- 1Instituto de Investigaciones Biotecnológicas-Instituto Tecnológico de Chascomús (CONICET-UNSAM), Buenos Aires, Argentina
- 2Institut Pasteur, Platforme de Cristallographie and CNRS UMR 3528, Paris, France
- 3Department of Biotechnology, University of Verona, Verona, Italy
- 4Department of Biological Sciences, George Washington University, Washington, DC, United States
- 5Laboratori d'Ictiologia Genètica, Departament de Biologia, Universitat de Girona, Girona, Spain
Kisspeptin receptors are G-Protein-Coupled Receptors that regulate GnRH synthesis and release in vertebrates. Here, we report the gene structure of two kisspeptin receptors (kissr2 and kissr3) in pejerrey fish. Genomic analysis exposed a gene structure with 5 exons and 4 introns for kissr2 and 6 exons and 5 introns for kissr3. Two alternative variants for both genes, named kissr2_v1 and _v2, and kissr3_v1 and v2, were revealed by gene expression analyses of several tissues. For both receptors, these variants were originated by alternative splicing retaining intron 3 and intron 4 for kissr2_v2 and kissr3_v2, respectively. In the case of kissr2, the intron retention introduced two stop codons leading to a putatively truncated protein whereas for kissr3, the intron retention produced a reading shift leading to a stop codon in exon 5. Modeling and structural analysis of Kissr2 and Kissr3 spliced variants revealed that truncation of the proteins may lead to non-functional proteins, as the structural elements missing are critical for receptor function. To understand the functional significance of splicing variants, the expression pattern for kissr2 was characterized on fish subjected to different diets. Fasting induced an up-regulation of kissr2_v1 in the hypothalamus, a brain region implicated in control of reproduction and food intake, with no expression of kissr2_v2. On the other hand, fasting did not elicit differential expression in testes and habenula. These results suggest that alternative splicing may play a role in regulating Kissr2 function in pejerrey.
Introduction
G-protein-coupled receptors (GPCRs) play key roles in many physiological processes and have been associated with multiple human diseases (1). Since changes in normal GPCRs signaling affect many pathophysiological mechanisms, these receptors have been targets for several drug therapies (1–3). The superfamily of GPCRs is characterized by having seven-transmembrane (7TM) α-helices connected by three extracellular loops (ECLs) and three intracellular loops (ICLs), an extracellular amino-terminal segment, and an intracellular carboxy-terminal tail (4). According to their amino acid sequence, GPCRs are classified into five major classes (families): (i) A or Rhodopsin-like (the largest group), (ii) B or Secretin receptor, (iii) C or Metabotropic Glutamate receptor, (iv) adhesion, and (v) frizzled/taste (5).
In 2001, a member of the Rhodopsin family, the kisspeptin receptor KISS1R (previously named GPR54) was shown to be activated by polypeptides kisspeptin-54,-14,-13, and-10 (6–8). A few years later, kisspeptin and its receptor were regarded as essential regulators of the reproductive axis, since hypogonadotropic hypogonadism in both humans and mice was shown to be associated with mutations of KISS1R (9, 10). Moreover, kisspeptin and its receptor were linked to other functions such as insulin secretion (11), vasoconstriction (12), tumor biology and the metastatic process (13), antioxidant function in oxidative stress (14), anticoagulation (15), and brain sex differentiation (16).
During the last decade, many studies on vertebrate reproduction readily identified kisspeptin receptors in a large number of species facilitated by the highly conserved structure of their 7TMs domains. Only one gene, known now as Kiss1r, has been reported in placental mammals (17). In contrast, two paralogous kissrs (namely kissr2 and kissr3) are frequently detected in teleost fishes, likely originating from the teleost-specific whole-genome duplication in the common ancestor of teleosts (17–19). Of particular interest is the case of the Senegalese Sole (Solea senegalensis), for which a single kissr2 but no kissr3 genes were reported (20). However, two kissr2 transcripts were identified in this species, a short one named kissr2_v1 corresponding to the normally-spliced messenger, and a long kissr2_v2 (putatively non-functional) transcript characterized by retaining the entire intron 3 (20). Subsequently, the presence of alternatively spliced variants of kissr2 was documented in other teleost species such as the Southern Bluefin Tuna (Thunnus maccoyii) and the Yellowtail Kingfish (Seriola lalandi) (21). Alternative transcripts or spliced variants for kissr3 (previously known as kiss1rb) also were described for zebrafish (Danio rerio) (22) and the European eel (Anguilla anguilla) (23). Although some studies have suggested preservation of functionality of truncated GPCR transcripts (22, 24), evidence to support this claim remains elusive.
A functional relationship between food intake and reproduction has been well established among fishes (25), however little is known about the putative role, if any, that the kisspeptin system may have to modulate this interaction. The first report supporting such a role was a study on Senegalese sole that showed up-regulation of hypothalamic kiss2 and kissr2 expression during starvation, in concert with an increase of transcript levels of gonadotropins in the pituitary (26). Similarly, food restriction was shown to enhance hypothalamic kiss2 and kissr2 gene expression and to increase mRNA levels of follicle-stimulating hormone and luteinizing hormone β subunits (fshb and lhb) in the pituitary of the European sea bass (Dicentrarchus labrax) during spermatogenesis (27).
In the present study, we report the predicted structure of two kissr genes, kissr2 and kissr3 in pejerrey fish (Odontesthes bonariensis). We also identify new alternative spliced variants for each receptor and provide preliminary evidence suggesting loss of function of variants due to intron retention. We also test the expression pattern of kissr2_v1 and kissr2_v2 in pejerrey hypothalamus after fasting, because a similar condition was reported to increase not only hypothalamic kiss2 but also kissr2 in S. senegalensis (26).
Our findings suggest a novel kissr2 gene regulatory mechanism in the hypothalamus involving expression of alternatively spliced variants with intron retention that produce potentially non-functional proteins.
Materials and Methods
Fish and Tissue Sample Collection for Gene Characterization
Adult pejerrey (Odontesthes bonariensis) were maintained in outdoor tanks of the IIB-INTECH aquatic facilities under natural photoperiod and water temperature of 17 ± 2°C. They were fed daily with fish commercial pellets (Shulet®, Argentina). For each of the experimental purposes, fish were anesthetized with an over-dose of benzocaine and then decapitated. The different tissues and organs were quickly dissected under clean conditions, immediately frozen in liquid nitrogen and then stored at −80°C until used. The fish were handled in accordance with the UFAW Handbook on the Care and Management of Laboratory Animals (http://www.ufaw.org.uk) and IIB-INTECH internal institutional regulations. These animal protocols were approved by a professional board.
Gene Structure of Kissr2 and Kissr3 in Pejerrey
The BLAST 2.2.29 algorithm was used to retrieve the genomic sequences of kissr2 and kissr3 from the pejerrey genome database (28) using pejerrey partial sequences of both genes (29). To assess the genomic gene structure, the coding sequences of both genes (29) were amplified by the primer combinations kissr2-Ex1-F/kissr2-Ex5-R and kissr3-Ex1-F/kissr3-Ex6-R (Table 1) using complementary DNA (cDNA) as template. Genomic DNA was extracted according to Aljanabi and Martinez (30). Briefly, a portion of muscle (~50 mg of tissue) was obtained and homogenized in 400 μl of saline buffer (NaCl 0.4 M, Tris-HCl 10 mM pH 8, EDTA 2 mM pH 8), then 40 μl of SDS 10% and 8 μl of proteinase K (10 mg/ml) were added and the mixture incubated at 65°C for 1 h. After incubation, 300 μl of NaCl 6 M were added and the solution was centrifuged at 10,000 g for 15 min. Finally, the supernatant was obtained and precipitated with 95% ethanol, washed with 70% ethanol and dissolved in 50 μl of ultrapure water (Invitrogen™, USA).
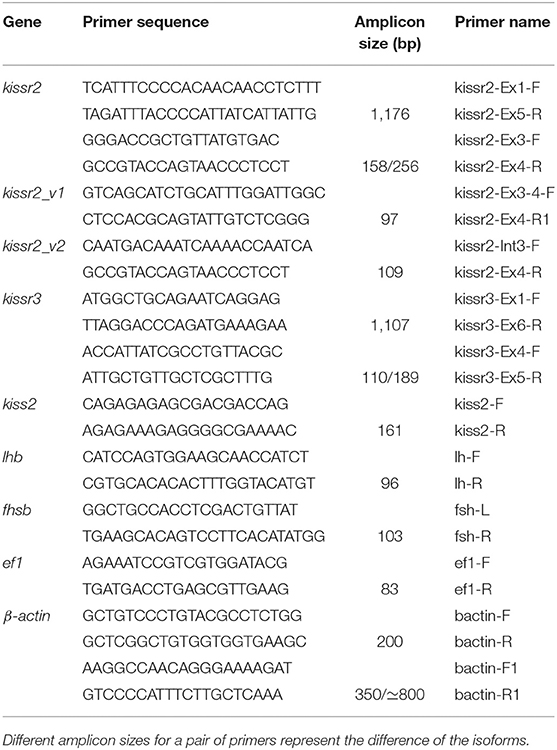
Table 1. Gene-specific primers used to build the gene structure organization, detection of alternative splicing and qPCR studies.
RNA Isolation and cDNA Synthesis
Total RNA was isolated with TRIZOL® Reagent (Invitrogen™, USA) from different organs and tissues. Quality of RNA was assessed in all samples using 1% agarose-formaldehyde gels and their quantity measured with a Biotek H1 synergy analyzer (Biotek®, USA). All RNAs were treated with DNaseI (Invitrogen™, USA) to remove any possible genomic DNA contamination. Subsequently, cDNA was synthesized using ~500 ng of RNA with Superscript II Reverse Transcriptase (Invitrogen™, USA), RNaseOUT recombinant ribonuclease inhibitor (Invitrogen™, USA), and oligo dT universal adaptor primer in 20 μl reaction volume, following the manufacturer's instructions.
Detection of Kissr2 and Kissr3 Alternatively Spliced Variants in Pejerrey
Complementary DNA from hypothalamus, testis, and habenula were used as templates to search for alternatively spliced variants of kissr2 and kissr3 in adult animals by RT-PCR. In the case of kissr2, primers located between exons 3 and 4, including intron III (kissr2-Ex3-F and kissr2-Ex4-R) were specifically designed to amplify both splicing variants. For kissr3, primers between exons 4 and 5, including intron IV were designed with the same purpose (kissr3-Ex4-F and kissr3-Ex5-R; Table 1). PCR amplifications of these transcripts were performed using an initial heat denaturation step at 94°C for 5 min, followed by 40 cycles of 30 s at 94°C, 30 s at 55°C, and 1 min at 72°C, and finished with a final extension step at 72°C for 5 min. PCR products were visualized by 1% agarose gel electrophoresis.
The tissue expression pattern of kissr2 and kissr3 splicing variants were analyzed by RT–PCR in different pejerrey tissues and organs. For the brain we separated three different regions after dissection: rostral (including the olfactory bulbs, telencephalon, and preoptic area), medial (including the optic tectum, thalamus, pineal gland, and hypothalamus), and caudal (cerebellar body, vagal lobe, and the medulla oblongata). The following tissues and organs also were analyzed for specimens of both sexes: pituitary gland, gonads, olfactory epithelium, retina, lateral line, liver, gills, muscle, heart, foregut, midgut, hindgut, kidney, and spleen. Total RNA isolation and cDNA synthesis were carried out following the above described protocols. The resulting cDNA was used to amplify kissr2 and kissr3 by RT-PCR using GoTaq® DNA Polymerase (Promega, USA). The PCR cycling conditions were: 5 min at 94°C; 40 cycles of 30 s at 94°C, 30 s at 60°C, 30 s at 72°C and a final extension of 5 min at 72°C. RNA quality and genomic DNA contamination in cDNA were checked by amplification of the β-actin gene with a primer combination encompassing an intron (bactin-F1 and bactin-R1) (Table 1, Figure 3C). No template controls (NTC) were included to ensure that no contamination occurred. All PCR products were run on 1% agarose gels.
Kissr Homology Model and Docking
The Kissr2_v1 and Kissr3_v1 structural models and their interaction with ligand peptides were inferred using GOMoDO (http://molsim.sci.univr.it/cgi-bin/cona/begin.php). The peptides were docked in the predicted binding cavities by using the Haddock program, accessible through the GOMoDo server (31). Figures depicting 2D and 3D receptor models were produced with Topdraw (32) and Chimera programs (http://www.cgl.ucsf.edu/chimera/), respectively.
Fasting Effect on Kissr2 Alternative Splicing
We choose the hypothalamus as a target organ to evaluate the relationship between expression levels of kissr2_v1 and kissr2_v2, because differential expression of kissr2 has been reported during fasting in other fish species (26, 27). In addition, the mRNA expression patterns of kissr2_v1 and kissr2_v2 (kissr2-Ex3-4-F1/kissr2-Ex4-R1 and kissr2_Int3_F/kissr2_Ex4_R primer pairs) (Table 1; Supplementary Figure 1) were analyzed in the testes and habenula, together with lhb and fshb in the pituitary gland. Adult pejerrey males (119.86 ± 9.21 g) were transferred to 300 l indoor tanks with similar conditions to outdoor tanks. Fish were distributed into different tanks (n = 8 per tank) and acclimated for 1 week before starting experimental manipulations. After acclimation, one group was starved and the other (control) was feed ad libitum three times a day for 15 days. These experimental procedures were performed in duplicate. Fish were sacrificed as described above, the hypothalamus and habenula were dissected from the brain, and the pituitary gland and testes also were sampled as controls. Total RNA was extracted and cDNA obtained according to described protocols. The expression patterns were analyzed by relative quantitative PCR (qPCR). All primers used for qPCR designed to amplify each kissr2 splicing variant are listed in Table 1 and their quality values in Supplementary Table 1. The qPCR amplification reaction mixture contained 2 μl of diluted cDNA (1:20), 300 nM of each primer, and 5 μl of FastStart Universal SYBR Green Master (Rox) (Roche Diagnostics, Germany) in a final volume of 10 μl. The thermal cycling conditions were 95°C for 10 min, 40 cycles at 95°C for 15 s, and 60°C for 1 min. At the end of the PCR cycles, the qualities of qPCR products were analyzed using a dissociation curve step to confirm that only a single PCR product was amplified. NTC reactions for every primer pair also were included on each reaction plate to ensure no external DNA contamination. The amplification efficiency (E) of each primer set/target gene was assessed as E = 10(−1/slope) as determined by linear regression of a series of dilutions of the input RNA. The qPCR reactions were performed with a Step-one Real-time PCR System (Applied Biosystems, USA). Fold change (the relative quantification, RQ) was calculated from the ΔΔCt (33). Determinations were carried out in technical triplicates for all the genes and normalized against the reference genes (ef1 and β-actin) (ef1-F/ef1-R and bactin-F/bactin-R primer pairs) (Table 1). The RQ values for each sample were averaged and the standard error of the mean (SEM) was calculated. Controls without cDNA template (Supplementary Figure 1B), and a melt curve analysis (Supplementary Figure 1C) were used to determine the specificity of the amplification.
Statistical Analyses
The SPSS v20 program was used to perform all the statistical analyses and results were expressed as mean ± SEM. Statistical significance of pairwise comparisons of body weight and mRNA levels was determined using Student t-test (*p < 0.01, *p < 0.05).
Results
Genomic Structure of Kissr2 and Kissr3 Genes in Pejerrey
We used the cDNA sequences of the coding region of kissr2 in pejerrey, previously reported by Tovar Bohórquez et al. (29), to scan the pejerrey genome (28) and locate the genomic sequence of the duplicated kissr genes, kissr2 and kissr3. The gene structures were constructed using the nucleotide and predicted peptide sequences of kissr2 and kissr3 compared with known kissr sequences available in GenBank. Localization of the intron-exon boundaries sites was based on alignment of the cDNA and genomic DNA for each receptor. The structure of the kissr2 gene exhibits five exons (with 252, 129, 136, 239, and 384 bp, respectively) and four introns (3,346, 1,948, 98, and 1,749 bp, respectively) (Figure 1). The structure of kissr3 is composed of six exons (with 219, 129, 132, 237, 153, and 237 bp, respectively) and five introns (with 355, 1,116, 238, 79, and 1,080 bp, respectively) (Figure 2).
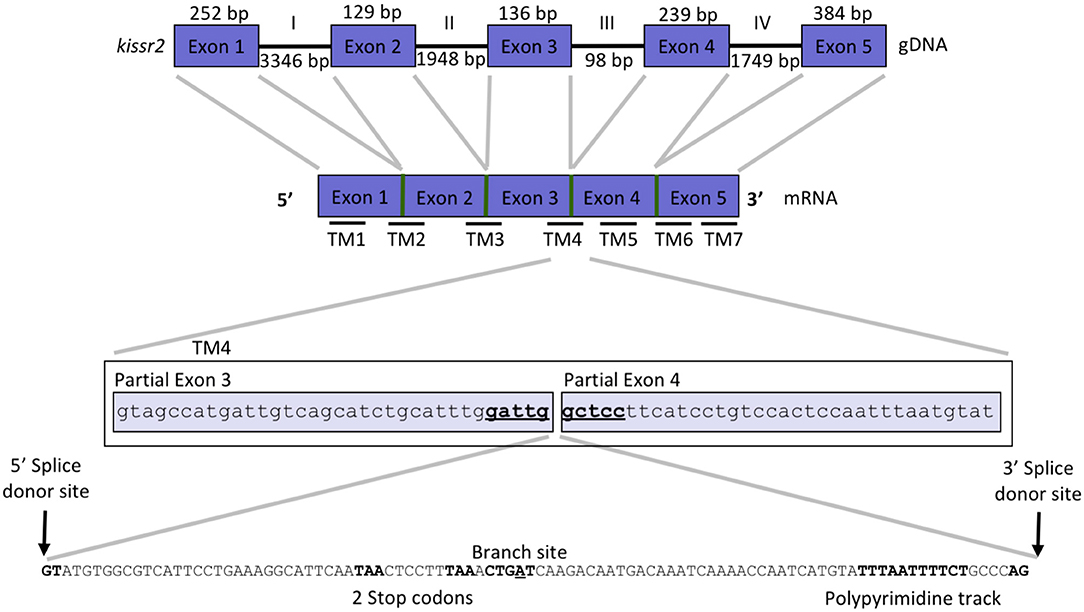
Figure 1. Gene structure of kissr2 in pejerrey. Exons are displayed as blue boxes and introns as thick lines. Thinner horizontal lines at the bottom of the cDNA scheme, represent the site of the transmembrane domains (TMs). A detail of pejerrey intron 3 of kissr2 sequence with features consistent with the presence of a mechanism for alternative splicing is shown.
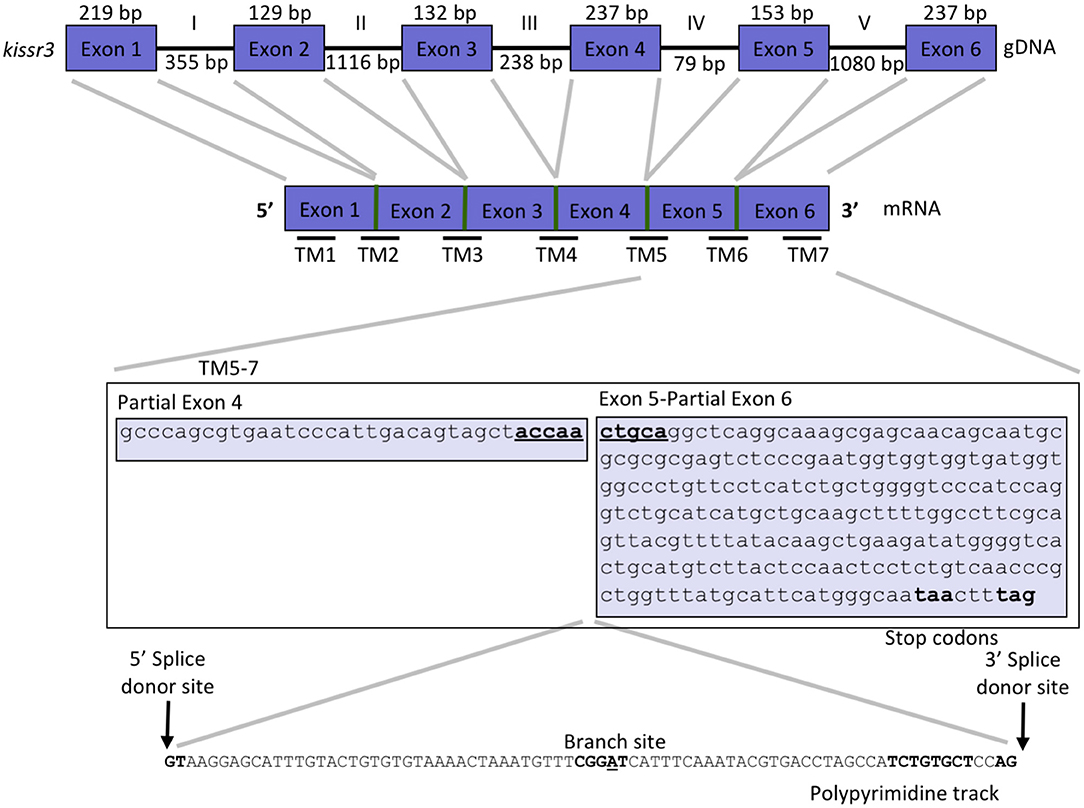
Figure 2. Gene structure of kissr3 in pejerrey. Exons are displayed as blue boxes and introns as thick lines. Thinner horizontal lines at the bottom of the cDNA scheme, represent the site of the transmembrane domains (TMs). A detail of pejerrey intron 4 of kissr3 sequence with features consistent with the presence of a mechanism for alternative splicing is shown.
Transcriptomic sequence analysis revealed that the longer transcript kissr2_v2 retained the entire intron 3 and displayed the presence of several consensus features of alternative splicing mechanisms in the introns: a 5′ donor splice site, potential branch points, polypyrimidine tracks and 3′ acceptor splice sites (Figure 1). Translation of the kissr2_v2 isoform DNA to its putative amino acid sequence revealed the presence of two premature stop codons. In the case of the longer kissr3_v2 isoform, retention of intron 4 caused a shift in the reading frame that included two premature stop codons in exon 5 (Figure 2).
Alternatively Spliced Variants of Kissr2 and Kissr3
Two sets of specific primers that amplified regions encompassing intron 3 in kissr2 and intron 4 in kissr3 were used for sequence analyses (Table 1). These primers generated two different amplicons for each gene (see Supplementary Figure 1A), as follows: kissr2_v1 (158 bp), kissr2_v2 (256 bp), kissr3_v1 (110 bp), and kissr3_v2 (189 bp).
In males, the rostral, medium and caudal brain, testis, gills, muscle, and foregut showed expression of both kissr2 transcripts (_v1 and _v2). The retina, lateral line, heart, and midgut only showed kissr2_v2 expression while pituitary, liver, hindgut, kidney, and spleen did not show expression of either spliced variant (Figure 3A). In females, kissr2_v1 was clearly visualized in rostral, medial and caudal brain, pituitary, and gonads; and dimly in retina and lateral line, whereas kissr2_v2 transcript was detected in the olfactory epithelium, retina, liver, gill, muscle, heart, foregut, midgut, hindgut, kidney, and spleen (Figure 3A). In the case of kissr3, in males, kissr3_v1 was detected in the rostral brain, medial brain, caudal brain, pituitary, testis, retina, gill, heart, hindgut, and kidney, meanwhile kissr3_v2 was only observed in the liver and hindgut (Figure 3B). In females, kissr3_v1 was observed in the rostral brain, medial brain, caudal brain, ovary, olfactory epithelium, gill, heart, and spleen, while kissr3_v2 was not detected (Figure 3B).
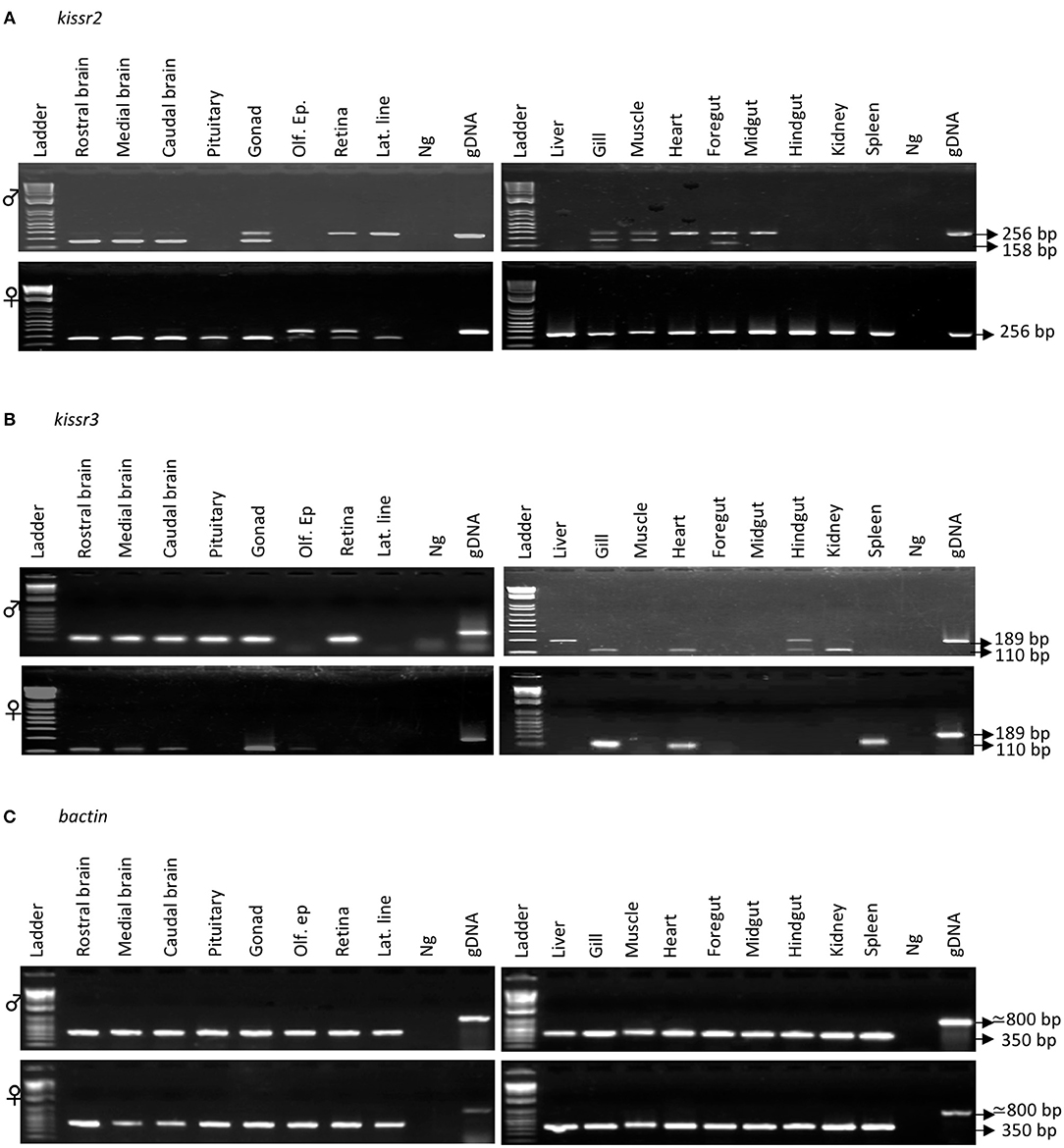
Figure 3. Tissue expression of the kissr2 (A) and kissr3 (B) was analyzed by RT-PCR in mature pejerrey. Total RNA was prepared from rostral brain, medial brain, caudal brain, pituitary, testis (in males), ovary (in females), olfactory epithelium (Olf. Ep), retina, lateral line, liver, gills, muscle, heart, foregut, midgut, hindgut, kidney, and spleen from one male and one female. Messenger RNAs of the two isoforms were detected by RT-PCR (kissr2-Ex3-F/kissr2-Ex4-R and kissr3-Ex4-F/kissr3-Ex5-R primers). Lane 1, 100 bp ladder; Lane 2-8/9, tissues cDNA; Lane 9/10, Negative control (Ng); Lane 10/11, genomic DNA (gDNA). (C) β-actin gene was amplified to check the absence of gDNA and as a control of the cDNA integrity.
Homology Modeling and Molecular Docking of Kisspeptins and Their Receptors
Homology 3D models of pejerrey Kissr2 and Kissr3-structures were built using the on-line platform GOMoDo (Figure 4, Supplementary Figure 2 for Kissr2 and Supplementary Figure 3 for Kissr3). Their respective peptides (Supplementary Figure 4) were then docked in the predicted binding cavities by using the Haddock program accessible also through the GOMoDo server (Supplementary Table 2). From the models it can be observed that: (i) in Kissr2_v1 the putative ligand binding cavity is formed by residues of TM3 (Gln125, Gln126, Val129, Gln130), ECL3 (Tyr197, Cys198, Glu200), TM5 (Gln215, Tyr220), TM6 (Leu276, Trp281, Ile284, Gln285), and TM7 (Asn311, Tyr315) (Figure 4A); and (ii) in pejerrey the Kissr3_v1 putative binding cavity is formed by residues of TM3 (Gln114, Gln115, Ala118, Gln119), ECL3 (Gln183, Thr184, Cys186), TM5 (Ser203, Tyr208), TM6 (Leu264, Trp269, Ile272, Gln273), and TM7 (His296, Tyr300) (Figure 4B). It is important to note that in both receptors the residues that are putatively crucial for ligand and G-protein binding (according to the prediction of the method used) belong to helices TM5-7, just like several other GPCRs analyzed before (34). This evidence suggests that loss of these helices in variants kissr2_v2 and kissr3_v2 could compromise receptor structure, function, or dimerization (Figures 4C,D).
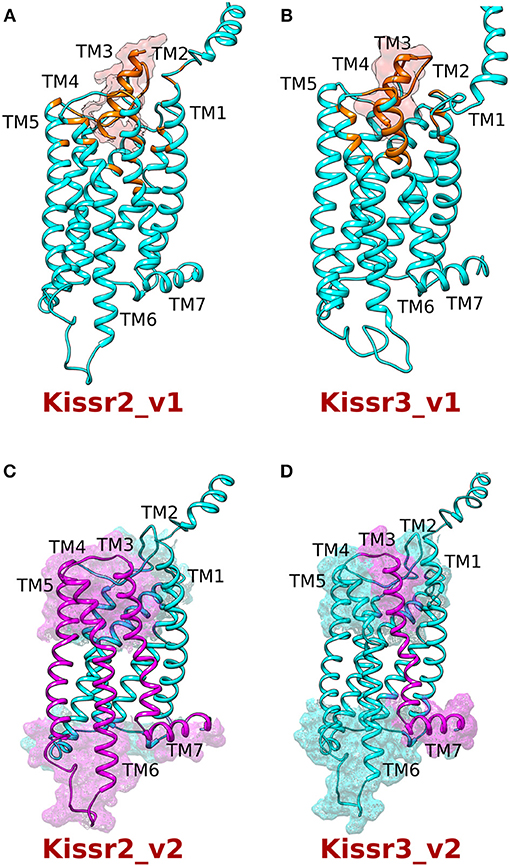
Figure 4. Homology modeling of the kisspeptin receptors (Kissr2 and Kissr3) with their respective peptides kiss1 and kiss2. (A) Kissr2_v1 receptor (cyan) and (B) Kissr3_v1 receptor (cyan) with their respective peptides (orange). The residues located within 5 Å from the peptides are shown in orange. (C,D) The truncated region of both the receptors is shown in violet. While receptor Kissr2_v2, lacks TM5-TM7 helixes; Kissr3_v2 loses TM7 and portion of the extracellular loop 3.
Effect of Fasting on Kissr2_v1 and Kissr2_v2 Expression Levels
Food deprivation resulted in a significant reduction of ~10 % in body weight (p < 0.001) in the starved adult pejerrey males (Figure 5A). Fasting increased mRNA levels of kiss2 (p = 0.014) in the hypothalamus (Figure 5B) and increased levels of lhb and fshb in the pituitary (p = 0.032 and p = 0.048) (Figure 5C).
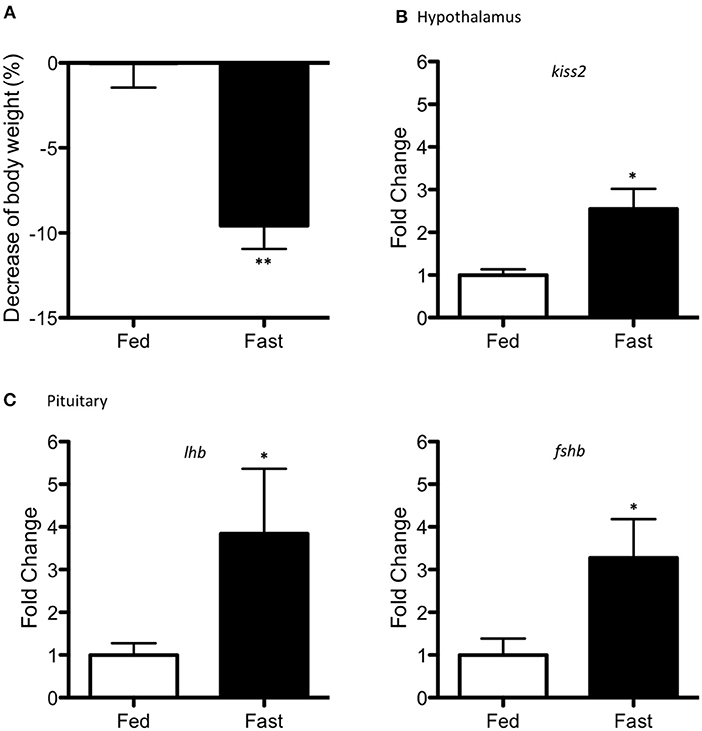
Figure 5. Effects of fasting (15 days period) in adult pejerrey. (A) Decrease of percentage in body weight during feeding and fasting conditions. (B) Expression levels of kiss2 in the hypothalamus. (C) Expression levels of lhb and fshb in the pituitary. Results are represented as fold change after data normalization against β-actin levels Asterisks indicate statistically significant differences after the Student's t-test. **p < 0.01; *p < 0.05. Data shown are expressed as mean ± S.E.M. (n = 7–8).
Changes in mRNA levels also were measured for kissr2_v1 and kissr2_v2 (Figure 6). In the hypothalamus, only kissr2_v1 was detected in both control and fasted fish, with higher expression in fasted fish than controls (p = 0.001, Figure 6A). However, kissr2_v2 was not detected in either case (Figure 6A). In testes and habenula both isoforms were observed in both feeding treatments, with no significant difference in expression level between isoforms (Figures 6B,C).
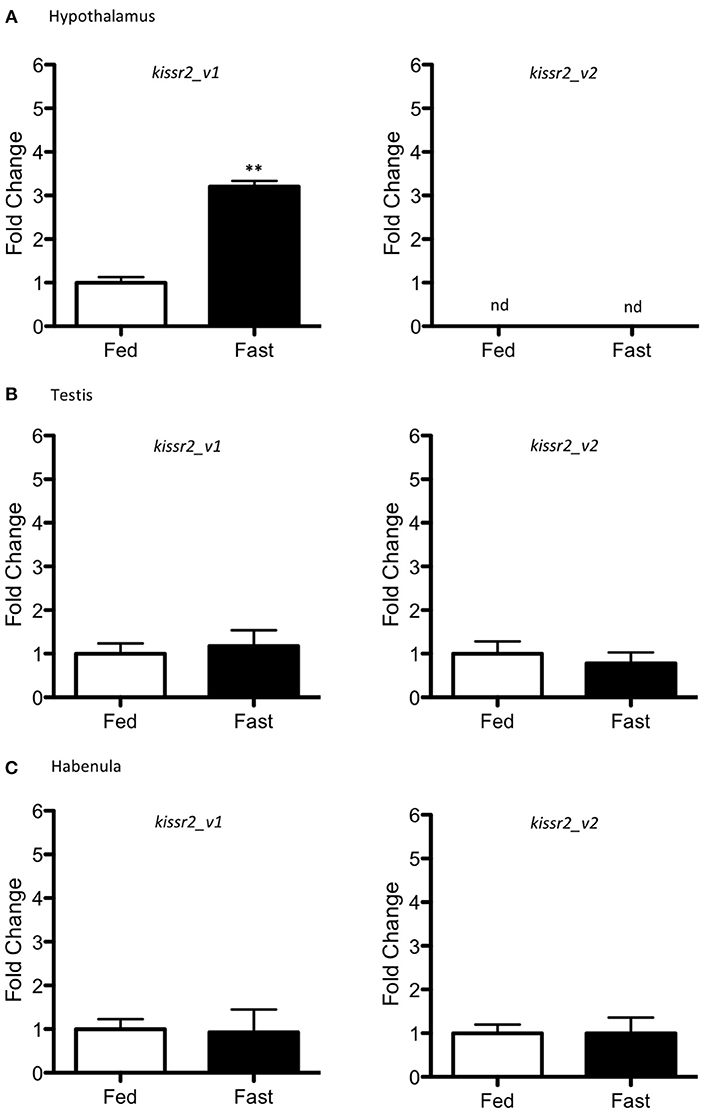
Figure 6. Gene expression in different tissues of male pejerrey. Expression levels of kissr2_v1 and kissr2_v2 isoforms in the hypothalamus (A), testis (B), and habenula (C). Results are represented as fold change after data normalization against β-actin levels. Asterisks indicate statistically significant differences after the Student's t-test. **p < 0.01. n.d. non-detected. Data shown are expressed as mean ± S.E.M. (n = 7).
Discussion
We have previously characterized two full-length cDNAs encoding kissr2 and kissr3 in pejerrey (29). In this study, we present additional analysis of their genomic structures and expression patterns in different tissues and under different experimental conditions. In agreement with a previous study of Senegalese Sole kissr2 (20) and an in silico analysis in Nile Tilapia (35), the inferred structure of this gene in pejerrey consists of five exons and four introns. The pejerrey kissr2 gene structure also is similar to its mammalian ortholog KISSR1 (35). On the other hand, pejerrey kissr3 presented six exons and five introns, similar to the situation observed in medaka (Oryzias latipes) and sea bass (36).
In pejerrey, kissr2 and kissr3 encode proteins of 379 and 369 aminoacids, respectively (29). These two paralogous proteins shared high similarity in their transmembrane domains, but low similarity in their ICL and ECL regions, similar to the situation observed in goldfish (37). Additionally, pejerrey Kissr2 and Kissr3 contain some typical features of the rhodopsin family, such as the NPXXY and DRY motifs (38).
Both receptors, Kissr2 and Kissr3 belong to the largest gene subfamily (Rhodopsin-like) within the GPCRs superfamily. Although exponential increase in our knowledge of crystallographic GPCRs structures during the last decade (39) helped to characterize many genes, lack of structural data still hampers a deep characterization of their function (40, 41). Indeed, solving protein structure remains a problematic issue due the limitations in protein production and purification, protein stability, and homogeneity (42) and, in some cases, low expression levels (43). Thus, computational tools are key to generate reliable protein structure modeling that may help in the characterization/elucidation of protein structure/function (31).
It is also becoming increasingly evident that a high percentage of GPCRs undergo alternative splicing events (2); however, only in a few fish species alternative Kissr isoforms have been described (20–22). The present study documents alternative Kissr2 isoforms in pejerrey (Kissr2_v1 and Kissr2_v2). Furthermore, we show that the kissr2_v2 isoform originates by retention of the entire intron 3, similar to the situation in the Senegalese sole (20). It is interesting to note that, in the case of foregut, the size of the smaller band looks shorter than the expected Kissr2_v1. At this moment, we cannot discard the possibility of the existence of a third isoform as it has been reported in other fish species (21, 22). Furthermore, in pejerrey, a species with two kissr paralogous genes, an alternative isoform of kissr3 mRNA was also observed in male liver and hindgut, and the alternative isoform originates by retention of the entire intron 4. Unlike other species where alternative spliced variants originate by deletion of exons, such as yellowtail kingfish (21) and zebrafish (22), this mechanism is not present in pejerrey. However, more studies on the mechanism of splicing of the kissr3 gene must be performed to get a better understanding of the role of splicing of this gene in different pejerrey tissues. The splicing mechanism, originating truncated isoforms in Kissr2 and/or Kissr3 in several teleost species appears to be a conserved feature and may represent a regulatory mechanism for controlling gene expression (44). We summarize the evidence reported so far for splicing events for these genes in teleost fish in Figure 7 and Supplementary Figure 5.
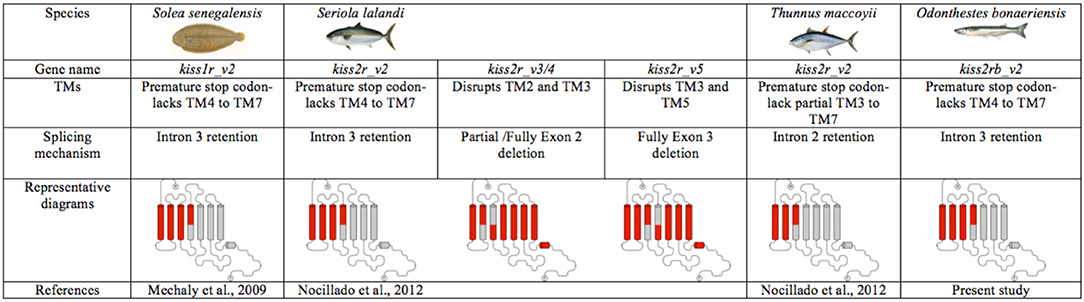
Figure 7. Different mechanisms of alternative splicing present in the kissr2 gene of teleost fish due to intron retention and transmembrane domain (TM) deletion in kissr2.
Alternative splicing is a mechanism that increases variability in protein products from a single gene (45). Although the physiological functions of alternative transcript isoforms are not completely understood, in some cases this mechanism could produce new products with defined functions (46). However, generation of variant products from alternative splicing not always results in functional proteins (47, 48). For example, if alternative splicing produces a large truncation, it is very unlikely that the synthesized polypeptide chain will fold properly (47). Moreover, most of the times, truncations are likely to produce dysfunctional proteins (49, 50). Accordingly, truncated GPCRs resulting from alternative splicing events are often retained in the endoplasmic reticulum (ER), preventing them to target the cell surface (24). For this reason, and to gain insights into the potential impact of alternative splicing on Kissr2 and Kissr3 structure/function, we generated structural models of the full-length receptors. Mapping of the missing regions of the spliced isoforms, Kissr2_v2 and Kissr3_v2 on the respective models, strongly suggests that the truncated receptors would be non-functional. Generally, in GPCRs, the missing structural elements (i.e., TM5–TM7) are involved either in ligand binding, signal transmission, or dimerization, thus they are critical for the correct functioning of the receptors (50). In some cases, mutations in rhodopsin receptors presented problems in the normal trafficking from the ER to the cell surface (51). Interestingly, in zebrafish the truncated isoform (called as KRBDP3) has been suggested to be functional from a regulatory point of view because it enters into the nucleus and presents ligand-independent transactivation activity (22). In the pejerrey case, however, we consider that the alternatively spliced receptor is non-functional, at least from the signaling point of view, similar to what has been observed in human KISSR1. Actually, single nucleotide mutations in this receptor were shown to be correlated with hypogonadotropic hypogonadism (HH) revealing that the alteration of the normal structure of this receptor will lead to lack of function (52, 53). Recently, a new alternative splicing mechanism in the 5′-untranslated regions (UTR) of kissr2 gene in Cynoglossus semilaevis was described. In that study, genomic analysis of kissr2 allowed the detection of three cDNA variants with common open reading frame (ORF) and 3′-UTR sequences, but with different 5′-UTR sequences (54). In this context, the alternative splicing events detected in pejerrey and in other teleost species (Figure 7) together with the existence of multiple promoters (54) seems to be a plausible mechanism to regulate kissr2 expression in teleosts.
In mammals, the reproductive axis also is regulated by energy balance (35) and kisspeptin is related to food intake and growth (55, 56). Although several recent studies have proposed that kisspeptins regulate reproduction in teleosts, little is known about their role in the control of food intake and energy balance. The response of fasting in kisspeptin genes was first evidenced in the Senegalese sole in which an up-regulation of both kiss2 and kissr2 was observed during fasting (26). In the present study, not only kiss2, but also kissr2_v1 was up-regulated after fasting while kissr2_v2 showed no expression, suggesting that kissr2_v1 is the functional form. Similar to that observed in the Senegalese sole (26) and a South American cichlid (57), an increase in pituitary Lh and Fsh mRNA was detected. It is important to say that we did not measure kissr3 expression in fasting animals because not only kissr3 gene was reported for Senegalese sole but also no kissr3 expression was observed in pejerrey hypothalamus. Taken together, these findings suggest that a short period of food restriction can trigger the reproductive axis in fish. This is probably because in some fish species, the absence or limited food supply is associated with the beginning of a period where fish start their reproductive season. Our results also support previous observations of the possible orexigenic role of the kissr2 (26, 27). However, in order to demonstrate the putative orexigenic role kisspeptins may an in fish, peptide-administration trials to test concomitant increase in foraging behavior need to be performed.
In summary, in this study, we have obtained the full genomic sequence of kissr2 and kissr3 in pejerrey and provided the first evidence for alternative splicing in both paralogous genes. Analysis of the Kissr2 and Kissr3 protein structures by 3D-models suggest that the alternative isoforms should give rise to non-functional GPCRs. The emergence of Next-generation sequencing (NGS), particularly RNAseq approaches, can offer promising avenues to discover novel isoforms of kisspeptin genes in other species and provide new information to study gene regulatory mechanism via alternative splicing in vertebrates.
Author Contributions
ASM and GMS conceived and designed the experiments. ASM, MT, AEM, MP, and AG performed the experiments. ASM, MT, AEM, ES, MP, AG, JV, and GMS analyzed the data. ASM and GMS wrote the paper. MT, AEM, AG, JV, and GO provided comments on the manuscript.
Funding
This study was funded by projects grants by the Agencia Nacional de Promoción Científica y Tecnológica (ANPCYT, Argentina) to ASM (PICT-2014-0515) and to GMS (PICT-2012-2619 and PICT-2015-2783).
Conflict of Interest Statement
The authors declare that the research was conducted in the absence of any commercial or financial relationships that could be construed as a potential conflict of interest.
Acknowledgments
Thanks are due to Javier Herdman (IIB-INTECH) for the fish maintenance used in this study. The Agencia Nacional de Promoción Científica y Tecnológica (ANPCYT) and the Argentine National Research Council (CONICET) are also acknowledge.
Supplementary Material
The Supplementary Material for this article can be found online at: https://www.frontiersin.org/articles/10.3389/fendo.2018.00604/full#supplementary-material
Supplementary Table 1. Reaction efficiencies slope and correlation r2 values of real-time quantitative PCR assays. The efficiency and conditions for primer sets for kiss2, lhb, and, fshb were already reported in Tovar Bohórquez et al. (29) and Elisio et al. (58).
Supplementary Table 2. Predicted receptor-ligand interactions.
Supplementary Figure 1. (A) Schematic representation of a portion of kissr2 gene in pejerrey showing the primer combinations used in this study. C1: kissr2-Ex3-4-F/kissr2-Ex4-R1, used to specifically amplify kissr2_v1, C2: kissr2-Int3-F/kissr2-Ex4-R, used to specifically amplify v2 isoform and C3: kissr2-Ex3-F/kissr2-Ex4-R, used to determine the presence of both isoforms in the tissue distribution experiments. (B) Representative agarose gel showing the expression of the kissr2 gene in mature pejerrey. Messenger RNA of the two isoforms was detected by RT-PCR (C3 primer combination). Lane 1, 100 bp ladder (Ld); Lane 2, genomic DNA (gDNA); Lane 3, testis cDNA; Lane 4, gill cDNA; Lane 5, rostral brain cDNA; Lane 6, medial brain cDNA; Lane 7, testis RNA with DNase; Lane 8, gill RNA with DNase; Lane 9, rostral brain RNA with DNase; Lane 10, medial brain RNA with DNase. (C) A melt curve analysis was run after each amplification cycle to confirm specificity of the reaction of kissr2_v1 (C1) and kissr2_v2 (C2) and to confirm the presence of two peaks in the case of primers that are able to detect two isoforms (C3).
Supplementary Figure 2. Homology model of the Kissr2. Pejerrey Kiss1 sequence is shown in red.
Supplementary Figure 3. Homology model of the Kissr3. Pejerrey Kiss2 sequence is shown in red.
Supplementary Figure 4. Weblogo 3 (http://weblogo.threeplusone.com/) representation of the sequence alignments in teleost fish peptides. (A) Teleost Kiss1-15: pjKiss1-15: QDVSSYNLNSFGLRY. (B) Teleost Kiss2-12: pjKiss2-12: SKFNYNPFGLRF.
Supplementary Figure 5. Summary of alternative splicing due to intron retention and exon deletion detected in kissr3 gene of teleost fish.
References
1. Venkatakrishnan AJ, Deupi X, Lebon G, Tate CG, Schertler GF, Babu MM. Molecular signatures of G-protein-coupled receptors. Nature (2013) 494:185–194. doi: 10.1038/nature11896
2. Markovic D, Challiss RAJ. Alternative splicing of G protein-coupled receptors: physiology and pathophysiology. Cell Mol Life Sci. (2009) 66:3337–52. doi: 10.1007/s00018-009-0093-4
3. Jacobson KA. New paradigms in GPCR drug discovery. Biochem Pharmacol. (2015) 98:541–55. doi: 10.1016/j.bcp.2015.08.085
4. Millar RP, Newton CL. The year in G protein-coupled receptor research. Mol Endocrinol. (2010) 24:261–74. doi: 10.1210/me.2009-0473
5. Fredriksson R, Lagerstrom MC, Lundin LG, Schioth HB. The G-protein-coupled receptors in the human genome form five main families. Phylogenetic analysis, paralogon groups, and fingerprints. Mol Pharmacol. (2003) 63:1256–72. doi: 10.1124/mol.63.6.1256
6. Kotani M, Detheux M, Vandenbogaerde A, Communi D, Vanderwinden JM, Le Poul E, et al. The metastasis suppressor gene KISS-1 encodes kisspeptins, the natural ligands of the orphan G protein-coupled receptor GPR54. J Biol Chem. (2001) 276:34631–6. doi: 10.1074/jbc.M104847200
7. Muir AI, Chamberlain L, Elshourbagy NA, Michalovich D, Moore DJ, Calamari A, et al. Axor12, a novel human G protein-coupled receptor, activated by the peptide KISS-1. J Biol Chem. (2001) 276:28969–75. doi: 10.1074/jbc.M102743200
8. Ohtaki T, Shintani Y, Honda S, Matsumoto H, Hori A, Kanehashi K, et al. Metastasis suppressor gene kiss-1encodes peptide ligand of a G-protein-coupled receptor. Nature (2001) 411:613–7. doi: 10.1038/35079135
9. De Roux N, Genin E, Carel JC, Matsuda F, Chaussain JL, Milgrom E. Hypogonadotropic hypogonadism due to loss of function of the KiSS1-derived peptide receptor GPR54. Proc Natl Acad Sci USA. (2003) 100:10972–6. doi: 10.1073/pnas.1834399100
10. Seminara SB, Messager S, Chatzidaki EE, Thresher RR, Acierno JS Jr, Shagoury JK, et al. The GPR54 gene as a regulator of puberty. N Engl J Med. (2003) 349:1614–27. doi: 10.1056/NEJMoa035322
11. Hauge-Evans AC, Richardson CC, Milne HM, Christie MR, Persaud SJ, Jones PM. A role for kisspeptin in islet function. Diabetologia (2006) 49:2131–5. doi: 10.1007/s00125-006-0343-z
12. Mead EJ, Maguire JJ, Kuc RE, Davenport AP. Kisspeptins are novel potent vasoconstrictors in humans, with a discrete localization of their receptor, G protein-coupled receptor 54, to atherosclerosis-prone vessels. Endocrinology (2007) 148:140–7. doi: 10.1210/en.2006-0818
13. Makri A, Pissimissis N, Lembessis P, Polychronakos C, Koutsilieris M. The kisspeptin (KiSS1-1)/Gpr54 system in cancer biology. Cancer Treat Rev. (2008) 34:682–92. doi: 10.1016/j.ctrv.2008.05.007
14. Aydin M, Oktar S, Yonden Z, Ozturk OH, Yilmaz B. Direct and indirect effects of kisspeptin on liver oxidant and antioxidant systems in young male rats. Cell Biochem Funct. (2010) 28:293–9. doi: 10.1002/cbf.1656
15. Qureshi IZ, Kanwal S. Novel role of puberty onset protein kisspeptin as an anticoagulation peptide. Blood Coagul Fibrinolysis (2012) 22:40–9. doi: 10.1097/MBC.0b013e328340e6a7
16. Clarkson J, Busby ER, Kirilov M, Schutz G, Sherwood NM, Herbison AE. Sexual differentiation of the brain requires perinatal kisspeptin-GnRH neuron signaling. J Neurosci. (2014) 34:15297–305. doi: 10.1523/JNEUROSCI.3061-14.2014
17. Pasquier J, Lafont AG, Tostivint H, Vaudry H, Rousseau K, Dufour S. Comparative evolutionary histories of kisspeptins and kisspeptin receptors in vertebrates reveal both parallel and divergent features. Front Endocrinol. (2012) 3:173. doi: 10.3389/fendo.2012.00173
18. Mechaly AS, Viñas J, Piferrer F. The kisspeptin system genes in teleost fish, their structure and regulation, with particular attention to the situation in Pleuronectiformes. Gen Comp Endocrinol. (2013) 188:258–68. doi: 10.1016/j.ygcen.2013.04.010
19. Pasquier J, Kamech N, Lafont AG, Vaudry H, Rousseau K, Dufour S. Molecular evolution of GPCRs: kisspeptin/kisspeptin receptors. J Mol Endocrinol. (2014) 52:101–17. doi: 10.1530/JME-13-0224
20. Mechaly AS, Viñas J, Piferrer F. Identification of two isoforms of the Kisspeptin-1 receptor (kiss1r) generated by alternative splicing in a modern teleost, the Senegalese sole (Solea senegalensis). Biol Reprod. (2009) 80:60–9. doi: 10.1095/biolreprod.108.072173
21. Nocillado JN, Biran J, Lee YY, Levavi-Sivan B, Mechaly AS, Zohar Y, et al. The Kiss2 receptor (Kiss2r) gene in Southern Bluefin Tuna, Thunnus maccoyii and in Yellowtail Kingfish, Seriola lalandi – Functional analysis and isolation of transcript variants. Mol Cell Endocrinol. (2012) 362:211–20. doi: 10.1016/j.mce.2012.06.024
22. Onuma TA, Duan C. Duplicated Kiss1 receptor genes in zebrafish: distinct gene expression patterns, different ligand selectivity, and a novel nuclear isoform with transactivating activity. FASEB J. (2012) 26:2941–50. doi: 10.1096/fj.11-201095
23. Pasquier J, Lafont AG, Jeng SR, Morini M, Dirks R, van den Thillart G, et al. Multiple kisspeptin receptors in early Osteichthyans provide new insights into the evolution of this receptor family. PLoS ONE (2012) 7:e48931. doi: 10.1371/journal.pone.0048931
24. Wise H. The roles played by highly truncated splice variants of G protein-coupled receptors. J Mol Signal. (2012) 7:13. doi: 10.1186/1750-2187-7-13
25. Volkoff H. The neuroendocrine regulation of food intake in fish: a review of current knowledge. Front Neurosci. (2016) 10:540. doi: 10.3389/fnins.2016.00540
26. Mechaly AS, Viñas J, Piferrer F. Gene structure analysis of kisspeptin-2 (Kiss2) in the Senegalese sole (Solea senegalensis): characterization of two splice variants of Kiss2, and novel evidence for metabolic regulation of kisspeptin signaling in non-mammalian species. Mol Cell Endocrinol. (2011) 339:14–24. doi: 10.1016/j.mce.2011.03.004
27. Escobar S, Felip A, Zanuy S, Carrillo M. Is the kisspeptin system involved in responses to food restriction in order to preserve reproduction in pubertal male sea bass (Dicentrarchus labrax)? Comp Biochem Physiol A (2016) 199:38–46. doi: 10.1016/j.cbpa.2016.05.005
28. Campanella D, Caler E, Miller J, Lorenzi H, Fernandino JI, Valenzuela N, et al. Phylogenetic context, whole genome sequencing, assembly and annotation of a new model species with Temperature-dependent Sex Determination. Abstracts. In: The 8th International Conference on Genomics. Shenzhen (2013).
29. Tovar Bohórquez MO, Mechaly AS, Hughes LC, Campanella D, Ortí G, Canosa LF, et al. Kisspeptin system in pejerrey fish (Odontesthes bonariensis). Characterization and gene expression pattern during early developmental stages. Comp Biochem Physiol A (2017) 204:146–56. doi: 10.1016/j.cbpa.2016.11.014
30. Aljanabi SM, Martinez I. Universal and rapid salt-extraction of high quality genomic DNA for PCR-based techniques. Nucleic Acids Res. (1997) 25:4692–3. doi: 10.1093/nar/25.22.4692
31. Sandal M, Duy TP, Cona M, Zung H, Carloni P, Musiani F, et al. GOMoDo: A GPCRs online modeling and docking webserver. PLoS ONE (2013) 8:e74092. doi: 10.1371/journal.pone.0074092
32. Bond CS. TopDraw: a sketchpad for protein structure topology cartoons. Bioinformatics (2003) 19:311–2. doi: 10.1093/bioinformatics/19.2.311
33. Bustin SA. Absolute quantification of mRNA using real-time reverse transcription polymerase chain reaction assays. J Mol Endocrinol. (2000) 25:169–93. doi: 10.1677/jme.0.0250169
34. Szczepek M, Beyrière F, Hofmann KP, Elgeti M, Kazmin R, Rose A, et al. Crystal structure of a common GPCR-binding interface for G protein and arrestin. Nat Commun. (2004) 5:4801. doi: 10.1038/ncomms5801
35. Parhar IS, Ogawa S, Sakuma Y. Laser-captured single digoxigenin-labeled neurons of gonadotropin-releasing hormone types reveal a novel G protein – coupled receptor (Gpr54) during maturation in cichlid fish. Endocrinology (2004) 145:3613–8. doi: 10.1210/en.2004-0395
36. Tena-Sempere M, Felip A, Gómez A, Zanuy S, Carrillo M. Comparative insights of the kisspeptin/kisspeptin receptor system: lessons from non-mammalian vertebrates. Gen Comp Endocrinol. (2012) 175:234–43. doi: 10.1016/j.ygcen.2011.11.015
37. Li S, Zhang Y, Liu Y, Huang X, Huang W, Lu D, et al. Structural and functional multiplicity of the kisspeptin/GPR54 system in goldfish (Carassius auratus). J Endocrinol. (2009) 201:407–18. doi: 10.1677/JOE-09-0016
38. Schwartz TW, Frimurer TM, Holst B, Rosenkilde MM, Elling CE. Molecular mechanism of 7TM receptor activation-a global toggle switch model. Ann Rev Pharmacol Toxicol. (2006) 46:481–519. doi: 10.1146/annurev.pharmtox.46.120604.141218
39. Zhang D, Zhao Q, Wu B. Structural studies of G protein-coupled receptors. Mol Cells (2015) 38:836–842. doi: 10.14348/molcells.2015.0263
40. Palczewski K, Kumasaka T, Hori T, Behnke CA, Motoshima H, Fox BA, et al. Crystal structure of rhodopsin: a G protein-coupled receptor. Science (2000) 289:739–45. doi: 10.1126/science.289.5480.739
41. Shonberg J, Kling RC, Gmeiner P, Löber S. GPCR crystal structures: medicinal chemistry in the pocket. Bioorg Med Chem. (2015) 23:3880–906. doi: 10.1016/j.bmc.2014.12.034
42. Kobilka BK. The structure and function of G-protein-coupled receptors. Biochem Biophys Acta (2007) 1768:794–807. doi: 10.1016/j.bbamem.2006.10.021
43. Rosenbaum DM, Rasmussen SG, Kobilka BK. The structure and function of G-protein-coupled receptors. Nature (2009) 459:356–63. doi: 10.1038/nature08144
44. Cáceres JF, Kornblihtt AR. Alternative splicing: multiple control mechanisms and involvement in human disease. Trends Genet. (2002) 8:186–93. doi: 10.1016/S0168-9525(01)02626-9
45. Black DL. Protein diversity from alternative splicing: a challenge for bioinformatics and post-genome biology. Cell (2000) 103:367–70. doi: 10.1016/S0092-8674(00)00128-8
46. Kouza M, Hansmann UHE. Folding simulations of the A and B domain of Protein GJ. Phys Chem B (2012) 116:6645–53. doi: 10.1021/jp210497h
47. Tress ML, Martelli PL, Frankish A, Reeves GA, Wesselink JJ, Yeats C, et al. The implications of alternative splicing in the ENCODE protein complement. Proc Natl Acad Sci USA. (2007) 104:5495–500. doi: 10.1073/pnas.0700800104
48. Tress ML, Abascal F, Valencia A. Alternative splicing may not be the key to proteome complexity. Trends Biochem Sci. (2017) 42:98–110. doi: 10.1016/j.tibs.2016.08.008
49. Lareau LF, Brenne SE. Regulation of splicing factors by alternative splicing and NMD is conserved between kingdoms yet evolutionarily flexible. Mol Biol Evol. (2015) 32:1072–9. doi: 10.1093/molbev/msv002
50. Xue L, Rovira X, Scholler P, Zhao H, Liu J, Pin JP, et al. Major ligand-induced rearrangement of the heptahelical domain interface in a GPCR dimer. Nat Chem Biol. (2015) 11:134–40. doi: 10.1038/nchembio.1711
51. Drake MT, Shenoy SK, Lefkowitz RJ. Trafficking of G protein-coupled receptors. Circ Res. (2006) 99:570–82. doi: 10.1161/01.RES.0000242563.47507.ce
52. Breuer O, Abdulhadi-Atwan M, Zeligson S, Fridman H, Renbaum P, Levy-Lahad E, et al. A novel severe N-terminal splice site KISS1R gene mutation causes hypogonadotropic hypogonadism but enables a normal development of neonatal external genitalia. Eur J Endocrinol. (2012) 167:209–16. doi: 10.1530/EJE-12-0127
53. Silveira LG, Latronico AC, Seminara SB. Kisspeptin and clinical disorders. Adv Exp Med Biol. (2013) 784:187–99. doi: 10.1007/978-1-4614-6199-9_9
54. Song H, Wand M, Wang Z, Liu J, Qi J, Zhang Q. Characterization of kiss2 and kissr2 genes and the regulation of kisspeptin on the HPG axis in Cynoglossus semilaevis. Fish Physiol Biochem. (2017) 43:731–53. doi: 10.1007/s10695-016-0328-x
55. Foradori CD, Whitlock BK, Daniel JA, Zimmerman AD, Jones MA, Read CC, et al. Kisspeptin stimulates growth hormone release by utilizing neuropeptide Y pathways and is dependent on the presence of ghrelin in the ewe. Endocrinology (2017) 158:3526–39. doi: 10.1210/en.2017-00303
56. Talbi R, Laran-Chich MP, Magoul R, El Ouezzani S, Simonneaux V. Kisspeptin and RFRP-3 differentially regulate food intake and metabolic neuropeptides in the female desert jerboa. Sci Rep UK (2016) 6:36057. doi: 10.1038/srep36057
57. Pérez Sirkin DI, Di Yorio MP, Delgadin TH, Karp PJ, Somoza GM, Vissio PG. Food restriction stimulates final maturation of spermatogenesis and induces variation in leptin-a expression in the cichlid fish Cichlasoma dimerus. In: 10th International Symposium on Reproductive Physiology of Fish (Olhão) (2014).
Keywords: kissr2, kissr3, kisspeptin receptors, pejerrey fish, alternative splicing, modeling, GoMoDo, fasting
Citation: Mechaly AS, Tovar Bohórquez MO, Mechaly AE, Suku E, Pérez MR, Giorgetti A, Ortí G, Viñas J and Somoza GM (2018) Evidence of Alternative Splicing as a Regulatory Mechanism for Kissr2 in Pejerrey Fish. Front. Endocrinol. 9:604. doi: 10.3389/fendo.2018.00604
Received: 24 January 2018; Accepted: 21 September 2018;
Published: 17 October 2018.
Edited by:
Ishwar Parhar, Monash University Malaysia, MalaysiaReviewed by:
Takashi Kitahashi, Niigata University, JapanWei Hu, Institute of Hydrobiology (CAS), China
Copyright © 2018 Mechaly, Tovar Bohórquez, Mechaly, Suku, Pérez, Giorgetti, Ortí, Viñas and Somoza. This is an open-access article distributed under the terms of the Creative Commons Attribution License (CC BY). The use, distribution or reproduction in other forums is permitted, provided the original author(s) and the copyright owner(s) are credited and that the original publication in this journal is cited, in accordance with accepted academic practice. No use, distribution or reproduction is permitted which does not comply with these terms.
*Correspondence: Alejandro S. Mechaly, amechaly@inidep.edu.ar
Gustavo M. Somoza, somoza@intech.gov.ar
†Present Address: Alejandro S. Mechaly, Instituto Nacional de Investigación y Desarrollo Pesquero, Mar del Plata, Argentina