- Department of Pharmacology, Faculty of Medicine, Kagawa University, Kagawa, Japan
The EMPA-REG OUTCOME study revealed that a sodium-glucose cotransporter 2 (SGLT2) inhibitor, empagliflozin, can remarkably reduce cardiovascular (CV) mortality and heart failure in patients with high-risk type 2 diabetes. Recently, the CANVAS program also showed that canagliflozin, another SGLT2 inhibitor, induces a lower risk of CV events. However, the precise mechanism by which an SGLT2 inhibitor elicits CV protective effects is still unclear. Possible sympathoinhibitory effects of SGLT2 inhibitor have been suggested, as significant blood pressure (BP) reduction, following treatment with an SGLT2 inhibitor, did not induce compensatory changes in heart rate (HR). We have begun to characterize the effects of SGLT2 inhibitor on BP and sympathetic nervous activity (SNA) in salt-treated obese and metabolic syndrome rats, who develop hypertension with an abnormal circadian rhythm of BP, a non-dipper type of hypertension, and do not exhibit a circadian rhythm of SNA. Treatment with SGLT2 inhibitors significantly decreased BP and normalized circadian rhythms of both BP and SNA, but did not change HR; this treatment was also associated with an increase in urinary sodium excretion. Taken together, these data suggest that an SGLT2 inhibitor decreases BP by normalizing the circadian rhythms of BP and SNA, which may be the source of its beneficial effects on CV outcome in high-risk patients with type 2 diabetes. In this review, we briefly summarize the effects of SGLT2 inhibitors on BP and HR, with a special emphasis on SNA.
Introduction
Sodium-glucose cotransporter 2 (SGLT2) is located at the S1 and S2 segments of the proximal tubule epithelium, which reabsorbs approximately 90% of filtered glucose (1). SGLT2 inhibitors induce glycosuria (2) and are widely used as antihyperglycemic agents in patients with type 2 diabetes (3). Recently, the EMPA-REG OUTCOME study demonstrated that treatment with empagliflozin, an SGLT2 inhibitor, significantly decreased the primary composite outcome of cardiovascular (CV) events, thereby reducing CV mortality by 38% (4). Further studies have shown that empagliflozin reduced heart failure hospitalization and CV death, with a consistent benefit in patients with and without baseline heart failure (5). The CANVAS program has also shown that canagliflozin, another SGLT2 inhibitor, lowers the risk of CV events by providing renal protection in type 2 diabetes patients (6). Moreover, in the large, multinational CVD-REAL study, treatment with an SGLT2 inhibitor was associated with lower rates of hospitalization for heart failure and death, compared with other glucose lowering drugs, implying CV benefits from SGLT2 inhibitor usage (7). The underlying mechanism by which an SGLT2 inhibitor improves CV disease is not clear; however, which the mechanism may not be limited to effects on metabolic parameters, body weight, and blood pressure (BP) (4).
There were close links and interactions between sympathetic nervous activity (SNA) and metabolic syndrome (8). And patients with obesity, hypertension, or diabetes exhibit high CV risk, which is associated with an inappropriate augmentation of SNA (9). A systematic meta-analysis revealed that SGLT2 inhibitors decrease systolic blood pressure (SBP) and diastolic blood pressure (DBP) from baseline (−4.0 mmHg, and −1.6 mmHg, respectively) (2). However, clinical trials have failed to show notable changes or compensatory increases in heart rate (HR), following the administration of SGLT2 inhibitors (2, 10). These data suggest a possible sympathoinhibitory effect from an SGLT2 inhibitor, which may contribute in part to the cardioprotective effects of SGLT2 inhibitor therapy. In this review, we briefly summarize the effects of SGLT2 inhibitors on BP and HR in patients with type 2 diabetes. We also discuss the hypothesis that SGLT2 inhibitors elicit SNA inhibition.
Effects of SGLT2 Inhibitors on BP
Most clinical studies have shown that treatment with SGLT2 inhibitors, either as mono- or add-on therapies, significantly decreases both SBP and DBP in patients with type 2 diabetes (Table 1); however, some studies have shown no notable change in DBP (3, 23). Meta-analyses have revealed that SGLT2 inhibitors induce statistically significant reductions in SBP and DBP (2, 39). And Reed et al. (10) showed reasonable explanation of BP-lowering effects of SGLT2 inhibitors in type 2 diabetes. Interestingly, the extent of antihypertensive efficacy for each SGLT2 inhibitor differs according to patient background. For example, in a study of 1,031 type 2 diabetic patients who were divided into 5 groups based on body mass index (BMI, kg/m3) level [low-to-medium (<22.5, n = 222); medium (22.5–24.9, n = 270); high-level 1 (25–27.4, n = 262); high-level 2 (27.5–29.9, n = 142); and very-high (≥30, n = 135)], treatment with luseogliflozin significantly decreased SBP and DBP, relative to baseline, in all groups. However, reductions in SBP and DBP were greater in groups with higher BMI levels (40), suggesting that an SGLT2 inhibitor effectively decreases BP in high BMI, type 2 diabetic patients. Another clinical trial with ipragliflozin (50 mg/day for 24 weeks) showed no significant change in BP in 50 patients with type 2 diabetes. However, in 23 patients with poorly controlled BP (SBP ≥ 140 mmHg and/or DBP ≥ 90 mmHg), treatment with ipragliflozin significantly reduced SBP and DBP (−6.6 mmHg and, −3.0 mmHg, respectively) (41). Similarly, treatment with empagliflozin for 12 weeks resulted in a greater BP reduction in hypertensive patients with type 2 diabetic who exhibited higher baseline BP (17). Taken together, these results suggest that SGLT2 inhibitors are effective for BP reduction in poorly controlled hypertensive patients with type 2 diabetes.
Effects of SGLT2 Inhibitors on Dipping Pattern of BP
The restoration and maintaining a normal circadian rhythm is crucial to CV health (42). Diminished nocturnal decline in BP has been established as an important determinant for CV risk, independent of overall BP during a 24-h period (43). We have recently shown that SGLT2 inhibitors improve disrupted circadian rhythms of BP in metabolic syndrome rats [SHR/NDmcr-cp(+/+) rats; SHRcp] (37) and salt-treated obese Otsuka Long Evans Tokushima Fatty (OLETF) rats (44), both of which show non-dipper type of hypertension. Rahman et al. (37) showed a significant BP-lowering effect from luseogliflozin therapy in SHRcp rats. Interestingly, significant differences in BP levels appeared between dark and light periods, following treatment with an SGLT2 inhibitor, suggesting that the SGLT2 inhibitor altered the circadian rhythm of SBP, from a non-dipper type to a dipper type. Similar effects were reported by Takeshige et al. (44) in salt-treated obese OLETF rats, following use of another SGLT2 inhibitor, empagliflozin. In these obese animals, high salt treatment increased BP and abolished differences in BP between dark and light periods, suggesting a non-dipper type of hypertension. Treatment with empagliflozin prevented the development of salt-induced hypertension and reversed their circadian rhythm of BP, from a non-dipper pattern to a dipper pattern. In SHRcp (37) and salt-treated obese rats (44), SGLT2 inhibitor-induced normalization of disrupted circadian rhythm of BP was associated with increased urinary excretion of sodium. Overall, these data suggest that an SGLT2 inhibitor induces natriuresis, which plays an important role in the improvement of the circadian rhythm of BP in type 2 diabetes (45).
Recently, a clinical case study examined the effect of dapagliflozin (5 mg/day) in patients with type 2 diabetes who exhibited a non-dipper type (sleep-time mean SBP > 90% of awake-time mean) of hypertension. Administration of dapagliflozin significantly decreased BP and altered the circadian dipping pattern of BP, from a non-dipper type to a dipper type (sleep-time mean SBP ≤ 90% of awake-time mean) (46). Another empagliflozin clinical trial also revealed that the reduction in BP was greater during sleep-time, than during wake-time, in type 2 diabetes patients with non-dipper hypertension (47). These data indicate that BP reduction by an SGLT2 inhibitor is associated with restoration of a disrupted circadian rhythm of BP, from a non-dipper pattern to a dipper pattern, in hypertensive patients with type 2 diabetes.
Effects of SGLT2 Inhibitors on HR
As shown in Table 1, many clinical studies have investigated the effects of SGLT2 inhibitors on BP and HR in patients with type 2 diabetes. Many clinical trials have shown that SGLT2 inhibitors significantly decrease BP in patients with type 2 diabetes; however, no study has reported any meaningful change or compensatory increase in HR. We have also recently monitored BP and HR, using a telemetry system, in hypertensive animals. We found that luseogliflozin significantly decreased BP, but did not change HR, in SHRcp rats (37). Recently, Sano et al. (48) reviewed clinical data regarding luseogliflozin treatment in Japanese patients with type 2 diabetes; their report showed that luseogliflozin significantly decreased HR in patients with high baseline HR levels (≥ 70/min before treatment). The authors of that study hypothesize that reduction in HR, by treatment with an SGLT2 inhibitor, is induced by the sympathoinhibitory effect of an SGLT2 inhibitor, in these patients.
Effects of SGLT2 Inhibitors on SNA
As discussed above, both clinical and animal studies indicate that SGLT2 inhibitors decrease BP without changing HR. The absence of HR changes, along with the reduction in BP, supports the notion that SGLT2 inhibitors elicit inhibitory effects on SNA; importantly, SNA strongly correlates with CV mortality (49). Previous studies have revealed that an SGLT2 inhibitor decreases SNA: Chiba et al. (50) showed that acute administration of dapagliflozin significantly suppressed norepinephrine turnover in brown adipose tissue of mice, which reflects SNA in brown adipose tissue. Further, Yoshikawa et al. (51) assessed the effects of ipragliflozin on arterial pressure and low frequency (LF, 0.04–0.60 Hz) of systolic arterial pressure, which reflects the level of sympathetic vasoconstrictor activity, in diabetes mellitus rats; their study demonstrated that inhibition of SGLT2 attenuated the arterial pressure lability associated with sympathoinhibition during the working period. Matthews et al. (52) concluded that SNA was upregulated in obesity and type 2 diabetes, and showed that dapagliflozin reduced SNA markers, such as tyrosine hydroxylase and noradrenaline, in the kidney and heart of C57BL6/J mice; these markers were routinely elevated by high-fat diet treatment. A rising in muscle SNA is usual during hypovolemia, like diuretic effects (53). Jordan et al. (54) demonstrated that there was no significant changes in muscle SNA despite increases in urine volume after short-term treatment of empagliflozin in type 2 diabetes, which suggested a possible inhibitory effects of SGLT2 inhibitor on SNA. However, Kusaka et al. (55) utilized a telemetry system to show that empagliflozin did not elicit significant changes in averaged 24-h SBP, DBP, or HR in SHRcp rats. They also measured LF (0.25–0.75 Hz) of SBP, and showed that treatment with empagliflozin did not alter LF of SBP, or its circadian rhythm, in those animals. Recently, Rahman et al. (37) showed that treatment with luseogliflozin tended to decrease the LF of SBP in SHRcp rats, but these were not statistically significant changes. However, when the LF of SBP was separately analyzed during dark (working) and light (sleeping) time periods, the investigators found that luseogliflozin significantly decreased LF of SBP only during the sleeping period, but not during the working period.
To confirm whether the sympathoinhibitory effect of an SGLT2 inhibitor is dependent on its class-effect or drug-effect, similar experiments were performed to examine the effects of another SGLT2 inhibitor, empagliflozin, in obese OLETF rats. Twenty male OLETF rats (13 weeks old) were implanted with radiotelemetry devices. After 2 weeks of acclimatization, animals were treated with vehicle (0.5% carboxymethylcellulose, n = 7), high salt (1% NaCl in drinking water, n = 5), or high salt plus empagliflozin (10 mg/kg per day, n = 8), for 5 weeks. We analyzed the 24-h SBP (Figure 1) and LF (0.25–0.75 Hz) of SBP (Figure 2), respectively; we found that high salt treatment significantly increased 24-h SBP, while empagliflozin inhibited this salt-induced increase in SBP (Figures 2A,B). Interestingly, differences in BP between dark and light periods were not observed in high salt-treated obese animals, suggesting a lack of circadian rhythm of BP in these animals. However, obvious circadian rhythms of SBP appeared upon administration of empagliflozin to high salt-treated obese rats (Figures 1C,D). Conversely, empagliflozin did not change HR (data not shown). Empagliflozin also tended to decrease the 24-h averaged LF of SBP; however, differences among the groups were not statistically significant (Figures 2A,B). Further, empagliflozin significantly decreased LF of SBP only during the sleeping period, and differences between working and sleeping periods were elevated. Consequently, circadian rhythms in the LF of SBP were quite clear after empagliflozin administration in high salt-treated obese rats (Figures 2C,D). These results support the hypothesis that inhibition of SGLT2 improves the circadian rhythm of SNA through its sympathoinhibitory class-effect during the sleeping period.
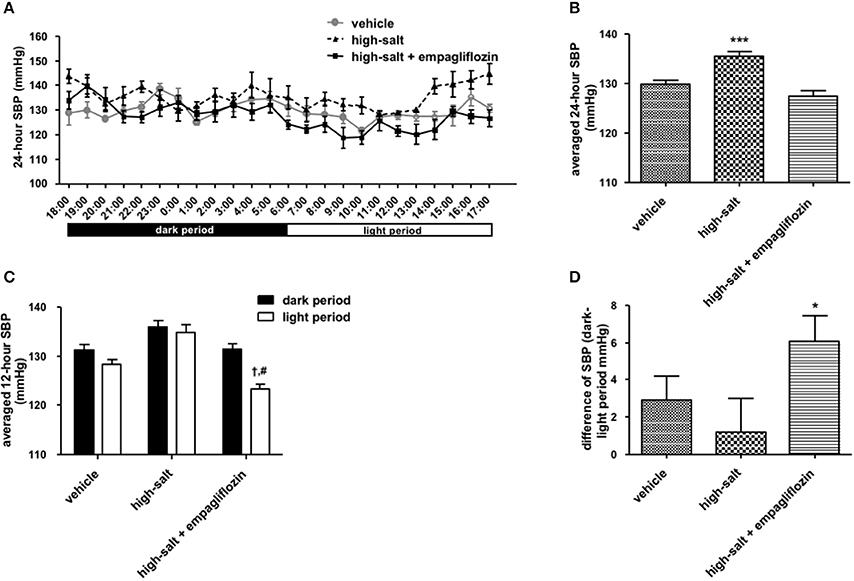
Figure 1. Effects of empagliflozin treatment on systolic blood pressure (SBP), and on circadian rhythm of SBP, in Otsuka Long Evans Tokushima Fatty (OLETF) rats. (A) 24-h SBP. (B) Average of 24-h SBP. (C) SBP in dark and light periods. (D) Differences between dark and light period in SBP. OLETF rats were treated with vehicle (vehicle, n = 7), 1% NaCl drinking water (high-salt, n = 5), or 1% NaCl drinking water and empagliflozin (high-salt + empagliflozin, n = 8), for 5 weeks. Values are mean ± SEM. ***P < 0.0001 vs. vehicle and high-salt + empagliflozin (one-way analysis of variance followed by Tukey's multiple comparison test), †P < 0.0001 vs. high-salt + empagliflozin dark period (2-way analysis), #P < 0.0001 vs. high-salt light period (t-test), *P < 0.05 vs. high-salt (t-test).
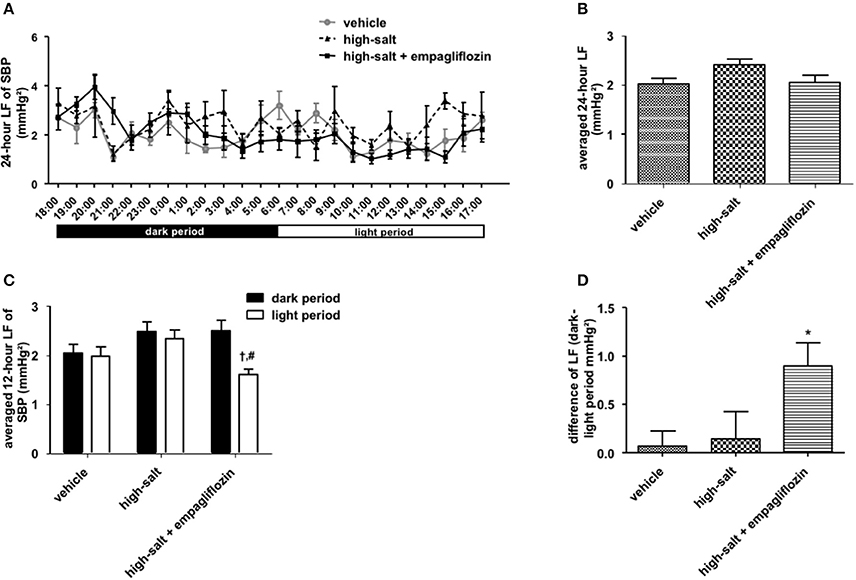
Figure 2. Effects of empagliflozin treatment on low frequency (LF) of systolic blood pressure (SBP), and on circadian rhythm of LF of SBP, in Otsuka Long Evans Tokushima Fatty (OLETF) rats. (A) 24-h LF of SBP. (B) Average of 24-h LF of SBP. (C) LF of SBP in dark and light period. (D) Differences between dark and light period in LF of SBP. OLETF rats were treated with vehicle (vehicle, n = 7), 1% NaCl drinking water (high-salt, n = 5), or 1% NaCl drinking water and empagliflozin (high-salt + empagliflozin, n = 8), for 5 weeks. Values are mean ± SEM. †P < 0.001 vs. high-salt + empagliflozin dark period (2-way analysis), #P < 0.001 vs. high-salt light period (t-test), *P < 0.05 vs. vehicle (one-way analysis of variance followed by Tukey's multiple comparison test).
Conclusions
Here, we have summarized clinical data regarding the effects of SGLT2 inhibitors on BP and HR in patients with type 2 diabetes. During treatment with an SGLT2 inhibitor, BP reduction is not accompanied by compensatory increases or notable changes in HR. Further, SGLT2 inhibitors exhibit beneficial influences on the circadian rhythms of BP and SNA. Thus, these effects of SGLT2 inhibitors may be important in their CV protective effects, as shown in the EMPA-REG OUTCOME and CANVAS programs (4–6). The precise mechanism by which an SGLT2 inhibitor normalizes disrupted circadian rhythms of BP and SNA is not clear; however, multiple processes may be involved, including reduction of blood glucose level and body weight, improvement of insulin resistance, and initiation of natriuresis (8, 56–60) (Figure 3). Further studies are necessary to determine the mechanism responsible for the effects of SGLT2 inhibitors on SNA.
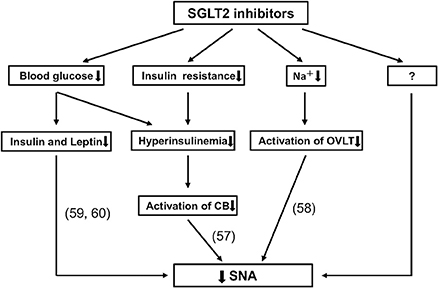
Figure 3. Possible mechanisms for reducing sympathetic nervous activity (SNA) through use of sodium-glucose cotransporter 2 (SGLT2) inhibitors. Recent studies have suggested that SGLT2 inhibitors elicit a reduction in SNA by decreasing insulin, leptin (59, 60) and blood glucose levels; and by improving insulin resistance and hyperinsulinemia, which could reduce the activation of carotid body (CB) (57); as well as by reducing sodium volume, which inhibits the activation of organum vasculosum laminae terminalis (OVLT) (58). Importantly, there are likely to be other mechanisms that have not been described.
Author Contributions
NW and AN analyzed previous clinical data. NW and AR performed the animal experiments and analyzed all experimental data. NW, HH, and AN wrote the manuscript. AN and HH supervised the study and revised the manuscript. All authors have read and approved the final manuscript.
Conflict of Interest Statement
Empagliflozin was provided by Boehringer Ingelheim Co., Ltd. (to AN). This is a collaborative study, in part, with Boehringer Ingelheim Co., Ltd.
Acknowledgments
This work was supported by the Hoansha Foundation (to AN), Alumni Association of Kagawa University Medical School (Sanjukai to AN), and the Naito Foundation (to AN). We thank Ryan Chastain-Gross, Ph.D., from Edanz Group (www.edanzediting.com/ac) for editing a draft of this manuscript.
References
1. Vallon V, Thomson SC. Targeting renal glucose reabsorption to treat hyperglycaemia: the pleiotropic effects of SGLT2 inhibition. Diabetologia (2017) 60:215–25. doi: 10.1007/s00125-016-4157-3
2. Baker WL, Smyth LR, Riche DM, Bourret EM, Chamberlin KW, White WB. Effects of sodium-glucose co-transporter 2 inhibitors on blood pressure: a systematic review and meta-analysis. J Am Soc Hypertens (2014) 8:L262–75.e9. doi: 10.1016/j.jash.2014.01.007
3. List JF, Whaley JM. Glucose dynamics and mechanistic implications of SGLT2 inhibitors in animals and humans. Kidney Int Suppl. (2011) 79:S20–7. doi: 10.1038/ki.2010.512
4. Abdul-Ghani M, Del Prato S, Chilton R, DeFronzo RA. SGLT2 inhibitors and cardiovascular risk: lessons learned from the EMPA-REG OUTCOME study. Diabetes Care (2016) 39:L717–25. doi: 10.2337/dc16-0041
5. Fitchett D, Zinman B, Wanner C, Lachin JM, Hantel S, Salsali A, et al. Heart failure outcomes with empagliflozin in patients with type 2 diabetes at high cardiovascular risk: results of the EMPA-REG OUTCOME(R) trial. Eur Heart J. (2016) 37:1526–34. doi: 10.1093/eurheartj/ehv728
6. Neal B, Perkovic V, Mahaffey KW, de Zeeuw D, Fulcher G, Erondu N, et al. Canagliflozin and Cardiovascular and Renal Events in Type 2 Diabetes. N Engl J Med. (2017) 377:644–57. doi: 10.1056/NEJMoa1611925
7. Kosiborod M, Cavender MA, Fu AZ, Wilding JP, Khunti K, Holl RW, et al. Lower risk of heart failure and death in patients initiated on sodium-glucose cotransporter-2 inhibitors versus other glucose-lowering drugs: the CVD-REAL study (comparative effectiveness of cardiovascular outcomes in new users of sodium-glucose cotransporter-2 inhibitors). Circulation (2017) 136:249–59. doi: 10.1161/CIRCULATIONAHA.117.029190
8. Schlaich M, Straznicky N, Lambert E, Lambert G. Metabolic syndrome: a sympathetic disease? Lancet Diabetes Endocrinol. (2015) 3:148–57. doi: 10.1016/S2213-8587(14)70033-6
9. Masuo K, Rakugi H, Ogihara T, Esler MD, Lambert GW. Cardiovascular and renal complications of type 2 diabetes in obesity: role of sympathetic nerve activity and insulin resistance. Curr Diabetes Rev. (2010) 6:58–67. doi: 10.2174/157339910790909396
10. Reed JW. Impact of sodium-glucose cotransporter 2 inhibitors on blood pressure. Vascular Health Risk Manag. (2016) 12:393–405. doi: 10.2147/VHRM.S111991
11. Cherney DZ, Perkins BA, Soleymanlou N, Har R, Fagan N, Johansen OE, et al. The effect of empagliflozin on arterial stiffness and heart rate variability in subjects with uncomplicated type 1 diabetes mellitus. Cardiovasc Diabetol. (2014) 13:28. doi: 10.1186/1475-2840-13-28
12. Haring HU, Merker L, Seewaldt-Becker E, Weimer M, Meinicke T, Woerle HJ, et al. Empagliflozin as add-on to metformin plus sulfonylurea in patients with type 2 diabetes: a 24-week, randomized, double-blind, placebo-controlled trial. Diabetes Care (2013) 36:3396–404. doi: 10.2337/dc12-2673
13. Chilton R, Tikkanen I, Cannon CP, Crowe S, Woerle HJ, Broedl UC, et al. Effects of empagliflozin on blood pressure and markers of arterial stiffness and vascular resistance in patients with type 2 diabetes. Diabetes Obes Metab. (2015) 17:1180–93. doi: 10.1111/dom.12572
14. Kovacs CS, Seshiah V, Swallow R, Jones R, Rattunde H, Woerle HJ, et al. Empagliflozin improves glycaemic and weight control as add-on therapy to pioglitazone or pioglitazone plus metformin in patients with type 2 diabetes: a 24-week, randomized, placebo-controlled trial. Diabetes Obesity Metab. (2014) 16:147–58. doi: 10.1111/dom.12188
15. Nishimura R, Tanaka Y, Koiwai K, Inoue K, Hach T, Salsali A, et al. Effect of empagliflozin monotherapy on postprandial glucose and 24-hour glucose variability in Japanese patients with type 2 diabetes mellitus: a randomized, double-blind, placebo-controlled, 4-week study. Cardiovasc Diabetol. (2015) 14:11. doi: 10.1186/s12933-014-0169-9
16. Haring HU, Merker L, Seewaldt-Becker E, Weimer M, Meinicke T, Broedl UC, et al. Empagliflozin as add-on to metformin in patients with type 2 diabetes: a 24-week, randomized, double-blind, placebo-controlled trial. Diabetes Care (2014) 37:1650–9. doi: 10.2337/dc13-2105
17. Tikkanen I, Narko K, Zeller C, Green A, Salsali A, Broedl UC, et al. Empagliflozin reduces blood pressure in patients with type 2 diabetes and hypertension. Diabetes Care (2015) 38:420–8. doi: 10.2337/dc14-1096
18. Rosenstock J, Jelaska A, Zeller C, Kim G, Broedl UC, Woerle HJ. Impact of empagliflozin added on to basal insulin in type 2 diabetes inadequately controlled on basal insulin: a 78-week randomized, double-blind, placebo-controlled trial. Diabetes Obesity Metab. (2015) 17:936–48. doi: 10.1111/dom.12503
19. Rosenstock J, Jelaska A, Frappin G, Salsali A, Kim G, Woerle HJ, et al. Improved glucose control with weight loss, lower insulin doses, and no increased hypoglycemia with empagliflozin added to titrated multiple daily injections of insulin in obese inadequately controlled type 2 diabetes. Diabetes Care (2014) 37:1815–23. doi: 10.2337/dc13-3055
20. Ferrannini E, Berk A, Hantel S, Pinnetti S, Hach T, Woerle HJ, et al. Long-term safety and efficacy of empagliflozin, sitagliptin, and metformin: an active-controlled, parallel-group, randomized, 78-week open-label extension study in patients with type 2 diabetes. Diabetes Care (2013) 36:4015–21. doi: 10.2337/dc13-0663
21. Wilding JP, Woo V, Rohwedder K, Sugg J, Parikh S. Dapagliflozin in patients with type 2 diabetes receiving high doses of insulin: efficacy and safety over 2 years. Diabetes Obesity Metab. (2014) 16:124–36. doi: 10.1111/dom.12187
22. Nauck MA, Del Prato S, Meier JJ, Duran-Garcia S, Rohwedder K, Elze M, et al. Dapagliflozin versus glipizide as add-on therapy in patients with type 2 diabetes who have inadequate glycemic control with metformin: a randomized, 52-week, double-blind, active-controlled noninferiority trial. Diabetes Care (2011) 34:2015–22. doi: 10.2337/dc11-0606
23. List JF, Woo V, Morales E, Tang W, Fiedorek FT. Sodium-glucose cotransport inhibition with dapagliflozin in type 2 diabetes. Diabetes Care (2009) 32:650–7. doi: 10.2337/dc08-1863
24. Sjostrom CD, Johansson P, Ptaszynska A, List J, Johnsson E. Dapagliflozin lowers blood pressure in hypertensive and non-hypertensive patients with type 2 diabetes. Diabetes Vasc Dis Res. (2015) 12:352–8. doi: 10.1177/1479164115585298
25. Wilding JP, Woo V, Soler NG, Pahor A, Sugg J, Rohwedder K, et al. Long-term efficacy of dapagliflozin in patients with type 2 diabetes mellitus receiving high doses of insulin: a randomized trial. Ann Internal Med. (2012) 156:405–15. doi: 10.7326/0003-4819-156-6-201203200-00003
26. Cefalu WT, Leiter LA, Yoon KH, Arias P, Niskanen L, Xie J, et al. Efficacy and safety of canagliflozin versus glimepiride in patients with type 2 diabetes inadequately controlled with metformin (CANTATA-SU): 52 week results from a randomised, double-blind, phase 3 non-inferiority trial. Lancet (2013) 382:941–50. doi: 10.1016/S0140-6736(13)60683-2
27. Devineni D, Morrow L, Hompesch M, Skee D, Vandebosch A, Murphy J, et al. Canagliflozin improves glycaemic control over 28 days in subjects with type 2 diabetes not optimally controlled on insulin. Diabetes Obesity Metab. (2012) 14:539–45. doi: 10.1111/j.1463-1326.2012.01558.x
28. Rosenstock J, Aggarwal N, Polidori D, Zhao Y, Arbit D, Usiskin K, et al. Dose-ranging effects of canagliflozin, a sodium-glucose cotransporter 2 inhibitor, as add-on to metformin in subjects with type 2 diabetes. Diabetes Care (2012) 35:1232–8. doi: 10.2337/dc11-1926
29. Leiter LA, Yoon KH, Arias P, Langslet G, Xie J, Balis DA, et al. Canagliflozin provides durable glycemic improvements and body weight reduction over 104 weeks versus glimepiride in patients with type 2 diabetes on metformin: a randomized, double-blind, phase 3 study. Diabetes Care (2015) 38:355–64. doi: 10.2337/dc13-2762
30. Sha S, Devineni D, Ghosh A, Polidori D, Hompesch M, Arnolds S, et al. Pharmacodynamic effects of canagliflozin, a sodium glucose co-transporter 2 inhibitor, from a randomized study in patients with type 2 diabetes. PLoS ONE (2014) 9:e105638. doi: 10.1371/journal.pone.0105638
31. Lavalle-Gonzalez FJ, Januszewicz A, Davidson J, Tong C, Qiu R, Canovatchel W, et al. Efficacy and safety of canagliflozin compared with placebo and sitagliptin in patients with type 2 diabetes on background metformin monotherapy: a randomised trial. Diabetologia (2013) 56:2582–92. doi: 10.1007/s00125-013-3039-1
32. Stenlof K, Cefalu WT, Kim KA, Alba M, Usiskin K, Tong C, et al. Efficacy and safety of canagliflozin monotherapy in subjects with type 2 diabetes mellitus inadequately controlled with diet and exercise. Diabetes Obes Metab. (2013) 15:372–82. doi: 10.1111/dom.12054
33. Wilding JP, Charpentier G, Hollander P, Gonzalez-Galvez G, Mathieu C, Vercruysse F, et al. Efficacy and safety of canagliflozin in patients with type 2 diabetes mellitus inadequately controlled with metformin and sulphonylurea: a randomised trial. Int J Clin Pract (2013) 67:1267–82. doi: 10.1111/ijcp.12322
34. Schernthaner G, Gross JL, Rosenstock J, Guarisco M, Fu M, Yee J, et al. Canagliflozin compared with sitagliptin for patients with type 2 diabetes who do not have adequate glycemic control with metformin plus sulfonylurea: a 52-week randomized trial. Diabetes Care (2013) 36:2508–15. doi: 10.2337/dc12-2491
35. Forst T, Guthrie R, Goldenberg R, Yee J, Vijapurkar U, Meininger G, et al. Efficacy and safety of canagliflozin over 52 weeks in patients with type 2 diabetes on background metformin and pioglitazone. Diabetes Obesity Metab. (2014) 16:467–77. doi: 10.1111/dom.12273
36. Yale JF, Bakris G, Cariou B, Yue D, David-Neto E, Xi L, et al. Efficacy and safety of canagliflozin in subjects with type 2 diabetes and chronic kidney disease. Diabetes Obes Metab. (2013) 15:463–73. doi: 10.1111/dom.12090
37. Rahman A, Fujisawa Y, Nakano D, Hitomi H, Nishiyama A. Effect of a selective SGLT2 inhibitor, luseogliflozin, on circadian rhythm of sympathetic nervous function and locomotor activities in metabolic syndrome rats. J Clin Exp Pharmacol Physiol (2017) 44:522–5. doi: 10.1111/1440-1681.12725
38. Maegawa H, Tobe K, Tabuchi H, Nakamura I. Baseline characteristics and interim (3-month) efficacy and safety data from STELLA-LONG TERM, a long-term post-marketing surveillance study of ipragliflozin in Japanese patients with type 2 diabetes in real-world clinical practice. Expert Opin Pharmacother. (2016) 17:1985–94. doi: 10.1080/14656566.2016.1217994
39. Vasilakou D, Karagiannis T, Athanasiadou E, Mainou M, Liakos A, Bekiari E, et al. Sodium-glucose cotransporter 2 inhibitors for type 2 diabetes: a systematic review and meta-analysis. Ann Int Med. (2013) 159:262–74. doi: 10.7326/0003-4819-159-4-201308200-00007
40. Sakai S, Kaku K, Seino Y, Inagaki N, Haneda M, Sasaki T, et al. Efficacy and safety of the SGLT2 inhibitor luseogliflozin in Japanese patients with type 2 diabetes mellitus stratified according to baseline body mass index: pooled analysis of data from 52-week phase III trials. Clin Ther. (2016) 38:843–62.e9. doi: 10.1016/j.clinthera.2016.01.017
41. Ito D, Ikuma-Suwa E, Inoue K, Kaneko K, Yanagisawa M, Inukai K, et al. Effects of ipragliflozin on diabetic nephropathy and blood pressure in patients with type 2 diabetes: an open-label study. J Clin Med Res. (2017) 9:154–62. doi: 10.14740/jocmr2875w
42. Chen L, Yang G. Recent advances in circadian rhythms in cardiovascular system. Front Pharmacol. (2015) 6:71. doi: 10.3389/fphar.2015.00071
43. Ohkubo T, Hozawa A, Yamaguchi J, Kikuya M, Ohmori K, Michimata M, et al. Prognostic significance of the nocturnal decline in blood pressure in individuals with and without high 24-h blood pressure: the Ohasama study. J Hypertens. (2002) 20:2183–9. doi: 10.1097/00004872-200211000-00017
44. Takeshige Y, Fujisawa Y, Rahman A, Kittikulsuth W, Nakano D, Mori H, et al. A sodium-glucose co-transporter 2 inhibitor empagliflozin prevents abnormality of circadian rhythm of blood pressure in salt-treated obese rats. Hypertens Res. (2016) 39:415–22. doi: 10.1038/hr.2016.2
45. Rahman A, Hitomi H, Nishiyama A. Cardioprotective effects of SGLT2 inhibitors are possibly associated with normalization of the circadian rhythm of blood pressure. Hypertens Res. (2017) 40:535–40. doi: 10.1038/hr.2016.193
46. Mori H, Okada Y, Kawaguchi M, Tanaka Y. A case of type 2 diabetes with a change from a non-dipper to a dipper blood pressure pattern by dapagliflozin. J UOEH (2016) 38:149–53. doi: 10.7888/juoeh.38.149
47. Chilton R, Tikkanen I, Hehnke U, Woerle HJ, Johansen OE. Impact of empagliflozin on blood pressure in dipper and non-dipperpatients with type 2 diabetes mellitus and hypertension. Diabetes Obesity Metab. (2017) 19:1620–4. doi: 10.1111/dom.12962
48. Sano M. Hemodynamic effects of sodium-glucose cotransporter 2 inhibitors. J Clin Med Res. (2017) 9:457–60. doi: 10.14740/jocmr3011w
49. Wenzel RR, Spieker L, Qui S, Shaw S, Luscher TF, Noll G. I1-imidazoline agonist moxonidine decreases sympathetic nerve activity and blood pressure in hypertensives. Hypertension (1998) 32:1022–7.
50. Chiba Y, Yamada T, Tsukita S, Takahashi K, Munakata Y, Shirai Y, et al. Dapagliflozin, a sodium-glucose co-transporter 2 inhibitor, acutely reduces energy expenditure in BAT via neural signals in mice. PLoS ONE (2016) 11:e0150756. doi: 10.1371/journal.pone.0150756
51. Yoshikawa T, Kishi T, Shinohara K, Takesue K, Shibata R, Sonoda N, et al. Arterial pressure lability is improved by sodium-glucose cotransporter 2 inhibitor in streptozotocin-induced diabetic rats. Hypertens Res. (2017) 40:646–51. doi: 10.1038/hr.2017.14
52. Matthews VB, Elliot RH, Rudnicka C, Hricova J, Herat L, Schlaich MP. Role of the sympathetic nervous system in regulation of the sodium glucose cotransporter 2. J Hypertens (2017) 35:2059–68. doi: 10.1097/HJH.0000000000001434
53. Kimmerly DS, Shoemaker JK. Hypovolemia and neurovascular control during orthostatic stress. Am J Physiol. (2002) 282:H645–55. doi: 10.1152/ajpheart.00535.2001
54. Jordan J, Tank J, Heusser K, Heise T, Wanner C, Heer M, et al. The effect of empagliflozin on muscle sympathetic nerve activity in patients with type II diabetes mellitus. J Am Soc Hypertens. (2017) 11:604–12. doi: 10.1016/j.jash.2017.07.005
55. Kusaka H, Koibuchi N, Hasegawa Y, Ogawa H, Kim-Mitsuyama S. Empagliflozin lessened cardiac injury and reduced visceral adipocyte hypertrophy in prediabetic rats with metabolic syndrome. Cardiovasc Diabetol. (2016) 15:157. doi: 10.1186/s12933-016-0473-7
56. Heise T, Jordan J, Wanner C, Heer M, Macha S, Mattheus M, et al. Pharmacodynamic effects of single and multiple doses of empagliflozin in patients with type 2 diabetes. Clin Ther. (2016) 38:2265–76. doi: 10.1016/j.clinthera.2016.09.001
57. Conde SV, Sacramento JF, Guarino MP, Gonzalez C, Obeso A, Diogo LN, et al. Carotid body, insulin, and metabolic diseases: unraveling the links. Front Physiol. (2014) 5:418. doi: 10.3389/fphys.2014.00418
58. Guyenet PG. Putative mechanism of salt-dependent neurogenic hypertension: cell-autonomous activation of organum vasculosum laminae terminalis neurons by hypernatremia. Hypertension (2017) 69:20–22. doi: 10.1161/HYPERTENSIONAHA.116.08470
59. Rahmouni K. Leptin-induced sympathetic nerve activation: signaling mechanisms and cardiovascular consequences in obesity. Curr Hypertens Rev. (2010) 6:104–209. doi: 10.2174/157340210791170994
Keywords: sodium-glucose cotransporter 2 (SGLT2) inhibitor, EMPA-REG OUTCOME trial, CANVAS program, blood pressure, heart rate, sympathetic nervous activity
Citation: Wan N, Rahman A, Hitomi H and Nishiyama A (2018) The Effects of Sodium-Glucose Cotransporter 2 Inhibitors on Sympathetic Nervous Activity. Front. Endocrinol. 9:421. doi: 10.3389/fendo.2018.00421
Received: 12 March 2018; Accepted: 04 July 2018;
Published: 26 July 2018.
Edited by:
Åke Sjöholm, Gävle Hospital, SwedenReviewed by:
Markus Peter Schlaich, Baker Heart and Diabetes Institute, AustraliaShunsuke Kiuchi, Toho University Omori Medical Center, Japan
Copyright © 2018 Wan, Rahman, Hitomi and Nishiyama. This is an open-access article distributed under the terms of the Creative Commons Attribution License (CC BY). The use, distribution or reproduction in other forums is permitted, provided the original author(s) and the copyright owner(s) are credited and that the original publication in this journal is cited, in accordance with accepted academic practice. No use, distribution or reproduction is permitted which does not comply with these terms.
*Correspondence: Akira Nishiyama, akira@med.kagawa-u.ac.jp