- 1Institute of Desertification Studies, Chinese Academy of Forestry, Beijing, China
- 2Oregon State University Agriculture Program at Eastern Oregon University, Oregon State University, La Grande, OR, United States
Summer rain is changeable in the semi-arid climate and is the limiting factor to the survival and growth of sand-fixing vegetation, especially in the Tibetan Plateau. Caragana intermedia is the dominant sand-binding shrub on a sand dune in the northeast Tibetan Plateau. Stable hydrogen and oxygen isotopes of xylem water, soil water, and groundwater were examined to study the rainwater uptake process by C. intermedia in mid-summer. Soil water content was monitored continuously before and after a 13.9-mm rain. Contribution of each water source was analyzed by the MixSIAR model. The results showed that the surface soil water (0−10 cm) was replenished by rainwater through increasing soil water content and reducing its stable isotope. Caragana intermedia mainly used surface soil water (0−10 cm) replenished by rainwater one day after the rain, accounting for about 60% of its total water source. As water availability decreased in upper soil layers, it used soil water at deeper levels (10−150 cm), the same water source as that one day before the rain. The rapid and efficient uptake of rainwater by C. intermedia reflected its response to summer rain, which is an adaptive strategy to the semi-arid environment. Therefore, C. intermedia could survive on a sand dune by shallow soil water replenished from rainwater in the Tibetan Plateau.
Introduction
In drylands, water is an important restricting factor for the growth, survival, and reproduction of plant species (Hou et al., 2013), where precipitation is low and changeable, potential evaporation is high, and drought is frequent. Based on the prediction of the climate change model, the extreme precipitation in the arid and semi-arid zones would increase in frequency under global warming. Moreover, total water resource would decrease and thus result in negative influence on the ecosystem (IPCC, 2013). Precipitation reduction combined with warming will modify water sources used by trees (Grossiord et al., 2017). Therefore, the reliance on rainwater in the growing season is important for plant phenology (flowering and fruit maturation) in semi-arid zones (Xia and Wan, 2012). Water use adaptation of plant species affects many ecological processes including plant growth, population regeneration, vegetation dynamics, and ecosystem water cycle. For most plant species, stable isotopes of hydrogen and oxygen usually do not fractionate when root systems absorb water from soil and plants transport water from root systems to branches. Therefore, the water source of a plant could be determined by comparing δD and δ18O of sap water and its possible water sources (Ehleringer, 1993). The possible water sources for plants in different seasons were rainwater (Zhou et al., 2015), snow melt (Yang et al., 2011), soil water, and/or groundwater (Kray et al., 2012; Song et al., 2014; Su et al., 2014; Liu et al., 2015; Barbeta and Peñuelas, 2017).
Precipitation is a driving factor of soil water availability in drylands, where small rain (2−5 mm) is the most common occurrence. Its contribution to total precipitation is quite constant for years. The importance of small rain is significantly related to mean annual precipitation and air temperature, which was most at the coolest and driest sites. However, large rain above 30 mm varies significantly among years and is a major source of annual variability in ecological functioning (Loik et al., 2004). Generally, snow in winter and early spring and large rain in summer could infiltrate to a deeper soil depth if their duration time is longer than small rain (Schwinning and Sala, 2004). The uptake of precipitation by plant species is related to its seasonal distribution (Yang et al., 2011). Many woody plants primarily used shallow soil water replenished by rainwater in spring and summer, such as Senecio filaginoides and Mulinum spinosum in the Patagonian steppe (Kowaljow and Fernández, 2011) and Haloxylon ammodendron in Gurbantonggut Desert (Dai et al., 2015). However, some woody plants mainly used deeper soil water replenished by snow in winter or early spring and large rain in summer, such as Sarcobatus vermiculatus in Colorado (Kray et al., 2012), Nitraria sibirica in Gurbantonggut Desert (Zhou et al., 2015) and Caragana microphylla in the Inner Mongolia steppe (Zheng et al., 2015).
Rain amount not only affects the depth and time of water infiltration into soil (Yang et al., 2014) but also affects the contribution of rainwater to plant growth (Duan et al., 2008). The interspecific difference of rain amount that plants use is related to their life forms (Dodd et al., 1998; Antunes et al., 2018). Annual plants initially use small rain (2−5 mm). Perennial grasses or herbs and small shrubs use light rain (10−20 mm). However, shrubs or trees might use heavy rain (65.3 mm) (Cheng et al., 2006). Water is absorbed primarily by root systems; therefore, the duration of soil water use depends on root distribution especially in arid zones (Zhou et al., 2015). Shallow-rooted plants with fine root systems are sensitive to small rain (Zhou et al., 2018). Deep-rooted plants or plants with a tap root respond to large rain after a few days (Li et al., 2007). However, some woody plants have dimorphic root systems and thus they can change water sources in different periods and use shallow soil water after precipitation but extract deeper soil water, even groundwater, during drought (Weltzin and Tissue, 2003). Thus, they can benefit from small or large rain allowing the plant to access water sources in different seasons based on availability (Kowaljow and Fernández, 2011; Zhou et al., 2015).
In the semi-arid zone of the Tibetan Plateau with 250−500 mm annual precipitation, water is the limiting factor for vegetation restoration in land desertification. Plants in different habitats may have different water use strategies based on water availability. For shrubs on interdunes, deep soil water replenished by groundwater is available, such as Salix cheilophila and Salix psammophila (Zhu et al., 2016). However, shrubs on sand dunes may rely on soil water replenished by rainwater, such as Caragana intermedia. Our earlier research indicated older C. intermedia (9- and 25-year-old) used deeper soil water in summer than younger C. intermedia (5-year-old) to adapt to the semi-arid climate (Jia et al., 2012). Moreover, this shrub had a dynamic water use strategy to access different depths of soil water and groundwater (Zhu, 2016). In this study, rainwater uptake of C. intermedia was investigated by stable hydrogen and oxygen isotopes before and after a summer rain. Our purpose was to evaluate the response of C. intermedia to summer rain and the contribution of rainwater to its water sources. This study will help to evaluate its stability on sand dunes under a semi-arid climate and give theoretical support to desertification restoration in the Tibetan Plateau.
Materials and Methods
Site Description
A field survey was conducted in Shazhuyu Town, Gonghe County, Qinghai Province (36°16′N, 100°16′E, and elevation 2874 m), located in the northeast Tibetan Plateau. The area has a temperate climate in a semi-arid zone. The average annual air temperature is 2.4°C, and the average air temperature is 12.6°C from May to September. The average annual precipitation is 246.3 mm, mainly occurring in summer and autumn. The average monthly precipitation was 29.3, 51.5, 70.5, 39.8, and 41.8 mm from May to September. The average annual potential evaporation is 1716.1 mm. There are only 91 frost-free days per year. The main soil type is aeolian sand, containing 6.86% clay, 8.93% silt, and 84.21% sand, with the pH of 8.61 (Li et al., 2016). The contents of soil organic matter, nitrogen, phosphorus, and potassium on the surface (0−5 cm) are 2.29−3.49, 0.35−0.55, 0.21−0.44, and 11.84−14.82 g⋅kg–1, respectively (Li et al., 2015). The natural vegetation is dominated by grassland (Achnatherum splendens). Shrub land is distributed on sand dunes and river banks (Artemisia arenaria, Nitraria sibirica, and Caragana tibetica). Sand-fixing shrubs in Caragana and Salix were planted between oasis and sand dunes to protect farms, roads, and villages.
Plant Species
Caragana intermedia Kuang et H.C.Fu (Caragana liouana Zhao Y. Chang & Yakovlev) is a leguminous shrub with a height of 1.5 m (Flora of China, 1993). The branches are yellowish gray–green. The leaves are pinnate with 6−16 foliolate. The leaflet blades are elliptic to obovate–elliptic, with sizes of 3−10 mm × 4−6 mm, whitish green, and villous in both surfaces. The corolla is yellow and 2−2.5 cm long. The pod is flat, lanceolate to oblong–lanceolate, with size of 2.5−3.5 cm × 6−7 mm. It mainly distributes on fixed and semi-fixed sand dunes in Gansu, North Hebei, Inner Mongolia, Ningxia, Shaanxi, and west Shanxi. It was used widely in sand-fixing and water and soil conservation in North China. It was introduced in the 1980s from Ordos City of Inner Mongolia to control land desertification on the Tibetan Plateau. This shrub blooms in May, and its legume matures in July in our study site. Caragana intermedia were seeded with the density of one plant per 1 m2, after the straw checkerboard was constructed on moving sand dunes in the spring of 2000. The root system of C. intermedia is 1.30 m deep (Jia et al., 2012). A few grasses and herbs grew in the C. intermedia community, including Leymus secalinus, Bassia dasyphylla, and Chenopodium foetidum.
Field Sample and Lab Measurements
Field samples were taken in July 2016. On July 13, 20 C. intermedia plants were selected randomly to measure their height and crown diameter. The mean plant height of C. intermedia was 1.51 ± 0.22 m, with the mean crown diameter of 1.42 ± 0.19 m (E–W) and 1.63 ± 0.25 m (S–N), which was defined as standard plant. Four plots with 5 m × 5 m size were set randomly in the C. intermedia community for field sampling. Twig samples were taken from four plants per plot which were similar to the standard plant. Lignified, 2-year-old twigs 5 cm in length and 3–5 mm in diameter were clipped from the sunny side of four shrubs on July 13, 15, 19, and 27, which was one day before and 1, 5, and 13 days after the rain (13.9 mm) on July 14. The bark was removed because the phloem water is isotopically enriched by photosynthesis and the samples of xylem were kept in 8-mL glass vials (National Scientific Company, Rockwood, TN, United States). Rainwater was collected in July in Shazhuyu Town. Groundwater was replaced by well water and collected on July 17 from a well with a 5-m water table, which was located 1 km north of the study site and not bailed during the previous 2 months. Three replicates of rainwater and groundwater were kept in 8-mL glass vials, respectively. All vials were immediately sealed with Parafilm® (Alcan Packaging, Chicago, IL, United States) and kept in a 16-L medical cooler containing dry ice.
Soil samples were collected at the middle point of each plot using a soil auger with 6.99 cm diameter (AMS Inc., American Falls, ID, United States). Soil sampling depths were 10, 25, 50, 75, 100, and 150 cm, based on the rooting depth of C. intermedia. Some soil samples with four replicates were kept and stored as similar to xylem samples. Other soil samples with four replicates were kept in aluminum cans. Wet soil mass was measured by an electronic balance (± 0.01 g) and dried at 105°C for 24 h, and dry soil mass was measured, then soil water content (g kg–1) was calculated as the loss of water.
Soil and xylem samples were stored frozen (−18°C) in the laboratory. Water in soil and xylem samples was extracted by the LI-2000 water vacuum extract system (LICA United Technology Limited, Beijing, China). Rainwater and groundwater samples were filtered prior to analysis. The δD and δ18O of water samples were measured with a Flash 2000 HT elemental analyzer and a Finnigan MAT 253 mass spectrometer (Thermo Fisher Scientific Co., Ltd., Bremen, Germany) in the Stable Isotope Ecology Laboratory, Department of Earth System Science, Tsinghua University. The measurement accuracy for stable hydrogen and oxygen isotope was ±1 and ±0.2%, respectively. Each water sample was measured three times, and their mean was used for analysis.
Statistical Analysis
Soil water content, δD, and δ18O were expressed as mean ± SE. One-sample Kolmogorov–Smirnov test and log transformations were used to check and satisfy normality, then one-way ANOVA in SPSS 19.0 (IBM Corp., Armonk, NY, United States) was applied to analyze the effects of time and depth on soil water content. If there was a significant effect (P < 0.05), Duncan’s multiple-range test was applied to analyze the difference between times or depths. The water use ratio to different sources was analyzed by the MixSIAR Bayesian isotope mixing model (v2.1.3) (Stock et al., 2018). The input data of the model were δD and δ18O of xylem water, soil water in three layers (0−10, 10−50, and 50−150 cm), and groundwater. The discrimination value was set to 0 for both isotopes, because isotopes usually do not fractionate during plant uptake water (Ehleringer, 1993). Parameter configuration was referred to Ma and Song (2016), including run length of Markov Chain Monte Carlo occurrence, the error options of “residual error” and “process error.” Trace plots and the diagnostic tests Gelman–Rubin, Heidelberger–Welch, and Geweke were applied to define if the model converged or not. The estimated median proportion was analyzed for comparisons, which was the median source contribution for each water source. The contribution of rainwater was assumed to be present at surface soil water (0−10 cm).
Results
Soil Water Dynamics Before and After the Rain
Soil water content in the C. intermedia community was significantly influenced by sampling date (P < 0.001) and soil depth (P < 0.001). One day before the rain and on day one and day five afterward, soil water content was significantly higher at 10−50 cm than at 75−150 cm. Thirteen days after the rain, soil water content was significantly higher at 10 cm than at 25−150 cm. Soil water content at 0−25 cm was significantly higher one day after the rain than on other days (Figure 1).
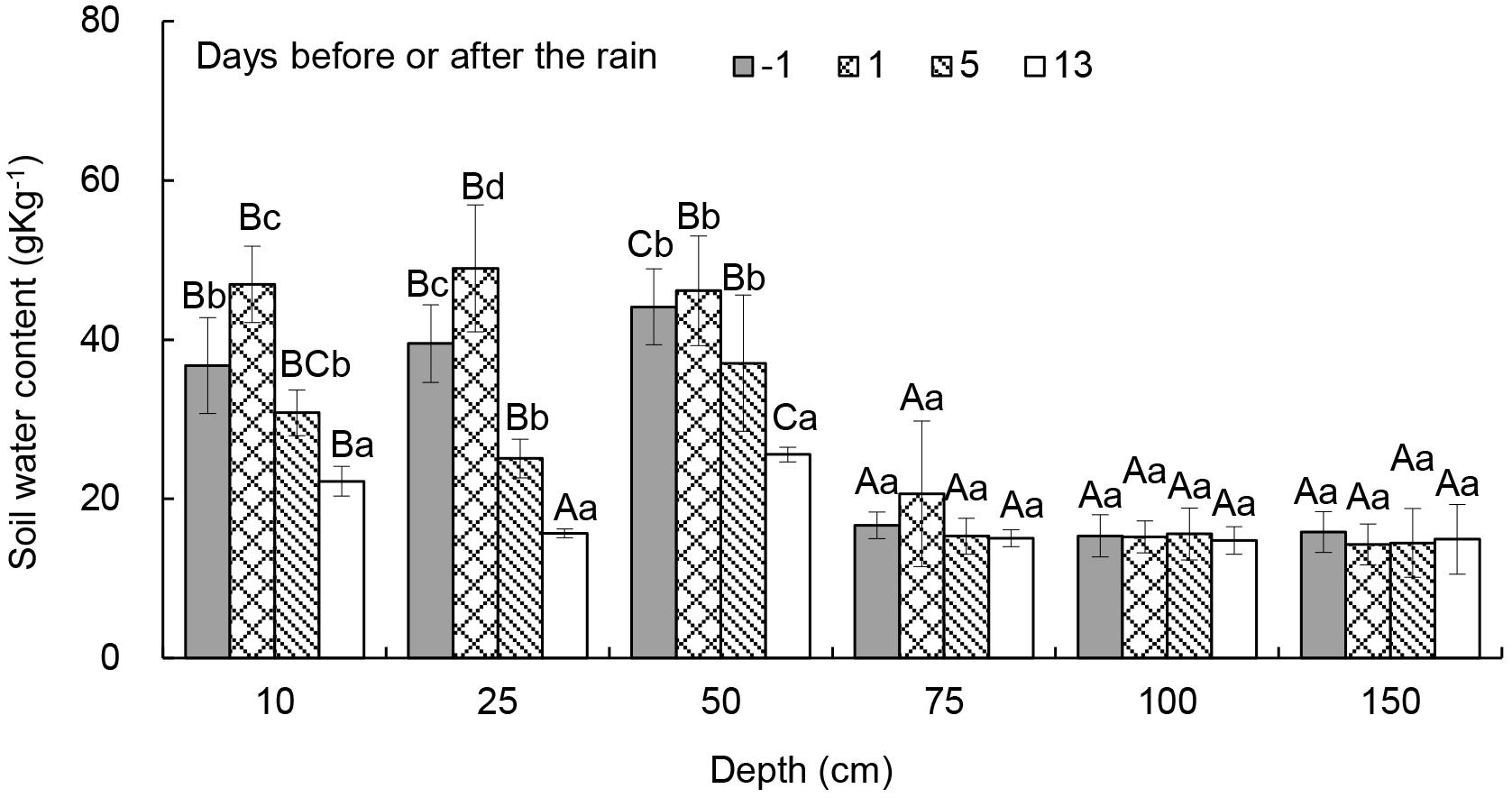
Figure 1. Soil water content in the Caragana intermedia community 1 day before and 1, 5, and 13 days after the rain on July 14, 2016. Different uppercase letters mean significant difference between days; different lowercase letters mean significant difference between depths.
The δD and δ18O of groundwater were similar to some soil water (150 cm), indicating that groundwater replenished deep soil water (Figure 2). On July 15, one day after the rain, the δD and δ18O of surface soil water (10 cm) were similar to rainwater on July 14 (13.9 mm) (Figure 3). On July 27, the δD and δ18O of surface soil water were similar to rainwater on July 22 (10.1 mm), indicating rainwater replenished surface soil water.
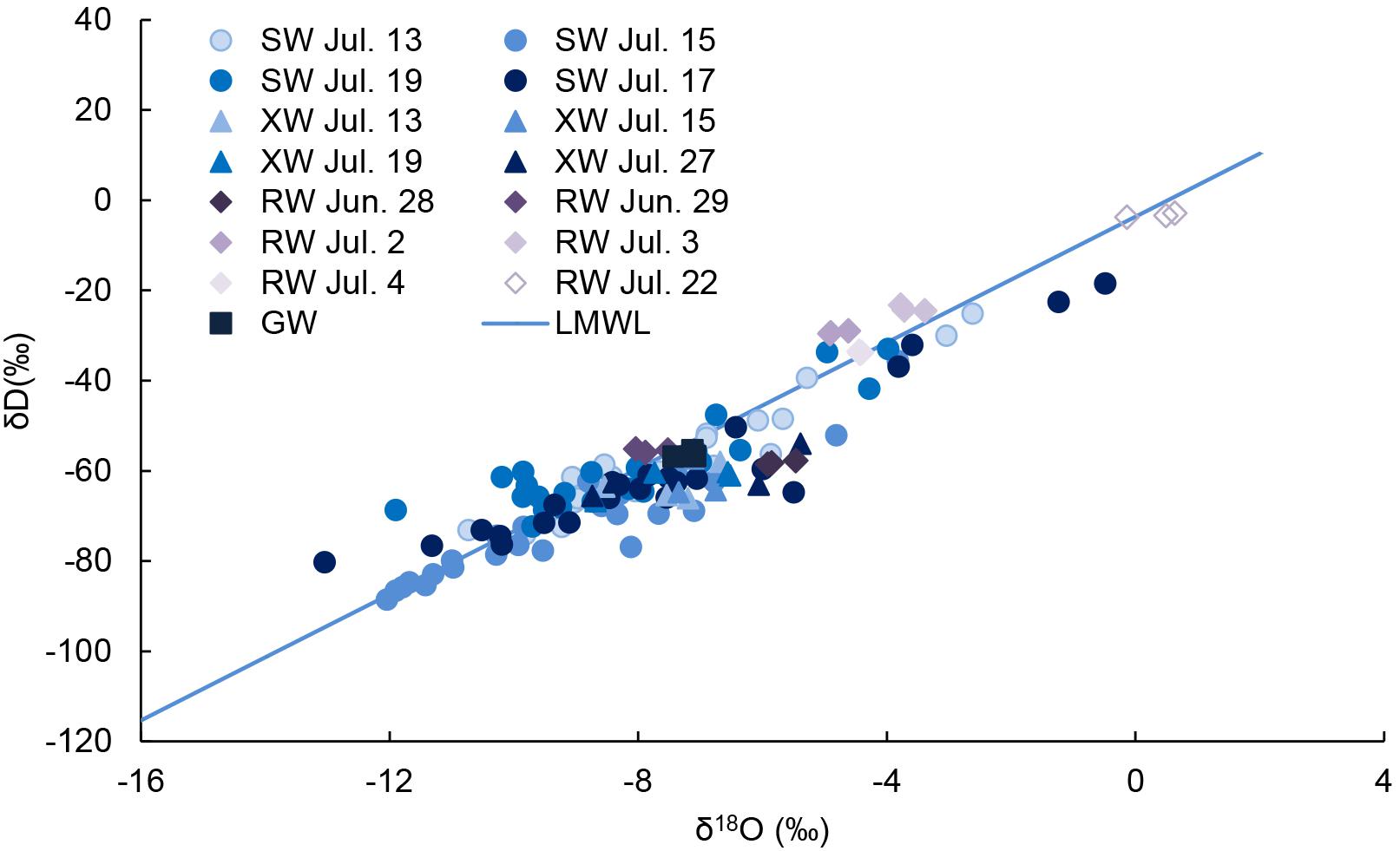
Figure 2. The δD and δ18O of xylem water (XW) of Caragana intermedia, soil water (SW), rainwater (RW), and groundwater (GW) in July 2016. LMWL is the local meteoric water line (δD = 6.977 × δ18O–3.668, R2 = 0.849, P < 0.001).
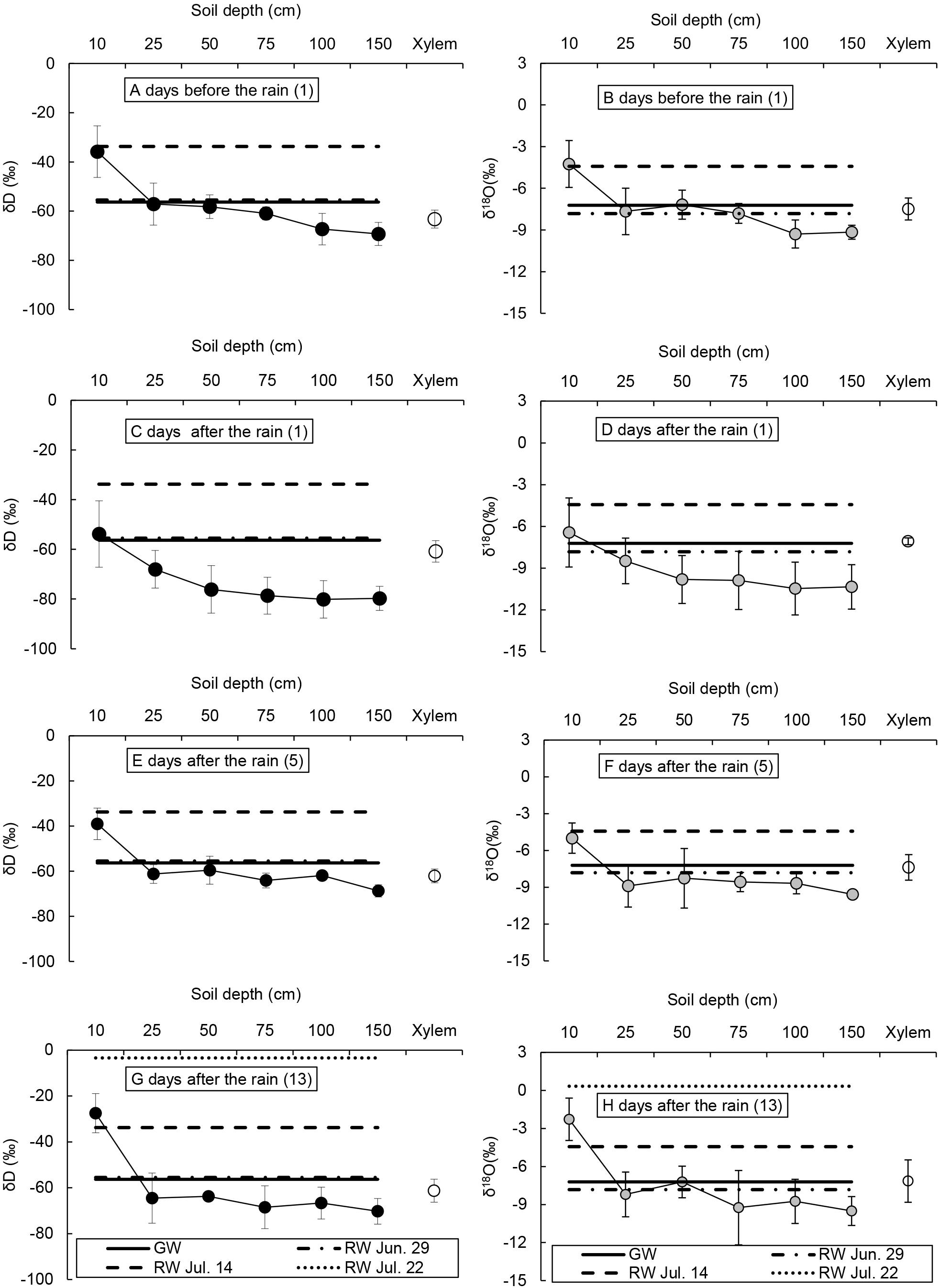
Figure 3. The δD (A,C,E,G) and δ18O (B,D,F,H) of xylem water and soil water of the Caragana intermedia community, rainwater (RW), and groundwater (GW) in the summer of 2016.
Water Sources of Caragana intermedia
The δ18O of xylem water in C. intermedia was located under the local meteoric water line (Figure 2), indicating its water source was influenced by isotope enrichment which resulted from evaporation. The δD and δ18O of xylem water of C. intermedia were similar to those of groundwater, indicating it extracted some groundwater.
One day before the rain, the δD and δ18O of xylem water of C. intermedia were similar to soil water at 25 cm and 75 − 150 cm (Figures 3A,B). One day after the rain, the δD and δ18O of xylem water of C. intermedia were similar to soil water at 10 − 25 cm and groundwater (Figures 3C,D). Five days after the rain, the δD and δ18O of xylem water of C. intermedia were similar to soil water at 25−100 cm and groundwater (Figures 3E,F). After 2 weeks, the δD and δ18O of C. intermedia xylem water were similar to soil water at 25−150 cm and groundwater (Figures 3G,H).
The Contribution of Different Water Sources
Caragana intermedia changed its main water source before and after the rain. It mainly used 10−150 cm soil water and groundwater one day before the rain, accounting for 96.3% of the total water source (Figure 4). One day after the rain, it mainly used 0−50 cm soil water and groundwater, accounting for 93.4% of its total water source. With the time elapsed after the rain, the water source of this shrub moved into deeper soil profiles. Five days after the rain, the water source of this shrub returned to pre-rain status and mainly used 10−150 cm soil water and groundwater, accounting for 94.5% of its total water source. Two weeks after the rain, this shrub mainly used 10−150 cm soil water and groundwater, accounting for 91.6% of its total water source. The contribution of 0−10 cm surface soil water (replenished by rainwater) was 59.9% of its total water source one day after the rain. Moreover, C. intermedia always used groundwater before and after the rain, accounting for 18.5−21.3% of its total water source.
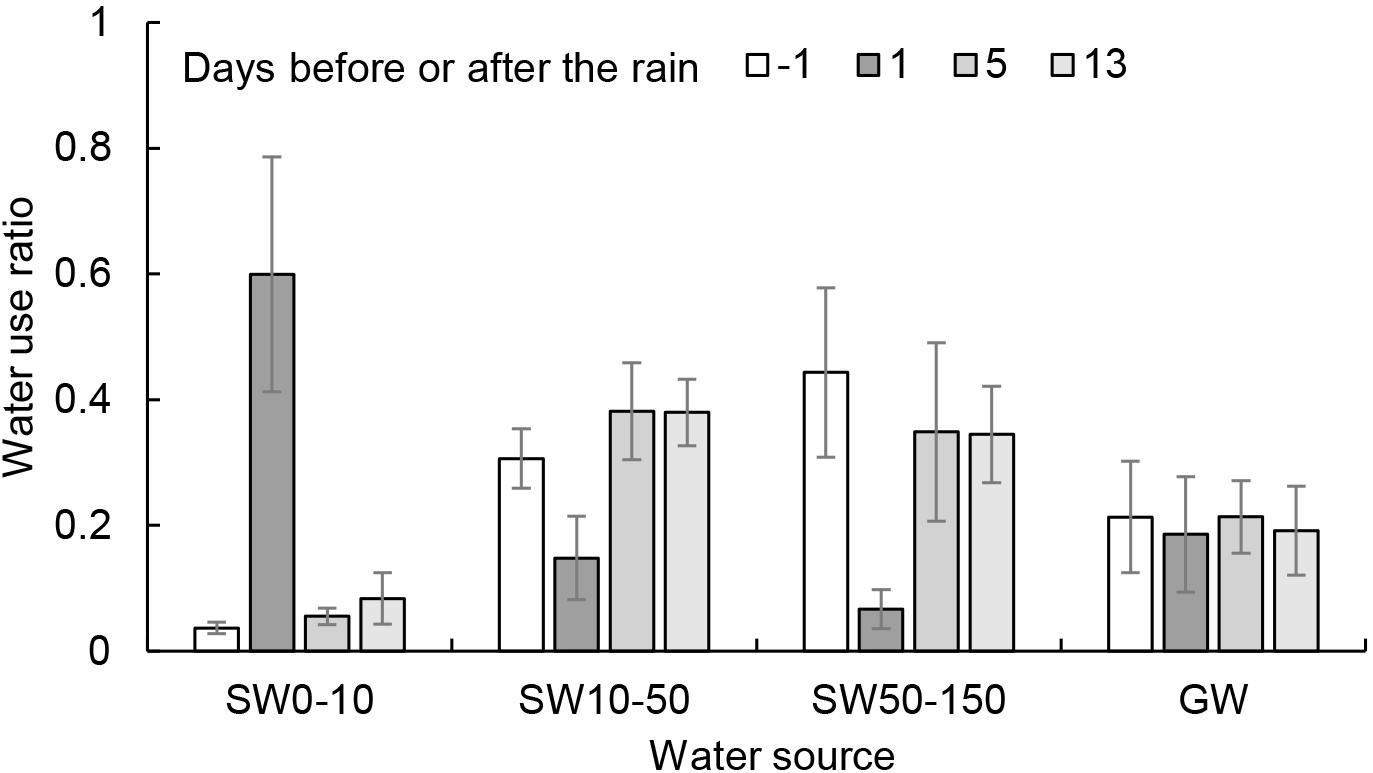
Figure 4. Water use ratio (mean ± SD) of Caragana intermedia to different layers of soil water (SW) and groundwater (GW).
Discussion
Our research showed that shallow soil water was replenished by rainwater in the C. intermedia community. One day after the rain, soil water content was increased at shallow depths (0−25 cm) than one day before the rain. Moreover, rainwater decreased the δD and δ18O of surface soil water. However, 5 days after the rain, soil water content at shallow depths was restored; meanwhile, the δD and δ18O of shallow soil water recovered to those one day before the rain. Therefore, the 13.9 mm rain had influence on soil water of sand dunes only for one day. Soil water depletion and isotope enrichment at shallow depths resulted from evaporation, rainwater infiltration, and plant consumption, similarly on sand dunes in Gurbantonggut Desert (Dai et al., 2015) and Tengger Desert (Huang and Zhang, 2015).
Caragana intermedia was responsive to summer rain and used rainwater one day after the rain. The contribution of 0−10 cm surface soil water replenished by rainwater accounted for approximately 60% of its total water source. When soil water was depleted in the shallow profile, C. intermedia used deeper soil water (10−150 cm). The rapid uptake of surface soil water replenished by rainwater was an adaptation for the growth of C. intermedia. It not only maximized the extraction of shallow soil water before evaporation loss but also had the opportunity for uptake nutrients in shallow soil (Antunes et al., 2018). Caragana intermedia used some groundwater before and after the rain, accounting for about 20% of its total water source. The consistent uptake of groundwater in summer may be important for the survival, especially the maintenance of metabolic activity of fine roots in shallow soil during drought (Antunes et al., 2018). For C. intermedia, summer rain is a changeable water source under a semi-arid climate, however, groundwater is a relatively reliable water source. The ability to use both rainwater and groundwater ensures the stability of the C. intermedia community on sand dune. Therefore, C. intermedia has a resource-dependent water use function adapted to a semi-arid climate. Other Caragana species also used rain in arid and semiarid zones. For example, C. microphylla in the Inner Mongolia steppe used summer rain, accounting for 42.65–63.92% of its water source (Zheng et al., 2015). After a 9.5 mm rainfall in southeast Tengger Desert, Caragana korshinskii mainly used water from deeper soil layers (100–150 cm) with soil moisture infiltration (Huang and Zhang, 2015). However, C. korshinskii used surface soil water replenished by rainwater in spring and autumn on sand dune in the Tibetan Plateau (Zhu, 2016). Therefore, Caragana shrubs appear to have different water use strategies based on water availability to adapt to drylands.
Many shrubs or trees use soil water replenished by precipitation in different seasons in drylands. Some shrubs use surface soil water replenished by rainwater in spring or summer. For example, rainwater accounted for 42.6% of xylem water of Nitraria tangutorum in July in Golmud, Tibetan Plateau (Duan et al., 2008). The proportion of surface pulse water to total water uptake was 29−38 and 22−32% for Senecio filaginoides and Mulinum spinosum in early summer in the Patagonia steppe, respectively (Kowaljow and Fernández, 2011). Sarcobatus vermiculatus used precipitation from soil layers after large rainfall in Colorado (Kray et al., 2012). Artemisia ordosica intensively depended on shallow soil water (10−20 cm) after a 9.5 mm summer rain in southeast Tengger Desert (Huang and Zhang, 2015). In interdunes in the Tibetan Plateau, Salix psammophila and Salix cheilophila depended on 0−25 cm shallow soil water replenished by rainwater in spring and summer, respectively (Zhu et al., 2016). In the Heihe River Basin of northwest China, Nitraria sphaerocarpa used summer rainwater more rapidly than Reaumuria soongorica (Zhang et al., 2017). Xerophytic shrubs relied on 10 cm top soil water in spring and summer in semi-arid coastal dune systems of southwest Spain (Antunes et al., 2018). In addition, other shrubs use soil moisture replenished by snow in winter or early spring. For example, Pinus edulis and Juniperus osteosperma utilized winter-derived shallow moisture during spring in South Utah (West et al., 2007). Nitraria sibirica mainly used water from snow melt in Gurbantonggut Desert (Zhou et al., 2015). In early spring after snow melt, Haloxylon ammodendron on interdune primarily used shallow soil water (0−40 cm) in Gurbantonggut Desert (Dai et al., 2015). Therefore, plants could survive in drylands depending on soil water replenished by precipitation in the growing season.
Conclusion
In the Tibetan Plateau, surface soil water (0−10 cm) on sand dune was replenished and its hydrogen and oxygen isotopes were reduced by a 13.9 mm rain. Caragana intermedia was rapidly responsive to rainfall and mainly used surface soil water one day after the rain. The contribution of rainwater to C. intermedia was approximately 60% of its total water source. With the decrease in soil water availability at shallow depths, this shrub was able to access soil water at deeper depths (25−150 cm). The rapid uptake of summer rain of C. intermedia is an adaptation to a semi-arid climate, which may benefit nutrient uptake and its survival. Therefore, summer rain is an important water source to maintain the stability of sand-fixing vegetation on sand dune in the Tibetan Plateau.
Data Availability Statement
The raw data supporting the conclusions of this article will be made available by the authors, without undue reservation. Requests to access the datasets should be directed to YZ, zhuyajuan8005@sohu.com.
Author Contributions
YZ designed this study, collected the sample, analyzed the data, prepared the figures, and wrote the main manuscript. GW contributed to improve the manuscript. Both authors reviewed the manuscript.
Funding
This study was financially supported by the Fundamental Research Funds for the Central Non-profit Research Institute of Chinese Academy of Forestry (CAFZC2017M006) and the National Natural Science Foundation of China (41301095).
Conflict of Interest
The authors declare that the research was conducted in the absence of any commercial or financial relationships that could be construed as a potential conflict of interest.
Acknowledgments
The authors thank Ms. Anne Nyren and Mr. Paul Nyren from North Dakota State University for the contribution in refining the manuscript. We thank the two reviewers for their valuable suggestions.
References
Antunes, C., Díaz-Barradas, M. C., Zunzunegui, M., Vieira, S., and Máguas, C. (2018). Water source partitioning among plant functional types in a semi-arid dune ecosystem. J. Veg. Sci. 29, 671–683. doi: 10.1111/jvs.12647
Barbeta, A., and Peñuelas, J. (2017). Relative contribution of groundwater to plant transpiration estimated with stable isotopes. Sci. Rep. 7:10580.
Cheng, X., An, S., Li, B., Chen, J., Lin, G., Liu, Y., et al. (2006). Summer rain pulse size and rainwater uptake by three dominant desert plants in a desertified grassland ecosystem in northwestern China. Plant Ecol. 184, 1–12. doi: 10.1007/s11258-005-9047-6
Dai, Y., Zheng, X.-J., Tang, L.-S., and Li, Y. (2015). Stable oxygen isotopes reveal distinct water use patterns of two Haloxylon species in the Gurbantonggut Desert. Plant Soil 389, 73–87. doi: 10.1007/s11104-014-2342-z
Dodd, M. B., Lauenroth, W. K., and Welker, J. M. (1998). Differential water resource use by herbaceous and woody plant life forms in a short grass steppe community. Oecologia 117, 504–512. doi: 10.1007/s004420050686
Duan, D.-Y., Ouyang, H., Song, M.-H., and Hu, Q.-W. (2008). Water sources of dominant species in three alpine ecosystems on the Tibetan Plateau. China. J. Integr. Plant Biol. 50, 257–264. doi: 10.1111/j.1744-7909.2007.00633.x
Ehleringer, J. R. (1993). “Carbon and water relations in desert plants: an isotopic perspective,” in Stable Isotope and Plant Carbon-Water Relations, eds J. R. Ehleringer, A. E. Hall, and G. D. Farquhar (San Diego: Academic Press), 155–172. doi: 10.1016/b978-0-08-091801-3.50018-0
Flora of China (1993). Caragana Intermedia. Avaliable at: http://foc.iplant.cn/content.aspx?TaxonId=250094187 (accessed January 9, 2020).
Grossiord, C., Sevanto, S., Dawson, T. E., Adams, H. D., Collins, A. D., Dickman, L. T., et al. (2017). Warming combined with more extreme precipitation regimes modifies the water sources used by trees. New Phytol. 213, 584–596. doi: 10.1111/nph.14192
Hou, Y., Zhou, G., Xu, Z., Liu, T., and Zhang, X. (2013). Interactive effects of warming and increased precipitation on community structure and composition in an annual forb dominated desert steppe. PLoS One 8:e70114. doi: 10.1371/journal.pone.0070114
Huang, L., and Zhang, A. (2015). Stable isotopic analysis on water utilization of two xerophytic shrubs in a revegetated desert area: tengger desert, China. Water 7, 1030–1045. doi: 10.3390/w7031030
IPCC (2013). Climate Change 2013. The Physical Science Basis. New York, NY: Cambridge University Press.
Jia, Z., Zhu, Y., and Liu, L. (2012). Different water use strategies of juvenile and adult Caragana intermedia plantations in the Gonghe Basin, Tibet Plateau. PLoS One 7:e45902. doi: 10.1371/journal.pone.0045902
Kowaljow, E., and Fernández, R. J. (2011). Different utilization of a shallow-water pulse by six shrub species in the Patagonian steppe. J. Arid Environ. 75, 211–214. doi: 10.1016/j.jaridenv.2010.10.004
Kray, J. A., Cooper, D. J., and Sanderson, J. S. (2012). Groundwater use by native plants in response to changes in precipitation in an intermountain basin. J. Arid Environ. 83, 25–34. doi: 10.1016/j.jaridenv.2012.03.009
Li, Q., Jia, Z., Zhu, Y., Wang, Y., Li, H., Yang, D., et al. (2015). Spatial heterogeneity of soil nutrients after the establishment of Caragana intermedia plantation on sand dunes in alpine sandy land of the Tibet Plateau. PLoS One 10:e124456. doi: 10.1371/journal.pone.0124456
Li, S., Romero-Saltos, H., Tsujimura, M., Sugimoto, A., Sasaki, L., Davaa, G., et al. (2007). Plant water sources in the cold semiarid ecosystem of the upper Kherlen River catchment in Mongolia: a stable isotope approach. J. Hydrol. 333, 109–117. doi: 10.1016/j.jhydrol.2006.07.020
Li, S., Wang, X. Q., Gao, Q., Bao, Y., and Yin, S. (2016). Influence of soil heterogeneity based on vegetation recovery in alpine sandy land. Forest Res. 29, 553–559.
Liu, S., Chen, Y., Chen, Y., Friedman, J. M., Hati, J. H. A., and Fang, G. (2015). Use of 2H and 18O stable isotopes to investigate water sources for different ages of Populus euphratica along the lower Heihe River. Ecol. Res. 30, 581–587. doi: 10.1007/s11284-015-1270-6
Loik, M. E., Breshears, D. D., Lauenroth, W. K., and Belnap, J. (2004). A multi-scale perspective of water pulses in dryland ecosystems: climatology and ecohydrology of the western USA. Oecologia 141, 269–281. doi: 10.1007/s00442-004-1570-y
Ma, Y., and Song, X. F. (2016). Using stable isotopes to determine seasonal variations in water uptake of summer maize under different fertilization treatments. Sci. Total Environ. 550, 471–483. doi: 10.1016/j.scitotenv.2016.01.148
Schwinning, S., and Sala, O. E. (2004). Hierarchy of responses to resource pulses in arid and semi-arid ecosystems. Oecologia 141, 211–220. doi: 10.1007/s00442-004-1520-8
Song, L., Zhu, J., Li, M., and Yu, Z. (2014). Water utilization of Pinus sylvestris var. mongolica in a sparse wood grassland in the semiarid sandy region of Northeast China. Trees 28, 971–982. doi: 10.1007/s00468-014-1010-5
Stock, B. C., Jackson, A., Ward, E. J., Parnell, A. C., Phillips, D. I., and Semmens, B. X. (2018). Analyzing mixing systems using a new generation of Bayesian tracer mixing models. PeerJ 6: e5096. doi: 10.7717/peerj.5096
Su, H., Li, Y., Liu, W., Xu, H., and Sun, J. O. (2014). Changes in water use with growth in Ulmus pumila in semiarid sandy land of northern China. Trees 28, 41–52. doi: 10.1007/s00468-013-0928-3
Weltzin, J. F., and Tissue, D. T. (2003). Resource pulses in arid environments – patterns of rain, patterns of life. New Phytol. 157, 171–173. doi: 10.1046/j.1469-8137.2003.00672.x
West, A. G., Hultine, K. R., Burtch, K. G., and Ehleringer, J. R. (2007). Seasonal variation in moisture use in a piñon-juniper woodland. Oecologia 153, 787–798. doi: 10.1007/s00442-007-0777-0
Xia, J., and Wan, S. (2012). The effects of warming-shifted plant phenology on ecosystem carbon exchange are regulated by precipitation in a semi-arid grassland. PLoS One 7:e32088. doi: 10.1371/journal.pone.0032088
Yang, H., Auerswald, K., Bai, Y., and Han, X. (2011). Complementarity in water sources among dominant species in typical steppe ecosystems of inner mongolia. China Plant Soil 340, 141–155. doi: 10.1007/s11104-010-0307-4
Yang, W. B., Tang, J. N., Liang, H. R., Dang, H. Z., and Li, W. (2014). Deep soil water infiltration and its dynamic variation in the shifting sandy land of typical deserts in China. Sci. China Earth Sci. 57, 1816–1824. doi: 10.1007/s11430-014-4882-8
Zhang, C., Li, X., Wu, H., and Wang, P. (2017). Differences in water-use strategies along an aridity gradient between two coexisting desert shrubs (Reaumuria soongorica and Nitraria sphaerocarpa): isotopic approaches with physiological evidence. Plant Soil 419, 169–187. doi: 10.1007/s11104-017-3332-8
Zheng, X. R., Zhao, G. Q., Li, X. Y., Li, L., Wu, H. W., Zhang, S. Y., et al. (2015). Application of stable hydrogen isotope in study of water sources for Caragana microphylla bushland in Nei Mongol. Chin. J. Plant Ecol. 39, 184–196. doi: 10.17521/cjpe.2015.0018
Zhou, H., Zhao, W., Zheng, X., and Li, S. (2015). Root distribution of Nitraria sibirica with seasonally varying water sources in a desert habitat. J. Plant Res. 128, 613–622. doi: 10.1007/s10265-015-0728-5
Zhou, Y., Boutton, T. W., Wu, X. B., Wright, C. L., and Dion, A. L. (2018). Rooting strategies in a subtropical savanna: a landscape-scale three-dimensional assessment. Oecologia 186, 1127–1135. doi: 10.1007/s00442-018-4083-9
Zhu, Y. (2016). “Water use strategy of four desert shrubs in Gonghe Basin, Qinghai-Tibetan Plateau,” in Water Stress in Plants, eds I. M. Rahman, Z. A. Begum, and H. Hasegawa (Croatia: InTech), 81–98. doi: 10.5772/63195
Keywords: desertification restoration, δD, δ18O, sand-fixing vegetation, water source
Citation: Zhu Y and Wang G (2020) Rainwater Use Process of Caragana intermedia in Semi-Arid Zone, Tibetan Plateau. Front. Earth Sci. 8:231. doi: 10.3389/feart.2020.00231
Received: 09 January 2020; Accepted: 29 May 2020;
Published: 31 July 2020.
Edited by:
Xian Xue, Northwest Institute of Eco-Environment and Resources (CAS), ChinaReviewed by:
Jian Sun, Chinese Academy of Sciences, ChinaXueyong Zhao, Northwest Institute of Eco-Environment and Resources (CAS), China
Copyright © 2020 Zhu and Wang. This is an open-access article distributed under the terms of the Creative Commons Attribution License (CC BY). The use, distribution or reproduction in other forums is permitted, provided the original author(s) and the copyright owner(s) are credited and that the original publication in this journal is cited, in accordance with accepted academic practice. No use, distribution or reproduction is permitted which does not comply with these terms.
*Correspondence: Yajuan Zhu, zhuyj@caf.ac.cn; zhuyajuan8005@sohu.com