- Section of Pulmonary and Critical Care Medicine, Department of Medicine, The University of Chicago, Chicago, IL, United States
Air pollution is a complex mixture of gaseous and particulate components, each of which has detrimental effects on human health. While the composition of air pollution varies greatly depending on the source, studies from across the world have consistently shown that air pollution is an important modifiable risk factor for significantly increased morbidity and mortality. Moreover, clinical studies have generally shown a greater impact of particulate matter (PM) air pollution on health than the gaseous components. PM has wide-ranging deleterious effects on human health, particularly on the cardiovascular system. Both acute and chronic exposure to PM air pollution is associated with increased risk of death from cardiovascular diseases including ischemic heart disease, heart failure, and ischemic/thrombotic stroke. Particulate matter has also been shown to be an important endocrine disrupter, contributing to the development of metabolic diseases such as obesity and diabetes mellitus, which themselves are risk factors for cardiovascular disease. While the epidemiological evidence for the deleterious effects of PM air pollution on health is increasingly accepted, newer studies are shedding light on the mechanisms by which PM exerts its toxic effects. A greater understanding of how PM exerts toxic effects on human health is required in order to prevent and minimize the deleterious health effects of this ubiquitous environmental hazard. Air pollution is a growing public health problem and mortality due to air pollution is expected to double by 2050. Here, we review the epidemiological evidence for the cardiovascular effects of PM exposure and discuss current understanding about the biological mechanisms, by which PM exerts toxic effects on cardiovascular system to induce cardiovascular disease.
Introduction
Ambient air pollution is a growing global health problem estimated to contribute to as many as 3.1 million all-cause deaths per year (1–3). Exposure to air pollution is the largest environmental health risk and ranks ninth among modifiable disease risk factors, above other common factors such as low physical activity, high cholesterol, and drug use (2). Most of the excess deaths attributable to air pollution exposure are due to acute ischemic/thrombotic cardiovascular events. In addition to excess mortality, air pollution is associated with significant reductions in healthy life years and worker productivity (2, 4). Air pollution may also be an important endocrine disrupter, contributing to the development of metabolic diseases such as obesity and diabetes mellitus (5). While the developing world is most burdened by air pollution-associated health effects, the association between air pollution and mortality is still evident in developed countries where pollution levels are well below target standards (6, 7). The purpose of this article is (1) to introduce the reader to the major studies that have established the link between particulate matter (PM) air pollution and human cardiovascular and metabolic disease and (2) to discuss the mechanisms by which PM mediates its biologic effects. For systematic review of the connection between air pollution and human disease, we refer the reader to several recent systematic reviews and meta-analyses (8–14).
Air Pollution
Air pollution is a complex mixture of gaseous and particulate components, each of which has detrimental effects on cardiovascular and respiratory systems. The composition of air pollution varies greatly, depending on the source, emission rate, and sunlight and wind conditions. Gaseous components of air pollution include nitrogen dioxide (NO2), nitric oxide (NO), sulfur dioxide (SO2), ozone (O3), and carbon monoxide (CO) (2, 15, 16). Particulate matter (PM) components of air pollution consist of carbonaceous particles with associated adsorbed organic chemicals and reactive metals. Common components of PM include nitrates, sulfates, polycyclic aromatic hydrocarbons, endotoxin, and metals such as iron, copper, nickel, zinc, and vanadium (2, 15, 17). PM is subclassified according to particle size into (a) coarse (PM10, diameter <10μm), (b) fine (PM2.5, diameter <2.5μm), and (c) ultrafine (PM0.1, diameter <0.1μm). Coarse particles derive from numerous natural and industrial sources and generally do not penetrate beyond the upper bronchus. Fine and ultrafine particles are produced through the combustion of fossil fuels and represent a greater threat to health than coarse particles as they penetrate into the small airways and alveoli (16–19). While the organic and metal components of particles vary with location, levels of PM2.5 have consistently correlated with negative cardiovascular outcomes regardless of location (15).
Epidemiological Studies Linking Pm Exposure to Morbidity and Mortality in Humans
The association between high levels of PM air pollution and adverse health outcomes has been known since the first half of the twentieth century. Smog incidents in Meuse Valley, Belgium (1930), Donora, Pennsylvania (1948), and London, UK (1952) acutely caused increased hospitalizations and deaths, particularly in the elderly and those with preexisting cardiac and respiratory diseases. An estimated 4,000 people died as a direct result of the London smog with 100,000 more suffering adverse health effects (20, 21). These incidents resulted in policy changes including the implementation of Clean Air Act in 1970 (22). The reduction in PM levels have led to gradual reduction in PM-associated morbidity and mortality; however, recent epidemiologic studies still consistently show a link between PM exposure and cardiopulmonary mortality.
Short-Term Exposure Studies
The increased deaths due to the smog in Meuse Valley, Donora, and London clearly suggested that acute exposure to air pollution is associated with adverse health outcomes. These classic cases of air pollution-induced mortality represent extreme examples, with the London smog reaching air PM concentrations of 4.5 mg/m3 (World Health Organization current safety guideline is 25 μg/m3) (21). A large number of short-term exposure studies have evaluated the associations between less extreme levels of air pollution and daily changes in mortality (15, 18). A recent meta-analysis of 110 peer-reviewed studies revealed that every 10 μg/cm3 increase in PM2.5 concentration was associated with a 1.04% (95% CI 0.52%-1.56%) increase in all-cause mortality (10). Hospitalizations and mortality due to cardiovascular and respiratory illnesses were positively correlated with increases in PM2.5 concentrations.
Several large, multi-city studies have been conducted in both North America and Europe, the largest being the NMMAPS (National Morbidity, Mortality, and Air Pollution Study) (23–25) and APHEA (Air Pollution and Health: A European Approach) (26, 27) studies. Findings from these studies were remarkably consistent and demonstrated that PM levels are significantly associated with daily all-cause, cardiovascular, and pulmonary mortality. Seasonal and regional variations existed in both studies possibly attributable to different sources of pollutants, meteorological conditions, and population differences. For example, the APHEA study found a stronger effect of PM on daily mortality in cities with a larger contribution of traffic emissions to total PM. This is in agreement with a recent study on triggers of myocardial infarction (MI) in which traffic exposure was found to be as significant of a trigger of MI as physical exertion and alcohol use (28). The NMMAPS study also found that the relationship between PM exposure and mortality was independent of gaseous co-pollutants, including NO2, CO, and SO2.
Studies carried out in Asia and the developing world have generally shown smaller effects on daily mortality due to PM than studies from the United States and Europe. A recent meta-analysis of 85 studies from 12 low- and middle-income countries showed a 0.47% (95% CI 0.34-0.61) increase for cardiovascular mortality and 0.57% (95% CI 0.28-0.86) increase for respiratory mortality for every 10 μg/cm3 increase in PM2.5 concentration (14). The cities covered by this analysis have mean PM2.5 levels ranging from 56 to 179 μg/cm3, which is significantly higher than the mean the PM2.5 levels in cities in the US and Europe. The reduced concentration-response relationship between PM2.5 levels and mortality in these countries is likely due to the higher baseline PM level seen in these countries. Indeed, current evidence suggests that the concentration-response relationship between PM2.5 levels and mortality is biphasic (29–33). A steep concentration-response function is observed at lower PM concentrations, while the curve flattens at higher concentrations. A recent study from Beijing, China found that while the slope of the concentration-response curve flattened at higher PM concentrations, there was no saturation for increased risk of ischemic heart disease mortality, even at PM concentrations as high as 500 μg/cm3 (33).
The biphasic relationship between PM concentration and adverse health outcomes means that the major health benefits from reducing PM levels will occur in countries with already cleaner air and that improvements in cardiovascular health will be more difficult to achieve in countries with higher levels of air pollution unless they can achieve a drastic improvement in PM concentrations. The results of the NMMAPS and APHEA studies suggest that there is no “safe” threshold under which increases in PM are not associated with increased deaths.
Long-Term Exposure Studies
In addition to studies on the acute effects of PM exposure, studies on the effect of chronic exposure to PM have revealed negative effects on long-term health outcomes. The first of these was the Harvard Six Cities study, which prospectively measured the effect of air pollution on mortality in a cohort of 8,111 adults while controlling for individual risk factors, including smoking, body mass index, occupational exposures, hypertension, and diabetes (34). The adjusted mortality rate ratio for the most polluted cities compared with the least polluted cities was 1.26 (95% CI 1.08-1.47). Air pollution, particularly PM2.5 and sulfates was positively associated with death from lung cancer and cardiopulmonary diseases.
A larger study, the ACS Cancer Prevention II study linked risk factor data for 552,138 adults with air pollution data and mortality statistics (35, 36). Both PM2.5 and SO2 were positively correlated with all-cause, lung cancer, and cardiopulmonary mortality and every 10 μg/cm3 increase in PM2.5 was associated with a 4, 6 and 8% increased risk of all-cause, cardiopulmonary, and lung cancer mortality, respectively. Coarse particles and gaseous co-pollutants other than SO2 were not significantly related to mortality.
A study on 22 European cohorts within the multicenter European Study of Cohorts for Air Pollution Effects (ESCAPE) found an increased hazard ratio for all-cause mortality of 1.07 (95% CI 1.02-1.13) per 5 μg/cm3 PM2.5 (37). Significant associations persisted even among participants exposed to PM2.5 levels below the European annual mean limit value of 25 μg/cm3.
Overall, the evidence from both short-term and long-term exposure studies demonstrates a consistent association between increased air pollution exposure and mortality. While the magnitude of this effect is small, the ubiquity of air pollution exposure makes it a significant source of early mortality. A global assessment of mortality attributable to several risk factors, including air pollution was carried out in the Global Burden of Diseases, Injuries, and Risk Factors Study 2015 (GBD 2015) (38). This study estimated that PM2.5 is the fifth-ranking mortality risk factor, leading to 4.2 million deaths and 103.1 million disability-adjusted life-years in 2015. The largest number of deaths attributable to air pollution occurred in China with an estimated 1.11 million deaths. These numbers are similar to the findings of a recent study from China that attributed 40.3% of deaths due to stroke, 26.8% of deaths due to ischemic heart disease, 23.9% of deaths due to lung cancer, and 18.7% of deaths due to chronic obstructive pulmonary disease (COPD) to PM2.5 exposure (39). According to the GBD 2015 study, these represent the 1st, 2nd, 4th, and 5th leading causes of death in China, respectively (12).
Susceptibility to PM-Induced Morbidity and Mortality
Enhanced risk of cardiovascular death from PM exposure has been linked to old age, low socioeconomic status, preexisting heart and lung disease, and smoking. The APHENA (Air Pollution and Health: A Combined European and North American Approach) study, which analyzed data from the NMMAPS and APHEA studies found that the elderly and unemployed are at higher risk for the deleterious health effects associated with short-term exposure to PM (40). The ACS study found that mortality from ischemic heart disease was positively correlated with chronic PM2.5 exposure among never smokers, former smokers, and current smokers (41). However, the risk for death due to arrhythmia, hearth failure, and cardiac arrest was not elevated by PM2.5 for never smokers, but significantly elevated for former and current smokers.
Studies have not shown a clear association between race and susceptibility to PM-induced health effects (42–44). However, air pollution in non-white neighborhoods tends to be higher than in majority-white areas, resulting in exposure disparities (45). Indeed, inter-city gradients of PM (i.e., gradients among communities within a city) are associated with larger negative health effects than the average PM measurements within a city (46, 47).
Finally, it has been suggested that women may be more susceptible than men to the PM-induced health effects. Particularly, robust risk estimates have been reported for studies that include only women. The Women's Health Initiative Observational Study found that every 10 μg/cm3 increase in PM2.5 was associated with a 76% increase in fatal cardiovascular events while the Nurses' Health Study found that every 10 μg/cm3 increase in PM10 was associated with a 43% increase fatal coronary heart disease (48, 49). More recent large studies have given conflicting results (42, 43). On a global scale, exposure disparities may play a role in increased risk for women as use of biomass fuels for cooking in sub-Saharan Africa and south Asia expose women to disproportionately high levels of indoor air pollution (50).
Interventional Studies
The implementation of the 1970 Clean Air Act and following amendments resulted in a progressive decline in PM2.5 levels in the United States. As would be expected from the concentration-response curve of PM2.5 vs. mortality, extended analysis of the NMMAPS and Harvard Six Cities Study, among others, have revealed that reductions in PM2.5 concentrations over time are associated with reductions in mortality risk (51–53). Pope et al. showed that a reduction of 10 μg/cm3 in PM2.5 levels increased the life expectancy by 0.61 ± 0.20 years (54). Similar reductions in mortality have been seen after policy changes regulating the use of diesel in Tokyo, Japan and coal in Dublin, Ireland (55, 56).
Exposure to Pm and Cardiovascular Disease
Deaths due to air pollution exposure result primarily from cardiovascular causes, with stronger associations with adverse effects of PM compared with gaseous co-pollutants (2, 7, 15). Chronic and acute exposure to elevated PM2.5 levels is closely associated with elevated risks for ischemic heart disease, heart failure, and cerebrovascular disease. Air pollution exacerbates existing heart conditions and appears to have a role in disease development.
Both long- and short-term studies have associated PM2.5 exposure with increased risk of fatal and non-fatal ischemic heart disease (33, 41, 48, 57). Risk of myocardial infarction is also associated with PM2.5 exposure (28, 58). The ACS study found increased risk of heart failure with PM2.5 exposure, although to a lesser degree than the association with ischemic heart disease. Additional studies and meta-analyses have associated both chronic and acute PM2.5 exposure with heart failure (9, 41, 59, 60). Significant associations also exist between PM2.5 exposure and cerebrovascular disease (48, 61). Short-term studies have shown that elevations in pollution increase the risk of ischemic, but not hemorrhagic stroke (8, 62).
Subclinical Effects
Exposure to PM air pollution is also correlated with subclinical pathologies underlying cardiovascular disease. These include systemic inflammation and oxidative stress, atherosclerosis, thrombosis, endothelial dysfunction, hypertension, cardiac remodeling, and arrhythmia.
Inflammation, Oxidative Stress, and Atherosclerosis
PM inhalation induces inflammatory responses both within the lung and systemically. Exposure of human volunteers to PM via inhalation for 2 h resulted in increased pulmonary neutrophil numbers (63). Circulating levels of C-reactive protein, fibrinogen, IL-1β (interleukin-1β), IL-6 (interleukin-6), GM-CSF (Granulocyte-Macrophage Colony Stimulating Factor), and TNF-α (Tumor Necrosis Factor-α) have been shown to correlate with environmental PM exposure levels (63–66).
PM exposure is also associated with systemic markers of oxidative stress, including atherogenic precursors such as oxidized lipids (67–70). Using carotid artery intima-media thickness as a surrogate for atherosclerotic progression, several studies, including the Multi-Ethnic Study of Atherosclerosis (MESA) have shown that intima-media thickness correlates positively with long-term exposure to PM (71–74). Other studies have shown that coronary artery calcification correlates with residence in a city center or near a major roadway (75, 76).
Studies in the atherosclerosis model apolipoprotein E (ApoE) knockout mice have shown that exposure to PM results in elevated levels of oxidized low-density lipoproteins, lipid peroxidation, and systemic oxidative stress. This is associated with increased atheroma burden, and increased plaque cellularity and lipid content (77–80).
Hypercoagulability and Thrombosis
Exposure to PM has been shown to induce a prothrombotic state, which may play a role in its ability to cause arterial thrombotic (myocardial infarction, ischemic/thrombotic cerebrovascular events) and venous thrombotic events (deep venous thrombosis) (81, 82). Exposure to PM induces the production of fibrinogen, and other factors that play a role in hemostasis including Von Willebrand factor, sCD62P, and sCD40L (65, 83–85). In addition to prothrombotic pathways, antifibrinolytic pathways are also activated by PM exposure. Plasminogen Activator Inhibitor-1 (PAI1) has been shown to be upregulated by PM exposure and tissue Plasminogen Activator (t-PA) activity is inhibited (85–88). These findings correlate with previous reports of PM-associated increases in plasma viscosity, platelet activation, and ex vivo coagulation (89–92).
The 2008 Summer Olympics in Beijing, China offered a unique opportunity to study the effects of PM exposure on cardiovascular biomarkers. As government-mandated restrictions on industrial and vehicular emissions were enacted, particulate and gaseous pollutants decreased. In test subjects, this corresponded with decreases in circulating levels of sCD62P and Von Willebrand factor. When restrictions were eased after the games, levels of these factors increased to pre-Olympic levels (84).
Endothelial Dysfunction, Increased Blood Pressure, and Cardiac Remodeling
Both short- and long-term exposure to PM has been correlated with changes in vascular function. Controlled exposure to diesel exhaust or concentrated ambient particles leads to vascular dysfunction characterized by acute arterial vasoconstriction and inhibition of response to vasodilators (86, 93–96). The MESA study found that chronic exposure to PM2.5 correlated with decreased flow-mediated dilation of the brachial artery and retinal arteriolar narrowing (97, 98).
Several studies have reported associations between chronic PM exposure and development of hypertension (99, 100). Controlled-exposure studies using acute exposure of humans to concentrated ambient particles or diesel exhaust have demonstrated rapid increases in systolic blood pressure following exposure (101, 102). Exposure to PM has also been shown to increase the risk of gestational hypertension and pre-eclampsia (11, 103, 104).
Finally, traffic exposure has been associated with both left and right ventricular hypertrophy, suggesting that pollution-associated vasoconstriction and hypertension may exacerbate congestive heart failure (105, 106). Similar results have been found in mice. A 3-month exposure of mice to concentrated ambient particles exacerbates cardiac hypertrophy and fibrosis in response to angiotensin II infusion (107). A longer, 9-month exposure of mice to concentrated ambient particles was sufficient to result in increased ventricular size, systolic and diastolic dysfunction, and myocardial fibrosis (108).
Cardiac Electrical Changes and Irregular Heart Rhythm
In patients with implantable cardioverter defibrillators, positive associations have been made between short-term increases in air pollution and incidence of cardiac arrhythmias including atrial fibrillation, ventricular fibrillation, and ventricular tachycardia (109–112). Exposure to air pollution is also associated with, increased heart rate, electric instability, ectopic beats, ST-segment depression, repolarization irregularities, and changes in heart-rate variability (65, 113–120).
The strongest correlations between arrhythmia and pollution exposure have been found when analysis was restricted to a subgroup of patients with frequent arrhythmias, suggesting that risk of arrhythmia is restricted to the most susceptible individuals (109). Similarly, a murine study found that wild-type mice did not exhibit arrhythmias after exposure to PM; however, significant arrhythmias were seen in mice engineered to exhibit cardiomyopathic changes that closely resemble congestive heart failure (121). In rats, greater effects of PM exposure on arrhythmogenesis were seen in animals previously injected with monocrotaline to induce pulmonary vascular inflammation and hypertension (122).
Metabolic Syndrome and Insulin Resistance
Several clinical studies have linked PM with insulin resistance and type II diabetes mellitus (DM) suggesting PM as a modifiable risk factor for DM, an important risk factor for cardiovascular disease. Significant positive correlations between PM exposure and fasting insulin levels and insulin resistance have been found in both adults and children (123–125). A large study conducted using data from both the United States Centers for Disease Control and Prevention and the Environmental Protection Agency found that diabetes prevalence increases by 1% with each 10 μg/m3 PM2.5 (126). Another study of over 3,500 individuals in Germany revealed that each 1 μg/m3 of traffic-related PM2.5 was associated with a relative risk for type II DM of 1.36 (95% CI.97-1.89) after adjusting for variables including age, gender, BMI, and socioeconomic status (127). This effect size was similar to that obtained by comparing individuals living close to a major road with those that live farther than 200 meters from a major road (127). A recent meta-analysis suggests that the correlation of PM with DM is stronger in women (13). How PM-associated insulin resistance and type II DM may interact with other PM-associated health effects to affect cardiovascular system is a complex question. For example, diabetics have been shown to be more susceptible to PM-associated endothelial dysfunction (128).
Animal studies have confirmed the effect of PM exposure on insulin sensitivity. Mice genetically susceptible to type II DM, or mice fed high-fat diet and exposed to PM exhibit increased insulin resistance, glucose intolerance, elevated fasting glucose, and increased visceral adiposity when compared with mice exposed to filtered air (129–131). Interestingly, young mice exposed to PM beginning at 3 weeks of age developed homeostatic insulin resistance after 10 weeks of exposure without additional stress indicating a developmental window of susceptibility to the effects of PM (132).
Biological Mechanisms
Recent controlled exposure studies in both humans and animals have shed light on the biological mechanisms behind PM-induced cardiovascular disease (Figure 1). There are presently three hypotheses on the mechanisms by which PM exposure exerts its biological effect (7, 15, 16, 133). The first hypothesis proposes that PM inhalation activates inflammatory responses in the lung leading to a “spillover” effect and systemic inflammation, which promotes thrombosis, endothelial dysfunction, and atherosclerosis. The second hypothesis suggests that inhaled PM activates sensory receptors in the lung, leading to imbalance of the autonomic nervous system (ANS), favoring sympathetic pathways and leading to alterations in heart rate, vasoconstriction, endothelial dysfunction, and hypertension. The third hypothesis proposes that some particles, particularly ultrafine particles (PM0.1) can enter the circulation from the lung and interact directly with target tissues; however, this mechanism remains controversial. Recent evidence suggests that majority of ultrafine particles are cleared from the lung in a similar manner as larger particles (i.e., alveolar macrophage-mediated clearance to the larynx) (134, 135). Nevertheless, soluble material adsorbed to the surface of inhaled particles may pass into the circulation. Further studies will be required to determine whether any portion of inhaled particles is translocated into the bloodstream and if so, whether these translocated particles contribute to PM-associated pathologies. We will discuss the evidence for inflammatory and ANS signaling as regulators of the biologic effects of PM exposure. It should be noted that these pathways are not mutually exclusive. In fact, there is significant evidence that while each plays an important role in mediating the cardiovascular effects of pollution exposure, they may also interact to drive the PM-induced health effects.
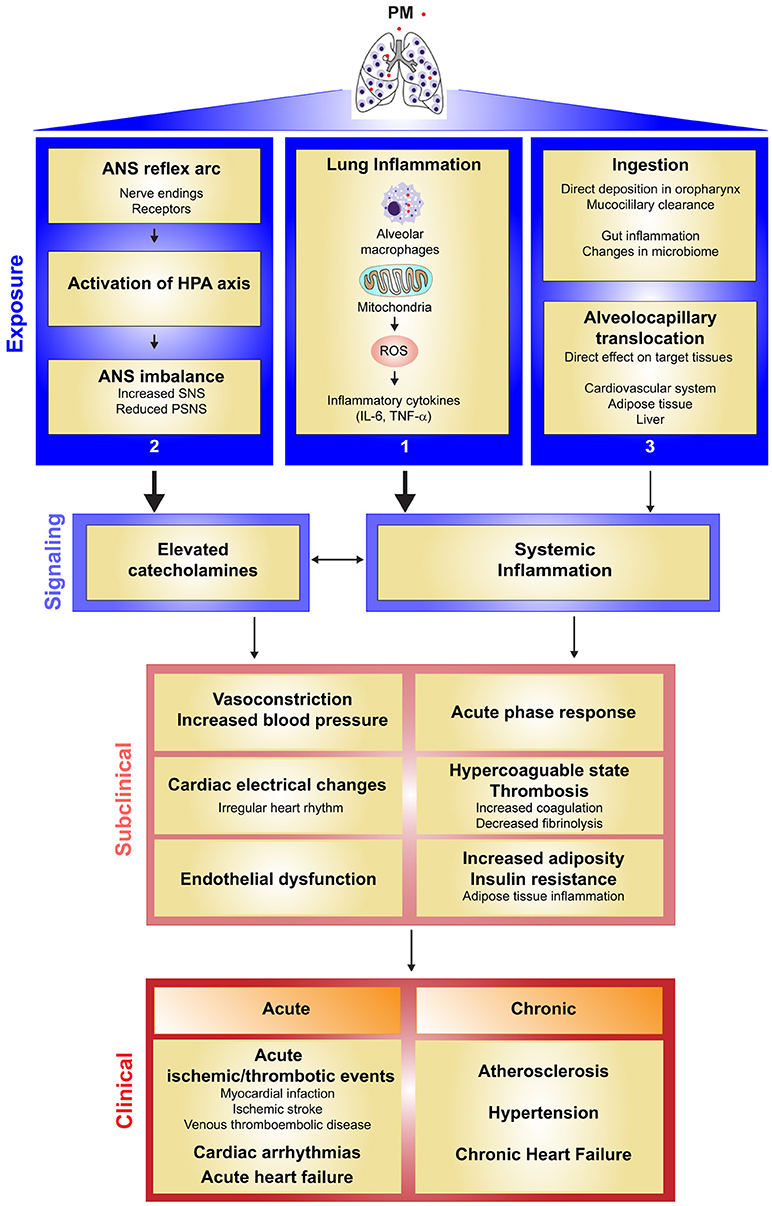
Figure 1. Current evidence for the mechanisms by which particulate matter air pollution causes cardiovascular health effects.
Exposure Level: PM exposure is hypothesized to exert its effects on the cardiovascular system by three routes: (1) PM induces an inflammatory response in the lung. PM acts on the cells of the lung, including alveolar macrophages, leading to mitochondrial reactive oxygen species (mROS)-dependent pro-inflammatory cytokine production. (2) Inhaled PM acts on sensory receptors in the lung, promoting activation of the hypothalamic pituitary adrenal (HPA) axis and sympathetic pathway activation in the autonomic nervous system (ANS). (3) Other effects of PM exposure may be mediated by translocation of particles into the circulatory system, or by particle ingestion, which may promote inflammation in the gut.
Signaling Level: Cytokines produced into the lung “spillover” into the circulation, leading to a systemic state of inflammation. Translocated particles as well as inflammation resulting from particle injection may also contribute to a general state of systemic inflammation. Sympathetic activation leads to elevated levels of circulating catecholamines.
Subclinical Level: Systemic inflammation and elevated catecholamine levels act on target cells leading to acute phase response, hypercoagulable state (activation of coagulation, and suppressed fibrinolysis), vasoconstriction, increased blood pressure, cardiac electrical changes, endothelial dysfunction, and increased adiposity and insulin resistance complicated by adipose tissue inflammation. Elevated catecholamine levels due to ANS imbalance further increase inflammation. Sympathetic activation leads to increased catecholamine production, which increases heart rate and promotes vasoconstriction, endothelial dysfunction, and hypertension.
Clinical Level: The combined effects of systemic inflammation and sympathetic activation on their cellular targets lead to the clinical effects of PM on cardiovascular disease. These effects are seen at both the acute level (acute ischemic/thrombotic events, cardiac arrhythmias, or acute heart failure), or at the chronic level (atherosclerosis, hypertension, and chronic heart failure).
Reactive Oxygen Species and Mitochondria
It is widely accepted that PM exerts many of its biologic effects via the generation of reactive oxygen species (ROS) and induction of oxidative stress responses (19, 136). Exposure to air pollution is associated with systemic markers of oxidative stress (67, 69, 70). At the cellular level, many cell types have been shown to respond to in vitro PM exposure with elevations in cellular ROS levels and oxidative stress. This includes nasal, airway, and lung epithelial cells (137–141), macrophages (142–144), endothelial cells (145, 146), cardiomyocytes (147, 148), gastrointestinal epithelial cells (149), epidermal keratinocytes (150), and corneal epithelial cells (151). Moreover, elevated ROS levels are required for PM-induced biologic effects as antioxidant treatment or inhibition of oxidant production is sufficient to inhibit downstream pathways including proinflammatory cytokine production and induction of apoptosis (137, 138, 152–155).
While PM-adsorbed chemicals and metals are capable of generating free radicals inside cells, cells can respond to stimuli with generation of ROS as signaling molecules (156). Mitochondrial generation of ROS has been found to be an important signaling regulator of the cellular response to PM. Indeed exposure to PM has been found to alter mitochondrial morphology and function (142, 151, 157, 158). PM exposure leads to oxidation of redox probes specifically targeted to mitochondria (149, 159). Furthermore, cells genetically engineered to lack mitochondrial ROS production or cells treated with mitochondria-targeted antioxidants or respiratory chain inhibitors have inhibited responses to PM, strongly supporting the role of mitochondria-derived ROS in PM-induced biologic effects (138, 153, 159–161).
Alveolar Macrophages
Alveolar Macrophages (AMs) reside on the luminal epithelial surface of alveoli and are crucial for lung development, surfactant homeostasis, and immune surveillance (162). These cells also represent a critical signaling node for the effects of PM on target organs such as heart and vasculature. Treatment of AMs in vitro with PM elicits a transcriptional upregulation of inflammatory cytokines including TNFα, IL-1β, IL-6, IL-8 and GM-CSF (64, 163). Lung epithelial cells also respond to PM treatment in vitro and the interaction between AMs and epithelial cells may regulate their response to PM (164, 165); however, studies in animals suggest that the response of AMs to PM exposure is required and sufficient for downstream cardiovascular effects.
Elimination of AMs in mice using liposomal clodronate inhibits both pulmonary and systemic accumulation of IL-6 or TNFα protein after exposure to PM (166, 167). The prothrombotic and endothelial-activating effects of PM exposure were also inhibited by clodronate, suggesting that the pro-inflammatory responses initiated by AMs in the lung promote the systemic and cardiovascular effects of PM exposure (166, 167). The ability of AMs to influence systemic responses to PM is supported by studies on bone marrow activation in rabbits. Instillation of PM into the lungs of rabbits results in increased release of polymorphonuclear leukocytes from bone marrow, with elevated numbers of circulating band cells, a marker of bone marrow activation (168). Similar responses have been seen in humans (169). Instillation of supernatants from human AMs treated with PM ex vivo has a similar ability to activate rabbit bone marrow as instillation of PM suggesting that PM-induced inflammatory responses in AMs regulate the systemic effects of PM on target cells and organs (170).
Pro-inflammatory Cytokines
Interleukin 6
Initially identified as a regulator of T cell activation and B cell differentiation, IL-6 is a pleotropic cytokine with key roles in diverse biological processes such as immune responses, the acute phase response and inflammation, hematopoiesis, vascular function, lipid metabolism, and neuroendocrine regulation (171, 172).
IL-6 is a major regulator of the acute phase response and stimulates hepatocytes to synthesize acute phase proteins, particularly C-reactive protein and coagulation factors (173, 174). IL-6 has been shown to increase the expression of coagulation factors including fibrinogen, tissue factor, Factor VIII and von Willebrand factor, and decrease anticoagulants including protein S and antithrombin (174). Recent reports in IL-6-deficient mice have demonstrated the critical role of IL-6 in promoting thrombotic events downstream of PM exposure. Exposure of wild-type mice to PM led to accelerated blood clotting and vascular thrombosis after FeCl3 application (166, 175). This accelerated clotting was associated with increased platelet count, increased Factor VIII activity, increased plasma thrombin antithrombin complexes, increased lung tissue factor levels, reduced prothrombin time, and reduced activated partial thromboplastin time. In IL-6 deficient mice, no increase in clotting capability was seen after exposure to PM, demonstrating the key role that IL-6 plays in regulating the prothrombotic effects of PM exposure.
Elevations in IL-6 may also promote vascular dysfunction after PM exposure. Administration of IL-6 to mice promotes endothelial dysfunction and impaired endothelium-dependent vasodilation (176). As in humans, exposure of mice to PM impairs vasodilation in response to acetylcholine. No significant change in vascular response was noted in IL-6 deficient mice after exposure to PM (177). Interestingly, in this same report, the authors demonstrated that instillation of recombinant IL-6 into the lungs of IL-6 knockout mice resulted in systemic elevations in IL-6 after endotoxin-mediated lung injury (177). While endotoxin is a stronger inducer of lung injury than PM, this finding provides support for the hypothesis that inflammatory cytokine induction in the lung can spillover into the circulation to promote systemic effects.
Tumor Necrosis Factor-α
TNFα is a pleiotropic cytokine originally identified as an endotoxin-inducible molecule with anti-cancer activity (178). TNFα has since been shown to be a critical regulator of the cytokine cascade in many inflammatory diseases and is a therapeutic target for a number of chronic inflammatory diseases (179, 180). Similar to IL-6, TNFα expression is induced in both AMs and lung epithelial cells after exposure to PM (64, 163, 165). TNFα also accumulates in both the lung and systemically after exposure of mice to PM (167, 175, 181, 182). Elimination of AMs with liposomal clodronate inhibited the accumulation of TNFα in plasma after exposure of mice to PM.
Surprisingly, studies in TNFα-deficient mice demonstrate that PM-mediated recruitment of neutrophils to the lung, as well as induction of cytokines, including IL-6, IL-8, and MCP-1 (monocyte chemoattractant protein 1), are independent of TNFα. (183, 184). This is despite the fact that TNFα is a known regulator of IL-6 expression (185, 186). TNFα was shown to be required for accumulation of PAI1 after exposure of mice to PM; however, TNFα remained dispensable for PM-mediated pro-thrombotic effects (175). More recent studies have shown that pulmonary T-cell recruitment is impaired after PM exposure in TNFα deficient mice (182) and that endothelial activation and impaired cardiac contractile function after PM exposure can be rescued by treatment with a TNFα-neutralizing antibody (167, 181).
Sympathetic Activation and Endogenous Catecholamines
The effects of PM exposure on heart rate variability and blood pressure indicate that air pollution may regulate the balance between the sympathetic and parasympathetic arms of the autonomic nervous system. Indeed, a study of Brazilian sugarcane harvesters found that during harvest time, when ambient PM is high due to sugarcane burning, workers' blood pressure and heart rate variability measurements correlated significantly with sympathetic nerve activity measured by microneurography (187). A more recent study found that elevated exposure to PM was associated with increased serum levels of norepinephrine and epinephrine, among other stress hormones (188).
Increased catecholamine levels have also been found in mice exposed to PM and this sympathetic activation has been shown to augment the inflammatory response and prothrombotic effects downstream of PM exposure (153). Deletion of β-adrenergic receptors either globally or in AMs alone resulted in reduced IL-6 accumulation after PM exposure. Inhibition of β-adrenergic receptors either genetically or pharmacologically prevented the prothrombotic effects of PM exposure while treatment of mice with the β-agonist formoterol further increased IL-6 accumulation and thrombosis after PM exposure (153). These findings from an experimental study have recently been confirmed in humans (188). Collectively, these results suggest that the PM-induced inflammation and modulation of the autonomic nervous system both contribute to the prothrombotic effects of PM exposure.
Increased Adiposity and Adipose Inflammation
Animal studies have shown that long-term PM exposure leads to increased adipocyte size and increased visceral fat mass (129, 189). PM exposure induced genes associated with lipogenesis in adipose tissue, impaired adipose mitochondrial function, and led to changes in circulating levels of leptin and adiponectin (130, 189–191). This increased adiposity was also associated with associated with increased macrophage infiltration into adipose tissue and induction of pro-inflammatory programs (129, 189).
Adipose inflammation is linked with insulin resistance (192). Indeed mice deficient for the NADPH oxidase subunit p47phox exhibited improved adipose inflammation and insulin resistance in response to PM exposure (132). Similar findings were found in mice deficient for the chemokine receptor CCR2 (131)
In humans, living near a major roadway (<60 m) is associated a 0.37 kg/m2 (95% CI: 0.10 to 0.65 kg/m2) increase in body mass index (BMI) when compared with those who live over 440 m away from a major road (193). The finding that inflammation is associated with PM-induced insulin resistance in mice is consistent with the findings of the SALIA (Study on the Influence of Air Pollution on Lung Inflammation and Aging), which demonstrated that Complement C3c, a marker of subclinical inflammation, is associated with PM exposure in a cohort of non-diabetic women. Elevated C3c was a strong independent predictor of diabetes development (194).
Animal studies have confirmed the effect of PM exposure on insulin sensitivity. Mice genetically susceptible to type II DM, or mice fed high-fat diet and exposed to PM exhibit increased insulin resistance, glucose intolerance, elevated fasting glucose, and increased visceral adiposity when compared with mice exposed to filtered air (129–131). Interestingly, young mice exposed to PM beginning at 3 weeks of age developed homeostatic insulin resistance after 10 weeks of exposure without additional stress indicating a developmental window of susceptibility to the effects of PM (132).
Epigenetic Changes
How the effects of air pollution exposure may endure after exposure is not clear; however studies from mice suggest that exposure early in life can have long lasting effects. Exposure of pregnant mice to diesel exhaust resulted in an increased susceptibility to pressure overload-induced heart failure in pups raised to adulthood (195). While the mechanisms behind this susceptibility is unknown, a potential mechanism for long-term disease susceptibility may lie in epigenetic changes that occur during exposure.
Epigenetic regulation of gene expression can result in transient, and potentially permanent changes in tissue function. Although studies are limited, air pollution exposure has been shown to affect multiple epigenetic mechanisms, including alterations in DNA methylation and histone modifications. Hypermethylation was observed in the DNA from sperm collected from mice exposed to particulate air pollution for 10 weeks when compared with mice exposed to filtered air (196). These changes were still evident when mice were examined 6 weeks after termination of exposure. In humans, hypomethylation in DNA repetitive elements has been seen in circulating leukocytes after exposure to particles (197, 198). Furthermore hypomethylation of LINE-1 elements correlates with increased risk for ischemic heart disease, stroke, and all-cause mortality (199).
Epigenetic regulation of certain genes by PM has been seen in cultured lung epithelial cells (200, 201); however, a genome-wide assessment of epigenetic changes induced in various tissues by PM exposure is yet to be carried out. A new large-scale study, sponsored by the National Institute of Environmental Health Sciences seeks to determine the genome-wide epigenetic effects of exposure to various environmental pollutants, including PM, on multiple tissues (202). The data collected by the TaRGET II (Toxicant Exposures and Responses by Genomic and Epigenomic Regulators of Transcription) Consortium will greatly advance the knowledge of the effects of air pollution exposure on the epigenome.
Conclusions and Future Directions
There is abundant evidence that air pollution is a major contributor to cardiovascular morbidity and mortality. Exposure to pollution is a major modifiable risk factor in the prevention and management of cardiovascular disease; however, the health effects of air pollution are not limited to the cardiovascular system. PM also appears to be an important contributor to development of metabolic diseases including obesity and type II diabetes. Emerging evidence suggests that PM exposure affects timing of puberty and reproductive health in both men and women (203–208). Furthermore, air pollution exposure may affect other systems including the central nervous system as well as the gastrointestinal tract and microbiome. (149, 209, 210). At current projections, premature mortality due to air pollution exposure is expected to double by 2050 (1). Reducing the effect of air pollution on public health will require both policy efforts to reduce production of air pollution as well individual efforts to limit exposure, particularly for those with preexisting susceptibility to cardiovascular disease.
The effects of PM exposure on catecholamine levels, insulin resistance, adiposity, and reproductive health, suggest that PM exposure is an important endocrine disruptor. Mechanistically, little is known about how PM exposure affects the endocrine system. Endocrine disrupting compounds are found in both the gaseous and particulate components of air pollution (211–214), however, further research will be required to determine if these compounds are the specific causes for the adverse health outcomes associated with PM exposure.
The totality of the evidence suggests that there is no “safe” level of PM exposure. Therefore, in addition to efforts to reduce PM production and exposure, future studies should increasingly focus on mechanistic investigations to better understand how PM causes adverse health effects. Further exploration of the signaling mediators and epigenetic regulators of the effects of air pollution on health may lead to pharmacological agents capable of mitigating the detrimental effects of air pollution on health. This effort will require cell-based and animal studies utilizing real-life exposures to PM as well as translational research in humans.
Finally, there should be increased efforts at public education on the harmful effects of air pollution exposure, particularly by physicians with at risk patients. Air pollution exposure should be seen as a major modifiable risk factor for cardiovascular disease. The United States Environmental Protection Agency provides daily ozone and PM level readings for cities in the US, Canada, and Mexico. Greater dissemination of these readings may not only help those at risk for PM-related health effects, but also increase awareness of the impact of PM exposure on health, possibly increasing demand for policy changes to reduce air pollution production.
Author Contributions
RBH and GMM contributed equally and wrote the manuscript together.
Funding
NIH K01 AR066579 and an ATS Foundation Unrestricted Grant (RBH) and R01 ES015024, U01 ES026718 and P30 ES027792 (GMM).
Conflict of Interest Statement
The authors declare that the research was conducted in the absence of any commercial or financial relationships that could be construed as a potential conflict of interest.
References
1. Lelieveld J, Evans JS, Fnais M, Giannadaki D, Pozzer A. The contribution of outdoor air pollution sources to premature mortality on a global scale. Nature (2015) 525:367–71. doi: 10.1038/nature15371
2. Newby DE, Mannucci PM, Tell GS, Baccarelli AA, Brook RD, Donaldson K, et al. Expert position paper on air pollution and cardiovascular disease. Eur Heart J. (2015) 36:83–93b. doi: 10.1093/eurheartj/ehu458
3. Brook RD, Newby DE, Rajagopalan S. The global threat of outdoor ambient air pollution to cardiovascular health: time for intervention. JAMA Cardiol. (2017) 2:353–4. doi: 10.1001/jamacardio.2017.0032
4. Zivin JG, Neidell M. Air pollution's hidden impacts. Science (2018) 359:39–40. doi: 10.1126/science.aap7711
5. Darbre PD. Overview of air pollution and endocrine disorders. Int J Gen Med. (2018) 11:191–207. doi: 10.2147/IJGM.S102230
6. Mills NL, Donaldson K, Hadoke PW, Boon NA, MacNee W, Cassee FR, et al. Adverse cardiovascular effects of air pollution. Nat Clin Pract Cardiovasc Med. (2009) 6:36–44. doi: 10.1038/ncpcardio1399
7. Cosselman KE, Navas-Acien A, Kaufman JD. Environmental factors in cardiovascular disease. Nat Rev Cardiol. (2015) 12:627–42. doi: 10.1038/nrcardio.2015.152
8. Mustafic H, Jabre P, Caussin C, Murad MH, Escolano S, Tafflet M, et al. Main air pollutants and myocardial infarction: a systematic review and meta-analysis. JAMA (2012) 307:713–21. doi: 10.1001/jama.2012.126
9. Shah AS, Langrish JP, Nair H, McAllister DA, Hunter AL, Donaldson K, et al. Global association of air pollution and heart failure: a systematic review and meta-analysis. Lancet (2013) 382:1039–48. doi: 10.1016/S0140-6736(13)60898-3
10. Atkinson RW, Kang S, Anderson HR, Mills IC, Walton HA. Epidemiological time series studies of PM2.5 and daily mortality and hospital admissions: a systematic review and meta-analysis. Thorax (2014) 69:660–5. doi: 10.1136/thoraxjnl-2013-204492
11. Pedersen M, Stayner L, Slama R, Sorensen M, Figueras F, Nieuwenhuijsen MJ, et al. Ambient air pollution and pregnancy-induced hypertensive disorders: a systematic review and meta-analysis. Hypertension (2014) 64:494–500. doi: 10.1161/HYPERTENSIONAHA.114.03545
12. Collaborators GBDRF, Forouzanfar MH, Alexander L, Anderson HR, Bachman VF, Biryukov S, et al. Global, regional, and national comparative risk assessment of 79 behavioural, environmental and occupational, and metabolic risks or clusters of risks in 188 countries, 1990-2013: a systematic analysis for the Global Burden of Disease Study 2013. Lancet (2015) 386:2287–323. doi: 10.1016/S0140-6736(15)00128-2
13. Eze IC, Hemkens LG, Bucher HC, Hoffmann B, Schindler C, Kunzli N, et al. Association between ambient air pollution and diabetes mellitus in Europe and North America: systematic review and meta-analysis. Environ Health Perspect. (2015) 123:381–9. doi: 10.1289/ehp.1307823
14. Newell K, Kartsonaki C, Lam KBH, Kurmi OP. Cardiorespiratory health effects of particulate ambient air pollution exposure in low-income and middle-income countries: a systematic review and meta-analysis. Lancet Planet Health (2017) 1:e368–80. doi: 10.1016/S2542-5196(17)30166-3
15. Brook RD, Rajagopalan S, Pope CA III, Brook JR, Bhatnagar A, Diez-Roux AV, et al. Particulate matter air pollution and cardiovascular disease: an update to the scientific statement from the American Heart Association. Circulation (2010) 121:2331–78. doi: 10.1161/CIR.0b013e3181dbece1
16. Chin MT. Basic mechanisms for adverse cardiovascular events associated with air pollution. Heart (2015) 101:253–6. doi: 10.1136/heartjnl-2014-306379
17. Brook RD, Franklin B, Cascio W, Hong Y, Howard G, Lipsett M, et al. Air pollution and cardiovascular disease: a statement for healthcare professionals from the Expert panel on population and prevention science of the american heart association. Circulation (2004) 109:2655–71. doi: 10.1161/01.CIR.0000128587.30041.C8
18. Pope CA III. Epidemiology of fine particulate air pollution and human health: biologic mechanisms and who's at risk? Environ Health Perspect (2000) 108 (Suppl. 4):713–23. doi: 10.1289/ehp.108-1637679
19. Miller MR, Shaw CA, Langrish JP. From particles to patients: oxidative stress and the cardiovascular effects of air pollution. Future Cardiol. (2012) 8:577–602. doi: 10.2217/fca.12.43
21. Stanek LW, Brown JS, Stanek J, Gift J, Costa DL. Air pollution toxicology–a brief review of the role of the science in shaping the current understanding of air pollution health risks. Toxicol Sci. (2011) 120 (Suppl. 1):S8–27. doi: 10.1093/toxsci/kfq367
22. Berger RE, Ramaswami R, Solomon CG, Drazen JM. Air pollution still kills. N Engl J Med. (2017) 376:2591–2. doi: 10.1056/NEJMe1706865
23. Samet JM, Dominici F, Curriero FC, Coursac I, Zeger SL. Fine particulate air pollution and mortality in 20 U.S. cities, 1987-1994. N Engl J Med. (2000) 343:1742–9. doi: 10.1056/NEJM200012143432401
24. Peng RD, Dominici F, Pastor-Barriuso R, Zeger SL, Samet JM. Seasonal analyses of air pollution and mortality in 100 US cities. Am J Epidemiol. (2005) 161:585–94. doi: 10.1093/aje/kwi075
25. Bell ML, Ebisu K, Peng RD, Walker J, Samet JM, Zeger SL, et al. Seasonal and regional short-term effects of fine particles on hospital admissions in 202 US counties, 1999-2005. Am J Epidemiol. (2008) 168:1301–10. doi: 10.1093/aje/kwn252
26. Katsouyanni K, Touloumi G, Spix C, Schwartz J, Balducci F, Medina S, et al. Short-term effects of ambient sulphur dioxide and particulate matter on mortality in 12 European cities: results from time series data from the APHEA project. Air Pollution and Health (1997) 314:1658–63.
27. Katsouyanni K, Touloumi G, Samoli E, Gryparis A, Le Tertre A, Monopolis Y, et al. Confounding and effect modification in the short-term effects of ambient particles on total mortality: results from 29 European cities within the APHEA2 project. Epidemiology (2001) 12:521–31. doi: 10.1097/00001648-200109000-00011
28. Nawrot TS, Perez L, Kunzli N, Munters E, Nemery B. Public health importance of triggers of myocardial infarction: a comparative risk assessment. Lancet (2011) 377:732–40. doi: 10.1016/S0140-6736(10)62296-9
29. Pope CA III, Burnett RT, Krewski D, Jerrett M, Shi Y, Calle EE, et al. Cardiovascular mortality and exposure to airborne fine particulate matter and cigarette smoke: shape of the exposure-response relationship. Circulation (2009) 120:941–8. doi: 10.1161/CIRCULATIONAHA.109.857888
30. Burnett RT, Pope CA III, Ezzati M, Olives C, Lim SS, Mehta S, et al. An integrated risk function for estimating the global burden of disease attributable to ambient fine particulate matter exposure. Environ Health Perspect. (2014) 122:397–403. doi: 10.1289/ehp.1307049
31. Apte JS, Marshall JD, Cohen AJ, Brauer M. Addressing global mortality from ambient PM2.5. Environ Sci Technol. (2015) 49:8057–66. doi: 10.1021/acs.est.5b01236
32. Shin HH, Cohen AJ, Pope CA III, Ezzati M, Lim SS, Hubbell BJ, et al. Meta-analysis methods to estimate the shape and uncertainty in the association between long-term exposure to ambient fine particulate matter and cause-specific mortality over the global concentration range. Risk Anal. (2015) 36:1813–25. doi: 10.1111/risa.12421
33. Xie W, Li G, Zhao D, Xie X, Wei Z, Wang W, et al. Relationship between fine particulate air pollution and ischaemic heart disease morbidity and mortality. Heart (2015) 101:257–63. doi: 10.1136/heartjnl-2014-306165
34. Dockery DW, Pope CA III, Xu X, Spengler JD, Ware JH, Fay ME, et al. An association between air pollution and mortality in six U.S. cities. N Engl J Med. (1993) 329:1753–9. doi: 10.1056/NEJM199312093292401
35. Pope CA III, Thun MJ, Namboodiri MM, Dockery DW, Evans JS, Speizer FE, et al. Particulate air pollution as a predictor of mortality in a prospective study of U.S. adults. Am J Respir Crit Care Med. (1995). 151:669–74. doi: 10.1164/ajrccm/151.3_Pt_1.669
36. Pope CA III, Burnett RT, Thun MJ, Calle EE, Krewski D, Ito K, et al. Lung cancer, cardiopulmonary mortality, and long-term exposure to fine particulate air pollution. JAMA (2002) 287:1132–41. doi: 10.1001/jama.287.9.1132
37. Beelen R, Raaschou-Nielsen O, Stafoggia M, Andersen ZJ, Weinmayr G, Hoffmann B, et al. Effects of long-term exposure to air pollution on natural-cause mortality: an analysis of 22 European cohorts within the multicentre ESCAPE project. Lancet (2014) 383:785–95. doi: 10.1016/S0140-6736(13)62158-3
38. Cohen AJ, Brauer M, Burnett R, Anderson HR, Frostad J, Estep K, et al. Estimates and 25-year trends of the global burden of disease attributable to ambient air pollution: an analysis of data from the Global Burden of Diseases Study 2015. Lancet (2017) 389:1907–18. doi: 10.1016/S0140-6736(17)30505-6
39. Song C, He J, Wu L, Jin T, Chen X, Li R, et al. Health burden attributable to ambient PM2.5 in China. Environ Pollut. (2017) 223:575–86. doi: 10.1016/j.envpol.2017.01.060
40. Samoli E, Peng R, Ramsay T, Pipikou M, Touloumi G, Dominici F, et al. Acute effects of ambient particulate matter on mortality in Europe and North America: results from the APHENA study. Environ Health Perspect. (2008) 116:1480–6. doi: 10.1289/ehp.11345
41. Pope CA III, Burnett RT, Thurston GD, Thun MJ, Calle EE, Krewski D, et al. Cardiovascular mortality and long-term exposure to particulate air pollution: epidemiological evidence of general pathophysiological pathways of disease. Circulation (2004) 109:71–7. doi: 10.1161/01.CIR.0000108927.80044.7F
42. Di Q, Dai L, Wang Y, Zanobetti A, Choirat C, Schwartz JD, et al. Association of short-term exposure to air pollution with mortality in older adults. JAMA (2017) 318:2446–56. doi: 10.1001/jama.2017.17923
43. Di Q, Wang Y, Zanobetti A, Wang Y, Koutrakis P, Choirat C, et al. Air pollution and mortality in the medicare population. N Engl J Med. (2017) 376:2513–22. doi: 10.1056/NEJMoa1702747
44. Parker JD, Kravets N, Vaidyanathan A. Particulate matter air pollution exposure and heart disease mortality risks by race and ethnicity in the United States: 1997 to 2009 national health interview survey with mortality follow-up through 2011. Circulation (2018) 137:1688–97. doi: 10.1161/CIRCULATIONAHA.117.029376
45. Clark LP, Millet DB, Marshall JD. Changes in transportation-related air pollution exposures by race-ethnicity and socioeconomic status: outdoor nitrogen dioxide in the United States in 2000 and 2010. Environ Health Perspect (2017) 125:097012. doi: 10.1289/EHP959
46. Hoek G, Brunekreef B, Goldbohm S, Fischer P, van den Brandt PA. Association between mortality and indicators of traffic-related air pollution in the Netherlands: a cohort study. Lancet (2002) 360:1203–9. doi: 10.1016/S0140-6736(02)11280-3
47. Jerrett M, Burnett RT, Ma R, Pope CA III, Krewski D, Newbold KB, et al. Spatial analysis of air pollution and mortality in Los Angeles. Epidemiology (2005) 16:727–36. doi: 10.1097/01.ede.0000181630.15826.7d
48. Miller KA, Siscovick DS, Sheppard L, Shepherd K, Sullivan JH, Anderson GL, et al. Long-term exposure to air pollution and incidence of cardiovascular events in women. N Engl J Med. (2007) 356:447–58. doi: 10.1056/NEJMoa054409
49. Puett RC, Schwartz J, Hart JE, Yanosky JD, Speizer FE, Suh H, et al. Chronic particulate exposure, mortality, and coronary heart disease in the nurses' health study. Am J Epidemiol. (2008) 168:1161–8. doi: 10.1093/aje/kwn232
50. Landrigan PJ, Fuller R, Acosta NJR, Adeyi O, Arnold R, Basu NN, et al. The Lancet Commission on pollution and health. Lancet (2018) 391:462–512. doi: 10.1016/S0140-6736(17)32345-0
51. Laden F, Schwartz J, Speizer FE, Dockery DW. Reduction in fine particulate air pollution and mortality: extended follow-up of the Harvard six cities study. Am J Respir Crit Care Med. (2006) 173:667–72. doi: 10.1164/rccm.200503-443OC
52. Dominici F, Peng RD, Zeger SL, White RH, Samet JM. Particulate air pollution and mortality in the United States: did the risks change from 1987 to 2000? Am J Epidemiol. (2007) 166:880–8. doi: 10.1093/aje/kwm222
53. Schwartz J, Coull B, Laden F, Ryan L. The effect of dose and timing of dose on the association between airborne particles and survival. Environ Health Perspect. (2008) 116:64–9. doi: 10.1289/ehp.9955
54. Pope CA III, Ezzati M, Dockery DW. Fine-particulate air pollution and life expectancy in the United States. N Engl J Med. (2009) 360:376–86. doi: 10.1056/NEJMsa0805646
55. Clancy L, Goodman P, Sinclair H, Dockery DW. Effect of air-pollution control on death rates in Dublin, Ireland: an intervention study. Lancet (2002) 360:1210–4. doi: 10.1016/S0140-6736(02)11281-5
56. Yorifuji T, Kashima S, Doi H. Fine-particulate air pollution from diesel emission control and mortality rates in Tokyo: a quasi-experimental study. Epidemiology (2016) 27:769–78. doi: 10.1097/EDE.0000000000000546
57. Cesaroni G, Forastiere F, Stafoggia M, Andersen ZJ, Badaloni C, Beelen R, et al. Long term exposure to ambient air pollution and incidence of acute coronary events: prospective cohort study and meta-analysis in 11 European cohorts from the ESCAPE Project. BMJ (2014) 348:f7412. doi: 10.1136/bmj.f7412
58. Madrigano J, Kloog I, Goldberg R, Coull BA, Mittleman MA, Schwartz J. Long-term exposure to PM2.5 and incidence of acute myocardial infarction. Environ Health Perspect. (2013) 121:192–6. doi: 10.1289/ehp.1205284
59. Wellenius GA, Bateson TF, Mittleman MA, Schwartz J. Particulate air pollution and the rate of hospitalization for congestive heart failure among medicare beneficiaries in Pittsburgh, Pennsylvania. Am J Epidemiol. (2005) 161:1030–6. doi: 10.1093/aje/kwi135
60. Atkinson RW, Carey IM, Kent AJ, van Staa TP, Anderson HR, Cook DG. Long-term exposure to outdoor air pollution and incidence of cardiovascular diseases. Epidemiology (2013) 24:44–53. doi: 10.1097/EDE.0b013e318276ccb8
61. Stafoggia M, Cesaroni G, Peters A, Andersen ZJ, Badaloni C, Beelen R, et al. Long-term exposure to ambient air pollution and incidence of cerebrovascular events: results from 11 European cohorts within the ESCAPE project. Environ Health Perspect. (2014) 122:919–25. doi: 10.1289/ehp.1307301
62. Wellenius GA, Burger MR, Coull BA, Schwartz J, Suh HH, Koutrakis P, et al. Ambient air pollution and the risk of acute ischemic stroke. Arch Intern Med. (2012) 172:229–34. doi: 10.1001/archinternmed.2011.732
63. Ghio AJ, Kim C, Devlin RB. Concentrated ambient air particles induce mild pulmonary inflammation in healthy human volunteers. Am J Respir Crit Care Med. (2000) 162:981–8. doi: 10.1164/ajrccm.162.3.9911115
64. van Eeden SF, Tan WC, Suwa T, Mukae H, Terashima T, Fujii T, et al. Cytokines involved in the systemic inflammatory response induced by exposure to particulate matter air pollutants [PM(10)]. Am J Respir Crit Care Med. (2001) 164:826–30. doi: 10.1164/ajrccm.164.5.2010160
65. Riediker M, Cascio WE, Griggs TR, Herbst MC, Bromberg PA, Neas L, et al. Particulate matter exposure in cars is associated with cardiovascular effects in healthy young men. Am J Respir Crit Care Med. (2004) 169:934–40. doi: 10.1164/rccm.200310-1463OC
66. Tsai DH, Amyai N, Marques-Vidal P, Wang JL, Riediker M, Mooser V, et al. Effects of particulate matter on inflammatory markers in the general adult population. Part Fibre Toxicol. (2012) 9:24. doi: 10.1186/1743-8977-9-24
67. Moller P, Loft S. Oxidative damage to DNA and lipids as biomarkers of exposure to air pollution. Environ Health Perspect. (2010) 118:1126–36. doi: 10.1289/ehp.0901725
68. Jacobs L, Emmerechts J, Hoylaerts MF, Mathieu C, Hoet PH, Nemery B, et al. Traffic air pollution and oxidized LDL. PLoS ONE (2011) 6:e16200. doi: 10.1371/journal.pone.0016200
69. Huang W, Wang G, Lu SE, Kipen H, Wang Y, Hu M, et al. Inflammatory and oxidative stress responses of healthy young adults to changes in air quality during the Beijing Olympics. Am J Respir Crit Care Med. (2012) 186:1150–9. doi: 10.1164/rccm.201205-0850OC
70. Yin F, Lawal A, Ricks J, Fox JR, Larson T, Navab M, et al. Diesel exhaust induces systemic lipid peroxidation and development of dysfunctional pro-oxidant and pro-inflammatory high-density lipoprotein. Arterioscler Thromb Vasc Biol. (2013) 33:1153–61. doi: 10.1161/ATVBAHA.112.300552
71. Kunzli N, Jerrett M, Mack WJ, Beckerman B, LaBree L, Gilliland F, et al. Ambient air pollution and atherosclerosis in Los Angeles. Environ Health Perspect. (2005) 113:201–6. doi: 10.1289/ehp.7523
72. Diez Roux AV, Auchincloss AH, Franklin TG, Raghunathan T, Barr RG, Kaufman J, et al. Long-term exposure to ambient particulate matter and prevalence of subclinical atherosclerosis in the Multi-Ethnic Study of Atherosclerosis. Am J Epidemiol. (2008) 167:667–75. doi: 10.1093/aje/kwm359
73. Bauer M, Moebus S, Mohlenkamp S, Dragano N, Nonnemacher M, Fuchsluger M, et al. Urban particulate matter air pollution is associated with subclinical atherosclerosis: results from the HNR (Heinz Nixdorf Recall) study. J Am Coll Cardiol. (2010) 56:1803–8. doi: 10.1016/j.jacc.2010.04.065
74. Adar SD, Sheppard L, Vedal S, Polak JF, Sampson PD, Diez Roux AV, et al. Fine particulate air pollution and the progression of carotid intima-medial thickness: a prospective cohort study from the multi-ethnic study of atherosclerosis and air pollution. PLoS Med. (2013) 10:e1001430. doi: 10.1371/journal.pmed.1001430
75. Hoffmann B, Moebus S, Mohlenkamp S, Stang A, Lehmann N, Dragano N, et al. Residential exposure to traffic is associated with coronary atherosclerosis. Circulation (2007) 116:489–96. doi: 10.1161/CIRCULATIONAHA.107.693622
76. Lambrechtsen J, Gerke O, Egstrup K, Sand NP, Norgaard BL, Petersen H, et al. The relation between coronary artery calcification in asymptomatic subjects and both traditional risk factors and living in the city centre: a DanRisk substudy. J Intern Med. (2012) 271:444–50. doi: 10.1111/j.1365-2796.2011.02486.x
77. Sun Q, Wang A, Jin X, Natanzon A, Duquaine D, Brook RD, et al. Long-term air pollution exposure and acceleration of atherosclerosis and vascular inflammation in an animal model. JAMA (2005) 294:3003–10. doi: 10.1001/jama.294.23.3003
78. Araujo JA, Barajas B, Kleinman M, Wang X, Bennett BJ, Gong KW, et al. Ambient particulate pollutants in the ultrafine range promote early atherosclerosis and systemic oxidative stress. Circ Res. (2008) 102:589–96. doi: 10.1161/CIRCRESAHA.107.164970
79. Campen MJ, Lund AK, Knuckles TL, Conklin DJ, Bishop B, Young D, et al. Inhaled diesel emissions alter atherosclerotic plaque composition in ApoE(-/-) mice. Toxicol Appl Pharmacol. (2010) 242:310–7. doi: 10.1016/j.taap.2009.10.021
80. Bai N, Kido T, Suzuki H, Yang G, Kavanagh TJ, Kaufman JD, et al. Changes in atherosclerotic plaques induced by inhalation of diesel exhaust. Atherosclerosis (2011) 216:299–306. doi: 10.1016/j.atherosclerosis.2011.02.019
81. Baccarelli A, Martinelli I, Pegoraro V, Melly S, Grillo P, Zanobetti A, et al. Living near major traffic roads and risk of deep vein thrombosis. Circulation (2009) 119:3118–24. doi: 10.1161/CIRCULATIONAHA.108.836163
82. Signorelli SS, Ferrante M, Gaudio A, Fiore V. Deep vein thrombosis related to environment (Review). Mol Med Rep. (2017) 15:3445–8. doi: 10.3892/mmr.2017.6395
83. Riediker M, Devlin RB, Griggs TR, Herbst MC, Bromberg PA, Williams RW, et al. Cardiovascular effects in patrol officers are associated with fine particulate matter from brake wear and engine emissions. Part Fibre Toxicol. (2004) 1:2. doi: 10.1186/1743-8977-1-2
84. Rich DQ, Kipen HM, Huang W, Wang G, Wang Y, Zhu P, et al. Association between changes in air pollution levels during the Beijing Olympics and biomarkers of inflammation and thrombosis in healthy young adults. JAMA (2012) 307:2068–78. doi: 10.1001/jama.2012.3488
85. Liu C, Cai J, Qiao L, Wang H, Xu W, Li H, et al. The acute effects of fine particulate matter constituents on blood inflammation and coagulation. Environ Sci Technol. (2017) 51:8128–37. doi: 10.1021/acs.est.7b00312
86. Mills NL, Tornqvist H, Robinson SD, Gonzalez M, Darnley K, MacNee W, et al. Diesel exhaust inhalation causes vascular dysfunction and impaired endogenous fibrinolysis. Circulation (2005) 112:3930–6. doi: 10.1161/CIRCULATIONAHA.105.588962
87. Chuang KJ, Chan CC, Su TC, Lee CT, Tang CS. The effect of urban air pollution on inflammation, oxidative stress, coagulation, and autonomic dysfunction in young adults. Am J Respir Crit Care Med. (2007) 176:370–6. doi: 10.1164/rccm.200611-1627OC
88. Mills NL, Tornqvist H, Gonzalez MC, Vink E, Robinson SD, Soderberg S, et al. Ischemic and thrombotic effects of dilute diesel-exhaust inhalation in men with coronary heart disease. N Engl J Med. (2007) 357:1075–82. doi: 10.1056/NEJMoa066314
89. Peters A, Doring A, Wichmann HE, Koenig W. Increased plasma viscosity during an air pollution episode: a link to mortality? Lancet (1997) 349:1582–7. doi: 10.1016/S0140-6736(97)01211-7
90. Lucking AJ, Lundback M, Mills NL, Faratian D, Barath SL, Pourazar J, et al. Diesel exhaust inhalation increases thrombus formation in man. Eur Heart J. (2008) 29:3043–51. doi: 10.1093/eurheartj/ehn464
91. Jacobs L, Emmerechts J, Mathieu C, Hoylaerts MF, Fierens F, Hoet PH, et al. Air pollution related prothrombotic changes in persons with diabetes. Environ Health Perspect. (2010) 118:191–6. doi: 10.1289/ehp.0900942
92. Lucking AJ, Lundback M, Barath SL, Mills NL, Sidhu MK, Langrish JP, et al. Particle traps prevent adverse vascular and prothrombotic effects of diesel engine exhaust inhalation in men. Circulation (2011) 123:1721–8. doi: 10.1161/CIRCULATIONAHA.110.987263
93. Brook RD, Brook JR, Urch B, Vincent R, Rajagopalan S, Silverman F. Inhalation of fine particulate air pollution and ozone causes acute arterial vasoconstriction in healthy adults. Circulation (2002) 105:1534–6. doi: 10.1161/01.CIR.0000013838.94747.64
94. Tornqvist H, Mills NL, Gonzalez M, Miller MR, Robinson SD, Megson IL, et al. Persistent endothelial dysfunction in humans after diesel exhaust inhalation. Am J Respir Crit Care Med. (2007) 176:395–400. doi: 10.1164/rccm.200606-872OC
95. Peretz A, Sullivan JH, Leotta DF, Trenga CA, Sands FN, Allen J, et al. Diesel exhaust inhalation elicits acute vasoconstriction in vivo. Environ Health Perspect. (2008) 116:937–42. doi: 10.1289/ehp.11027
96. Barath S, Mills NL, Lundback M, Tornqvist H, Lucking AJ, Langrish JP, et al. Impaired vascular function after exposure to diesel exhaust generated at urban transient running conditions. Part Fibre Toxicol. (2010) 7:19. doi: 10.1186/1743-8977-7-19
97. Adar SD, Klein R, Klein BE, Szpiro AA, Cotch MF, Wong TY, et al. Air Pollution and the microvasculature: a cross-sectional assessment of in vivo retinal images in the population-based multi-ethnic study of atherosclerosis (MESA). PLoS Med. (2010) 7:e1000372. doi: 10.1371/journal.pmed.1000372
98. Krishnan RM, Adar SD, Szpiro AA, Jorgensen NW, Van Hee VC, Barr RG, et al. Vascular responses to long- and short-term exposure to fine particulate matter: MESA Air (Multi-Ethnic Study of Atherosclerosis and Air Pollution). J Am Coll Cardiol. (2012) 60:2158–66. doi: 10.1016/j.jacc.2012.08.973
99. Coogan PF, White LF, Jerrett M, Brook RD, Su JG, Seto E, et al. Air pollution and incidence of hypertension and diabetes mellitus in black women living in Los Angeles. Circulation (2012) 125:767–72. doi: 10.1161/CIRCULATIONAHA.111.052753
100. Chen H, Burnett RT, Kwong JC, Villeneuve PJ, Goldberg MS, Brook RD, et al. Spatial association between ambient fine particulate matter and incident hypertension. Circulation (2014) 129:562–9. doi: 10.1161/CIRCULATIONAHA.113.003532
101. Cosselman KE, Krishnan RM, Oron AP, Jansen K, Peretz A, Sullivan JH, et al. Blood pressure response to controlled diesel exhaust exposure in human subjects. Hypertension (2012) 59:943–8. doi: 10.1161/HYPERTENSIONAHA.111.186593
102. Bellavia A, Urch B, Speck M, Brook RD, Scott JA, Albetti B, et al. DNA hypomethylation, ambient particulate matter, and increased blood pressure: findings from controlled human exposure experiments. J Am Heart Assoc. (2013) 2:e000212. doi: 10.1161/JAHA.113.000212
103. Vinikoor-Imler LC, Gray SC, Edwards SE, Miranda ML. The effects of exposure to particulate matter and neighbourhood deprivation on gestational hypertension. Paediatr Perinat Epidemiol. (2012) 26:91–100. doi: 10.1111/j.1365-3016.2011.01245.x
104. Dadvand P, Ostro B, Amato F, Figueras F, Minguillon MC, Martinez D, et al. Particulate air pollution and preeclampsia: a source-based analysis. Occup Environ Med. (2014) 71:570–7. doi: 10.1136/oemed-2013-101693
105. Van Hee VC, Adar SD, Szpiro AA, Barr RG, Bluemke DA, Diez Roux AV, et al. Exposure to traffic and left ventricular mass and function: the Multi-Ethnic Study of Atherosclerosis. Am J Respir Crit Care Med. (2009) 179:827–34. doi: 10.1164/rccm.200808-1344OC
106. Leary PJ, Kaufman JD, Barr RG, Bluemke DA, Curl CL, Hough CL, et al. Traffic-related air pollution and the right ventricle. The multi-ethnic study of atherosclerosis. Am J Respir Crit Care Med. (2014) 189:1093–100. doi: 10.1164/rccm.201312-2298OC
107. Ying Z, Yue P, Xu X, Zhong M, Sun Q, Mikolaj M, et al. Air pollution and cardiac remodeling: a role for RhoA/Rho-kinase. Am J Physiol Heart Circ Physiol. (2009) 296:H1540–1550. doi: 10.1152/ajpheart.01270.2008
108. Wold LE, Ying Z, Hutchinson KR, Velten M, Gorr MW, Velten C, et al. Cardiovascular remodeling in response to long-term exposure to fine particulate matter air pollution. Circ Heart Fail (2012) 5:452–61. doi: 10.1161/CIRCHEARTFAILURE.112.966580
109. Dockery DW, Luttmann-Gibson H, Rich DQ, Link MS, Mittleman MA, Gold DR, et al. Association of air pollution with increased incidence of ventricular tachyarrhythmias recorded by implanted cardioverter defibrillators. Environ Health Perspect. (2005) 113:670–4. doi: 10.1289/ehp.7767
110. Rich DQ, Mittleman MA, Link MS, Schwartz J, Luttmann-Gibson H, Catalano PJ, et al. Increased risk of paroxysmal atrial fibrillation episodes associated with acute increases in ambient air pollution. Environ Health Perspect. (2006) 114:120–3. doi: 10.1289/ehp.8371
111. Link MS, Luttmann-Gibson H, Schwartz J, Mittleman MA, Wessler B, Gold DR, et al. Acute exposure to air pollution triggers atrial fibrillation. J Am Coll Cardiol. (2013) 62:816–25. doi: 10.1016/j.jacc.2013.05.043
112. Folino F, Buja G, Zanotto G, Marras E, Allocca G, Vaccari D, et al. Association between air pollution and ventricular arrhythmias in high-risk patients (ARIA study): a multicentre longitudinal study. Lancet Planet Health (2017) 1:e58–64. doi: 10.1016/S2542-5196(17)30020-7
113. Pope CA III, Verrier RL, Lovett EG, Larson AC, Raizenne ME, Kanner RE, et al. Heart rate variability associated with particulate air pollution. Am Heart J. (1999) 138:890–9.
114. Gold DR, Litonjua A, Schwartz J, Lovett E, Larson A, Nearing B, et al. Ambient pollution and heart rate variability. Circulation (2000) 101:1267–73. doi: 10.1161/01.cir.101.11.1267
115. Pekkanen J, Peters A, Hoek G, Tiittanen P, Brunekreef B, de Hartog J, et al. Particulate air pollution and risk of ST-segment depression during repeated submaximal exercise tests among subjects with coronary heart disease: the Exposure and Risk Assessment for Fine and Ultrafine Particles in Ambient Air (ULTRA) study. Circulation (2002) 106:933–8. doi: 10.1161/01.CIR.0000027561.41736.3C
116. Henneberger A, Zareba W, Ibald-Mulli A, Ruckerl R, Cyrys J, Couderc JP, et al. Repolarization changes induced by air pollution in ischemic heart disease patients. Environ Health Perspect. (2005) 113:440–6. doi: 10.1289/ehp.7579
117. Zanobetti A, Stone PH, Speizer FE, Schwartz JD, Coull BA, Suh HH, et al. T-wave alternans, air pollution and traffic in high-risk subjects. Am J Cardiol. (2009) 104:665–70. doi: 10.1016/j.amjcard.2009.04.046
118. Zanobetti A, Gold DR, Stone PH, Suh HH, Schwartz J, Coull BA, et al. Reduction in heart rate variability with traffic and air pollution in patients with coronary artery disease. Environ Health Perspect. (2010) 118:324–30. doi: 10.1289/ehp.0901003
119. He F, Shaffer ML, Rodriguez-Colon S, Yanosky JD, Bixler E, Cascio WE, et al. Acute effects of fine particulate air pollution on cardiac arrhythmia: the APACR study. Environ Health Perspect. (2011) 119:927–32. doi: 10.1289/ehp.1002640
120. Cakmak S, Dales R, Kauri LM, Mahmud M, Van Ryswyk K, Vanos J, et al. Metal composition of fine particulate air pollution and acute changes in cardiorespiratory physiology. Environ Pollut. (2014) 189:208–14. doi: 10.1016/j.envpol.2014.03.004
121. Wang T, Lang GD, Moreno-Vinasco L, Huang Y, Goonewardena SN, Peng YJ, et al. Particulate matter induces cardiac arrhythmias via dysregulation of carotid body sensitivity and cardiac sodium channels. Am J Respir Cell Mol Biol. (2012) 46:524–31. doi: 10.1165/rcmb.2011-0213OC
122. Watkinson WP, Campen MJ, Costa DL. Cardiac arrhythmia induction after exposure to residual oil fly ash particles in a rodent model of pulmonary hypertension. Toxicol Sci. (1998) 41:209–16. doi: 10.1006/toxs.1997.2406
123. Brook RD, Xu X, Bard RL, Dvonch JT, Morishita M, Kaciroti N, et al. Reduced metabolic insulin sensitivity following sub-acute exposures to low levels of ambient fine particulate matter air pollution. Sci Total Environ. (2013) 448:66–71. doi: 10.1016/j.scitotenv.2012.07.034
124. Thiering E, Cyrys J, Kratzsch J, Meisinger C, Hoffmann B, Berdel D, et al. Long-term exposure to traffic-related air pollution and insulin resistance in children: results from the GINIplus and LISAplus birth cohorts. Diabetologia (2013) 56:1696–704. doi: 10.1007/s00125-013-2925-x
125. Wolf K, Popp A, Schneider A, Breitner S, Hampel R, Rathmann W, et al. Association between long-term exposure to air pollution and biomarkers related to insulin resistance, subclinical inflammation, and adipokines. Diabetes (2016) 65:3314–26. doi: 10.2337/db15-1567
126. Pearson JF, Bachireddy C, Shyamprasad S, Goldfine AB, Brownstein JS. Association between fine particulate matter and diabetes prevalence in the U.S. Diabetes Care (2010) 33:2196–201. doi: 10.2337/dc10-0698
127. Weinmayr G, Hennig F, Fuks K, Nonnemacher M, Jakobs H, Mohlenkamp S, et al. Long-term exposure to fine particulate matter and incidence of type 2 diabetes mellitus in a cohort study: effects of total and traffic-specific air pollution. Environ Health (2015) 14:53. doi: 10.1186/s12940-015-0031-x
128. O'Neill MS, Veves A, Zanobetti A, Sarnat JA, Gold DR, Economides PA, et al. Diabetes enhances vulnerability to particulate air pollution-associated impairment in vascular reactivity and endothelial function. Circulation (2005) 111:2913–20. doi: 10.1161/CIRCULATIONAHA.104.517110
129. Sun Q, Yue P, Deiuliis JA, Lumeng CN, Kampfrath T, Mikolaj MB, et al. Ambient air pollution exaggerates adipose inflammation and insulin resistance in a mouse model of diet-induced obesity. Circulation (2009) 119:538–46. doi: 10.1161/CIRCULATIONAHA.108.799015
130. Liu C, Bai Y, Xu X, Sun L, Wang A, Wang TY, et al. Exaggerated effects of particulate matter air pollution in genetic type II diabetes mellitus. Part Fibre Toxicol. (2014) 11:27. doi: 10.1186/1743-8977-11-27
131. Liu C, Xu X, Bai Y, Wang TY, Rao X, Wang A, et al. Air pollution-mediated susceptibility to inflammation and insulin resistance: influence of CCR2 pathways in mice. Environ Health Perspect. (2014) 122:17–26. doi: 10.1289/ehp.1306841
132. Xu X, Yavar Z, Verdin M, Ying Z, Mihai G, Kampfrath T, et al. Effect of early particulate air pollution exposure on obesity in mice: role of p47phox. Arterioscler Thromb Vasc Biol. (2010) 30:2518–27. doi: 10.1161/ATVBAHA.110.215350
133. Franklin BA, Brook R, Arden Pope C III Air pollution and cardiovascular disease. Curr Probl Cardiol. (2015) 40:207–38. doi: 10.1016/j.cpcardiol.2015.01.003
134. Semmler M, Seitz J, Erbe F, Mayer P, Heyder J, Oberdorster G, et al. Long-term clearance kinetics of inhaled ultrafine insoluble iridium particles from the rat lung, including transient translocation into secondary organs. Inhal Toxicol. (2004) 16:453–9. doi: 10.1080/08958370490439650
135. Semmler-Behnke M, Takenaka S, Fertsch S, Wenk A, Seitz J, Mayer P, et al. Efficient elimination of inhaled nanoparticles from the alveolar region: evidence for interstitial uptake and subsequent reentrainment onto airways epithelium. Environ Health Perspect. (2007) 115:728–33. doi: 10.1289/ehp.9685
136. Rao X, Zhong J, Brook RD, Rajagopalan S. Effect of particulate matter air pollution on cardiovascular oxidative stress pathways. Antioxid Redox Signal (2018) 28:797–818. doi: 10.1089/ars.2017.7394
137. Mutlu GM, Snyder C, Bellmeyer A, Wang H, Hawkins K, Soberanes S, et al. Airborne particulate matter inhibits alveolar fluid reabsorption in mice via oxidant generation. Am J Respir Cell Mol Biol. (2006) 34:670–6. doi: 10.1165/rcmb.2005-0329OC
138. Soberanes S, Panduri V, Mutlu GM, Ghio A, Bundinger GR, Kamp DW. p53 mediates particulate matter-induced alveolar epithelial cell mitochondria-regulated apoptosis. Am J Respir Crit Care Med. (2006) 174:1229–38. doi: 10.1164/rccm.200602-203OC
139. Manzo ND, LaGier AJ, Slade R, Ledbetter AD, Richards JH, Dye JA. Nitric oxide and superoxide mediate diesel particle effects in cytokine-treated mice and murine lung epithelial cells–implications for susceptibility to traffic-related air pollution. Part Fibre Toxicol. (2012) 9:43. doi: 10.1186/1743-8977-9-43
140. Hong Z, Guo Z, Zhang R, Xu J, Dong W, Zhuang G, et al. Airborne fine particulate matter induces oxidative stress and inflammation in human nasal epithelial cells. Tohoku J Exp Med. (2016) 239:117–25. doi: 10.1620/tjem.239.117
141. Wang J, Huang J, Wang L, Chen C, Yang D, Jin M, et al. Urban particulate matter triggers lung inflammation via the ROS-MAPK-NF-kappaB signaling pathway. J Thorac Dis. (2017) 9:4398–412. doi: 10.21037/jtd.2017.09.135
142. Li N, Sioutas C, Cho A, Schmitz D, Misra C, Sempf J, et al. Ultrafine particulate pollutants induce oxidative stress and mitochondrial damage. Environ Health Perspect. (2003) 111:455–60. doi: 10.1289/ehp.6000
143. Ohyama K, Kubo H, Harada M, Sasahara Y, Nozaki A, Takei N, et al. Comparison of 3 Tesla whole heart coronary MRA (WHCA) with 1.5 Tesla. Nihon Hoshasen Gijutsu Gakkai Zasshi (2008) 64:1540–6. doi: 10.6009/jjrt.64.1540
144. Zhao Q, Chen H, Yang T, Rui W, Liu F, Zhang F, et al. Direct effects of airborne PM2.5 exposure on macrophage polarizations. Biochim Biophys Acta (2016) 1860:2835–43. doi: 10.1016/j.bbagen.2016.03.033
145. Li R, Ning Z, Cui J, Khalsa B, Ai L, Takabe W, et al. Ultrafine particles from diesel engines induce vascular oxidative stress via JNK activation. Free Radic Biol Med. (2009) 46:775–82. doi: 10.1016/j.freeradbiomed.2008.11.025
146. Montiel-Davalos A, Ibarra-Sanchez Mde J, Ventura-Gallegos JL, Alfaro-Moreno E, Lopez-Marure R. Oxidative stress and apoptosis are induced in human endothelial cells exposed to urban particulate matter. Toxicol In Vitro (2010) 24:135–41. doi: 10.1016/j.tiv.2009.08.004
147. Cao J, Qin G, Shi R, Bai F, Yang G, Zhang M, et al. Overproduction of reactive oxygen species and activation of MAPKs are involved in apoptosis induced by PM2.5 in rat cardiac H9c2 cells. J Appl Toxicol. (2016) 36:609–17. doi: 10.1002/jat.3249
148. Yang X, Feng L, Zhang Y, Hu H, Shi Y, Liang S, et al. Cytotoxicity induced by fine particulate matter (PM2.5) via mitochondria-mediated apoptosis pathway in human cardiomyocytes. Ecotoxicol Environ Saf. (2018) 161:198–207. doi: 10.1016/j.ecoenv.2018.05.092
149. Mutlu EA, Engen PA, Soberanes S, Urich D, Forsyth CB, Nigdelioglu R, et al. Particulate matter air pollution causes oxidant-mediated increase in gut permeability in mice. Part Fibre Toxicol. (2011) 8:19. doi: 10.1186/1743-8977-8-19
150. Jin SP, Li Z, Choi EK, Lee S, Kim YK, Seo EY, et al. Urban particulate matter in air pollution penetrates into the barrier-disrupted skin and produces ROS-dependent cutaneous inflammatory response in vivo. J Dermatol Sci. (2018). doi: 10.1016/j.jdermsci.2018.04.015
151. Park EJ, Chae JB, Lyu J, Yoon C, Kim S, Yeom C, et al. Ambient fine particulate matters induce cell death and inflammatory response by influencing mitochondria function in human corneal epithelial cells. Environ Res. (2017) 159:595–605. doi: 10.1016/j.envres.2017.08.044
152. Imrich A, Ning Y, Lawrence J, Coull B, Gitin E, Knutson M, et al. Alveolar macrophage cytokine response to air pollution particles: oxidant mechanisms. Toxicol Appl Pharmacol. (2007) 218:256–64. doi: 10.1016/j.taap.2006.11.033
153. Chiarella SE, Soberanes S, Urich D, Morales-Nebreda L, Nigdelioglu R, Green D, et al. beta(2)-Adrenergic agonists augment air pollution-induced IL-6 release and thrombosis. J Clin Invest. (2014) 124:2935–46. doi: 10.1172/JCI75157
154. Tripathi P, Deng F, Scruggs AM, Chen Y, Huang SK. Variation in doses and duration of particulate matter exposure in bronchial epithelial cells results in upregulation of different genes associated with airway disorders. Toxicol In Vitro (2018) 51:95–105. doi: 10.1016/j.tiv.2018.05.004
155. Xu C, Shi Q, Zhang L, Zhao H. High molecular weight hyaluronan attenuates fine particulate matter-induced acute lung injury through inhibition of ROS-ASK1-p38/JNK-mediated epithelial apoptosis. Environ Toxicol Pharmacol. (2018) 59:190–8. doi: 10.1016/j.etap.2018.03.020
156. Hamanaka RB, Chandel NS. Mitochondrial reactive oxygen species regulate cellular signaling and dictate biological outcomes. Trends Biochem Sci. (2010) 35:505–13. doi: 10.1016/j.tibs.2010.04.002
157. Li R, Kou X, Geng H, Xie J, Yang Z, Zhang Y, et al. Effect of ambient PM(2.5) on lung mitochondrial damage and fusion/fission gene expression in rats. Chem Res Toxicol. (2015) 28:408–18. doi: 10.1021/tx5003723
158. Jin X, Xue B, Zhou Q, Su R, Li Z. Mitochondrial damage mediated by ROS incurs bronchial epithelial cell apoptosis upon ambient PM2.5 exposure. J Toxicol Sci. (2018) 43:101–11. doi: 10.2131/jts.43.101
159. Soberanes S, Urich D, Baker CM, Burgess Z, Chiarella SE, Bell EL, et al. Mitochondrial complex III-generated oxidants activate ASK1 and JNK to induce alveolar epithelial cell death following exposure to particulate matter air pollution. J Biol Chem. (2009) 284:2176–86. doi: 10.1074/jbc.M808844200
160. Huang YC, Soukup J, Harder S, Becker S. Mitochondrial oxidant production by a pollutant dust and NO-mediated apoptosis in human alveolar macrophage. Am J Physiol Cell Physiol. (2003) 284:C24–32. doi: 10.1152/ajpcell.00139.2002
161. Soberanes S, Misharin AV, Jairaman A, Morales-Nebreda L, McQuattie-Pimentel AC, Cho T, et al. Metformin targets mitochondrial electron transport to reduce air-pollution-induced thrombosis. Cell Metab. (2018). doi: 10.1016/j.cmet.2018.09.019. [Epub ahead of print].
162. Morales-Nebreda L, Misharin AV, Perlman H, Budinger GR. The heterogeneity of lung macrophages in the susceptibility to disease. Eur Respir Rev. (2015) 24:505–9. doi: 10.1183/16000617.0031-2015
163. Soukup JM, Becker S. Human alveolar macrophage responses to air pollution particulates are associated with insoluble components of coarse material, including particulate endotoxin. Toxicol Appl Pharmacol. (2001) 171:20–6. doi: 10.1006/taap.2000.9096
164. Fujii T, Hayashi S, Hogg JC, Vincent R, Van Eeden SF. Particulate matter induces cytokine expression in human bronchial epithelial cells. Am J Respir Cell Mol Biol. (2001) 25:265–71. doi: 10.1165/ajrcmb.25.3.4445
165. Fujii T, Hayashi S, Hogg JC, Mukae H, Suwa T, Goto Y, et al. Interaction of alveolar macrophages and airway epithelial cells following exposure to particulate matter produces mediators that stimulate the bone marrow. Am J Respir Cell Mol Biol. (2002) 27:34–41. doi: 10.1165/ajrcmb.27.1.4787
166. Mutlu GM, Green D, Bellmeyer A, Baker CM, Burgess Z, Rajamannan N, et al. Ambient particulate matter accelerates coagulation via an IL-6-dependent pathway. J Clin Invest. (2007) 117:2952–61. doi: 10.1172/JCI30639
167. Marchini T, Wolf D, Michel NA, Mauler M, Dufner B, Hoppe N, et al. Acute exposure to air pollution particulate matter aggravates experimental myocardial infarction in mice by potentiating cytokine secretion from lung macrophages. Basic Res Cardiol. (2016) 111:44. doi: 10.1007/s00395-016-0562-5
168. Mukae H, Vincent R, Quinlan K, English D, Hards J, Hogg JC, et al. The effect of repeated exposure to particulate air pollution (PM10) on the bone marrow. Am J Respir Crit Care Med. (2001) 163:201–9. doi: 10.1164/ajrccm.163.1.2002039
169. Tan WC, Qiu D, Liam BL, Ng TP, Lee SH, van Eeden SF, et al. The human bone marrow response to acute air pollution caused by forest fires. Am J Respir Crit Care Med. (2000) 161:1213–7. doi: 10.1164/ajrccm.161.4.9904084
170. Goto Y, Ishii H, Hogg JC, Shih CH, Yatera K, Vincent R, et al. Particulate matter air pollution stimulates monocyte release from the bone marrow. Am J Respir Crit Care Med. (2004) 170:891–7. doi: 10.1164/rccm.200402-235OC
171. Mihara M, Hashizume M, Yoshida H, Suzuki M, Shiina M. IL-6/IL-6 receptor system and its role in physiological and pathological conditions. Clin Sci. (2012) 122:143–59. doi: 10.1042/CS20110340
172. Hunter CA, Jones SA. IL-6 as a keystone cytokine in health and disease. Nat Immunol. (2015) 16:448–57. doi: 10.1038/ni.3153
173. Heinrich PC, Castell JV, Andus T. Interleukin-6 and the acute phase response. Biochem J. (1990) 265:621–36.
174. Kerr R, Stirling D, Ludlam CA. Interleukin 6 and haemostasis. Br J Haematol. (2001) 115:3–12. doi: 10.1046/j.1365-2141.2001.03061.x
175. Budinger GR, McKell JL, Urich D, Foiles N, Weiss I, Chiarella SE, et al. Particulate matter-induced lung inflammation increases systemic levels of PAI-1 and activates coagulation through distinct mechanisms. PLoS ONE (2011) 6:e18525. doi: 10.1371/journal.pone.0018525
176. Wassmann S, Stumpf M, Strehlow K, Schmid A, Schieffer B, Bohm M, et al. Interleukin-6 induces oxidative stress and endothelial dysfunction by overexpression of the angiotensin II type 1 receptor. Circ Res. (2004) 94:534–41. doi: 10.1161/01.RES.0000115557.25127.8D
177. Kido T, Tamagawa E, Bai N, Suda K, Yang HH, Li Y, et al. Particulate matter induces translocation of IL-6 from the lung to the systemic circulation. Am J Respir Cell Mol Biol. (2011) 44:197–204. doi: 10.1165/rcmb.2009-0427OC
178. Carswell EA, Old LJ, Kassel RL, Green S, Fiore N, Williamson B. An endotoxin-induced serum factor that causes necrosis of tumors. Proc Natl Acad Sci USA (1975) 72:3666–70.
179. Sedger LM, McDermott MF. TNF and TNF-receptors: from mediators of cell death and inflammation to therapeutic giants - past, present and future. Cytokine Growth Factor Rev. (2014) 25:453–72. doi: 10.1016/j.cytogfr.2014.07.016
180. Kalliolias GD, Ivashkiv LB. TNF biology, pathogenic mechanisms and emerging therapeutic strategies. Nat Rev Rheumatol. (2016) 12:49–62. doi: 10.1038/nrrheum.2015.169
181. Marchini T, D'Annunzio V, Paz ML, Caceres L, Garces M, Perez V, et al. Selective TNF-alpha targeting with infliximab attenuates impaired oxygen metabolism and contractile function induced by an acute exposure to air particulate matter. Am J Physiol Heart Circ Physiol. (2015) 309:H1621–1628. doi: 10.1152/ajpheart.00359.2015
182. Kumar S, Joos G, Boon L, Tournoy K, Provoost S, Maes T. Role of tumor necrosis factor-alpha and its receptors in diesel exhaust particle-induced pulmonary inflammation. Sci Rep. (2017) 7:11508. doi: 10.1038/s41598-017-11991-7
183. Saber AT, Bornholdt J, Dybdahl M, Sharma AK, Loft S, Vogel U, et al. Tumor necrosis factor is not required for particle-induced genotoxicity and pulmonary inflammation. Arch Toxicol. (2005) 79:177–82. doi: 10.1007/s00204-004-0613-9
184. Saber AT, Jacobsen NR, Bornholdt J, Kjaer SL, Dybdahl M, Risom L, et al. Cytokine expression in mice exposed to diesel exhaust particles by inhalation. Role of tumor necrosis factor. Part Fibre Toxicol. (2006) 3:4. doi: 10.1186/1743-8977-3-4
185. Kohase M, Henriksen-DeStefano D, May LT, Vilcek J, Sehgal PB. Induction of beta 2-interferon by tumor necrosis factor: a homeostatic mechanism in the control of cell proliferation. Cell (1986) 45:659–66.
186. Shalaby MR, Waage A, Aarden L, Espevik T. Endotoxin, tumor necrosis factor-alpha and interleukin 1 induce interleukin 6 production in vivo. Clin Immunol Immunopathol. (1989) 53:488–98.
187. Barbosa CM, Terra-Filho M, de Albuquerque AL, Di Giorgi D, Grupi C, Negrao CE, et al. Burnt sugarcane harvesting - cardiovascular effects on a group of healthy workers, Brazil. PLoS ONE (2012) 7:e46142. doi: 10.1371/journal.pone.0046142
188. Li H, Cai J, Chen R, Zhao Z, Ying Z, Wang L, et al. Particulate matter exposure and stress hormone levels: a randomized, double-blind, crossover trial of air purification. Circulation (2017) 136:618–27. doi: 10.1161/CIRCULATIONAHA.116.026796
189. Mendez R, Zheng Z, Fan Z, Rajagopalan S, Sun Q, Zhang K. Exposure to fine airborne particulate matter induces macrophage infiltration, unfolded protein response, and lipid deposition in white adipose tissue. Am J Transl Res. (2013) 5:224–34.
190. Xu X, Liu C, Xu Z, Tzan K, Zhong M, Wang A, et al. Long-term exposure to ambient fine particulate pollution induces insulin resistance and mitochondrial alteration in adipose tissue. Toxicol Sci. (2011) 124:88–98. doi: 10.1093/toxsci/kfr211
191. Xu Z, Xu X, Zhong M, Hotchkiss IP, Lewandowski RP, Wagner JG, et al. Ambient particulate air pollution induces oxidative stress and alterations of mitochondria and gene expression in brown and white adipose tissues. Part Fibre Toxicol. (2011) 8:20. doi: 10.1186/1743-8977-8-20
192. Shoelson SE, Lee J, Goldfine AB. Inflammation and insulin resistance. J Clin Invest. (2006) 116:1793–801. doi: 10.1172/JCI29069
193. Li W, Dorans KS, Wilker EH, Rice MB, Schwartz J, Coull BA, et al. Residential proximity to major roadways, fine particulate matter, and adiposity: the framingham heart study. Obesity (2016) 24:2593–9. doi: 10.1002/oby.21630
194. Kramer U, Herder C, Sugiri D, Strassburger K, Schikowski T, Ranft U, et al. Traffic-related air pollution and incident type 2 diabetes: results from the SALIA cohort study. Environ Health Perspect. (2010) 118:1273–9. doi: 10.1289/ehp.0901689
195. Weldy CS, Liu Y, Liggitt HD, Chin MT. In utero exposure to diesel exhaust air pollution promotes adverse intrauterine conditions, resulting in weight gain, altered blood pressure, and increased susceptibility to heart failure in adult mice. PLoS ONE (2014) 9:e88582. doi: 10.1371/journal.pone.0088582
196. Yauk C, Polyzos A, Rowan-Carroll A, Somers CM, Godschalk RW, Van Schooten FJ, et al. Germ-line mutations, DNA damage, and global hypermethylation in mice exposed to particulate air pollution in an urban/industrial location. Proc Natl Acad Sci USA (2008) 105:605–10. doi: 10.1073/pnas.0705896105
197. Baccarelli A, Wright RO, Bollati V, Tarantini L, Litonjua AA, Suh HH, et al. Rapid DNA methylation changes after exposure to traffic particles. Am J Respir Crit Care Med. (2009) 179:572–8. doi: 10.1164/rccm.200807-1097OC
198. Madrigano J, Baccarelli A, Mittleman MA, Wright RO, Sparrow D, Vokonas PS, et al. Prolonged exposure to particulate pollution, genes associated with glutathione pathways, and DNA methylation in a cohort of older men. Environ Health Perspect. (2011) 119:977–82. doi: 10.1289/ehp.1002773
199. Baccarelli A, Wright R, Bollati V, Litonjua A, Zanobetti A, Tarantini L, et al. Ischemic heart disease and stroke in relation to blood DNA methylation. Epidemiology (2010) 21:819–28. doi: 10.1097/EDE.0b013e3181f20457
200. Gilmour PS, Rahman I, Donaldson K, MacNee W. Histone acetylation regulates epithelial IL-8 release mediated by oxidative stress from environmental particles. Am J Physiol Lung Cell Mol Physiol. (2003) 284:L533–540. doi: 10.1152/ajplung.00277.2002
201. Soberanes S, Gonzalez A, Urich D, Chiarella SE, Radigan KA, Osornio-Vargas A, et al. Particulate matter air pollution induces hypermethylation of the p16 promoter Via a mitochondrial ROS-JNK-DNMT1 pathway. Sci Rep. (2012) 2:275. doi: 10.1038/srep00275
202. Wang T, Pehrsson EC, Purushotham D, Li D, Zhuo X, Zhang B, et al. The NIEHS TaRGET II Consortium and environmental epigenomics. Nat Biotechnol. (2018) 36:225–7. doi: 10.1038/nbt.4099
203. Dejmek J, Selevan SG, Benes I, Solansky I, Sram RJ. Fetal growth and maternal exposure to particulate matter during pregnancy. Environ Health Perspect. (1999) 107:475–80.
204. Selevan SG, Borkovec L, Slott VL, Zudova Z, Rubes J, Evenson DP, et al. Semen quality and reproductive health of young Czech men exposed to seasonal air pollution. Environ Health Perspect. (2000) 108:887–94. doi: 10.1289/ehp.00108887
205. Pedersen M, Giorgis-Allemand L, Bernard C, Aguilera I, Andersen AM, Ballester F, et al. Ambient air pollution and low birthweight: a European cohort study (ESCAPE). Lancet Respir Med. (2013) 1:695–704. doi: 10.1016/S2213-2600(13)70192-9
206. Slama R, Bottagisi S, Solansky I, Lepeule J, Giorgis-Allemand L, Sram R. Short-term impact of atmospheric pollution on fecundability. Epidemiology (2013) 24:871–9. doi: 10.1097/EDE.0b013e3182a702c5
207. Huang JV, Leung GM, Schooling CM. The association of air pollution with pubertal development: evidence from hong kong's “children of 1997” birth cohort. Am J Epidemiol. (2017) 185:914–23. doi: 10.1093/aje/kww200
208. Janssen BG, Saenen ND, Roels HA, Madhloum N, Gyselaers W, Lefebvre W, et al. Fetal thyroid function, birth weight, and in utero exposure to fine particle air pollution: a birth cohort study. Environ Health Perspect. (2017) 125:699–705. doi: 10.1289/EHP508
209. Block ML, Calderon-Garciduenas L. Air pollution: mechanisms of neuroinflammation and CNS disease. Trends Neurosci. (2009) 32:506–16. doi: 10.1016/j.tins.2009.05.009
210. Mutlu EA, Comba IY, Cho T, Engen PA, Yazici C, Soberanes S, et al. Inhalational exposure to particulate matter air pollution alters the composition of the gut microbiome. Environ Pollut. (2018) 240:817–30. doi: 10.1016/j.envpol.2018.04.130
211. Takeda K, Tsukue N, Yoshida S. Endocrine-disrupting activity of chemicals in diesel exhaust and diesel exhaust particles. Environ Sci. (2004) 11:33–45.
212. Oh SM, Ryu BT, Chung KH. Identification of estrogenic and antiestrogenic activities of respirable diesel exhaust particles by bioassay-directed fractionation. Arch Pharm Res. (2008) 31:75–82. doi: 10.1007/s12272-008-1123-8
213. Novak J, Jalova V, Giesy JP, Hilscherova K. Pollutants in particulate and gaseous fractions of ambient air interfere with multiple signaling pathways in vitro. Environ Int (2009) 35:43–9. doi: 10.1016/j.envint.2008.06.006
Keywords: particulate matter, cardiovascular, lung, macrophage, inflammation, interleukin-6, thrombosis, coagulation
Citation: Hamanaka RB and Mutlu GM (2018) Particulate Matter Air Pollution: Effects on the Cardiovascular System. Front. Endocrinol. 9:680. doi: 10.3389/fendo.2018.00680
Received: 04 July 2018; Accepted: 30 October 2018;
Published: 16 November 2018.
Edited by:
Robert Sargis, University of Illinois at Chicago, United StatesReviewed by:
Abby Fleisch, Maine Medical Center Research Institute, United StatesLicio A. Velloso, Universidade Estadual de Campinas, Brazil
Copyright © 2018 Hamanaka and Mutlu. This is an open-access article distributed under the terms of the Creative Commons Attribution License (CC BY). The use, distribution or reproduction in other forums is permitted, provided the original author(s) and the copyright owner(s) are credited and that the original publication in this journal is cited, in accordance with accepted academic practice. No use, distribution or reproduction is permitted which does not comply with these terms.
*Correspondence: Gökhan M. Mutlu, gmutlu@medicine.bsd.uchicago.edu