- Department of Orthopedic Surgery, Shanghai Jiao Tong University Affiliated Sixth People's Hospital, Shanghai, China
Objective: Nonalcoholic fatty liver disease (NAFLD) is related to several inflammatory or metabolic diseases. However, findings of previous studies investigating the association between NAFLD and BMD are inconsistent. Only one study reported a potential association between NAFLD and osteoporotic fracture. This study investigated whether NAFLD in older participants (>55 years) was associated with osteoporotic fracture risk.
Materials and Methods: This cross-sectional, observational study included 2,695 participants (35.7% men, 614 cases of NAFLD, and 383 fractures). Standardized questionnaires, laboratory tests, and physical and ultrasonic examinations were completed.
Results: After adjusting for various factors including serum triglycerides (TG), high-density cholesterol (HDL-C), and low-density lipoprotein cholesterol (LDL-C), multivariate logistic regression models revealed a marginal association between NAFLD and osteoporotic fracture risk in men (odds ratio [OR], 1.86; 95% confidence interval [CI], 1.06–3.27; P = 0.030) but no association in women (OR, 1.05; 95% CI, 0.74–1.48; P = 0.800). Further stratified analyses showed a significant association between NAFLD and osteoporotic fracture risk in men without high TG, low HDL-C, and high LDL-C.
Conclusions: There was a significant association between NAFLD and osteoporotic fracture risk in older Chinese men, particularly men without dyslipidemia.
Introduction
Osteoporosis is a systemic skeletal disease characterized by bone loss and bone microstructure degradation that leads to low energy fractures (1, 2). The incidence of osteoporosis and osteoporotic fractures is increasing, resulting in increased morbidity, increased mortality, and heavy burdens on families in terms of social status and economic status (1, 2). The risk of osteoporotic fractures is associated with many factors, including metabolic factors, inflammatory state, disease status, lifestyle, and genetic factors (3–5). Recently, the relationship between inflammation, metabolism, and osteoporosis has been elucidated (6, 7), and risk factors for osteoporotic fracture such as subclinical thyroid dysfunction, metabolic syndrome, and insulin resistance have been further explored (8, 9).
Nonalcoholic fatty liver disease (NAFLD), typically presents as mild or heavy steatosis, is the most common pathological disorder of the liver. It is associated with end-stage liver disease and liver-related morbidity and mortality (10). NAFLD is considered to be a multisystem disease that may affect many extrahepatic organs, is associated with various metabolic abnormalities, and can influence and participate in multiple metabolic pathways (10). Moreover, NAFLD is associated with several risk factors that affect bone mineral density (BMD), such as chronic inflammatory disease, metabolic syndrome, cardiovascular disease, diabetes mellitus, and chronic kidney disease (10, 11–13). However, findings of previous studies investigating the association between NAFLD and osteopenia/osteoporosis are inconsistent (14–17).
To our knowledge, there is only one cross-sectional study that has investigated the association between NAFLD and risk of osteoporotic fractures in adults aged ≥40 years. After adjusting for age, smoking, alcohol intake, physical activity, metabolic risk factors, kidney function, and use of glucocorticoids or osteoporosis medications, this study found that NAFLD may significantly increase the odds ratio of osteoporotic fracture risk in men (14). However, this study included both middle-aged and elderly participants; therefore, confounding factors, such as menopause and BMD, may have affected the real association between NAFLD and risk of osteoporotic fractures.
Therefore, the objectives of this study were to investigate whether NAFLD in elderly participants (aged >55 years) was associated with osteoporotic fracture risk and to further examine whether other factors may affect this association.
Materials and Methods
Study Population and Design
This population-based, cross-sectional study recruited 3,657 participants (4,000 total participants and 343 dropouts) aged 55–85 years from the Shanghai metropolitan area in China between June 2014 and June 2016. This study was approved by the Ethics Committee of Shanghai Sixth People's Hospital, and all participants signed an informed consent form. The exclusion criteria were as follows: (1) history of hepatitis, liver cirrhosis, or any cancer or malignancy (n = 285); (2) history of kidney disease (n = 82); (3) regular treatment for osteoporosis (n = 192); (4) excessive alcohol consumption (≥140 g/week for men and ≥70 g/week for women; n = 311); and (5) absence of completed questionnaires (n = 128). Therefore, a total of 2,659 (950 men and 1,709 women) participants were included in the analysis (Figure S1). The study design was described in our previous article (15).
Participant Characteristics
Standardized self-administered questionnaires were used to collect participant characteristics such as demographic characteristics, lifestyle, disease history, and medication history. Chronic disease history, including cardiovascular disease, diabetes, hypertension, chronic kidney disease, dyslipidemia, and fractures, was also recorded. Smoking status was categorized as nonsmoker, current smoker, or ex-smoker. Alcohol consumption status was categorized as never, current (drinking within the past 6 months), or previous (cessation of more than 6 months). The type, frequency, and dose of alcohol consumption were collected and mean daily alcohol consumption was calculated. Physical activity was classified as <30 min per day, 30–60 min per day, 1–2 h per day, 2–4 h per day, 4–6 h per day, and >6 h per day.
Physical examination was performed according to a standardized protocol and by a trained inspector. Standing height and body weight were measured while participants wore lightweight clothing and no shoes. Systolic blood pressure and diastolic blood pressure were measured with an automatic sphygmomanometer after at least 5 min of seated rest. Body mass index (BMI), which was used as an index of body fat, was calculated as weight divided by height (kg/m2). Waist circumference was measured horizontally at the umbilicus level. Total hip BMD was measured by dual energy X-ray absorptiometry using a Lunar Prodigy GE densitometer (Lunar Corp, Madison, WI, USA).
Laboratory tests included triglycerides (TG), total cholesterol (TC), high-density lipoprotein cholesterol (HDL-C), and low-density lipoprotein cholesterol (LDL-C). All values were measured by an automated analyzer (Modular E170; F. Hoffmann-La Roche Ltd, Basel, Switzerland) using standard methods. Dyslipidemia was defined as having high TG (≥1.7 mmol/L), high TC (≥5.0 mmol/L), high LDL-C (≥2.6 mmol/L), and low HDL-C (≤0.9 mmol/L in men and ≤1.1 mmol/L in women) (16, 17).
Definition of NAFLD
NAFLD was diagnosed based on the results of a liver ultrasonic examination. Two ultrasonographers examined all participants and were blinded to their clinical information. Fatty liver disease was diagnosed when characteristics of increased diffuse echogenicity, ultrasound beam attenuation, and poor visualization of intrahepatic architecture were observed on ultrasound examination (18).
Assessment of Fractures
According to interviewer-assisted, standardized, self-administered questionnaires, the history of fractures was collected. Data regarding fracture sites and the age when the fracture occurred were also collected. In this study, osteoporotic fractures were defined as low-trauma fractures that occurred at age ≥45 years due to falling from a standing height or shorter, a trip or slip, or falling out of bed (fracture of the hip, wrist, spine) (19, 20). All pathologic (due to cancer, bone tuberculosis, etc.) and traumatic fractures (defined as a fracture that resulted from a fall from higher than a standing height or an accident due to a motor vehicle collision or sports, etc.) were excluded (19, 20). We verified the fracture based on the hospital diagnosis or previous radiographic data.
Statistical Analysis
In accordance with previous studies, we performed all analyses integrally and separately for men and women because sex may be an important influencing factor for the association between NAFLD and osteoporotic fracture. Continuous variables were presented as mean ± standard deviation, and categorical variables were presented as number and proportions. Comparisons between groups were performed using chi-squared tests for categorical variables, one-way analysis of variance (ANOVA) for normally distributed continuous variables, and the Kruskal Wallis test for skewed continuous variables. Odds ratios (OR) and corresponding 95% confidence intervals (CI) for the associations between NAFLD and osteoporotic fracture were calculated using logistic regression analyses. Both unadjusted and multivariate-adjusted logistic regression analyses were performed: model 1 was adjusted for age; model 2 was model 1 plus smoking status and alcohol status; model 3 was model 2 plus physical activity (<30 min/day, 30–60 min/day, >60 min/day); model 4 was model 3 plus diabetes, hypertension, cardiovascular events, and family history of fracture; model 5 was model 4 plus waist circumference, BMI, and dyslipidemia; and model 6 was model 5 plus TG, TC, HDL-C, and LDL-C. Two-sided P < 0.050 was considered significant. Statistical analyses were performed using PASW Statistics 18.0 software (SPSS Inc., Chicago, IL, USA), Empower (R) (www.empowerstats.com; X&Y Solutions Inc., Boston, MA, USA), and R packages (http://www.r-project.org; The R Foundation for Statistical Computing, Vienna, Austria).
Results
Participant Characteristics
Of the 2,659 participants included in the current analyses, 614 had NAFLD (226 men and 388 women) and 383 had fractures (96 men and 287 women). The clinical and laboratory characteristics, stratified by NAFLD status, are shown in Table 1. Compared to participants without NAFLD, participants with NAFLD had a significantly higher prevalence of fractures (13.30 vs. 18.08%; P = 0.003) and comorbidities, including hypertension (37.73 vs. 59.02%; P < 0.001), cardiovascular events (23.14 vs. 38.40%; P < 0.001), hypertension (39.40 vs. 62.70%; P < 0.001), and diabetes mellitus (13.43 vs. 24.14%; P < 0.001).
Men with NAFLD were younger (72.97 ± 5.63 vs. 74.71 ± 5.93 years; P < 0.001) than men without NAFLD. Men with NAFLD had a significantly larger waist circumference (90.39 ± 8.94 vs. 85.30 ± 8.79 cm; P < 0.001), significantly higher BMI (25.34 ± 2.68 vs. 23.45 ± 2.96 kg/m2; P < 0.001), serum TG (1.92 ± 1.04 vs. 1.57 ± 0.87 mmol/L; P < 0.001), and LDL-C (2.75 ± 0.74 vs. 2.64 ± 0.68 mmol/L; P = 0.030), and significantly lower high-density lipoprotein cholesterol (HDL-C) (1.34 ± 0.28 vs. 1.39 ± 0.36 mmol/L; P = 0.025) than men without NAFLD. Additionally, men with NAFLD had a higher frequency of fractures compared to men without NAFLD (14.60 vs. 8.70%; P = 0.010). Similarly, women with NAFLD had a significantly larger waist circumference (84.31 ± 9.21 vs. 80.73 ± 8.65 cm; P < 0.001), significantly higher BMI (25.20 ± 3.31 vs. 23.38 ± 3.62 kg/m2; P < 0.001) and TG (2.07 ± 1.17 vs. 1.71 ± 0.93 mmol/L; P < 0.001), and significantly lower HDL-C (1.47 ± 0.31 vs. 1.57 ± 0.40 mmol/L; P < 0.001) than women without NAFLD. Furthermore, there was a borderline difference in the prevalence of osteoporotic fractures in women with NAFLD compared with women without NAFLD (15.82 vs. 20.10%; P = 0.047).
Association Between NAFLD and Osteoporotic Fracture
Table 2 shows the association between NAFLD and osteoporotic fracture. For all participants, NAFLD was significantly associated with increased odds of osteoporotic fractures in the unadjusted model (OR, 1.45; 95% CI, 1.14–1.86; P = 0.003). However, after adjusting for age, smoking status, alcohol status, physical activity, diabetes, hypertension, cardiovascular events, family history of fracture, dyslipidemia, waist circumference, BMI, TG, TC, HDL-C, LDL-C, and BMD (model 7), the association was no longer significant (OR, 1.25; 95% CI, 0.93–1.68; P = 0.1365). A similar pattern of associations was also detected for women (unadjusted model: OR, 1.34; 95% CI, 1.00–1.79; P = 0.048; model 7: OR, 1.05; 95% CI, 0.74–1.48; P = 0.800).
Conversely, for men, NAFLD was significantly associated with increased odds of osteoporotic fractures in the unadjusted model (OR, 1.79; 95% CI, 1.14–2.82; P = 0.011) and marginally associated with increased odds of osteoporotic fracture risk in model 7 (OR, 1.86; 95% CI, 1.06–3.27; P = 0.030).
Association Between Serum Cholesterol Level and Fracture Type
Table 3 shows the association between NAFLD and osteoporotic fracture type. There was a significant association between NAFLD and lumbar fractures in all participants and in men alone, whereas no association was observed in women. Moreover, there were no significant associations between NAFLD and radius fractures, humerus fractures, or hip fractures in both men and women.
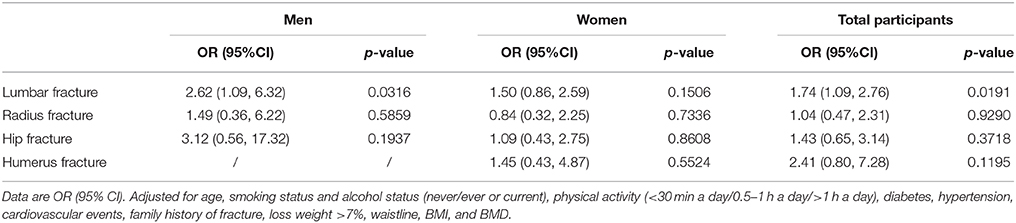
Table 3. Association between NAFLD and lumbar fracture, radius fracture, hip fracture and humerus fracture.
Stratified Analysis of the Association Between NAFLD and Osteoporotic Fractures for Different Dyslipidemia Statuses
We examined whether dyslipidemia status affected the association between NAFLD and osteoporotic fractures. As shown in Table 4, we observed a differential association between NAFLD and osteoporotic fractures between men with and without high TG, low HDL-C, and high LDL-C. After adjusting for age, smoking status, alcohol status, physical activity, diabetes, hypertension, cardiovascular events, family history of fractures, dyslipidemia, waist circumference, BMI, TG, TC, HDL-C, LDL-C, and BMD, no significant interactive effects were detected (interaction P > 0.05 for all).
Discussion
In the present cross-sectional study, NAFLD was significantly associated with risk of osteoporotic fractures in men 55 years or older; however, no association was observed in women. A further stratified analysis showed that there was a significant association between NAFLD and risk of osteoporotic fractures in men without dyslipidemia.
According to the unadjusted model, NAFLD was significantly associated with increased odds of osteoporotic fractures for women. However, after adjusting for age, smoking status, alcohol status, physical activity, diabetes, hypertension, cardiovascular events, family history of fracture, dyslipidemia, waist circumference, BMI, TG, TC, HDL-C, LDL-C, and BMD, the association was no longer significant. Moreover, according to a dyslipidemia status subgroup analysis, a significant association between NAFLD and risk of osteoporotic fractures only remained for men without dyslipidemia status, which might imply a true effect of age.
Previous studies have primarily focused on the relationship between NAFLD and BMD and have reported contradictory results. For example, many observational studies reported that there was a significant association between NAFLD and osteopenia/osteoporosis (21, 22). Conversely, a meta-analysis of five cross-sectional studies showed no significant difference in BMD between patients with and without NAFLD (n = 1,276 participants, 638 cases of NAFLD); however, obesity and insulin resistance may have affected this association (23). On the contrary, a prospective cohort study consisting of 1,659 participants found that there was a significant inverse association between liver fat content and lumbar spine, hip, and whole-body BMD (β = −0.123, P = 0.001; β = −0.101, P = 0.008; β = −0.130, P < 0.001, respectively) and osteocalcin (β = −0.116, P = 0.001) for men; however, no associations were observed for postmenopausal women (β = −0.068, P = 0.068 for lumbar spine BMD; β = −0.029, P = 0.429 for hip BMD; β = −0.041, P < 0.224 for whole-body BMD; β = −0.025, P < 0.498 for osteocalcin) (21). Moreover, a large, retrospective, cross-sectional study comprising 6,634 participants showed that there was a significant negative association between femoral neck BMD and NAFLD for men (β = −0.013, P = 0.029), but that there was a positive association between lumbar spine BMD and NAFLD for postmenopausal women (β = 0.022, P = 0.005) (24).
Our analyses showed similar but generally stronger associations between NAFLD and osteoporotic fracture risk compared to those indicated by the only available cross-sectional study of this issue that found that NAFLD may have increased the OR of osteoporotic fracture risk for men (14). This study suggested that there may be other factors that interfere with the association between NAFLD and risk of osteoporotic fractures. However, the authors did not exclude middle-aged participants (especially postmenopausal women); therefore, some confounding factors, such as menopause, may have affected the real association between NAFLD and risk of osteoporotic fractures, which may have reduced the strength of their conclusions. Moreover, the authors did not examine whether dyslipidemia status affected the association. To our knowledge, our study is the first observational study involving older men and women (age ≥55 years) that examined the association between NAFLD and risk of osteoporotic fracture and that evaluated whether dyslipidemia status affects this association. Therefore, our study reported several new findings.
To date, the pathophysiological links between NAFLD and osteoporosis and related fractures are unclear (13, 25– 27). Therefore, exploring potential mechanisms that link NAFLD and osteoporosis is very important and may potentially lead to additional and novel prevention and treatment strategies (Figure 1).
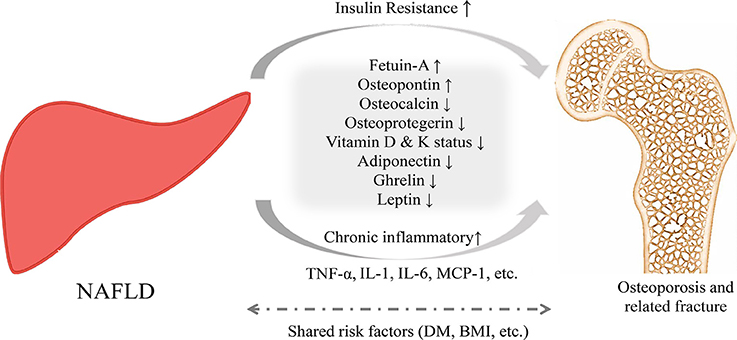
Figure 1. Proposed pathophysiological mechanisms for the relationship between NAFLD and osteoporosis and related fractures.
For example, insulin resistance is considered to be a major risk factor for NAFLD, and this may also be observed in patients with lower BMI or normal glucose tolerance. Accumulation of intrahepatic lipids is associated with a decrease in insulin sensitivity in the liver, bone, muscle, and fat (28). Previous studies reported that higher fasting serum insulin and insulin resistance may be associated with higher risk of lower BMD and lower femoral neck strength (29, 30). However, this association remains controversial (31, 32). Experimental studies showed that in rats consuming a high-fat diet, development of insulin resistance could decrease osteoblast proliferation and differentiation and increase osteoblast apoptosis, resulting in decreased jaw bone density (33).
Chronic inflammation may be another important cross-talk mechanism linking NAFLD and osteopenia/osteoporosis. For example, higher concentrations of interleukin-1, interleukin-6, and tumor necrosis factor-α were found with several liver diseases, such as cirrhosis, hepatitis, and alcoholic liver disease. Moreover, these inflammatory cytokines may enhance the activity of osteoclasts, inhibit the apoptosis of osteoclasts, and stimulate osteoclastogenesis (34–37).
Vitamin D is a fat-soluble vitamin that is involved in bone-related metabolism (38–40), and 25-hydroxyvitamin D, which is the most important circulating and storing metabolite of vitamin D, is produced in the liver. Previous studies have shown that vitamin D deficiency has had a role in the pathogenesis of both NAFLD and osteoporosis (41, 42). Further well-designed, randomized clinical trials are needed to investigate the potential for vitamin D supplementation in NAFLD patients to reduce the risk of lower BMD and fractures.
Osteocalcin, a marker of bone formation, is mainly expressed by osteoblasts. Osteocalcin−/− knockout mice have exhibited increased fat mass and insulin resistance and decreased expression of insulin target genes and adiponectin genes (43). Additionally, several epidemiological studies investigated the association between osteocalcin and NAFLD (44–47). In a Chinese cohort study, an inverse correlation was found between serum osteocalcin level and the scale of NAFLD (r = −0.150, P < 0.010) (45). Other studies also confirmed this inverse relationship between osteocalcin and NAFLD in both male and female participants (44, 46).
Osteoprotegerin (OPG), an important coordinator in the balance between bone formation and bone resorption, is associated with obesity and insulin resistance (48). The possible role of OPG in the pathogenesis of NAFLD is controversial. Epidemiological studies reported that serum OPG in patients with NAFLD was much lower than that in patients without NAFLD (49, 50). However, other studies have yielded contradictory results (51). The role of OPG in relation to NAFLD and BMD needs further investigation.
Our study had several limitations. First, the current study had a cross-sectional design, which cannot draw a causal inference. Further well-designed, prospective cohort studies are needed to investigate a potential causal relationship. Second, recall bias is innate and there is the possibility for confusion regarding data from participants' medication history and dietary records. Third, our study used verified, interviewer-assisted, standardized, self-administered questionnaires for the assessment of fractures, thereby possibly missing vertebral fractures. Fourth, risk of osteoporotic fractures is associated with many factors, such as lifestyle, disease status, metabolic factors, inflammatory states, and genetic factors (3–5, 52, 53). Although wide epidemiologic and clinical covariables were included in the adjustment, we could not exclude the possibility of residual confounding variables, such as bone markers, vitamin D/calcium, decreased glucose levels, and muscle strength, in the analyses. Therefore, additional well-designed and stratified cohort studies that include sufficient controls and account for confounding factors are needed to elucidate the link between NAFLD and the risk of osteoporotic fractures. Fifth, our study was underpowered for the assessment of NAFLD. Liver biopsy is the gold standard for the diagnosis of NAFLD. In our study, the diagnosis of NAFLD was based on ultrasonic examination, which may have underestimated the incidence of NAFLD. However, due to its invasiveness and the potential for complications, liver biopsy is not commonly used in epidemiological studies or in the clinic. Ultrasonography is a noninvasive and simple tool for the diagnosis of NAFLD. According to previous epidemiological studies, ultrasonic examination was the most commonly used assessment for NAFLD. Finally, although a wide variety of epidemiologic and clinical covariates were included in the adjustment, we cannot exclude the possibility of residual confounders in the analyses. Therefore, our results should be interpreted with caution.
In conclusion, our study demonstrated that there was a significant association between NAFLD and osteoporotic fracture risk in older Chinese men, and particularly in men without dyslipidemia. Additional well-designed and stratified cohort studies with a wide range of controls for confounding factors are required to elucidate the link between NAFLD and the risk of osteoporotic fractures.
Author Contributions
YW, CH, and YC: contributed to the study design; YW, GW, RZ, WZ, and SL: performed data collection, interpretation, and analysis; CH and YC: performed critical review. All authors performed data collection, case diagnoses, and confirmation of case diagnoses.
Funding
The project was supported by a grant from the National Natural Science Foundation of China (No. 81572122).
Conflict of Interest Statement
The authors declare that the research was conducted in the absence of any commercial or financial relationships that could be construed as a potential conflict of interest.
Supplementary Material
The Supplementary Material for this article can be found online at: https://www.frontiersin.org/articles/10.3389/fendo.2018.00408/full#supplementary-material
Abbreviations
95% CI, 95% confidence interval; BMD, bone mineral density; BMI, body mass index; HDL-C, high-density lipoprotein cholesterol; LDL-C, low-density lipoprotein cholesterol; NAFLD, nonalcoholic fatty liver disease; OR, odds ratio; TC, total cholesterol; TG, serum triglycerides.
References
1. Khosla S, Hofbauer LC. Osteoporosis treatment: recent developments and ongoing challenges. Lancet Diabetes Endocrinol. (2017) 5:898–907. doi: 10.1016/S2213-8587(17)30188-2
2. Rachner TD, Khosla S, Hofbauer LC. Osteoporosis: now and the future. Lancet. (2011) 377:1276–87. doi: 10.1016/S0140-6736(10)62349-5
3. Ensrud KE, Crandall CJ. Osteoporosis. Ann Intern Med. (2017) 167:ITC17–32. doi: 10.7326/AITC201708010
4. Lems WF. Fracture risk estimation may facilitate the treatment gap in osteoporosis. Ann Rheum Dis. (2015) 74:1943–5. doi: 10.1136/annrheumdis-2015-208245
5. Hendrickx G, Boudin E, Van Hul W. A look behind the scenes: the risk and pathogenesis of primary osteoporosis. Nat Rev Rheumatol. (2015) 11:462–74. doi: 10.1038/nrrheum.2015.48
6. Tilg H, Moschen AR, Kaser A, Pines A, Dotan I. Gut, inflammation and osteoporosis: basic and clinical concepts. Gut (2008) 57:684–94. doi: 10.1136/gut.2006.117382
7. Mundy GR. Osteoporosis and inflammation. Nutr Rev. (2007) 65:S147–51. doi: 10.1111/j.1753-4887.2007.tb00353.x
8. Blum MR, Bauer DC, Collet TH, Fink HA, Cappola AR, da Costa BR, et al. Subclinical thyroid dysfunction and fracture risk: a meta-analysis. JAMA (2015) 313:2055–65. doi: 10.1001/jama.2015.5161
9. Qin L, Yang Z, Zhang W, Gu H, Li X, Zhu L, et al. Metabolic syndrome and osteoporotic fracture: a population-based study in China. BMC Endocr Disord. (2016) 16:27. doi: 10.1186/s12902-016-0106-x
10. Adams LA, Anstee QM, Tilg H, Targher G. Non-alcoholic fatty liver disease and its relationship with cardiovascular disease and other extrahepatic diseases. Gut (2017) 66:1138–53. doi: 10.1136/gutjnl-2017-313884
11. Targher G, Byrne CD. Non-alcoholic fatty liver disease: an emerging driving force in chronic kidney disease. Nat Rev Nephrol. (2017) 13:297–310. doi: 10.1038/nrneph.2017.16
12. Francque SM, van der Graaff D, Kwanten WJ. Non-alcoholic fatty liver disease and cardiovascular risk: pathophysiological mechanisms and implications. J Hepatol. (2016) 65:425–43. doi: 10.1016/j.jhep.2016.04.005
13. Yilmaz Y. Review article: non-alcoholic fatty liver disease and osteoporosis–clinical and molecular crosstalk. Aliment Pharmacol Ther. (2012) 36:345–52. doi: 10.1111/j.1365-2036.2012.05196.x
14. Li M, Xu Y, Xu M, Ma L, Wang T, Liu Y, et al. Association between nonalcoholic fatty liver disease (NAFLD) and osteoporotic fracture in middle-aged and elderly Chinese. J Clin Endocrinol Metab. (2012) 97:2033–8. doi: 10.1210/jc.2011-3010
15. Wang Y, Dai J, Zhong W, Hu C, Lu S, Chai Y. Association between serum cholesterol level and osteoporotic fractures. Front Endocrinol (Lausanne). (2018) 9:30. doi: 10.3389/fendo.2018.00030
16. Nuotio J, Oikonen M, Magnussen CG, Viikari JS, Hutri-Kahonen N, Jula A, et al. Adult dyslipidemia prediction is improved by repeated measurements in childhood and young adulthood. The Cardiovascular Risk in Young Finns Study. Atherosclerosis (2015) 239:350–7. doi: 10.1016/j.atherosclerosis.2015.02.004
17. Perk J, De Backer G, Gohlke H, Graham I, Reiner Z, Verschuren M, et al., European guidelines on cardiovascular disease prevention in clinical practice (version 2012). The Fifth Joint Task Force of the European Society of Cardiology and Other Societies on Cardiovascular Disease Prevention in Clinical Practice (constituted by representatives of nine societies and by invited experts). Eur Heart J. (2012) 33:1635–701. doi: 10.1093/eurheartj/ehs092
18. Fan JG, Farrell GC. Epidemiology of non-alcoholic fatty liver disease in China. J Hepatol. (2009) 50:204–10. doi: 10.1016/j.jhep.2008.10.010
19. Gregg EW, Cauley JA, Seeley DG, Ensrud KE, Bauer DC. Physical activity and osteoporotic fracture risk in older women. Study of Osteoporotic Fractures Research Group. Ann Intern Med. (1998) 129:81–8.
20. van Meurs JB, Dhonukshe-Rutten RA, Pluijm SM, van der Klift M, de Jonge R, Lindemans J, et al. Homocysteine levels and the risk of osteoporotic fracture. N Engl J Med. (2004) 350:2033–41. doi: 10.1056/NEJMoa032546
21. Xia MF, Lin HD, Yan HM, Bian H, Chang XX, Zhang LS, et al. The association of liver fat content and serum alanine aminotransferase with bone mineral density in middle-aged and elderly Chinese men and postmenopausal women. J Transl Med. (2016) 14:11. doi: 10.1186/s12967-016-0766-3
22. Yang HJ, Shim SG, Ma BO, Kwak JY. Association of nonalcoholic fatty liver disease with bone mineral density and serum osteocalcin levels in Korean men. Eur J Gastroenterol Hepatol. (2016) 28:338–44. doi: 10.1097/MEG.0000000000000535
23. Upala S, Jaruvongvanich V, Wijarnpreecha K, Sanguankeo A. Nonalcoholic fatty liver disease and osteoporosis: a systematic review and meta-analysis. J Bone Miner Metab. (2016) 35:685–93. doi: 10.1007/s00774-016-0807-2
24. Lee JW, Han HS, Han KJ, Park J, Jeon H, Ok MR, et al. Long-term clinical study and multiscale analysis of in vivo biodegradation mechanism of Mg alloy. Proc Natl Acad Sci USA. (2016) 113:716–21. doi: 10.1073/pnas.1518238113
25. Targher G, Lonardo A, Rossini M. Nonalcoholic fatty liver disease and decreased bone mineral density: is there a link? J Endocrinol Invest. (2015) 38:817–25. doi: 10.1007/s40618-015-0315-6
26. Poggiogalle E, Donini LM, Lenzi A, Chiesa C, Pacifico L. Non-alcoholic fatty liver disease connections with fat-free tissues: A focus on bone and skeletal muscle. World J Gastroenterol. (2017) 23:1747–57. doi: 10.3748/wjg.v23.i10.1747
27. Eshraghian A. Bone metabolism in non-alcoholic fatty liver disease: vitamin D status and bone mineral density. Minerva Endocrinol. (2017) 42:164–72. doi: 10.23736/S0391-1977.16.02587-6
28. Gaggini M, Morelli M, Buzzigoli E, DeFronzo RA, Bugianesi E, Gastaldelli A. Non-alcoholic fatty liver disease (NAFLD) and its connection with insulin resistance, dyslipidemia, atherosclerosis and coronary heart disease. Nutrients (2013) 5:1544–60. doi: 10.3390/nu5051544
29. Shin D, Kim S, Kim KH, Lee K, Park SM. Association between insulin resistance and bone mass in men. J Clin Endocrinol Metab. (2014) 99:988–95. doi: 10.1210/jc.2013-3338
30. Ahn SH, Kim H, Kim BJ, Lee SH, Koh JM. Insulin resistance and composite indices of femoral neck strength in Asians: the fourth Korea National Health and Nutrition Examination Survey (KNHANES IV). Clin Endocrinol (Oxf). (2015) 84:185–93. doi: 10.1111/cen.12958
31. Ishii S, Cauley JA, Crandall CJ, Srikanthan P, Greendale GA, Huang MH, et al. Diabetes and femoral neck strength: findings from the hip strength across the menopausal transition study. J Clin Endocrinol Metab. (2012) 97:190–7. doi: 10.1210/jc.2011-1883
32. Reid IR, Evans MC, Cooper GJ, Ames RW, Stapleton J. Circulating insulin levels are related to bone density in normal postmenopausal women. Am J Physiol. (1993) 265:E655–9. doi: 10.1152/ajpendo.1993.265.4.E655
33. Pramojanee SN, Phimphilai M, Kumphune S, Chattipakorn N, Chattipakorn SC. Decreased jaw bone density and osteoblastic insulin signaling in a model of obesity. J Dent Res. (2013) 92:560–5. doi: 10.1177/0022034513485600
34. Fox SW, Fuller K, Chambers TJ. Activation of osteoclasts by interleukin-1: divergent responsiveness in osteoclasts formed in vivo and in vitro. J Cell Physiol. (2000) 184:334–40. doi: 10.1002/1097-4652(200009)184:3<334::AID-JCP7>3.0.CO;2-U
35. Hofbauer LC, Heufelder AE. Intercellular chatter: osteoblasts, osteoclasts and interleukin 6. Eur J Endocrinol. (1996) 134:425–6. doi: 10.1530/eje.0.1340425
36. Li H, Hong S, Qian J, Zheng Y, Yang J, Yi Q. Cross talk between the bone and immune systems: osteoclasts function as antigen-presenting cells and activate CD4+ and CD8+ T cells. Blood (2010) 116:210–7. doi: 10.1182/blood-2009-11-255026
37. Soysa NS, Alles N. NF-kappaB functions in osteoclasts. Biochem Biophys Res Commun. (2009) 378:1–5. doi: 10.1016/j.bbrc.2008.10.146
38. Gunton JE, Girgis CM, Baldock PA, Lips P. Bone muscle interactions and vitamin D. Bone (2015) 80:89–94. doi: 10.1016/j.bone.2015.02.029
39. Lieben L, Carmeliet G. Vitamin D signaling in osteocytes: effects on bone and mineral homeostasis. Bone (2013) 54:237–43. doi: 10.1016/j.bone.2012.10.007
40. Marcelli C, Chavoix C, Dargent-Molina P. Beneficial effects of vitamin D on falls and fractures: is cognition rather than bone or muscle behind these benefits? Osteoporos Int. (2015) 26:1–10. doi: 10.1007/s00198-014-2829-8
41. Wang X, Li W, Zhang Y, Yang Y, Qin G. Association between vitamin D and non-alcoholic fatty liver disease/non-alcoholic steatohepatitis: results from a meta-analysis. Int J Clin Exp Med. (2015) 8:17221–34.
42. Eliades M, Spyrou E, Agrawal N, Lazo M, Brancati FL, Potter JJ, et al. Meta-analysis: vitamin D and non-alcoholic fatty liver disease. Aliment Pharmacol Ther. (2013) 38:246–54. doi: 10.1111/apt.12377
43. Lee NK, Sowa H, Hinoi E, Ferron M, Ahn JD, Confavreux C, et al. Endocrine regulation of energy metabolism by the skeleton. Cell (2007) 130:456–69. doi: 10.1016/j.cell.2007.05.047
44. Sinn DH, Gwak GY, Rhee SY, Cho J, Son HJ, Paik YH, et al. Association between serum osteocalcin levels and non-alcoholic fatty liver disease in women. Digestion (2015) 91:150–7. doi: 10.1159/000369789
45. Liu JJ, Chen YY, Mo ZN, Tian GX, Tan AH, Gao Y, et al. Relationship between serum osteocalcin levels and non-alcoholic fatty liver disease in adult males, South China. Int J Mol Sci. (2013) 14:19782–91. doi: 10.3390/ijms141019782
46. Dou J, Ma X, Fang Q, Hao Y, Yang R, Wang F, et al. Relationship between serum osteocalcin levels and non-alcoholic fatty liver disease in Chinese men. Clin Exp Pharmacol Physiol. (2013) 40:282–8. doi: 10.1111/1440-1681.12063
47. Aller R, Castrillon JL, de Luis DA, Conde R, Izaola O, Sagrado MG, et al. Relation of osteocalcin with insulin resistance and histopathological changes of non alcoholic fatty liver disease. Ann Hepatol. (2011) 10:50–5.
48. Blazquez-Medela AM, Lopez-Novoa JM, Martinez-Salgado C. Osteoprotegerin and diabetes-associated pathologies. Curr Mol Med. (2011) 11:401–16. doi: 10.2174/156652411795976565
49. Yilmaz Y, Yonal O, Kurt R, Oral AY, Eren F, Ozdogan O, et al. Serum levels of osteoprotegerin in the spectrum of nonalcoholic fatty liver disease. Scand J Clin Lab Invest. (2010) 70:541–6. doi: 10.3109/00365513.2010.524933
50. Niu Y, Zhang W, Yang Z, Li X, Fang W, Zhang H, et al. Plasma osteoprotegerin levels are inversely associated with nonalcoholic fatty liver disease in patients with type 2 diabetes: a case-control study in China. Metabolism (2016) 65:475–81. doi: 10.1016/j.metabol.2015.12.005
51. Monseu M, Dubois S, Boursier J, Aube C, Gagnadoux F, Leftheriotis G, et al. Osteoprotegerin levels are associated with liver fat and liver markers in dysmetabolic adults. Diabetes Metab. (2016) 42:364–7. doi: 10.1016/j.diabet.2016.02.004
52. Forstein DA, Bernardini C, Cole RE, Harris ST, Singer A. Before the breaking point: reducing the risk of osteoporotic fracture. J Am Osteopath Assoc. (2013) 113:S5–24; quiz S5.
Keywords: nonalcoholic fatty liver disease, fracture, cross-sectional studies, risk factor, dyslipidemia
Citation: Wang Y, Wen G, Zhou R, Zhong W, Lu S, Hu C and Chai Y (2018) Association of Nonalcoholic Fatty Liver Disease With Osteoporotic Fractures: A Cross-Sectional Retrospective Study of Chinese Individuals. Front. Endocrinol. 9:408. doi: 10.3389/fendo.2018.00408
Received: 28 January 2018; Accepted: 02 July 2018;
Published: 23 July 2018.
Edited by:
Ann Schwartz, University of California, San Francisco, United StatesReviewed by:
Emma M. Clark, University of Bristol, United KingdomBjörn E. Rosengren, Lund University, Sweden
Copyright © 2018 Wang, Wen, Zhou, Zhong, Lu, Hu and Chai. This is an open-access article distributed under the terms of the Creative Commons Attribution License (CC BY). The use, distribution or reproduction in other forums is permitted, provided the original author(s) and the copyright owner(s) are credited and that the original publication in this journal is cited, in accordance with accepted academic practice. No use, distribution or reproduction is permitted which does not comply with these terms.
*Correspondence: Chengfang Hu, cfhu6thhosp@163.com
Yimin Chai, ymchai@sjtu.edu.cn
†These authors have contributed equally to this work.