- 1Department of Research and Development, Danish Meteorological Institute, Copenhagen, Denmark
- 2Leibniz Institute for Baltic Sea Research Warnemünde, Rostock, Germany
- 3Swedish Meteorological and Hydrological Institute, Norrköping, Sweden
- 4Institute of Oceanology Polish Academy of Sciences (IOPAN), Sopot, Poland
- 5Department of Marine Systems, Tallinn University of Technology, Tallinn, Estonia
- 6Marine Sciences, Federal Maritime and Hydrographic Agency, Hamburg, Germany
- 7Marine Research Unit, Finnish Meteorological Institute, Helsinki, Finland
Two important communities related to oceanography in the Baltic Sea are those working on operational oceanography and Earth system science, with focusing on the same water body but different temporal scales. They have been coordinated through two organizations/programs: the Baltic Sea Operational Oceanographic System (BOOS) and the Baltic Sea Experiment (BALTEX) and its successor, the Baltic Earth Program (Earth system science for the Baltic Sea region), respectively. Although the two communities have archived significant progresses in their own fields since early 1990s, there were few interactions between the communities. Rapid advancements of operational oceanography on ocean monitoring, data sharing, modeling, and historical ocean state reconstruction in the last decade have provided a wide range of data, products and modeling tools which may be used in Earth system and climate change research. This is especially true when operational oceanography in the Baltic Sea is in a transition to a seamless service, i.e., from basin to local scales, from synoptic to climate scales and from physical to biogeochemical and biological systems. On the other hand, the Baltic Sea Earth system research can help to improve operational oceanography by contributing research observations and transferring their research achievements to the operational system. Based on a review of state-of-the-art of BOOS monitoring and modeling capabilities and on-going BOOS research, this paper will highlight topics and areas which are related to the Baltic Earth Grand Challenges, i.e., salinity dynamics, land-sea biogeochemical linkages, natural hazards and extreme events, sea level dynamics, coastal morphology and erosion, regional variability of water and energy exchanges, and multi-drivers of regional Earth system changes. Potential win-win cooperation and interaction between the BOOS and the Baltic Earth communities are also proposed and discussed.
Introduction
Oceanographic monitoring and research has a long history in the Baltic Sea. The earliest sea level observations started from 1770s (Ekman, 2009). After early German and Swedish expeditions in 1871 (“Pommerania” from Kiel) and 1877 (Swedish expedition by G. Ekman and O. Petterson), the Baltic Sea countries Denmark, Finland, Germany, Russia and Sweden signed in 1892 a resolution on international cooperation in Baltic Sea monitoring and in 1898 an agreement on simultaneous investigations on a regular basis at a few selected deep stations in the Baltic Sea. With the start of the International Council of the Exploration of the Sea (ICES) in 1902 a systematic monitoring of the Baltic Sea was established. In the past two decades, community coordination of the Baltic Sea oceanography has been organized mainly in three areas: marine environment monitoring and protection—coordinated by The Baltic Marine Environment Protection Commission (HELCOM) since 1992, operational oceanography—coordinated by the Baltic Sea Operational Oceanographic System (BOOS) since 1998 and oceanography research related to climate variability and Baltic Sea system science—coordinated by the Baltic Earth program (Earth system science for the Baltic Sea region), the successor program of the Baltic Sea Experiment (BALTEX) since 1993. In addition, fishery monitoring and data management has been coordinated by ICES and DG MARE in the Baltic-North Sea and European scale, respectively. Due to their different mandates, HELCOM members are mainly conducting offline, physical-biogeochemical and biological monitoring for environment assessment, protection, and ecosystem-based management (HELCOM, 2013); BOOS members are mainly responsible for online, physical-biogeochemical monitoring for operational oceanographic services, e.g., forecast, nowcast and hindcast (Buch and Dahlin, 2000; Buch et al., 2006); ICES members mainly carry out offline monitoring ranging from hydrographic to biological parameters for fishery management; ICOS (Integrated Carbon Observation System) is a European research infrastructure to quantify and understand the greenhouse gas balance of the European continent and of adjacent regions and is built up as a collaboration of nationally operated measurement stations in 12 European countries. Most of the monitoring activities in the above categories are regular.
BALTEX/Baltic Earth has a much wider focus. BALTEX was founded in 1993 as a network of operational weather services, climate centers, and universities with the aim to exchange both operational and climate data of the atmosphere, ocean, and land surface and to analyze water, energy and matter cycles in the Baltic Sea region (Raschke et al., 2001; Omstedt et al., 2004, 2014; Reckermann et al., 2011). BALTEX/Baltic Earth is a Regional Hydroclimate Project (RHP) within the Global Energy and Water Cycle Exchanges Project (GEWEX) of the World Climate Research Programme (WRCP). Baltic Earth research aims to fill knowledge gaps of the entire regional Earth system (Meier et al., 2014). However, in this review we limit the discussion mainly to the oceanographic component of Baltic Earth following Omstedt et al. (2004, 2014).
Since their establishments, both BALTEX/Baltic Earth and BOOS have reached significant achievements in their own fields. For operational oceanography, advanced monitoring, and forecasting capacities have been developed at local, sub-basin, and sea basin scales. Observations and forecasts are shared in real or near real time. With sustained observing, the ocean state is identified and new phenomena and related knowledge are discovered and transferred to the operational hindcast and forecast modeling platforms for optimizing existing models; by assimilating observations into the operational models, the capacity on reconstructing historical and forecasting future ocean states is improved. The products generated have been used in marine service for blue economy, ocean health, and climate change adaptation and mitigation (She, 2018a). Currently the BOOS monitoring network provides a significant amount of real time observations from e.g., tide gauge stations, FerryBox lines, mooring buoys, fixed stations, Argo profilers and research vessels (She, 2018b; Siiriä et al., 2018). The quality of operational ocean-ice-wave-biogeochemical models has been significantly improved. Based on the models developed, operational forecasting service has been made both in national and regional levels (She and Murawski, 2018; Tuomi et al., 2018). Data assimilation has been developed to ingrate modeling and observations to derive a better initial field for the forecasting models and reanalysis for reconstructing the history of the Baltic Sea (Zhuang et al., 2011; Fu et al., 2012; Axell, 2013; Liu et al., 2017). Major sources for the development of the operational oceanography in the Baltic Sea have been the Member States. In the past decades, programs from European commission, e.g., Operational Oceanography Cluster in the Framework Programs 5 and 6 (Cieślikiewicz et al., 2007), MyOcean (Bahurel et al., 2010) and following Copernicus Marine Environment Monitoring Service (CMEMS) Program have strongly supported the integration of Baltic Sea operational oceanography in advancing the operational service at the sea basin scale.
Baltic Earth is an independent and open research network with the following vision: “Baltic Earth strives to achieve an improved Earth System understanding of the Baltic Sea region as the basis for science-based management in the face of climatic, environmental, and human impact in the region. Baltic Earth brings together a broad international research community around core scientific issues identified as fundamental to informing societal efforts to achieve sustainability in the region. These “Grand Challenges (GC)” are tackled through joint research efforts, workshops, conferences, and capacity building events accompanied by a continuous process of synthesis of the current state of knowledge. Communication with stakeholders and research funders aims to ensure impact and relevance of the research. Baltic Earth targets the atmosphere, land, and marine environment of the Baltic Sea, its drainage basin and nearby areas with relevance for the Baltic Sea region.” (Baltic Earth Science Plan Writing Team, 2017). Following this vision, Baltic Earth fosters interdisciplinary and international collaboration on processes in the atmosphere, on land and in the sea and also in the anthroposphere by organizing conferences, workshops, seminars, dedicated sessions at conferences, etc. Dedicated working groups (WGs) identify at Baltic Earth conferences and by using assessments of existing research new GCs. Currently, the Baltic Earth community has identified six GCs for the Baltic Earth system research: (GC1) salinity dynamics, (GC2) land-sea biogeochemical linkages, (GC3) natural hazards and extreme events, (GC4) sea level dynamics, coastal morphology and erosion, (GC5) regional variability of water and energy exchanges, and (GC6) multi-drivers of regional Earth system changes. For each of the GCs, WGs were installed. In addition, WGs on outreach and communication, education, and regional climate system modeling are active. A new WG on climate and environmental ocean observing systems such as the Boknis Eck Time-Series Station is in the planning.
Another important aspect of Baltic Earth are thematic assessments that provide an overview over knowledge gaps which need to be filled, e.g., by funded projects. Two assessments of climate change of the Baltic Sea region, which are research community efforts such as the regular assessments of the Intergovernmental Panel on Climate Change (IPCC) of past, present and future climate, have been performed, and a third one is on the way (BACC Author Team, 2008; BACC II Author Team, 2015). Further assessments focussed on Baltic Sea models (Eilola et al., 2011; Placke et al., 2018) and ensembles of scenario simulations with coupled physical-biogeochemical models (Meier et al., 2018a, 2019), both in past and future climates (Meier et al., 2012). Recently, a more comprehensive Baltic Sea Model Inter-comparison Project (BMIP) including also process-based assessments has started.
For the closure of knowledge gaps identified by Baltic Earth assessments, several projects funded by national and European Union (EU) programs have been carried out under the umbrella of Baltic Earth. Selected examples are the BONUS projects AMBER, BALTIC-C, ECOSUPPORT, INTEGRAL, BalticAPP, and SHEBA (see http://baltic.earth). Baltic Earth is coordinated by the International Baltic Earth Secretariat at Helmholtz Zentrum Geesthacht, the Baltic Earth Science Steering Group and the Baltic Earth Advisory Board.
However, the BOOS and BALTEX/Baltic Earth communities had few interactions in the past two decades. Now it is time to enhance cooperation and integration between operational oceanography and Earth system science communities. This is in line with the recent trend of development in several international initiatives such as seamless prediction of the Earth system from the World Meteorological Organization (WMO, 2015) where observing and modeling development at the synoptic scale will be integrated with the climate scale. In the Global Ocean Observing System (GOOS), ocean observing has been extended from mainly for operational service to cover also climate change and ocean health. It is expected that GOOS Regional Alliances (GRAs, e.g., EuroGOOS) and Regional Ocean Observing Systems (ROOSs) will follow this vision to further integrate the ocean observing in operational oceanography, climate change and ocean health fields, as indicated by recent development of a sustained European Ocean Observing System (EOOS, http://www.eoos-ocean.eu). For the assessment and services in climate change adaptation and mitigation, long-term change of extreme events are more and more emphasized which needs calibrated high quality models to perform trustworthy simulations of climate projections.
In addition, the European Commission has asked for “responsive research and innovation” in its research policy in the FPs (Rodriguez et al., 2013; von Schomberg, 2013). Integration and interactions between the operational oceanography community and research community will certainly enhance the responsiveness of our research.
The purpose of this paper is to introduce the state-of-the-art of operational oceanography in the Baltic Sea, set up the scene and identify potential areas of collaboration between the operational oceanography community and the Baltic Earth community. The paper is organized as follows: section Operational oceanography in the Baltic Sea reviews the state-of-the-art of operational oceanography in the Baltic Sea, including operational observing and modeling. Section BALTEX/Baltic Earth marine research reviews the state-of-the-art of Baltic Earth system science while section Operational oceanography and Baltic Earth research—interactions identifies potential collaboration areas between the two communities. Section Discussions and recommendations gives recommendations for future BOOS—Baltic Earth cooperation. Acronyms used in this study are explained in Table 1.
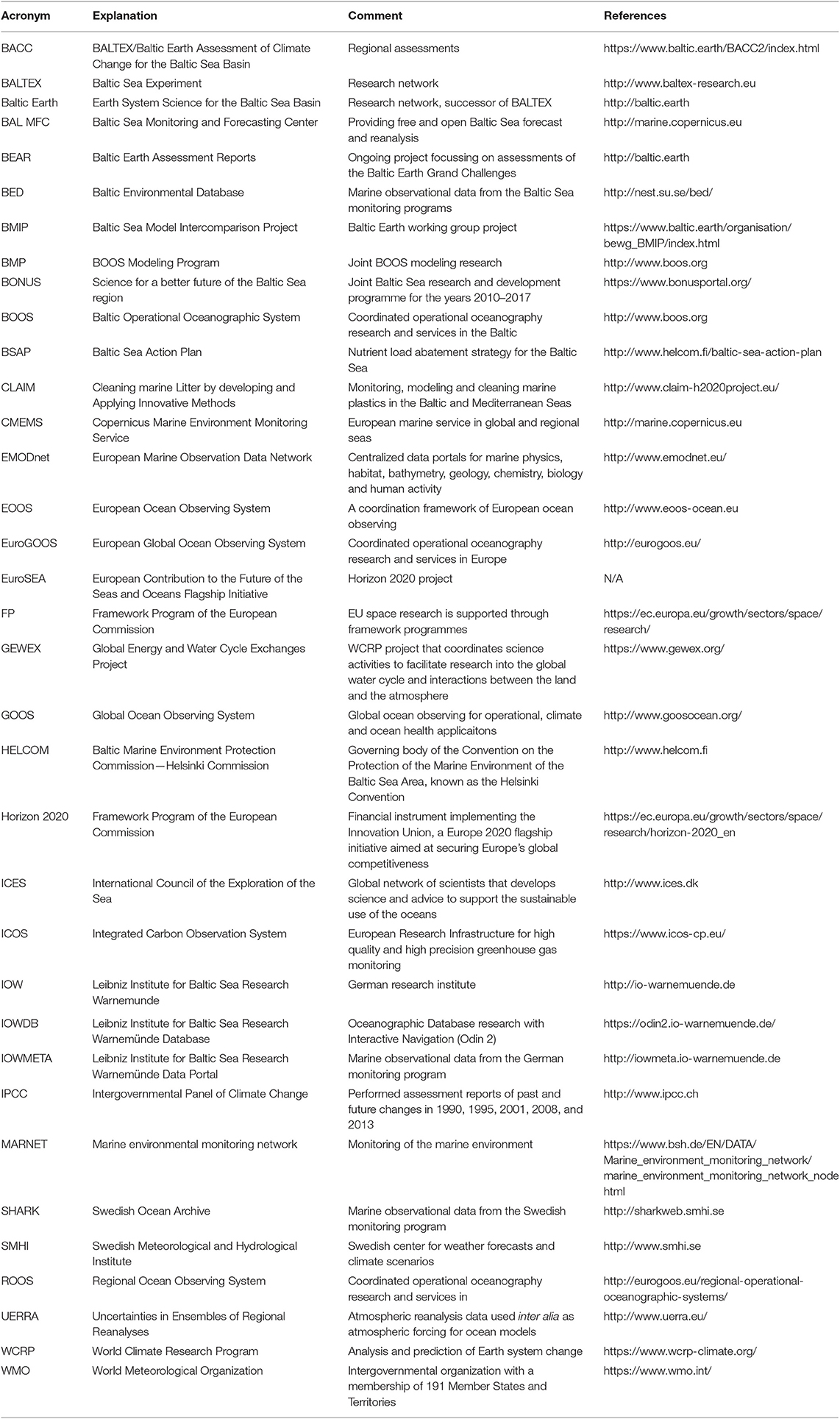
Table 1. List of frequently used acronyms, their explanation, and references (in alphabetical order, assessed on 2019-01-13).
Operational Oceanography in The Baltic Sea
Operational Observing—Current Status and Major Challenges
Ocean observing value chain includes three components: observing, data management, and data usage. The observing aims at generating cost-effective and fit-for-purpose observations; the data management is responsible for providing user friendly data access while the data usage component will transfer data into information products for user applications, in many cases through integrating satellite and in-situ observations with models. The Baltic Sea has been monitored with comprehensive, self-coordinated monitoring programs: operational monitoring is coordinated by BOOS, environmental monitoring by HELCOM and fishery monitoring by ICES. The research monitoring activities are not coordinated but the regional and EU research programs (e.g., BONUS and Horizon 2020) have their own data policies.
Operational Monitoring
The operational observing system in the Baltic Sea provides real time (RT) and near real time (NRT) observations by BOOS members to fit for the purpose of model validation and data assimilation for the improvement of the operational forecasting and reanalysis. The observations include sea level, temperature/salinity (T/S), currents, waves, dissolved oxygen (DO), and chlorophyll a, etc. The station locations and types of platforms are illustrated in Figure 1. High resolution data are generated by tide gauges (sea level, in 1–60 min sampling interval), FerryBox (spatial continuous sampling of surface temperature, salinity, DO and fluorescence etc. in 1–7 day sampling interval), moorings (hourly sampling of met-ocean variables, T/S, currents, turbidity, DO and chlorophyll fluorescence) and shallow water Argo profilers (T/S, DO and chlorophyll fluorescence in 1–7 day sampling interval). Low frequency (mostly monthly or less frequent) observations are made by research vessels. Most of the operational observations cover the coastal water, only a few moorings, Argo profiler and ship stations cover the open Baltic Sea. In recent years, the shallow water Argo profiler has been demonstrated as an efficient tool for monitoring hydrographic conditions in operational mode (Haavisto et al., 2018). There is also a potential to derive the currents from the shallow water Argo profilers (Roiha et al., 2018).
A recent survey on the observational infrastructure to BOOS members showed that the member institutes (not including Russian) own or have access to 211 tide gauges, 7 shallow water Argo floats, 29 buoys, 22 Research Vessels (R/Vs), 6 Remotely Operated Vehicles (ROVs, e.g., gliders), 22 Acoustic Doppler Current Profilers (ADCPs), 25 other fixed stations and 23 FerryBoxes. Not all of them are used for operational observing. Most of the R/Vs are used for research and/or regular basin wide environmental monitoring, coordinated by HELCOM.
Data Management
BOOS partners share their observations through a ftp-network. This forms a basis for Baltic Sea in-situ Thematic Assembling Center (BAL INS-TAC) in CMEMS and EMODnet (European Marine Observation Data Network) Physics, which provide open and free operational data access to users.
Major Challenges
The final goal for Baltic Sea observing is to build up a sustainable, integrated, and cost-effective observing system, which can fit for multi-purpose for operational, climate, commercial, and ecological applications. BOOS observing will be an important subsystem. Major gaps in the existing BOOS observational network for operational oceanography are lack of current measurements and profile observations (especially for biogeochemical variables) in the open Baltic Sea (She, 2018b; Le Traon et al., 2019). Key questions have to be answered: how observations from other sectors can be used to fill the gaps; if new observations are needed, which sampling schemes and technologies should be applied and how to be combined to generate a cost-effective gap-filling solution.
Operational Modeling—Current Status and Major Challenges
The operational modeling activities in the Baltic Sea is coordinated by BOOS Modeling Program (BMP) and CMEMS BAL MFC (Baltic Sea Monitoring and Forecasting Center), including joint research on operational model system development, data assimilation, model calibration and validation (cal/val), multi-model ensemble forecast, products generation, and services.
Model Development
Operational modeling has a long history in the Baltic Sea. The ocean wave forecast model (WAM) was developed in Europe in the 1980s (WAMDI, 1988). Operational wave forecasts were implemented in the late 1990s in Denmark and Finland (She and Nielsen, 1999; Tuomi et al., 1999) based on the WAM model. Ocean, ice and oil drift forecast models BSH-Cmod, BSH-Dmod, HIROMB, and HELMI had been developed and operationalized in the early and mid-1990s (Haapala and Leppäranta, 1996; Dick et al., 2001; Wilhelmsson, 2002; Funkquist and Kleine, 2007). They are currently updated by more advanced coupled ocean-ice forecasting systems HBM (HIROMB-BOOS Model, Berg and Poulsen, 2012), NEMO-Nordic (Hordoir et al., 2018), and GETM (General Estuarine Transport Model, Burchard and Bolding, 2002; Büchmann and Söderkvist, 2016). HBM is a dynamically two-way nested model with excellent hybrid parallel computing performance (Poulsen et al., 2014). NEMO is the European operational model with the largest user community. GETM has advantages in resolving the coastal-estuary continuum with specific advances in turbulence closure schemes and reduced diapycnal mixing due to the usage of vertically adaptive coordinates (Burchard et al., 2009). Biogeochemical models such as ERGOM (Neumann, 2000; Maar et al., 2011) and SCOBI (Swedish Coastal and Ocean BIogeochemical model, Eilola et al., 2009) have been developed in this century for setting up operational ecological service. The former has been used to provide basin-scale biogeochemical forecasts for CMEMS since 2009 (Tuomi et al., 2018) while the latter was used for producing biogeochemical reanalysis (Liu et al., 2017). The above operational model systems have been applied for basin-scale forecasts in 0.5–1 nautical mile (nm) resolution and a up to 60 m resolution for local scale forecasts (She and Murawski, 2018). Coupled model development, especially wave related coupling processes, e.g., ocean-wave, atmosphere-wave, and wave-ice interaction, is an on-going activity by BOOS partners. In the Phase II of CMEMS BAL MFC (2018–2021), a fully coupled ocean-wave-ice-biogeochemical model system NEMO-LIM-WAM-ERGOM together with PDAF (Parallel Data Assimilation Framework) assimilation is under development.
Data Assimilation
Significant data assimilation capacity has also been developed in the operational community, ranging from a simplified Kalman Filter for sea surface temperature (SST) assimilation in BSH-Cmod (Larsen et al., 2007), pre-operational 3DVAR (3D Variational method), and EnOI (Ensemble OI) for T/S assimilation and reanalysis production in HBM (Zhuang et al., 2011; Fu et al., 2012) to the ensemble variational method and PDAF simplified Karman filter for physical-biogeochemical reanalysis production in RCO-SCOBI and NEMO-SCOBI (Axell and Liu, 2016; Liu et al., 2017). Currently the Baltic Sea data assimilation collaboration in CMEMS focuses on developing physical and biogeochemical assimilation systems by using the PDAF both for operational NRT forecast and also for reanalyses. Assimilation schemes for SST, T/S, sea ice and nutrients are relatively mature. New schemes are developed for assimilating sea level and satellite ocean-color data (Tuomi et al., 2018).
Model Quality and Validation
Before 2009, the operational model validation and quality assessment were mainly done at national level with different methods and quality standards. Common cal/val methodology had been developed and applied in the MyOcean projects (2009–2015), including cal/val metrics definition and error statistics calculation and presentation for new model version, NRT validation and reanalysis quality assessment. The cal/val has been part of the BAL MFC operational activities since 2015. Before release of each new BAL MFC model version—both forecasting and reanalysis systems, a correspondent QUID (Quality Information Document) report has to be released to demonstrate the quality of the new version products (e.g., Golbeck et al., 2017). The NRT validation for the BAL MFC forecast products (ocean-ice-wave-biogeochemical parameters) is shown online at the BOOS website. The cal/val method and toolbox developed in the BAL MFC is now further extended to a BOOS model quality and validation cooperation.
Multi-Model Ensemble (MME) Forecasting
Based on NRT exchange of both model and observational data via the BOOS ftp network, a MME forecast system has been developed for sea level, SST, sea surface salinity (SSS), T/S and currents (Golbeck et al., 2015). By weighting the individual forecast related to its forecasting error, a weighted MME method is used to generate the MME forecast. The results, shown online at the BOOS website, demonstrate superior quality of the MME forecast to the individual ones. The MME is a joint achievement of ROOSs and MFCs in the Baltic and North Sea. Currently the BOOS MME Working Group aims at extending the current MME system for national forecasting use.
Major Challenges
Future direction of the operational modeling in the Baltic Sea is seamless modeling in spatial, temporal, and state variable dimensions (WMO, 2015; She and Murawski, 2018). In spatial scales, the modeling capacity will be extended from basin scale to local coastal-estuary scale and from mesoscale to sub-mesoscale. In temporal scales, the synoptic and climate scales will be resolved by the same operational modeling framework. For state variables, future operational modeling capacity (including forecast, reanalysis, and scenario-based projections) will be extended to cover sedimentation, movements of pollutants (either as particles or Eulerian tracers) and biological parameters. In this dimension, operational ecological modeling will be developed, different modeling sectors will be coupled, e.g., hydrological-ocean coupling, wave-ocean and wave-ice coupling, and ocean-optical coupling.
There still exist many knowledge gaps toward the establishment of the seamless operational service. Monitoring and accurate modeling of water and biogeochemical mass transport caused by coastal-estuary interaction, inter-sub-basin exchange and meso- and submeso-scale eddies is still a challenge. Capacities for precisely predicting currents, upwelling, extreme sea level and waves in icing waters, skin temperature, algae bloom, and oxygen depletion are yet to be improved.
Baltex/Baltic Earth Marine Research
In the following, a few selected research highlights from BALTEX/Baltic Earth are presented, documenting the progress in physical oceanography of the Baltic Sea during 2003–2014 (Omstedt et al., 2004, 2014; see also BACC Author Team, 2008; BACC II Author Team, 2015) and after. One of the main motivations for the foundation of BALTEX in the 1990s was the exchange of data between eastern and western Baltic Sea countries. Due to the Iron Curtain after World War II, the exchange of scientific information was limited. Hence, after the rise of the Iron Curtain in the 1990s BALTEX aimed to enhance international collaboration between the Baltic Sea countries and to increase the exchange of especially observational data.
Process Understanding
With the help of project-oriented research data and process modeling, our knowledge about oceanographic processes and the interactions of the ocean with the other components of the Earth system such as atmosphere, land surface and sediments has considerably increased since the start of BALTEX (Omstedt et al., 2004, 2014). For instance, the importance of surface waves in air–sea interaction of heat, momentum, and matter is better understood, and Stokes drift and Langmuir circulation have been identified as likely playing an important role in surface water mixing explaining the underestimation of mixed layer depth in many Baltic Sea models (Reissmann et al., 2009).
Research on water exchange between the Baltic Sea and North Sea and saltwater inflows into the Baltic has a long tradition. Today we know that, on average, half of the total amount of salt imported into the Baltic is supplied by barotropic inflows of highly saline water (Mohrholz, 2018). In particular, the well-observed, exceptionally strong Major Baltic Inflow (MBI) of 2014 (Mohrholz et al., 2015) enabled unprecedented, detailed studies of the dynamics of saltwater inflow events and of their implications for the ecosystem (e.g., Gräwe et al., 2015; Schmale et al., 2016; Holtermann et al., 2017; Bergen et al., 2018). In a long-term average MBIs contribute 20 to 25% to the total salt import into the Baltic (Mohrholz, 2018), beside this they are the solely mechanism for deep water ventilation of the central Baltic (Meier et al., 2006). Despite the decrease of nutrient supply after the 1980s, recently observed oxygen consumption rates are higher than ever observed (Meier et al., 2018b). According to model results, oxygen consumption in the water column has increased relatively more than oxygen consumption in the sediment. Subsequently, natural ventilation has become less effective representing a positive feedback for hypoxia (Meier et al., 2018b).
Observations from field campaigns from the northern Baltic Sea suggested that the flow regimes are intermittent and that hydraulic control occurs in only about 55% of the cases, i.e., less frequently than anticipated (Green et al., 2006). Further, in wider gravitational flows, transverse Ekman circulation was identified to be an important process for the generation of mixing (Umlauf and Arneborg, 2009a,b).
Recently, there has been increased research into the Baltic Sea coastal zone, particularly into upwelling, nutrient retention and the coastal filter capacity of nutrients (e.g., Edman et al., 2018). Estimates suggest that the coastal filter of the entire Baltic Sea removes 16% of nitrogen and 53% of phosphorus inputs from land (Asmala et al., 2017). Simulated long-term nutrient retention was found to be associated with the physical characteristics of a water body, such as the surface area, depth and residence time of the water.
Progress was also made in understanding the large-scale circulation, water mass transformations, and mixing processes in the Baltic Sea using high-resolution ocean circulation models that were running for many decades together with Eulerian concentration and age tracers (e.g., Meier, 2005, 2007). The model results illustrate possible pathways and ages of either inflowing saline water from the North Sea or freshwater originating from the various rivers. Freshwater is found to be subject to an efficient recirculation in the Baltic (e.g., Rodhe and Winsor, 2002). These simulations are complementary to an interesting tracer release experiment in the deep water of the central Gotland Basin showing a considerable increase in vertical mixing rates after the tracer reached the lateral boundaries of the basin (Holtermann and Umlauf, 2012; Holtermann et al., 2012). Hence, boundary mixing is perhaps the key process of basin-scale vertical mixing. For further details, the reader is referred to the review article by Omstedt et al. (2014) and the original literature cited therein.
Climate and Environmental Observations and Reanalyses
Nowadays, meteorological databases (both station data and high-resolution gridded datasets) are freely available with high quality to force ocean models on decadal and even centennial time scales. For instance, the regional reanalysis project Uncertainties in Ensembles of Regional ReAnalyses (UERRA, http://www.uerra.eu) delivers homogenous atmospheric surface fields for the period 1961 until today. In addition, oceanographic data became more easily accessible and new important measurement platforms, such as the MARNET stations (https://www.io-warnemuende.de/marnet-en.html), long-term moorings, e.g., in the Gotland Deep region, FerryBoxes, and satellites, have provided temporally and spatially better resolved observations. River runoff data are crucial for the understanding of the Baltic Sea dynamics and new catchment-wide high-resolution datasets based on process-based hydrological modeling calibrated to available station data are now available. However, a homogeneous hydrological dataset that covers the entire period from the 1960s to the present day comparable to atmospheric reanalysis data is still missing. Further, available nutrient load and other environmental data are nowadays collected and stored in publicly available databases.
A big step forward to understand climate variability in the Baltic Sea region was the development of historical reconstructions of atmospheric, hydrological and oceanic datasets since around 1850. With the help of Baltic Sea models, the impact of increasing nutrient loads and climate change on the marine ecosystem was detected and attributed to the various drivers of the system. We have now a better understanding of the natural variability in the Baltic Sea region and how large-scale atmospheric circulation affects the Baltic Sea climate variability (e.g., Börgel et al., 2018). During recent decades, changes in large-scale atmospheric circulation have caused a north-eastward shift in low-pressure tracks consistent with a more zonal circulation over the Baltic Sea basin (e.g., Trenberth et al., 2007). The decadal and multi-decadal regional variability of the past climate is partly explained by the North Atlantic Oscillation (NAO, mainly during winter) and the Atlantic Multi-decadal Oscillation (AMO). Despite the pronounced internal variability, trends were detected that could probably be attributed to anthropogenic climate change on centennial time scale (e.g., Kniebusch et al., 2019a,b). A highlight was the revision of the empirically derived barotropic saltwater inflow statistics for 1887 until present that shows no statistically significant trend but the same multi-decadal variability as in precipitation data (Mohrholz, 2018). Further, based upon model results it was concluded that stagnation periods such as the one between 1983 and 1992 are part of the natural variability of the system and occur once per 100 years on average (Schimanke and Meier, 2016).
While atmospheric reanalysis data have long been used to force ocean models, long-term reanalyses for the ocean on multi-decadal time scales became only recently available including both physical and biogeochemical variables (e.g., Liu et al., 2017). Ocean reanalysis data play an important role for the development and evaluation of ocean models (Placke et al., 2018).
Climate and Environmental Modeling
Within BALTEX, the first coupled atmosphere-ice-ocean regional models were developed about 20 years ago (Gustafsson et al., 1998; Hagedorn et al., 2000; Döscher et al., 2002; Schrum et al., 2003). Nowadays several coupled models for the Baltic Sea—North Sea system are under development (e.g., Gröger et al., 2013; Tian et al., 2013, 2016; Van Pham et al., 2014; Wang et al., 2015; Ho-Hagemann et al., 2017). Regional climate models contributed to a better quantitative understanding of the energy and water cycles of the Baltic Sea basin. However, especially processes important for the regional water cycle are still not well-understood causing, inter alia, precipitation and runoff biases over the catchment area in long-term atmosphere climate simulations with considerable impact on the quality of ocean climate simulations (Meier et al., 2019).
These models will be the future tools to investigate the dynamics of regional Earth systems. Within Baltic Earth a dedicated WG on regional climate system models (RCSMs) is planning and performing coordinated experiments of an ensemble of RCSMs with the aim to improve coupled models, to exchange expertise and to investigate the added value of RCSMs. Based upon the dynamical downscaling approach using RCSMs with lateral boundary data from global climate models, paleo-climate simulations of the past 1,000 years (e.g., Schimanke et al., 2012) and projections of the twenty-first century were performed (e.g., Meier et al., 2018a; Dieterich et al., 2019; Gröger et al., 2019). As the sizes of the ensembles were relatively large, they allowed to estimate uncertainty ranges and to identify the sources of uncertainties. For further details, the reader is referred to the review article by Meier et al. (2019) and the original literature cited therein.
Ongoing Activities
Following the original idea of identifying knowledge gaps (Baltic Earth Science Plan Writing Team, 2017), currently a series of extensive Baltic Earth Assessment Reports (BEAR) is in preparation. For each of the GCs, a team of experts from the Baltic Earth network has started to collect information from scientific publications to summarize the current state of knowledge in the respective research fields and to identify knowledge gaps. In addition, one of these assessments will be the BACC III report, an update of the knowledge recently gained after the publication of the comprehensive BACC I and II reports (BACC Author Team, 2008; BACC II Author Team, 2015). As for the previous two BACC reports, a close collaboration between Baltic Earth and HELCOM is envisaged. For the update of the Baltic Sea Action Plan (BSAP), climate change will be considered. Moreover, Baltic Earth scientists participate in the HELCOM—Baltic Earth Expert Network on Climate Change (EN CLIME) that will produce a Climate Change Fact Sheet for policy makers and the public based upon BACC results. Assessments of our knowledge on the regional Earth system (including aspects of processes, climate, and environment) are an integral part of Baltic Earth.
Operational Oceanography and Baltic Earth Research—Interactions
Use of Operational Observing for Baltic Earth Research
Due to the operational feature of the observation production, quality control and open and free dissemination, the operational data are useful to all kinds of users, ranging from research, ecosystem-based management, climate change adaptation, and mitigation to blue economy information service. For the Baltic Earth research, the BOOS observations are especially valuable due to three reasons: (i) long history: operational monitoring of temperature, salinity, sea level, currents and ice started 100 years ago; (ii) the high resolution observations provide rich information on hydrographical and biogeochemical processes, and (iii) NRT delivery of data ensures timely access. The historical operational observations are the major data source of the climate data archive in the sea. However, a significant part of them has not been digitized, e.g., Finnish ice charts (since 1915) and Danish sea level, T/S, currents and ice measurements before 1930. High-resolution SST and sea ice products in the past 100 years are essential in the reconstruction of accurate atmosphere-ocean states. The high-frequency sea level observations can be used for studying important ocean processes in scales of hours to a few weeks, e.g., storm surges, coastal waves, basin-scale sea level dynamics (GCs 1, 3, and 4). FerryBox and shallow water Argo floats data can be used for investigating processes of sub-mesoscale and mesoscale eddies, river plumes and coastal-estuary interaction, inter-subbasin water exchange, upwelling, ocean heat content anomaly, algae bloom and oxygen depletion etc. Mooring observations, with hourly measurements and more parameters, in addition to the above usages, are also suitable for studying the diurnal variation of SST, chl-a and trophic layer optical features.
Considering the free and 24/7 (all time) availability of the BOOS data, any research field campaign should use them as background observations, for the design of the campaign sampling schemes whenever necessary. The research observing program can also consider joint observing activities by mobilizing the observing infrastructure from the BOOS members.
Use of Operational Modeling for Baltic Earth Research
The operational modeling platforms, products (both short- and long-term) and data assimilation and cal/val tools, as described in section BALTEX/Baltic Earth marine research, can be used for the Baltic Earth research, for example,
1. Reconstruction of past hydrodynamic and biogeochemical state: decadal ocean-ice-wave-biogeochemical reanalysis and reprocessed satellite and in-situ observation products from CMEMS provide ready to use data for the GCs 1, 3, 4, and 5.
2. The operational cal/val toolbox developed in the BAL MFC can be applied and further developed for evaluating climate models
3. Downscaled operational models are capable of predicting small-scale variability in up to tens-of-meter resolution. Some of them are computationally so efficient that they can be applied in climate simulations with very high resolution (GCs 2, 5, and 6)
4. The short-term operational products, e.g., forecast, interim reanalysis, can be used for studying the natural hazards and extreme events (GC3)
5. End-to-end modeling: the solid operational products can provide robust inputs (ocean-ice-wave-biogeochemical variables) to end-to-end modeling (GC6)
Potential Contribution From Baltic Earth Community to Operational Oceanography
Operational Observing
Research observations from the Baltic Earth community, if they can be adapted to meet operational requirements, will be very useful for filling the gaps of the BOOS observational network. ICES database collects ship observations from the HELCOM monitoring program, fishery monitoring and some research projects, which has a much better coverage then the BOOS network in the open Baltic Sea. Most of the research observations are not in real or near real time. The research observing program can be made to fit for the operational application through open data policy and NRT data delivery, which will fill the observational data gaps. For example, research data from classical observations like CTD can be made available in NRT before the final processing for (climate) research. Vice versa, good quality operational observations can be used for (climate) research after appropriate quality control and exposing it to appropriate processing in delayed mode.
Operational Modeling
The research on the six GCs by the Baltic Earth community is highly relevant to improve the operational modeling capacity. By revealing important factors controlling the salinity and sea level dynamics, results from the GCs 1, 4, and 5 can be used to improve the long-term performance of operational ocean models. The GC2 research can be used to improve the downscaled models for resolving coastal-estuary continuum. The GC3 may find new features and knowledge regarding to the extreme events, which are always challenge cases in the operational modeling. The GC6 and GC2 research may benefit emerging areas of operational modeling, e.g., operational ecological modeling, coupled ocean-hydrological modeling and sediment transport modeling etc.
In order to benefit the operational modeling, there should be a platform to transform the Baltic Earth research results into the operational models. This is similar to the “Service Evolution” element in the CMEMS where dedicated, short-term mini-R&D projects are funded to transfer the best practice in modeling and observing research into CMEMS system.
Discussions and Recommendations
The Baltic operational oceanography community and the BALTEX/Baltic Earth community have co-existed in the past two/three decades. However, only very preliminary interactions have been carried out because differing objectives of both communities hampered an intensive collaboration as outlined below.
Through analysis of the state-of-the-art of operational oceanography and regional Earth system research, it was found that the operational observations, modeling platforms, and products can significantly benefit the Baltic Earth research, e.g., in the areas of the six grand challenges, while the Baltic Earth research can also benefit operational oceanography. Most of the research databases such as the Baltic Environmental Database (BED, http://nest.su.se/bed) at Stockholm University, the Swedish Ocean Archive (SHARK, http://sharkweb.smhi.se) and all other environmental databases operated by the Swedish Meteorological and Hydrological Institute (SMHI) and the German Baltic Sea monitoring data archive (IOWDB, http://iowmeta.io-warnemuende.de and https://odin2.io-warnemuende.de/) operated by the Leibniz Institute for Baltic Sea Research Warnemünde (IOW) are already open access. In the future, a common database including both operational and research data may be established. In Europe, EMODnet has integrated marine observations, both online and offline data, in the entire parameter domain ranging from physical, biogeochemical, biological to human activities. In future, it may play a more important and active role for linking research observations and operational oceanography. However, for the research of, for instance, detecting ocean changes high accuracy of measurements is needed which is today not assured by all operational data products. The calibration of measurement devices is time consuming and expensive. Quality control is also the reason why not all research data of IOWDB and other databases can be provided in real or near real time.
Research observations can be an important complementary in emerging observations for developing operational ecology, predicting the fate of visible, and invisible marine plastics in the Baltic Sea, modeling and forecasting sediment transport, underwater noise etc. For these areas, operational observing capacity has not been established yet. They will need to heavily rely on data from research projects.
As mentioned above, reanalysis data sets are useful for climate analysis and for the evaluation of climate models used within Baltic Earth. However, current reanalysis data sets are usually not based on first principles, i.e., the conservation of mass, energy and momentum. Hence, many data assimilation schemes may cause problems, for instance, for budget and flux calculations of nutrients (Liu et al., 2017). This may lead to problems when using reanalysis for trend and long-term variability analyses. Hence, reanalysis products should be improved for the climate research purpose by using more mass and energy conserved assimilation method, e.g., 4D variational assimilation.
Research observing infrastructure should be made more usable for various applications. Examples of observing systems that fulfill such a criterion are the Australian IMOS (Integrated Marine Observing System, Hill et al., 2009) system, the German COSYNA (Coastal Observing System for the Northern and Arctic Seas, Baschek et al., 2017) and the IOW long-term monitoring program outside the German territorial waters. Although funded as a research infrastructure, the programs provide openly accessible observations, which can be used for many other purposes. These systems have been operated for more than 10 years.
The new knowledge made from the Baltic Earth research, both on the processes and model system development, can be transferred to the operational models, which will fill the knowledge and technological gaps in the operational modeling. The two communities should have regular joint meetings to identify topics with common interests and make the technology transfer from regional Earth system research to operational oceanography. For such a working group Baltic Earth would provide an ideal discussion platform. Unfortunately, state-of-the-art climate and operational ocean model versions usually differ although some institutes aim to have only one version for both applications. However, in reality two model versions are still needed because not all processes are well-enough understood such that their parameterizations fulfill the requirements of both applications on short and long time scales. An example is diapycnical mixing. Climate models require that long-term simulations do not artificially drift whereas data assimilation can always compensate shortcomings in operational mode. The development of operational oceanography in the last two decades, by integrating field monitoring, research (knowledge generation), operation and service, has demonstrated successfully the value chain of ocean research. In the Baltic Sea region, the future research is moving to an Earth system scale and be more responsive, the operational and adaptive level of the services for marine, climate, environment, and fishery are evolving. The value chain practiced by the operational oceanography is similar to other areas, e.g., Earth system research and environment protection. Seamless monitoring, modeling, and service provides a unified platform for integrating the research and services.
Next generation operational service system needs capacities on reconstructing, forecasting and projecting the states of marine ecosystems in basin and coastal waters. Significant new knowledge is needed for understanding and forecasting ocean processes such as inter-basin and sub-basin transport, sub-mesoscale eddies, coast-estuary interaction, sediment transport, algae bloom, oxygen depletion, and marine litter transportation. An idea solution is to carry out monitoring-modeling integrated and targeted research programs in the Baltic Sea scale (She et al., 2016). Joint force from BOOS and Baltic Earth community is a great advantage for responsive and collaborative research on the development of seamless service capacities, from synoptic to climate scales, from open sea to local waters, from physical to biological subsystems. During the procedure, scientific issues e.g., Baltic Earth GCs and interactions and coupling between different subsystems, will be addressed. Well-designed research programs should cover not only dedicated field experiment and new knowledge generation but also knowledge transfer into seamless operational model system and product service.
Naturally BOOS and Baltic Earth will consider to engage other important Baltic networks such as HELCOM, ICOS, and ICES in their cooperation as Baltic Earth and BOOS already collaborate with them. Within EN CLIME Baltic Earth and HELCOM are working together to produce a climate fact sheet and HELCOM and ICES representatives are members of the Baltic Earth Advisory Board. In the upcoming EuroSea project, BOOS and HELCOM partners will work together on improving fast delivery of HELCOM data to BOOS and a delivery of a better reanalysis to HELCOM by BOOS to assimilating HELCOM data. HELCOM is also an important stakeholder for both BOOS and Baltic Earth communities. Many projects carried out by BOOS and Baltic Earth partners, such as Baltic Sea Check Point, CLAIM, ECOSUPPORT, address HELCOM goals. HELCOM monitoring is an important source of biogeochemical and biological (lower trophic level) observations for both research and operational services. Carbon observations from ICOS and biological observations collected by ICES are important to BOOS future priority on operational ecology and Baltic Earth System studies.
Recommendations
BOOS and Baltic Earth communities share similar basic research instruments, i.e., monitoring and modeling. Their human resources are also overlapping to a certain degree: most of BOOS partners are also Baltic Earth partners. Collaboration between BOOS and Baltic Earth can help in understanding the climate change influence on the Baltic Sea region in many aspects. For example, adaptation of well-calibrated, high resolution operational models (both local and basin scales) to climate scales can help to reach the last mile to the end users in climate applications. High resolution BOOS observations, e.g., from moorings, ferrybox and shallow water Argo profilers, can benefit for Baltic Earth system process studies, especially for extreme events e.g., salty water inflow, storm surge, marine heat wave, oxygen depletion and algae bloom. The CMEMS ocean reanalysis, with an operational update to months, can provide comprehensive data for most recent changes of climate signals especially statistics of extreme events, although trend analysis from the reanalysis products are problematic because the number of observations changes with time.
Hence, under the background of WMO seamless prediction of Earth systems, GOOS and EuroGOOS focus on provision of information not only for operational services but also for climate change and ocean health and for the UN Decade of Ocean Science, the two communities are motivated to have joint forces in the following two areas:
1. Harmonization and integration of existing research and infrastructure:
- Between synoptic and climate scales:
° Harmonizing model calibration and validation methods for operational and climate scales: Most of the operational models running by BOOS partners have regular calibration and validation. In BAL MFC, such procedure has been standardized based on a continuous effort since 2004 for developing Copernicus Marine Service. This includes models both for near real time forecast and long-term reanalysis. Also the Baltic Earth community has organized several multi-model inter-comparisons and validation studies, mainly for climate scales. The most recent Baltic Earth project is BMIP. The two task forces should harmonize the efforts and aim to reach a more general, standardized calibration and validation procedure for both operational and climate models.
° Adapting calibrated operational models for climate study: Although many institutes still use different models for operational forecast and climate study, there is a trend that more and more models are developed for both forecast and climate modeling. However, there still exist technical challenges when using an operational model for climate purpose. Some adaptations of the operational models to climate modeling are still needed.
° Extending the operational forecast range from synoptic to climate scales (seasonal and decadal predictions): The Baltic Sea is dominated by many slow processes such as seasonal stratification and sea ice, saltwater inflows, water stagnation, and ventilation. Hence, the predictability of seasonal to decadal variability should be investigated in an Earth system framework and forecasting capability should be developed for the corresponding scales with high predictability.
- From physical ocean to ocean system and Earth system:
° End-to-end Earth system modeling: BOOS and Baltic Earth partners from weather and climate, environmental and fishery institutes should work together to jointly develop end-to-end Baltic Earth system modeling framework, including human pressure models, atmospheric models, physical and biogeochemical ocean models, higher trophic level food-web models and socioeconomic models. Such modeling framework can be used both at basin and national levels to support ecosystem-based management and climate change adaptation.
° Data integration: Multi-disciplinary observations, ranging from air, water, seabed and human activity, should be collected and disseminated centrally to serve multiple operational and research purposes. Currently, BOOS and Baltic Earth are working independently for their own purposes in this area. Hence, it is recommended that a common data infrastructure for both research and operational observations should be established.
° Extending forecast from physical ocean to marine ecosystem and to the entire Earth system: operational (coupled) Earth system model should be developed for the Baltic Sea, considering the interactions between atmosphere-ocean-wave-sea ice-marine biogeochemistry-land surface systems.
- Between local and basin scales:
° Stakeholders concern in many cases local applications in relation to climate change, e.g., in the coastal-estuary-catchment continuum. Basin-scale model systems are too coarse for these applications. BOOS partners have developed very high resolution operational models to address local challenges such as storm surges, coastal flooding and eutrophication. However, these local model systems are mainly applied for short time scales. Hence, also for climate applications high-resolution coastal zone models two-way nested into basin-wide Earth system models should be developed.
2. Optimizing research instruments and products:
- Monitoring network: The Baltic Sea monitoring network, including operational, environmental, fishery, and research should be harmonized and optimized through fit-for-purpose assessment, breaking institutional barriers and cost-effective sampling design. Especially, requirements and current gaps for observations for operational Earth system prediction and long-term Earth system study should be identified.
- Modeling platforms: Standards for the next generation of Baltic Earth system models should be defined; common technical challenges for both operational, and climate models should be identified. Joint forces can be made for improving especially high performance computational efficiency, grid flexibility, and model coupling interfaces for targeted model systems.
Author Contributions
All authors contributed in writing, with JS as lead author and main author on operational oceanography, HM as main author on Baltic Earth and VH as main author on BAL MFC.
Funding
Funds for supporting this publication is from Danish Meteorological Institute.
Conflict of Interest
The authors declare that the research was conducted in the absence of any commercial or financial relationships that could be construed as a potential conflict of interest.
References
Asmala, E., Carstensen, J., Conley, D. J., Slomp, C. P., Stadmark, J., and Voss, M. (2017). Efficiency of the coastal filter: nitrogen and phosphorus removal in the Baltic Sea. Limnol. Oceanogr. 62, S222–S238. doi: 10.1002/lno.10644
Axell, L. (2013). BSRA-15: A Baltic Sea Reanalysis 1990-2004. Reports Oceanography, 45. Swedish Meteorological and Hydrological Institute, Norrköping, Sweden.
Axell, L., and Liu, Y. (2016). Application of 3-D ensemble variational data assimilation to a Baltic Sea reanalysis 1989–2013. Tellus A 68:1. doi: 10.3402/tellusa.v68.24220
BACC Author Team (2008). Assessment of Climate Change for the Baltic Sea Basin. Regional Climate Studies. Berlin; Heidelberg: Springer-Verlag.
BACC II Author Team (2015). Second Assessment of Climate Change for the Baltic Sea Basin. Cham: Springer.
Bahurel, P., Adragna, F., Bell, M. J., Jacq, F., Johannessen, J. A., Le Traon, P.-Y., et al. (2010). “Ocean Monitoring and Forecasting Core Services, the European MyOcean Example,” in Proceedings of OceanObs'09: Sustained Ocean Observations and Information for Society, eds J. Hall, D. E. Harrison, and D. Stammer (Venice: ESA Publication WPP-306). doi: 10.5270/OceanObs09.pp.02
Baltic Earth Science Plan Writing Team (2017). Baltic Earth Science Plan 2017. International Baltic Earth Secretariat Publication No. 11, February 2017.
Baschek, B., Schroeder, F., Brix, H., Riethmüller, R., Badewien, T. H., Breitbach, et al. (2017). The coastal observing system for northern and arctic seas (COSYNA). Ocean Sci. 13, 379–410. doi: 10.5194/os-13-379-2017
Berg, P., and Poulsen, J. W. (2012). Implementation Details for HBM. DMI Technical Report No. 12–11 (Copenhagen), 149. Available online at: www.dmi.dk/fileadmin/Rapporter/TR/tr12-11.pdf.
Bergen, B., Naumann, M., Herlemann, D. P. R., Gräwe, U., Labrenz, M., and Jürgens, K. (2018). Impact of a Major inflow event on the composition and distribution of bacterioplankton communities in the Baltic Sea. Front. Mar. Sci. 5:383. doi: 10.3389/fmars.2018.00383
Börgel, F., Frauen, C., Neumann, T., Schimanke, S., and Meier, H. E. M. (2018). Impact of the Atlantic Multidecadal Oscillation on Baltic Sea Variability. Geophys. Res. Lett. 45, 9880–9888. doi: 10.1029/2018GL078943
Buch, E., and Dahlin, H. (2000). BOOS Plan. Baltic Operational Oceanographic System 1999–2003. EuroGOOS Publications nr, 14.
Buch, E., Elken, J., Gajewski, J., Haakansson, B., Kahma, K., and Soetje, K. (2006). “Baltic Operational Oceanographic System BOOS,” in 2006 IEEE US/EU Baltic International Symposium on Integrated Ocean Observation Syst. for Managing Global & Regional Ecosys. Marine Resch, 1–5. doi: 10.1109/BALTIC.2006.7266162
Büchmann, B., and Söderkvist, J. (2016). Internal variability of a 3-D ocean model. Tellus A 68. doi: 10.3402/tellusa.v68.30417
Burchard, H., and Bolding, K. (2002). GETM A General Estuarine Transport Model. Scientific Documentation. Technical Report EUR 20253 EN. Ispra: European Commission. Available online at: https://getm.eu/
Burchard, H. F., Janssen, K., Bolding, L., and Rennau, H. (2009). Model simulations of dense bottom currents in the Western Baltic Sea. Continental Shelf Res. 29, 205–220. doi: 10.1016/j.csr.2007.09.010
Cieślikiewicz, W., Connolly, N., Ollier, G., and O'Sullivan, G. (editors.) (2007). Proceedings of the EurOCEAN 2004 European Conference on Marine Science & Ocean Technology. Published by European Commission, 420.
Dick, S., Kleine, E., and Müller-Navarra, S. (2001). The Operational Circulation Model of BSH (BSH cmod). Model description and validation. Berichte des Bundesamtes für Seeschifffahrt und Hydrographie 29/2001, 48.
Dieterich, C., Wang, S., Schimanke, S., Gröger, M., Klein, B., Hordoir, R., et al. (2019). Surface heat budget over the north sea in climate change simulations. Atmosphere 10:272. doi: 10.3390/atmos10050272
Döscher, R., Willén, U., Jones, C., Rutgersson, A., Meier, H. E. M., Hansson, U., et al. (2002). The development of the regional coupled ocean-atmosphere model RCAO. Boreal Environ. Res. 7, 183–192.
Edman, M., Eilola, K., Almroth-Rosell, E., Meier, H. E. M., Wåhlström, I., and Arneborg, L. (2018). Nutrient retention along the Swedish coastline. Front. Marine Sci. 5:415. doi: 10.3389/fmars.2018.00415
Eilola, K., Gustafson, B. G., Kuznetsov, I., Meier, H. E. M., Neumann, T., and Savchuk, O. P. (2011). Evaluation of biogeochemical cycles in an ensemble of three state-of-the-art numerical models of the Baltic Sea during 1970-2005. J. Marine Systems 88, 267–284, doi: 10.1016/j.jmarsys.2011.05.004
Eilola, K., Meier, H. E. M., and Almroth, E. (2009). On the dynamics of oxygen, phosphorus and cyanobacteria in the Baltic Sea; a model study. J. Marine Syst. 75, 163–184. doi: 10.1016/j.jmarsys.2008.08.009
Ekman, M. (2009). The Changing Level of the Baltic Sea during 300 Years: A Clue to Understanding the Earth. Published by the Summer Institute for Historical Geophysics.
Fu, W., She, J., and Dobrynin, M. (2012). A 20-year reanalysis experiment in the Baltic Sea using three-dimensional variational (3DVAR) method. Ocean Sci. 8, 827–844. doi: 10.5194/os-8-827-2012
Funkquist, L., and Kleine, E. (2007). HIROMB: An Introduction to HIROMB, an Operational Baroclinic Model for the Baltic Sea. Report Oceanography, 37. Swedish Meteorological and Hydrological Institute, Norrkoping, Sweden.
Golbeck, I., Izotova, J., Jandt, S., Janssen, F., Lagemaa, P., Brüning, T., et al (2017). Quality Information Document (QUID) Baltic Sea Physical Analysis and Forecasting Product BALTICSEA_ANALYSIS_FORECAST_PHY_003_006: Issue 4.0. Available online at: http://marine.copernicus.eu/documents/QUID/CMEMS-BAL-QUID-003-006.pdf
Golbeck, I., Li, X., Janssen, F., Brüning, T., and Nielsen, J. W. (2015). Uncertainty estimation for operational ocean forecast products – a multi-model ensemble for the North Sea and the Baltic Sea. Ocean Dynam. 65, 1603–1631. doi: 10.1007/s10236-015-0897-8
Gräwe, U., Naumann, M., Mohrholz, V., and Burchard, H. (2015). Anatomizing one of the largest saltwater inflows into the B altic S ea in D ecember 2014. J. Geophys. Res. 120, 7676–7697. doi: 10.1002/2015JC011269
Green, J. M., Liljebladh, B., and Omstedt, A. (2006). Physical oceanography and water exchange in the Northern Kvark Strait. Continental Shelf Res. 26, 721–732. doi: 10.1016/j.csr.2006.01.012
Gröger, M., Arneborg, L., Dieterich, C., Höglund, A., and Meier, H. E. M. (2019). Hydrographic changes in the North Sea and Baltic Sea projected in an ensemble of climate scenarios downscaled with a coupled regional ocean-sea ice-atmosphere model. Climate Dynam. 53, 5945–5966. doi: 10.1007/s00382-019-04908-9
Gröger, M., Maier-Reimer, E., Mikolajewicz, U., Moll, A., and Sein, D. (2013). NW European shelf under climate warming: implications for open ocean-shelf exchange, primary production, and carbon absorption. Biogeosciences 10, 3767–3792. doi: 10.5194/bg-10-3767-2013
Gustafsson, N., Nyberg, L., and Omstedt, A. (1998). Coupling of a high-resolution Atmospheric Model and an Ocean Model for the Baltic Sea. Monthly Weather Rev. 126, 2822–2846. doi: 10.1175/1520-0493(1998)126<2822:COAHRA>2.0.CO;2
Haapala, J., and Leppäranta, M. (1996). Simulating the Baltic Sea ice season with a coupled ice-ocean model. Tellus A 48, 622–643. doi: 10.1034/j.1600-0870.1996.t01-4-00003.x
Haavisto, N., Tuomi, L., Roiha, P., Siiriä, S.-M., Alenius, P., and Purokoski, T. (2018). Argo floats as a novel part of the monitoring the hydrography of the Bothnian Sea. Front. Mar. Sci. 5:324. doi: 10.3389/fmars.2018.00324
Hagedorn, R., Lehmann, A., and Jacob, D. (2000). A coupled high resolution atmosphere-ocean model for the BALTEX region. Meteorol. Zeitschr. 9, 7–20. doi: 10.1127/metz/9/2000/7
HELCOM (2013). HELCOM Monitoring and Assessment Strategy, 37. Available online at: http://www.helcom.fi/Documents/Action%20areas/Monitoring%20and%20assessment/Monitoring%20and%20assessment%20strategy/Monitoring%20and%20assessment%20strategy.pdf
Hill, K. L., Moltmann, T., Proctor, R., and Allen, S. (2009). Australia's integrated marine observing system. Meteorol. Technol. Int. 1, 114–120.
Ho-Hagemann, H. T. M., Gröger, M., Rockel, B., Geyer, B., Zahn, M., and Meier, H. E. M. (2017). Effects of air-sea coupling over the North Sea and the Baltic Sea on simulated summer precipitation over Central Europe. Climate Dynam. 49, 3851–3876. doi: 10.1007/s00382-017-3546-8
Holtermann, P. L., Prien, R., Naumann, M., Mohrholz, V., and Umlauf, L. (2017). Deepwater dynamics and mixing processes during a major inflow event in the central Baltic Sea. J. Geophys. Res. 122, 6648–6667. doi: 10.1002/2017JC013050
Holtermann, P. L., and Umlauf, L. (2012). The Baltic Sea tracer release experiment: 2. Mixing processes. J. Geophys. Res. 117:C01022. doi: 10.1029/2011JC007445
Holtermann, P. L., Umlauf, L., Tanhua, T., Schmale, O., Rehder, G., and Waniek, J. J. (2012). The Baltic Sea tracer release experiment: 1. Mixing rates. J. Geophys. Res. 117:C01021. doi: 10.1029/2011JC007439
Hordoir, R., Axell, L., Höglund, A., Dieterich, C., Fransner, F., and Andersson, M. (2018). Nemo-Nordic 1.0: a NEMO based ocean model for Baltic & North Seas, research and operational applications. Geosci. Model Dev. Discuss. 11, 2353–2371. doi: 10.5194/gmd-2018-2
Kniebusch, M., Meier, H. E. M., and Neumann, T. (2019a). Temperature variability of the Baltic Sea since 1850 in model simulations and observations and attribution to atmospheric forcing. J. Geophys. Res. 124, 4168–4187. doi: 10.1029/2018JC013948
Kniebusch, M., Meier, H. E. M., and Radtke, H. (2019b). Changing salinity gradients in the Baltic Sea as a consequence of altered freshwater budgets. Geophys. Res. Lett. 46, 9739–9747. doi: 10.1029/2019GL083902
Larsen, J., Høyer, J. L., and She, J. (2007). Validation of a hybrid optimal interpolation and Kalman filter scheme for sea surface temperature assimilation. J. Mar. Sys. 65, 122–133. doi: 10.1016/j.jmarsys.2005.09.013
Le Traon, P.-Y., Reppucci, A., Fanjul, E. A., Aouf, L., Behrens, A., Belmonte, M., et al. (2019). From observation to information and users: the Copernicus Marine Service perspective. Front. Mar. Sci. 6:234. doi: 10.3389/fmars.2019.00234
Liu, Y., Meier, H. E. M., and Eilola, K. (2017). Nutrient transports in the Baltic Sea – results from a 30-year physical–biogeochemical reanalysis. Biogeosciences 14, 2113–2131. doi: 10.5194/bg-14-2113-2017
Maar, M., Møller, E. F., Larsen, J., Madsen, K. S., Wan, Z., She, J., et al. (2011). Ecosystem modelling across a salinity gradient from the North Sea to the Baltic Sea. Ecol. Model. 10:1696–1711. doi: 10.1016/j.ecolmodel.2011.03.006
Meier, H. E. M. (2005). Modeling the age of Baltic Seawater masses: quantification and steady state sensitivity experiments. J. Geophys. Res. 110:C02006, doi: 10.1029/2004JC002607
Meier, H. E. M. (2007). Modeling the pathways and ages of inflowing salt- and freshwater in the Baltic Sea. Estuarine Coastal Shelf Sci. 744, 717–734. doi: 10.1016/j.ecss.2007.05.019
Meier, H. E. M., Andersson, H. C., Arheimer, B., Blenckner, T., Chubarenko, B., Donnelly, C., et al. (2012). Comparing reconstructed past variations and future projections of the Baltic Sea ecosystem - first results from multi-model ensemble simulations. Environ. Res. Lett. 7:034005. doi: 10.1088/1748-9326/7/3/034005
Meier, H. E. M., Edman, M., Eilola, K., Placke, M., Neumann, T., Andersson, H., et al. (2018a). Assessment of eutrophication abatement scenarios for the Baltic Sea by multi-model ensemble simulations. Front. Mar. Sci. 5:440. doi: 10.3389/fmars.2018.00440
Meier, H. E. M., Edman, M., Eilola, K., Placke, M., Neumann, T., and Savchuk, H. (2019). Assessment of uncertainties in scenario simulations of biogeochemical cycles in the Baltic Sea. Front. Mar. Sci. 6:46. doi: 10.3389/fmars.2019.00046
Meier, H. E. M., Feistel, R., Piechura, J., Arneborg, L., Burchard, H., Fiekas, V., et al. (2006). Ventilation of the Baltic Sea deep water: a brief review of present knowledge from observations and models. Oceanologia 48, 133–164.
Meier, H. E. M., Rutgersson, A., and Reckermann, M. (2014). Baltic Earth - a new earth system science program for the Baltic Sea Region. EOS Trans. AGU 95, 109–110. doi: 10.1002/2014EO130001
Meier, H. E. M., Väli, G., Naumann, M., Eilola, K., and Frauen, C. (2018b). Recently accelerated oxygen consumption rates amplify deoxygenation in the Baltic Sea. J. Geophys. Res. 123, 3227–3240. doi: 10.1029/2017JC013686
Mohrholz, V. (2018). Major Baltic Inflow Statistics – Revised. Front. Marine Sci. 5:384. doi: 10.3389/fmars.2018.00384
Mohrholz, V., Naumann, M., Nausch, G., Krüger, S., and Gräwe, U. (2015). Fresh oxygen for the Baltic Sea—An exceptional saline inflow after a decade of stagnation. J. Marine Syst. 148, 152–166. doi: 10.1016/j.jmarsys.2015.03.005
Neumann, T. (2000). Towards a 3d-ecosystem model of the Baltic Sea. J. Mar. Sys. 25, 405–419. doi: 10.1016/S0924-7963(00)00030-0
Omstedt, A., Elken, J., Lehmann, A., Leppäranta, M., Meier, H. E. M., Myrberg, K., et al. (2014). Progress in physical oceanography of the Baltic Sea during the 2003–2014 period. Progress Oceanogr. 128, 139–171. doi: 10.1016/j.pocean.2014.08.010
Omstedt, A., Elken, J., Lehmann, A., and Piechura, J. (2004). Knowledge of the Baltic Sea physics gained during the BALTEX and related programmes. Progress Oceanogr. 63, 1–28. doi: 10.1016/j.pocean.2004.09.001
Placke, M., Meier, H. E. M., Gräwe, U., Neumann, T., and Liu, Y. (2018). Long-term mean circulation of the Baltic Sea as represented by various ocean circulation models. Front. Mar. Sci. 5:287. doi: 10.3389/fmars.2018.00287
Poulsen, J. W., Berg, P., and Raman, K. (2014). “Chapter 3 - Better concurrency and SIMD on HBM,” in High Performance Parallelism Pearls: Multicore and Many-core Programming Approaches, eds J. Jeffers and J. Reinders (Morgan Kaufmann Publishing), 43–67. doi: 10.1016/B978-0-12-802118-7.00003-0
Raschke, E., Meywerk, J., Warrach, K., Andrea, U., Bergström, S., Beyrich, F., et al. (2001). The Baltic Sea Experiment (BALTEX) a European contribution to the investigation of the energy and water cycle over a large drainage basin. Bull. Am. Meteorol. Soc. 82, 2389–2413. doi: 10.1175/1520-0477(2001)082<2389:TBSEBA>2.3.CO;2
Reckermann, M., Langner, J., Omstedt, A., von Storch, H., Keevallik, S., and Hünicke, B. (2011). BALTEX - An interdisciplinary research network for the Baltic Sea region. Environ. Res. Lett. 6:045205. doi: 10.1088/1748-9326/6/4/045205
Reissmann, J. H., Burchard, H., Feistel, R., Hagen, E., Lass, H. U., Mohrholz, V., et al. (2009). Vertical mixing in the Baltic Sea and consequences for eutrophication–A review. Progress Oceanogr. 82, 47–80. doi: 10.1016/j.pocean.2007.10.004
Rodhe, J., and Winsor, P. (2002). On the influence of the freshwater supply on the Baltic Sea mean salinity. Tellus A 54, 175–186. doi: 10.3402/tellusa.v54i2.12134
Rodriguez, H., Fisher, E., and Schuurbiers, D. (2013). Integrating science and society in European Framework Programmes: trends in project-level solicitations. Res. Policy 42, 1126–1137. doi: 10.1016/j.respol.2013.02.006
Roiha, P., Siiriä, S.-M, Haavisto, N., Alenius, P., Westerlund, A., and Purokoski, T. (2018). Estimating currents from argo trajectories in the bothnian Sea, Baltic Sea. Front. Mar. Sci. 5:308. doi: 10.3389/fmars.2018.00308
Schimanke, S., and Meier, H. E. M. (2016). Decadal-to-centennial variability of salinity in the Baltic Sea. J. Climate 29, 7173–7188. doi: 10.1175/JCLI-D-15-0443.1
Schimanke, S., Meier, H. E. M., Kjellström, E., Strandberg, G., and Hordoir, R. (2012). The climate in the Baltic Sea region during the last millennium simulated with a regional climate model. Climate Past 8, 1419–1433. doi: 10.5194/cp-8-1419-2012
Schmale, O., Krause, S., Holtermann, P., Power Guerra, N. C., and Umlauf, L. (2016). Dense bottom gravity currents and their impact on pelagic methanotrophy at oxic/anoxic transition zones. Geophys. Res. Lett. 43, 5225–5232. doi: 10.1002/2016GL069032
Schrum, C., Hübner, U., Jacob, D., and Podzun, R. (2003). A coupled atmosphere/ice/ocean model for the North Sea and the Baltic Sea. Climate Dynam. 21, 131–151. doi: 10.1007/s00382-003-0322-8
She, J. (2018a). “Emerging needs on operational oceanography to serve sustainable development in Baltic-North Sea,” in Proceedings of the Eight EuroGOOS International Conference, eds E. Buch, V. Fernández, D. Eparkhina, P. Gorringe, and G. Nolan (Belgium : EuroGOOS, 463–472.
She, J. (2018b). “Assessment of Baltic Sea observations for operational oceanography,” in Proceedings of the Eight EuroGOOS International Conference, eds E. Buch, V. Fernández, D. Eparkhina, P. Gorringe and G. Nolan (Brussels: EuroGOOS, 79–87.
She, J., Allen, I., Buch, E., Crise, A., Johannessen, J. A., Le Traon, P.-Y., et al. (2016). Developing European operational oceanography for Blue Growth, climate change adaptation and mitigation, and ecosystem-based management. Ocean Sci. 12, 953–976. doi: 10.5194/os-12-953-2016
She, J., and Murawski, J. (2018). “Towards seamless modelling for the Baltic Sea. Operational Oceanography serving Sustainable Marine Development,” in Proceedings of the Eight EuroGOOS International Conference, eds E. Buch, V. Fernández, D. Eparkhina, P. Gorringe, and G. Nolan (Belgium: EuroGOOS, 233–241.
She, J., and Nielsen, J. W. (1999). Operational Wave Forecasts Over Baltic and North Sea. Scientific Report 99-7, Danish Meteorological Institute, Copenhagen, Denmark.
Siiriä, S., Roiha, P., Tuomi, L., Purokoski, T., Haavisto, N., and Alenius, P. (2018). Applying area-locked, shallow Argo floats in the Baltic Sea monitoring. J. Operat. Oceanogr. 12, 58–72. doi: 10.1080/1755876X.2018.1544783
Tian, T., Boberg, F., Christensen, O. B., Christensen, J. H., She, J., and Vihma, T. (2013). Resolved complex coastlines and land–sea contrasts in a high-resolution regional climate model: a comparative study using prescribed and modelled SSTs. Tellus A 65:19951. doi: 10.3402/tellusa.v65i0.19951
Tian, T., Su, T., Boberg, F., Yang, S., and Schmith, T. (2016). Estimating uncertainty caused by ocean heat transport to the North Sea: experiments downscaling EC-Earth. Climate Dynam. 46, 99–110. doi: 10.1007/s00382-015-2571-8
Trenberth, K. E., Jones, P. D., Ambenje, P., Bojariu, R., Easterling, D., Klein Tank, A., et al. (2007). “Observations: surface and atmospheric climate change,” in Climate Change 2007: The Physical Science Basis. Contribution of Working Group I to the Fourth Assessment Report of the Intergovernmental Panel on Climate Change, eds S. Solomon, D. Qin, M. Manning, Z. Chen, M. Marquis, K. B. Averyt, M. Tignor, and H. L. Miller (Cambridge: Cambridge University Press). 235–336.
Tuomi, L., Pettersson, H., and Kahma, K. (1999). Preliminary Results From the WAM Wave Model Forced by the Mesoscale EUR-HIRLAM Atmospheric Model. MERI – Report series of the Finnish Institute of Marine Research 40, 19–23.
Tuomi, L., She, J., Lorkowski, I., Axell, L., Lagemaa, P., Schwichtenberg, F., et al. (2018). “Overview of CMEMS BAL MFC Service and Developments,” in Proceedings of the Eigth EuroGOOS International Conference, eds E. Buch, V. Fernández, D. Eparkhina, P. Gorringe, and G. Nolan (Brussels; Belgium: EuroGOOS, 261–268.
Umlauf, L., and Arneborg, L. (2009a). Dynamics of rotating shallow gravity currents passing through a channel. Part I: observation of transverse structure. J. Phys. Oceanogr. 39, 2385–2401. doi: 10.1175/2009JPO4159.1
Umlauf, L., and Arneborg, L. (2009b). Dynamics of rotating shallow gravity currents passing through a channel. Part II: analysis. J. Phys. Oceanogr. 39, 2402–2416. doi: 10.1175/2009JPO4164.1
Van Pham, T., Brauch, J., Dieterich, C., Frueh, B., and Ahrens, B. (2014). New coupled atmosphere-ocean-ice system COSMO-CLM/NEMO: assessing air temperature sensitivity over the North and Baltic Seas. Oceanologia 56, 167–189. doi: 10.5697/oc.56-2.167
von Schomberg, R. (2013). “A vision of responsible research and innovation,” in Responsible Innovation, eds R. Owen, J. Bessant, and M. Heintz (London: John Wiley), 51–74. doi: 10.1002/9781118551424.ch3
WAMDI (1988). The WAM model - A third generation ocean wave prediction model. J. Phys. Oceanogr. 18, 1775–1810. doi: 10.1175/1520-0485(1988)018<1775:TWMTGO>2.0.CO;2
Wang, S., Dieterich, C., Döscher, R., Höglund, A., Hordoir, R., Meier, H. E. M., et al. (2015). Development and evaluation of a new regional coupled atmosphere–ocean model in the North Sea and Baltic Sea. Tellus A 67:24284. doi: 10.3402/tellusa.v67.24284
Wilhelmsson, T. (2002). Parallellization of the HIROMB Ocean Model. Licentiate Thesis. Royal Institute of Technology, Department of Numerical and Computer Science, Stockholm, Sweden.
WMO (2015). World Meteorological Organisation. Seamless Prediction of the Earth System: From Minutes to Months. Available online at: https://library.wmo.int/opac/doc_num.php?explnum_id=3546
Keywords: operational oceanography, Baltic Sea, BOOS, observational networks, seamless modeling, earth system study, Baltic Earth
Citation: She J, Meier HEM, Darecki M, Gorringe P, Huess V, Kouts T, Reissmann JH and Tuomi L (2020) Baltic Sea Operational Oceanography—A Stimulant for Regional Earth System Research. Front. Earth Sci. 8:7. doi: 10.3389/feart.2020.00007
Received: 14 January 2019; Accepted: 16 January 2020;
Published: 05 February 2020.
Edited by:
Klaus D. Joehnk, Commonwealth Scientific and Industrial Research Organisation (CSIRO), AustraliaReviewed by:
Anna Rutgersson, Uppsala University, SwedenBeata Szymczycha, Institute of Oceanology (PAN), Poland
Copyright © 2020 She, Meier, Darecki, Gorringe, Huess, Kouts, Reissmann and Tuomi. This is an open-access article distributed under the terms of the Creative Commons Attribution License (CC BY). The use, distribution or reproduction in other forums is permitted, provided the original author(s) and the copyright owner(s) are credited and that the original publication in this journal is cited, in accordance with accepted academic practice. No use, distribution or reproduction is permitted which does not comply with these terms.
*Correspondence: Jun She, js@dmi.dk