. doi: 10.1007/s10528-017-9794-9
116. Yang W, Xu T, Qiu P, Xu G. Caveolin-1 promotes pituitary adenoma cells migration and invasion by regulating the interaction between EGR1 and KLF5. Exp Cell Res. (2018) 367:7–14. doi: 10.1016/j.yexcr.2018.01.008
117. Zhang Q, Peng C, Song J, Zhang Y, Chen J, Song Z, et al. Germline mutations in CDH23, encoding cadherin-related 23, are associated with both familial and sporadic pituitary adenomas. Am J Hum Genet. (2017) 100:817–23. doi: 10.1016/j.ajhg.2017.03.011
118. Song W, Qian L, Jing G, Jie F, Xiaosong S, Chunhui L, et al. Aberrant expression of the sFRP and WIF1 genes in invasive non-functioning pituitary adenomas. Mol Cell Endocrinol. (2018) 474:168–75. doi: 10.1016/j.mce.2018.03.005
119. Gu YH, Feng YG. Down-regulation of TGF-beta RII expression is correlated with tumor growth and invasion in non-functioning pituitary adenomas. J Clin Neurosci. (2018) 47:264–8. doi: 10.1016/j.jocn.2017.07.033
120. Wang J, Liu Q, Gao H, Wan D, Li C, Li Z, et al. EGFL7 participates in regulating biological behavior of growth hormone-secreting pituitary adenomas via Notch2/DLL3 signaling pathway. Tumour Biol. (2017) 39:1010428317706203. doi: 10.1177/1010428317706203
121. Liu Q, Wang J, Yang H, Gao H, Li C, Lan X, et al. Attenuation of EGFL7 expression inhibits growth hormone-producing pituitary adenomas growth and invasion. Hum Gene Ther. (2018) 29:1396–406. doi: 10.1089/hum.2017.200
122. Yu G, Li C, Xie W, Wang Z, Gao H, Cao L, et al. Long non-coding RNA C5orf66-AS1 is downregulated in pituitary null cell adenomas and is associated with their invasiveness. Oncol Rep. (2017) 38:1140–8. doi: 10.3892/or.2017.5739
123. Lu T, Yu C, Ni H, Liang W, Yan H, Jin W. Expression of the long non-coding RNA H19 and MALAT-1 in growth hormone-secreting pituitary adenomas and its relationship to tumor behavior. Int J Dev Neurosci. (2018) 67:46–50. doi: 10.1016/j.ijdevneu.2018.03.009
124. Woloschak M, Yu A, Post KD. Frequent inactivation of the p16 gene in human pituitary tumors by gene methylation. Mol Carcinog. (1997) 19:221–4. doi: 10.1002/(SICI)1098-2744(199708)19:4<221::AID-MC1>;3.0.CO;2-F
125. Jaffrain-Rea ML, Ferretti E, Toniato E, Cannita K, Santoro A, Di Stefano D, et al. p16 (INK4a, MTS-1) gene polymorphism and methylation status in human pituitary tumours. Clin Endocrinol. (1999) 51:317–25. doi: 10.1046/j.1365-2265.1999.00774.x
126. Simpson DJ, Bicknell JE, McNicol AM, Clayton RN, Farrell WE. Hypermethylation of the p16/CDKN2A/MTSI gene and loss of protein expression is associated with non-functional pituitary adenomas but not somatotrophinomas. Genes Chromosomes Cancer (1999) 24:328–36. doi: 10.1002/(SICI)1098-2264(199904)24:4<328::AID-GCC6>;3.0.CO;2-P
127. Seemann N, Kuhn D, Wrocklage C, Keyvani K, Hackl W, Buchfelder M, et al. CDKN2A/p16 inactivation is related to pituitary adenoma type and size. J Pathol. (2001) 193:491–7. doi: 10.1002/path.833
128. Ogino A, Yoshino A, Katayama Y, Watanabe T, Ota T, Komine C, et al. The p15(INK4b)/p16(INK4a)/RB1 pathway is frequently deregulated in human pituitary adenomas. J Neuropathol Exp Neurol. (2005) 64:398–403. doi: 10.1093/jnen/64.5.398
129. Pease M, Ling C, Mack WJ, Wang K, Zada G. The role of epigenetic modification in tumorigenesis and progression of pituitary adenomas: a systematic review of the literature. PLoS ONE (2013) 8:e82619. doi: 10.1371/journal.pone.0082619
130. Yacqub-Usman K, Richardson A, Duong CV, Clayton RN, Farrell WE. The pituitary tumour epigenome: aberrations and prospects for targeted therapy. Nat Rev Endocrinol. (2012) 8:486–94. doi: 10.1038/nrendo.2012.54
131. Lan X, Gao H, Wang F, Feng J, Bai J, Zhao P, et al. Whole-exome sequencing identifies variants in invasive pituitary adenomas. Oncol Lett. (2016) 12:2319–28. doi: 10.3892/ol.2016.5029
132. Chen Y, Chuan HL, Yu SY, Li CZ, Wu ZB, Li GL, et al. A novel invasive-related biomarker in three subtypes of non-functioning pituitary adenomas. World Neurosurg. (2017) 100:514–21. doi: 10.1016/j.wneu.2017.01.010
Keywords: angiogenesis, endocrinology, invasiveness, molecular network, pituitary adenoma
Citation: Yang Q and Li X (2019) Molecular Network Basis of Invasive Pituitary Adenoma: A Review. Front. Endocrinol. 10:7. doi: 10.3389/fendo.2019.00007
Received: 28 August 2018; Accepted: 09 January 2019;
Published: 24 January 2019.
Edited by:
Adam Mamelak, Cedars-Sinai Medical Center, United StatesReviewed by:
Manuel Dos Santos Faria, Universidade Federal do Maranhão, BrazilMurat Aydin Sav, Yeditepe University, Turkey
Copyright © 2019 Yang and Li. This is an open-access article distributed under the terms of the Creative Commons Attribution License (CC BY). The use, distribution or reproduction in other forums is permitted, provided the original author(s) and the copyright owner(s) are credited and that the original publication in this journal is cited, in accordance with accepted academic practice. No use, distribution or reproduction is permitted which does not comply with these terms.
*Correspondence: Xuejun Li, lxjneuro@csu.edu.cn