- 1Department of Clinical and Experimental Medicine, University of Pisa, Pisa, Italy
- 2Department of Pharmacy, University of Pisa, Pisa, Italy
- 3Department of Translational Research and of New Technologies in Medicine and Surgery, University of Pisa, Pisa, Italy
- 4Department of Experimental Medicine, “Sapienza” University of Rome, Rome, Italy
- 5Department of Surgical, Medical, Molecular Pathology and Critical Area, University of Pisa, Pisa, Italy
Anaplastic thyroid carcinoma (ATC) is a malignant tumor of the thyroid gland, infrequent but with a very poor prognosis, as it rapidly causes death (mean survival of about 6 months). ATC treatment includes a multimodal protocol consisting of surgery, chemotherapy (doxorubicin and cisplatin), and hyperfractionated accelerated external beam radiotherapy (median patient survival of 10 months). For this reason, the identification of an effective systemic treatment for ATC would be a major advance in the management of this deadly thyroid cancer. The opportunity to test the sensitivity to different drugs of primary cells from ATC (pATC) cultures, obtained from each patients, could improve the effectiveness of the treatment. Then, the administration of inactive therapeutics could be avoided. Our aim is to investigate the antineoplastic effect of two tyrosine kinase inhibitors (TKIs; lenvatinib, vandetanib) in pATC obtained both from biopsy (biop-pATC), and from fine needle aspiration (FNA-pATC). The antiproliferative activity of lenvatinib and vandetanib was evaluated in 6 ATC patients, on biop-pATC, such as on FNA-pATC. A significant reduction of proliferation (obtained by WST-1 assay) vs. control was shown with lenvatinib and vandetanib in FNA-pATC, as well as in biop-pATC. The percentage of apoptosis in FNA-pATC, or biop-pATC, increased with both compounds dose-dependently. pATC cells from FNA, or biopsy, had a similar sensitivity to lenvatinib and vandetanib. In conclusion, primary cells (biop-pATC or FNA-pATC) have a similar sensitivity to TKIs, and lenvatinib and vandetanib are effective in reducing cell growth, increasing apoptosis in ATC. The possibility to test the sensitivity to different TKIs in each patient could open the way to personalized treatments, avoiding the administration of ineffective, and potentially dangerous, drugs.
Introduction
Anaplastic thyroid carcinoma (ATC) is a malignant tumor of the thyroid gland, infrequent but with a very poor prognosis, as it rapidly causes death (mean survival of about 6 months) (1–4).
Anaplastic thyroid carcinoma treatment includes a multimodal protocol consisting of surgery (5), chemotherapy (doxorubicin and cisplatin), and hyperfractionated accelerated external beam radiotherapy (6) (median patient survival of 10 months) (6).
For these reasons, it could be useful to identificate an effective systemic treatment for ATC, to ameliorate the management of this deadly thyroid cancer (TC) (7).
Aurora kinase inhibitors and tyrosine kinase inhibitors (TKIs) (8), as imatinib (9) or sorafenib (10), are promising future treatments, while other studies (11–15) have evaluated antiangiogenic agents, like PTK787/ZK222584, aplidin, combretastatin A4 phosphate, and human vascular endothelial growth factor (VEGF) monoclonal antibodies (bevacizumab, cetuximab).
Moreover, small-molecule adenosine triphosphate (ATP) competitive inhibitors directed intracellularly at epidermal growth factor receptor (EGFR)'s tyrosine kinase (such as erlotinib, or gefitinib) (16, 17) are under evaluation.
The antitumor activity of CLM94 [a new cyclic amide, with antiangiogenic effect and anti-VEGF receptor (R)-2], has been shown in vitro and in vivo in primary (p)ATC cells (18), such as a potent antitumor activity of the new “pyrazolo[3,4-d]pyrimidine” compounds (CLM29 and CLM24), with an antiangiogenic action and able to inhibit EGFR, the RET tyrosine kinase, VEGFR, in 8305C and pATC cells (19).
Moreover, CLM3 (with antiangiogenic activity and suggested for a multiple signal transduction inhibition, on EGFR, the RET tyrosine kinase, and VEGFR), has shown antitumor and antiangiogenic activity in pATC cells (20).
Recently, the combination of dabrafenib plus trametinib has been recently approved for the treatment of ATC with V600EBRAF mutation (21, 22).
Moreover, we have recently shown, that lenvatinib, and vandetanib, have a significant antineoplastic effect, in vitro in ATC cells, and in xenotrasplants of ATC in vivo in nude mice (23, 24).
Despite these new therapeutic strategies against ATC, more researches are required to identify therapies able to control and to cure this disease.
Testing the sensitivity of pATC cells from each subject to different drugs could give the possibility to increase the effectiveness of the treatment in the next future, for the personalization of the therapy.
By disease-orientated in vitro drug testing conducted in human neoplastic cell lines, predictive values for the activity of clinical responses can be obtained (25, 26). A 60% positive predictive value and a 90% negative predictive value have been reported (27). Therefore, in vitro drug testing could avoid to administer patients with inactive chemotherapeutics.
Until now, pATC have been obtained after surgery (biop-pATC) for therapeutic or diagnostic techniques. However, it has been shown the possibility to obtain pATC from fine-needle aspiration (FNA), avoiding worthless surgical procedures and allowing the evaluation of the sensitivity to different chemotherapeutic agents in each patient (28–30).
In this study, we evaluate the antineoplastic effect of lenvatinib, and vandetanib, in pATC obtained from biop-pATC, or from FNA-pATC.
Methods
Drugs and Supplements
Lenvatinib (E7080, Lenvima; 1 nM, 100 nM, 1, 10, 25, and 50 μM), and vandetanib (ZD6474, Caprelsa; 1 nM, 100 nM, 1, 10, 25, and 50 μM), were evaluated in pATC cell cultures.
Most of chemicals and supplements were obtained from Sigma-Aldrich (Merck KGaA, Darmstadt, Germany).
Patients Source for Thyroid Tissue
Thyroidal tissues were obtained from 6 patients with ATC at the time of surgery. The diagnosis was done following generally recognized clinical, laboratory, and histological criteria (28–30).
Absence of thyroid-stimulating hormone (TSH) receptor, thyroperoxidase (TPO), thyroglobulin (Tg), and Sodium/Iodide Symporter (NIS) expression has been shown by immunohistochemistry.
Microdissection and DNA extraction, detection of BRAF mutation by PCR Single Strand Conformation Polymorphism (PCR-SSCP) and direct DNA sequencing were performed using conventional methods previously described (28–30).
Informed consent to the study was obtained from all the subjects, and the approval was received from the local ethical committee of the University of Pisa.
Primary ATC Cells
FNA-pATC
Fine-needle aspiration was conducted in 6 ATC patients by FNA cytology (23 gauge needle). About 10,000 cells were seeded in RPMI 1640 containing 20 μg/ml gentamicin, 100 IU/ml penicillin G, 1% w/v glutamine, 20% v/v Fetal Calf Serum (FCS) (Seromed, Biochrom, Berlin, Germany). After 2 weeks, cells were propagated in DMEM medium containing 50 μg/ml penicillin/streptomycin, 1% w/v glutamine and 20% v/v FCS, then incubated at 37°C in 5% CO2.
To have a sufficient number of cells, chemosensitivity tests were performed at the 4th passage, after 4–5 weeks of controlled in vitro growth.
Biop-pATC
Neoplastic tissues (1–3 mm in size) were obtained, and washed in M-199 media containing 500 IU/ml penicillin, 500 IU/ml streptomycin, and 1,000 IU/ml nystatin, then suspended in DMEM with 50 μg/ml penicillin/streptomycin, 1% w/v glutamine and 20% v/v FCS and maintained in 5% CO2 at 37°C.
At the third cell passage reached in primary tissue-culture flasks, cells were coated in methocel (31) to evaluate the colony-forming efficiencies. The biggest colonies were expanded and chemosensitivity tests were carried out when cells reached the 4th passage.
The absence of TSH receptor, TPO, Tg, and NIS expression was confirmed by immunohistochemistry.
A partial and focal positivity for cytokeratin was obtained by immunocytochemistry on de-stained smears in FNA-pATC.
DNA fingerprinting showed a pattern identical to that of the original neoplastic tissue (28–30).
WST-1 Assay
Cell viability and proliferation were assessed by the WST-1 assay [3-[4,5-dimethylthiazol-2-yl]-2,5-diphenyltetrazolium bromide, used in the MTT assay, by Roche Diagnostics, Almere, The Netherlands] (28, 29, 32).
Different concentrations of lenvatinib or vandetanib (1 nM, 100 nM, 1, 10, 25, and 50 μM), or their vehicle alone, were added in quadruplicates to cells, that were treated for 24 h. Then IC50 values were determined by linear interpolation. The experiments were performed in triplicate for each cell preparation.
For comparison, proliferation was evaluated also by the cell number counting (28, 29, 32).
Apoptosis Evaluation
ATC cells (35,000 cells/mL) were plated and treated with lenvatinib, or vandetanib, for 48 h in a humidified atmosphere (37°C, 5% CO2). Then, pATC were stained with Hoechst 33342, as earlier described (32).
The apoptosis index (ratio between apoptotic and total cells) x100 was calculated.
Moreover, the cells were seeded in Lab-tekII Chamber Slide System (Nalge Nunc International), treated with lenvatinib, or vandetanib, for 48 h, and then treated with Annexin V binding assay (32).
Data Analysis
Values are given as mean ± SD for normally distributed variables, otherwise as median and [interquartile range]. The experiments were repeated 3 times with the cells from each donor. The mean of the experiments in the 6 specimens from different donors is reported. The mean group values were compared by one-way ANOVA for normally distributed variables, otherwise by the Mann-Whitney U or Kruskal-Wallis test. Proportions were compared by the χ2 test. Post-hoc comparisons on normally distributed variables were carried out using the Bonferroni-Dunn test. Data about apoptosis were analyzed by one-way ANOVA with Newman–Keuls multiple comparisons test.
Results
FNA-pATC Cells
Viability and Proliferation Assay
In FNA-pATC cells, a significant reduction of proliferation (vs. control) was observed with lenvatinib at 1 h (data not shown) and at 2 h (from the beginning of tetrazolium reaction; P < 0.01, for both, ANOVA; Figure 1A), as confirmed by cell counting, too.
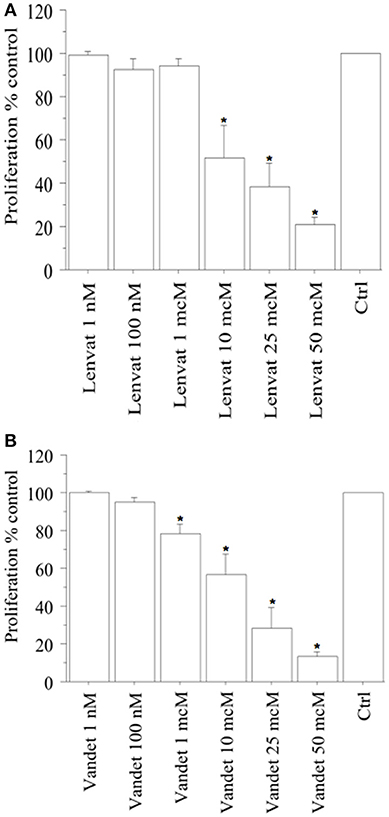
Figure 1. WST-1 test (at 2 h from the beginning of tetrazolium reaction) in FNA-pATC cells treated with lenvatinib (A) or vandetanib (B) for 24 h. Lenvatinib or vandetanib had a concentration-dependent antiproliferative effect on the FNA-pATC cells with an IC50 of 12 or 16 μM, respectively. Bars are mean ± SD. *P < 0.05 or less (by Bonferroni–Dunn test, vs. Control).
In ATC the cell number was 19,405 ± 985/100 μL, per well; 19,589 ± 990 (101%) with lenvatinib 1 nM; 17,850 ± 1,010 (92%) with lenvatinib 100 nM; 18,251 ± 998 (94%) with lenvatinib 1 μM; 10,090 ± 1,115 (52%) with lenvatinib 10 μM; 7,568 ± 1,120 (39%) with lenvatinib 25 μM; 3,687 ± 915 (19%) with lenvatinib 50 μM; (P < 0.01, ANOVA). For lenvatinib, IC50 was 12 μM (by linear interpolation).
Moreover, also a significant reduction of proliferation (vs. control) was reported with vandetanib at 1 h (data not shown) and at 2 h (from the beginning of tetrazolium reaction; P < 0.01, for both, ANOVA; Figure 1B), and confirmed by cell counting.
In ATC the cell number was 19,680 ± 925/100 μL, per well; 19,589 ± 990 (101%) with vandetanib 1 nM; 18,893 ± 995 (96%) with vandetanib 100 nM; 15,744 ± 1,020 (80%) with vandetanib 1 μM; 11,415 ± 1,118 (58%) with vandetanib 10 μM; 5,510 ± 1,120 (28%) with vandetanib 25 μM; 2,755 ± 1,010 (14%) with vandetanib 50 μM; (P < 0.01, ANOVA). For vandetanib, IC50 was 16 μM (by linear interpolation).
BRAF and Proliferation
The V600EBRAF mutation was present in 2 FNA-pATCs; RET/PTC1 and RET/PTC3 by real-time PCR were not revealed in FNA-pATCs.
Regarding the inhibition of proliferation in FNA-pATCs, lenvatinib, and vandetanib gave similar results, considering tumors in presence or absence of the V600EBRAF mutation (data not shown).
Apoptosis Determination
Apoptotic cells (expressed in %) in FNA-pATC rised in a dose-dependent manner: 21% of the cells treated with lenvatinib 1 μM were apoptotic; with the higher lenvatinib concentrations of 10 μM, 25 μM or 50 μM the apoptotic percentage increased up to 42, 51, and 88%, respectively (P < 0.001, ANOVA; Figure 2A).
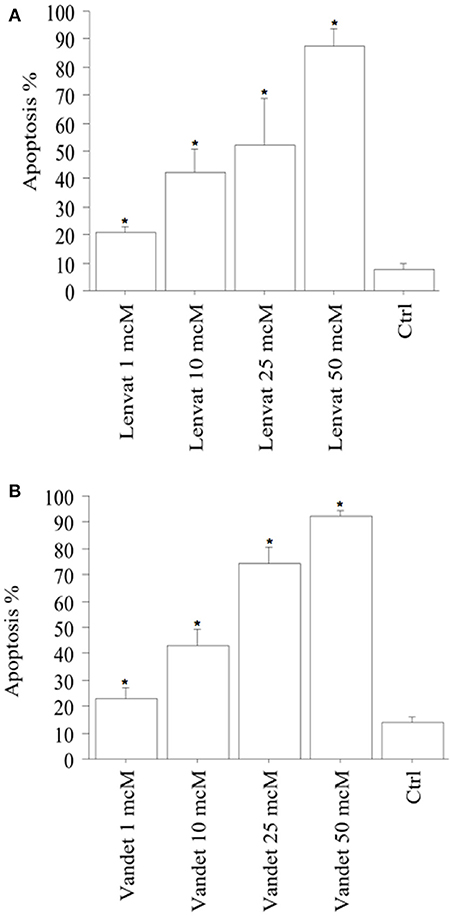
Figure 2. Apoptosis in FNA-pATC cells after the treatment with lenvatinib (A) or vandetanib (B) for 48 h (mean ± SD of all samples). Apoptosis index was obtained by Hoechst staining. The % of apoptotic cells increased strongly and dose-dependently. Data were analyzed by one-way ANOVA with Newman–Keuls multiple comparisons test and with a test for linear trend (*P < 0.001 vs. Control).
Also vandetanib increased apoptosis in FNA-pATC in a dose-dependent manner: 22% of the cells were apoptotic after treatment with vandetanib 1 μM; with the higher vandetanib concentrations of 10, 25, or 50 μM the apoptotic percentage increased up to 42, 72, and 91%, respectively (P < 0.001; by ANOVA; Figure 2B). To confirm the induced cell apoptosis, annexin V staining was used (data not shown).
Biop-ATC Cells
Similar results were obtained in biop-pATC and in FNA-pATC cells, too.
Viability and Proliferation Assay
In biop-pATC cells, a significant reduction of proliferation (vs. control) was observed with lenvatinib at 1 h (data not shown) and at 2 h (from the beginning of tetrazolium reaction; P < 0.01, for both, ANOVA; Figure 3A), as confirmed by the cell counting.
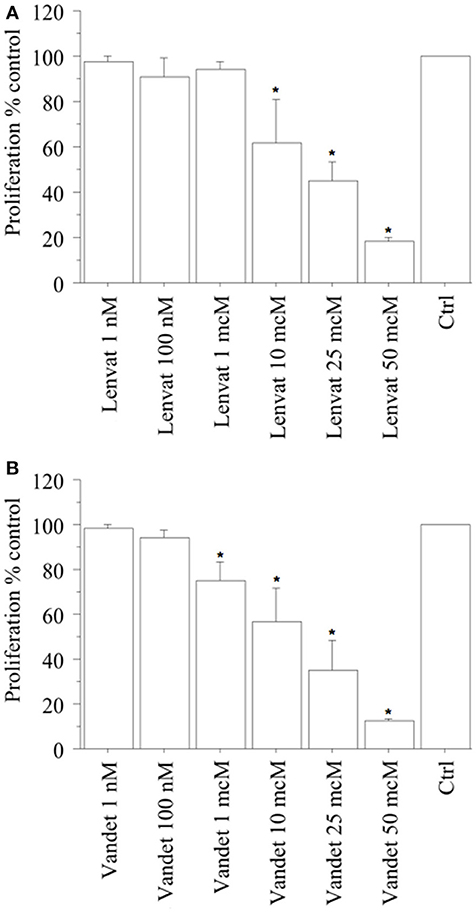
Figure 3. WST-1 test (at 2 h from the beginning of tetrazolium reaction) in biop-pATC cells treated with lenvatinib (A) or vandetanib (B) for 24 h. Lenvatinib or vandetanib had a concentration-dependent antiproliferative effect on the biop-pATC cells with an IC50 of 17 or 18 μM, respectively. Bars are mean ± SD. *P < 0.05 or less (by Bonferroni–Dunn test, vs. Control).
In ATC the cell number was 19,520 ± 980/100 μL, per well; 19,130 ± 985 (98%) with lenvatinib 1 nM; 17,570 ± 1,132 (90%) with lenvatinib 100 nM; 18,544 ± 996 (95%) with lenvatinib 1 μM; 11,712 ± 11,145 (60%) with lenvatinib 10 μM; 8,589 ± 1,020 (44%) with lenvatinib 25 μM; 4,100 ± 910 (21%) with lenvatinib 50 μM; (P < 0.01, ANOVA). For lenvatinib, IC50 was 17 μM (by linear interpolation).
Moreover, also a significant reduction of proliferation (vs. control) was reported with vandetanib at 1 h (data not shown) and at 2 h (from the beginning of tetrazolium reaction; P < 0.01, for both, ANOVA; Figure 3B), confirmed by cell counting.
In ATC the cell number was 19,270 ± 890/100 μL, per well; 19,070 ± 898 (99%) with vandetanib 1 nM; 18,499 ± 902 (96%) with vandetanib 100 nM; 14,450 ± 998 (75%) with vandetanib 1 μM; 10,984 ± 1,121 (57%) with vandetanib 10 μM; 6,360 ± 1,120 (33%) with vandetanib 25 μM; 2,120 ± 900 (11%) with vandetanib 50 μM; (P < 0.01, ANOVA). For vandetanib, IC50 was 18 μM (by linear interpolation).
BRAF and Proliferation
The V600EBRAF mutation was observed in 2 biop-pATC cells; RET/PTC1 and RET/PTC3 by real-time PCR were not revealed in biop-pATCs.
Considering the inhibition of proliferation in biop-pATCs, lenvatinib, and vandetanib, gave similar results in tumors with/without V600EBRAF mutation (data not shown).
Apoptosis Determination
Apoptotic cells (expressed in %) in biop-pATC cells rised in a dose-dependent manner: 27% of the cells were apoptotic after treatment with lenvatinib 1 μM; with the higher lenvatinib concentrations of 10, 25, or 50 μM the apoptotic percentage increased up to 44, 59, and 92%, respectively (P < 0.001, ANOVA; Figure 4A).
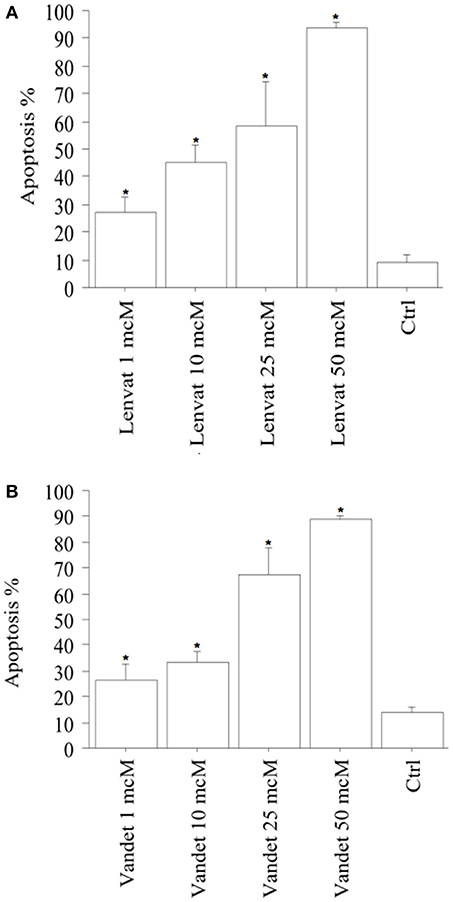
Figure 4. Apoptosis in biop-pATC cells after the treatment with lenvatinib (A) or vandetanib (B) for 48 h (mean ± SD of all samples). Apoptosis index was obtained by Hoechst staining. The % of apoptotic cells increased strongly and dose-dependently. Data were analyzed by one-way ANOVA with Newman–Keuls multiple comparisons test and with a test for linear trend (*P < 0.001 vs. Control).
Also vandetanib increased apoptosis in biop-pATC dose-dependently: 28% of the cells treated with vandetanib 1 μM were apoptotic; with the higher vandetanib concentrations of 10, 25, or 50 μM the apoptotic percentage increased up to 33, 68, and 89%, respectively (P < 0.001; by ANOVA; Figure 4B).
To confirm the induced cell apoptosis, annexin V staining was used (data not shown).
No significant differences in sensitivity to lenvatinib, and vandetanib were observed between the tested cells obtained from FNA or biopsy.
Discussion
Lenvatinib, and vandetanib are able to exert an antineoplastic action in TC, and ATC. With this study we contribute to understand the lenvatinib, and vandetanib anticancer activity, in ATC, in fact: (1) to the best of our knowledge, this is the first study showing the possibility to screen the antineoplastic activity of lenvatinib, and vandetanib in vitro in primary neoplastic cells obtained from cytological samples of FNA; (2) moreover, primary cells from FNA showed a chemosensitivity to TKIs (lenvatinib, and vandetanib) considerably similar to the one in primary cells from biopsy.
Lenvatinib is an oral, multitargeted TKI of VEGFR1-VEGFR3, RET, fibroblast growth factor receptors 1–4 (FGFR1-FGFR4), PDGFRα, and v-kit Hardy-Zuckerman 4 feline sarcoma viral oncogene homolog (KIT) signaling networks involved in tumor angiogenesis (33).
In vitro studies evaluated the action of lenvatinib in preclinical models. Lenvatinib had an antineoplastic effect in xenograft models of different cell lines [5 differentiated thyroid cancer (DTC), 5 ATC, and 1 medullary thyroid cancer (MTC)], and had an antiangiogenic effect in 5 DTC and 5 ATC xenografts, while the antiproliferative activity was shown in vitro only in 2/11 thyroid cancer cell lines (i.e., RO82-W-1 and TT cells) (34). Moreover, it inhibited RET phosphorylation in TT cells with the activating mutation C634W (34).
In vivo phase II (35, 36), and phase III (37) studies in patients with aggressive DTC not responsive to radioiodine showed that lenvatinib administration ameliorated progression-free survival (PFS; median PFS 18.2 vs. 3.6 months with placebo). Following the results of this phase III study, lenvatinib has been approved for the treatment of patients with locally recurrent or metastatic, progressive, radioactive iodine refractory DTC (38).
Other anecdotal studies and a phase II clinical study have an antitumor effect of lenvatinib in ATC (39–43). Furthermore, we have recently reported a significant anticancer activity in vitro, and in vivo, in experimental models (23, 24).
Vandetanib is an oral once-daily TKI, with a strong antiangiogenic activity, and able to inhibit the activation of RET, EGFR, VEGFR-2, VEGFR-3, and a little of VEGFR-1 (44). A potent antineoplastic action of vandetanib was shown against transplantable MTC in nude mice (45). In patients with aggressive MTC, a phase III clinical study showed vandetanib improved PFS (30.5 vs. 19.3 months in the control group) (46). Food and Drug Administration, and European Medicines Agency approved it in 2011 in patients with locally advanced or metastatic MTC (47) and encouraging data have been shown also in aggressive DTC patients not responsive to the usual therapies (48, 49).
The results of this study agree with the ones of another paper reporting that vandetanib inhibits 8305C cells growth in vivo, and stops angiogenesis, decreasing vascular permeability (50), and also with our previous study (24).
Moreover, the results obtained in this study sustain the concept that lenvatinib and vandetanib have antiangiogenesis activity and are suggested for a multiple signal transduction inhibition (on EGFR, the RET tyrosine kinase, VEGFR) (51).
Considering that TKIs inhibitory effects can be bypassed by the activation of other kinases (52), multikinase inhibitors are more useful as they can block more than one single kinase in this way avoiding resistance (53–55).
It is interesting that the anti-proliferative action of lenvatinib and vandetanib did not depend on the presence/absence of V600EBRAF mutation in pATC.
To summarize we can hypothesize that, as shown in vivo (23, 24, 56), the antitumor effect of lenvatinib, and vandetanib in the tumoral cells could be linked to the following combination: (1) the antiproliferative action associated with the rise in apoptosis; (2) the inhibition of ERK1/2 phosphorylation (20); (3) the inhibition of tumor neovascularization (18, 56).
By disease-orientated in vitro drug testing conducted in human neoplastic cell lines, predictive values for the activity of clinical responses can be obtained (25, 26). A negative predictive value of 90% can avoid to administer patients with inactive chemotherapeutics and a positive preditive value of 60% can predict effectiveness in 60% of cases in vivo (27).
The observed disparity between in vitro and in vivo data can be caused by several factors: the metabolization and/or inactivation of the drugs in the tumor or by different organs in the body (as kidney and liver, etc.); the cellular resistance to drugs; the response to chemotherapeutics that is determined also by the growth curve of tumors (29).
Up to now primary ATC cells have been obtained from surgical materials obtained for therapeutic or diagnostic tecniques. In this study we obtain primary cells from FNA cytology in ATC.
Primary cultures have been obtained by needle aspiration biopsy in only 1 patient (57), and some papers reported of cutaneous needle track seeding after needle aspiration biopsy in TC patients (58, 59), but FNA cytology bypasses this problem and no signs of needle track seeding after FNA has been shown in our patients.
As FNA permits to collect material from a limited area of the tumor, that is the expression of a restricted cell population, this could select a cellular population not representative of the whole tumor. To rule out this possibility, the experiments were repeated with primary cell cultures obtained from bioptical samples in the same conditions. The results were quite similar to those observed in FNA-pATC, in this way excluding the hypothesis that FNA sampling might have brought to a cell population selection.
In conclusion: (1) primary cells obtained from FNA-pATC or biop-pATC, have a similar sensitivity to TKIs; (2) lenvatinib, and vandetanib are are able to decrease cell growth, increasing apoptosis in ATC; (3) the opportunity to test the sensitivity to different TKIs in each patient could avoid to administer ineffective (or even dangerous) drugs to patients, ameliorating also the effectiveness of the therapy; (4) this preclinical evaluation could permit to increase the effectiveness of lenvatinib and vandetanib in patients with ATC in whom the sensitivity has been shown in primary cells in vitro.
Ethics Statement
This study was carried out in accordance with the recommendations of the local ethical committee of the University of Pisa with written informed consent from all subjects. All subjects gave written informed consent in accordance with the Declaration of Helsinki. The protocol was approved by the the local ethical committee of the University of Pisa.
Author Contributions
SMF, CLM, SU, GM, PF, and AA gave substantial contribution in the conception and design of the work, and in writing the paper. AA and CLM revised it critically for important intellectual content. SMF, CLM, GE, FR, IR, LQ, SRP, SP, AP, SU, EB, GM, PF, and AA gave the final approval of the version to be published and agreed to be accountable for all aspects of the work in ensuring that questions related to the accuracy or integrity of any part of the work are appropriately investigated and resolved.
Conflict of Interest Statement
The authors declare that the research was conducted in the absence of any commercial or financial relationships that could be construed as a potential conflict of interest.
Abbreviations
ATC, anaplastic thyroid carcinoma; ATP, adenosine triphosphate; biop-pATC, primary cells from anaplastic thyroid cancer obtained from biopsy; EGFR, epidermal growth factor receptor; FCS, Fetal Calf Seurum; FNA-pATC, primary cells from anaplastic thyroid cancer obtained from fine needle aspiration; NIS, Sodium/Iodide Symporter; pATC, primary cells from anaplastic thyroid cancer; PCR-SSCP, PCR Single Strand Conformation Polymorphism; PFS, progression free survival; TKIs, tyrosine kinase inhibitors; TSH, thyroid-stimulating hormone; TPO, thyroperoxidase; Tg, thyroglobulin; VEGF, vascular endothelial growth factor; VEGFR, vascular endothelial growth factor receptor.
References
1. O'Neill JP, O'Neill B, Condron C, Walsh M, Bouchier-Hayes D. Anaplastic (undifferentiated) thyroid cancer: improved insight and therapeutic strategy into a highly aggressive disease. J Laryngol Otol. (2005) 119:585–91. doi: 10.1258/0022215054516197
2. Are C, Shaha AR. Anaplastic thyroid carcinoma: biology, pathogenesis, prognostic factors, and treatment approaches. Ann Surg Oncol. (2006) 13:453–64. doi: 10.1245/ASO.2006.05.042
4. Neff RL, Farrar WB, Kloos RT, Burman KD. Anaplastic thyroid cancer. Endocrinol Metab Clin North Am. (2008) 37:525–38. doi: 10.1016/j.ecl.2008.02.003
5. Miccoli P, Antonelli A, Spinelli C, Ferdeghini M, Fallahi P, Baschieri L. Completion total thyroidectomy in children with thyroid cancer secondary to the Chernobyl accident. Arch Surg. (1998) 133:89–93.
6. De Crevoisier R, Baudin E, Bachelot A, Leboulleux S, Travagli JP, Caillou B, et al. Combined treatment of anaplastic thyroid carcinoma with surgery, chemotherapy, and hyperfractionated accelerated external radiotherapy. Int J Radiat Oncol Biol Phys. (2004) 60:1137–43. doi: 10.1016/j.ijrobp.2004.05.032
7. Wartofsky L. Highlights of the American Thyroid Association Guidelines for patients with thyroid nodules or differentiated thyroid carcinoma: the 2009 revision. Thyroid (2009) 19:1139–43. doi: 10.1089/thy.2009.1599
8. Antonelli A, Fallahi P, Ferrari SM, Mancusi C, Colaci M, Santarpia L, et al. RET TKI: potential role in thyroid cancers. Curr Oncol Rep. (2012) 14:97–104. doi: 10.1007/s11912-012-0217-0
9. Podtcheko A, Ohtsuru A, Tsuda S, Namba H, Saenko V, Nakashima M, et al. The selective tyrosine kinase inhibitor, STI571, inhibits growth of anaplastic thyroid cancer cells. J Clin Endocrinol Metab. (2003) 88:1889–96. doi: 10.1210/jc.2002-021230
10. Nagaiah G, Fu P, Wasman JK, Cooney MM, Mooney C, Afshin D, et al. Phase II trial of sorafenib (bay 43-9006) in patients with advanced anaplastic carcinoma of the thyroid (ATC). J Clin Oncol. (2009) 27(suppl. 15):A6058. doi: 10.1200/jco.2009.27.15s.6058
11. Dziba JM, Marcinek R, Venkataraman G, Robinson JA, Ain KB. Combretastatin A4 phosphate has primary antineoplastic activity against human anaplastic thyroid carcinoma cell lines and xenograft tumors. Thyroid (2002) 12:1063–70. doi: 10.1089/105072502321085153
12. Straight AM, Oakley K, Moores R, Bauer AJ, Patel A, Tuttle RM, et al. Aplidin reduces growth of anaplastic thyroid cancer xenografts and the expression of several angiogenic genes. Cancer Chemother Pharmacol. (2006) 57:7–14. doi: 10.1007/s00280-005-0014-7
13. Schoenberger J, Grimm D, Kossmehl P, Infanger M, Kurth E, Eilles C. Effects of PTK787/ZK222584, a tyrosine kinase inhibitor, on the growth of a poorly differentiated thyroid carcinoma: an animal study. Endocrinology (2004) 145:1031–8. doi: 10.1210/en.2003-1228
14. Bauer AJ, Terrell R, Doniparthi NK, Patel A, Tuttle RM, Saji M, et al. Vascular endothelial growth factor monoclonal antibody inhibits growth of anaplastic thyroid cancer xenografts in nude mice. Thyroid (2002) 12:953–61. doi: 10.1089/105072502320908286
15. Prichard CN, Kim S, Yazici YD, Doan DD, Jasser SA, Mandal M, et al. Concurrent cetuximab and bevacizumab therapy in a murine orthotopic model of anaplastic thyroid carcinoma. Laryngoscope (2007) 117:674–9. doi: 10.1097/MLG.0b013e318031055e
16. Schiff BA, McMurphy AB, Jasser SA, Younes MN, Doan D, Yigitbasi OG, et al. Epidermal growth factor receptor (EGFR) is overexpressed in anaplastic thyroid cancer, and the EGFR inhibitor gefitinib inhibits the growth of anaplastic thyroid cancer. Clin Cancer Res. (2004) 10:8594–602. doi: 10.1158/1078-0432.CCR-04-0690
17. Nobuhara Y, Onoda N, Yamashita Y, Yamasaki M, Ogisawa K, Takashima T, et al. Efficacy of epidermal growth factor receptor-targeted molecular therapy in anaplastic thyroid cancer cell lines. Br J Cancer (2005) 92:1110–6. doi: 10.1038/sj.bjc.6602461
18. Antonelli A, Bocci G, La Motta C, Ferrari SM, Fallahi P, Ruffilli I, et al. CLM94, a novel cyclic amide with anti-VEGFR-2 and antiangiogenic properties, is active against primary anaplastic thyroid cancer in vitro and in vivo. J Clin Endocrinol Metab. (2012) 97:E528–36. doi: 10.1210/jc.2011-1987
19. Fallahi P, Ferrari SM, La Motta C, Materazzi G, Bocci G, Da Settimo F, et al. CLM29 and CLM24, pyrazolopyrimidine derivatives, have antitumoral activity in vitro in anaplastic thyroid cancer, with or without BRAF mutation. Endocrine (2016) 53:136–44. doi: 10.1007/s12020-015-0717-4
20. Antonelli A, Bocci G, Fallahi P, La Motta C, Ferrari SM, Mancusi C, et al. CLM3, a multitarget tyrosine kinase inhibitor with antiangiogenic properties, is active against primary anaplastic thyroid cancer in vitro and in vivo. J Clin Endocrinol Metab. (2014) 99:E572–81. doi: 10.1210/jc.2013-2321
21. Iyer PC, Dadu R, Ferrarotto R, Busaidy NL, Habra MA, Zafereo M, et al. Real-world experience with targeted therapy for the treatment of anaplastic thyroid carcinoma. Thyroid (2018) 28:79–87. doi: 10.1089/thy.2017.0285
22. Subbiah V, Kreitman RJ, Wainberg ZA, Cho JY, Schellens JHM, Soria JC, et al. Dabrafenib and trametinib treatment in patients with locally advanced or metastatic BRAF V600-mutant anaplastic thyroid cancer. J Clin Oncol. (2018) 36:7–13. doi: 10.1200/JCO.2017.73.6785
23. Ferrari SM, Bocci G, Di Desidero T, Elia G, Ruffilli I, Ragusa F, et al. Lenvatinib exhibits antineoplastic activity in anaplastic thyroid cancer in vitro and in vivo. Oncol Rep. (2018) 39:2225–34. doi: 10.3892/or.2018.6306
24. Ferrari SM, Bocci G, Di Desidero T, Ruffilli I, Elia G, Ragusa F, et al. Vandetanib has antineoplastic activity in anaplastic thyroid cancer, in vitro and in vivo. Oncol Rep. (2018) 39:2306–14. doi: 10.3892/or.2018.6305
25. Newell DR. Flasks, fibres and flanks–pre-clinical tumour models for predicting clinical antitumour activity. Br J Cancer (2001) 84:1289–90. doi: 10.1054/bjoc.2001.1797
26. Dollner R, Granzow C, Werner JA, Dietz A. Is there a role for chemosensitivity tests in head and neck cancer? Onkologie (2004) 27:310–5. doi: 10.1159/000077984
27. Schroyens W, Tueni E, Dodion P, Bodecker R, Stoessel F, Klastersky J. Validation of clinical predictive value of in vitro colorimetric chemosensitivity assay in head and neck cancer. Eur J Cancer (1990) 26:834–8.
28. Antonelli A, Ferrari SM, Fallahi P, Berti P, Materazzi G, Minuto M, et al. Thiazolidinediones and antiblastics in primary human anaplastic thyroid cancer cells. Clin Endocrinol. (2009) 70:946–53. doi: 10.1111/j.1365-2265.2008.03415.x
29. Antonelli A, Ferrari SM, Fallahi P, Berti P, Materazzi G, Marchetti I, et al. Evaluation of the sensitivity to chemotherapeutics or thiazolidinediones of primary anaplastic thyroid cancer cells obtained by fine-needle aspiration. Eur J Endocrinol. (2008) 159:283–91. doi: 10.1530/EJE-08-0190
30. Antonelli A, Ferrari SM, Fallahi P, Berti P, Materazzi G, Barani L, et al. Primary cell cultures from anaplastic thyroid cancer obtained by fine-needle aspiration used for chemosensitivity tests. Clin Endocrinol. (2008) 69:148–52. doi: 10.1111/j.1365-2265.2008.03182.x
31. Basolo F, Fiore L, Calvo S, Falcone V, Conaldi PG, Fontanini G, et al. Defective interleukin six expression and responsiveness in human mammary cells transformed by an adeno 5/SV40 hybrid virus. Br J Cancer (1996) 73:1356–61.
32. Antonelli A, Ferrari SM, Fallahi P, Frascerra S, Piaggi S, Gelmini S, et al. Dysregulation of secretion of CXC alpha-chemokine CXCL10 in papillary thyroid cancer: modulation by peroxisome proliferator-activated receptor-gamma agonists. Endocr Relat Cancer (2009) 16:1299–311. doi: 10.1677/ERC-08-0337
33. Costa R, Carneiro BA, Chandra S, Pai SG, Chae YK, Kaplan JB, et al. Spotlight on lenvatinib in the treatment of thyroid cancer: patient selection and perspectives. Drug Des Devel Ther. (2016) 10:873–84. doi: 10.2147/DDDT.S93459
34. Tohyama O, Matsui J, Kodama K, Hata-Sugi N, Kimura T, Okamoto K, et al. Antitumor activity of lenvatinib (e7080): an angiogenesis inhibitor that targets multiple receptor tyrosine kinases in preclinical human thyroid cancer models. J Thyroid Res. (2014) 2014:638747. doi: 10.1155/2014/638747
35. Cabanillas ME, Schlumberger M, Jarzab B, Martins RG, Pacini F, Robinson B, et al. A phase 2 trial of lenvatinib (E7080) in advanced, progressive, radioiodine-refractory, differentiated thyroid cancer: a clinical outcomes and biomarker assessment. Cancer (2015) 121:2749–56. doi: 10.1002/cncr.29395
36. Schlumberger M, Jarzab B, Cabanillas ME, Robinson B, Pacini F, Ball DW, et al. A phase II trial of the multitargeted tyrosine kinase inhibitor lenvatinib (E7080) in advanced medullary thyroid cancer. Clin Cancer Res. (2016) 22:44–53. doi: 10.1158/1078-0432.CCR-15-1127
37. Schlumberger M, Tahara M, Wirth LJ, Robinson B, Brose MS, Elisei R, et al. Lenvatinib versus placebo in radioiodine-refractory thyroid cancer. N Engl J Med. (2015) 372:621–30. doi: 10.1056/NEJMoa1406470
38. Nair A, Lemery SJ, Yang J, Marathe A, Zhao L, Zhao H, et al. FDA approval summary: lenvatinib for progressive, radio-iodine-refractory differentiated thyroid cancer. Clin Cancer Res. (2015) 21:5205–8. doi: 10.1158/1078-0432.CCR-15-1377
39. Yamazaki H, Shimizu S, Iwasaki H, Yoshida T, Suganuma N, Yamanaka T, et al. [Efficacy and Safety of Lenvatinib for Unresectable Anaplastic Thyroid Cancer]. Gan To Kagaku Ryoho (2017) 44:695–7.
40. Iñiguez-Ariza NM, Ryder MM, Hilger CR, Bible KC. Salvage lenvatinib therapy in metastatic anaplastic thyroid cancer. Thyroid (2017) 27:923–7. doi: 10.1089/thy.2016.0627
41. Oishi K, Takabatake D, Shibuya Y. Efficacy of lenvatinib in a patient with anaplastic thyroid cancer. Endocrinol Diabetes Metab Case Rep. (2017) 2017:136. doi: 10.1530/EDM-16-0136
42. Fukuhara T, Donishi R, Koyama S, Miyake N, Matsuda E, Fujiwara K, et al. Significant amelioration of tracheal stenosis following lenvatinib in a patient who has anaplastic thyroid carcinoma with bronchomediastinal infiltration: a case report. Case Rep Oncol. (2017) 10:175–81. doi: 10.1159/000457831
43. Tahara M, Kiyota N, Yamazaki T, Chayahara N, Nakano K, Inagaki L, et al. Lenvatinib for Anaplastic Thyroid Cancer. Front Oncol. (2017) 7:25. doi: 10.3389/fonc.2017.00025
44. Jasim S, Ozsari L, Habra MA. Multikinase inhibitors use in differentiated thyroid carcinoma. Biologics (2014) 8:281–91. doi: 10.2147/BTT.S57619
45. Johanson V, Ahlman H, Bernhardt P, Jansson S, Kölby L, Persson F, et al. A transplantable human medullary thyroid carcinoma as a model for RET tyrosine kinase-driven tumorigenesis. Endocr Relat Cancer (2007) 14:433–44. doi: 10.1677/ERC-06-0033
46. Wells SA Jr, Robinson BG, Gagel RF, Dralle H, Fagin JA, Santoro M, et al. Vandetanib in patients with locally advanced or metastatic medullary thyroid cancer: a randomized, double-blind phase III trial. J Clin Oncol. (2012) 30:134–41. doi: 10.1200/JCO.2011.35.5040
47. Cooper MR, Yi SY, Alghamdi W, Shaheen DJ, Steinberg M. Vandetanib for the treatment of medullary thyroid carcinoma. Ann Pharmacother. (2014) 48:387–94. doi: 10.1177/1060028013512791
48. Leboulleux S, Bastholt L, Krause T, de la Fouchardiere C, Tennvall J, Awada A, et al. Vandetanib in locally advanced or metastatic differentiated thyroid cancer: a randomised, double-blind, phase 2 trial. Lancet Oncol. (2012) 13:897–905. doi: 10.1016/S1470-2045(12)70335-2
49. AstraZeneca. Evaluation of Efficacy, Safety of Vandetanib in Patients With Differentiated Thyroid Cancer (VERIFY) [ClinicalTrials.gov Identifier: NCT01876784]. (2016). Available online at: https://www.clinicaltrials.gov/ct2/show/NCT01876784. Last Update Posted: September 6, 2017
50. Gule MK, Chen Y, Sano D, Frederick MJ, Zhou G, Zhao M, et al. Targeted therapy of VEGFR2 and EGFR significantly inhibits growth of anaplastic thyroid cancer in an orthotopic murine model. Clin Cancer Res. (2011) 17:2281–91. doi: 10.1158/1078-0432.CCR-10-2762
51. Taliani S, La Motta C, Mugnaini L, Simorini F, Salerno S, Marini AM, et al. Novel N2-substituted pyrazolo[3,4-d]pyrimidine adenosine A3 receptor antagonists: inhibition of A3-mediated human glioblastoma cell proliferation. J Med Chem. (2010) 53:3954–63. doi: 10.1021/jm901785w
52. Stommel JM, Kimmelman AC, Ying H, Nabioullin R, Ponugoti AH, Wiedemeyer R, et al. Coactivation of receptor tyrosine kinases affects the response of tumor cells to targeted therapies. Science (2007) 318:287–90. doi: 10.1126/science.1142946
53. van Amerongen R, Berns A. Targeted anticancer therapies: mouse models help uncover the mechanisms of tumor escape. Cancer Cell (2008) 13:5–7. doi: 10.1016/j.ccr.2007.12.019
54. Knight ZA, Lin H, Shokat KM. Targeting the cancer kinome through polypharmacology. Nat Rev Cancer (2010) 10:130–7. doi: 10.1038/nrc2787
55. Vitagliano D, De Falco V, Tamburrino A, Coluzzi S, Troncone G, Chiappetta G, et al. The tyrosine kinase inhibitor ZD6474 blocks proliferation of RET mutant medullary thyroid carcinoma cells. Endocr Relat Cancer (2010) 18:1–11. doi: 10.1677/ERC-09-0292
56. Antonelli A, Bocci G, La Motta C, Ferrari SM, Fallahi P, Fioravanti A, et al. Novel pyrazolopyrimidine derivatives as tyrosine kinase inhibitors with antitumoral activity in vitro and in vivo in papillary dedifferentiated thyroid cancer. J Clin Endocrinol Metab. (2011) 96:E288–96. doi: 10.1210/jc.2010-1905
57. Bravo SB, García-Rendueles ME, Seoane R, Dosil V, Cameselle-Teijeiro J, López-Lázaro L, et al. Plitidepsin has a cytostatic effect in human undifferentiated (anaplastic) thyroid carcinoma. Clin Cancer Res. (2005) 11:7664–73. doi: 10.1158/1078-0432.CCR-05-0455
58. Karwowski JK, Nowels KW, McDougall IR, Weigel RJ. Needle track seeding of papillary thyroid carcinoma from fine needle aspiration biopsy. a case report. Acta Cytol. (2002) 46:591–5. doi: 10.1159/000326884
Keywords: anaplastic thyroid cancer, fine needle aspiration, lenvatinib, primary anaplastic thyroid cancer cells, tyrosine kinase inhibitors, vandetanib
Citation: Ferrari SM, La Motta C, Elia G, Ragusa F, Ruffilli I, Quattrini L, Paparo SR, Piaggi S, Patrizio A, Ulisse S, Baldini E, Materazzi G, Fallahi P and Antonelli A (2018) Antineoplastic Effect of Lenvatinib and Vandetanib in Primary Anaplastic Thyroid Cancer Cells Obtained From Biopsy or Fine Needle Aspiration. Front. Endocrinol. 9:764. doi: 10.3389/fendo.2018.00764
Received: 02 October 2018; Accepted: 04 December 2018;
Published: 18 December 2018.
Edited by:
Bernadette Biondi, University of Naples Federico II, ItalyReviewed by:
Michele Minuto, Università degli Studi di Genova, ItalyRoberto Vita, Università degli Studi di Messina, Italy
Copyright © 2018 Ferrari, La Motta, Elia, Ragusa, Ruffilli, Quattrini, Paparo, Piaggi, Patrizio, Ulisse, Baldini, Materazzi, Fallahi and Antonelli. This is an open-access article distributed under the terms of the Creative Commons Attribution License (CC BY). The use, distribution or reproduction in other forums is permitted, provided the original author(s) and the copyright owner(s) are credited and that the original publication in this journal is cited, in accordance with accepted academic practice. No use, distribution or reproduction is permitted which does not comply with these terms.
*Correspondence: Alessandro Antonelli, YWxlc3NhbmRyby5hbnRvbmVsbGlAbWVkLnVuaXBpLml0