- 1Division of Developmental Paediatrics, Department of Paediatrics and Child Health, Red Cross War Memorial Children’s Hospital, University of Cape Town, Cape Town, South Africa
- 2Department of Clinical Research, London School of Hygiene & Tropical Medicine, London, United Kingdom
- 3Unit on Child and Adolescent Health, South African Medical Research Council (SAMRC), Cape Town, South Africa
- 4Department of Paediatrics and Child Health, Red Cross War Memorial Children’s Hospital, University of Cape Town, Cape Town, South Africa
- 5Department of Psychiatry and Mental Health, University of Cape Town, Cape Town, South Africa
- 6Department of Psychiatry and Behavioral Sciences, Feinberg School of Medicine, Northwestern University, Chicago, IL, United States
- 7Department of Physiology, Feinberg School of Medicine, Northwestern University, Chicago, IL, United States
- 8Unit on Risk and Resilience in Mental Disorders, South African Medical Research Council (SAMRC), Cape Town, South Africa
Introduction: Fetal alcohol spectrum disorders (FASD) have an estimated global prevalence of 2–5% of births, but prevalence is reported to be as high as 15.5% for FASD in certain high-risk communities in South Africa. Preclinical studies demonstrate that alcohol consumption during pregnancy interferes with thyroid hormone availability and function and negatively impacts exposed offspring. Very little is currently reported on this phenomenon in humans.
Methods: This pilot study was embedded in the Drakenstein Child Health Study, a multi-disciplinary longitudinal birth cohort study investigating the early biological and psychosocial determinants of child health in South Africa. Twenty one mothers and their children with moderate–severe prenatal alcohol exposure (PAE) and 19 mothers and their children with no alcohol exposure were investigated. Maternal exposure history and blood samples were collected in mid-pregnancy and analyzed for serum-free thyroxin (FT4), free triiodothyronine (FT3), and thyroid stimulating hormone (TSH). Children were assessed with formally measured growth parameters and development was evaluated using the Bayley III Scales of Infant and Toddler Development (BSID III) at 6 and 24 months of age.
Results: While there were no significant differences in serum TSH and FT4 between groups, FT3 levels were significantly higher in mothers with moderate–severe prenatal alcohol use. In abstinent pregnant women, levels of FT4 were significantly correlated with infants’ scores on cognitive measures at 6 and 24 months of age and with levels of gross motor skills at 24 months. However, in mothers with alcohol use, FT4 levels were not correlated with any cognitive or motor skills, but FT3 levels were significantly associated with scores on children’s social-emotional development at 24 months of age.
Discussion: Thyroid function in PAE is sufficiently disrupted to lead to alterations in serum FT3 levels. The contrast in findings between PAE and abstinent dyads in their association of maternal thyroid function and infant development further suggests that such disruption is present and may contribute to adverse neurodevelopment. Further work is needed to determine the relationship between peripheral thyroid indices during pregnancy and neurodevelopmental outcomes in the context of PAE.
Introduction
The World Health Organization (1) states that harmful alcohol usage ranks in the top five global contributors to disease, disability, and mortality. Fetal alcohol syndrome (FAS), the most severe of all fetal alcohol spectrum disorders (FASD), is further recognized as one of the major disease and disability categories within alcohol-related disorders (2, 3). The global prevalence of fetal FASD is estimated at 2–5% of births (4–7), but in South Africa, prevalence is reported to be as high as 6.3% for FAS and 15.5% for FASD in certain high-risk communities (8).
Alcohol may directly harm the developing fetus, or act indirectly by, among other mechanisms, suppressing maternal and fetal thyroid function (9–11). There are a number of preclinical studies, which demonstrate that maternal alcohol consumption during pregnancy interferes with thyroid hormone availability or function (12–18). In humans, excessive alcohol consumption may decrease serum levels of triiodothyronine (T3) (19, 20), thyroxine (T4) (21–23), free T3 (FT3), and free T4 (FT4) (24, 25) and increase thyroid stimulating hormone (TSH) levels (26). Alcohol use during pregnancy has also been reported to lead to a significant suppression of newborn TSH levels (27, 28). One contrasting report found that newborn T4 and TSH values were considered normal, though demonstrated a wide range of values (T4: 2.7–42 µg/dl, and TSH: 3.0–114.0 ng/dl), perhaps reflecting the 48 h postpartum sampling range in this study (29). However, no prior work has reported on the relationship between thyroid function during pregnancy in alcohol dependent women and infant developmental outcomes.
Thyroid hormones are essential for normal healthy growth and neurodevelopment. The effect of subclinical hypothyroidism during the antenatal period on pregnancy outcomes has been a focus of attention (30–36). The largest study to date suggested an association of subclinical hypothyroidism with increased risk of premature delivery (37). Studies reporting on the effects of maternal thyroid hypofunction on fetal neurocognitive development have yielded inconsistent findings including one highly powered study showing no significant adverse effect on the intelligence quotient of 3- and 5-year-old children whose mothers had subclinical hypothyroidism in pregnancy (38, 39). In contrast, maternal subclinical hypothyroxinemia has overwhelmingly been reported as significantly associated with lower motor and intellectual development in children (40–53). While, a recent meta-analysis concluded that low maternal FT4 in pregnancy is associated with a threefold risk of delayed cognitive development in children (54), much remains to be learned about the relevant contributing mechanisms, particularly as it relates to maternal alcohol consumption. Our hypothesis is that alcohol consumption in pregnancy alters not only the maternal thyroid function, but that of the mother–fetus dyad. Thus, child neurodevelopment of alcohol consuming mothers may be affected even if maternal thyroid hormone levels are within the normal range.
Animal studies have provided some important insights into the relationships between alcohol exposure during pregnancy and thyroid function. Such studies have identified that placental levels of the thyroid hormone metabolizing enzyme, iodothyronine deiodinase 3 (Dio3), are increased following prenatal alcohol exposure (PAE) (14). Thus, with increased levels of placental Dio3, more T4 and T3 are metabolized into the biologically inactive reversed T3 and T2, respectively, leading to reduced amounts of T4 and T3 reaching the fetus. This is concerning, given the association between maternal FT4 levels and cognition of children at 3–5 years of age (52). The determining factor in thyroid hormone-dependent fetal brain development is the amount of the bioactive T3 in the brain, which is converted locally from T4. Since Dio3 expression is increased in the hippocampus of the PAE offspring, just as in the placenta, an additional decrease of hippocampal T3 is very likely, which is exaggerated by the reduced levels of T4 reaching the brain (16, 55, 56). These events can be precipitated by alcohol during development even in the face of adequate maternal peripheral T4 levels. Some of these mechanisms cannot be and others have not yet been explored in humans.
The current study investigated the impact of peripheral thyroid function of alcohol-consuming pregnant women on child developmental outcomes. We assessed serum FT4, FT3, and TSH levels in pregnant women with moderate to severe alcohol consumption and in those without significant alcohol consumption. We further assessed growth parameters at birth and neurodevelopmental outcomes of their children at 6 and 24 months of age.
Materials and Methods
Participants
The study was embedded in the Drakenstein Child Health Study (DCHS), a multi-disciplinary longitudinal birth cohort study investigating the early biological and psychosocial determinants of child health in two communities in the Western Cape Province, South Africa (57, 58). Both communities are largely low socioeconomic status and are characterized by a high prevalence of psychosocial risk factors. These include high rates of drug and alcohol usage (57), maternal psychological distress and depression (59), community exposure to violence and intimate partner violence (60), and low levels of employment and educational attainment (57). The population is stable, with little immigration or emigration. In excess of 90% of people in the district use the public-sector health system.
The methods of the larger DCHS are described elsewhere (57, 58). Briefly, the cohort consisted of a South African sample of English, Afrikaans, or isiXhosa speaking mother–infant dyads. Mothers were recruited into this population-based cohort study from two clinics between March 2012 and March 2015 while attending for antenatal appointments. Women were enrolled in the DCHS at 20–28 weeks’ gestation and were followed through birth and postnatally. Pregnant mothers were considered eligible for inclusion if they were 18 years or older, were attending antenatal care at one of the two clinics and planned to remain resident in the region for at least 1 year. Mothers who were eligible and agreed to be included, provided informed written consent, in their preferred language, at enrollment and were re-consented annually following childbirth. Informed consent forms described the scope and aims of the study, including potential harm or benefits. The study was approved by the faculty of Health Sciences, Human Research Ethics Committee, University of Cape Town (401/2009), Stellenbosch University (N12/02/0002), and the Western Cape Provincial Health Research committee (2011RP45).
In this pilot study, 21 mothers and their children with moderate–severe (PAE, as defined by mothers drinking at least twice a week and at least two drinks per occasion during any stage of pregnancy prior to recruitment) and 19 mothers and their children with no alcohol exposure (controls) were assessed for maternal thyroid function and child growth and developmental outcomes (61).
Child Assessment
Children were assessed by formally measured growth parameters, and a subgroup had their development evaluated using the Bayley III Scales of Infant Development (BSID III) at 6 and 24 months of age (62). Assessors were blinded to alcohol and thyroid hormone status of the mothers. This assessment of infant development has been widely used globally and has been further validated in a South African population (63). The BSID-III consists of five subscales that were evaluated through direct observation by a trained assessor: cognitive, receptive language and expressive language, fine and gross motor. In addition, two additional scales were assessed through a caregiver questionnaire: Social-Emotional and Adaptive Behavior that can be used to indicate emotional and behavioral dysregulation. Scaled scores adjusted for age are reported, standardized mean 10, SD 3. Quality control was performed on all the BSID-III assessments. Infants were excluded if born <36 weeks or had a low Apgar score (<7 at 5 min) and/or admission for hypoxic ischemic encephalopathy or other significant neonatal complication (such as neonatal jaundice requiring phototherapy). Infants were also excluded if they had an identified genetic syndrome or congenital abnormality.
Thyroid Function Measures
Maternal blood samples were collected in mid-pregnancy, in moderate to severe alcohol-consuming women (PAE) at 5.36 ± 0.92 months, and in abstinent women at 5.18 ± 1.14 months of pregnancy.
For the thyroid function measurements, electrochemiluminescence immunoassays “ECLIA” (Roche Diagnostics GmbH, D-68305 Mannheim) were used on the Elecsys e-immunoanalyzers. The serum TSH assay measures 0.005–100 μIU/mL TSH with 2–8% interassay coefficient of variation. The euthyroid values for non-pregnant test subjects are 0.270–4.20 μIU/mL, given by the manufacturer. The FT4 II assay can quantify 0.3–100 pmol/L FT4 with less than 4% interassay coefficient of variation. The euthyroid values for non-pregnant test subjects are given by the manufacturer as 12–22 pmol/L (2.5th and 97.5th percentile). Finally, the serum FT3 III assay range is 0.6–50 pmol/L FT3 with less than 3% interassay coefficient of variation. The euthyroid values for non-pregnant test subjects are given by the manufacturer as 3.1–6.8 pmol/L (2.5th and 97.5th percentile).
Statistics
We generated descriptive statistics for maternal sociodemographic characteristics, infant anthropometric data, and BSID III Scaled Scores. The data were compared using Student’s t-tests for normally distributed continuous variables (e.g., maternal age), Pearson’s chi-square tests for categorical variables (e.g., ethnicity), and Mann–Whitney U tests for continuous variables that did not meet the assumptions for parametric testing (e.g., BSID Scaled Scores). Statistical analyses for this information was generated using SPSS (version 24).
Serum hormone levels in PAE and abstinent cases were compared using Student’s t-test for the complete dataset or for the upper and lower 50th percentile in the case of serum TSH and FT4, respectively. Please note that Bonferroni correction was not applied in the descriptive statistics (Table 1) and in the maternal thyroid function data (Figure 1). A significant body of literature argues that while the Bonferroni correction directly targets the Type 1 error problem, it does so at the expense of Type 2 error. Therefore, many statisticians recommend reporting all of the individual p values and making it clear that no mathematical correction was made for multiple comparisons (64).
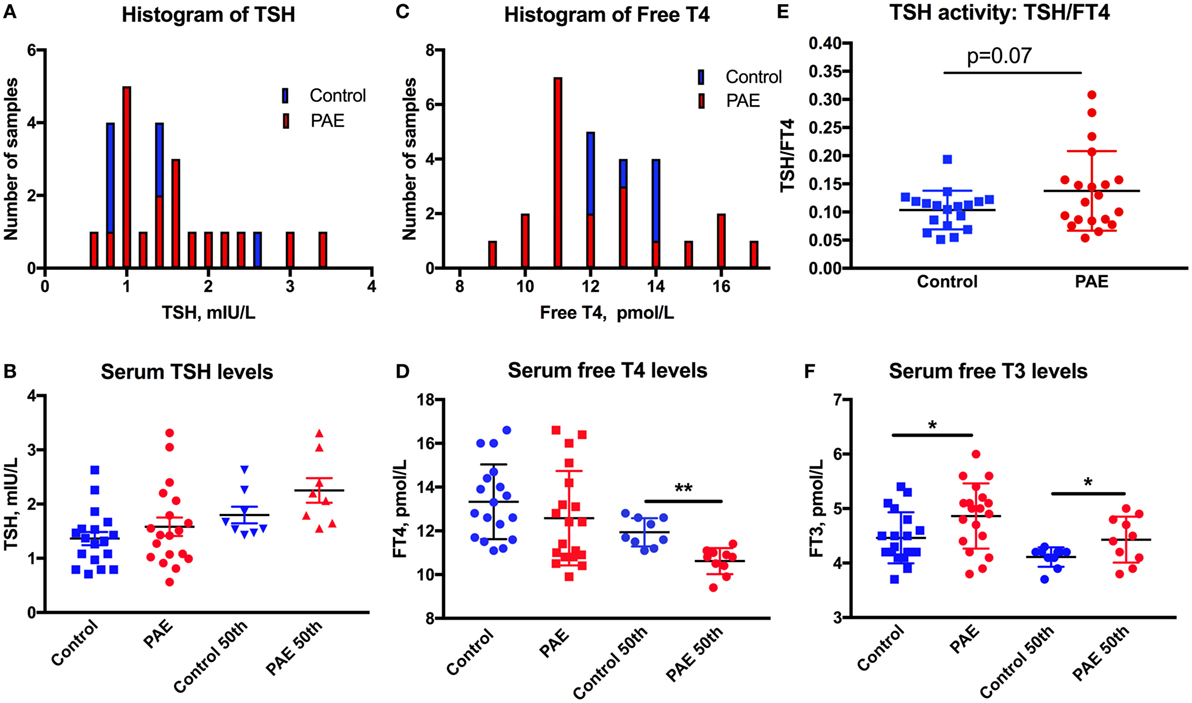
Figure 1. Thyroid function in pregnant women. The distribution is shifted toward higher levels of serum TSH (A), toward lower levels of FT4 (C), and greater ratio of TSH/FT4 (E) in pregnant women with moderate to severe alcohol consumption [prenatal alcohol exposure (PAE)] compared to no alcohol use women (Controls). Means and SEM of serum (B) TSH, (D) FT4, and (F) FT3 levels in PAE and control women, and the highest 50th percentile of TSH, lowest 50th percentile FT4 and FT3, respectively. *p < 0.05; **p < 0.01. Trending significance is shown by the actual p-value.
Pearson correlations were used to assess associations between serum hormone levels and child developmental measurements. FDR-corrections were employed for multiple comparisons, significance was set at p < 0.05. Linear regression analyses were carried out for maternal thyroid function measures and child developmental measures. Statistical analyses were carried out by Graphpad (Prism 7.0).
Results
Table 1 presents the demographic characteristics of the study participants. The two groups of mothers were well matched for age and reasonably matched for ethnicity. Performance on the BSID III in this small cohort did not show significant group differences, with the exception of expressive communication at 6 months of age, but this pilot study was not designed or adequately powered to address this particular question.
Thyroid function of the pregnant women was assessed by serum TSH, FT4, and FT3 measurements. One women from the PAE group and two from the abstinent group had serum TSH levels above 3.5 mIU/L (3.65, 3.57, and 5.76 mIU/L), and so were removed from the dataset. The histograms of serum TSH and FT4 (Figures 1A,C) show the propensity of TSH values to spread toward the higher, and FT4 levels toward the lower levels in the PAE pregnant women. TSH levels did not show significant differences between PAE and abstinent women (Figure 1B). However, while FT4 levels did not differ between the groups, the lower 50th percentile of FT4 values were significantly lower in the PAE compared to abstinent women [t(17) = 4.6, p < 0.01; Figure 1D]. Similarly, the TSH to FT4 ratio tended to be greater in the PAE group [t(36) = 1.86, p = 0.07; Figure 1E], consistent with an exaggeration in the inverse relationship of serum TSH and FT4 in the PAE group. In contrast to FT4, serum FT3 levels were significantly higher in the PAE group compared to abstinent pregnant women [whole group: t(36) = 2.30, p < 0.05; lowest 50th percentile: t(17) = 2.20, p < 0.05; Figure 1F]. The increased serum levels of FT3 and unchanged or decreased FT4 suggest enhanced T4 to T3 conversion in the periphery.
We investigated the maternal thyroid function and infant developmental measures separately for abstinent women and infant dyad and for the alcohol-consuming mother infant dyad. As alcohol affects not only maternal thyroid function but also the developing fetus combining abstinent and alcohol-consuming mothers’ thyroid indices and their infants’ developmental measures may be misleading. In the abstinent women, correlation between maternal thyroid hormone indices during pregnancy and newborn and child measures found that increased maternal FT4 is significantly associated with greater scores on cognitive measures at 6 and 24 months of age and with increased performance in gross motor skills at 24 months of age (Table 2). In contrast, these relationships were not found in PAE dyads (Table 3), but in this group maternal FT3 levels significantly correlated with children’s social-emotional developmental scores at 24 months of age. Linear regression analyses between maternal FT4 and TSH, and cognitive, and language developmental measures of their children at 6 months of age are shown in Figure 2. Higher maternal FT4 levels in abstinent pregnant women predict greater cognitive and communicative functions of their children at 6 month of age [cognition 6 m: F(1, 10) = 6.08; p < 0.05; receptive communication 6 m: F(1, 10) = 9.09; p = 0.01; Figure 2A]. Maternal serum TSH shows the predicted inverse trend with these measures, albeit not significantly [cognition 6 m: F(1, 10) = 3.67; p = 0.08; receptive communication 6 m: F(1, 10) = 2.06; p = 0.18; Figure 2B]. Neither FT4 nor TSH of the pregnant alcohol-consuming women showed a significant relationship with these measures, which displayed a distinctly greater scatter than in the abstinent dyads as represented in Figures 2C,D.
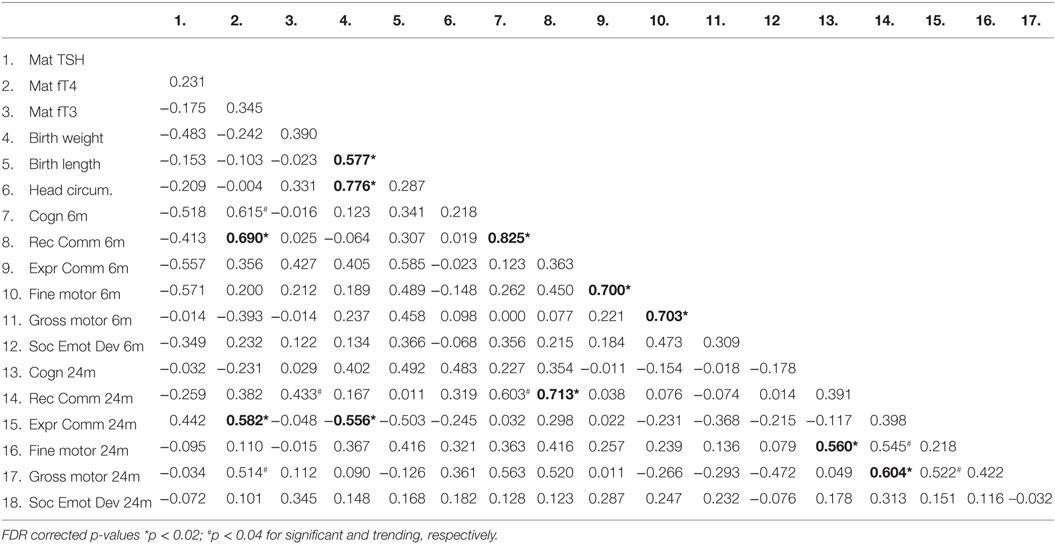
Table 2. Correlation coefficients (Pearson) between thyroid function measures in abstinent pregnant women and their children’s birth and neurobehavioral parameters.
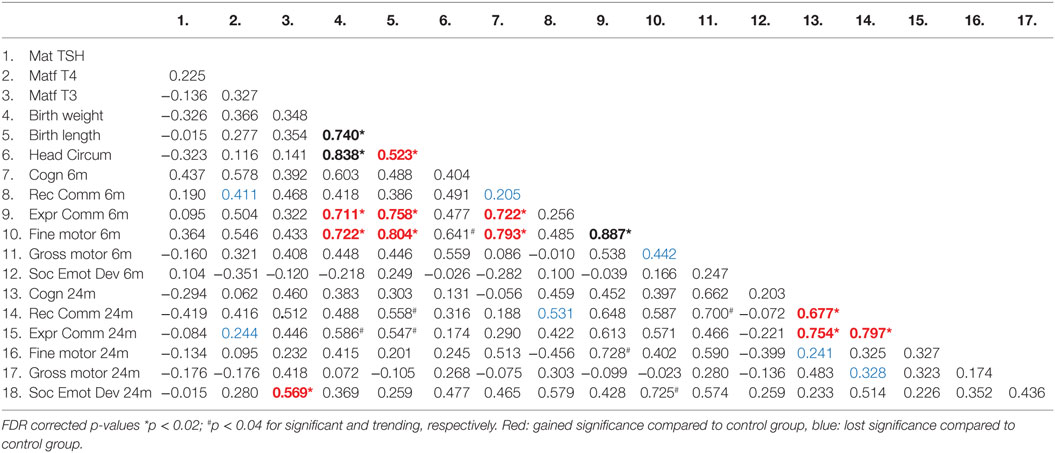
Table 3. Correlation coefficients (Pearson) between thyroid function measures in the moderate to severe alcohol consuming pregnant women and their children’s birth and neurobehavioral parameters.
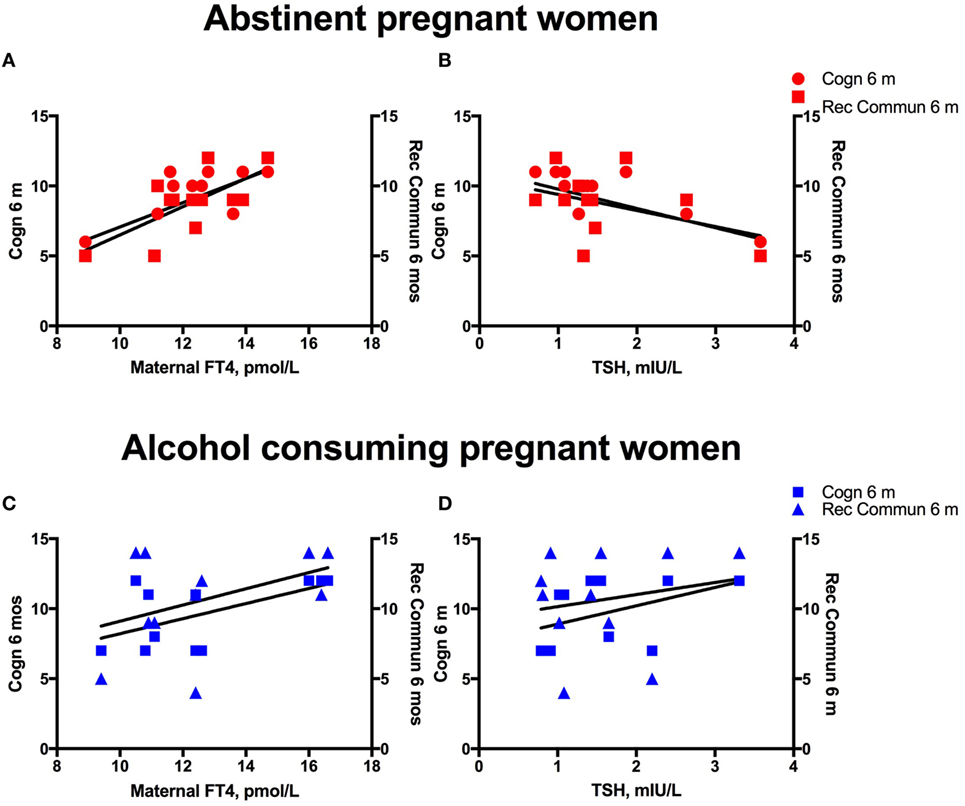
Figure 2. Alcohol consumption interferes with the predictive association between maternal thyroid measures and child development. Linear regression analyses between maternal serum FT4 and TSH, and cognitive, and language developmental measures of their children at 6 months of age in women abstinent during pregnancy (A,B) and pregnant women with moderate to severe alcohol consumption (C,D).
Discussion
This pilot study suggests that moderate-to-severe alcohol consumption during pregnancy is associated with alterations in maternal thyroid function, particularly with increased serum FT3 levels. Increased serum FT4 levels in abstinent pregnant women are significantly associated with higher scores on cognitive measures in early childhood in their children. In contrast, in women with moderate-to-severe alcohol consumption, this association is not present, but instead, increased serum FT3 levels are significantly associated with altered social-emotional development at 24 months of age.
Altered thyroid function in pregnant women who consumed alcohol was reflected in increased FT3. Furthermore, serum TSH tended to be higher, and FT4 tended to be lower in PAE women. Increased serum FT3 in alcohol consuming pregnant women is a novel and unexpected finding. In the light of the tendency of alcohol lowering FT4, increased serum FT3 levels could be due to greater proportion of T4 converted to T3 by increased expression of deiodinase 2 (Dio2) after alcohol exposure. Chronic alcohol exposure is known to increase deiodinase 1 in the liver (65) and deiodinase 2 in the pituitary and the frontal cortex (66) as well as the amygdala (67). Furthermore, in a positive feedforward regulation, T3 is known to induce Dio2 expression in brain regions (68) and in the brown adipose tissue as well (69). An example of how increased Dio2 can coexist with decreased T4 and increased T3, at least in the brain, is shown by the effects of a single dose lithium (67).
Importantly, with greater T4 to T3 conversion in the periphery, less T4 is available to cross to the placenta to the fetal brain. Since only a portion of brain T3 originates from the periphery and the main source is local conversion from T4, the potentially reduced amount of T4 reaching the fetal brain can induce a state of hypothyroidism in the fetal brain. Furthermore, some thyroid hormone transporters prefer T4 to T3; therefore, brain region-specific hypothyroidism can occur in the presence of decreased serum FT4 and elevated FT3 (70). Interestingly, decreased serum FT4 and increased FT3 has been described in patients with posttraumatic stress disorder (PTSD) (71) and in women at risk for postpartum depression (72). Whether that finding is related to alteration in cortisol levels in subjects with PTSD (73), which is also known to occur in alcohol consuming pregnant animals (74, 75) is not known. Additionally, elevated FT3 has been shown to correlate with increases in preterm birth (76), which is very frequent in PAE pregnancies.
The finding here of a correlation between FT4 and cognitive outcomes is consistent with much work showing the reverse relationship: adverse outcomes in children born to mothers with isolated hypothyroxinemia (30, 35, 38, 40, 41, 50, 77–90). Adverse outcomes of hypothyroxinemia reported in populations across the world, include prematurity, lower IQ, general cognitive developmental deficit, language delay, impaired motor function, smaller head circumference among others. In the present study, serum FT4 levels of abstinent women in pregnancy were linearly correlated with their children’s cognitive performance in infancy in the predicted direction. However, this seemingly direct linear relationship between euthyroxinemic maternal milieu and child cognitive development was abolished by alcohol consumption during pregnancy in our pilot cohort. The possibility that an increased proportion of FT4 converted to FT3 in the PAE women suggests potentially decreased FT4 crossing into the placenta. Should placental levels of DIO3 be increased after PAE in humans, as it has been shown in animal studies, even less FT4 and FT3 would reach the fetus. Thus, even in the context of non-significant differences in FT4, in the presence of PAE during this important developmental window, the placental transfer of FT4 and fetal thyroid hormone utilization may differ. Whether subclinical hypothyroidism or hypothyroxinaemia during pregnancy is an additional risk factor for alcohol-induced neurodevelopmental disorder is important to explore. Unfortunately, there remains great controversy about the significance of subclinical hypothyroidism in general, but in pregnancy, in particular. Should alcohol consumption in pregnancy alter not only the maternal thyroid function but that of the mother–fetus dyad, then neurodevelopment of the children alcohol consuming mothers may be affected even in the presence of maternal thyroid hormone levels within the normal range. This is the proposed potential mechanism (at least in part) for the effects of alcohol exposure on the developing fetus, and a potential pathway for treatment.
The finding of linear association between the elevated serum FT3 levels in the alcohol consuming pregnant women and their infant’s social and emotional development is difficult to interpret. Without detailed interpretation of this developmental measurement, one can venture the opinion that this relationship may cover multiple aspects of emotional development. Specifically, very young children with FASD have been described as being “excessively friendly and fearless” (91), “friendly and trusting with strangers” (92), thus clearly indicating differences in their emotional, social development from the control population. This verbal, but impulsive, disposition may be the signature that is being picked up in this domain (93). The actual items measured in this domain at this age include requesting physical and social need through verbal or visual means. Thus, a more demanding or forceful child may score highly on these particular items without socio-emotional flexibility, which is one of the hallmark deficits of the FASD profile. As with all early developmental outcome studies, the answers may only manifest with larger samples.
A number of limitations to this pilot study should be emphasized. In particular, the small sample size, the lack of maternal thyroid indices at earlier time points in pregnancy and additional measurements of peripheral thyroid function, resulting in the conservative interpretation of the findings. Nevertheless, these are the first data to report child developmental outcomes in relation to maternal thyroid indices in the context of PAE. These findings are supported by animal work where thyroid hormone efficacy and metabolism is affected by alcohol exposure during pregnancy with an impact on the offspring cognitive outcomes compared to those infants born to non-alcohol consuming mothers (55, 56). Future studies, in addition to expanding the sample size, will investigate changes in maternal and child thyroid hormone profile in the context of PAE and in healthy unexposed pregnancies over the course of pregnancy. Additionally, longitudinal developmental trajectories into later childhood should be used to investigate the mediating role of thyroid function in pregnancy on child development in future studies involving abstinent and moderate to severe alcohol consuming women.
Ethics Statement
The study was approved by the faculty of Health Sciences, Human Research Ethics Committee, University of Cape Town (401/2009), Stellenbosch University (N12/02/0002), and the Western Cape Provincial Health Research committee (2011RP45). All subjects gave written informed consent in accordance with the Declaration of Helsinki.
Author Contributions
ER, DS, and KD conceived the research question, refined the approach for this specific project. ER performed the analysis. KD and ER provided the interpretation of the results and jointly wrote the first draft of the manuscript. HZ conceived and is lead investigator of the umbrella birth cohort and DS the psychosocial arm in which this pilot analysis was embedded and as such facilitated the data collection and study design. WB, NH, and CW were all involved in data collection, quality control, and interpretation of both maternal and infant outcome measures in specific content areas. All authors reviewed and approved the final draft of this manuscript.
Conflict of Interest Statement
The authors declare that the research was conducted in the absence of any commercial or financial relationships that could be construed as a potential conflict of interest.
Acknowledgments
We thank the study staff in Paarl, the study data team and lab teams, the clinical and administrative staff of the Western Cape Government Health Department at Paarl Hospital and at the clinics for support of the study. We acknowledge the advice from members of the study International Advisory Board and thank our collaborators. We thank the families and children who participated in this study.
Funding
The study was funded by the Bill and Melinda Gates Foundation (OPP 1017641). Additional support for HZ and DS by the MRC of South Africa. Additional aspects of the work reported here are supported by the South African NRF and MRC, by an Academy of Medical Sciences Newton Advanced Fellowship (NAF002\1001), funded by the UK Government’s Newton Fund, by NIAAA via (R21AA023887) and by the Collaborative Initiative on Fetal Alcohol Spectrum Disorders (CIFASD) developmental grant (U24 AA014811), by the US Brain and Behaviour Foundation Independent Investigator grant (24467) and by NIAAA (RO1AA017978). WB is supported by the SAMRC National Health Scholars programme, and CW is supported by the Wellcome Trust through a Research Training Fellowship (203525/Z/16/Z).
References
1. World Health Organization. Global Status Report on Alcohol and Health. 2014 ed. Geneva: World Health Organization Press (2014).
2. Lupton C, Burd L, Harwood R. Cost of fetal alcohol spectrum disorders. Am J Med Genet (2004) 127(1):42–50. doi:10.1002/ajmg.c.30015
3. Navarro HJ, Doran CM, Shakeshaft AP. Measuring costs of alcohol harm to others: a review of the literature. Drug Alcohol Depend (2011) 114(2):87–99. doi:10.1016/j.drugalcdep.2010.11.009
4. May PA, Baete A, Russo J, Elliott AJ, Blankenship J, Kalberg WO, et al. Prevalence and characteristics of fetal alcohol spectrum disorders. Pediatrics (2014) 134(5):855–66. doi:10.1542/peds.2013-3319
5. May PA, Blankenship J, Marais AS, Gossage JP, Kalberg WO, Joubert B. Maternal alcohol consumption producing fetal alcohol spectrum disorders (FASD): quantity, frequency, and timing of drinking. Drug Alcohol Depend (2013) 133(2):502–12. doi:10.1016/j.drugalcdep.2013.07.013
6. May PA, Fiorentino D, Gossage JP, Kalberg WO, Hoyme HE, Robinson LK. Epidemiology of FASD in a province in Italy: prevalence and characteristics of children in a random sample of schools. Alcohol Clin Exp Res (2006) 30:1562–75. doi:10.1111/j.1530-0277.2006.00188.x
7. May PA, Gossage JP, Kalberg WO, Robinson LK, Buckley DG, Manning M. The prevalence and epidemiologic characteristics of FASD from various research methods with an emphasis on in-school studies. Dev Disabil Res Rev (2009) 15:176–92. doi:10.1002/ddrr.68
8. May PA, De Vries MM, Marais AS, Kalberg WO, Buckley D, Adnams CM, et al. Replication of high fetal alcohol spectrum disorders prevalence rates, child characteristics, and maternal risk factors in a second sample of rural communities in South Africa. Int J Environ Res Public Health (2017) 14(5):E522. doi:10.3390/ijerph14050522
9. Gabriel K, Hofmann C, Glavas M, Weinberg J. The hormonal effects of alcohol use on the mother and fetus. Alcohol Health Res World (1998) 22(3):170.
10. Rachdaoui N, Sarkar DK. Effects of alcohol on the endocrine system. Endocrinol Metab Clin North Am (2013) 42(3):593–615. doi:10.1016/j.ecl.2013.05.008
11. Zhang X, Sliwowska JH, Weinberg J. Prenatal alcohol exposure and fetal programming: effects on neuroendocrine and immune function. Exp Biol Med (Maywood) (2005) 230:376–88. doi:10.1177/15353702-0323006-05
12. Gottesfeld Z, Silverman PB. Developmental delays associated with prenatal alcohol exposure are reversed by thyroid hormone treatment. Neurosci Lett (1990) 109(1–2):42–7. doi:10.1016/0304-3940(90)90535-H
13. Scott HC, Sun GY, Zoeller RT. Prenatal ethanol exposure selectively reduces the mα-1 thyroid hormone receptor in fetal rat brain. Alcohol Clin Exp Res (1998) 22:2111–7. doi:10.1111/j.1530-0277.1998.tb05924.x
14. Shukla PK, Sittig LJ, Ullmann TM, Redei EE. Candidate placental biomarkers for intrauterine alcohol exposure. Alcohol Clin Exp Res (2011) 35(3):559–65. doi:10.1111/j.1530-0277.2010.01373.x
15. Sittig LJ, Redei EE. Paternal genetic contribution influences fetal vulnerability to maternal alcohol consumption in a rat model of fetal alcohol spectrum disorder. PLoS One (2010) 5:e10058. doi:10.1371/journal.pone.0010058
16. Tunc-Ozcan E, Harper KM, Graf EN, Redei EE. Thyroxine administration prevents matrilineal intergenerational consequences of in utero ethanol exposure in rats. Horm Behav (2016) 82:1–10. doi:10.1016/j.yhbeh.2016.04.002
17. Wilcoxon J, Kuo A, Disterhoft J, Redei E. Behavioral deficits associated with fetal alcohol exposure are reversed by prenatal thyroid hormone treatment: a role for maternal thyroid hormone deficiency in FAE. Mol Psychiatry (2005) 10(10):961–71. doi:10.1038/sj.mp.4001694
18. Wilcoxon JS, Redei EE. Prenatal programming of adult thyroid function by alcohol and thyroid hormones. Am J Physiol Endocrinol Metab (2004) 287(2):E318–26. doi:10.1152/ajpendo.00022.2004
19. Hegedus L, Rasmussen N, Ravn V, Kastrup J, Krogsgaard K, Aldershvile J. Independent effects of liver disease and chronic alcoholism on thyroid function and size: the possibility of a toxic effect of alcohol on the thyroid gland. Metabolism (1988) 37(3):229–33. doi:10.1016/0026-0495(88)90100-X
20. Liappas I, Piperi C, Malitas PN, Tzavellas EO, Zisaki A, Liappas AI, et al. Interrelationship of hepatic function, thyroid activity and mood status in alcohol-dependent individuals. In Vivo (2006) 20(2):293–300.
21. Geurts J, Glinoer D, Prigogine T, Corvilain J, Demeester-Mirkine N, Fernandez-Deville M. Alterations in circulating thyroid hormones and thyroxine binding globulin in chronic alcoholism. Clin Endocrinol (Oxf) (1981) 14:113–8. doi:10.1111/j.1365-2265.1981.tb00605.x
22. Heinz A, Bauer M, Kuhn S, Rommelspacher H, Krüger F, Gräf K. Long-term observation of the hypothalamic-pituitary-thyroid (HPT) axis in alcohol-dependent patients. Acta Psychiatr Scand (1996) 93(6):470–6. doi:10.1111/j.1600-0447.1996.tb10679.x
23. Hermann D, Heinz A, Mann K. Dysregulation of the hypothalamic-pituitary-thyroid axis in alcoholism. Addiction (2002) 97(11):1369–81. doi:10.1046/j.1360-0443.2002.00200.x
24. Ozsoy S, Esel E, Izgi HB, Sofuoglu S. Thyroid function in early and late alcohol withdrawal: relationship with aggression, family history, and onset age of alcoholism. Alcohol Alcohol (2006) 41(5):515–21. doi:10.1093/alcalc/agl056
25. Papineni JK, Pinnelli VBK, Davanum R. Thyroid hormone levels in chronic alcoholic liver disease patients before and after treatment. J Clin Diagn Res (2017) 11(7):BC13–6. doi:10.7860/JCDR/2017/24552.10276
26. Lee MR, Schwandt ML, Bollinger JW, Dias AA, Oot EN, Goldman D, et al. Effect of functionally significant deiodinase single nucleotide polymorphisms on drinking behavior in alcohol dependence: an exploratory investigation. Alcohol Clin Exp Res (2015) 39(9):1665–70. doi:10.1111/acer.12814
27. Herbstman J, Apelberg BJ, Witter FR, Panny S, Goldman LR. Maternal, infant, and delivery factors associated with neonatal thyroid hormone status. Thyroid (2008) 18:67–76. doi:10.1089/thy.2007.0180
28. Hernandez JT, Hoffman L, Weavil S, Cvejin S, Prange AJ. The effect of drug exposure on thyroid hormone levels of newborns. Biochem Med Metab Biol (1992) 48(3):255–62. doi:10.1016/0885-4505(92)90072-7
29. Hannigan JH, Martier SS, Naber JM. Independent associations among maternal alcohol consumption and infant thyroxine levels and pregnancy outcome. Alcohol Clin Exp Res (1995) 19(1):135–41. doi:10.1111/j.1530-0277.1995.tb01481.x
30. Karakosta P, Alegakis D, Georgiou V, Roumeliotaki T, Fthenou E, Vassilaki M, et al. Thyroid dysfunction and autoantibodies in early pregnancy are associated with increased risk of gestational diabetes and adverse birth outcomes. J Clin Endocrinol Metab (2012) 97(12):4464–72. doi:10.1210/jc.2012-2540
31. Allan WC, Haddow JE, Palomaki GE, Williams JR, Mitchell ML, Hermos RJ, et al. Maternal thyroid deficiency and pregnancy complications: implications for population screening. J Med Screen (2000) 7(3):127–30. doi:10.1136/jms.7.3.127
32. Mannisto T, Surcel HM, Ruokonen A, Vaarasmaki M, Pouta A, Bloigu A, et al. Early pregnancy reference intervals of thyroid hormone concentrations in a thyroid antibody-negative pregnant population. Thyroid (2011) 21(3):291–8. doi:10.1089/thy.2010.0337
33. Negro R, Schwartz A, Gismondi R, Tinelli A, Mangieri T, Stagnaro-Green A. Increased pregnancy loss rate in thyroid antibody negative women with TSH levels between 2.5 and 5.0 in the first trimester of pregnancy. J Clin Endocrinol Metab (2010) 95(9):E44–8. doi:10.1210/jc.2010-0340
34. Stagnaro-Green A, Chen X, Bogden JD, Davies TF, Scholl TO. The thyroid and pregnancy: a novel risk factor for very preterm delivery. Thyroid (2005) 15(4):351–7. doi:10.1089/thy.2005.15.351
35. Su PY, Huang K, Hao JH, Xu YQ, Yan SQ, Li T, et al. Maternal thyroid function in the first twenty weeks of pregnancy and subsequent fetal and infant development: a prospective population-based cohort study in China. J Clin Endocrinol Metab (2011) 96(10):3234–41. doi:10.1210/jc.2011-0274
36. Schneuer FJ, Nassar N, Tasevski V, Morris JM, Roberts CL. Association and predictive accuracy of high TSH serum levels in first trimester and adverse pregnancy outcomes. J Clin Endocrinol Metab (2012) 97(9):3115–22. doi:10.1210/jc.2012-1193
37. Casey BM, Dashe JS, Spong CY, McIntire DD, Leveno KJ, Cunningham GF. Perinatal significance of isolated maternal hypothyroxinemia identified in the first half of pregnancy. Obstet Gynecol (2007) 109(5):1129–35. doi:10.1097/01.AOG.0000262054.03531.24
38. Lazarus JH, Bestwick JP, Channon S, Paradice R, Maina A, Rees R, et al. Antenatal thyroid screening and childhood cognitive function. N Engl J Med (2012) 366(6):493–501. doi:10.1056/NEJMoa1106104
39. Casey BM, Thom EA, Peaceman AM, Varner MW, Sorokin Y, Hirtz DG, et al. Treatment of subclinical hypothyroidism or hypothyroxinemia in pregnancy. N Engl J Med (2017) 376(9):815–25. doi:10.1056/NEJMoa1606205
40. Korevaar TI, Schalekamp-Timmermans S, de Rijke YB, Visser WE, Visser W, de Muinck Keizer-Schrama SM, et al. Hypothyroxinemia and TPO-antibody positivity are risk factors for premature delivery: the generation R study. J Clin Endocrinol Metab (2013) 98(11):4382–90. doi:10.1210/jc.2013-2855
41. Leon G, Murcia M, Rebagliato M, Alvarez-Pedrerol M, Castilla AM, Basterrechea M, et al. Maternal thyroid dysfunction during gestation, preterm delivery, and birthweight. The Infancia y Medio Ambiente Cohort, Spain. Paediatr Perinat Epidemiol (2015) 29(2):113–22. doi:10.1111/ppe.12172
42. Henrichs J, Bongers-Schokking JJ, Schenk JJ, Ghassabian A, Schmidt HG, Visser TJ, et al. Maternal thyroid function during early pregnancy and cognitive functioning in early childhood: the generation R study. J Clin Endocrinol Metab (2010) 95(9):4227–34. doi:10.1210/jc.2010-0415
43. Craig WY, Allan WC, Kloza EM, Pulkkinen AJ, Waisbren S, Spratt DI, et al. Mid-gestational maternal free thyroxine concentration and offspring neurocognitive development at age two years. J Clin Endocrinol Metab (2012) 97(1):E22–8. doi:10.1210/jc.2011-1772
44. Roman GC, Ghassabian A, Bongers-Schokking JJ, Jaddoe VW, Hofman A, de Rijke YB, et al. Association of gestational maternal hypothyroxinemia and increased autism risk. Ann Neurol (2013) 74(5):733–42. doi:10.1002/ana.23976
45. Finken MJ, van Eijsden M, Loomans EM, Vrijkotte TG, Rotteveel J. Maternal hypothyroxinemia in early pregnancy predicts reduced performance in reaction time tests in 5- to 6-year-old offspring. J Clin Endocrinol Metab (2013) 98(4):1417–26. doi:10.1210/jc.2012-3389
46. Julvez J, Alvarez-Pedrerol M, Rebagliato M, Murcia M, Forns J, Garcia-Esteban R, et al. Thyroxine levels during pregnancy in healthy women and early child neurodevelopment. Epidemiology (2013) 24(1):150–7. doi:10.1097/EDE.0b013e318276ccd3
47. Ghassabian A, El Marroun H, Peeters RP, Jaddoe VW, Hofman A, Verhulst FC, et al. Downstream effects of maternal hypothyroxinemia in early pregnancy: nonverbal IQ and brain morphology in school-age children. J Clin Endocrinol Metab (2014) 99(7):2383–90. doi:10.1210/jc.2013-4281
48. Gyllenberg D, Sourander A, Surcel HM, Hinkka-Yli-Salomaki S, McKeague IW, Brown AS. Hypothyroxinemia during gestation and offspring schizophrenia in a national birth cohort. Biol Psychiatry (2016) 79(12):962–70. doi:10.1016/j.biopsych.2015.06.014
49. Modesto T, Tiemeier H, Peeters RP, Jaddoe VW, Hofman A, Verhulst FC, et al. Maternal mild thyroid hormone insufficiency in early pregnancy and attention-deficit/hyperactivity disorder symptoms in children. JAMA Pediatr (2015) 169(9):838–45. doi:10.1001/jamapediatrics.2015.0498
50. Medici M, Timmermans S, Visser W, de Muinck Keizer-Schrama SM, Jaddoe VW, Hofman A, et al. Maternal thyroid hormone parameters during early pregnancy and birth weight: the Generation R Study. J Clin Endocrinol Metab (2013) 98(1):59–66. doi:10.1210/jc.2012-2420
51. Korevaar TI, Muetzel R, Medici M, Chaker L, Jaddoe VW, de Rijke YB. Association of maternal thyroid function during early pregnancy with offspring IQ and brain morphology in childhood: a population-based prospective cohort study. Lancet Diabetes Endocrinol (2016) 4:35–43. doi:10.1016/S2213-8587(15)00327-7
52. Li Y, Shan Z, Teng W, Yu X, Li Y, Fan C, et al. Abnormalities of maternal thyroid function during pregnancy affect neuropsychological development of their children at 25–30 months. Clin Endocrinol (Oxf) (2010) 72(6):825–9. doi:10.1111/j.1365-2265.2009.03743.x
53. Andersen SL, Andersen S, Liew Z, Vestergaard P, Olsen J. Maternal thyroid function in early pregnancy and neuropsychological performance of the child at 5 years of age. J Clin Endocrinol Metab (2017) 103(2):660–70. doi:10.1210/jc.2017-02171
54. Wang P, Gao J, Zhao S, Guo Y, Wang Z, Qi F. Maternal thyroxine levels during pregnancy and outcomes of cognitive development in children. Mol Neurobiol (2016) 53(4):2241–8. doi:10.1007/s12035-015-9189-z
55. Tunc-Ozcan E, Ullmann TM, Shukla PK, Redei EE. Low-dose thyroxine attenuates autism-associated adverse effects of fetal alcohol in male offspring’s social behavior and hippocampal gene expression. Alcohol Clin Exp Res (2013) 37:1986–95. doi:10.1111/acer.12183
56. Tunc-Ozcan E, Wert SL, Lim PH, Ferreira A, Redei EE. Hippocampus-dependent memory and allele-specific gene expression in adult offspring of alcohol-consuming dams after neonatal treatment with thyroxin or metformin. Mol Psychiatry (2017) 1–9. doi:10.1038/mp.2017.129
57. Stein DJ, Koen N, Donald KA, Adnams CM, Koopowitz S, Lund C, et al. Investigating the psychosocial determinants of child health in Africa: the Drakenstein Child Health Study. J Neurosci Methods (2015) 252:27–35. doi:10.1016/j.jneumeth.2015.03.016
58. Zar HJ, Barnett W, Myer L, Stein DJ, Nicol MP. Investigating the early-life determinants of illness in Africa: the Drakenstein Child Health Study. Thorax (2015) 70(6):592–4. doi:10.1136/thoraxjnl-2014-206242
59. Dewing S, Tomlinson M, le Roux IM, Chopra M, Tsai AC. Food insecurity and its association with co-occurring postnatal depression, hazardous drinking, and suicidality among women in peri-urban South Africa. J Affect Disord (2013) 150(2):460–5. doi:10.1016/j.jad.2013.04.040
60. Groves AK, Moodley D, McNaughton-Reyes L, Martin SL, Foshee V, Maman S. Prevalence, rates and correlates of intimate partner violence among South African women during pregnancy and the postpartum period. Matern Child Health J (2015) 19(3):487–95. doi:10.1007/s10995-014-1528-6
61. Myers B, Koen N, Donald KA, Nhapi RT, Workman L, Barnett W, et al. Effect of hazardous alcohol use during pregnancy on growth outcomes at birth: findings from a South African Cohort Study. Alcohol Clin Exp Res (2018) 42(2):369–77. doi:10.1111/acer.13566
62. Bayley N. Technical Manual of the Bayley Scales of Infant and Toddler Development. 3rd ed. San Antonio, TX: Harcourt Assessment Inc (2006).
63. Ballot DE, Potterton J, Chirwa T, Hilburn N, Cooper PA. Developmental outcome of very low birth weight infants in a developing country. BMC Pediatr (2012) 12:11. doi:10.1186/1471-2431-12-11
64. Gelman A, Hill J, Yajima M. Why we (usually) don’t have to worry about multiple comparisons. J Res Educ Eff (2012) 5(2):189–211. doi:10.1080/19345747.2011.618213
65. Nikodémová M, Benický J, Brtko J, Štrbák V. Chronic ethanol drinking and food deprivation affect rat hypothalamic-pituitary-thyroid axis and TRH in septum. Endocrine (1998) 9(2):213–8. doi:10.1385/ENDO:9:2:213
66. Jimenez J, Osuna C, Rubio A, Guerrero J. Effect of chronic ethanol administration on the rat pineal N-acetyltransferase and thyroxine type II 5′-deiodinase activities. Biosci Rep (1993) 13(2):91–8. doi:10.1007/BF01145961
67. Eravci M, Pinna G, Meinhold H, Baumgartner A. Effects of pharmacological and nonpharmacological treatments on thyroid hormone metabolism and concentrations in rat brain. Endocrinology (2000) 141(3):1027–40. doi:10.1210/endo.141.3.7358
68. Leonard JL, Kaplan MM, Visser TJ, Silva JE, Larsen PR. Cerebral cortex responds rapidly to thyroid hormones. Science (1981) 214(4520):571–3. doi:10.1126/science.7291997
69. Martinez-deMena R, Hernández A, Obregón M-J. Triiodothyronine is required for the stimulation of type II 5′-deiodinase mRNA in rat brown adipocytes. Am J Physiol Endocrinol Metab (2002) 282(5):E1119–27. doi:10.1152/ajpendo.00433.2001
70. Gereben B, McAninch EA, Ribeiro MO, Bianco AC. Scope and limitations of iodothyronine deiodinases in hypothyroidism. Nat Rev Endocrinol (2015) 11(11):642–52. doi:10.1038/nrendo.2015.155
71. Prange AJ Jr. Thyroid axis sustaining hypothesis of posttraumatic stress disorder. Psychosom Med (1999) 61(2):139–40. doi:10.1097/00006842-199903000-00002
72. Le Donne M, Settineri S, Benvenga S. Early pospartum alexithymia and risk for depression: relationship with serum thyrotropin, free thyroid hormones and thyroid autoantibodies. Psychoneuroendocrinology (2012) 37(4):519–33. doi:10.1016/j.psyneuen.2011.08.001
73. Seng JS, Li Y, Yang JJ, King AP, Low LMK, Sperlich M, et al. Gestational and postnatal cortisol profiles of women with posttraumatic stress disorder and the dissociative subtype. J Obstet Gynecol Neonatal Nurs (2017) 47(1):12–22. doi:10.1016/j.jogn.2017.10.008
74. Ramadoss J, Tress U, Chen WJ, Cudd TA. Maternal adrenocorticotropin, cortisol, and thyroid hormone responses to all three-trimester equivalent repeated binge alcohol exposure: ovine model. Alcohol (2008) 42(3):199–205. doi:10.1016/j.alcohol.2007.12.004
75. Slone JL, Redei EE. Maternal alcohol and adrenalectomy: asynchrony of stress response and forced swim behavior. Neurotoxicol Teratol (2002) 24(2):173–8. doi:10.1016/S0892-0362(01)00186-6
76. Johns LE, Ferguson KK, McElrath TF, Mukherjee B, Seely EW, Meeker JD. Longitudinal profiles of thyroid hormone parameters in pregnancy and associations with preterm birth. PLoS One (2017) 12(1):e0169542. doi:10.1371/journal.pone.0169542
77. Kumru P, Erdogdu E, Arisoy R, Demirci O, Ozkoral A, Ardic C, et al. Effect of thyroid dysfunction and autoimmunity on pregnancy outcomes in low risk population. Arch Gynecol Obstet (2015) 291(5):1047–54. doi:10.1007/s00404-014-3533-9
78. Casey BM, Dashe JS, Wells CE, McIntire DD, Byrd W, Leveno KJ, et al. Subclinical hypothyroidism and pregnancy outcomes. Obstet Gynecol (2005) 105(2):239–45. doi:10.1097/01.AOG.0000152345.99421.22
79. Sahu MT, Das V, Mittal S, Agarwal A, Sahu M. Overt and subclinical thyroid dysfunction among Indian pregnant women and its effect on maternal and fetal outcome. Arch Gynecol Obstet (2010) 281(2):215–20. doi:10.1007/s00404-009-1105-1
80. Ong GS, Hadlow NC, Brown SJ, Lim EM, Walsh JP. Does the thyroid-stimulating hormone measured concurrently with first trimester biochemical screening tests predict adverse pregnancy outcomes occurring after 20 weeks gestation? J Clin Endocrinol Metab (2014) 99(12):E2668–72. doi:10.1210/jc.2014-1918
81. Chen LM, Du WJ, Dai J, Zhang Q, Si GX, Yang H, et al. Effects of subclinical hypothyroidism on maternal and perinatal outcomes during pregnancy: a single-center cohort study of a Chinese population. PLoS One (2014) 9(10):e109364. doi:10.1371/journal.pone.0109364
82. Godoy GA, Korevaar TI, Peeters RP, Hofman A, de Rijke YB, Bongers-Schokking JJ, et al. Maternal thyroid hormones during pregnancy, childhood adiposity and cardiovascular risk factors: the Generation R Study. Clin Endocrinol (Oxf) (2014) 81(1):117–25. doi:10.1111/cen.12399
83. Pakkila F, Mannisto T, Pouta A, Hartikainen AL, Ruokonen A, Surcel HM, et al. The impact of gestational thyroid hormone concentrations on ADHD symptoms of the child. J Clin Endocrinol Metab (2014) 99(1):E1–8. doi:10.1210/jc.2013-2943
84. Benhadi N, Wiersinga WM, Reitsma JB, Vrijkotte TG, Bonsel GJ. Higher maternal TSH levels in pregnancy are associated with increased risk for miscarriage, fetal or neonatal death. Eur J Endocrinol (2009) 160(6):985–91. doi:10.1530/EJE-08-0953
85. Liu H, Shan Z, Li C, Mao J, Xie X, Wang W, et al. Maternal subclinical hypothyroidism, thyroid autoimmunity, and the risk of miscarriage: a prospective cohort study. Thyroid (2014) 24(11):1642–9. doi:10.1089/thy.2014.0029
86. Cleary-Goldman J, Malone FD, Lambert-Messerlian G, Sullivan L, Canick J, Porter TF, et al. Maternal thyroid hypofunction and pregnancy outcome. Obstet Gynecol (2008) 112(1):85–92. doi:10.1097/AOG.0b013e3181788dd7
87. Mannisto T, Vaarasmaki M, Pouta A, Hartikainen AL, Ruokonen A, Surcel HM, et al. Thyroid dysfunction and autoantibodies during pregnancy as predictive factors of pregnancy complications and maternal morbidity in later life. J Clin Endocrinol Metab (2010) 95(3):1084–94. doi:10.1210/jc.2009-1904
88. Mannisto T, Vaarasmaki M, Pouta A, Hartikainen AL, Ruokonen A, Surcel HM, et al. Perinatal outcome of children born to mothers with thyroid dysfunction or antibodies: a prospective population-based cohort study. J Clin Endocrinol Metab (2009) 94(3):772–9. doi:10.1210/jc.2008-1520
89. Henrichs J, Ghassabian A, Peeters RP, Tiemeier H. Maternal hypothyroxinemia and effects on cognitive functioning in childhood: how and why? Clin Endocrinol (Oxf) (2013) 79(2):152–62. doi:10.1111/cen.12227
90. Taylor PN, Minassian C, Rehman A, Iqbal A, Draman MS, Hamilton W, et al. TSH levels and risk of miscarriage in women on long-term levothyroxine: a community-based study. J Clin Endocrinol Metab (2014) 99(10):3895–902. doi:10.1210/jc.2014-1954
91. Kully-Martens K, Denys K, Treit S, Tamana S, Rasmussen C. A review of social skills deficits in individuals with fetal alcohol spectrum disorders and prenatal alcohol exposure: profiles, mechanisms, and interventions. Alcohol Clin Exp Res (2012) 36(4):568–76. doi:10.1111/j.1530-0277.2011.01661.x
92. Streissguth A, Clarren S, Jones K. Natural history of the fetal alcohol syndrome: a 10-year follow-up of eleven patients. Lancet (1985) 326(8446):85–91. doi:10.1016/S0140-6736(85)90189-8
Keywords: prenatal thyroid function, prenatal alcohol exposure, fetal alcohol spectrum disorders, infant development, pregnancy thyroid, thyroid function, PAE, child-development
Citation: Donald KA, Wedderburn CJ, Barnett W, Hoffman N, Zar HJ, Redei EE and Stein DJ (2018) Thyroid Function in Pregnant Women With Moderate to Severe Alcohol Consumption Is Related to Infant Developmental Outcomes. Front. Endocrinol. 9:294. doi: 10.3389/fendo.2018.00294
Received: 15 February 2018; Accepted: 17 May 2018;
Published: 05 June 2018
Edited by:
Barbara Anne Demeneix, Centre national de la recherche scientifique (CNRS), FranceReviewed by:
Roberto Vita, Università degli Studi di Messina, ItalySarah Mattson, San Diego State University, United States
Copyright: © 2018 Donald, Wedderburn, Barnett, Hoffman, Zar, Redei and Stein. This is an open-access article distributed under the terms of the Creative Commons Attribution License (CC BY). The use, distribution or reproduction in other forums is permitted, provided the original author(s) and the copyright owner are credited and that the original publication in this journal is cited, in accordance with accepted academic practice. No use, distribution or reproduction is permitted which does not comply with these terms.
*Correspondence: Kirsten A. Donald, a2lyc3R5LmRvbmFsZEB1Y3QuYWMuemE=
†Joint last author.