- 1Max Planck Institute for Biogeochemistry, Jena, Germany
- 2Thüringer Landesanstalt für Umwelt und Geologie, Jena, Germany
The origin, molecular composition and fate of dissolved organic matter (DOM) provides essential information that links surface and subsurface processes and explores the functioning of the Critical Zone. Therefore it is important to identify specific marker compounds that provide information on the temporal and spatial linkages in the Critical Zone. Here, we used ultra-high resolution mass spectrometry and accelerator mass spectrometry in order to identify markers for fast transport of surface-derived DOM through the Critical Zone. We assessed the molecular composition and radiocarbon age of solid phase extracted DOM (SPE-DOM) from forest top soils. The 14C ages of SPE-DOM in our study were similar to that of bulk DOM. Calibrated ages ranged from 0 to 49 years and the within-site variability was larger than between sampling sites. Spearman rank correlation between the 14C ages and the DOM composition identified 129 sum formulae that were significantly correlated to 14C age. We found that molecular entities with younger 14C ages had lower molecular weight, higher unsaturation and less oxygen and heteroatoms than those associated with older 14C ages. A chemical library search suggested that phenylpropanoids and compounds, which are known to be lignin derived, are key molecular species for terrestrial DOM with young 14C ages. Among them, lignin dimers emerged as prominent surface-derived compounds that can potentially be used as markers for fast transport of water and DOM into the subsurface and groundwater.
Introduction
Processes that are taking place in the Critical Zone (CZ) are mirrored in the chemical composition of dissolved organic matter (DOM) in surface-, soil- and groundwater (Wickland et al., 2007; Kaiser and Kalbitz, 2012; Gabor et al., 2014; Roth et al., 2015; Seifert et al., 2016). Major sources of DOM in terrestrial ecosystems include young carbon inputs from the decomposition of plant litter and leaching of microbially modified (Kallenbach et al., 2016), aged soil organic matter (SOM). Composition and fate of DOM are strongly influenced by the ecosystem as a whole (Nebbioso and Piccolo, 2013; Miller et al., 2016) and the discovery of terrestrial ecosystem-specificity of DOM spectra highlights their close connection (Roth et al., 2014). As a result, tracing surface signals over time has emerged as a key objective in CZ research (Küsel et al., 2016). Specific markers for 14C young and surface-derived inputs could help to trace surface signals into the subsurface. Their degradability could further make the occurrence of these marker compounds in groundwater an indicator for fast transport of water and DOM through the CZ.
The search for compositional marker molecules that identify ecosystem constitution and source characteristics (Kujawinski et al., 2009; Roth et al., 2014; Dubinenkov et al., 2015; Kothawala et al., 2015) is strongly aided by the integration of non-targeted Fourier transform ion cyclotron resonance mass spectrometry (FT-ICR-MS). FT-ICR-MS allows study of the molecular composition of DOM in unprecedented detail, providing chemical information about thousands of mass-resolved peaks due to accurate determination of their exact mass (Hertkorn et al., 2013).
Correlating the molecular composition of DOM with 14C ages of the respective samples has led to the identification of degradation states of marine DOM on the basis of individual sum formulae (Flerus et al., 2012). However, marine derived marker compounds cannot be readily applied to terrestrial systems due to terrestrial ecosystem heterogeneity and DOM representing a snapshot of ecosystem activities (Roth et al., 2014). Generally, marine DOM is composed of a wide range of compounds ranging from labile carbohydrates to refractory black carbon (Moran et al., 2016), while terrestrial DOM contains linear terpenoids and carboxyl-rich-alicyclic molecules in high proportions (Hertkorn et al., 2006; Arakawa and Aluwihare, 2015). Thus, new markers applicable for terrestrial ecosystem processes and characteristics are required.
To achieve traceability of 14C young DOM through the subsurface, potential markers for CZ DOM must link to the major constituents of plant biomass. Plant litter is generally composed of the biopolymers cellulose and lignin as well as monomeric natural compounds such as carbohydrates and terpenes (Thomson, 1993). During the process of decomposition lignin was suggested to be comparatively recalcitrant in a variety of environments (Melillo et al., 1982; Hedges et al., 1985; Akin and Benner, 1988). However, more recent investigations highlight that lignin structures are not recalcitrant (Gleixner et al., 2002; Schmidt et al., 2011; Waggoner et al., 2017). Lignin phenols were further shown to be young components in both sediments and DOM of arctic rivers (Feng et al., 2013, 2015, 2017). Potential pathways for the removal of lignin structures include photochemical alteration (Waggoner et al., 2017), microbial degradation (Ward et al., 2013) in aquatic systems as well as sorption onto minerals (Kaiser et al., 2004; Hernes et al., 2013) and microbial degradation in soils (Gleixner et al., 2002). As a consequence, lignin-derived compounds may be used for tracing fast flow processes of recent biomass through the CZ.
In non-targeted ultra-high resolution studies of DOM current limitations for the identification of new and specific marker compounds arise from lack of information on the structure of specific molecules and the multitude of structural isomers per molecular formula (Zark et al., 2017). Our efforts were therefore targeted at receiving direct molecular information from the sum formulae level on the basis of chemical databases and annotated information on their biogeochemical relevance (Degtyarenko et al., 2008).
In this study we explored the feasibility of combining ultra-high resolution molecular composition data with 14C ages to identify marker compounds for the transport of water and DOM in the CZ from the surface into the subsurface. We hypothesize that (1) leaching from plant biomass governs the molecular composition of 14C-young carbon inputs and (2) non-recalcitrance of lignin molecules allows selection of potential 14C-young marker compounds that relate to their plant litter source.
Materials and Methods
Sampling
Soil water samples were collected in March, May, and November of 2005 at three different long term monitoring sites in Germany. The first site is a deciduous old-growth forest in the Hainich National Park [51°04′46″N, 10°27′08 ″E, 440 m above sea level (a.s.l.)], Thuringia (Knohl et al., 2003; Gleixner et al., 2009; Tefs and Gleixner, 2012). The forest is unmanaged and dominated by European beech (Fagus sylvatica, 65%) and ash (Fraxinus excelsior, 25%). Understory vegetation includes Alium ursinium, Mercurialis perennis, and Anemone nemorosa. The soil column consists of a 5 to 15 cm deep A horizon which is followed by a clayish T horizon on 50 to 60 cm deep cambisol. The second site is a maple (Acer pseudoplatanus), pine and spruce (Pinus sylvestris and Picea abies) mixed forest stand near the village of Thann (49°19′24″N, 12°27′37″E), Bavaria. A 5 to 7 cm deep organic layer overlays a sandy cambisol developed on granite parent material (Sachse et al., 2009). The third site is the Wetzstein forest (50°27′13″N, 11°27′27″E, 785 m a.s.l.) in Thuringia. This managed forest consists mostly of ca. 50 year old Norway spruce (Picea abies) trees on an acidic and carbonate-free podzol (Schulze et al., 2005; Moyano et al., 2008; Kindler et al., 2011). Setup and sampling was conducted with permission of the national park administration, the land owner and the forest management administration, respectively, for the sites. The diversity in vegetation and soil parameters within the sample set results in a relatively wide coverage of top soil DOM conditions. Together with the replicated sampling throughout the growing season we aim at providing overarching trends in DOM composition not biased through site-specific effects.
Sample Preparation and FT-ICR-MS Analysis
The water samples were taken during a regular biweekly sampling scheme using glass ceramic suction plates with a pore size of 1–1.6 μm which were permanently installed at 5 cm soil depth. If samples were unavailable at 5 cm on a specific sampling location, we used samples from 10 cm depth. Bottles connected to the suction plates were evacuated to 20 kPa such that free flowing soil water was sucked through the ceramic plate into the bottle until pressure equilibrium was reached. As a result of the biweekly sampling scheme, each sampling time point integrates the water flow of 2 weeks. Water samples were immediately freeze-dried in the lab and stored at room temperature in the dark. After re-dissolution, DOM was subjected to solid phase extraction using PPL (styrene-divinylbenzene polymer) cartridges according to previously established protocols (Dittmar et al., 2008), with an average extraction efficiency of 63%. A procedural blank of ultrapure water (pH 2) was stored and extracted together with samples.
FT-ICR-MS analysis was performed at the University of Oldenburg, Germany (Roth et al., 2015). The FT-ICR-MS (Bruker Solarix, 15 T) measurement was conducted in negative electrospray ionization mode at a continuous injection flow of 120 μL/h and ESI needle voltage of -4 KV. 500 scans of m/z 150–2000 and 0.25 s ion accumulation time were combined into one spectrum. Peaks with signal/noise (S/N)> 3 and within 150–800 m/z were used for further analysis. Ions with m/z> 800 were not detected. Peaks found in the procedural blank were removed from the peak list of the samples. For molecular formula assignment the elements C, H, O, N, and S (N ≤ 2, S ≤ 1) were utilized in accordance with previous studies (Herzsprung et al., 2012; Riedel et al., 2012). Compounds with successful molecular formula assignment were considered for further data interpretation (Supplementary Table S4).
Radiocarbon Analysis
PPL extracts were dried into tin capsules for combustion to CO2 in an elemental analyzer, graphitization and subsequent radiocarbon analysis on a 3 MV Tandetron 14C-AMS at the MPI-BGC in Jena, Germany together with size-matched modern (Oxalic Acid II) and 14C-depleted standard materials (Steinhof et al., 2004, 2010). The δ13C values of the samples were determined online in the AMS. Age calibration was performed on the background corrected fraction modern [F14C; (Reimer et al., 2004; Steinhof, 2013)] values using OxCal 4.3 (Bronk Ramsey, 2009) and the NH1 bomb 2013 curve (Hua et al., 2013). Resulting median calAD dates were subtracted from the year of sampling (2005) to give an age estimate.
14C System Blank of SPE-DOM
A system blank test was conducted using DOM from the Wetzstein site that was (i) non-extracted, (ii) PPL extracted, (iii) PPL extracted, dried and re-dissolved in ultrapure water once, and (iv) re-dissolved three times. For (i) DOM material from the Wetzstein site was de-carbonified by acidifying with HCl and bubbling with N2 in order to remove inorganic carbon from affecting the 14C/12C DOM signal. For (ii) the same original material as in (i) was extracted onto PPL cartridges and eluted with methanol (Dittmar et al., 2008). For (iii) and (iv) aliquots of the eluate from (ii) were dried and re-dissolved in ultrapure water in order to break any methyl ester bonds that might have formed to the methanol solvent and would skew the 14C/12C isotopic ratio.
Statistical Analysis and Correlation
Spearman rank correlation was employed on relative intensities of mass peaks with respect to the 14C calibrated ages of the samples. Rank correlation was used to account for known competitive ionization efficiencies that affect the ion intensities of the individual masses (Hertkorn et al., 2008; Herzsprung et al., 2012). Only mass peaks present in at least 8 out of 10 samples were considered for correlation in order to identify bulk trends. Statistical data analysis and correlation was done in R 3.4.1 using the R package “vegan” 2.4–5. Principal component analysis (PCA) was performed on the matrix of relative intensities of m/z values per sample, which was centered and scaled by the standard deviations. The environmental parameters pH, cal 14C age, DOC concentration and length of the growing season were fitted to the PC values as vectors. For characterization of molecular entities matching of sum formulae with the ChEBI database was performed using the web interface at http://www.ebi.ac.uk/chebi/ (Hastings et al., 2016). We chose the ChEBI database for its focus on “small” chemical compounds that have biological relevance, its links and integration with similar chemical databases [(for example PubChem (Kim et al., 2016) and KEGG (Kanehisa et al., 2017)], free availability and general ease of use for the extraction of annotated information and chemical structures.
Results and Discussion
14C System Blank of SPE-DOM
Solvent residues in carbon extracts resulting from elution steps can influence the 14C/12C ratio in organic matter samples. As for PPL extraction, the methanol solvent could esterify free carboxyl groups in the organic matter (McIntyre and McRae, 2005), thereby introducing 14C-depleted carbon to the sample, which persists even after evaporating to dryness. Depending on the abundance of carboxyl groups in the sample, the percentage of modern carbon would be expected to be significantly reduced especially if samples are comprised entirely of modern carbon whereas commercially bought methanol is usually produced from fossil carbon sources. Since methyl ester bonds are highly susceptible to hydrolysis, re-dissolution in water could help to remove potential solvent residues. Testing our extraction method for changes of 14C/12C before and after extraction as well as after hydrolysis of residual ester bound methanol yielded highly similar percentages of modern carbon (pMC; Figure 1). A one-way analysis of variance (ANOVA) revealed that there was no significant difference (α = 0.05) between the groups. We conclude that the 14C/12C ratios of SPE-DOM in our study are not influenced by residual ester bound MeOH and likely represent the 14C/12C ratios of bulk DOM.
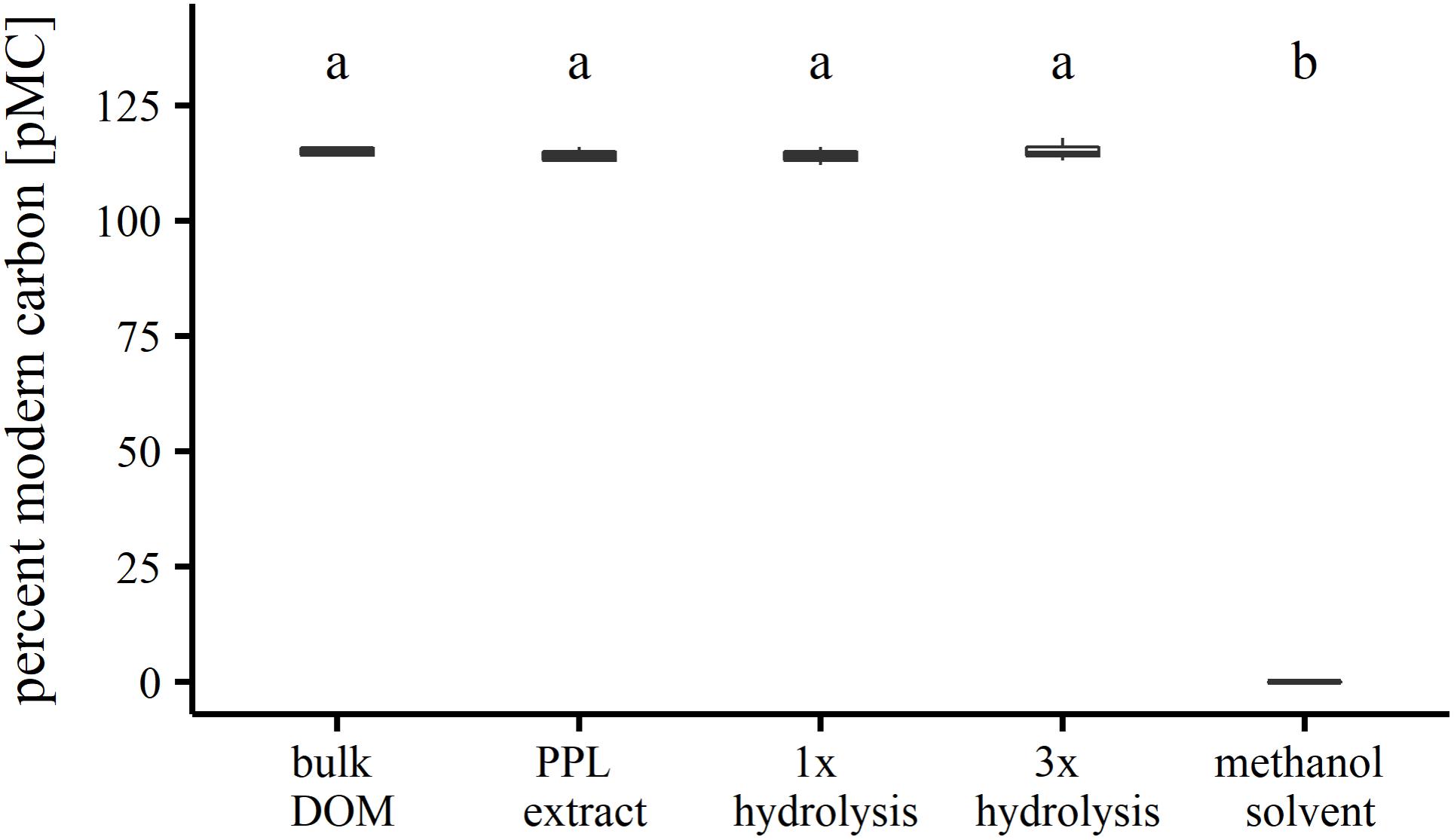
FIGURE 1. Radiocarbon analysis of SPE-DOM before and after extraction and after hydrolysis of potential residual ester-bound methanol.
Age Correlation of Molecular Entities
Principal component analysis (PCA) using the exact masses of molecules with annotated sum formulae summarized 68.2% of the variability in the molecular data by the first two principal components (PC): PC1 49.2%, PC2 19.0%. The PCA produced a well-separated clustering reflecting the 3 sampling sites (Figure 2). The fitted environmental parameters DOC concentration and pH were correlated with PC1. Cal 14C age was correlated to PC2. In a previous assessment none of the considered environmental parameters were correlated to PC2 (Roth et al., 2015). Our results show that cal 14C age can help to interpret additional variability in DOM composition.
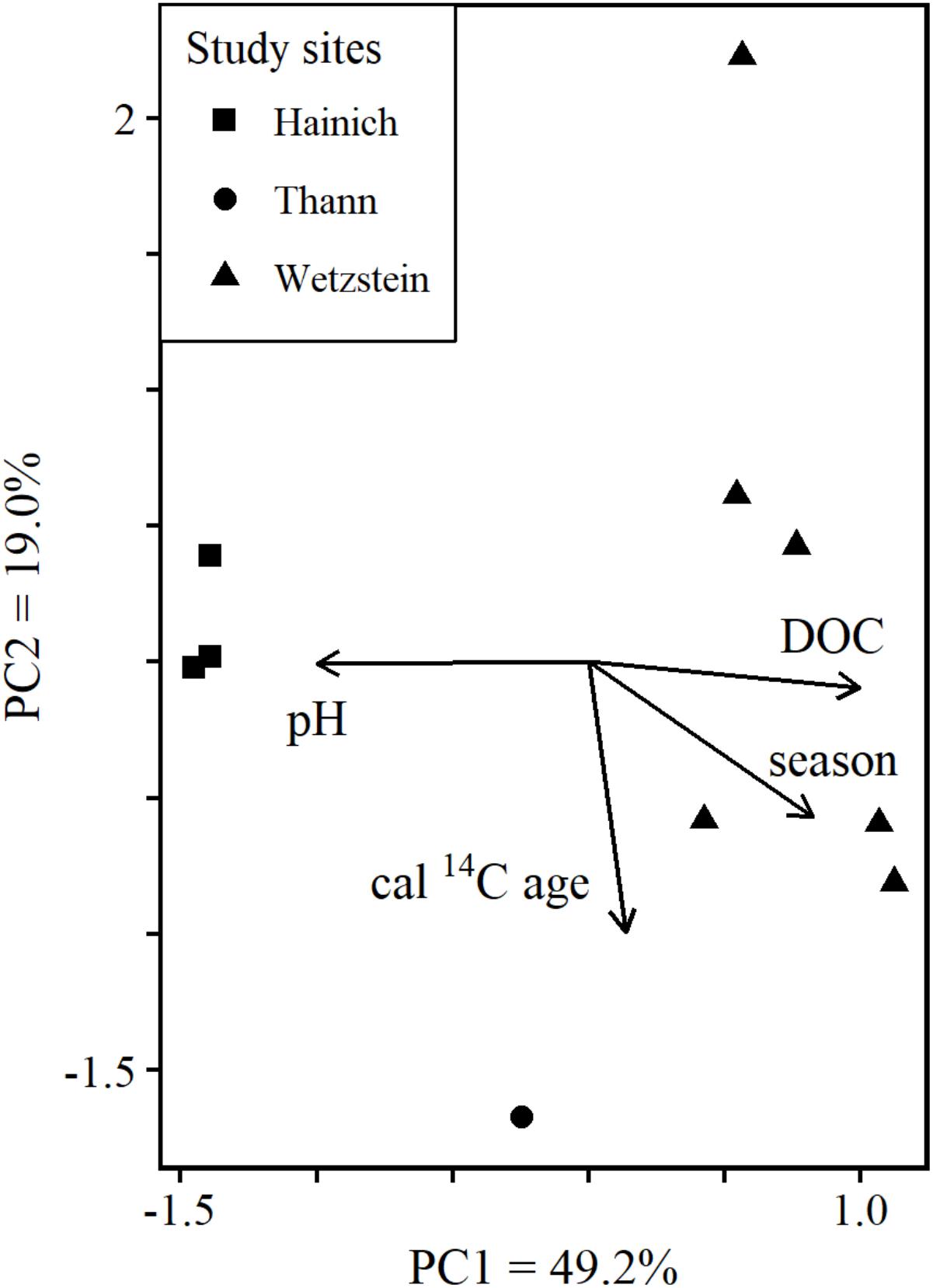
FIGURE 2. Ordination plot from PCA based on the molecular composition of samples with cal. age data. All masses with molecular formula assignment and their normalized FT-ICR-MS intensities were used for calculating the PCA. DOC concentration, pH, growing season and cal. age were fitted as environmental vectors.
The radiocarbon activity of the samples ranged from 102 to 117 percent Modern Carbon (pMC). All samples were “modern” and the calibrated ages ranged between 0 and 49 years (Table 1). The within-site variability was higher than between sampling sites, suggesting that radiocarbon ages in this study are not biased through site-effects.
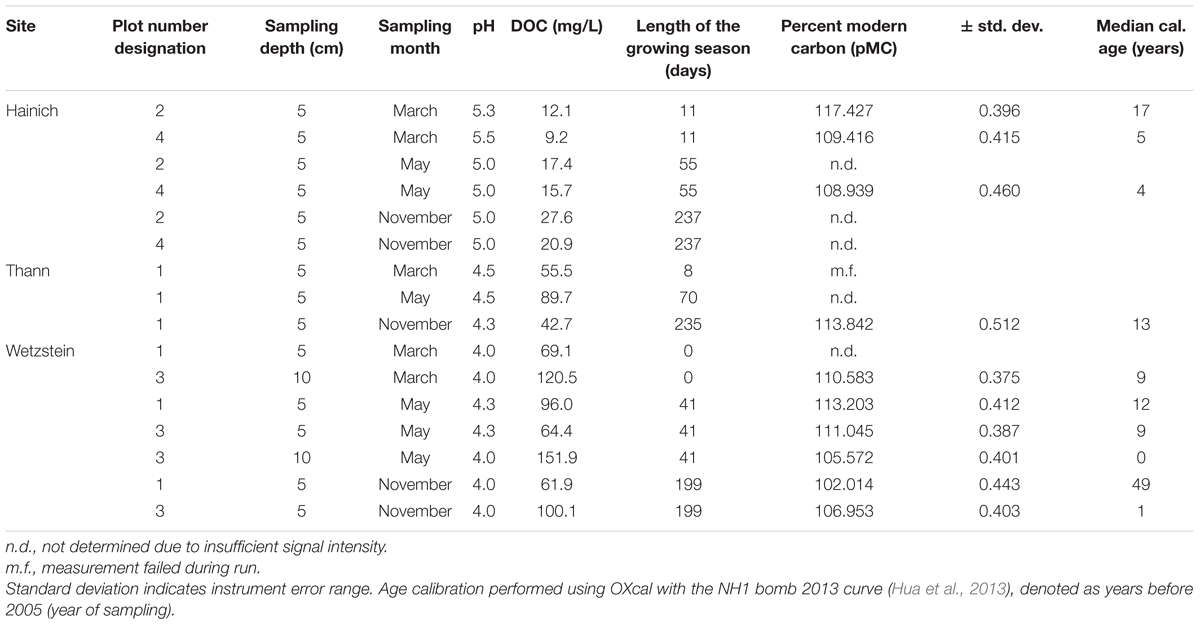
TABLE 1. Overview of the sample sites regarding pH, DOC concentration, length of the growing season and radiocarbon ages of SPE-DOM.
From here on we refer to negative/positive correlation with age of a molecular formula in cases when the rank relative intensity distribution of the respective sum formula was significantly descending/ascending between samples from low cal 14C age to samples with higher cal 14C age, respectively. We observed distinct differences in the m/z ranges as well as H/C and O/C elemental ratios between the group of negatively and positively correlated compounds (Figure 3). The masses of negatively correlated formulae ranged from 250 to 450 m/z while the positively correlated formulae ranged from 450 to 650 m/z. This indicates that inputs into soil water DOM containing recently assimilated carbon are likely of lower molecular weight compared to molecular entities comprised of 14C-older carbon. The full distribution of masses and respective rank correlation strength values further suggests a continuous mass-age relation rather than fractionated sub pools of young and old DOM (Supplementary Figure S1). The finding of lower molecular weight compounds being associated with more recent SPE-DOM is consistent with results from an assessment of marine SPE-DOM aging trends (Flerus et al., 2012). Previous studies of marine DOM size fractions have suggested decreasing molecular weight with increasing age (Kaiser and Benner, 2009; Benner and Amon, 2015). Yet, direct comparisons between SPE-DOM and studies using size-fractionation cannot be drawn due to the differences in sample preparation and resulting observed mass range.
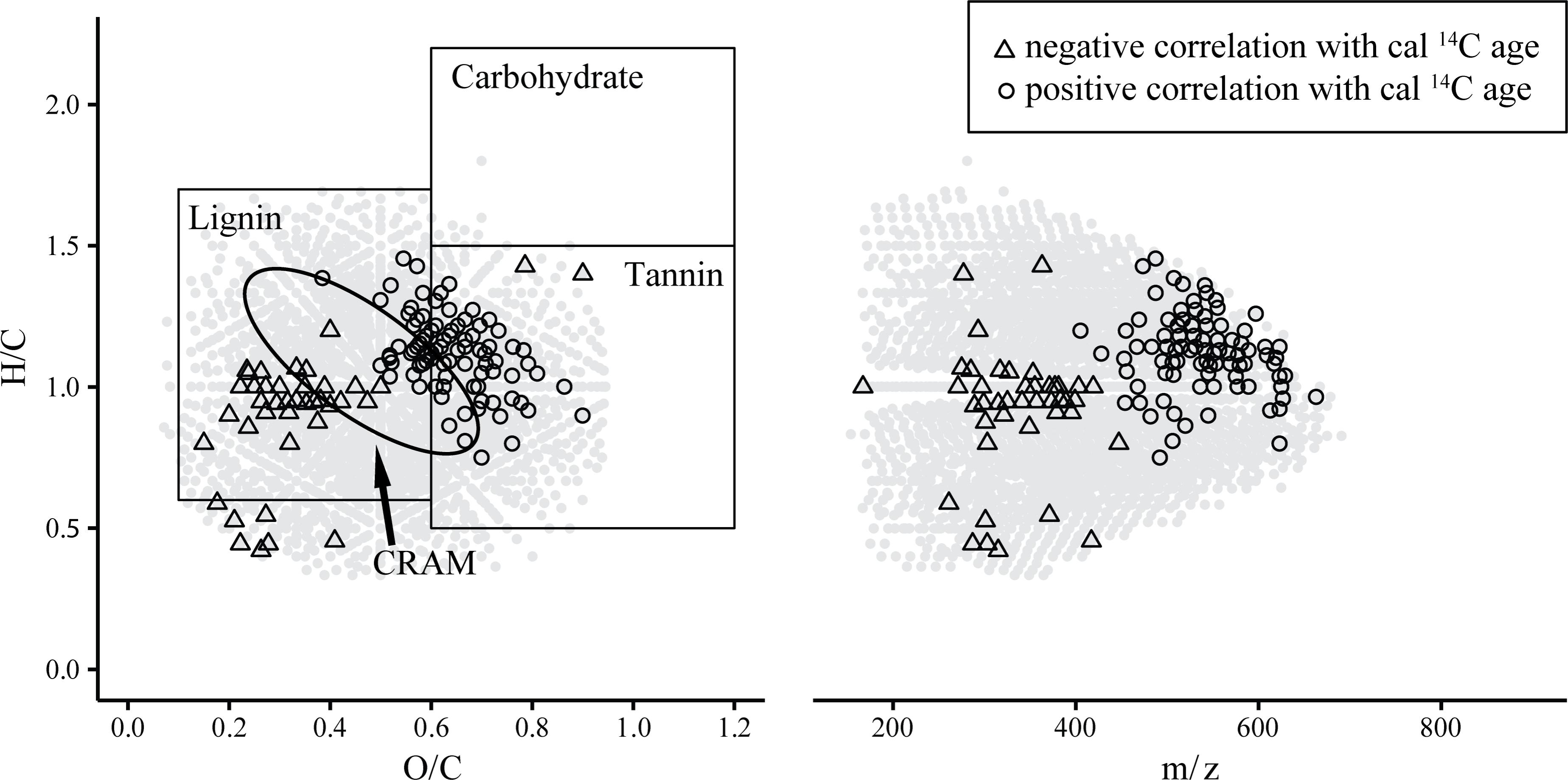
FIGURE 3. Van Krevelen and m/z plots highlighting sum formulae that were significantly (p < 0.05) positively or negatively correlated to cal 14C age. Circles in gray represent all sum formulae that were detected in at least 8 out of 10 samples. Structure suggestions in the van Krevelen diagram adapted from Antony et al. (2014).
In the forest environment one of the most prevalent sources of young carbon is plant litter. Consequently, lignin-derived molecules associated with the young carbon inputs are expected. In the van Krevelen diagram indeed many of the negatively correlated sum formulae can be estimated to represent lignin-type structures from their elemental composition [H/C: 0.6 – 1.7, O/C: 0.1 – 0.6; (Antony et al., 2014)]. A search within the ChEBI library revealed that the group of negatively 14C-age correlated formulae could possibly be mostly comprised of methoxylated phenylpropanoid-derivatives (Table 2 and Supplementary Tables S1, S2). Lignin dimers were prominent suggestions among flavonoids, coumarins and stilbenes, all of which result from the phenylpropanoid pathway (Vogt, 2010). This suggests that the group of negatively correlated compounds represents inputs of plant biomass derived molecules that feature recently assimilated carbon and are likely leaching from litter decay processes.
Positively 14C-age correlated sum formulae were more saturated and more oxygenated, their molecular weight was higher and in some cases they contained nitrogen or sulfur as heteroatoms (Table 2). From the elemental composition in the van Krevelen diagram the positively correlated sum formulae plot in the tannin-like area [H/C: 0.5 – 1.5, O/C: 0.6 – 1.2; (Antony et al., 2014)]. However, database search did not select tannin-type structures for positively correlated sum formulae even though tannin structures are annotated in the ChEBI library and in contrast structures of glycosylated phenylpropanoid derivatives were suggested (Supplementary Tables S1, S3). The tannin area in the van Krevelen diagram lies aside the lignin and carbohydrate areas [H/C: 1.5 – 2.2, O/C: 0.6 – 1.2; (Antony et al., 2014)] and consequently the structure suggestions are very likely (Figure 3).
The observed 14C-age related trends regarding the elemental composition and structure suggestions for the molecular entities differ from aging trends in marine DOM, likely due to largely different timescales of DOM aging. There, the composition converged toward carboxyl rich alicyclic molecules (CRAM) (Hertkorn et al., 2006; Lechtenfeld et al., 2014). Marker compounds related to marine DOM degradation state (Flerus et al., 2012) were entirely absent within the data set of this study.
From our assessment of the suggested structures of molecular entities (Supplementary Tables S2, S3) that were associated with 14C young and old carbon samples we identified that plant-derived phenylpropanoid compounds were core structural units for both groups, although it is probable that there are other structural units that may be suppressed during ionization (Nebbioso et al., 2010). Molecular entities associated with 14C-young or old DOM were characterized by a high degree of methoxylation and glycosylation, respectively. This finding is consistent with a previous investigation of plant-derived SOM (Gleixner et al., 2002).
While demethylation is a long known processes during lignin degradation (Jin and Kirk, 1990), it has recently been reported that glycosylation of phenylpropanoids greatly affects their bioavailability (Le Roy et al., 2016). In this study we cannot disentangle if the observed glycosylated phenylpropanoids remain from the original substrate or are synthesized in the environment. Yet, a combination of both processes is probable. Glycosylated phenylpropanoids are widely present in plants and glycosylated lignin oligomers can be stored in leaf vacuoles (Dima et al., 2015). Due to the increased solubility in water compared to their respective aglycones, the glycosides might be preferentially leached during biomass decomposition and accumulate in DOM over time. Furthermore, glycosylation of phenylpropanoids increases their stability by weakening their nucleophilic character as well as their tendency to polymerize. Because polymerization and reactions of phenylpropanoids with biomolecules are potentially harmful if they happen in cells, active glycosylation of these compounds could be a way for organisms to tolerate elevated levels of otherwise toxic lignin-derived molecules. This detoxification effect and the solubility increase are especially crucial for lignin decomposers since degradation products resulting from the activity of extracellular enzymes must be taken up by the mycelium (Jeffries, 1994; Sanchez, 2009).
The introduction of 14C young molecules derived from lignin degradation, their detoxification and stabilization can provide a framework for 14C age related marker compounds. The differing evolution of individual molecular entities over time is highlighted in the relative intensity distributions of two possible ferulic acid derivatives (Figure 4). Their final identification needs of course further structural investigation. However, the preliminary identification of C20H20O7 and C22H30O14 is likely as ferulic acid is a key component of lignocellulose (de Oliveira et al., 2015). On the one hand glycosmisic acid, a lignin dimer comprised of a ferulic acid and a coniferyl alcohol substructure showed linearly decreasing relative intensities with respect to increasing age of the samples, suggesting continuous degradation. On the other hand ferulic acid in its form as a disaccharide showed strongly increasing relative intensities. From not being detected in two samples with zero and one year of cal 14C-age, the ferulic acid disaccharide entity built up to stable levels within ∼10 years. The observed build-up of persisting lignin derivatives supports recent advances in dissolved lignin analysis, suggesting lability-based pools of lignin-derived molecules (Feng et al., 2017).
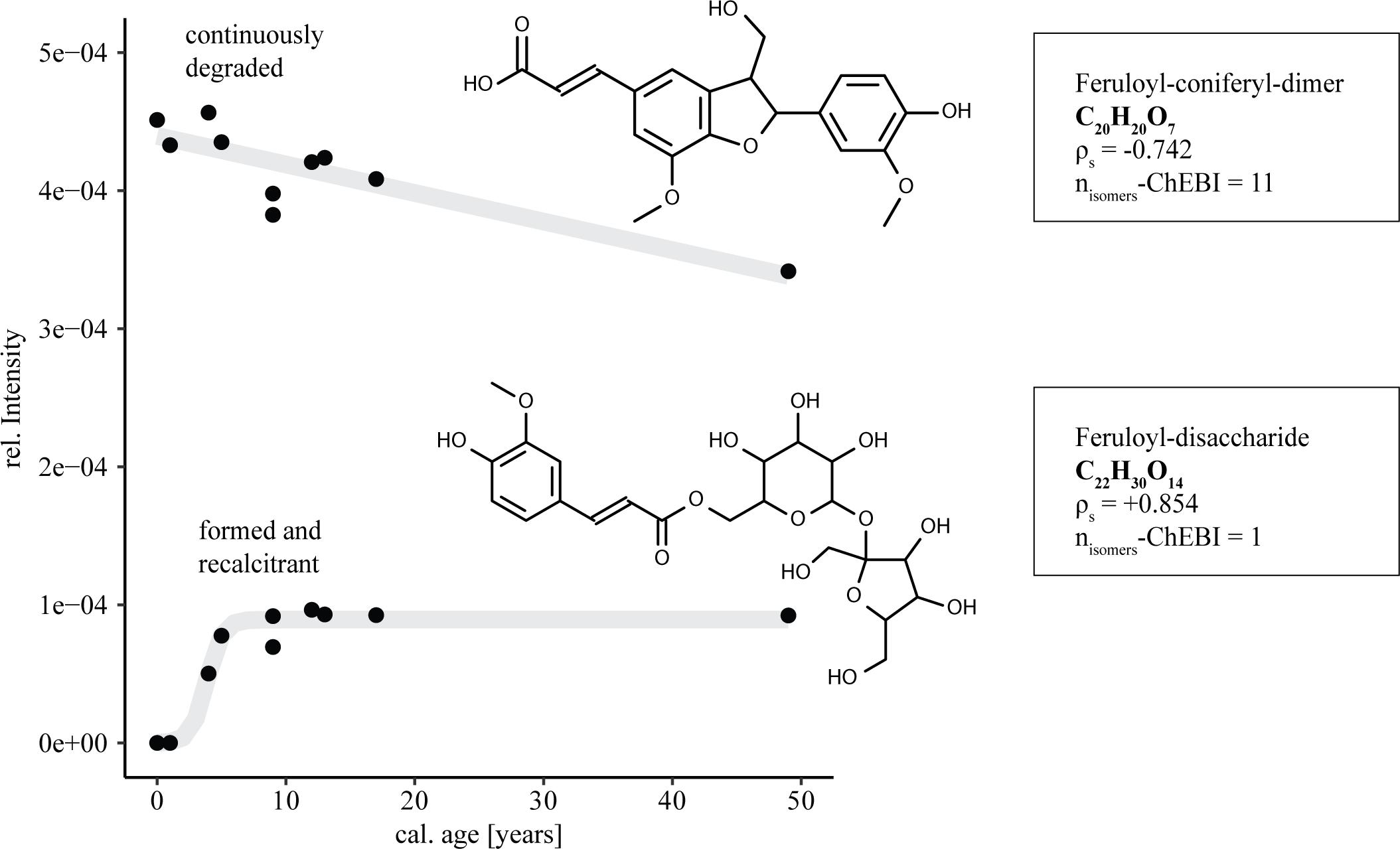
FIGURE 4. Effect of DOM age on FT-ICR-MS relative intensity distributions of ferulic acid derivatives. Trend lines are given in gray for visual support (top: linear function, bottom: logistic function). ρs: spearman correlation coefficient; nisomers-ChEBI: number of suggested structural isomers for the respective sum formula.
The decrease in the relative intensities of the feruloyl-coniferyl-dimer highlights the potential usability of lignin dimer molecules as fast flow markers for 14C young, surface-derived DOM inputs in the CZ. High abundances of this new marker in ground water could indicate fast transport of water and DOM through the subsurface. As aromatic organic matter is known to interact with mineral surfaces, the potential applicability of the proposed molecular markers in groundwater will depend on the mineral structure of the respective subsurface environment (Klotzbücher et al., 2016). Recent investigations show that a considerable proportion of lignin-derived phenols might be irreversibly sorbed to minerals (Kaiser and Guggenberger, 2000; Hernes et al., 2013), thereby limiting the potential traceability of lignin-derived markers into groundwater. Low pH conditions, as are present in sandy forest soils, can further favor strong OM sorption to mineral surfaces (Kleber et al., 2015). Therefore further studies focusing on subsurface DOM are required in order to decide which of the proposed potential marker compounds are applicable in the respective pedologic settings.
Conclusion
14C age is a strong driver of DOM composition in the CZ. 14C-young DOM molecules linking to inputs from decaying plant biomass could be identified by correlating individual sum formulae to 14C ages of SPE-DOM. Since this approach was applied only on water from forest top soils, it is important to expand this method to other CZ compartments. Groundwater DOM studies are needed to verify the applicability of lignin derived molecules as potential marker compounds for fast DOM transport.
We propose lignin derived molecules as potential markers for 14C young DOM in the CZ as a result of their prominence in chemical database suggestions for highly 14C age correlated sum formulae and their direct link to the forest environment. Still, they represent only a fraction of all 14C correlated DOM compounds in our study. In general, chemical transformation of phenylpropanoids so far seems to be an underestimated factor when considering aging and transport of terrestrial DOM. Their bioavailability and detoxification in the environment emerged as a potential key driver in early DOM evolution. However, further research using multidimensional mass spectrometric experiments is needed to verify the molecular identifications based on our database searches (e.g., Ball and Aluwihare, 2014). Further recent methodological advances such as liquid chromatography coupling (Hawkes et al., 2018) and ion fragmentation (Osterholz et al., 2015; Zark and Dittmar, 2018) have been successfully applied in DOM research and can be applied in context to this study. Possible follow-up studies using targeted approaches could be designed similarly to recent investigations of root exudates with UPLC/ESI-QTOFMS, which also detected C20H20O7, the sum formula of the feruloyl-coniferyl-dimer highlighted in Figure 4 (Strehmel et al., 2014).
Overall, our findings support recent advances in biomarker research, proposing degradability of lignin derived molecules and using resulting leachates as marker compounds. Applying our methodology in groundwater and tracing the already identified potential markers will further improve our knowledge on transport and evolution of DOM in the subsurface.
Author Contributions
V-NR and GG conceived the study idea. SB managed the samples and analyses and wrote the manuscript. SB and YL analyzed the data. SB, YL, V-NR, and GG interpreted the data and improved the manuscript.
Funding
YL and V-NR received financial support by the Zwillenberg-Tietz foundation and the German research foundation funded Collaborative Research Centre SFB 1076 AquaDiva. SB acknowledges the IMPRS-gBGC for funding.
Conflict of Interest Statement
The authors declare that the research was conducted in the absence of any commercial or financial relationships that could be construed as a potential conflict of interest.
Acknowledgments
We thank the radiocarbon group of A. Steinhof and the FT-ICR-MS group of T. Dittmar for measurement cooperations. We also thank the reviewers for their helpful comments which greatly improved this manuscript.
Supplementary Material
The Supplementary Material for this article can be found online at: https://www.frontiersin.org/articles/10.3389/feart.2018.00168/full#supplementary-material
References
Akin, D. E., and Benner, R. (1988). Degradation of polysaccharides and lignin by ruminal bacteria and fungi. Appl. Environ. Microbiol. 54, 1117–1125.
Antony, R., Grannas, A. M., Willoughby, A. S., Sleighter, R. L., Thamban, M., and Hatcher, P. G. (2014). Origin and sources of dissolved organic matter in snow on the East Antarctic ice sheet. Environ. Sci. Technol. 48, 6151–6159. doi: 10.1021/es405246a
Arakawa, N., and Aluwihare, L. (2015). Direct identification of diverse alicyclic terpenoids in Suwannee River Fulvic Acid. Environ. Sci. Technol. 49, 4097–4105. doi: 10.1021/es5055176
Ball, G. I., and Aluwihare, L. I. (2014). CuO-oxidized dissolved organic matter (DOM) investigated with comprehensive two dimensional gas chromatography-time of flight-mass spectrometry (GC × GC-TOF-MS). Org. Geochem. 75, 87–98. doi: 10.1016/j.orggeochem.2014.06.010
Benner, R., and Amon, R. M. (2015). The size-reactivity continuum of major bioelements in the ocean. Ann. Rev. Mar. Sci. 7, 185–205. doi: 10.1146/annurev-marine-010213-135126
Bronk Ramsey, C. (2009). Bayesian analysis of radiocarbon dates. Radiocarbon 51, 337–360. doi: 10.1017/S0033822200033865
de Oliveira, D. M., Finger-Teixeira, A., Mota, T. R., Salvador, V. H., Moreira-Vilar, F. C., Molinari, H. B., et al. (2015). Ferulic acid: a key component in grass lignocellulose recalcitrance to hydrolysis. Plant Biotechnol. J. 13, 1224–1232. doi: 10.1111/pbi.12292
Degtyarenko, K., De Matos, P., Ennis, M., Hastings, J., Zbinden, M., Mcnaught, A., et al. (2008). ChEBI: a database and ontology for chemical entities of biological interest. Nucleic Acids Res. 36, D344–D350.
Dima, O., Morreel, K., Vanholme, B., Kim, H., Ralph, J., and Boerjan, W. (2015). Small glycosylated lignin oligomers are stored in Arabidopsis leaf vacuoles. Plant Cell 27, 695–710. doi: 10.1105/tpc.114.134643
Dittmar, T., Koch, B. P., Hertkorn, N., and Kattner, G. (2008). A simple and efficient method for the solid-phase extraction of dissolved organic matter (SPE-DOM) from seawater. Limnol. Oceanogr. 6, 230–235. doi: 10.4319/lom.2008.6.230
Dubinenkov, I. V., Flerus, R., Schmitt-Kopplin, P., Kattner, G., and Koch, B. P. (2015). Origin-specific molecular signatures of dissolved organic matter in the Lena Delta. Biogeochemistry 123, 1–14. doi: 10.1007/s10533-014-0049-0
Feng, X., Gustafsson,Ö, Holmes, R. M., Vonk, J. E., Van Dongen, B. E., Semiletov, I. P., et al. (2015). Multimolecular tracers of terrestrial carbon transfer across the pan-Arctic:14C characteristics of sedimentary carbon components and their environmental controls. Glob. Biogeochem. Cycles 29, 1855–1873. doi: 10.1002/2015GB005204
Feng, X., Vonk, J. E., Griffin, C., Zimov, N., Montluçon, D. B., Wacker, L., et al. (2017). 14C Variation of dissolved lignin in Arctic River Systems. ACS Earth Space Chem. 1, 334–344. doi: 10.1021/acsearthspacechem.7b00055
Feng, X., Vonk, J. E., Van Dongen, B. E., Gustafsson,Ö, Semiletov, I. P., Dudarev, O. V., et al. (2013). Differential mobilization of terrestrial carbon pools in Eurasian Arctic river basins. Proc. Natl. Acad. Sci. U.S.A. 35, 14168–14173. doi: 10.1073/pnas.1307031110
Flerus, R., Lechtenfeld, O. J., Koch, B. P., Mccallister, S. L., Schmitt-Kopplin, P., Benner, R., et al. (2012). A molecular perspective on the ageing of marine dissolved organic matter. Biogeosciences 9, 1935–1955. doi: 10.5194/bg-9-1935-2012
Gabor, R. S., Eilers, K., Mcknight, D. M., Fierer, N., and Anderson, S. P. (2014). From the litter layer to the saprolite: chemical changes in water-soluble soil organic matter and their correlation to microbial community composition. Soil Biol. Biochem. 68, 166–176. doi: 10.1016/j.soilbio.2013.09.029
Gleixner, G., Poirier, N., Bol, R., and Balesdent, J. (2002). Molecular dynamics of organic matter in a cultivated soil. Org. Geochem. 33, 357–366. doi: 10.1016/j.aca.2013.09.031
Gleixner, G., Tefs, C., Albrecht, J., Hammer, M., Wirth, C., Nueske, A., et al. (2009). “Soil carbon accumulation in old-growth forests,” in Old-Growth Forests: Function, Fate and Value, ed. M. Heimann (Berlin: Springer), 231–266.
Hastings, J., Owen, G., Dekker, A., Ennis, M., Kale, N., Muthukrishnan, V., et al. (2016). ChEBI in 2016: improved services and an expanding collection of metabolites. Nucleic Acids Res. 44, D1214–D1219. doi: 10.1093/nar/gkv1031
Hawkes, J. A., Patriarca, C., Sjöberg, P. J. R., Tranvik, L. J., and Bergquist, J. (2018). Extreme isomeric complexity of dissolved organic matter found across aquatic environments. Limnol. Oceanogr. Lett. 3, 21–30. doi: 10.1002/lol2.10064
Hedges, J. I., Cowie, G. L., Ertel, J. R., Barbour, R. J., and Hatcher, P. G. (1985). Degradation of carbohydrates and lignins in buried woods. Geochim. Cosmochim. Acta 49, 701–711. doi: 10.1016/j.wasman.2007.11.006
Hernes, P. J., Kaiser, K., Dyda, R. Y., and Cerli, C. (2013). Molecular trickery in soil organic matter: hidden lignin. Environ. Sci. Technol. 47, 9077–9085. doi: 10.1021/es401019n
Hertkorn, N., Benner, R., Frommberger, M., Schmitt-Kopplin, P., Witt, M., Kaiser, K., et al. (2006). Characterization of a major refractory component of marine dissolved organic matter. Geochim. Cosmochim. Acta 70, 2990–3010. doi: 10.1016/j.gca.2006.03.021
Hertkorn, N., Frommberger, M., Witt, M., Koch, B. P., Schmitt-Kopplin, P., and Perdue, E. M. (2008). Natural organic matter and the event horizon of mass spectrometry. Anal. Chem. 80, 8908–8919. doi: 10.1021/ac800464g
Hertkorn, N., Harir, M., Koch, B. P., Michalke, B., and Schmitt-Kopplin, P. (2013). High-field NMR spectroscopy and FTICR mass spectrometry: powerful discovery tools for the molecular level characterization of marine dissolved organic matter. Biogeosciences 10, 1583–1624. doi: 10.5194/bg-10-1583-2013
Herzsprung, P., Von Tumpling, W., Hertkorn, N., Harir, M., Buttner, O., Bravidor, J., et al. (2012). Variations of DOM quality in inflows of a drinking water reservoir: linking of van Krevelen diagrams with EEMF spectra by rank correlation. Environ. Sci. Technol. 46, 5511–5518. doi: 10.1021/es300345c
Hua, Q., Barbetti, M., and Rakowski, A. Z. (2013). Atmospheric radiocarbon for the period 1950–2010. Radiocarbon 55, 2059–2072. doi: 10.2458/azu_js_rc.v55i2.16177
Jeffries, T. W. (1994). “Biodegradation of lignin and hemicelluloses,” in Biochemistry of Microbial Degradataion, ed. C. Ratledge (Dordrecht: Springer).
Jin, L., and Kirk, T. K. (1990). Mineralization of the methoxyl carbon of isolated lignin by brown-rot fungi under solid substrate conditions. Wood Sci. Technol. 24, 263–276. doi: 10.1007/BF01153559
Kaiser, K., and Benner, R. (2009). Biochemical composition and size distribution of organic matter at the Pacific and Atlantic time-series stations. Mar. Chem. 113, 63–77. doi: 10.1016/j.marchem.2008.12.004
Kaiser, K., and Guggenberger, G. (2000). The role of DOM sorption to mineral surfaces in the preservation of organic matter in soils. Org. Geochem. 31, 711–725. doi: 10.1016/S0146-6380(00)00046-2
Kaiser, K., Guggenberger, G., and Haumaier, L. (2004). Changes in dissolved lignin-derived phenols, neutral sugars, uronic acids, and amino sugars with depth in forested Haplic Arenosols and Rendzic Leptosols. Biogeochemistry 70, 135–151. doi: 10.1023/B:BIOG.0000049340.77963.18
Kaiser, K., and Kalbitz, K. (2012). Cycling downwards – dissolved organic matter in soils. Soil Biol. Biochem. 52, 29–32. doi: 10.1016/j.soilbio.2012.04.002
Kallenbach, C. M., Frey, S. D., and Grandy, A. S. (2016). Direct evidence for microbial-derived soil organic matter formation and its ecophysiological controls. Nat. Commun. 7:13630. doi: 10.1038/ncomms13630
Kanehisa, M., Furumichi, M., Tanabe, M., Sato, Y., and Morishima, K. (2017). KEGG: new perspectives on genomes, pathways, diseases and drugs. Nucleic Acids Res. 45, D353–D361. doi: 10.1093/nar/gkw1092
Kim, S., Thiessen, P. A., Bolton, E. E., Chen, J., Fu, G., Gindulyte, A., et al. (2016). PubChem substance and compound databases. Nucleic Acids Res. 44, D1202–D1213. doi: 10.1093/nar/gkv951
Kindler, R., Siemens, J., Kaiser, K., Walmsley, D. C., and Bernhofer, C. (2011). Dissolved carbon leaching from soils is a crucial component of the net ecosystem carbon balance. Glob. Change Biol. 17, 1167–1185. doi: 10.1111/j.1365-2486.2010.02282.x
Kleber, M., Eusterhues, K., Keiluweit, M., Mikutta, C., Mikutta, R., and Nico, P. S. (2015). “Mineral–organic associations: formation, properties, and relevance in soil environments,” in Advances in Agronomy, ed. D. L. Sparks (Amsterdam: Elsevier), 1–140.
Klotzbücher, T., Kalbitz, K., Cerli, C., Hernes, P. J., and Kaiser, K. (2016). Gone or just out of sight? The apparent disappearance of aromatic litter components in soils. Soil 2, 325–335. doi: 10.5194/soil-2-325-2016
Knohl, A., Schulze, E.-D., Kolle, O., and Buchmann, N. (2003). Large carbon uptake by an unmanaged 250-year-old deciduous forest in Central Germany. Agric. For. Meteorol. 118, 151–167. doi: 10.1016/S0168-1923(03)00115-1
Koch, B. P., and Dittmar, T. (2016). From mass to structure: an aromaticity index for high-resolution mass data of natural organic matter. Rapid Commun. Mass Spectrom. 30, 250–250. doi: 10.1002/rcm.7433
Kothawala, D. N., Ji, X., Laudon, H., Agren, A. M., Futter, M. N., Köhler, S. J., et al. (2015). The relative influence of land cover, hydrology, and in-stream processing on the composition of dissolved organic matter in boreal streams. J. Geophys. Res. Biogeosci. 120, 1491–1505. doi: 10.1002/2015JG002946
Kujawinski, E. B., Longnecker, K., Blough, N. V., Vecchio, R. D., Finlay, L., Kitner, J. B., et al. (2009). Identification of possible source markers in marine dissolved organic matter using ultrahigh resolution mass spectrometry. Geochim. Cosmochim. Acta 73, 4384–4399. doi: 10.1016/j.gca.2009.04.033
Küsel, K., Totsche, K. U., Trumbore, S. E., Lehmann, R., Steinhäuser, C., and Herrmann, M. (2016). How deep can surface signals be traced in the critical zone? Merging biodiversity with biogeochemistry research in a central german muschelkalk landscape. Front. Earth Sci. 4:32. doi: 10.3389/feart.2016.00032
Le Roy, J., Huss, B., Creach, A., Hawkins, S., and Neutelings, G. (2016). Glycosylation is a major regulator of phenylpropanoid availability and biological activity in plants. Front. Plant Sci. 7:735. doi: 10.3389/fpls.2016.00735
Lechtenfeld, O. J., Kattner, G., Flerus, R., Mccallister, S. L., Schmitt-Kopplin, P., and Koch, B. P. (2014). Molecular transformation and degradation of refractory dissolved organic matter in the Atlantic and Southern Ocean. Geochim. Cosmochim. Acta 126, 321–337. doi: 10.1016/j.gca.2013.11.009
McIntyre, C., and McRae, C. (2005). Proposed guidelines for sample preparation and ESI-MS analysis of humic substances to avoid self-esterification. Org. Geochem. 36, 543–553. doi: 10.1016/j.orggeochem.2004.11.002
Melillo, J. M., Aber, J. D., and Muratore, J. F. (1982). Nitrogen and lignin control of hardwood leaf litter decomposition dynamics. Ecology 63, 621–626. doi: 10.1007/BF00378913
Miller, M. P., Boyer, E. W., Mcknight, D. M., Brown, M. G., Gabor, R. S., Hunsaker, C. T., et al. (2016). Variation of organic matter quantity and quality in streams at Critical Zone Observatory watersheds. Water Resour. Res. 52, 8202–8216. doi: 10.1002/2016WR018970
Moran, M. A., Kujawinski, E. B., Stubbins, A., Fatland, R., Aluwihare, L. I., Buchan, A., et al. (2016). Deciphering ocean carbon in a changing world. Proc. Natl. Acad. Sci. U.S.A. 113, 3143–3151. doi: 10.1073/pnas.1514645113
Moyano, F. E., Kutsch, W. L., and Rebmann, C. (2008). Soil respiration fluxes in relation to photosynthetic activity in broad-leaf and needle-leaf forest stands. Agric. For. Meteorol. 148, 135–143. doi: 10.1016/j.agrformet.2007.09.006
Nebbioso, A., and Piccolo, A. (2013). Molecular characterization of dissolved organic matter (DOM): a critical review. Anal. Bioanal. Chem. 405, 109–124. doi: 10.1007/s00216-012-6363-2
Nebbioso, A., Piccolo, A., and Spiteller, M. (2010). Limitations of electrospray ionization in the analysis of a heterogeneous mixture of naturally occurring hydrophilic and hydrophobic compounds. Rapid Commun. Mass Spectrom. 24, 3163–3170. doi: 10.1002/rcm.4749
Osterholz, H., Niggemann, J., Giebel, H. A., Simon, M., and Dittmar, T. (2015). Inefficient microbial production of refractory dissolved organic matter in the ocean. Nat. Commun. 6:7422. doi: 10.1038/ncomms8422
Reimer, P. J., Brown, T. A., and Reimer, R. W. (2004). Discussion: reporting and calibration of post-bomb 14C data. Radiocarbon 46, 1299–1304. doi: 10.1017/S0033822200033154
Riedel, T., Biester, H., and Dittmar, T. (2012). Molecular fractionation of dissolved organic matter with metal salts. Environ. Sci. Technol. 46, 4419–4426. doi: 10.1021/es203901u
Roth, V.-N., Dittmar, T., Gaupp, R., and Gleixner, G. (2014). Ecosystem-specific composition of dissolved organic matter. Vadose Zone J. 13:vzj2013.09.0162. doi: 10.2136/vzj2013.09.0162
Roth, V. N., Dittmar, T., Gaupp, R., and Gleixner, G. (2015). The molecular composition of dissolved organic matter in forest soils as a function of pH and temperature. PLoS One 10:e0119188. doi: 10.1371/journal.pone.0119188
Sachse, D., Kahmen, A., and Gleixner, G. (2009). Significant seasonal variation in the hydrogen isotopic composition of leaf-wax lipids for two deciduous tree ecosystems (Fagus sylvativa and Acerpseudoplatanus). Org. Geochem. 40, 732–742. doi: 10.1016/j.orggeochem.2009.02.008
Sanchez, C. (2009). Lignocellulosic residues: biodegradation and bioconversion by fungi. Biotechnol. Adv. 27, 185–194. doi: 10.1016/j.biotechadv.2008.11.001
Schmidt, M. W., Torn, M. S., Abiven, S., Dittmar, T., Guggenberger, G., Janssens, I. A., et al. (2011). Persistence of soil organic matter as an ecosystem property. Nature 478, 49–56. doi: 10.1038/nature10386
Schulze, W. X., Gleixner, G., Kaiser, K., Guggenberger, G., Mann, M., and Schulze, E. D. (2005). A proteomic fingerprint of dissolved organic carbon and of soil particles. Oecologia 142, 335–343. doi: 10.1007/s00442-004-1698-9
Seifert, A. G., Roth, V. N., Dittmar, T., Gleixner, G., Breuer, L., Houska, T., et al. (2016). Comparing molecular composition of dissolved organic matter in soil and stream water: Influence of land use and chemical characteristics. Sci. Total Environ. 571, 142–152. doi: 10.1016/j.scitotenv.2016.07.033
Steinhof, A. (2013). Data analysis at the jena 14C laboratory. Radiocarbon 55, 282–293. doi: 10.1017/S0033822200057386
Steinhof, A., Adamiec, G., Gleixner, G., Van Klinken, G. J., and Wagner, T. (2004). The new 14C analysis laboratory in jena. Germany. Radiocarbon 46, 51–58. doi: 10.1017/S0033822200039345
Steinhof, A., Hejja, I., and Wagner, T. (2010). Improvements of the Jena AMS system. Nucl. Instrum. Methods Phys. Res. B 268, 902–905. doi: 10.1016/j.nimb.2009.10.060
Strehmel, N., Bottcher, C., Schmidt, S., and Scheel, D. (2014). Profiling of secondary metabolites in root exudates of Arabidopsis thaliana. Phytochemistry 108, 35–46. doi: 10.1016/j.phytochem.2014.10.003
Tefs, C., and Gleixner, G. (2012). Importance of root derived carbon for soil organic matter storage in a temperate old-growth beech forest – Evidence from C, N and 14C content. For. Ecol. Manage. 263, 131–137. doi: 10.1016/j.foreco.2011.09.010
Thomson, R. H. (1993). The Chemistry of Natural Products. Berlin: Springer Science & Business Media. doi: 10.1007/978-94-011-2144-6
Waggoner, D. C., Wozniak, A. S., Cory, R. M., and Hatcher, P. G. (2017). The role of reactive oxygen species in the degradation of lignin derived dissolved organic matter. Geochim. Cosmochim. Acta 208, 171–184. doi: 10.1016/j.gca.2017.03.036
Ward, N. D., Keil, R. G., Medeiros, P. M., Brito, D. C., Cunha, A. C., Dittmar, T., et al. (2013). Degradation of terrestrially derived macromolecules in the Amazon River. Nat. Geosci. 6, 530–533. doi: 10.1038/ngeo1817
Wickland, K. P., Neff, J. C., and Aiken, G. R. (2007). Dissolved organic carbon in alaskan boreal forest: sources, chemical characteristics, and biodegradability. Ecosystems 10, 1323–1340. doi: 10.1007/s10021-007-9101-4
Zark, M., Christoffers, J., and Dittmar, T. (2017). Molecular properties of deep-sea dissolved organic matter are predictable by the central limit theorem: evidence from tandem FT-ICR-MS. Mar. Chem. 191, 9–15. doi: 10.1016/j.marchem.2017.02.005
Keywords: FT-ICR-MS, radiocarbon, lignin, markers, carbon cycle
Citation: Benk SA, Li Y, Roth V-N and Gleixner G (2018) Lignin Dimers as Potential Markers for 14C-young Terrestrial Dissolved Organic Matter in the Critical Zone. Front. Earth Sci. 6:168. doi: 10.3389/feart.2018.00168
Received: 31 July 2018; Accepted: 28 September 2018;
Published: 16 October 2018.
Edited by:
Francien Peterse, Utrecht University, NetherlandsReviewed by:
Xiaojuan Feng, Institute of Botany (CAS), ChinaAllison Myers-Pigg, Memorial University of Newfoundland, Canada
Philippa Louise Ascough, University of Glasgow, United Kingdom
Copyright © 2018 Benk, Li, Roth and Gleixner. This is an open-access article distributed under the terms of the Creative Commons Attribution License (CC BY). The use, distribution or reproduction in other forums is permitted, provided the original author(s) and the copyright owner(s) are credited and that the original publication in this journal is cited, in accordance with accepted academic practice. No use, distribution or reproduction is permitted which does not comply with these terms.
*Correspondence: Gerd Gleixner, gerd.gleixner@bgc-jena.mpg.de