- 1Key Laboratory of Western China’s Environmental System, Ministry of Education, College of Earth and Environmental Sciences, Lanzhou University, Lanzhou, China
- 2Department of Engineering, Modeling and Applied Social Sciences, University Federal of ABC, São Paulo, Brazil
In arid central Asia, the geo-ecological environment of the well-vegetated high mountains differs from that of the extensive arid Gobi desert areas, with the forested areas having experienced a different pattern of humidity variations compared to the dryland regions. Therefore, the moisture history of the forest areas reconstructed by tree rings may differ from that of the dryland areas. In the extremely arid area of the western Qilian Mountains and the surrounding dryland areas, where forest is absent, it is unclear how humidity conditions have changed over the past several centuries. Here, we use a pollen record from Tian’E Lake, with a chronology based on 210Pb and 137Cs, and with an average temporal resolution of ∼2 years, to reconstruct the humidity changes over the past 300 years. The results show that the pollen assemblage is dominated by Artemisia and Amaranthaceae (=Chenopodiaceae), and therefore, the A/C (Artemisia/Chenopodiaceae) ratio can be used to reconstruct changes in humidity conditions. Based on the pollen A/C ratio, two relatively wet periods are identified: ∼1740–1750 and 1840–1980, and two dry intervals: ∼1750–1840 and 1980–2018. This pattern of variation is similar to that reconstructed from nearby humidity records based on tree-ring width adjacent to the Tian’E Lake area and with instrumental records from meteorological stations over the past several decades. However, there are significantly different records between pollen-based and tree-ring based humidity during ∼1760–1830, 1880–1910, and 1920–2018 in the Qilian Mountains on long timescales. We conclude that pollen-based humidity records from dryland areas may differ from those reconstructed from tree-ring widths in forested mountain areas, especially when the temperature was increasing. It was further suggested that there was an antiphased relationship in humidity conditions between westerlies-dominated central Asia and monsoon areas over the past century.
Introduction
Although instrumental climate records show a clear record of increasing temperature in the Northern Hemisphere during the 20th century, humidity variations exhibit complex spatial patterns at the regional scale (IPCC, 2007). Increased global warming could lead to more frequent and sustained droughts (Cook et al., 2004; Dai et al., 2004), with consequently increased aridity in arid and semiarid areas (Feng and Zhang, 2015; Huang et al., 2016). It has been shown that on the global scale, drylands have experienced a significant intensification of warming in the past 100 years, together with inter-decadal fluctuations in drier/wetter conditions (Li et al., 2007; Guan et al., 2019). The Qilian Mountains, in the northern part of the Qinghai–Tibetan Plateau, is located in the marginal area of the arid region in central Asia and the semiarid or semi-humid region in the east. The Qilian Mountains is climatically influenced by the westerlies in the west and the summer monsoon in the east (Chen et al., 2008). The high mountains of the region have diverse geo-ecological environments along vertical and longitudinal gradients, including forest, shrubland, grassland, tundra, and sparse desert-like vegetation. The precipitation in the Qilian Mountains decreases from east to west. In recent decades, the climate of the region has warmed, and there is an increasing frequency and intensity of severe drought events (Zou et al., 2005).
In the Qilian Mountains, numerous dendrochronological studies have been conducted, including reconstructions of moisture conditions at the lower tree line (Kang et al., 2003; Yang et al., 2011; Zhang et al., 2011; Deng et al., 2013; Sun and Liu, 2013; Yang et al., 2019) and temperature at the upper tree line (Wang et al., 2001; Gou et al., 2012). However, it can be concluded that the pattern of climate change in the Qilian Mountains reconstructed by tree-ring records is complex on both the spatial and temporal scales. Moreover, because of the absence of forest in the arid area of the western Qilian Mountains and the surrounding arid areas, it is unclear how humidity conditions have evolved over the past three centuries, in the context of the significant temperature variations.
Pollen records from closed alpine lakes are reliable indicators of regional changes in moisture conditions. Moisture reconstructions from such lakes can potentially fill the gaps in the spatial distribution of paleoclimate records from dryland areas in central Asia, which may have experienced a different pattern of moisture changes from those of forested areas. Tian’E Lake is an alpine lake located in the western Qilian Mountains. Zhang et al. (2018) used the A/C ratio from this lake to reconstruct the history of regional humidity, and it had a relatively wetting trend after AD 1600 with some fluctuations (all subsequent dates are AD). A geotropism index suggests that the region was relatively warm and dry during the Medieval Warm Period and relatively cold and wet during the Little Ice Age (LIA) (Yan et al., 2018; Zhang et al., 2018). The past 300 years is a critical interval bridging the gap between meteorological records and geological records, and it also corresponds to the transition period from the LIA to the Current Warming Period. The high sediment accumulation rate of the alpine Tian’E lake enables high-resolution paleoenvironmental records to be obtained. Based on pollen analysis of 128 samples from the sediments of the lake, we reconstructed the pattern of humidity changes in the surrounding dryland area over the past ∼300 years, and attempt to address whether the tree-ring records from the semiarid area have the representation of humidity over the large arid region in central Asia or not.
Study Area
Tian’E Lake (39°14′20″N, 97°55′26″E, 3,012 m.a.s.l.) is an alpine lake located in the arid western part of the Qilian Mountains (Figure 1), ∼35 km from the nearest forested area. The lake is ∼0.12 km2 in area and has a water depth of ∼14.5 m. The lake basin is closed and is mainly fed by groundwater from glaciers, and its sediments can potentially provide a record of regional climate change (Zhang et al., 2018). As recorded at the nearby Sunan Meteorological Station (150 km from the lake, at 2,330 m.a.s.l.), from 1981 to 2010, the regional average annual precipitation was ∼267 mm and the evaporation was ∼2,200 mm. At the same time, the mean January temperature was −9.4°C and the mean July temperature was 16.8°C (Figure 1B).
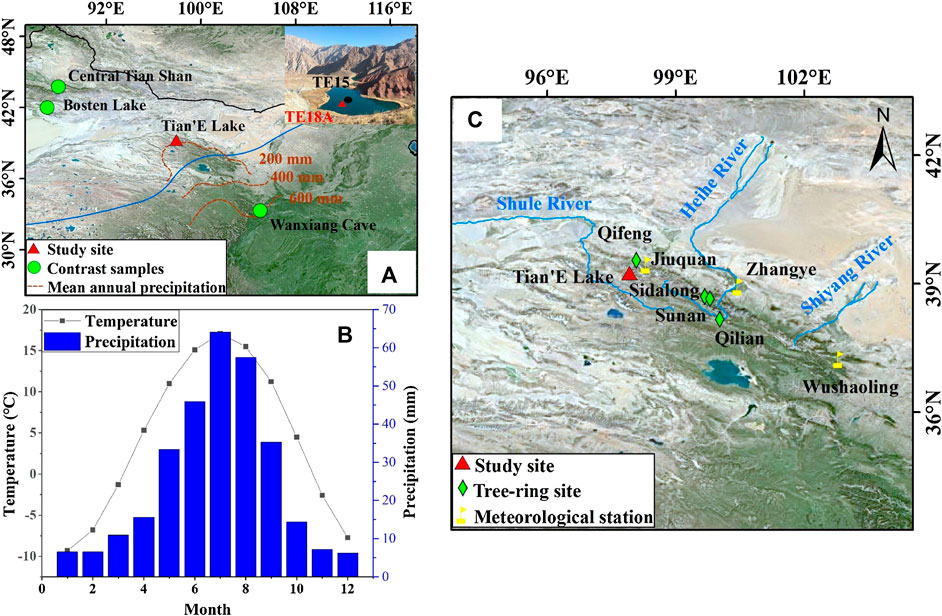
FIGURE 1. Location and environmental setting of the Tian’E Lake area. (A) Location of Tian’E Lake in northwestern China and locations of other sites mentioned in the text. Inset image is a photo of Tian’E Lake showing the location of cores TE18A and TE15. The blue line is the modern limit of the summer monsoon (Chen et al., 2008), and the red dashed lines denote the mean annual precipitation. (B) Monthly temperature and precipitation at the Sunan Meteorological Station during 1981–2010. (C) Location of Tian’E Lake and tree-ring records from the Qilian Mountains referenced in the text.
The vegetation coverage of the Tian’E Lake region is sparse, and forest is absent (Figure 1A). The region is characterized by a desert-steppe landscape, with the natural vegetation consisting of desert and dry grassland plants, very similar to the vegetation of the Hexi Corridor. The vegetation consists mainly of Artemisia and Amaranthaceae, together with Asteraceae, Poaceae, Ranunculaceae, Rosaceae, Fabaceae, Labiatae, Caryophyllaceae, and Brassicaceae (Yi and Wang, 2013).
Materials and Methods
Sediment Sampling and Chronology
A 66-cm-long sediment core (core TE18A) was collected from Tian’E Lake in October 2018 using a gravity corer (Figure 1A). The core was mainly subsampled at a 0.5-cm interval, with some samples (TE18A-81, 82, 83, and 84) at a 1-cm interval. The samples were transported to the laboratory and then freeze-dried.
Eighteen samples were analyzed for 210Pb and 137Cs at the Key Laboratory of Western China’s Environmental System, Ministry of Education, Lanzhou University. For 210Pb analysis, subsamples were freeze-dried and homogenized before being stored for three weeks to reach radioactive equilibrium. The samples were then analyzed for 210Pb using gamma spectrometry with a low background intrinsic germanium detector. Gamma emissions at 46.5 keV were used to determine the radioactivity level of 210Pb downcore. Unsupported 210Pb activity (210Pbex) was calculated by subtracting the activity of supported 210Pb (as 226Ra) from the total 210Pb activity. The final chronology was established using the constant rate of supply model (Appleby and Oldfield, 1978).
210Pbex decreases irregularly with increasing depth (Figure 2B). Equilibrium between the total 210Pb and supported 210Pb activity is reached at the depth of 18.5–19 cm. 137Cs first appears in the core sample at the depth of 12.0–12.5 cm and reaches a maximum value of 33.3 Bq kg−1 at the depth of 10.0–10.5 cm. Hence, these levels were dated to 1952 and 1963, respectively, which is in good agreement with the 210Pb chronology.
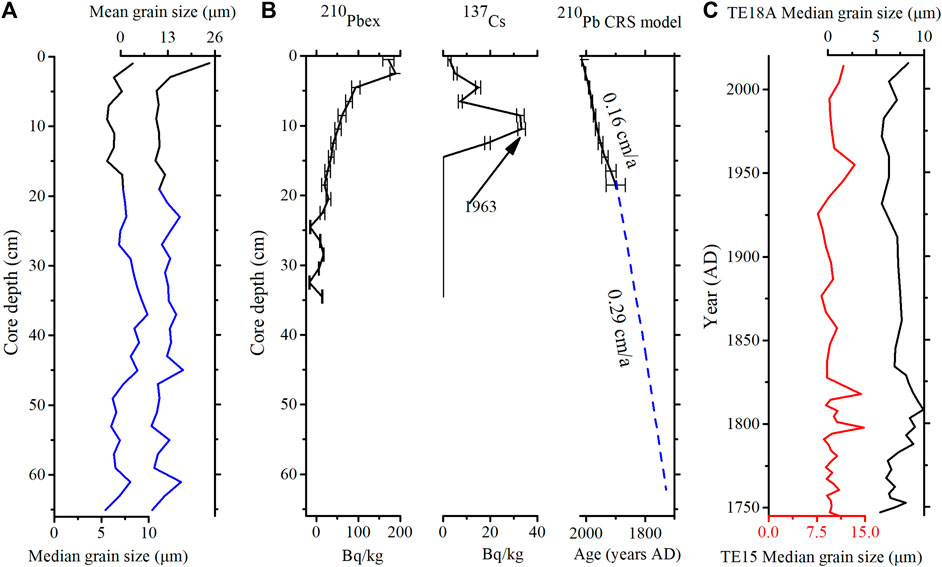
FIGURE 2. Age model for core TE18A from Tian’E Lake. (A) Depth profiles of median grain size and mean grain size of TE18A. Parts below 19 cm are shown in blue. (B) 210Pb and 137Cs activity and the constant rate of supply age-depth model. (C) Comparison of the median grain sizes of TE15 and TE18A.
Below 19 cm of TE18A, there are no direct AMS 14C dates based on plant material. Considering the lake has a stable sedimentation environment and the two sediment cores of TE15 and TE18A (Figure 1A) have close coring locations and a relatively small grain-size (Figure 2), we assume that the sedimentation rate was consistent and extrapolate the chronology of TE18A based on that of core TE15. The TE15 core has three radiocarbon dates of terrestrial plants in the upper 56 cm and four dates in the upper 166 cm (Zhang et al., 2018), so we use the average sedimentation rate of the top 100 cm of TE15 (0.29 cm/a) to calculate the age model of TE18A considering the errors of 14C dates and variable sedimentation rates based on 14C dates (Figure 2B). Based on this age model, the median grain-size data of TE18A have good consistency with TE15 (Figure 2C), which increased the confidence of this age model additionally.
Pollen Analysis
Pollen grains were extracted from 1 to 3 g of dried sediment using sequential treatments with 10% HCl, 40% HF, and 10% HCl. Before chemical pretreatment, tablets containing a known number of Lycopodium spores were added to estimate pollen concentrations. The samples were mounted in glycerin and counted using a light microscope at ×400–600 magnification. At least 300 terrestrial pollen grains were counted per sample, and pollen percentages were calculated based on the sum of total terrestrial pollen. Pollen identifications were based on photographs in Tang et al. (2016). A pollen diagram was produced using Tilia software (Grimm, 2011), and pollen assemblage zones were defined using stratigraphically constrained cluster analysis implemented with CONISS, using all pollen types (Grimm, 1987).
Results
A total of 128 samples from core TE18A were used for pollen analysis, and 27 pollen types were identified. The average temporal resolution of the pollen data is ∼2 years. The tree pollen percentages are relatively low, including some types of Pinus, Picea, Betula, and Salix; the main shrub pollen types are Ephedra, Nitraria, Rosaceae, and Zygophyllaceae; and the main herb pollen types are Artemisia, Amaranthaceae, Poaceae, Cyperaceae, Thalictrum, Polygonaceae, Asteraceae, Brassicaceae, Labiatae, and Ranunculaceae. The main aquatic pollen type is Typha. The pollen assemblages are divided into four main zones based on CONISS (Figure 3), and they are described below.
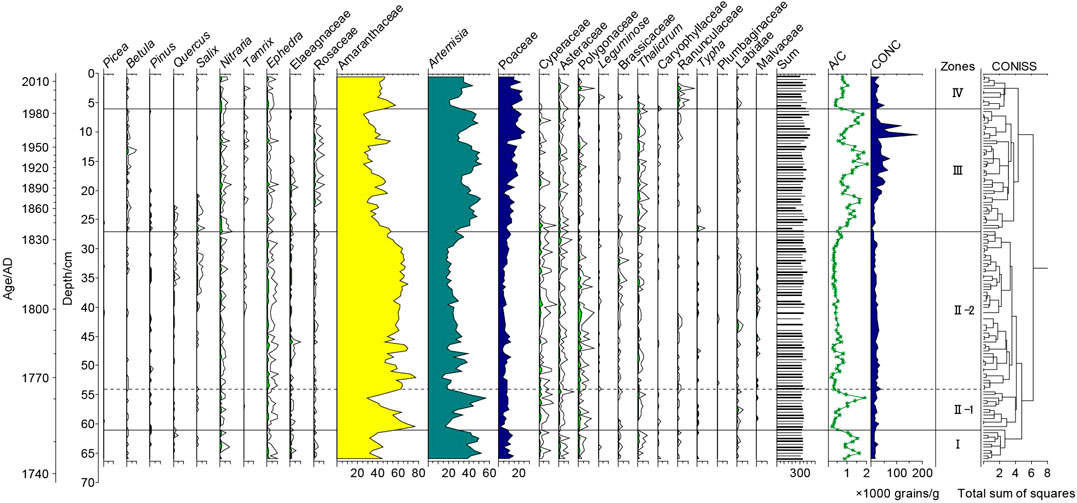
FIGURE 3. Pollen percentage diagram (%) for core TE18A spanning the last 300 years (open curves are a 5× exaggeration of scale).
Zone I (66–61 cm, ∼1740–1750)
Amaranthaceae (31.7–44.8%, average of 38.1%) and Artemisia (37.5–52.3%, average of 44.1%) are the main pollen types. Asteraceae (average of 1%), Cyperaceae (average of 1%), and Ephedra (average of 1%) are also represented. The A/C ratio is variable (0.87–1.65, average of 1.18). The pollen concentration ranges from 11,381 to 26,886 grains/g (average of 15,492 grains/g).
Zone II (61–27 cm, ∼1750–1840)
Amaranthaceae (29.4–76.9%, average of 58.9%) has a substantially increased representation, while Artemisia (16.9–56.9%) decreases to its lowest values. Other taxa with an average representation above 1% are Cyperaceae (1.2%), Ephedra (1.1%), and Polygonaceae (1%). Compared to Zone I, the representation of Artemisia is substantially decreased and that of Amaranthaceae is significantly increased. This zone has the lowest A/C ratio, with an average of 0.49. The pollen concentration decreases markedly, fluctuating within the range of 9,049–38,022 grains/g (average of 19,479 grains/g). Based on the percentages of contents of Artemisia and Amaranthaceae, the zone can be divided into two subzones, II-1 and II-2. The average representation of Amaranthaceae increases from 53.5% in subzone II-1 to 60.2% in subzone II-2 and that of Artemisia decreases from 32.1% in subzone II-1 to 24.9% in subzone II-2.
Zone III (27–6 cm, ∼1840–1980)
This zone is dominated by Amaranthaceae (25.8–53.2%, average of 37.3%) and Artemisia (26.6–52.9%, average of 41.3%). Compared to Zone II, the representation of Betula (average of 0.4%), Artemisia, and Rosaceae substantially increases, while that of Amaranthaceae and Typha decreases. The A/C ratio has the range of 0.5–2.05 and shows an increasing trend. The pollen concentration increases substantially compared with the previous zone, to 36,288 grains/g.
Zone IV (6–0 cm, ∼1980–2018)
Amaranthaceae (41.8–57.7%, average of 46.1%) and Artemisia (21.1–43.5%, average of 31.2%) are still the dominant pollen types throughout the zone. Compared to Zone III, Artemisia decreases slightly and Amaranthaceae increases. The A/C ratio averages 0.69, and there is a slight decrease throughout the zone. The pollen concentration has the range of 11,968–51,779 grains/g (average of 24,908 grains/g), and there is a decreasing trend compared with Zone III.
Discussion
Changes in Humidity Conditions in Western Qilian Mountains Over the Past 300 Years
Artemisia and Amaranthaceae are the main pollen types in the arid and semiarid areas, and both are overrepresented in pollen assemblages because of their high dispersal ability. Amaranthaceae percentages in areas with desert vegetation are generally higher than those of Artemisia (Xu et al., 2007), and the sum of the two pollen types typically exceeds 40%, indicating the occurrence of desert-steppe vegetation (Li et al., 2005; Xu et al., 2007). In core TE18A from Tian’E Lake, the average percentages of Amaranthaceae and Artemisia are 48.9 and 33.2%, respectively, and therefore, the vegetation is desert steppe at Tian’E Lake and the surrounding area. The A/C ratio is widely used as a proxy for reconstructing changes in humidity conditions in arid and semiarid areas (Tarasov et al., 1997; Huang et al., 2009; Zhao et al., 2009; Zhang et al., 2018). A paleoenvironmental study of a 50-year pollen record with a near-annual resolution from Gahai Lake in the Qaidam basin showed that the A/C ratio was well correlated with moisture data from the Delingha Meteorological Station (Zhao et al., 2008). A study of modern surface pollen in northwestern China demonstrated a statistically significant relationship between the A/C ratio and humidity when the sum percentage of the two pollen types exceeded 50%, with a higher A/C ratio indicating more humid conditions in a semiarid environment (Zhao et al., 2012).
To assess the reliability of humidity reconstruction, we compared it to the records from meteorological stations and historical documents. It was demonstrated that the A/C ratio fluctuations of TE18A were related to both the temperature and precipitation records from the Jiuquan station (Figure 4). The higher A/C ratio was related to lower temperature and higher precipitation during 1970–1980s, and the fast increases in temperature in the 1990s and 2010s led to lower humidity even with some higher precipitation (Figure 4). In addition, the moisture history revealed by the A/C ratio from Tian’E Lake was generally consistent with documentary records from the Hexi Corridor. For ∼1876–1878, the low A/C ratio indicates a dry interval, which corresponds to the drought event of “Ding-Wu Disaster”; this was a major drought in northern China in the early Guangxu reign period, which resulted in millions of deaths with extremely adverse social impacts (Li et al., 2018). According to the Jiuquan city annals (Jiuquan City Annals Compilation Committee, 2008), after 1953, there was a prolonged drought and insect disaster in Jinta County of Jiuquan city. As documented in the Dunhuang city annals (Dunhuang City Annals Compilation Committee, 1994), floods occurred in 1939, 1946, 1971, and 1979. The timing of the drought and flood years documented in historical Chinese texts was generally in accord with the pollen record from Tian’E Lake. Therefore, the A/C ratio was a tested reliable indicator of humidity in this region.
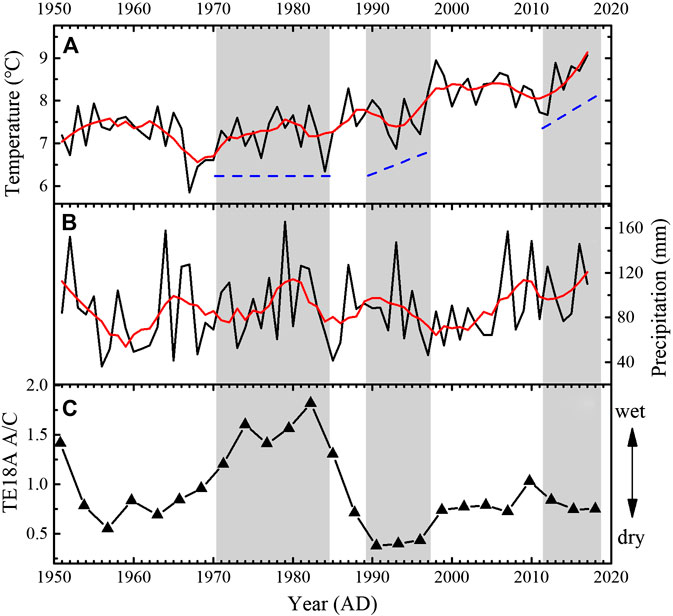
FIGURE 4. Comparison of the A/C ratio with the meteorological information from the Jiuquan station during 1951–2017. (A) Mean annual temperature (°C). (B) Mean annual precipitation (mm). (C) Pollen A/C ratio from Tian’E Lake. The red curve is the result of 10-point smoothing. The blue dashed line indicates the trend of temperature. Some high precipitation periods are indicated by gray shading.
In the small lake basin, Poaceae plants mainly include aquatic plants of Phragmites australis and terrestrial plants of Stipa and Achnatherum splendens, and therefore, it is difficult to use Poaceae pollen to reconstruct climatic signals. Ephedra and Nitraria are typical desert plants, and their increase in pollen assemblage indicates climatic drought. For example, Ephedra had a high content during ∼1750–1840 (Figure 3) and this period has the lowest A/C ratio, and both of them indicate drier climate.
The reconstructed humidity record for the western Qilian Mountains, based on the A/C ratio from Tian’E Lake, is presented in Figure 5H, together with other climate records for the region. For the interval of ∼1740–1750, the high A/C ratio indicates the occurrence of relatively high humidity at this time (Figure 5H). From ∼1750 to 1840, the A/C ratio decreased markedly and reached its lowest values, indicating that this interval was the driest within the entire sequence (Figure 5H), which is also reflected by an increase in the representation of Amaranthaceae, Ephedra, and Nitraria and a gradual decrease in the pollen concentration (Figure 3). Some of the tree-ring records from the Qilian Mountains also revealed a drought condition during ∼1760–1820s (Wang et al., 2001; Zhang et al., 2011; Kang et al., 2013). As indicated by the A/C ratio, this dry interval was interrupted by a notable wet period in ∼1760 (Figure 5H). The subsequent rapid increase in the A/C ratio indicates that the interval of ∼1840–1980 was the wettest in the western Qilian Mountains during the past 300 years (Figure 5H). During this period, the desert pollen types of Ephedra and Amaranthaceae decreased, while Artemisia and the total pollen concentration increased (Figure 3). During ∼1980–2018, the lower A/C ratio suggests that the climate became drier than the previous stage (Figure 5). The tree-ring record from Qifeng, in the western Qilian Mountains and close to our study area, indicates a drying trend during the recent decades (Gou et al., 2015; Yang et al., 2019).
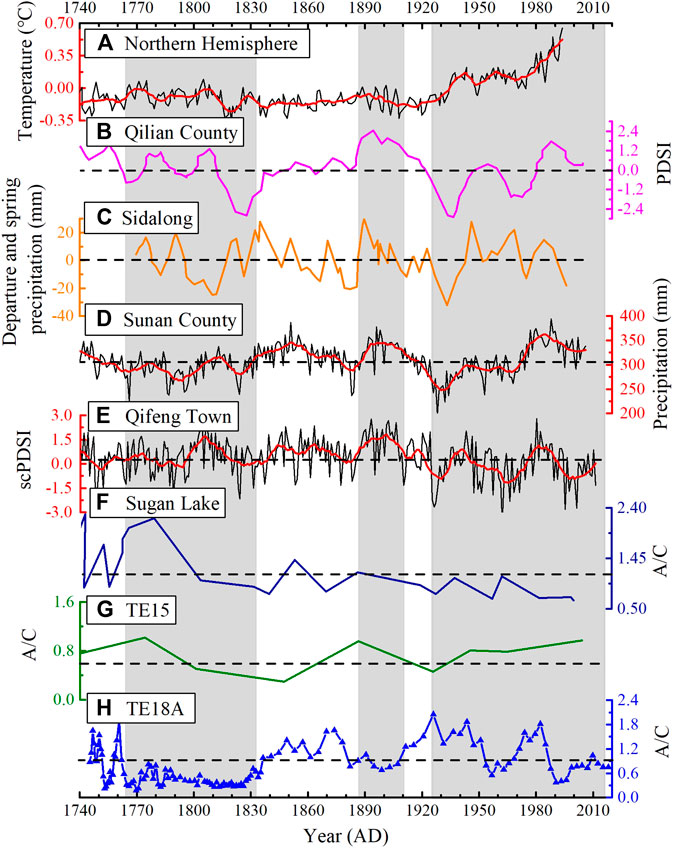
FIGURE 5. Comparison of the humidity reconstruction for Tian’E Lake based on the A/C ratio with tree-ring and pollen records from Qilian Mountains and other temperature signals. (A) Northern Hemisphere temperature (Mann et al., 1998). (B) Palmer Drought Severity Index (PDSI) from May to July, a tree-ring record from central Qilian Mountains (Sun and Liu, 2013). (C) Tree-ring-based precipitation anomaly from Sidalong, in the central Qilian Mountains (Wang et al., 2001). (D) Annual precipitation reconstruction since 775 based on tree-ring records from the Qilian Mountains (Zhang et al., 2011). (E) scPDSI-based tree-ring records from Qifeng in the western Qilian Mountains (Yang et al., 2019). (F) Pollen A/C ratio from Sugan Lake (Zhang et al., 2010). (G) Pollen A/C ratio from core TE15 of Tian’E Lake in the western Qilian Mountains (Zhang et al., 2018). (H) Pollen A/C ratio from core TE18A of Tian’E Lake in the western Qilian Mountains (this study). Horizontal dashed lines represent the average value. Significant differences between the pollen and tree-ring-based humidity reconstructions are indicated by gray shaded areas. The red curves are the result of 20-point smoothing.
Overall, the most obvious pattern of climatic changes in the western part of the Qilian Mountains during the last ∼300 years is alternation of warm-dry and cold-wet conditions; however, the changes in humidity conditions may have been affected by temperature fluctuations on the inter-decadal scale as that in the recent decades we discussed in Figure 4.
Differences Between the Pollen-Based Regional Humidity Record Evolution and Tree-Ring Records on Decadal to Centennial Timescales
Several moisture-sensitive tree-ring width series for the central and western Qilian Mountains (with gradients of humidity and precipitation) have been produced, and their reconstructed humidity/precipitation have consistencies and differences (Figures 1C,5) (Supplementary Table S1). Differences in reconstructed moisture conditions between the pollen and tree-ring records are evident during ∼1760–1830, 1890–1910, and 1920–2018 (Figure 5).
During ∼1760–1830, the low pollen A/C ratio indicates the occurrence of the most intense and extended dry interval within the last 300 years, whereas the intensity and timing of drought indicated by the tree-ring records differ from the pollen record. On the central Qilian Mountains, the lower Palmer Drought Severity Index (PDSI) indicates that it experienced severe drought conditions at this time (Sun and Liu, 2013) (Figure 5B), which is consistent with low precipitation records from tree rings, Sunan County (Zhang et al., 2011) (Figure 5D). And a low precipitation period also occurred in the 1800s (Wang et al., 2001) (Figure 5C). However, the duration of the drought is not so long as that indicated by the pollen record (Figure 5H). At the western Qilian Mountain area, tree-ring records indicate that the Hexi Corridor had a humid climate during this period of 1760–1830 (Yang et al., 2019) (Figure 5E). A 2,700-year high-resolution (ca. 10-year) pollen record from the annually varved Sugan Lake in the Qaidam basin was presented, and the pollen A/C ratio suggests a relatively moister climate during ∼1700–1800 and a drying trend from ∼1790 (Zhang et al., 2010) (Figure 5F). During ∼1890–1910, there was a slight increase in temperature in the Northern Hemisphere (Mann et al., 1998) (Figure 5A), and the pollen record from Tian’E Lake indicates a relatively dry climate (Figure 5H), whereas tree-ring records from the Qilian Mountains display high precipitation or wetter conditions (Wang et al., 2001; Hou et al., 2011; Zhang et al., 2011; Sun and Liu, 2013; Yang et al., 2019). Although the tree-ring record was obtained from a site closer to Tian’E Lake (Yang et al., 2019), the pattern of moisture changes differs substantially from that of Tian’E Lake. Furthermore, the moisture/precipitation conditions recorded by tree-ring records from the central Qilian Mountains spanning the intervals of ∼1920–2018 suggest a trend of wetting conditions with the temperature increasing rapidly (Wang et al., 2001; Zhang et al., 2011; Sun and Liu, 2013) (Figures 5B–D), while it shows a phase of drying recorded by pollen from Tian’E Lake and Sugan Lake (Zhang et al., 2010; Zhang et al., 2018) (Figures 5F–H) and tree-ring records from the western Qilian Mountain areas (Yang et al., 2019) (Figure 5E). Notably, three significant differences in the pollen records from Tian’E Lake compared with tree-ring records occurred during ∼1760–1830, 1890–1910, and 1920–2018 (Figure 5), and these three intervals broadly correspond to the warm periods in the Northern Hemisphere (Mann et al., 1998) (Figure 5A). The good correspondence suggests that the pollen-indicated desert-steppe vegetation in the arid region is more temperature sensitive than tree-ring-indicated forest.
In addition, the humidity reconstruction based on pollen records is similar to the nearest tree-ring records of the Qifeng site from the western Qilian Mountains in the last few decades, but substantially different for previous periods (Yang et al., 2019) (Figures 5E,H). Therefore, there are differences between tree-ring and pollen-inferred humidity on a longer timescale. Although an offset between the two types of records could be caused by dating uncertainties at Tian’E Lake, there are also different types of response modes of tree rings and pollen spectra to long-term climate change.
Tree rings have the advantages of covering wide areas, a high temporal resolution (seasonal to annual), precise dating, and overall high reliability (Esper et al., 2002). However, at the centennial scale, studies of tree-ring widths have revealed several influences which may introduce uncertainties into climate reconstructions, for example, the complex interactions between environmental factors such as soil water, temperature, soil, CO2 concentration, and changes in groundwater status caused by the thawing of frozen soil and glacier meltwater supply (Liu et al., 2011; Zhang et al., 2011; Gou et al., 2012). In addition, the response of woody and herbaceous plants to climatic seasonality is different. Tree-ring growth is more sensitive to winter precipitation, while desert grassland vegetation may be sensitive to spring or summer precipitation. For example, it was suggested that high temperatures favor tree growth and that the warming trend after the LIA resulted in an increase in tree density and an advance of the tree line in the eastern Qilian Mountains (Gou et al., 2012). The results of the correlation analysis showed that tree-ring growth in the central Qilian Mountains was most strongly related to total water-year precipitation from the prior August to the current July (Zhang et al., 2011). Liu et al. (2011) suggested that there was a significant increase in the water-use efficiency of Picea crassifolia with increasing atmospheric CO2 concentration at all sampling locations from east to west in the Qilian Mountains. Based on the above discussion, humidity conditions in dryland areas may be more sensitive to climatic warming than forested mountain areas which have a more variable environment. We infer that forested mountain areas reflect local climate and may experience a different pattern of humidity variations than extensive dryland areas on a longer timescale.
With regard to differences in humidity conditions indicated by the pollen and tree-ring records during ∼1920–2018 (Figure 5), in relation to increasing temperature, it is important to determine whether pollen records from desert-steppe environments reflect moisture changes and whether they differ from tree-ring records. The three meteorological stations of Wushaoling, Zhangye, and Jiuquan (from 1951 to 2017) provide climate records from east to west across the study area, and they indicate spatial differences in climate between the eastern, central, and western parts of the Qilian Mountains (Figure 6). The results from Wushaoling, Zhangye, and Jiuquan show that from 1951 to 2017, the average precipitation in the eastern, central, and western parts of the study area was 414.9, 129.1, and 88.4 mm, respectively (Figures 6B,E,H). It is clear that with rising temperature, there has been a fluctuating but overall increasing trend of precipitation in the Qilian Mountains (Figures 6B,E,H). Relative humidity in central and western Qilian Mountains shows a decreasing trend (Figures 6C,F), while there is an increasing trend in the eastern part (Figure 6I). It is obvious that there is a similar pattern of variation between PDSI and precipitation reconstruction based on different tree-ring records from the central Qilian Mountains (Figures 5B–D).
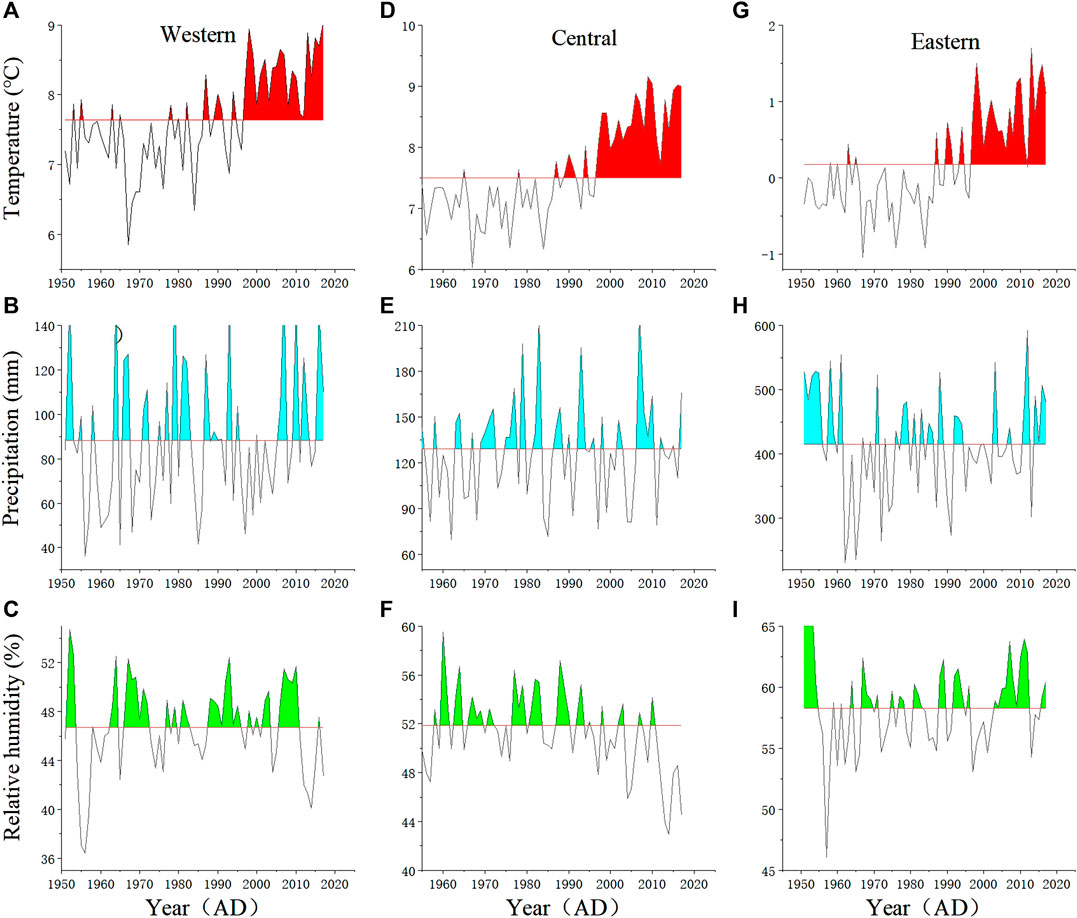
FIGURE 6. Annual precipitation (mm), annual average temperature (°C), and relative humidity (%) in the eastern, central, and western parts of the northern slopes of the Qilian Mountains (data from China Meteorological Data Network http://data.cma.cn/). (A) Annual temperature at the Jiuquan Meteorological Station during 1951–2017. (B) Annual precipitation at Jiuquan. (C) Relative humidity at Jiuquan. (D) Annual temperature at the Zhangye Meteorological Station during 1951–2017. (E) Annual precipitation at Zhangye. (F) Relative humidity at Zhangye. (G) Annual temperature at the Wushaoling Meteorological Station during 1951–2017. (H) Annual precipitation at Wushaoling. (I) Relative humidity at Wushaoling. Black horizontal lines represent the mean value.
The humidity of dryland areas is mainly controlled by a combination of temperature and precipitation, which is likely to be a dominant factor affecting vegetation growth in the dryland areas. And temperature plays a more important role in dryland areas than in forested areas, which are a more complex environment with more precipitation for tree growth. According to the records from meteorological stations, we further suggest that pollen from desert-steppe areas can reflect the humidity conditions of a broad area of dryland in the northwestern China.
Comparison of Various Moisture Reconstructions From Different Climate Regions
There were substantial differences in moisture conditions between westerlies-influenced Asia and mid-latitude monsoonal Asia during the Holocene, on suborbital, centennial, and decadal timescales (Chen et al., 2019). In addition, it has been suggested that the climate of the western Qilian Mountain region was alternately affected by the westerlies and Asian monsoon, and by both circulation systems together through time (Zhang et al., 2009).
In the westerlies-dominated region of the Tian Shan, records of tree-ring width indicate a trend of increasing moisture in the 20th century (Figure 7B), along with increasing temperature (Mann et al., 1998) (Figure 7A). In the lower Yanqi basin, adjacent to the Tian Shan Mountains, records of sedimentary carbonate content and pollen A/C ratio from Bosten Lake (Figure 7C) indicate a dry climate from the end of the 19th century onward (Chen et al., 2006, with new data of this study; Fontana et al., 2019), which was contrary to tree-ring records from the Tian Shan Mountains. Huang et al. (2018) studied the Holocene vegetation and climate dynamics in the Altai Mountains and surrounding areas and suggested that, compared with the lower basins/plains, the vegetation of mountain environments responded differently to temperature forcing.
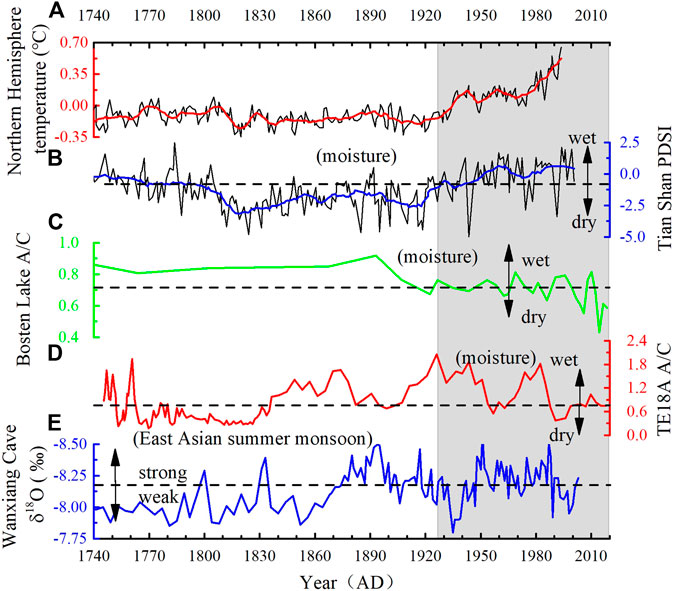
FIGURE 7. Comparison of various climate reconstructions from different climatic regions in northwestern China with Northern Hemisphere temperature. (A) Northern Hemisphere temperature (Mann et al., 1998); the red line represents 20-point smoothing. (B) April–June Palmer Drought Severity Index derived from tree rings from the Tian Shan area in northwestern China (Li et al., 2006); the blue line represents 20-point smoothing. (C) Pollen A/C ratio from Bosten Lake (Chen et al., 2006), which is extended to 2018 using new data from the new core BST19C-1 (this study). (D) Pollen A/C ratio from Tian’E Lake (this study). (E) Stalagmite δ18O record from Wanxiang Cave in northwestern China, reflecting variations in the intensity of the Asian summer monsoon circulation (Zhang et al., 2008). Horizontal dashed lines represent average values. Periods of rapid temperature increase are shown by gray shading.
In the Qaidam basin, the pollen A/C ratio at Hurleg and Toson lakes decreased substantially since 1700 (Zhao et al., 2010), and a tree-ring-based precipitation record from Delingha (∼30 km from Lake Kruk; 3,700–4,000 m.a.s.l.) shows the opposite trend (Shao et al., 2005). In the Qilian Mountains, tree-ring records of hydroclimatic variations also show substantial regional differences (Yang et al., 2019). The climate of the Hexi Corridor was generally dry during the Medieval Climate Anomaly, with a subsequent drying trend during the 20th century, whereas the Qaidam basin experienced high precipitation during the Medieval Climate Anomaly and the 20th century (Yang et al., 2019). Moreover, Yang et al. (2019) suggested that the Qaidam basin will receive more precipitation with ongoing global warming, whereas the Hexi Corridor will become drier in the future. Tree-ring records from the Helan Mountains, to the northeast of the Qilian Mountains, show a clear drying trend since the 1930s (Li et al., 2007), which is the opposite of the wetting trend in northwestern China (Li et al., 2006; Chen et al., 2014).
The pollen A/C ratio from Tian’E Lake indicates a trend of decreasing moisture conditions in the western Qilian Mountains from the 1920s onward (this study, Figure 7D), which is consistent with humidity records from Bosten Lake and Sugan Lake in the dryland areas (Figures 5F,7C). However, in the monsoon region, the oxygen isotope record from Wanxiang Cave indicates an interval of strong monsoon during the same interval (Zhang et al., 2008) (Figure 7E). Notably, the stalagmite δ18O record from Wanxiang Cave is out of phase with the climate reconstruction from Tian’E Lake. The pollen record from Tian’E Lake indicates that the humidity record of the vast dryland area of central Asia was different from that suggested by tree-ring and stalagmite records from the monsoon region (Figure 7E), especially during the period of rapid temperature rise (Figure 7A). These spatiotemporal discrepancies among different records may also reflect the interaction of the monsoon and the westerlies.
Conclusion
We have obtained a high-resolution pollen record spanning the last 300 years from the sediments of Tian’E Lake in the western Qilian Mountains which is used to reconstruct humidity changes in the extensive dryland area of central Asia. The record helps fill the spatial gap in recent climate records from the region. The A/C ratio indicates the occurrence of high humidity during ∼1740–1750 and dry conditions during ∼1750–1840, with a notable wet interval around 1760. The interval of 1840–1980 was the most extended and pronounced wet period in the western Qilian Mountains during the past 300 years. During 1980–2018, the lower A/C ratio suggests that the climate became drier than previously.
Over the past few decades, the humidity of dryland areas is mainly controlled by a combination of temperature and precipitation. And pollen-indicated desert-steppe vegetation in the arid region is more temperature sensitive than tree-ring-indicated forest. There are significantly different records between pollen-based and tree-ring-based humidity during ∼1760–1830, ∼1880–1910, and ∼1920–2018 in the Qilian Mountains. It was inferred that forested mountain areas may experience a different pattern of humidity variations than vast dryland areas at the background of substantial temperature changes on long timescales. We further suggest that pollen from desert-steppe areas can reflect the humidity conditions of a broad area of dryland in the northwestern China on the basis of evidences from meteorological stations.
A comparison of various climate records from westerlies-dominated central Asia and monsoon areas suggests that there was an antiphased relationship in humidity conditions between these two climatic domains over the past 100 years.
Data Availability Statement
The raw data supporting the conclusions of this article will be made available by the authors, without undue reservation.
Author Contributions
XH conceived this study. JW undertook the identification of pollen. JW and XH wrote the manuscript. JZ, LX, YX, LF, XR, and ZW discussed the data. LF improved the language.
Conflict of Interest
The authors declare that the research was conducted in the absence of any commercial or financial relationships that could be construed as a potential conflict of interest.
Funding
The study was funded by the Second Tibetan Plateau Scientific Expedition and Research (STEP) program (Grant No. 2019QZKK0601) and the NSFC key project (Grant No. 41790422).
Acknowledgments
We thank Davide Tiranti for his valuable comments on the manuscript. We gratefully acknowledge the friendly and constructive comments of the two reviewers.
References
Appleby, P. G., and Oldfield, F. (1978). The calculation of lead-210 dates assuming a constant rate of supply of unsupported 210Pb to the sediment. Catena 5, 1–8. doi:10.1016/S0341-8162(78)80002-2
Chen, F., Chen, J., Huang, W., Chen, S., Huang, X., Jin, L., et al. (2019). Westerlies Asia and monsoonal Asia: spatiotemporal differences in climate change and possible mechanisms on decadal to sub-orbital timescales. Earth Sci. Rev. 192, 337–354. doi:10.1016/j.earscirev.2019.03.005
Chen, F., Huang, X., Zhang, J., Holmes, J. A., and Chen, J. (2006). Humid Little Ice age in arid central Asia documented by Bosten Lake, Xinjiang, China. Sci. China Ser. D 49, 1280–1290. doi:10.1007/s11430-006-2027-4
Chen, F., Yu, Z., Yang, M., Ito, E., Wang, S., Madsen, D. B., et al. (2008). Holocene moisture evolution in arid central Asia and its out-of-phase relationship with Asian monsoon history. Quat. Sci. Rev. 27, 351–364. doi:10.1016/j.quascirev.2007.10.017
Chen, F., Yuan, Y.-J., Wei, W.-S., Zhang, T.-W., Shang, H.-M., and Zhang, R. (2014). Precipitation reconstruction for the southern Altay Mountains (China) from tree rings of Siberian spruce, reveals recent wetting trend. Dendrochronologia 32, 266–272. doi:10.1016/j.dendro.2014.06.003
Cook, E. R., Woodhouse, C. A., Eakin, C. M., Meko, D. M., and Stahle, D. W. (2004). Long-term aridity changes in the western United States. Science 306, 1015–1018. doi:10.1126/science.1102586
Dai, A., Trenberth, K. E., and Qian, T. (2004). A global dataset of Palmer Drought Severity Index for 1870–2002: relationship with soil moisture and effects of surface warming. J. Hydrometeorol. 5, 1117–1130. doi:10.1175/JHM-386.1
Deng, Y., Gou, X., Gao, L., Zhao, Z., Cao, Z., and Yang, M. (2013). Aridity changes in the eastern Qilian Mountains since AD 1856 reconstructed from tree-rings. Quat. Int. 283, 78–84. doi:10.1016/j.quaint.2012.04.039
Dunhuang City Annals Compilation Committee (1994). Dunhuang city annals. Beijing, China: Xinhua Publishing House [in Chinese].
Esper, J., Cook, E. R., and Schweingruber1, F. H. (2002). Low-frequency signals in long tree-ring chronologies for reconstructing past temperature variability. Science 295, 2250–2253. doi:10.1126/science.1066208
Feng, H., and Zhang, M. (2015). Global land moisture trends: drier in dry and wetter in wet over land. Sci. Rep. 5, 18018. doi:10.1038/srep18018
Fontana, L., Sun, M., Huang, X., and Xiang, L. (2019). The impact of climate change and human activity on the ecological status of Bosten Lake, NW China, revealed by a diatom record for the last 2,000 years. Holocene 29, 1871–1884. doi:10.1177/0959683619865586
Gou, X., Gao, L., Deng, Y., Chen, F., Yang, M., and Still, C. (2015). An 850-year tree-ring-based reconstruction of drought history in the western Qilian Mountains of northwestern China. Int. J. Climatol. 35, 3308–3319. doi:10.1002/joc.4208
Gou, X., Zhang, F., Deng, Y., Ettl, G. J., Yang, M., Gao, L., et al. (2012). Patterns and dynamics of tree-line response to climate change in the eastern Qilian Mountains, northwestern China. Dendrochronologia 30, 121–126. doi:10.1016/j.dendro.2011.05.002
Grimm, E. C. (1987). CONISS: a FORTRAN 77 program for stratigraphically constrained cluster analysis by the method of incremental sum of squares. Comput. Geosci. 13, 13–35. doi:10.1016/0098-3004(87)90022-7
Grimm, E. C. (2011). Tilia 1.7.16 software. Springfield, IL: Illinois State Museum, Research and Collection Center.
Guan, X., Ma, J., Huang, J., Huang, R., Zhang, L., and Ma, Z. (2019). Impact of oceans on climate change in drylands. Sci. China Earth Sci. 62, 891–908. doi:10.1007/s11430-018-9317-8
Hou, Y., Wang, N. A., Zhang, X. M., Cheng, H. Y., and Lu, J. W. (2011). Precipitation reconstruction from tree ring width over the eastern part of the Qilian Mountains, northwestern China. J. Mt. Sci. 29, 12–18 [in Chinese]. doi:10.16089/j.cnki.1008-2786.2011.01.006
Huang, J., Yu, H., Guan, X., Wang, G., and Guo, R. (2016). Accelerated dryland expansion under climate change. Nat. Clim. Change 6, 166–171. doi:10.1038/nclimate2837
Huang, X., Peng, W., Rudaya, N., Grimm, E. C., Chen, X., Cao, X., et al. (2018). Holocene vegetation and climate dynamics in the Altai Mountains and surrounding areas. Geophys. Res. Lett. 45, 6628–6636. doi:10.1029/2018GL078028
Huang, X. Z., Chen, F. H., Fan, Y. X., and Yang, M. L. (2009). Dry late-glacial and early Holocene climate in arid central Asia indicated by lithological and palynological evidence from Bosten Lake, China. Quat. Int. 194, 19–27. doi:10.1016/j.quaint.2007.10.002
IPCC (2007). “WGI fourth assessment report, summary for policymakers,” in The 10th session of working group I of the IPCC, February 2, Paris, France.
Jiuquan City Annals Compilation Committee (2008). Jiuquan city annals. Beijing, China: Fangzhi Publishing House [in Chinese].
Kang, S., Yang, B., Qin, C., Wang, J., Shi, F., and Liu, J. (2013). Extreme drought events in the years 1877-1878, and 1928, in the southeast Qilian Mountains and the air-sea coupling system. Quat. Int. 283, 85–92. doi:10.1016/j.quaint.2012.03.011
Kang, X. C., Cheng, G. D., Chen, F. H., and Gou, X. H. (2003). A record of rought and flood series by tree-ring data in the middle section of Qilian Mountain since 904 A.D. J. Glaciol. Geocryol. 25, 518–525 [in Chinese]. doi:10.3969/j.issn.1000-0240.2003.05.007
Li, J., Chen, F., Cook, E. R., Gou, X., and Zhang, Y. (2007). Drought reconstruction for North Central China from tree rings: the value of the Palmer drought severity index. Int. J. Climatol. 27, 903–909. doi:10.1002/joc.1450
Li, J., Gou, X., Cook, E. R., and Chen, F. (2006). Tree-ring based drought reconstruction for the central Tien Shan area in northwest China. Geophys. Res. Lett. 33, L07715. doi:10.1029/2006gl025803
Li, Y. C., Xu, Q. H., Yang, X. L., and Zheng, Z. H. (2005). Pollen assemblages of major steppe communities in China. Acta Ecol. Sin. 25, 169–178 [in Chinese]. doi:10.3321/j.issn:1000-0933.2005.03.024
Li, Y. K., Zhang, L., Ye, Y., and Lu, Y. J. (2018). Drought event in the midwest region of northwest China in 1876–1878. Sci. Geogr. Sin. 38, 780–789 [in Chinese]. doi:10.13249/j.cnki.sgs.2018.05.016
Liu, X. H., An, W. L., Liang, E. Y., Wang, W. Z., Shao, X. M., Huang, L., et al. (2011). Spatiotemporal variability in tree ring’s δ13C of Picea crassifolia in the Qilian Mountains: climatic significance and responses to rising CO2. Sci. Cold Arid Reg. 3, 93–102 doi:10.3724/SP.J.1226.2011.00093
Mann, M. E., Bradley, R. S., and Hughes, M. K. (1998). Global-scale temperature patterns and climate forcing over the past six centuries. Nature 392, 779–787. doi:10.1038/33859
Shao, X., Huang, L., Liu, H. B., Liang, E. Y., Fang, X. Q., and Wang, L. L. (2005). Reconstruction of precipitation variation from tree rings in recent 1,000 years in Delingha, Qinghai. Sci. China Ser. D 48, 939–949. doi:10.1360/03yd0146
Sun, J., and Liu, Y. (2013). Drought variations in the middle Qilian Mountains, northeast Tibetan Plateau, over the last 450 years as reconstructed from tree rings. Dendrochronologia 31, 279–285. doi:10.1016/j.dendro.2012.07.004
Tang, L. Y., Mao, L. M., Shu, J. W., Li, C. H., Shen, C. M., and Zhou, Z. Z. (2016). An illustrated handbook of quaternary pollen and spores in China. Beijing, China: Science Press [in Chinese]. ISBN
Tarasov, P. E., Jolly, D., and Kaplan, J. O. (1997). A continuous late glacial and Holocene record of vegetation changes in Kazakhstan. Palaeogeogr. Palaeoclimatol. Palaeoecol. 136, 281–292. doi:10.1016/S0031-0182(97)00072-2
Wang, Y. J., Chen, F. H., Gou, X. H., and Du, S. Y. (2001). Study on response relationship between tree ring width and climate factors and climate reconstruction in middle region of Qilian Mountains. J. Desert Res. 21, 135–140 [in Chinese].doi:10.3321/j.issn:1000-694X.2001.02.006
Xu, Q. H., Li, Y. C., Yang, X. L., and Zheng, Z. H. (2007). Quantitative relationships between modern pollen rain and vegetation in Northern China. Sci. China Earth Sci. 37, 192–205 [in Chinese]. doi:10.3969/j.issn.1674-7240.2007.02.007
Yan, T. L., Wang, Z. T., He, J. Q., Huang, X. Z., Xia, D. S., Wang, and Z. L. (2018). A 3500 Year environmental changes recorded by sediment of Tian’E Lake, central part of the Qilian Mountains, China. Acta Sedimentol. Sin. 36, 521–530 [in Chinese]. doi:10.14027/j.issn.1000-0550.2018.041
Yang, B., Qin, C., Bräuning, A., Burchardt, I., and Liu, J. (2011). Rainfall history for the Hexi Corridor in the arid northwest China during the past 620 years derived from tree rings. Int. J. Climatol. 31, 1166–1176. doi:10.1002/joc.2143
Yang, B., Wang, J., and Liu, J. (2019). A 1556 year-long early summer moisture reconstruction for the Hexi Corridor, Northwestern China. Sci. China Earth Sci. 62, 953–963. doi:10.1007/s11430-018-9327-1
Yi, Y. Y., and Wang, H. Y. (2013). Characteristics of vertical distribution of vegetation community in the north slope of Qilian Mountains. Protect. For. Sci. Technol. 10, 6–9 [in Chinese]. doi:10.3969/j.issn.1005-5215.2013.10.003
Zhang, J., Huang, X., Wang, Z., Yan, T., and Zhang, E. Y. (2018). A late-Holocene pollen record from the western Qilian Mountains and its implications for climate change and human activity along the Silk Road, Northwestern China. Holocene 28, 1141–1150. doi:10.1177/0959683618761548
Zhang, K., Zhao, Y., Yu, Z., and Zhou, A. (2010). A 2700-year high resolution pollen record of climate change from varved Sugan Lake in the Qaidam Basin, northeastern Tibetan Plateau. Palaeogeogr. Palaeoclimatol. Palaeoecol. 297, 290–298. doi:10.1016/j.palaeo.2010.08.008
Zhang, P., Cheng, H., Edwards, R. L., Chen, F., Wang, Y., Yang, X., et al. (2008). A test of climate, sun, and culture relationships from an 1810-year Chinese cave record. Science 322, 940–942. doi:10.1126/science.1163965
Zhang, Y., Gou, X., Chen, F., Tian, Q., Yang, M., Peng, J., et al. (2009). A 1232-year tree-ring record of climate variability in the Qilian Mountains, Northwestern China. IAWA J. 30, 407–420. doi:10.1163/22941932-90000228
Zhang, Y., Tian, Q., Gou, X., Chen, F., Leavitt, S. W., and Wang, Y. (2011). Annual precipitation reconstruction since AD 775 based on tree rings from the Qilian Mountains, northwestern China. Int. J. Climatol. 31, 371–381. doi:10.1002/joc.2085
Zhao, Y., Liu, H., Li, F., Huang, X., Sun, J., Zhao, W., et al. (2012). Application and limitations of the Artemisia/Chenopodiaceae pollen ratio in arid and semi-arid China. Holocene 22, 1385–1392. doi:10.1177/0959683612449762
Zhao, Y., Xu, Q., Huang, X., Guo, X., and Tao, S. (2009). Differences of modern pollen assemblages from lake sediments and surface soils in arid and semi-arid China and their significance for pollen-based quantitative climate reconstruction. Rev. Palaeobot. Palynol. 156, 519–524. doi:10.1016/j.revpalbo.2009.05.001
Zhao, Y., Yu, Z., Chen, F., Liu, X., and Ito, E. (2008). Sensitive response of desert vegetation to moisture change based on a near-annual resolution pollen record from Gahai Lake in the Qaidam Basin, northwest China. Global Planet. Change 62, 107–114. doi:10.1016/j.gloplacha.2007.12.003
Zhao, Y., Yu, Z., Liu, X., Zhao, C., Chen, F., and Zhang, K. (2010). Late Holocene vegetation and climate oscillations in the Qaidam Basin of the northeastern Tibetan plateau. Quat. Res. 73, 59–69 [in Chinese]. doi:10.1016/j.yqres.2008.11.007
Keywords: western Qilian Mountains, A/C ratio, tree rings, global warming, humidity changes
Citation: Wang J, Huang X, Zhang J, Xiang L, Xiao Y, Fontana L, Ren X and Wang Z (2020) Pollen Record of Humidity Changes in the Arid Western Qilian Mountains Over the Past 300 Years and Comparison With Tree-Ring Reconstructions. Front. Earth Sci. 8:562426. doi:10.3389/feart.2020.562426
Received: 18 May 2020; Accepted: 17 August 2020;
Published: 09 September 2020.
Edited by:
Davide Tiranti, Agenzia Regionale per la Protezione Ambientale (ARPA), ItalyReviewed by:
Santosh Kumar Shah, Birbal Sahni Institute of Palaeobotany, IndiaOlga Nikolaevna Solomina, Institute of Geography (RAS), Russia
Copyright © 2020 Wang, Huang, Zhang, Xiang, Xiao, Fontana, Ren and Wang. This is an open-access article distributed under the terms of the Creative Commons Attribution License (CC BY). The use, distribution or reproduction in other forums is permitted, provided the original author(s) and the copyright owner(s) are credited and that the original publication in this journal is cited, in accordance with accepted academic practice. No use, distribution or reproduction is permitted which does not comply with these terms.
*Correspondence: Xiaozhong Huang, xzhuang@lzu.edu.cn, Zongli Wang, zlwang@lzu.edu.cn