- 1Instituto Dom Luiz (IDL), Faculdade De Ciências, Universidade De Lisboa, Campo Grande, Portugal
- 2Departamento De Geologia, Faculdade De Ciências, Universidade De Lisboa, Campo Grande, Portugal
- 3Geosciences Barcelona (Geo3BCN-CSIC), Barcelona, Spain
- 4School of Earth and Environmental Sciences, Cardiff University, Cardiff, United Kingdom
- 5School of Earth Sciences, University of Bristol, Bristol, United Kingdom
- 6Lamont-Doherty Earth Observatory of Columbia University, Palisades, NY, United States
- 7Centro de Informação e Vigilância Sismovulcânica Dos Açores (CIVISA), Ponta Delgada, Portugal
- 8Instituto de Investigação Em Vulcanologia e Avaliação de Riscos (IVAR), Universidade Dos Açores, Ponta Delgada, Portugal
- 9GEOMAR Helmholtz Centre for Ocean Research, Kiel, Germany
- 10Department of Earth and Ocean Dynamics, Universitat de Barcelona, Barcelona, Spain
- 11CIBIO, Centro de Investigação Em Biodiversidade e Recursos Genéticos, InBio Laboratório Associado, Azores, Portugal
- 12Departamento de Biologia, Faculdade de Ciências e Tecnologias, Universidade Dos Açores, Ponta Delgada, Portugal
Lacustrine sequences from active volcanic settings usually hold a rich and continuous record of tephra layers, providing a critical source of information to reconstruct a most complete eruptive history of a region. Lake sedimentary records on volcanic islands are particularly useful as the typical small size of these islands and their steep subaerial and submarine slopes lead to a lower preservation of potential erodible pyroclastic deposits. Here we explore the lacustrine sedimentary record of Lagoa da Lomba, a crater lake in the central upland area of Flores Island (Azores), to gain insight into the recent eruptive history of this island. The strategic location of Lagoa da Lomba, half distance between the two clusters of recent volcanic activity of the island, together with its long-lasting record, back to 23.52 cal kyr BP, makes this lake a privileged site to investigate the Holocene volcanic history of Flores. Based on a detailed stratigraphic characterization of sediments from a lake transect of three cores, supported by glass shard geochemistry and radiocarbon dating, we recognized four Holocene eruptive events taking place between 6.28 and 2.36 cal kyr BP, demonstrating that the Holocene volcanic activity at Flores Island may have lasted longer than previously reported. Glass shard geochemistry from the different tephra layers suggests three populations, basaltic to trachybasaltic in composition, where the last eruption is the least evolved endmember. Two of the four eruptive events correlate with subaerially-exposed pyroclastic sequences, in terms of stratigraphy and geochemistry. The most recent event recorded at Lagoa da Lomba was constrained to 3.66 – 2.36 cal kyr BP and linked to an eruption sourced from Lagoa Comprida Volcanic System. The second most recent eruptive event was sourced from Lagoa Funda Volcanic System and dated at 3.66 cal kyr BP. Our observations show that Flores experienced vigorous volcanic activity during the Late Holocene. Therefore, contrary to what is assumed, the possibility of future eruptions should be properly considered, and the volcanic hazard here should not be underestimated. Moreover, we highlight the importance of tephrostratigraphy in recent lake sediments to reconstruct past volcanic activity, especially at small volcanic islands, such as Flores, where exposure is poor due to erosion within the limited subaerial area and the dense vegetation.
Introduction
Lakes are natural traps in drainage basins, and their sedimentary records are potentially continuous on time scales of several millennia. Depending on the age of the lake basin and the sediments, as well as on sedimentation rates, lakes may host crucial information to reconstruct climatic, ecological, and tectonic histories of volcanic regions (e.g., Björck et al., 2006; Sáez et al., 2007; Giralt et al., 2008; Stockhecke et al., 2014; Hernández et al., 2017; Vázquez-Loureiro et al., 2019). In these regions, lacustrine sequences are usually rich in tephra layers, which constitute one of the best means to investigate the frequency, size, and style of eruptions affecting that region, often with a detail that cannot be achieved using terrestrial deposits alone (e.g., Wulf et al., 2004; Stern 2008; Van Daele et al., 2014; Kutterolf et al., 2016; McNamara et al., 2018).
Lake records on volcanic islands may be particularly important, providing information that otherwise would not be accessible, due to the typical small size and irregular topography of these islands, which make the preservation of erodible pyroclastic deposits difficult on land, particularly in areas with high rainfall such as the Azores Archipelago. Moreover, in densely vegetated areas, access to outcrops is frequently limited (Kueppers et al., 2019). There is also a much smaller potential of preservation of pyroclastic deposits in the energetic shallow coastal waters or along the steep, unstable submarine flanks of the islands (Mitchell, 2003), and since deep-sea drilling is technically very challenging and expensive, we rarely have access to offshore records. As such, lake sedimentary records are key to improve our scientific knowledge of the recent eruptive history of many volcanic archipelagos, particularly those where crater and caldera lakes are very abundant and perennial, as it happens in the Azores Archipelago (Figure 1A). The potential of Azorean lacustrine sequences to reconstruct the volcanic activity of the islands is, to date, still unexplored. This study employs lacustrine sediment records from one of the Azores islands – Flores (Figure 1B) – to investigate its recent eruptive history and volcanic hazard potential.
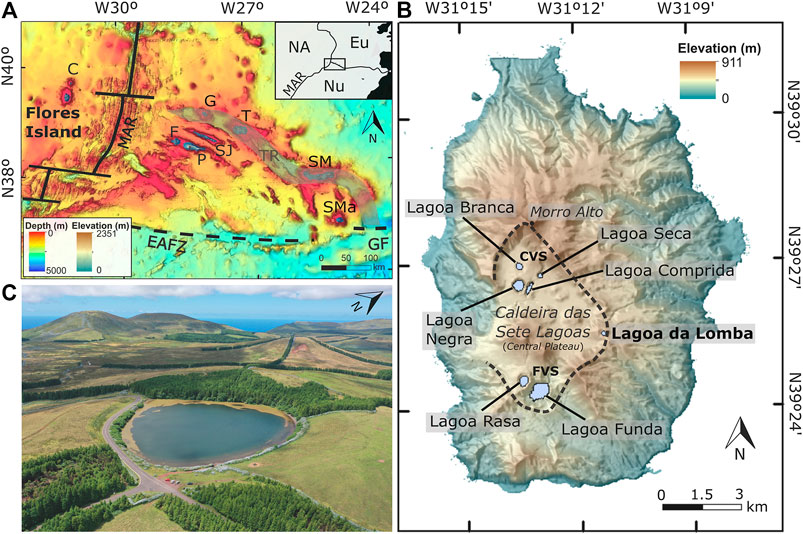
FIGURE 1. (A) Bathymetric map of the North Atlantic at the Azores triple junction, showing the geotectonic setting of Flores Island, located west of the Mid-Atlantic Ridge (MAR), as well as the main tectonic structures of the region: East Azores Fracture Zone (EAFZ), Gloria Fault (GF), and Terceira Rift (TR). The location of the other islands of the archipelago is also indicated: Corvo (C), Pico (P), Faial (F), São Jorge (SJ), Terceira (T), Graciosa (G), São Miguel (SM) and Santa Maria (SMa). Bathymetry from EMODnet Bathymetry Consortium (2018); subaerial topography was generated from a 1:5000 scale digital altimetric database from Secretaria Regional do Turismo e Transportes of the Azores Government. Upper right inset depicts the regional setting of the Azores Archipelago within the triple junction of the North American (NA), Eurasian (Eu) and Nubian (Nu) lithospheric plates (B) Digital elevation model of Flores Island showing the location of the seven lakes in the upland central area of the island (Caldeira das Sete Lagoas, outlined by the dashed line). Lagoa da Lomba is highlighted in the DEM (C) Aerial image of Lagoa da Lomba obtained during a fieldwork campaign in August 2020.
Despite being a young oceanic volcanic island, Flores did not experience any eruption since the Portuguese settlement in the 15th century and therefore its volcanic hazard is usually considered as low (Chester et al., 2017). Moreover, hydrogeochemical and diffuse CO2 degassing studies of Flores lakes show that, presently, there is no volcanic/hydrothermal signature (Cruz et al., 2006; Andrade et al., 2019), suggesting that volcanism is either dormant or extinct. However, the geological record of the island shows that a brief, but intense period of monogenetic volcanism occurred 2.9–3 kyr ago (Morisseau and Traineau, 1985; Azevedo and Portugal Ferreira, 2006). This volcanism is thought to be responsible for the formation of numerous scoria cones, and several maars and tuff rings. The latter are grouped in two clusters (Figure 1B): Lagoa Funda Volcanic System and Lagoa Comprida Volcanic System, hereafter abbreviated as FVS and CVS, respectively. Given this context, it is crucial to improve our knowledge about the recent volcanism of Flores to better evaluate the possibility of future eruptions and associated hazards. In 2017, a coring survey visited several lakes in Flores and Corvo islands to investigate the spatial and temporal climatic evolution of the western Azores Atlantic region. Using the existence of these cores, we conduct a detailed tephrostratigraphic, geochemical, and chronological characterization of the lacustrine sediment record of Lagoa da Lomba (Lomba Lake), an old crater lake in the upland central area of Flores Island. Lagoa da Lomba is located half distance between the two clusters of recent activity of the island (Figure 1B) and, as our study shows, its sedimentary record extends beyond the Holocene. Lagoa da Lomba thus presents the potential to host primary Holocene tephra deposits, being an ideal site to investigate the last volcanic eruptions on Flores.
Geological Setting
Flores Island, located in the North Atlantic Ocean, is part of the Azores Archipelago, which is a volcanic archipelago that straddles the triple junction of the North American, Eurasian and Nubian lithospheric plates (Figure 1A). The archipelago results from volcanic processes associated with the interaction of the lithospheric plates and eventually to the upwelling of unusually hot or volatile-enriched mantle (Métrich et al., 2014; Genske et al., 2016). Flores lies approximately 100 km west of the axis of the Mid-Atlantic Ridge, in the more stable North American plate. Together with the neighboring Corvo Island, it forms the western group of the Azores Archipelago. The islands of Flores and Corvo are the emergent portions of a NNE-SSW-trending volcanic ridge that is subparallel to the Mid-Atlantic Ridge (MAR) (Figure 1A).
Flores is a small island (142 km2) built by overlapping volcanic complexes that reach a maximum altitude of 911 m at Morro Alto (Figure 1B). Current constrains from K/Ar dating indicate that the island edifice formed during the late Pliocene, 2.15 Ma ago (Azevedo et al., 1991), on a 9 Ma old oceanic crust (Freire Luis et al., 1994). Flores Island is the result of a complex geological history with vertical movements and recurrent episodes of mass wasting (Azevedo et al., 1991; Hildenbrand et al., 2018). The island’s topography is characterized by a central upland area, the Central Plateau (500 m above sea level), surrounded by steep slopes and notched valleys. Two main stratigraphic groups characterize the island (Azevedo et al., 1991): the Base Complex, which corresponds to rocks formed during the proto-island stage; and the Upper Complex, which comprises the record of subaerial volcanism. The subaerial volcanic history of the island was dominated by three stages of volcanic activity separated by long periods of quiescence (Azevedo and Portugal Ferreira, 2006):
1) The first stage (700 – 500 ka) includes the most voluminous volcanism, which was characterized by effusive and explosive events;
2) The intermediate stage (400 – 200 ka) involved a larger number of small-scale feeder centers, with predominant effusive eruptions;
3) The final stage (3 – 2.9 ka) was short-lived and marked by Strombolian and subsequent phreatomagmatic activity.
The products of the Holocene volcanic activity crop out mainly in the middle of the Central Plateau, in an area locally known as Caldeira das Sete Lagoas (Figure 1B). According to Azevedo and Portugal Ferreira (2006), the Holocene Strombolian activity was centered at about 30 scoria cones, with gentle flanks and smooth craters, that produced widespread scoria lapilli and ash fall deposits. The most recent volcanic activity on the island is thought to have formed maars and tuff rings that are currently occupied by lakes. These volcanic vents are grouped into two clusters, one in the north part of Caldeira das Sete Lagoas and one in the south sector. The four main northern vents (Lagoa Comprida, Lagoa Negra, Lagoa Seca, and Lagoa Branca) are inferred to be associated with N25°E and N-S fractures, while the southern vents (Lagoa Funda and Lagoa Rasa) are possibly related to N40°W and N20°-25°E fractures. The maars (Lagoa Comprida, Lagoa Negra, Lagoa Seca, and Lagoa Funda) show great depths, steep slopes and diameters ranging from 280 m to ∼1 km, while the tuff rings (Lagoa Branca and Lagoa Rasa) have low crater rims, smooth slopes, and diameters in the order of 300–400 m (Figure 1B). The associated phreatomagmatic deposits are lithic-rich, showing lateral continuity for several hundreds of meters. From the seven crater lakes found in Caldeira das Sete Lagoas, only Lagoa da Lomba (N39°25′31″ W31°11′19″; Figure 1C) was formed in an early stage, prior to the Holocene, and therefore it has the potential to hold the longest lacustrine record on the island. This older topographic depression, located in the upland area of Flores Island (650 m high), has a surface area of 37,885 m2, a perimeter of 716 m and a volume of 143,000 m3. The average lake depth is relatively shallow (7.4 m), but Lagoa da Lomba has steep slopes (∼30° in some areas), reaching a maximum interior crater depth of 16 m (Figure 2; Direção Regional do Ambiente, 2001).
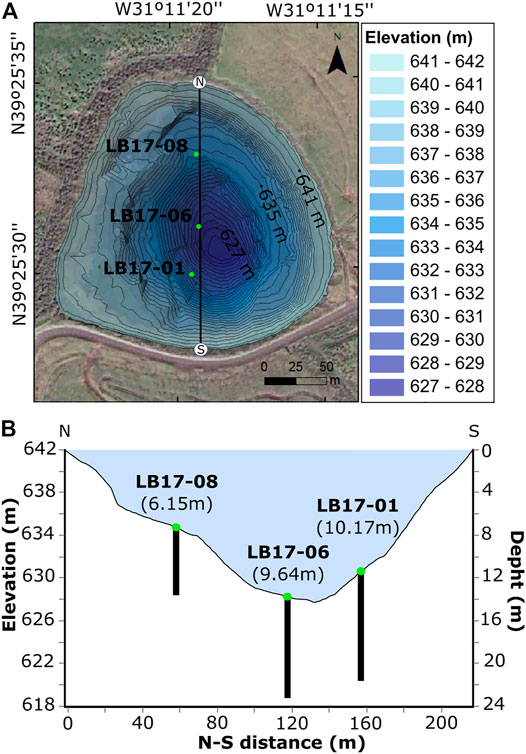
FIGURE 2. (A) Orthophotomap with the bathymetry of Lagoa da Lomba showing as green dots the location of cores LB17-01 (N39°25′29.92″ W31°11′19.55″), LB17-06 (N39°25′31.18″ W31°11′19.28″), and LB17-08 (N39°25′33.06″ W31°11′19.32″). Bathymetry from Direção Regional do Ambiente (2001). Light blue colors represent the shallowest areas and dark blue the deepest parts. Contour lines are spaced at every 50 cm (B) N-S profile of Lagoa da Lomba indicating the site where each core was collected, as well as the lengths (in brackets) of each recovered sequence. The left axis is the elevation relative to sea level, and the right axis the depth of Lagoa da Lomba. The horizontal axis represents the distance between north and south margins [N and S points of the panel (A)]. All values are in meters.
Methods
Coring Survey and Petrographic Analysis
Lagoa da Lomba sediments were drilled in 2017 using a UWITEC® piston corer installed on a platform raft. We performed a transect of three cores, from south to north, LB17-01 (N39°25′29.92″ W31°11′19.55″), LB17-06 (N39°25′31.18″ W31°11′19.28″), and LB17-08 (N39°25′33.06″ W31°11′19.32″) that were collected at lake depths of 10.1, 13.5, and 7.1 m, and whose total lengths are 10.17, 9.64, and 6.15 m, respectively (Figure 2).
Following the methodology employed by Hernández et al. (2017) and Vázquez-Loureiro et al. (2019), a detailed characterization of lacustrine volcanic and non-volcanic facies was conducted through visual core description, supported by smear slides observation under a petrographic microscope at GEO3BCN-CSIC, Barcelona, Spain. Variations in grain size, texture and components were carefully documented next to observed sedimentation features (lamination, boundary conditions, structures). Juvenile clasts (scoria lapilli) from tephra layers were sampled for subsequent geochemical microanalyses.
Field Observations
Two field campaigns taking place in the summer of 2019 and 2020 were conducted to test possible correlations between tephra layers found in cores of Lagoa da Lomba and subaerially-exposed volcanic sequences around the lake, as well as to identify potential sources for the inferred volcanic events. Given that the most recent eruptions at Flores are thought to have been centered at FVS and CVS (Morisseau and Traineau, 1985; Azevedo and Portugal Ferreira, 2006), we investigated the volcanic stratigraphy in the proximal areas of these vents for comparison with the lacustrine record. Accordingly, a detailed field survey was conducted in the Central Plateau area, and a small number of key exposures was selected for logging and sampling. Exposed sequences from field sites (named as FLxxMA) were described in detail regarding their stratigraphical, depositional, and textural characteristics (grain size, components, and thickness). Samples of juvenile scoria lapilli were selectively collected at different horizons for geochemical microanalyses.
Electron Microprobe Analysis
Juvenile scoria lapilli samples were crushed, and together with the lake sediment washed and sieved into 63–125 µm (4-3Φ) fraction. The resulting fragments and glass shards were embedded with epoxy resin into acrylic tablets and then polished. Glass shards (318 in total) were analyzed for major and minor elements on 18 epoxy embedded samples using a JEOL JXA 8200 wavelength dispersive EMP at GEOMAR Helmholtz Centre for Ocean Research, Kiel (Germany), utilizing the methods of Kutterolf et al. (2011). A calibrated measuring program was used based on international standards with a 10 µm electron beam to minimize sodium loss. Oxide concentrations were determined using the ZAF correction method. Accuracy was monitored by Lipari obsidian (Hunt and Hill, 2001) and Smithsonian basaltic standard VGA99 (Jarosewich et al., 1980) having two measurements on each standard after every sixty single glass shard measurements (∼15 per sample). Standard deviations are <0.5% for major and <10% for minor elements (except for MnO2 in samples >55 wt% SiO2). All analyses with totals >97 wt% were normalized to 100% to eliminate the effects of variable post-depositional hydration and minor deviations in focusing of the electron beam. The acceptable analyses of each sample were then averaged in order to characterize the elemental compositions of each tephra. Layers with heterogenous compositions but internal differentiation trends were included with their intra-sample deviation in the data base whereas widely heterogenous compositions outside any possible differentiation trend were excluded. All the resulting major element data and their respective errors are listed in Supplementary Tables S1 and S2.
Lake Chronology
A detailed chronological study was conducted for LB17-01 master core (the longest and most complete sequence recovered at Lagoa da Lomba), which was complemented with a few chronological analyses of LB17-06 and LB17-08 cores. Sediment samples were collected at different levels, particularly at the base of tephra layers to constrain the ages of the volcanic events. Accelerated mass spectrometer (AMS) radiocarbon ages were obtained from pollen enrichment extracts prepared by acid digestion (Rull et al., 2010). Pollen concentrates were prepared at the Laboratory of Sedimentology of the University of Barcelona (Spain) and sent to the Radiochronology Laboratory of the Centre d’Études Nordiques, University of Laval (Canada) for AMS 14C dating. The radiocarbon ages were calibrated with the CALIB 8.2 software, using the IntCal20 calibration curve (Stuiver et al., 2021).
The age-depth model for LB17-01 core was calculated with the R rbacon library v2.5.1 (Blaauw et al., 2021), using the AMS 14C dates as anchor points. This R script calibrated radiocarbon ages using the newest IntCal20 calibration curve (Stuiver et al., 2021). Layers of tephra and terrigenous gravel, as well as eventual reworked horizons, were considered as deposited instantaneously/flash events to avoid errors in the estimation of accumulation rates. The deposition of large amounts of tephra in both the lake and the catchment area can strongly modify the available amount of sediment that might be deposited in the lake. Therefore, we used a low value for the memory of the system. The reddish muddy sediments present between 640 and 623 cm of core depth together with the significant sediment age difference just before (14.12 cal kyr BP) and just afterwards (10.7 cal kyr BP) have been interpreted as temporal lake dry-up and incipient soil formation. Therefore, the top of these reddish sediments was considered as indicative of the existence of a hiatus. Due to model dating uncertainties, ages were rounded to whole numbers, i.e., to tens of years.
Results
Core Analysis and Facies Description
Three sedimentary facies were defined for the sequences of Lagoa da Lomba based on textural, lithological, and biological features (Figure 3A):
1) Organic-rich fine grained lacustrine facies (F1): This facies is composed of a dark-brown organic-rich mud, with high contents of organic matter (ca. 65%) and abundant bioclasts (ca. 30%, e.g., diatoms, sponge spicules and chironomids remains) with a small (ca. 5%) amount of dispersed lithoclasts and minerals, ranging in grain size from silts to very fine sands (∼20–100 µm).
2) Terrigenous/volcaniclastic fine to coarse grained facies (F2): This facies is mainly made up of clayey-silts to fine sandy-silts and well-sorted, sub-rounded gravel layers, with variable amounts of organic matter and varying degrees of oxidation. The lithoclastic and mineral fraction (ca. 60–80%) consists of volcanic lithics (lava fragments and weathered scoria clasts), as well as loose plagioclase, olivine, and pyroxene crystals. The organic component (ca. 20–40%) is essentially made up of amorphous organic matter with almost no bioclasts and particulate organic matter (both not exceeding 1%). Gravel levels are in most cases supported by a dark-brown muddy matrix, in some cases with dispersed plant macro-remains. Occasionally, the distinction between gravel and tephra layers was only possible using backscatter electron images (BSE), which helped to identify (or not) the presence of glass shards (e.g., LB17-06 gravel layers, Figures 3B–D).
3) Volcanic facies (F3): This facies corresponds to layers of tephra, which were labeled according to the core number and the sequential number of occurrence in the core (e.g. LBx-Tx), from base to top (Figure 3A). Thicknesses of tephra layers vary from 7.9 to 58.5 cm, and all consist of clast-supported scoria, fine to medium lapilli, with variable amounts of olivine (Ol) and plagioclase (Pl) crystals. Tephra layers are relatively homogeneous in terms of grain size, excepting LB1-T3 and LB8-T1 layers, which exhibit a very similar textural pattern, with grain size showing slightly normal to reverse symmetric grading from medium-to-fine lapilli and again back to medium lapilli, i.e., both tephra layers display a tenuous distinctive horizon (∼5 cm thick) of fine lapilli in the middle or in the upper third of the layer. Although some tephra layers are almost exclusively composed of juvenile clasts with pristine morphologies (i.e., very angular, lunate, bubble-wall and highly vesicular shapes, Figure 3B), others contain blocky, dense, poor vesiculated juveniles and occasional to frequent lithic clasts, sometimes reaching up to 50 vol% (e.g., Figure 3C). It is worth noting that of the ten individual tephra layers recognized, only two layers (LB1-T1 and LB1-T2) are exclusively composed of juvenile material (scoria lapilli), with all other layers exhibiting variable amounts of lithics. Except for tephra layers from LB17-08 core, which show a significant degree of oxidation, tephras from the other cores are well preserved. A detailed description of the textural features and components of the tephra layers identified on each core is summarized in Table 1.
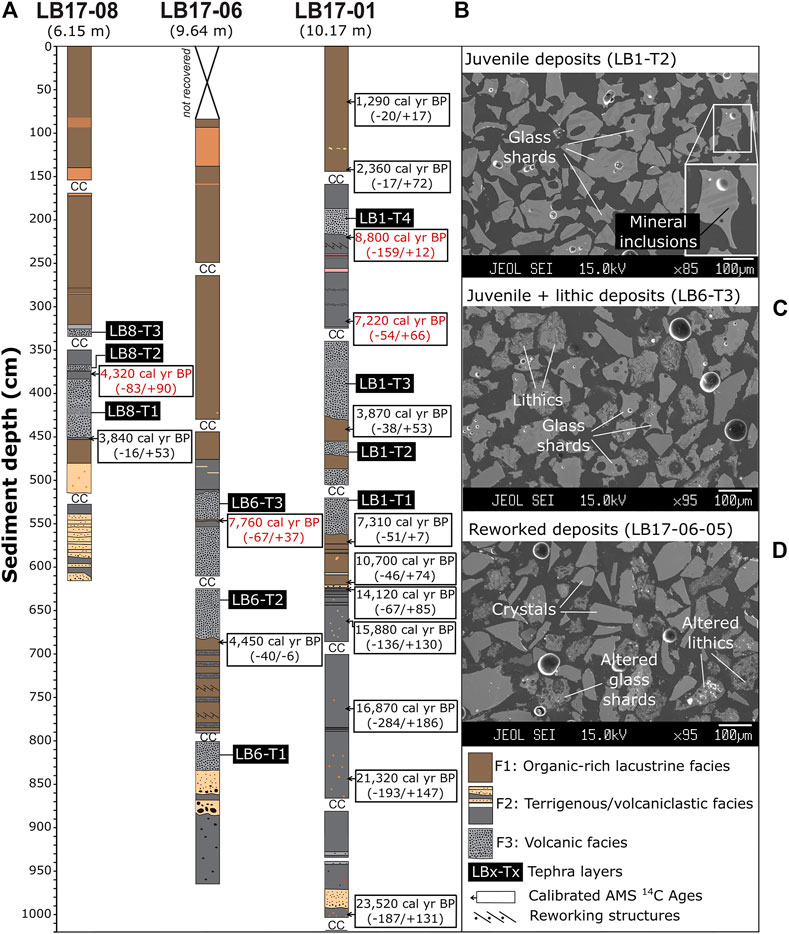
FIGURE 3. (A) Sediment logs of the core sequences collected at Lagoa da Lomba. Three facies are differentiated using texture, components, and volcanic origin. CC represents the potential length of the sediments retained by the core catcher. White boxes show the position of the obtained radiocarbon ages. Reversal AMS 14C ages are highlighted in red. Dark squares identify each tephra layer. BSE images showing (B) purely juvenile clasts with typical angular, lunate, bubble-walled and highly vesicular shapes (e.g., LB1-T2) (C) juvenile and lithic clasts (e.g., LB6-T3), and (D) altered lithic clasts and crystals (e.g., gravel, LB17-06).
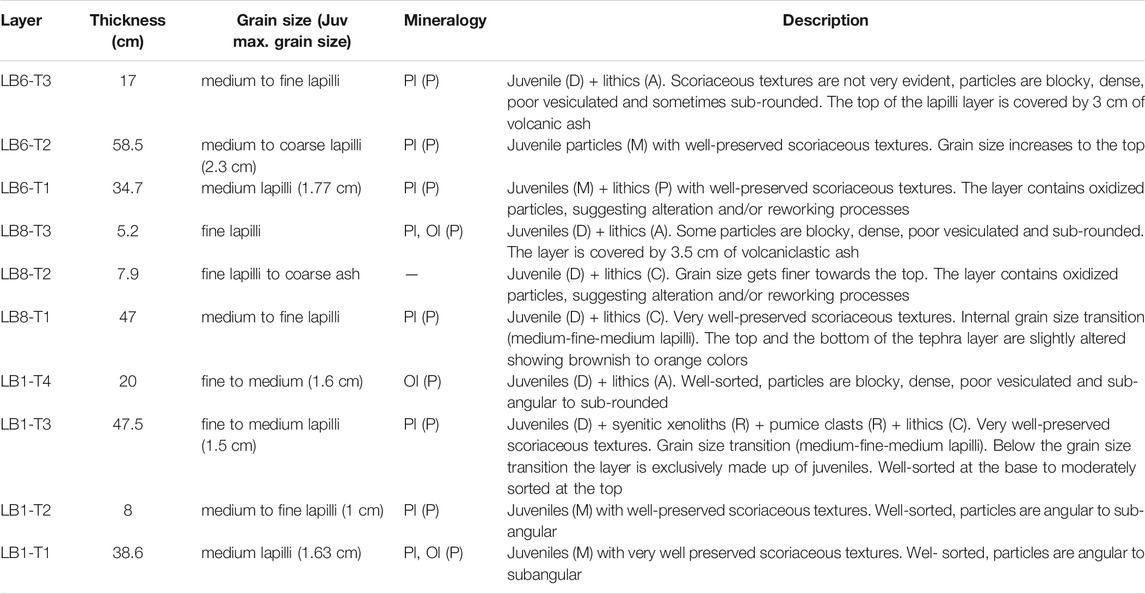
TABLE 1. Description of tephra layers from lacustrine sequences recovered at Lagoa da Lomba. M = major (>80%), D = dominant (50–80%), A = abundant (20–50%), C = common (5–20%), P = present (1–5%) and R = rare (0.1–1%). Pl = plagioclase, Ol = olivine.
Sedimentological and stratigraphical analyses of the three studied cores reveal that lake sediment deposition was in general continuous, except for the hiatus considered at 623 cm of LB17-01 sequence, and some intervals that exhibit reworking features (Figure 4):
1) Between LB1-T3 and LB1-T4, where we found reworked deposits (small mass movement deposits) composed of volcaniclastic clayey silts intercalated with thin beds of dispersed volcaniclastic sand and a few lenses of a light brown volcaniclastic clay. Bioclasts and organic matter are absent in this interval.
2) Between LB6-T1 and LB6-T2 some reworking structures are present, accompanied by thin mass movement deposits of volcaniclastic sand intercalated with biogenic clays. Between LB6-T2 and LB6-T3 there is a thin lens (5 cm) of volcaniclastic clayey-silts. This lens seems to be a sediment blob incorporated in the tephra deposit. The uppermost 2 cm of the lens consist of a biogenic clay, composed almost exclusively of diatoms and plant remains. Volcaniclastic material at the top of LB6-T3 seems to be resedimented material from the tephra layer itself, including transported sediments from the lake slopes.
3) The LB8-T1 and LB8-T2 tephra layers are separated by a thin sediment lens (7 cm-thick) of volcaniclastic material. It exhibits rounded edges suggesting this could be a sediment blob interbedded in the tephras.
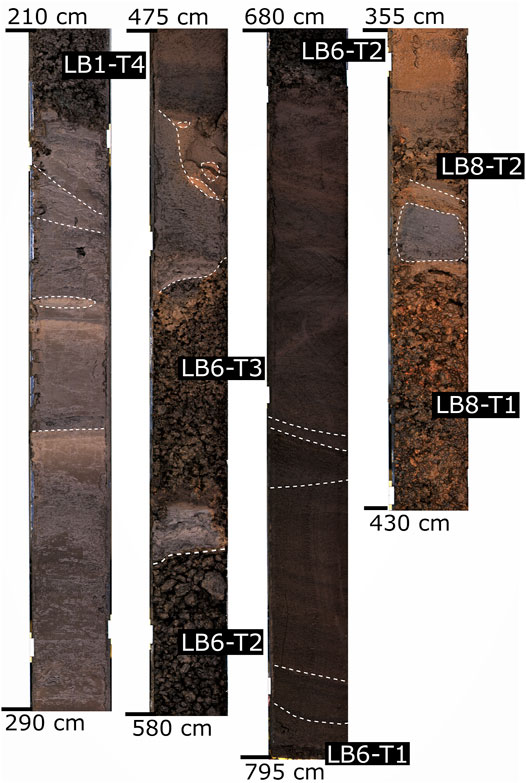
FIGURE 4. Photographs of selected core sections showing reworking structures (erosional surfaces, variation of the horizontality of the layers, small mass movement deposits and sediment pods). Sediment depth is specified at the top and at the bottom of each section. Dark rectangles indicate the nomenclature of each tephra layer.
Chronology
Radiocarbon geochronology shows that the sedimentary record of Lagoa da Lomba extends back to the late Pleistocene, as attested by the 23,520 (−187/+131) cal yr BP age obtained at the base of core LB17-01 (Figure 3A). The twelve AMS 14C dates from LB17-01 core (Table 2) are for the most part stratigraphically coherent, with only two reversals dated as 8,800 (−159/+12) and 7,220 (−54/+66) cal yr BP (Figure 3). It is assumed that such ages were imprinted by local reworking processes, which is supported by the presence of small mass movement deposits described in Core analysis and facies description. Moreover, since these two dates correspond to sediments trapped between tephra layers, punctual contributions of volcanic CO2 might have apparently aged the radiocarbon dated material. The same could be for overturned ages at LB17-06 and LB17-08 cores, where reversed radiocarbon ages also seem to be imprinted by reworking processes and related to older sediment ages in sediment blobs between tephra layers (Figure 3A). Since there are no signs of reworking at the base of LB8-T1 tephra layer, the reliability of the 3,840 (−16/+53) cal yr BP age is considerably high. Moreover, this age is compatible with the age obtained at a similar layer of LB17-01 (LB1-T3), that is dated to 3,870 (−38/+53) cal yr BP, suggesting a presumable correlation between these two tephra layers.
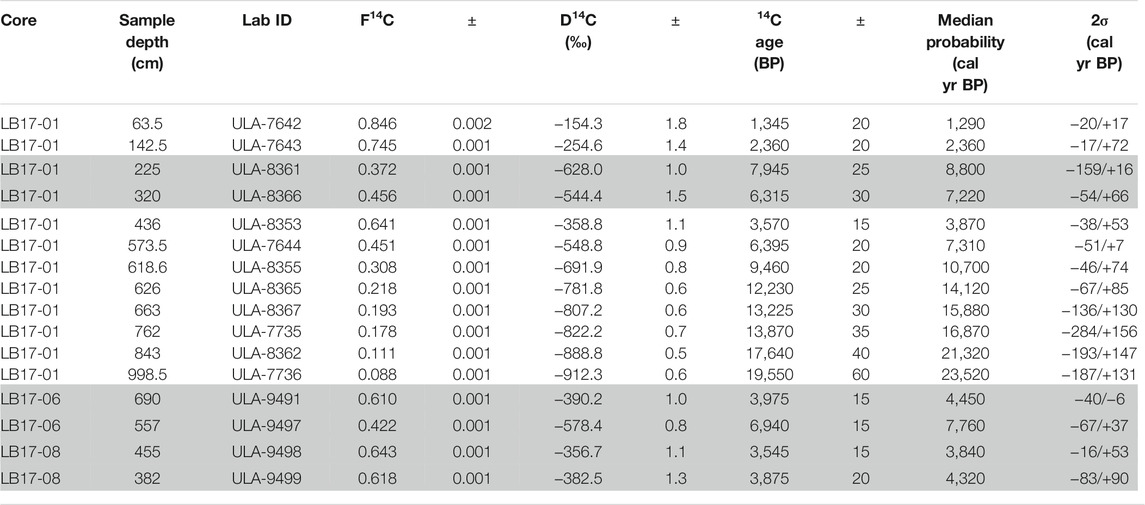
TABLE 2. Radiocarbon dates and calibrated ages for Lagoa da Lomba sediment cores. Shaded entries represent the ages not included in the construction of the depth-age model.
Except for the two reversals at 8.8 and 7.22 cal kyr BP, all the other AMS 14C ages obtained for LB17-01 were used to construct the Lagoa da Lomba age-depth model (Table 2). The age model curve is staircase-shaped between 0 and 6,280 (−578/+626) cal yr BP due to several tephra layers and reworked sediments, showing that sedimentation rates were much higher during periods of volcanic activity. In fact, ∼40% of the sedimentary sequence was deposited over the 3 kyr during which volcanic eruptions occurred at Flores, while the remaining 60% was deposited over 20 kyr (Figure 5). Based on the age-depth model, we estimate a precise age for the three oldest tephra layers, LB1-T1, LB1-T2 and LB1-T3, which have been deposited at 6,280 (−578/+626), 4,990 (−773/+685) and 3,660 (−210/+194) cal yr BP, respectively (Figure 5). Due to the reworked character of the sediments below LB1-T4, we cannot assign a precise age to this tephra layer. However, since LB1-T4 is conformably overlain by lacustrine sediments dated at 2,360 (−17/+72) cal yr BP (see Figure 3), we can constrain its age to the 3,660 (−220/+174) – 2,360 (−17/+72) cal yr BP interval (Figure 5).
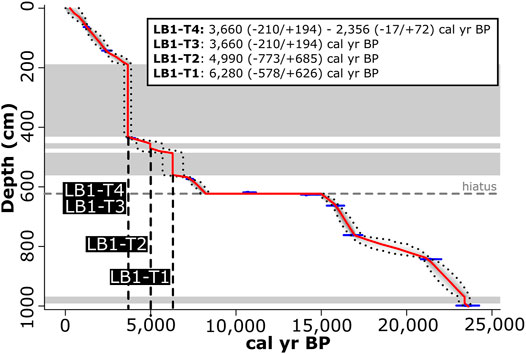
FIGURE 5. Age depth model for Lagoa da Lomba constructed with the R rbacon function (Blaauw et al., 2021), using the AMS 14C radiocarbon dates. The red line represents the age-depth function and dark doted lines correspond to the error of the model. The small blue traces indicate the AMS 14C radiocarbon dates used as anchor points and their uncertainties. Light grey bands represent abrupt events of sedimentation (tephra accumulation), which were not used to calculate the age model. A hiatus was considered at 623 cm depth. Vertical dashed lines are projecting the age obtained at the base of LB1-T1, LB1-T2, and LB1-T3, and LB1-T4 tephra layers, which are indicated on the top right box.
Geochemistry of Lacustrine Tephra Layers
Glass shards of scoriae from the tephra layers defined at Core analysis and facies description are characterized by abundant mineral micro-inclusions (Figure 3B). Glass compositions are quite homogeneous, with 45–50 wt% of SiO2 and 4–8 wt% of Na2O + K2O, mainly lying on the boundary between the trachybasalt and the tephrite/basanite fields. A group of three slightly more primitive compositions, namely samples from LB1-T4, LB6-T3, and LB8-T3 layers, which correspond to the uppermost tephra layer at each core, lay close to the triple junction of the basalt – trachybasalt – tephrite/basanite fields (Figure 6).
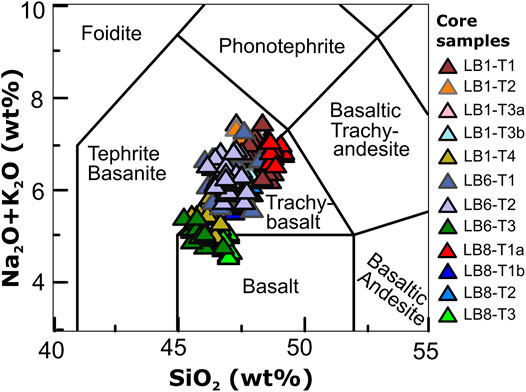
FIGURE 6. Total alkalis vs silica (TAS) diagram (Le Bas et al., 1986) for the classification of glasses of Lagoa da Lomba tephra layers. Glasses are normalized to 100% volatile free. Compositions cluster around the transition of the basalt, trachybasalt and tephrite/basanite fields.
Variation diagrams for the major elements vs CaO show three main geochemical populations, demonstrating that some tephra layers are geochemically distinct from others (Figure 7). These populations follow a general geochemical trend, with more primitive compositions towards the top of the sedimentary sequences, demonstrated by the increase in MgO and decrease in SiO2 contents. LB1-T3 and LB8-T1 are the only layers showing an internal geochemical variation, which coincides with a granulometric transition mentioned in the volcanic facies description (Core analysis and facies description).
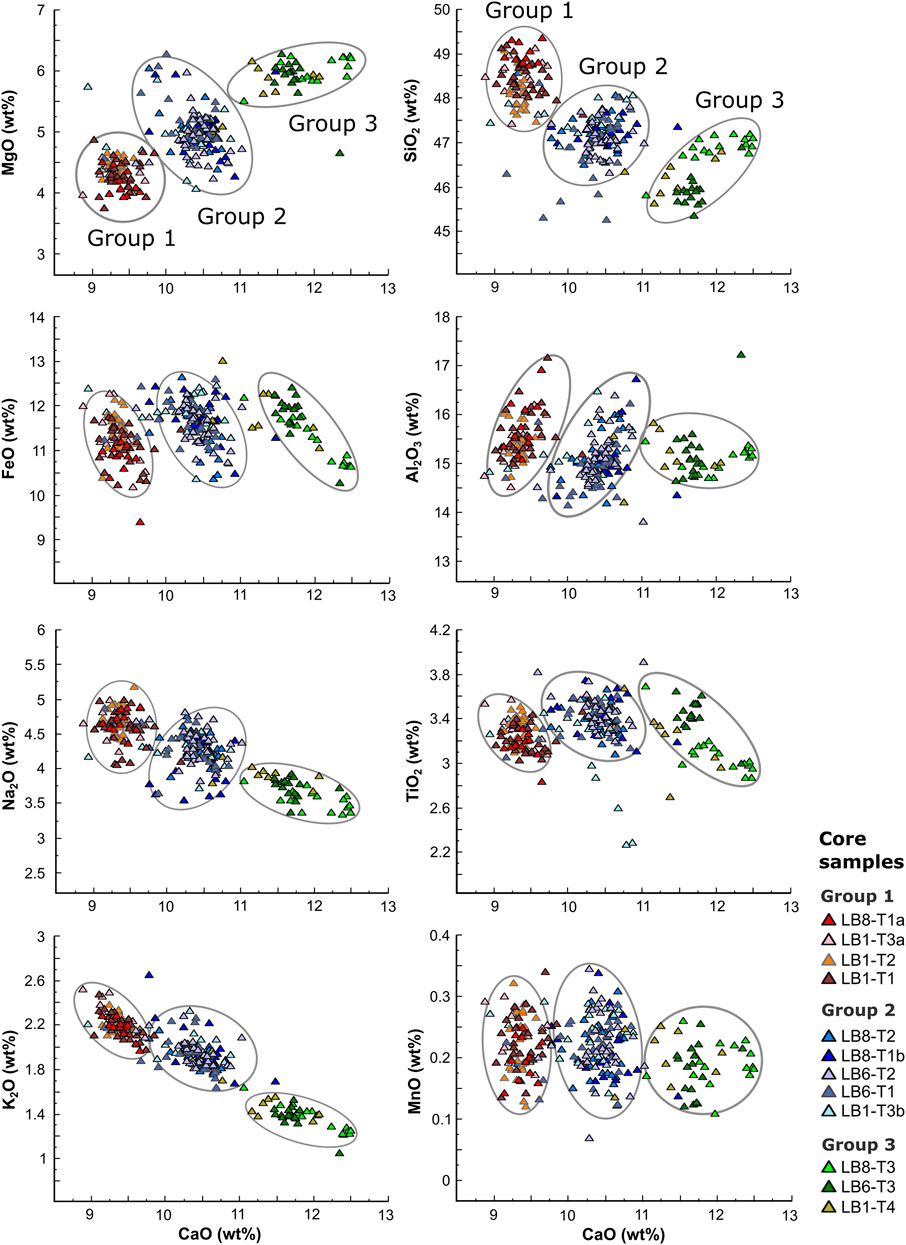
FIGURE 7. Variation diagrams of major elements (wt%) vs CaO (wt%) for the glasses of Lagoa da Lomba tephra layers. Glasses are normalized to 100% volatile free. Glass compositions plot in three different geochemical populations, with decreasing degree of evolution towards the most recent tephra layers.
Geochemical Group 1 includes LB1-T1, LB1-T2, LB1-T3a, and LB8-T1a tephra layers, and consistently has the lower CaO contents, varying between 9 and 9.8 wt%. Geochemical Group 2 has intermediate CaO contents, from 9.8 to 11 wt%, and includes LB1-T3b, LB8-T1b, LB8-T2, LB6-T1, and LB6-T2 layers. Finally, geochemical Group 3 has the higher CaO contents, from 11 to 12.5 wt%, and comprises the uppermost tephra layer of each core, which includes LB1-T4, LB6-T3 and LB8-T3 layers (Figure 7).
Characterization of Subaerially-Exposed Pyroclastic Sequences
Four field sites deemed the most representative of the recent volcanic stratigraphy of the study area were established (see Figure 8A) and studied in detail.
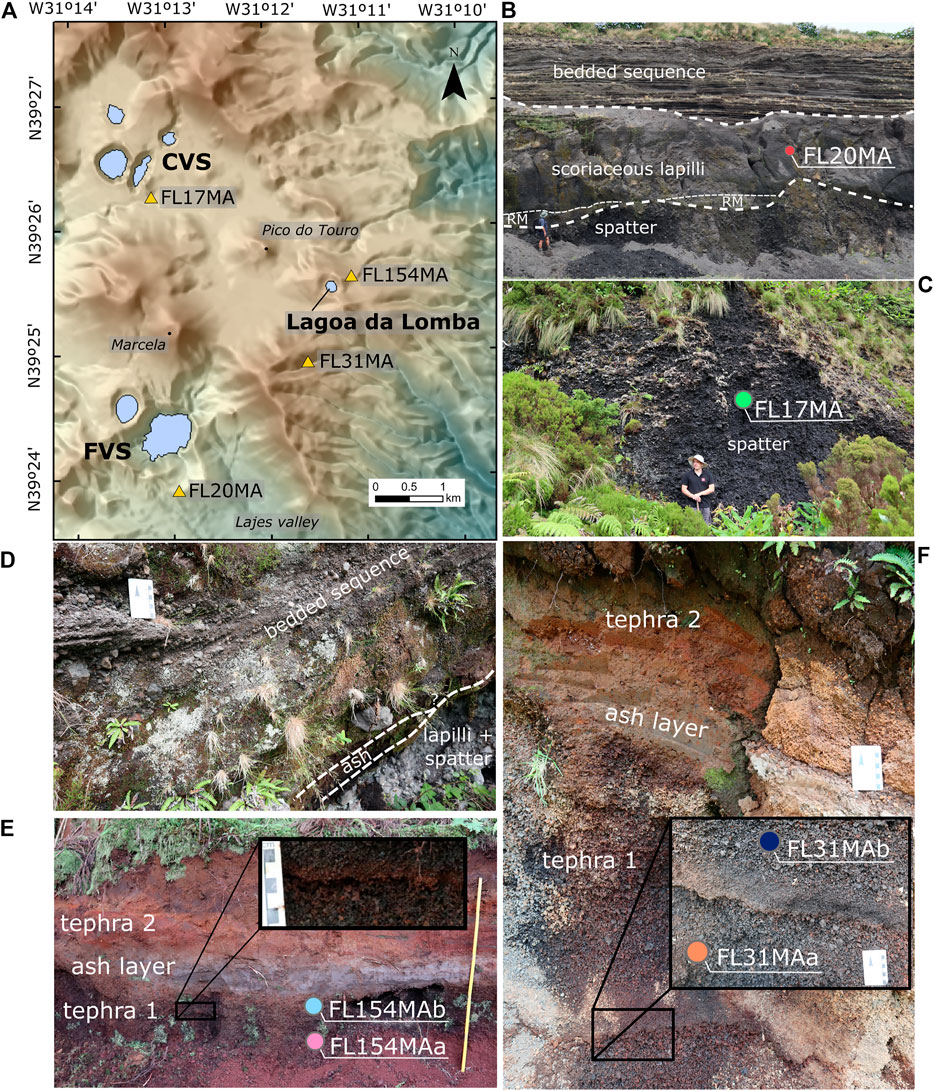
FIGURE 8. (A) Elevation map of Flores Island showing the location of Lagoa Funda and Lagoa Comprida Volcanic Systems (FVS and CVS, respectively) in relation to Lagoa da Lomba, as well as the location of four subaerially-exposed pyroclastic sequences used to investigate the origin of the tephra layers of Lagoa da Lomba lacustrine record (B) Photo of a proximal volcanic sequence on the SE rim of Lagoa Funda (FL20MA: N39°23′53.74″ W31°12′57.17″). Volcanic succession consists, from the base to the top, on spatter accumulation, remobilized material (RM), scoriaceous lapilli and lithic-rich sequence of ash intercalated with lapilli, bombs, and blocks (phreatomagmatic) (C) Photo of an outcrop of spatter and scoria produced during CVS eruptions. The volcanic sequence is located on the SE flank of Lagoa Comprida cone (FL17MA: N39°26′14.89″ W31°13′10.99″) (D) Photo of the upper part of the scoria cone produced during CVS eruptions showing that it is overlapped by a sequence of intercalated very fine ash layers and lithic lapilli and blocks layers (phreatomagmatic). Scale is 7 cm (E) Photo of the sequence located ∼200 m NE of Lagoa da Lomba (FL154MA: N39°25′34.86″ W31°11′8.12″). Shows an internal grain size transition on Tephra 1 deposit, which is similar to the one found at LB1-T3 tephra layer. On FL154MA sequence, this transition is accompanied by a thin oxidation crust. Scale is 1 m (F) Photo of the sequence located half-way between Lagoa da Lomba and FVS (FL31MA: N39°.24′54.04″ W31°11′35.02″). Shows a lapilli tephra deposit with the grain size transition and oxidation crust that characterizes tephra 1 deposit from FL154MA site and LB1-T3 layer. Scale is 7 cm.
FL20MA field site (N39°23′53.74″ W31°12′57.17″) corresponds to a quarry located on the southern flank of FVS, just below the rim of the southernmost crater, where a very proximal volcanic sequence crops out (Figure 8A). This quarry constitutes one of the best tephrostratigraphic exposures on the island and is a crucial site to reconstruct the stratigraphy of this volcanic system. In this exposure (Figure 8B), the basal unit consists of welded spatter accumulation that is >1.70 m thick. Large bombs with well-preserved fluidal shapes are observed in this unit, most of them containing large crystals of plagioclase. The spatter is overlapped by a unit of remobilized material, with variable thickness up to 80 cm (Figure 8B). The remobilized material is followed by a thick (>2.5 m) unit of scoriaceous coarse lapilli, also with well-preserved fluidal shapes and common olivine and plagioclase crystals. Overlapping these scoriae, there is a very thick (∼15 m), well-bedded sequence, heterogeneous in composition, made up of very fine ash layers, in places with accretionary lapilli, intercalated with ash layers rich in lithic clasts, up to coarse lapilli and blocks (Figure 8B). Juveniles are rare along these sequences and when present are usually dense, sub-rounded and blocky. Bomb sag structures are frequent and deform the underlying layers, wavy and cross-stratification is often present within and between fine-grained subunits. These features yield for a phreatomagmatic nature of the sequence. Glass compositions of the scoriaceous lapilli overlap with geochemical Group 1 of Lagoa da Lomba sedimentary record (Figure 9).
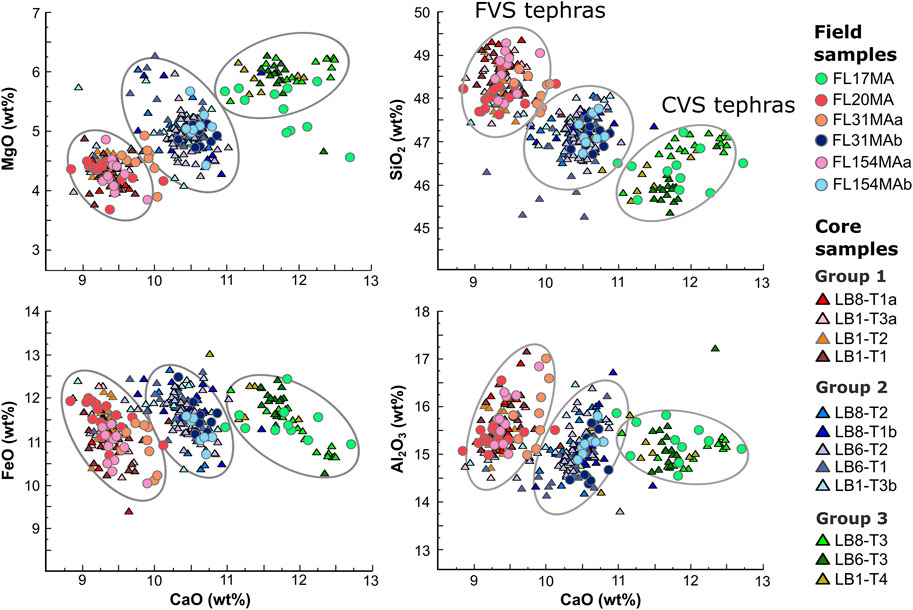
FIGURE 9. Variation diagrams of major elements (wt%) vs CaO (wt%) for the glasses of Lagoa da Lomba tephra layers and subaerial tephra deposits on Figure 8. Glasses are normalized to 100% volatile free. Glass compositions of subaerial deposits fit well in the three geochemical groups defined for Lagoa da Lomba lacustrine record. Geochemical Group 1 correlates with Lagoa Funda Volcanic System (FVS) deposits and Group 3 with Lagoa Comprida Volcanic System (CVS) deposits. The internal geochemical variation described for LB1-T3 and LB8-T1 tephra layers is also found on FL154MA and FL31MA deposits, with samples below the oxidation crust (FL31MAa and FL154MAa) lying on geochemical Group 1 and samples collected above the oxidation crust (FL31MAb and FL154MAb) fitting in geochemical Group 2.
The FL17MA field site (N39°26′14.89″ W31°13′10.99″) corresponds to an abandoned quarry located on the southeast flank of Comprida scoria cone (Figures 8A,C). The outcropping sequence (∼4 m-thick) consists of welded spatter accumulation showing a normal grading pattern, ranging from block-sized clasts at the base to lapilli-sized scoria and coarse scoriaceous ash towards the top. Although it is essentially made up of juvenile material, it contains occasional lithic blocks (up to 20 cm across), as well as syenitic xenoliths within the spatter. The upward decrease in the grain size of juvenile clasts is accompanied by a gradual increase of the lithic clast content. The Comprida sequence ends with a 1.70 m-thick, well-bedded, succession of fine ash layers intercalated with ash layers rich in lithic clasts, up to coarse lapilli and blocks (phreatomagmatic nature; Figure 8D). The sequence contains parallel to subparallel stratification, sometimes with wavy and cross-stratification (Figure 8D) and the ash layers frequently contain accretionary lapilli. Variable amounts of olivine and plagioclase crystals are present along the sequence. Glass compositions of scoria lapilli overlap with geochemical Group 3 of Lagoa da Lomba sedimentary record (Figure 9).
Outcrop FL154MA (N39°25′34.86″ W31°11′8.12″), located on the NE flank of Lagoa da Lomba (Figure 8A), consists of a subaerial stratigraphic sequence identical to that observed in core LB17-01. It contains two tephra deposits (Tephra 1, with 25 cm of thickness; and Tephra 2, with 93 cm of maximum thickness) separated by a thin (10 cm thick), weathered, very fine ash bed; Figure 8E). Tephra 1 exhibits a sharp grain size variation of scoria clasts from medium-to-fine lapilli, and back to medium lapilli towards the top. This grain size transition is accompanied by a thin oxidation crust where the fine lapilli are present. The glass compositions of the medium lapilli-sized scoriae below the oxidation crust (FL154MAa) overlap with Lagoa da Lomba geochemical Group 1, and the compositions of the scoria lapilli above the oxidation crust (FL154MAb) overlap with geochemical Group 2 of Lagoa da Lomba (Figure 9). Unfortunately, the intense weathering of tephra 2 scoriae precluded the geochemical analysis of the glass shards.
The same pattern is observed on FL31MA field site (N39°24′54.04″ W31°11′35.02’’; Figure 8A) located ∼1 km SSW of Lagoa da Lomba. In this field site, the outcropping tephra 1 (104 cm-thick) shows a very similar grain size variation to that of tephra 1 at FL154MA, with the fine lapilli scoria and a thin oxidation crust in the middle of the deposit (Figure 8F). Moreover, scoria lapilli above the crust (FL31MAb) yielded glass compositions that overlap with that of geochemical Group 2 of Lagoa da Lomba, whereas the scoriae below this horizon (FL31MAa) fit well with geochemical Group 1 (Figure 9). As well as in site FL154MA, the scoriae of tephra 1 from site FL31MA are overlapped by a 10 cm-thick fine ash bed, in turn covered by another scoriaceous tephra layer, tephra 2, which is 15 cm-thick (Figure 8F). As well as in site FL154MA, these scoriae are intensively weathered, making impractical the geochemical analysis of glass shards.
Discussion
Interpretation of Lagoa da Lomba Facies and Correlation Between Tephra Layers
Based on the distinctive features of the facies identified in Lagoa da Lomba, we propose different interpretations for their origin:
1) The muddy organic lacustrine facies (F1) was deposited during periods of volcanic quiescence, incorporating significant amounts of organic matter, mainly from lake biogenic production as well as fine clastic sediments from runoff on catchment during regular rains.
2) The terrigenous/volcaniclastic facies (F2) was deposited during periods of intense clastic inputs in the lake due to an increase of flood frequency and/or to an increase in the availability of materials in the basin catchment (either by intense soil weathering or deposition of pyroclastic fall deposits in the surroundings of Lagoa da Lomba).
3) The volcanic facies (F3), which includes all the tephra layers, are interpreted as pyroclastic fall deposits, thus representing periods of volcanic activity on the island. This interpretation is based on the clast-supported nature of the tephra layers and on the pristine morphologies of the juvenile clasts (scoria lapilli). The high content of lithic clasts of some tephra layers suggests the occurrence of phreatomagmatic activity and/or the enlargement and erosion of the conduit walls (Barberi et al., 1989; Sulpizio et al., 2005; Pimentel et al., 2015). The higher degree of oxidation observed in the tephra layers of LB17-08 may be related to its lake shallow conditions of deposition.
Separation of predominantly basaltic (sensu latu) eruptive events based on major element geochemistry alone is often problematic, on account of the generally similar geochemistry of basaltic magmas derived from the same source, i.e., same island and same volcanic system (e.g., Zanon and Pimentel, 2015; Zanon et al., 2020). However, our results clearly allowed to discriminate different geochemical populations that proved to be crucial to identifying volcanic events. The distinct glass compositions of juvenile material (scoria lapilli), together with lake core stratigraphy and radiocarbon ages, allowed the establishment of consistent correlations between the tephra layers (Figure 10), as detailed below, from the most recent to the oldest.
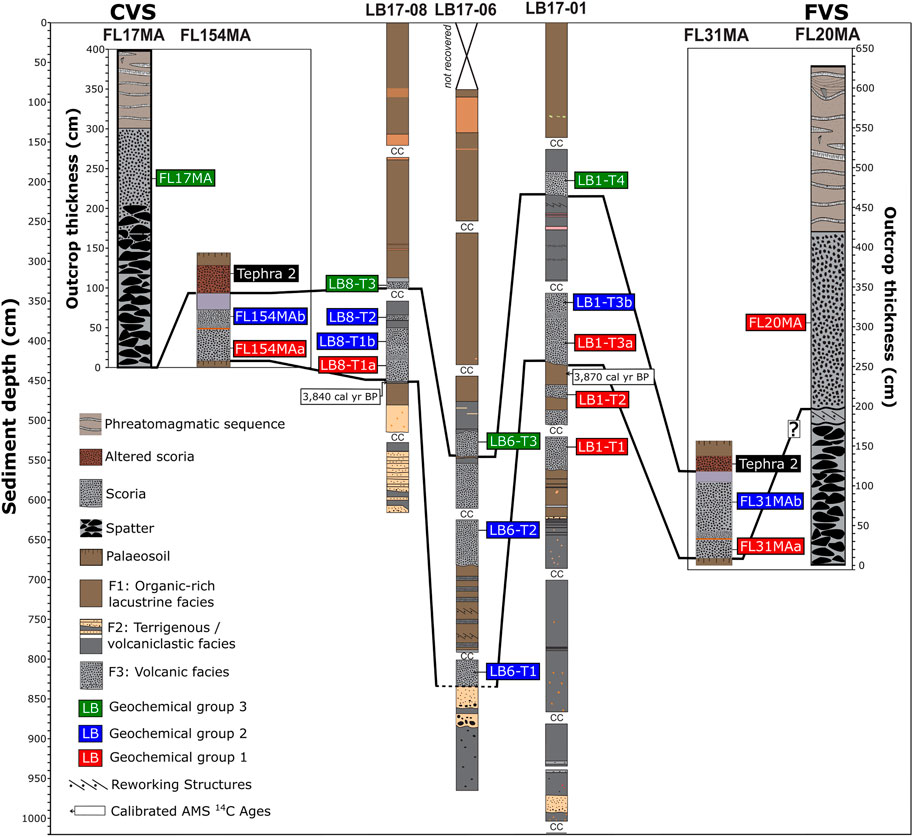
FIGURE 10. Correlation panel of the sedimentary sequences collected at Lagoa da Lomba and subaerial volcanic sequences. Red, blue and green colors indicate the geochemical Group to which each tephra layer belongs. The dashed line on the bottom of LB6-T1 tephra layer indicates a possible correlation that is uncertain. We named the tephras below and above the granulometric transition of LB8-T1 and LB1-T3 as a and as b, respectively.
We correlated the LB1-T4, LB6-T3, and LB8-T3 tephra layers (Figure 10) as corresponding to the same volcanic event based on their stratigraphic position (all constitute the uppermost tephra layer of each sequence and all lie on the top of a reworked section), but especially on their distinctive geochemical signature, which is exclusive of those tephras (the more primitive geochemical Group 3). Important to highlight is a very thin lens of organic-rich clay (F1) almost exclusively made up of diatoms and plant remains, just below LB6-T3. This suggests a period of high in-lake productivity, and therefore a short-time break in the volcanic activity immediately before the deposition of LB6-T3 tephra layer, during which lacustrine sediments were deposited in the deepest area of Lagoa da Lomba. Accordingly, LB1-T4, LB6-T3, and LB8-T3 tephra layers record the youngest volcanic eruption preserved at Lagoa da Lomba, and its distinct geochemistry may potentially be used to discriminate subaerially exposed tephras in the study area, as we demonstrate. Since all the material between LB1-T3 and LB1-T4 tephra layers is interpreted as reworked (F2), it is not possible to obtain a precise age for the most recent eruption. However, the absence of muddy and organic lacustrine deposits between these horizons suggests that the transport and sedimentation of volcaniclastic material was rapid, taking place at a much shorter time scale than regular lacustrine sediments. This rapid transport of material may have been initiated during the emplacement of LB1-T3 tephra layer either by lateral flow, creeping or just the pressure posed by the deposition of the lapilli on the water-saturated sediment. Therefore, it is assumed that the time interval between the deposition of LB1-T3 and LB1-T4 layers was considerably short. Given these considerations, and taking into account that tephra layer LB1-T4 is conformably overlain by lacustrine sediments dated at 2,360 (−17/+72) cal yr BP (see Figure 3), our data suggest that the eruption took place sometime in between 3,660 (−210/+194) cal yr BP and 2,360 (−17/+72) cal yr BP.
The subjacent volcanic event is recorded by both the LB1-T3 and LB8-T1 tephra layers, given the fact that the correlation between them is consistent. A thin sediment lens separates LB8-T1 and LB8-T2 tephra layers, which may have been emplaced by a syn-eruptive mass flow that ripped-up sediments from the lake slopes. In this case, instead of two tephra deposits, LB8-T1 and LB8-T2 would comprise a single deposit. Another possibility is that both the volcaniclastic lens and the LB8-T2 tephra layer are reworked material. Despite this, LB1-T3 and LB8-T1 tephra layers show a distinctive internal grain size variation that was not observed at any other lacustrine-hosted tephras and they are both overlain by reworked sediments (Figure 4). More importantly, they are similar in terms of glass compositions, as both show a more primitive composition at the upper part (geochemical Group 2) and a slightly more evolved composition (geochemical Group 1) at the base. Additionally, radiocarbon ages of the sediments at the base of LB1-T3 and LB8-T1 tephra layers (3,840 (−38/+53) cal yr BP and 3,870 (−16/+53) cal yr BP, respectively) are very similar, indicating that these tephras were deposited at the same time. Our age model suggests an approximate age of 3,660 (−210/+194) cal yr BP for this eruption(s).
Except for the LB6-T3 tephra layer, there is no other solid option for a correlation of the tephras from LB17-06 sequence with the LB17-01 and LB17-08 cores, as there is no characteristic grain size or geochemical transition in the tephra layers. The LB6-T2 tephra layer may not be a primary deposit, i.e., a fall deposit, but a volcaniclastic deposit that results from in-lake and/or near lake redeposition processes. This is supported by the small mass movement deposits occurring below LB6-T2, as well as by the repetition of the geochemical Group 2 on the older LB6-T1 tephra. Such repetition does not occur on LB17-01 sequence, which we assume to be continuous. Therefore, we do not expect to find a repetition of the geochemical Group 2 in the lake stratigraphy. Eventually, the top of LB6-T1 tephra layer could be correlated with the top of layers LB8-T1 and LB1-T3 given the fact that it constitutes the older deposit whose tephras lie on geochemical Group 2. However, due to its location on the central and deepest part of Lagoa da Lomba where the accumulation of sediments is higher and possibly more vulnerable to the deposition of reworked/slumped material, the Holocene period may not be completely recorded on LB17-06 sequence as it is on LB17-01. Thus, since it is not possible to establish any solid stratigraphic or geochemical correlation, we consider that LB6-T1 tephra layer most likely corresponds to remobilized tephras, which slumped downslope from a higher position. The great thickness of reworked sediment at LB17-06 core sequence may be explained by the steep subaqueous slopes of Lagoa da Lomba, which are close to 30° in some areas. This steepness promotes the flow of sediments along the slopes and consequently favors its deposition in the deepest area of the lake (see LB17-06 location in Figure 2).
In core LB17-01, two other tephra layers (LB1-T2 and LB1-T1) are interpreted as primary volcanic deposits and consequently are thought to be representative of two older eruptions, which – according to our age model – occurred approximately at 4,990 (−773/+685) and 6,280 (−578/+626) cal yr BP. These two tephra layers are very similar in composition, given that they both fall in geochemical Group 1, similarly to LB1-T3a.
In summary, the lacustrine record of Lagoa da Lomba attest to the occurrence of at least four different volcanic eruptions that took place at Flores during the Holocene (Figure 5). Furthermore, this record confirms that all four eruptions occurred within a time interval between 6,280 (−578/+626) and 2,360 (−17/+72) cal yr BP. Finally, it is worth mentioning that the 23,520 (−187/+131) cal yr BP age for the base of LB17-01 places an upper boundary for the eruption that formed the crater of Lagoa da Lomba itself, confirming it was formed during the late Pleistocene.
Correlation Between Lake and Subaerially-Exposed Sequences and Possible Sources
Considering the results and interpretations of the previous chapters, we confirm that the volcanic succession preserved at Lagoa da Lomba – and particularly that of the longest and most complete core LB17-01 – is generally consistent with the subaerially-exposed record. Moreover, the evidence presented above allows the identification of possible sources for the described eruptions, as we demonstrate below.
The youngest eruptive event – represented by LB1-T4, LB6-T3, LB8-T3 tephra layers – is recorded by the sequence of CVS (outcrop FL17MA; Figure 10), given that its geochemical signature lie within the more primitive geochemical Group 3. Despite the absence of geochemical analyses, based on their stratigraphic position we assume that this event is also recorded by tephra 2 from outcrops FL31MA and FL154MA (Figure 10). The age interval [3,660 (−210/+194) – 2,360 (−17/+76) cal yr BP] we infer for this event agrees well with the 3,050 (−206/+217) cal yr BP (2,900 ± 100 uncalibrated 14C yr BP) age proposed by Morisseau and Traineau (1985). We, therefore, confirm that the youngest volcanic eruption that took place at Flores Island was initiated from vents located in the northern volcanic cluster that includes the maars of Lagoa Comprida, Lagoa Negra, and Lagoa Seca, the Lagoa Branca tuff ring, and a few other smaller unnamed craters (Figure 1B). The sequences exposed on the flanks of Lagoa Comprida (FL17MA), suggest that this event started with a vigorous magmatic phase (Hawaiian/Strombolian style) and subsequently, transitioned (rather rapidly/instantaneously) to highly explosive phreatomagmatic activity that ultimately led to the formation of the maars and tuff rings. Such shifts of eruption style from Strombolian to phreatomagmatic were described at different volcanic islands, e.g., Fuencaliente and Tinguaton Tao volcanoes, Canary Islands (Clarke et al., 2009), Miyakejima volcano, Japan (Geshi et al., 2019), as well as in other volcanic regions, e.g., Garrinada and Montsacopa volcanoes (Martí et al., 2010), Pinacate volcanic field (Gutmann, 2002). The early magmatic phase of this eruption involved the extrusion of magma with a composition that lies at the junction of the basalt, trachybasalt and tephrite/basanite fields, being the less evolved of all magmas that fed the Holocene eruptions of Flores.
The second oldest eruptive event, in turn, is well-recorded by lake tephra layers LB1-T3 and LB8-T1, and tephra 1 of outcrops FL31MA and FL154MA (Figure 10). All these units exhibit a very distinctive petrographic and geochemical pattern characterized by a peculiar grain size variation and a composition that changed from a more evolved endmember (represented by geochemical Group 1) towards a less evolved endmember (represented by geochemical Group 2), with higher MgO contents and lower SiO2, CaO, and Na2O. This characteristic pattern provides a valuable tool to discriminate this event in the field, facilitating the identification of its source. Effectively, a preliminary field survey showed that the spatial distribution, thickness variation, and the size of the larger clasts of this deposit found within a large area between Lagoa Funda and Lagoa da Lomba, as well as to the east of Lagoa da Lomba, suggest that its source is located at FVS.
A comparison of glass compositions between lake-hosted tephra layers and scoriaceous lapilli at outcrop FL20MA (located in the southern flank of FVS) confirms that these overlap with LB1-T3a and LB8-T1a within geochemical Group 1 (Figure 9), with geochemical Group 2 being absent from this field sequence. The presence of remobilized material below the lapilli unit (Figure 8B), may suggest a time break in the eruption characterized by geochemical Group 1 (or probably two eruptions in close succession). Regardless of its absence, Group 2 is interpreted as an internal compositional variation of the deposit rather than a deposit from a different, individual eruption (or eruption phase). The grain size variations may either result from changes in the wind direction and/or velocity or from a decrease in the eruption rate, resulting in the deposition of finer material closer to the vent location (Carey and Sparks, 1986). In turn, the geochemical variation may result from the initial eruption of a more differentiated magma, stored at the upper part of the magmatic reservoir, followed by less evolved composition, which may have suffered (or not) an injection of new magma. Additionally, such internal changes in the deposit coincide with an increase in the lithic content, which may indicate an enlargement of the crater and erosion of the conduit walls (Sulpizio et al., 2005) and/or the initiation of a phreatomagmatic pulse (Barberi et al., 1989; Pimentel et al., 2015). This transition between contrasting eruptive styles, i.e., “dry” magmatic to “wet” phreatomagmatic, is dominantly controlled by variations in degassing patterns, magma ascent rates and degrees of interaction with external water (Houghton et al., 1999; Gutmann, 2002; Geshi et al., 2019). In an early stage, high rates of magma ascent may have inhibited groundwater from entering the conduit. Later, groundwater may have entered the system as a response of a widening of the conduit and/or a decrease in the eruption rate. This, would increase the explosivity of the eruption, resulting in the optimization of magma fragmentation, highlighted by the rapid decrease in grain size that characterize LB1-T3 and LB8-T1 deposits, as well as tephra 1 from FL154MA and FL31MA sites.
We infer that the second oldest eruptive event recorded at Lagoa da Lomba was sourced at FVS (Figure 10), and the absence of geochemical Group 2 in the proximal deposits is possibly due to an incomplete record at FL20MA. According to our age model, we estimate that this eruption was initiated at ca. 3,660 (−210/+194) cal yr BP, similar to the 3,360 (−283/+219) cal yr BP (3,150 ± 100 uncalibrated 14C yr BP) age suggested by Morisseau and Traineau (1985). Moreover, the sequence at FL20MA also suggests that this eruption experienced a rapid transition from an initial magmatic phase (Hawaiian/Strombolian style) to phreatomagmatic activity, ultimately leading to the formation of the maar of Lagoa Funda and the tuff ring of Lagoa Rasa and other adjacent unnamed craters (Figure 1B). The occurrence of phreatomagmatism is supported by the internal structure of the deposits, with parallel to subparallel stratification, in places gently undulated, the presence of very fine-grained ash layers, occasionally containing accretionary lapilli, and the high lithic content of some layers, which often show bomb sag features (Barberi et al., 1989; De Rita et al., 2002; Pimentel et al., 2015).
Due to limitations on the outcrop exposure, including high erosion rates of subaerial pyroclastic deposits on volcanic islands, the products of the two older eruptions (recorded by LB1-T1 and LB1-T2 tephra deposits) were not observed on land. For this reason, we were not able to assign the source of those eruptions. Based on the age-depth model, the eruptions occurred with an interruption of approximately 1,000 years, occurring between 6,280 (−578/+626) cal yr BP and 4,990 (−773/+685) cal yr BP. It is, however, important to highlight the difference in the products between these two older eruptions and the two most recent ones. While CVS and FVS tephras have a high lithic component, the deposits of the older eruptions are exclusively made up of scoria lapilli and ash (glass shards), and a few loose crystals. This may attest to the later phreatomagmatic behaviour of CVS and FVS eruptions, which contrasts with the older eruptions, which probably were exclusively magmatic. This is supported by the thick phreatomagmatic deposits that cap the sequences at both volcanic systems, which are directly associated with the formation of the visible maars/tuff rings that host the lakes. Based on this, we suggest that such deposits could have resulted from the eruption of monogenetic scoria cones described by Azevedo and Portugal Ferreira (2006), e.g., Pico do Touro or Marcela cones, which are the two closest volcanic sources to Lagoa da Lomba (Figure 8A). Another possibility would be that these events have been sourced from older vents at FVS, given that the sequence at FL20MA site suggests the occurrence of earlier magmatic, largely effusive events, such as the one that emitted the young lava flows that flowed down the adjacent Lajes valley (see Figure 8A for location) – a hypothesis to be confirmed in future studies.
The Holocene Volcanism of Flores and Hazard Implications
Compared to other islands of the Azores Archipelago, Flores Island lies on a more stable tectonic environment and did not experience historical eruptions but hitherto its volcanic history is still poorly studied. Until now, available ages for the Holocene eruptions at Flores are constrained to the 2.9 – 3.0 ka interval (Morisseau and Traineau, 1985). However, the lacustrine tephrostratigraphy described here shows that this period was considerably longer, extending at least up to ca. 6.7 ka ago.
During the Holocene period, at least four eruptions were recorded in Lagoa da Lomba lacustrine sequence. The most recent eruption was related to CVS and the second most recent related to FVS, with two earlier events from yet unknown sources. In terms of composition, there is no great variability amongst the Holocene eruptions, all being basaltic to trachybasaltic in composition. However, glass geochemistry of the erupted products shows a trend towards a more primitive basaltic composition as we move up in the stratigraphy. This suggests the gradual activation of a different magma feeding system over time.
Although the eruptive frequency was relatively low during the Holocene, Flores is an active volcanic island, and the possibility of future eruptions should not be ruled out. Given the small size of the island, remote geography and lack of adequate monitoring networks, the reconstruction of its volcanic history, including the size and style of the eruptions, assumes paramount importance for the development of long-term hazard assessment strategies. Our dataset demonstrates that different eruptive styles have occurred throughout the Holocene, with evidence of magmatic and phreatomagmatic activity. Phreatomagmatic eruptions may be highly hazardous phenomena, especially in basaltic systems where a sudden and unexpected shift of eruption style can transform a mild effusive eruption into a violent explosive event (Geshi et al., 2019). A future eruption on Flores with similar characteristic to the last two events (CVS and FVS) could have a devastating impact on the island and its 3,600 inhabitants. If a future eruptive event would occur, tephra fallout would probably affect all the central part of the island and could reach the inhabited coastal areas depending on the wind direction. In proximal areas, associated volcanic hazards would likely include ballistic projectiles (bombs), pyroclastic density currents, lahars and gas emissions. Ongoing studies are currently being undertaken to carefully access the long-term volcanic hazard at Flores Island.
Conclusion
Core sequences collected at Lagoa da Lomba (Flores Island) provide valuable insights to reconstruct the recent volcanic history of Flores Island. Based on tephrostratigraphy supported by glass geochemistry and radiocarbon ages, we correlated lacustrine-hosted tephras with subaerially-exposed sequences from key outcrops, resulting in a robust reconstruction of the eruptive events that took place in central part of Flores Island during the Holocene. Our reconstructions confirm that at least four eruptive events occurred at Flores, within a period comprising 2.36 and 6.28 cal kyr BP. The youngest volcanic event was sourced from the Lagoa Comprida Volcanic System (sometime between 2.36 and 3.66 cal kyr BP), and the second most recent was sourced at the Lagoa Funda Volcanic System, approximately at 3.66 cal kyr BP. Both events seem to have started with vigorous magmatic activity, of Hawaiian/Strombolian style, but rapidly changed to highly explosive phreatomagmatic activity. Two older eruptive events (6.28 and 4.99 cal kyr BP), exclusively magmatic in nature, are evident by deposits preserved on the Lagoa da Lomba sedimentary record but are not exposed onland. Our observations reinforce the importance of tephrostratigraphy in lake records to reconstruct past volcanic activity of volcanic islands, especially on small and highly vegetated islands like those of the Azores Archipelago. Moreover, the use of geochemical tools (glass compositions) proved to be very useful to confirm and establish stratigraphical correlations, opening a pathway for more detailed field studies. We also demonstrate that Flores experienced an intense period of volcanic activity during the Holocene, that extended longer than previously known. Therefore, additional work is needed to better understand the past volcanism of the island and ultimately assess the possibility of future eruptions and the associated hazards. Finally, these new insights into the Holocene history of Flores show that the island went through a vigorous volcanic activity during the Late Holocene, and therefore, contrary to what is typically assumed, the possibility of future eruptions and associated hazards should be properly considered.
Data Availability Statement
The original contributions presented in the study are included in the article/Supplementary Material, further inquiries can be directed to the corresponding author.
Author Contributions
MA, RSR, AP, and AH undertook the field work; AH, AS, PMR, and SG collected the sediment cores within the remit of the project PALEOMODES; MA, AH, AS, MB, and SG performed all core description and analyses; MA and SK performed all glass shard geochemical analyses and subsequent data processing; and MA led the writing of the manuscript with contributions from all authors.
Funding
MA acknowledges the financial support given through her doctoral grant funded by Fundação para a Ciência e Tecnologia (SFRH/BD/138261/2018). The acquisition of sediment cores was funded by the Spanish Ministry of Economy and Competitiveness through the research project PaleoModes (CGL 2016-75281-C2). The radiocarbon dating was partially funded by FCT through the research project DISCOVERAZORES (PTDC/CTA-AMB/28511/2017), PaleoModes and RapidNAO (CGL 2013-40608-R). RSR and PMR acknowledge their IF/01641/2015 and DL57/2016/ICETA/EEC 2018/25 fellowship funded by FCT, respectively. This work was also supported by projects FCT/UID/GEO/50019/2019 and FCT/UIDB/50019/2020 - IDL funded by FCT.
Conflict of Interest
The authors declare that the research was conducted in the absence of any commercial or financial relationships that could be construed as a potential conflict of interest.
Publisher’s Note
All claims expressed in this article are solely those of the authors and do not necessarily represent those of their affiliated organizations, or those of the publisher, the editors and the reviewers. Any product that may be evaluated in this article, or claim that may be made by its manufacturer, is not guaranteed or endorsed by the publisher.
Acknowledgments
We would like to acknowledge the GEO3BCN-CSIC and the University of Barcelona as well as the GEOMAR Helmholtz Centre for providing the facilities and technical support during the study of the lacustrine records and geochemical analyses, respectively. We thank M. Antunes at Secretaria Regional do Turismo e Transportes for providing the digital altimetric database used in this study. We also acknowledge Serviços Florestais das Flores e Corvo as well as Câmara Municipal das Lajes das Flores, for their support in field logistics and access to outcrops. We also acknowledge J. Madeira and J. Cabral for their informal review of the manuscript, prior to submission, and DR and DP, for their constructive reviews that helped to improve the overall quality of the manuscript.
Supplementary Material
The Supplementary Material for this article can be found online at: https://www.frontiersin.org/articles/10.3389/feart.2021.738178/full#supplementary-material
References
Andrade, C., Cruz, J., Viveiros, F., and Coutinho, R. (2019). CO2 Flux from Volcanic Lakes in the Western Group of the Azores Archipelago (Portugal). Water 11 (3), 599. doi:10.3390/w11030599
Azevedo, J. M. M., Ferreira, M. R., and Martins, J. Á. (1991). The Emergent Volcanism of Flores Islands, Azores (Portugal). Arquipélago-Life Earth Sci. 9, 37–46.
Azevedo, J. M. M., and Portugal Ferreira, M. R. (2006). The Volcanotectonic Evolution of Flores Island, Azores (Portugal). J. Volcanology Geothermal Res. 156 (1–2), 90–102. doi:10.1016/j.jvolgeores.2006.03.011
Barberi, F., Cioni, R., Rosi, M., Santacroce, R., Sbrana, A., and Vecci, R. (1989). Magmatic and Phreatomagmatic Phases in Explosive Eruptions of Vesuvius as Deduced by Grain-Size and Component Analysis of the Pyroclastic Deposits. J. Volcanology Geothermal Res. 38 (3-4), 287–307. doi:10.1016/0377-0273(89)90044-9
Bas, M. J. L., Maitre, R. W. L., Streckeisen, A., and Zanettin, B. (1986). A Chemical Classification of Volcanic Rocks Based on the Total Alkali-Silica Diagram. J. Petrol. 27, 745–750. doi:10.1093/petrology/27.3.745
Björck, S., Rittenour, T., Rosén, P., França, Z., Möller, P., Snowball, I., et al. (2006). A Holocene Lacustrine Record in the central North Atlantic: Proxies for Volcanic Activity, Short-Term NAO Mode Variability, and Long-Term Precipitation Changes. Quat. Sci. Rev. 25 (1-2), 9–32. doi:10.1016/j.quascirev.2005.08.008
Blaauw, M., Christen, J. A., and Aquino Lopez, M. A. (2021). Rbacon: Age-Depth Modelling Using Bayesian Statistics. R package version 2.5.5. Available at: https://CRAN.R-project.org/package=rbacon (Accessed June 17, 2021).
Carey, S., and Sparks, R. S. J. (1986). Quantitative Models of the Fallout and Dispersal of Tephra from Volcanic Eruption Columns. Bull. Volcanol 48 (2), 109–125. doi:10.1007/BF01046546
Chester, D., Duncan, A., Coutinho, R., Wallenstein, N., and Branca, S. (2017). “Communicating Information on Eruptions and Their Impacts from the Earliest Times until the Late Twentieth century,” in Observing the Volcano World (Cham: Springer), 419–443. doi:10.1007/11157_2016_30
Clarke, H., Troll, V. R., and Carracedo, J. C. (2009). Phreatomagmatic to Strombolian Eruptive Activity of Basaltic Cinder Cones: Montaña Los Erales, Tenerife, Canary Islands. J. Volcanology Geothermal Res. 180 (2-4), 225–245. doi:10.1016/j.jvolgeores.2008.11.014
Cruz, J. V., Antunes, P., Amaral, C., França, Z., and Nunes, J. C. (2006). Volcanic Lakes of the Azores Archipelago (Portugal): Geological Setting and Geochemical Characterization. J. Volcanology Geothermal Res. 156 (1-2), 135–157. doi:10.1016/j.jvolgeores.2006.03.008
De Rita, D., Giordano, G., Esposito, A., Fabbri, M., and Rodani, S. (2002). Large Volume Phreatomagmatic Ignimbrites from the Colli Albani Volcano (Middle Pleistocene, Italy). J. Volcanology Geothermal Res. 118 (1-2), 77–98. doi:10.1016/S0377-0273(02)00251-2
Direção Regional do Ambiente (2001). Plano Regional da água. Relatório técnico. Available at: http://sig-sraa.azores.gov.pt/SIG/(S(t3wmy4450zukatyelzq1p2v5))/MapViewer/SectionsViewer.aspx?id=34 (Accessed March 3, 2021).
EMODnet Bathymetry Consortium (2018). EMODnet Digital Bathymetry (DTM 2018). France: Hydrographic and oceanographic service of the navy (SHOM). doi:10.12770/18ff0d48-b203-4a65-94a9-5fd8b0ec35f6
Freire Luis, J., Miranda, J. M., Galdeano, A., Patriat, P., Rossignol, J. C., and Mendes Victor, L. A. (1994). The Azores Triple junction Evolution since 10 Ma from an Aeromagnetic Survey of the Mid-Atlantic Ridge. Earth Planet. Sci. Lett. 125 (1–4), 439–459. doi:10.1016/0012-821X(94)90231-3
Genske, F. S., Beier, C., Stracke, A., Turner, S. P., Pearson, N. J., Hauff, F., et al. (2016). Comparing the Nature of the Western and Eastern Azores Mantle. Geochimica et Cosmochimica Acta 172, 76–92. doi:10.1016/j.gca.2015.08.019
Geshi, N., Németh, K., Noguchi, R., and Oikawa, T. (2019). Shift from Magmatic to Phreatomagmatic Explosions Controlled by the Lateral Evolution of a Feeder dike in the Suoana-Kazahaya Eruption, Miyakejima Volcano, Japan. Earth Planet. Sci. Lett. 511, 177–189. doi:10.1016/j.epsl.2019.01.038
Giralt, S., Moreno, A., Bao, R., Sáez, A., Prego, R., Valero-Garcés, B. L., et al. (2008). A Statistical Approach to Disentangle Environmental Forcings in a Lacustrine Record: the Lago Chungará Case (Chilean Altiplano). J. Paleolimnol 40 (1), 195–215. doi:10.1007/s10933-007-9151-9
Gutmann, J. T. (2002). Strombolian and Effusive Activity as Precursors to Phreatomagmatism: Eruptive Sequence at Maars of the Pinacate Volcanic Field, Sonora, Mexico. J. Volcanology Geothermal Res. 113 (1-2), 345–356. doi:10.1016/S0377-0273(01)00265-7
Hernández, A., Sáez, A., Bao, R., Raposeiro, P. M., Trigo, R. M., Doolittle, S., et al. (2017). The Influences of the AMO and NAO on the Sedimentary Infill in an Azores Archipelago lake since Ca. 1350 CE. Glob. Planet. Change 154, 61–74. doi:10.1016/j.gloplacha.2017.05.007
Hildenbrand, A., Marques, F. O., and Catalão, J. (2018). Large-scale Mass Wasting on Small Volcanic Islands Revealed by the Study of Flores Island (Azores). Sci. Rep. 8 (1), 1–11. doi:10.1038/s41598-018-32253-0
Houghton, B. F., Wilson, C. J. N., and Smith, I. E. M. (1999). Shallow-seated Controls on Styles of Explosive Basaltic Volcanism: a Case Study from New Zealand. J. volcanology geothermal Res. 91 (1), 97–120. doi:10.1016/S0377-0273(99)00058-X
Hunt, J. B., and Hill, P. G. (2001). Tephrological Implications of Beam Size-Sample-Size Effects in Electron Microprobe Analysis of Glass Shards. J. Quat. Sci. 16 (2), 105–117. doi:10.1002/jqs.571
Jarosewich, E., Nelen, J. A., and Norberg, J. A. (1980). Reference Samples for Electron Microprobe Analysis*. Geostandards Newsl. 4 (1), 43–47. doi:10.1111/j.1751-908X.1980.tb00273.x
Kueppers, U., Pimentel, A., Ellis, B., Forni, F., Neukampf, J., Pacheco, J., et al. (2019). Biased Volcanic hazard Assessment Due to Incomplete Eruption Records on Ocean Islands: an Example of Sete Cidades Volcano, Azores. Front. Earth Sci. 7, 122. doi:10.3389/feart.2019.00122
Kutterolf, S., Freundt, A., and Burkert, C. (2011). Eruptive History and Magmatic Evolution of the 1.9 Kyr Plinian Dacitic Chiltepe Tephra from Apoyeque Volcano in West-central Nicaragua. Bull. Volcanol 73, 811–831. doi:10.1007/s00445-011-0457-0
Kutterolf, S., Schindlbeck, J. C., Anselmetti, F. S., Ariztegui, D., Brenner, M., Curtis, J., et al. (2016). A 400-ka Tephrochronological Framework for Central America from Lake Petén Itzá (Guatemala) Sediments. Quat. Sci. Rev. 150, 200–220. doi:10.1016/j.quascirev.2016.08.023
Martí, J., Planagumà, L., Geyer, A., Canal, E., and Pedrazzi, D. (2011). Complex Interaction between Strombolian and Phreatomagmatic Eruptions in the Quaternary Monogenetic Volcanism of the Catalan Volcanic Zone (NE of Spain). J. Volcanology Geothermal Res. 201 (1-4), 178–193. doi:10.1016/j.jvolgeores.2010.12.009
McNamara, K., Cashman, K. V., Rust, A. C., Fontijn, K., Chalié, F., Tomlinson, E. L., et al. (2018). Using lake Sediment Cores to Improve Records of Volcanism at Aluto Volcano in the Main Ethiopian Rift. Geochem. Geophys. Geosyst. 19 (9), 3164–3188. doi:10.1029/2018GC007686
Métrich, N., Zanon, V., Créon, L., Hildenbrand, A., Moreira, M., and Marques, F. O. (2014). Is the 'Azores Hotspot' a Wetspot? Insights from the Geochemistry of Fluid and Melt Inclusions in Olivine of Pico Basalts. J. Petrol. 55 (2), 377–393. doi:10.1093/petrology/egt071
Mitchell, N. C. (2003). Susceptibility of Mid-ocean ridge Volcanic Islands and Seamounts to Large-Scale Landsliding. J. Geophys. Res. 108 (B8). doi:10.1029/2002JB001997
Morisseau, M., and Traineau, H. (1985). Mise en évidence d’une activité hydromagmatique holocène sur l’île de Flores (Açores). C. R. Acad. Sc. Paris, T 301 (série II, no 18), 1309–1314.
Pimentel, A., Pacheco, J., and Self, S. (2015). The ∼1000-years BP Explosive Eruption of Caldeira Volcano (Faial, Azores): the First Stage of Incremental Caldera Formation. Bull. Volcanol 77 (5), 1–26. doi:10.1007/s00445-015-0930-2
Rull, V., Stansell, N. D., Montoya, E., Bezada, M., and Abbott, M. B. (2010). Palynological Signal of the Younger Dryas in the Tropical Venezuelan Andes. Quat. Sci. Rev. 29 (23-24), 3045–3056. doi:10.1016/j.quascirev.2010.07.012
Sáez, A., Valero-Garcés, B. L., Moreno, A., Bao, R., Pueyo, J. J., González-Sampériz, P., et al. (2007). Lacustrine Sedimentation in Active Volcanic Settings: the Late Quaternary Depositional Evolution of Lake Chungará (Northern Chile). Sedimentology 54 (5), 1191–1222. doi:10.1111/j.1365-3091.2007.00878.x
Stern, C. R. (2008). Holocene Tephrochronology Record of Large Explosive Eruptions in the Southernmost Patagonian Andes. Bull. Volcanol 70 (4), 435–454. doi:10.1007/s00445-007-0148-z
Stockhecke, M., Sturm, M., Brunner, I., Schmincke, H. U., Sumita, M., Kipfer, R., et al. (2014). Sedimentary Evolution and Environmental History of Lake Van (Turkey) over the Past 600 000 Years. Sedimentology 61 (6), 1830–1861. doi:10.1111/sed.12118
Stuiver, M., Reimer, P. J., and Reimer, R. W. (2021). CALIB 8.2. [WWW program] at: http://calib.org (accessed January 25, 2021).
Sulpizio, R., Mele, D., Dellino, P., and Volpe, L. L. (2005). A Complex, Subplinian-type Eruption from Low-Viscosity, Phonolitic to Tephri-Phonolitic Magma: the AD 472 (Pollena) Eruption of Somma-Vesuvius, Italy. Bull. Volcanol 67 (8), 743–767. doi:10.1007/s00445-005-0414-x
Van Daele, M., Moernaut, J., Silversmit, G., Schmidt, S., Fontijn, K., Heirman, K., et al. (2014). The 600 Yr Eruptive History of Villarrica Volcano (Chile) Revealed by Annually Laminated lake Sediments. Geol. Soc. America Bull. 126 (3-4), 481–498. doi:10.1130/B30798.1
Vázquez-Loureiro, D., Gonçalves, V., Sáez, A., Hernández, A., Raposeiro, P. M., Giralt, S., et al. (2019). Diatom-inferred Ecological Responses of an Oceanic lake System to Volcanism and Anthropogenic Perturbations since 1290 CE. Palaeogeogr. Palaeoclimatol. Palaeoecol. 534, 109285. doi:10.1016/j.palaeo.2019.109285
Wulf, S., Kraml, M., Brauer, A., Keller, J., and Negendank, J. F. W. (2004). Tephrochronology of the 100ka lacustrine sediment record of Lago Grande di Monticchio (southern Italy). Quat. Int. 122 (1), 7–30. doi:10.1016/j.quaint.2004.01.028
Zanon, V., Pimentel, A., Auxerre, M., Marchini, G., and Stuart, F. M. (2020). Unravelling the Magma Feeding System of a Young Basaltic Oceanic Volcano. Lithos 352-353, 105325. doi:10.1016/j.lithos.2019.105325
Keywords: ocean island volcanoes, lake tephrostratigraphy, glass geochemistry, holocene volcanism, lagoa da lomba, Flores Island, azores archipelago
Citation: Andrade M, Ramalho RS, Pimentel A, Hernández A, Kutterolf S, Sáez A, Benavente M, Raposeiro PM and Giralt S (2021) Unraveling the Holocene Eruptive History of Flores Island (Azores) Through the Analysis of Lacustrine Sedimentary Records. Front. Earth Sci. 9:738178. doi: 10.3389/feart.2021.738178
Received: 08 July 2021; Accepted: 15 September 2021;
Published: 29 September 2021.
Edited by:
Antonio Paonita, Istituto Nazionale di Geofisica e Vulcanologia (INGV), ItalyReviewed by:
Dmitri Rouwet, Istituto Nazionale di Geofisica e Vulcanologia, ItalyDario Pedrazzi, Instituto de Ciencias de la Tierra Jaume Almera (ICTJA), Spain
Copyright © 2021 Andrade, Ramalho, Pimentel, Hernández, Kutterolf, Sáez, Benavente, Raposeiro and Giralt. This is an open-access article distributed under the terms of the Creative Commons Attribution License (CC BY). The use, distribution or reproduction in other forums is permitted, provided the original author(s) and the copyright owner(s) are credited and that the original publication in this journal is cited, in accordance with accepted academic practice. No use, distribution or reproduction is permitted which does not comply with these terms.
*Correspondence: Mariana Andrade, bWRhbmRyYWRlQGZjLnVsLnB0