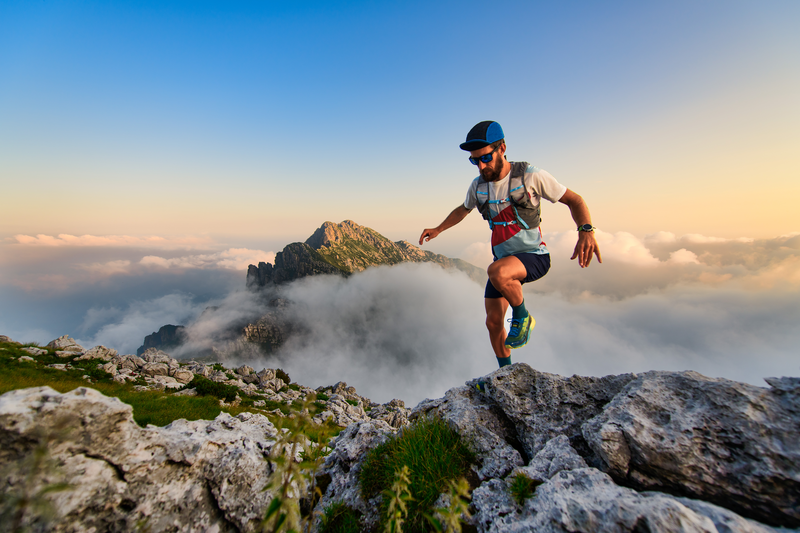
95% of researchers rate our articles as excellent or good
Learn more about the work of our research integrity team to safeguard the quality of each article we publish.
Find out more
SYSTEMATIC REVIEW article
Front. Endocrinol. , 03 August 2018
Sec. Thyroid Endocrinology
Volume 9 - 2018 | https://doi.org/10.3389/fendo.2018.00432
Background: Serum thyroid-stimulating hormone (TSH) and free thyroxine (FT4) change dynamically during pregnancy. Differences in geographic regions, populations, and manufacturer's methodologies can affect the reference intervals for thyroid function tests. The 2017 guidelines of the American Thyroid Association (ATA) recommended 4.0 mU/L as the cut-off point for the upper limit of serum TSH in early pregnancy. A systematic review is called for to establish practical, gestational-specific TSH and FT4 reference intervals for pregnant Chinese women and to explore whether the criteria are suitable for China.
Methods: English and Chinese articles published from inception to Aug 2017 were searched in the PubMed, EMBASE, and SCIE English-language databases and the CNKI, WanFang, and CQVIP Chinese databases. The relative descent or ascent rates of serum TSH and FT4 were calculated, after which Comprehensive Meta-Analysis V2.0 software was used to analyze the data.
Results: Eleven studies (6 in English and 5 in Chinese), five kits and 11,629 Chinese women from nine cities were considered in this meta-analysis. Compared with the reference ranges provided by manufacturers, serum TSH decreased in the first trimester, with the upper limit declining by 21.7% (5.0–36.6%), to a value close to 4.0 mU/L, and the lower limit declining by 85.7% (73.5–97.1%). It continued decreasing in the second trimester, with the upper limit declining by 24.0% (6.4–40.9%) and the lower limit declining by 40.7% (9.0–85.7%). For FT4, the upper limit fluctuated slightly, and the lower limit increased by 6.8% (1.0–14.6%) in the first trimester. Serum FT4 dropped gradually, with the upper limit declining by 21.8% (2.5–31.8%) and the lower limit declining by 12.7% (2.6–19.6%) in the second trimester. During the third trimester, the upper limit decreased by 25.1% (12.7–35.0%), while the lower limit decreased by 20.9% (14.8–27.3%).
Conclusions: Various regions, kits and test methods affect the gestational TSH and FT4 levels. The non-pregnant serum TSH upper limit minus 22% is very close to 4.0 mU/L, which can be used as a sub-optimal approach to represent the cut-off value for pregnant Chinese women in the first trimester.
Thyroid hormone is essential for the growth and development of the human body. It plays a vital role in promoting the development of the skeletal, nervous, and reproductive systems (1). Pregnancy affects the thyroid gland and its function profoundly. Human chorionic gonadotrophin (hCG) significantly increases in early pregnancy, sharing the same alpha-subunits and 80%-homologous beta-subunits with TSH. Therefore, hCG can stimulate thyroid hormonogenesis, which is the negative-feedback system to TSH secretion, causing the serum TSH level to decline during early pregnancy (2, 3).
Serum TSH and FT4 vary with gestational age. Several studies and guidelines have indicated that non-pregnant reference intervals of serum TSH and FT4 are not applicable for diagnosing thyroid diseases during pregnancy. By contrast, trimester- and method-specific reference ranges for thyroid testing have been strongly recommended because of their higher accuracy (3–6). Nevertheless, the formulation of gestational reference ranges is affected by many factors, limiting their feasibility (7, 8). The 2011 guidelines of the American Thyroid Association (ATA) suggested a specific upper limit cut-off (2.5 mU/L) for serum TSH in the first trimester of pregnancy (4). However, there are large differences in TSH and FT4 reference ranges between various populations, with 90% of the relevant studies having higher TSH upper limits than the TSH cut-off point of 2.5 mU/L. These inconsistencies could increase the misdiagnosis rate of overt and subclinical hypothyroidism in pregnancy (9). The 2017 ATA guidelines noted that if internal or transferable pregnancy-specific TSH reference intervals are unavailable, an upper reference limit of 4.0 mU/L may be used, representing the non-pregnant TSH upper limit minus 0.5 mU/L (5). However, it is uncertain whether this cut-off is appropriate for pregnant Chinese women. Similarly, gestational- and method-specific criteria are also recommended for serum FT4 (5), but the criteria for serum FT4 are as inconvenient as those of TSH to diagnose gestational hypothyroxinemia in clinical practice.
The aim of the current study was to systematically assess and summarize gestational- and method-specific serum TSH and FT4 reference ranges in various regions in China and determine their trends in early, middle and late pregnancy. We compared the differences between the reference ranges of Chinese pregnant women and the 2017 ATA guidelines recommendation of 4.0 mU/L. Finally, we aimed to provide feasible and practical reference intervals to diagnose hypothyroidism and hypothyroxinemia in pregnancy.
A systematic literature search (PubMed, EMBASE, SCIE, Chinese National Knowledge Infrastructure, Chinese Scientific Journals Full-text Database, Wanfang) was performed from inception to Aug 2017. The keywords “TSH” and “FT4” combined with the terms “reference range” or “reference interval,” “pregnant,” or “gestational,” and “China” or “Chinese” were used to search for potentially relevant studies in English and Chinese. The following is an example for PubMed: (((((#TSH AND #FT4))) AND ((#pregan* OR #gestation*)) AND ((#China OR #Chinese)) AND ((#reference range* OR #reference interval*)). To identify additional studies and expand our search, the reference lists of the retrieved articles were scanned.
The studies included in the meta-analysis conformed with the following conditions: All subjects were pregnant Chinese women. The study recruitment standards met the National Academy of Clinical Biochemistry (NACB) recommendations: (1) more than 120 subjects; (2) no TPOAb or TGAb positivity; (3) no family or personal history of thyroid disease; (4) no goiter; and (5) no medical history influencing thyroid function (except use of estrogens) (6).
Exclusion criteria were as follows: (1) subjects came from iodine-excessive or iodine-deficient regions; (2) the Newcastle-Ottawa quality assessment scale (NOS) quality score was <6 (10); (3) serum TSH- and FT4-related information could not be extracted; and (4) the study was a repeat of an earlier study. In addition, Li et al. (11) declared that the reference intervals for non-pregnant women should be used from 4 to 6 gestational weeks. Therefore, studies including 0–6 gestational weeks or average gestational week <9.3 weeks in the first trimester were excluded to improve the accuracy of the meta-analysis.
The NOS was selected to assess the quality of the included studies using the “star system.” Information regarding selection, comparability, and outcomes was evaluated with a maximum of 4 stars, 2 stars, and 3 stars, respectively. The total full score = 9. A study graded ≥6 stars was considered a high-quality study (10).
Two reviewers (Gao XT and Li YZ) abstracted the following data from all eligible studies independently: first author; publication year and journal; region(s) and hospital(s) of study; sample size; pregnancy stages; medians and percentiles (2.5th and 97.5th) of serum TSH and FT4; manufacturers; inter- and intra-assay coefficients of variation (CV) in the laboratory; normal range of the detection kit; normal range of the control group; and iodine status of the region.
We summarized the lower reference limits (2.5th) and the upper reference limits (97.5th) of serum TSH and FT4 in early, middle and late pregnancy. We calculated the relative descent or ascent rate of serum TSH and FT4 and compared these with the normal reference ranges provided by manufacturers involved in each enrolled study. The calculation formula can be written as:
Relative descent rate of lower limit = (2.5th in non-pregnancy−2.5th in pregnancy)/2.5th in non-pregnancy × 100%;
Relative descent rate of upper limit = (97.5th non-pregnancy−97.5th in pregnancy)/97.5th non-pregnancy × 100%.
The meta-analysis of the relative descent and ascent rates for the gestational reference intervals was accomplished using Comprehensive Meta-Analysis software (V2.0, Biostat, Englewood, NJ). The Z test was used to compare the difference between 0 and the relative change rates of TSH and FT4 reference intervals (p < 0.05, 0.05 < p < 0.1 and p > 0.1 indicated high, medium, and no difference between relative change rate and 0, respectively).
Factors affecting gestational TSH and FT4 were age, iodine nutrition status, ethnicity, sex, and hour of the day, in addition to the conditions referred to in the NACB (12). Our meta-analysis included pregnant women of appropriate age who came from adequate-iodine regions of China, and the blood samples were taken in morning in the fasting state.
A total of 265 studies were initially considered for inclusion, of which 4 were excluded due to duplication, and 219 articles were excluded after screening the titles and abstracts. After more detailed evaluation of the remaining 42 articles, 31 articles were excluded. Finally, the remaining 11 studies (6 published in English and 5 in Chinese) involving 5 types of kits and including 11,629 Chinese women met the inclusion criteria and were included in this meta-analysis (Figure 1). There were 4 studies on the application of Roche e600/601 with 1,920 pregnant Chinese women; 3 studies using the Bayer ADVIA Centaur with 3,441 pregnant Chinese women; 4 studies using the Beckman with 2,350 pregnant Chinese women; 2 studies using the Abbott Architect I 2,000 with 1,223 pregnant Chinese women, and 2 studies using the DPC Immulite 1,000 with 1,189 pregnant Chinese women. The qualified studies were published from 2008 to 2016 and proved to be of good quality in accordance with the NOS scoring system (Supplementary Table 1).
Table 1 displays the basic characteristics of the included studies regarding serum TSH. According to the median, serum TSH decreased in early pregnancy and showed an upward trend during middle and late pregnancy (Figure 2A).
Table 1. Gestational TSH reference intervals and relative descent or ascent rate compared with non-pregnancy in Chinese women.
Figure 2. The trend of serum TSH (A) and FT4 (B) median during pregnancy. T1, the first trimester of pregnancy; T2, the second trimester of pregnancy; T3, the third trimester of pregnancy. (A) The trend of median gestational serum TSH in each study (3 studies with Roche kits; 2 studies with Bayer kits; 2 studies with Abbott kits; 2 studies with DPC kits; 3 studies with Beckman kits). (B) The trend of gestational serum FT4 median in each study (2 studies with Roche kits; 2 studies with Bayer kits; 2 studies with Abbott kits; 1 study with DPC kits; 3 studies with Beckman kits).
Table 2 shows the basic characteristics of the included studies regarding serum FT4. In the first trimester, no obvious rule was derived for the serum FT4 upper limit (lower than the non-pregnant levels in six studies; higher than the non-pregnant levels in the other five studies). However, the lower limits were higher than those in non-pregnancy. In the second and third trimesters, both the upper and lower limits of serum FT4 were lower than those in non-pregnancy. The gestational serum FT4 medians exhibited a downward trend (Figure 2B).
Table 2. Gestational FT4 reference intervals and relative descent or ascent rate compared with non-pregnancy in Chinese women.
We used the random-effects model to summarize the descending rule of serum TSH in early pregnancy and the descending and ascending rules of serum FT4 in each gestational stage by meta-analysis.
Figure 3A shows the summarized relative descent rate [85.7%, 95% confidence interval (CI): 84.5, 86.8%] for the serum TSH lower limit in the first trimester from 2008 to 2016. The relative descent rate in each study ranged from 73.5% (95% CI: 68.3, 78.2%) to 97.1% (95% CI: 94.5, 98.5%). This suggested that the lower limit of serum TSH decreased in the first trimester compared with that in non-pregnancy, and the descent rate was 85.7% (73.5–97.1%).
Figure 3. Meta-analysis of the relative descent rate of TSH lower (A) and upper (B) reference limits in early pregnancy. Figure shows unadjusted relative descent rate of TSH lower (A) and upper (B) limit estimates in early pregnancy with 95% confidence limits for each study selected. Pooled relative descent rate estimates are represented as diamonds in this plot.
Figure 3B shows the summarized relative descent rate (21.7%, 95% CI: 20.4, 23.1%) for the serum TSH upper limit in the first trimester. The relative descent rate in individual studies ranged from 5.0% (95% CI: 2.9, 8.5%) to 36.6% (95% CI: 29.2, 44.8%), suggesting that, compared to the non-pregnant levels, the serum TSH upper limit decreased in early pregnancy, and the descent rate was 21.7% (5.0–36.6%).
Figure 4 shows the comparison of the serum TSH upper limits acquired in different conditions. If we subtract 0.5 mU/L from the upper limits provided by manufacturers (97.5th in non-pregnancy), the gestational TSH upper limits obtained (97.5th in non-pregnancy−0.5), which ranged from 3.45 to 5.14 mU/L, varied greatly, and the gaps around 4.0 mU/L, which ranged from −0.55 to 1.14 mU/L, were different from each other. The absolute values of the gaps were >1, suggesting that the fluctuation around 4.0 mU/L was obvious.
Figure 4. Comparison of serum TSH upper reference limits under different conditions. Figure shows the comparison among serum TSH upper reference limits acquired in different conditions and shows the gaps between the limits and 4.0 mU/L. T1, the first trimester of pregnancy; 97.5th, the upper reference limit; TSH, thyroid stimulating hormone; 97.5th in non-pregnancy, the normal TSH upper limits provided by 5 kinds of manufacturers (Roche, Bayer, Abbott, DPC, and Beckman); 97.5th in T1, The real serum TSH upper limits during early pregnancy provided by 11 enrolled studies. 97.5th in non-pregnancy–0.5, We subtracted 0.5 mU/L from the normal TSH upper limits provided by 5 kinds of manufacturers (Roche, Bayer, Abbott, DPC, and Beckman), according to 2017 ATA guidelines' recommendation that 4.0 mU/L represents a reduction in the non-pregnant TSH upper reference limit of ~0.5 mU/L (5); (1–22%) × 97.5th in non-pregnancy, In our meta-analysis, regardless of manufacture, the non-pregnant serum TSH upper limit decreased by 22% in the first trimester of pregnancy; (1–descent rate) × 97.5th in non-pregnancy, In our meta-analysis, the relative descent rate of the TSH upper limit during early pregnancy assayed by Roche, Bayer, Abbott, DPC and Beckman were 22.7, 18.3, 24.8, 17.6, and 25.5%, respectively. The relative descent rate of each kit is listed in Supplementary Table 2. We calculated the method-specific gestational upper limit by non-pregnant upper limit decreased by the relative descent rate.
By contrast, if we compare 4.0 mU/L with the gestational TSH upper limit, which was 22% lower than the non-pregnant upper limit, [(1–22%) × 97.5th in non-pregnancy], ranging from 3.12 to 4.40 mU/L, the gaps ranging from −0.88 to 0.40 mU/L were narrower than those of “97.5th in non-pregnancy – 0.5” ranging from −0.55 to 1.14 mU/L. Similarly, if we replace 22% with the relative descent rate of each kit (Roche, Bayer, Abbott, DPC and Beckman were 22.7, 18.3, 24.8, 17.6 and 25.5%, respectively) listed in Supplementary Table 2, the gestational upper limits obtained [(1–descent rate) × 97.5th in non-pregnancy] ranged from 3.30 to 4.36 mU/L. The gaps between 4.0 mU/L and ‘(1–descent rate) × 97.5th in non-pregnancy' were much narrower, which ranged from −0.70 to 0.36 mU/L. The absolute values of the gaps in both groups were less than 1, suggesting that the non-pregnant upper limit that declined by its relative descent rate was much closer to 4.0 mU/L.
Figure 4 also shows that the comparison between 4.0 mU/L and the TSH upper limits of the first trimester in Chinese women (97.5th in T1). If we subtract “97.5th in T1” from 4.0 mU/L, the gaps ranged from −0.85 to 1.0 mU/L. The absolute values of the gaps were ≤1. These results suggest that regardless of efforts to standardize the reference ranges, there were still differences in comparison to the real TSH upper limits of pregnant Chinese women, while the differences were not very significant.
Figure 5A shows the summarized relative descent rate (40.7%, 95% CI: 38.9, 42.5%) for the serum TSH lower limit in the second trimester. The relative descent rate in each study ranged from 9.0% (95% CI: 7.3, 10.9%) to 85.7% (95% CI: 81.3, 89.2%). This suggests that the lower limit of serum TSH decreased in the second trimester compared with that in non-pregnancy, and the descent rate was 40.7% (9.0–85.7%).
Figure 5. Meta-analysis of relative descent rate of TSH lower (A) and upper (B) reference limits in middle pregnancy. Figure shows unadjusted relative descent rate of TSH lower (A) and upper (B) limit estimates in middle pregnancy with 95% confidence limits for each study selected. Pooled relative descent rate estimates are represented as diamonds in this plot.
Figure 5B shows the summarized relative descent rate (24.0%, 95% CI: 22.6, 25.5%) for the serum TSH upper limit in the second trimester. The relative descent rate in individual studies ranged from 6.4% (95% CI: 5.0, 8.1%) to 40.9% (95% CI: 35.5, 46.5%), suggesting that, compared to the non-pregnant levels, the serum TSH upper limit decreased in middle pregnancy, and the descent rate was 24.0% (6.4–40.9%).
Table 1 lists the changing characteristics of the serum TSH lower limit in the third trimester. Seven studies showed that the lower limit increased compared with non-pregnant levels. By contrast, the lower limit decreased in the other 5 studies. The fluctuation range varied from down by 51.43% to up by 120.59%. Therefore, there was no definite change rule regarding the TSH lower limit in late pregnancy, and the fluctuation range was wide.
Table 1 also lists the changing characteristics of the serum TSH upper limit in late pregnancy. Five studies showed that the upper limit increased compared with non-pregnant levels. By contrast, the upper limit decreased in the other 7 studies. The fluctuation range varied from down by 34.46% to up by 27.33%. Therefore, there was no definite change rule regarding the TSH upper limit in the third trimester, and the fluctuation range was wide.
Figure 6 shows the summarized relative ascent rate (6.8%, 95% CI: 5.9, 7.7%) for the serum FT4 lower limit in the first trimester. The relative ascent rate in all studies ranged from 1.0% (95% CI: 0.2, 4.9%) to 14.6% (95% CI: 9.7, 21.4%), suggesting that the serum FT4 lower limit increased in early gestation compared to the non-pregnant levels, and the ascent rate was 6.8% (1.0–14.6%).
Figure 6. Meta-analysis of relative ascent rate of FT4 lower reference limits in early pregnancy. Figure shows unadjusted relative ascent rate of FT4 lower limit estimates in early pregnancy with 95% confidence limits for each study selected. Pooled relative ascent rate estimates are represented as diamonds in this plot.
Table 2 lists the changing characteristics of the serum FT4 upper limit in the first trimester. Six studies showed that the upper limit decreased compared with non-pregnant levels. By contrast, the upper limit increased in the other 5 studies. The fluctuation range varied from down by 16.65% to up by 8.76%. Therefore, there was no definite change rule regarding the FT4 upper limit in early pregnancy, and the fluctuation range was small.
Figure 7A summarizes the relative descent rate (12.7%, 95% CI: 11.5, 14.0%) with regard to the serum FT4 lower limit during the second trimester. The relative descent rate in the included studies ranged from 2.6% (95% CI: 1.4, 4.8%) to 19.6% (95% CI: 15.5, 24.4%). This suggested that the serum FT4 lower limit decreased in middle pregnancy compared with non-pregnant levels, and the descent rate was 12.7% (2.6–19.6%).
Figure 7. Meta-analysis of relative descent rate of FT4 lower (A) and upper (B) reference limits in middle pregnancy. Figure shows unadjusted relative descent rate of FT4 lower (A) and upper (B) limit estimates in middle pregnancy with 95% confidence limits for each study selected. Pooled relative descent rate estimates are represented as diamonds in this plot.
Figure 7B summarizes the relative descent rate (21.8%, 95% CI: 20.3, 23.5%) for the serum FT4 upper limit in the second trimester. The relative descent rate in the included studies ranged from 2.5% (95% CI: 1.0, 6.5%) to 31.8% (95% CI: 28.9, 34.7%). This suggested that the serum FT4 upper limit decreased in middle pregnancy compared with the non-pregnant levels, and the descent rate was 21.8% (2.5–31.8%).
Figure 8A shows the summarized relative descent rate (20.9%, 95% CI: 19.5, 22.3%) for the serum FT4 lower limit in the third trimester. The relative descent rate in the included studies ranged from 14.8% (95% CI: 11.5, 18.8%) to 27.3% (95% CI: 22.8, 32.4%). This suggested that the lower limit of serum FT4 decreased in the third trimester of pregnancy compared with the non-pregnant levels, and the descent rate was 20.9% (14.8–27.3%).
Figure 8. Meta-analysis of relative descent rate of FT4 lower (A) and upper (B) reference limits in late pregnancy. Figure shows unadjusted relative descent rate of FT4 lower (A) and upper (B) limit estimates in late pregnancy with 95% confidence limits for each study selected. Pooled relative descent rate estimates are represented as diamonds in this plot.
Figure 8B shows the summarized relative descent rate (25.1%, 95% CI: 23.6, 26.7%) for the serum FT4 upper limit in the third trimester. The relative descent rate in all studies ranged from 12.7% (95% CI: 9.5, 16.7%) to 35.0% (95% CI: 28.7, 41.8%), suggesting that the serum FT4 upper limit declined in late gestation compared with non-pregnant levels, and the descent rate was 25.1% (12.7–35.0%).
Compared with the non-pregnant reference ranges provided by manufacturers, serum TSH showed a downward trend during early pregnancy, with the upper limit decreasing by 21.7% and the lower limit decreasing by 85.7%. It maintained this descending trend in middle pregnancy, with the upper limit decreasing by 24.0% and the lower limit decreasing by 40.7%. Then, in late pregnancy, serum TSH gradually increased to non-pregnant levels. For serum FT4, the upper limit changed slightly, with the lower limit increasing by 6.8% compared to non-pregnant levels in early pregnancy. Then, serum FT4 gradually declined, with the upper limit decreasing by 21.8% and the lower limit decreasing by 12.7% in the second trimester. It kept decreasing in the third trimester, with the upper limit decreasing by 25.1% and the lower limit decreasing by 20.9%.
Pregnancy causes increases in renal iodine excretion, thyroxine binding proteins, and thyroid hormone production. A healthy thyroid adjusts thyroid hormone metabolism, iodine uptake, and the hypothalamic-pituitary-thyroid axis to mediate such changes. The peak rise in hCG also occurs during early pregnancy (13, 14). Maternal hCG plays a direct role in stimulating the TSH receptor to produce thyroid hormone, resulting in a decrease in serum TSH. Thus, serum hCG increases in association with a corresponding reduction in serum TSH (2, 3). Starting at gestational 6–8 weeks, maternal serum estrogens increase progressively until term, which is accompanied by total T4 increasing, FT4 decreasing, and TSH progressively increasing throughout the pregnancy (15). Therefore, the non-pregnant reference ranges for thyroid function tests are not applicable to pregnant women. National guidelines throughout the world have recommended the use of gestational- and population-specific serum TSH and FT4 reference ranges to diagnose thyroid disease during pregnancy (4, 5) (16–20). According to the 2017 ATA guidelines, 2.5 mU/L was no longer used as the serum TSH upper limit cut-off value to diagnose hypothyroidism in early pregnancy, and 4.0 mU/L was recommended when internal or transferable pregnancy-specific TSH reference intervals were unavailable (5). Since the serum TSH upper limit in the American general population is usually 4.5 mU/L, it generally decreased by 0.5 mU/L in the first trimester, resulting in the cut-off value of 4.0 mU/L (6).
Although the 2017 ATA guidelines provided a convenient and feasible method for determining the serum TSH upper limit in early pregnancy, whether 4.0 mU/L is suitable for pregnant Chinese women needs to be explored. First, serum TSH reference ranges vary among different ethnicities due to cultural, environmental, geographic and genetic factors (21–23). Second, sex differences exist in TSH circadian rhythms. Third, serum TSH values change throughout the 24-h cycle and progressively increase with age (12). Fourth, iodine is the main ingredient in the synthesis of thyroid hormones. Since the implementation of mandatory universal salt iodization in 1996, China has eliminated iodine deficiency and become an iodine-sufficient country (24). Epidemiological studies also found that the resident's average serum TSH level has risen due to the effects of increased iodine intake (25). A similar epidemiological survey reported by Korea showed that there was high iodine intake in Korea, resulting in serum TSH exhibiting a right-shifted distribution in that population (26).
TSH is regarded as one of the principal indicators to diagnose primary hyperthyroidism and hypothyroidism. Our study compared the gestational upper and lower limits for serum TSH with the non-pregnant reference intervals provided by the test manufacturers. We found that regardless the kind of kit or test method, the serum TSH upper limit decreased by ~22% and the lower limit decreased by ~85% in early pregnancy. What we found especially interesting was that the non-pregnant upper limit declined by 22% was very close to 4.0 mU/L. However, the difference between 4.0 mU/L and the non-pregnant TSH upper limit minus 0.5 mU/L, according to the 2017 ATA guideline's recommendation (5), was obvious. Although the difference between 4.0 mU/L and the real TSH upper limits of pregnant Chinese women cannot be eliminated, the difference was not significant. Our findings further suggest that if we use 4.0 mU/L as a sub-optimal approach to identify serum TSH upper limit in early pregnancy, this limit represents a relative descent rate in the non-pregnant TSH upper reference limit of 22% rather than a reduction of ~0.5 mU/L.
However, we must stress that the population of a local institute or laboratory and pregnancy-specific serum TSH reference ranges should optimally define the gestational-specific serum TSH reference range. If unavailable, pregnancy-specific TSH reference ranges obtained from similar patient populations and detected by similar test assays should be the alternatives. If the above two conditions are not available, 4.0 mU/L or the serum TSH upper limit, which is 22% lower than the non-pregnant level, may be used as a sub-optimal approach to identify the serum TSH reference ranges in pregnancy for diagnosing gestational thyroid diseases.
T4 is considered an important index for the diagnosis of overt gestational hypothyroidism and hypothyroxinemia. At present, serum FT4 is used as a diagnostic indicator for hypothyroidism and hypothyroxinemia in the majority of clinical laboratories. The 2017 ATA guidelines declared that the accuracy of detecting serum FT4 by indirect analog immunoassays was influenced by pregnancy and manufacturer diversity. Gestational- and method-specific serum FT4 reference ranges should be established, but they are difficult to implement (5). According to the studies we included, serum FT4 showed an upward trend in the first trimester compared to non-pregnant levels. The upper limit fluctuated slightly, while the lower limit increased by ~7.0%. Serum FT4 decreased in the second trimester, with the upper limit decreasing by ~20% and the lower limit decreasing by ~15%. Subsequently, serum FT4 declined more profoundly in the third trimester, with the upper limit decreasing by ~25% and the lower limit decreasing by ~20%. Thus, by comparing with the non-pregnant reference ranges provided by manufacturers or measurements in the local population, we can diagnose hypothyroxinemia once the serum FT4 lower limit decreases by more than 15% in middle pregnancy and 20% in late pregnancy.
Our analysis of the included studies found that the gestational TSH reference ranges are broader than those of the non-pregnant population, mainly because the serum TSH upper limit decreased less than the lower limit. One possible explanation for this phenomenon is that women with subclinical hypothyroidism have an impaired thyroidal response to hCG stimulation, and women with a lower thyroid functional capacity may already have high-normal TSH concentrations going into pregnancy (27). So, in the whole population, the TSH upper limit probably does not decrease steeply. The upper and lower limit of serum FT4 almost synchronously declined in pregnancy, resulting in no obvious change in the breadth of the reference range.
Our study had some limitations. We only included the studies from China, without considering other countries or ethnic groups. Our study represented the serum TSH and FT4 reference ranges of a pregnant Chinese population; due to the paucity of studies calculating good population-based reference ranges for non-pregnancy, we did not acquire accurate normal TSH and FT4 reference ranges, which can be seen as the gold standard for comparison (8). In addition, our meta-analysis only included kits published and meeting inclusion criteria. Kits such as the Bayer ASC 180, LIAISON, and TOSOH were not included because of few or no publications; A minimum of approximately 400 women is required, due to the high interindividual variability and skewness for TSH but also to some extent FT4 (9). In our meta-analysis, the number of women included in most of the studies was lower than 400.
Our meta-analysis found that serum TSH decreased in the first and second trimesters of pregnancy and exhibited an upward trend to non-pregnant levels in the third trimester. Furthermore, serum FT4 increased slightly in the first trimester and decreased gradually in the second and third trimesters. The relative descent or ascent rate compared with the non-pregnant reference intervals may explain the change rules of gestational serum TSH and FT4. In the first trimester, using 4.0 mU/L as the cut-off point of the serum TSH upper limit is a sub-optimal approach for pregnant Chinese women. Generally, this limit represents a relative descent rate in the non-pregnant TSH upper reference limit of 22%.
XG and YL: Conceived and designed the meta-analysis; XG, JL, and AL: Performed the meta-analysis; XG: Analyzed the data, wrote the manuscript, statistical analyses and paper writing; WS: Contributed material/analysis tools; XG and YL: Reference collection and data management; XG, ZS, and WT: Study design.
This work was supported by Chinese National Natural Science Foundation (grant number 81570709, grant number 81170730); The National Science and technology support program (grant number 2014BAI06B02); Research Foundation of Key laboratory of endocrine diseases, Department of education in Liaoning Province China (grant number LZ2014035); Key platform foundation of science and technology for the universities in Liaoning Province (grant number 16010) and Health and Medicine Research Foundation, Shenyang City (grant number 17-230-9-02).
The authors declare that the research was conducted in the absence of any commercial or financial relationships that could be construed as a potential conflict of interest.
We gratefully acknowledge Haoyu Wang, Xun Gong, Qingling Guo, Yuanyuan Hou for providing technical assistance to the meta-analysis.
The Supplementary Material for this article can be found online at: https://www.frontiersin.org/articles/10.3389/fendo.2018.00432/full#supplementary-material
1. Huang SA, Neves LADC. Thyroid Hormone Metabolism: Dekker, New York, NY: Chemical Rubber Company Press (1986).
2. Glinoer D. What happens to the normal thyroid during pregnancy? Thyroid Offic J Am Thyr Assoc. (1999) 9:631.
3. Glinoer D. The regulation of thyroid function in pregnancy: pathways of endocrine adaptation from physiology to pathology. Endocr Rev. (1997) 18:404. doi: 10.1210/edrv.18.3.0300
4. StagnaroGreen A, Abalovich M, Alexander E, Azizi F, Mestman J, Negro R, et al. Guidelines of the American thyroid association for the diagnosis and management of thyroid disease during pregnancy and postpartum. Thyroid (2011) 21:1081. doi: 10.1089/thy.2011.0087
5. Alexander EK, Pearce EN, Brent GA, Brown RS, Chen H, Dosiou C, et al. 2017 Guidelines of the American Thyroid Association for the diagnosis and management of thyroid disease during pregnancy and the postpartum. Thyroid (2017) 27:315–89. doi: 10.1089/thy.2016.0457
6. Demers LM, Spencer CA. Laboratory medicine practice guidelines: laboratory support for the diagnosis and monitoring of thyroid disease. Clin Endocrinol. (2003) 58:138–40. doi: 10.1046/j.1365-2265.2003.01681.x
7. Springer D, Bartos V, Zima T. Reference intervals for thyroid markers in early pregnancy determined by 7 different analytical systems. Scand J Clin Lab Invest. (2014) 74:95–101. doi: 10.3109/00365513.2013.860617
8. Korevaar TIM, Chaker L, Peeters RP. Improving the clinical impact of randomised trials in thyroidology. Lancet Diabet Endocrinol. (2017) 6:523–5. doi: 10.1016/S2213-8587(17)30316-9
9. Medici M, Korevaar TI, Visser WE, Visser TJ, Peeters RP. Thyroid function in pregnancy: what is normal? Clin Chem (2015) 61:704–13. doi: 10.1373/clinchem.2014.236646
10. Stang A. Critical evaluation of the Newcastle-Ottawa scale for the assessment of the quality of nonrandomized studies in meta-analyses. Eur J Epidemiol. (2010) 25:603–5. doi: 10.1007/s10654-010-9491-z
11. Li C, Shan Z, Mao J, Wang W, Xie X, Zhou W, et al. Assessment of thyroid function during first-trimester pregnancy: what is the rational upper limit of serum TSH during the first trimester in Chinese pregnant women? J Clin Endocrinol Metab. (2014) 99:73–9. doi: 10.1210/jc.2013-1674
12. Ehrenkranz J, Bach PR, Snow GL, Schneider A, Lee JL, Ilstrup S, et al. Circadian and circannual rhythms in thyroid hormones: determining the TSH and free T4 reference intervals based upon time of day, age, and sex. Thyroid (2015) 25:954–61. doi: 10.1089/thy.2014.0589
13. Carr SR. Medical complications during pregnancy. JAMA (1995) 274:1247. doi: 10.1001/jama.1995.03530150071041
15. Benvenga S, Di Bari F, Granese R, Borrielli I, Giorgianni G, Grasso L, et al. Circulating thyrotropin is upregulated by estradiol. J Clin Transl Endocrinol. (2018) 11:11–7. doi: 10.1016/j.jcte.2018.02.002
16. Maji R, Nath S, Lahiri S, Das MS, Bhattacharyya AR, Das HN. Establishment of trimester-specific reference intervals of serum TSH & fT4 in a Pregnant Indian population at North Kolkata. Indian J Clin Biochem. (2014) 29:167–73. doi: 10.1007/s12291-013-0332-1
17. Moon HW, Chung HJ, Park CM, Hur M, Yun YM. Establishment of trimester-specific reference intervals for thyroid hormones in Korean pregnant women. Ann Lab Med. (2015) 35:198–204. doi: 10.3343/alm.2015.35.2.198
18. Bestwick JP, John R, Maina A, Guaraldo V, Joomun M, Wald NJ, et al. Thyroid stimulating hormone and free thyroxine in pregnancy: expressing concentrations as multiples of the median (MoMs). Clin Chim Acta (2014) 430:33–7. doi: 10.1016/j.cca.2013.12.030
19. Tuazon MA, van Raaij JM, Hautvast JG, Barba CV. Energy requirements of pregnancy in the Philippines. Lancet (1987) 2:1129–31.
20. Bocos-Terraz JP, Izquierdo-Álvarez S, Bancalero-Flores JL, Álvarez-Lahuerta R, Aznar-Sauca A, Real-López E, et al. Thyroid hormones according to gestational age in pregnant Spanish women. BMC Res Notes (2009) 2:1–9. doi: 10.1186/1756-0500-2-237
21. Price A, Obel O, Cresswell J, Catch I, Rutter S, Barik S, et al. Comparison of thyroid function in pregnant and non-pregnant Asian and western Caucasian women. Clin Chim Acta (2001) 308:91–8. doi: 10.1016/S0009-8981(01)00470-3
22. Surks MI, Boucai L. Age- and race-based serum thyrotropin reference limits. J Clin Endocrinol Metab. (2010) 95:496. doi: 10.1210/jc.2009-1845
23. Korevaar TI, Medici M, de Rijke YB, Visser W, Sm KS, Jaddoe VW, et al. Ethnic differences in maternal thyroid parameters during pregnancy: the Generation R study. J Clin Endocrinol Metab. (2013) 98:3678–86. doi: 10.1210/jc.2013-2005
24. Shan Z, Chen L, Lian X, Liu C, Shi B, Shi L, et al. Iodine status and prevalence of thyroid disorders after introduction of mandatory universal salt iodization for 16 years in china: a cross-sectional study in 10 cities. Thyroid (2016) 26:1125. doi: 10.1089/thy.2015.0613
25. Liu P, Liu L, Shen H, Jia Q, Wang J, Zheng H, et al. The standard, intervention measures and health risk for high water iodine areas. PLoS ONE (2014) 9:e89608. doi: 10.1371/journal.pone.0089608
26. Jeon M, Kim WG, Kwon H, Kim M, Park S, Oh HS, et al. Excessive iodine intake and TSH reference interval: data from the Korean National Health and Nutrition Examination Survey. Thyroid (2017) 27:967–72. doi: 10.1089/thy.2017.0078
27. Korevaar TI, Steegers EA, de Rijke YB, Visser WE, Jaddoe VW, Visser TJ, et al. Placental angiogenic factors are associated with maternal thyroid function and modify hCG-mediated FT4 stimulation. J Clin Endocrinol Metab (2015) 100:E1328–34. doi: 10.1210/jc.2015-2553
28. Liu J, Yu X, Xia M, Cai H, Cheng G, Wu L, et al. Development of gestation-specific reference intervals for thyroid hormones in normal pregnant Northeast Chinese women: What is the rational division of gestation stages for establishing reference intervals for pregnancy women? Clin Biochem (2017) 50:309–17. doi: 10.1016/j.clinbiochem.2016.11.036
29. Wang Q, Yu B, Huang R, Cao F, Zhu Z, Sun D, et al. Assessment of thyroid function during pregnancy: the advantage of self-sequential longitudinal reference intervals. Arch Med Sci Ams (2011) 7:679–84. doi: 10.5114/aoms.2011.24139
30. Xu SJ, Fan JX, Yang S, Tao J, Qian W, Han M, et al. Variations in different thyroid stimulating hormone and free thyroxine detection kits for evaluating thyroid function during pregnancy. Chinese J Perinat Med. (2015) 18:81–6. doi: 10.3760/cma.j.issn.1007-9408.2015.02.001
31. Duan Y, Peng L, Cui Y, Jiang Y. Reference Intervals for Thyroid Function and the Negative Correlation between FT4 and HbA1c in Pregnant Women of West China. Clin Lab. (2015) 61:777–83. doi: 10.7754/Clin.Lab.2015.141235
32. Fan JX, Han M, Tao J, Luo J, Song MF, Yang S, et al. Reference intervals for common thyroid function tests, during different stages of pregnancy in Chinese women. Chin Med J. (2013) 126:2710–4. doi: 10.3760/cma.j.issn.0366-6999.20130767
33. Li J, Teng WP, Shan ZY, Li CY, Zhou WW, Gao B, et al. Gestational month-specific reference ranges for TSH and thyroxine in Han nationality women in iodine sufficient area of China. Chinese J Endocrinol Metab. (2008) 24:605–8. doi: 10.3321/j.issn:1000-6699.2008.06.004
34. Chen QQ, Wang ZC. Establishment of reference intervals of thyroid hormones in pregnant women for Ningbo city. Chinese J Gen Pract. (2016) 14:1013–6. doi: 10.16766/j.cnki.issn.1674-4152.2016.06.043
35. Chen L, Chang K, Pu XY, Luo SF, Peng ZY, Chen M, et al. Gestation-specific reference intervals for thyroid function tests and the clinical significance for thyroid function monitoring through different periods of pregnancy. Chinese J Lab Med. (2016) 39:511–5. doi: 10.3760/cma.j.issn.1009-9158.2016.07.010
36. Yan YQ, Dong ZL, Dong L, Wang FR, Yang XM, Jin XY, et al. Trimester- and method-specific reference intervals for thyroid tests in pregnant Chinese women: methodology, euthyroid definition and iodine status can influence the setting of reference intervals. Clin Endocrinol. (2011) 74:262–9. doi: 10.1111/j.1365-2265.2010.03910.x
Keywords: TSH, FT4, pregnancy, reference range, Chinese women
Citation: Gao X, Li Y, Li J, Liu A, Sun W, Teng W and Shan Z (2018) Gestational TSH and FT4 Reference Intervals in Chinese Women: A Systematic Review and Meta-Analysis. Front. Endocrinol. 9:432. doi: 10.3389/fendo.2018.00432
Received: 09 May 2018; Accepted: 13 July 2018;
Published: 03 August 2018.
Edited by:
Alex Stewart Stagnaro-Green, University of Illinois at Chicago, United StatesReviewed by:
Tim I. M. Korevaar, Erasmus Medical Center, Erasmus University Rotterdam, NetherlandsCopyright © 2018 Gao, Li, Li, Liu, Sun, Teng and Shan. This is an open-access article distributed under the terms of the Creative Commons Attribution License (CC BY). The use, distribution or reproduction in other forums is permitted, provided the original author(s) and the copyright owner(s) are credited and that the original publication in this journal is cited, in accordance with accepted academic practice. No use, distribution or reproduction is permitted which does not comply with these terms.
*Correspondence: Zhongyan Shan, c2hhbnpob25neWFuQG1lZG1haWwuY29tLmNu
Disclaimer: All claims expressed in this article are solely those of the authors and do not necessarily represent those of their affiliated organizations, or those of the publisher, the editors and the reviewers. Any product that may be evaluated in this article or claim that may be made by its manufacturer is not guaranteed or endorsed by the publisher.
Research integrity at Frontiers
Learn more about the work of our research integrity team to safeguard the quality of each article we publish.