- 1Institute of Geography, Russian Academy of Sciences, Moscow, Russia
- 2V.S. Sobolev Institute of Geology and Mineralogy, Siberian Branch of the Russian Academy of Sciences, Novosibirsk, Russia
Bottom sediments of the proglacial Lake Donguz-Orun situated at ∼2500 m a.s.l. in the Elbrus Region (Central Caucasus) reveal regular laminae, characteristic of proglacial varved lakes. This is the first laminated sediment sequence recorded in the region. However, visual counting of the layers was restricted due to partial indistinctness of the lamination. In order to confirm the annual sedimentary cyclicity and proceed with annually resolved data, in addition to the visual identification we used high-resolution geochemical markers. The upper 160 mm of the sediment core were scanned at 200 μm intervals using synchrotron radiation X-ray fluorescence analysis (SR-XRF). Additional ultrahigh resolution scanning at 30 μm increments was employed for the upper 20 mm of the core. The Rb/Sr and Zr/Rb ratios are interpreted to record annual changes in grain-size. Based on this geochemical assessment, we identified 88 annual layers covering the interval between 1922 and 2010, while visually we have been able to identify between 70 and 100 layers. The correctness of the geochemical results is confirmed by mean accumulation rates assessed by 137Cs and 210Pb dating. Cross-correlation between the ring width of local pine chronology and the layer thickness, identified as a distance between the annual Rb/Sr peaks, allowed for the accurate dating of the uppermost preserved year of the sediment sequence (AD 2010). Annually averaged elemental data were then compared with regional meteorological observations, glacier mass balance and tree-ring chronologies. The comparison revealed notable conformities: content of bromine is positively correlated with annual temperatures (r = 0.41, p < 0.01), content of terrigenous elements (major elements with the origin in watershed rocks) is positively correlated (up to r = 0.44, p < 0.01) with annual precipitation. A high statistically significant negative relationship is observed between the concentrations of terrigenous elements and tree-ring width of local pine chronology (up to r = -0.56, p < 0.01). Taken together, these data point to a common composite climatic signal in the two independent records (lake sediments and tree rings) and confirm that the laminae represent annual layers (i.e., varves). These findings open opportunities for high-resolution multiproxy climate reconstructions 300–350 years long using the longer sediment core and tree-ring records.
Introduction
Background
Documenting environmental changes at various scales and deciphering their causes and consequences is one of the urgent challenges of current research in geosciences (IPCC, 2013). Addressing the role of the anthropogenic factor in global change can only be done using paleoreconstructions based on natural archives, which allow the evaluation of variability prior to significant human interference. Since the record of instrumental meteorological observations covers only a short time period, reliable regional climate reconstructions calibrated against available instrumental records are vital for assessing the magnitude of regional climate changes compared to the natural “baseline” and for testing and tuning prognostic climate models.
Past environmental changes in the Caucasus, a mountainous border region between Europe and Asia stretching from the Caspian to the Black Sea, remain poorly documented, especially at a high temporal resolution (Solomina et al., 2016 and references therein). The total area of this mountainous region is ∼440 thousand km2 and the range of sharply differing environments is extraordinary. According to Gulisashvili et al. (1975) the Caucasus is comprised of 7 zones and 14 regional altitudinal sequences ranging from the subtropical to the alpine. Paleoclimatic and paleoecological studies of the Caucasus region are scarce, offering only isolated evidence of individual past phenomena. Assessing the overall regional picture of environmental change remains problematic due to insufficient expertise, especially the lack of high resolution reconstructions (see Solomina et al., 2016 for a review of the subject). Only the larger general tendencies of glacier variations and the history of climate such as the Little Ice Age and the Medieval Climatic Anomaly are identified. Tree ring records spanning the most recent four and a half centuries yield the only available high-resolution reconstruction of temperature in the Caucasus (Dolgova and Solomina, 2010; Dolgova, 2016). Long-term temperature and precipitation trends over the Holocene have been assessed from lake sediment and peat bog pollen and diatom analysis (Serebryanny et al., 1984). Beech pollen proportion was employed as an indicator by Knyazev et al. (1992), who created a paleoclimatic reconstruction for the second half of the Holocene, finding general warm periods, 7500–5500 and 5000–3100 years BP, and cold periods, 5500–5000, 4400–4000, 3700–3400, and 3000–2300 years BP. Based on pollen analyses in the Western Caucasus, Kvavadze and Efremov (1996) suggested two more recent shorter cold periods: 1300–1200 and 400–350 years BP. Variability in lake chemistry has been addressed in the studies by Moiseenko et al. (2012). These reconstructions are mostly qualitative rather than quantitative – mostly due to the lack of conventional analytical methods at the time.
Lake sediments in the Caucasus potentially offer a detailed picture of environmental changes in the region over Late Pleistocene – Holocene, i.e., a much longer time interval than tree-ring proxies can supply. According to Efremov (1984), there are around 1600 lakes in the Caucasus. Lake sediments have long been utilized as a source of multiproxy paleoenvironmental data (e.g., Karlén, 1976, 1981; Leonard, 1986; Leemann and Niessen, 1994; Matthews et al., 2000; Nesje et al., 2001; Bakke et al., 2005; Osborn et al., 2007), providing long continuous records of climatic and biogeochemical changes. The most valuable source of paleoclimatic information of high temporal resolution are annually laminated lake sediments – varves (Zolitschka et al., 2015).
In order to recover proxies of environmental changes recorded in the sediments of Lake Donguz-Orun a synchrotron-based scanning X-ray fluorescence analysis was used. Although we have previously reported some preliminary findings in a Russian journal in the format of a brief communication (Darin et al., 2015), a comprehensive account of the results and data analysis is given here for the first time. Prior to this work no annually laminated lake sediments have been reported for the Caucasus region.
The goal of this study is to assess the potential of the sediments of Lake Donguz-Orun for high resolution environmental reconstructions. Precisely, that means following two major hypotheses: first – that the laminae of the bottom sediment of the lake indeed represent annual layers, i.e., varves; second – that the sediment is capable of recording the information of past climatic and environmental change.
To fulfill this task, it was necessary:
• To identify the sediment accumulation rate using geochemical markers and radioisotopic dating;
• To analyze the reliability of annual boundaries identified visually and by means of geochemical data;
• To build an annually resolved chronology of geochemical characteristics of Donguz-Orun lake sediments, to compare it with meteorological, mass balance, and tree ring records and identify the markers that can be interpreted in terms of environmental changes.
Study Site
Lake Donguz-Orun (43°13′26″N; 42°29′35″E) is located within the Central Caucasus at an elevation of 2545 m (Figure 1). The length of the lake is ∼650 m, the width – ∼220 m, the surface area is ∼55,000 m2. The average depth of the lake is 4.5 m with a maximum depth reaching ∼15 m. The lake has been subject to minimal, if any direct anthropogenic modification.
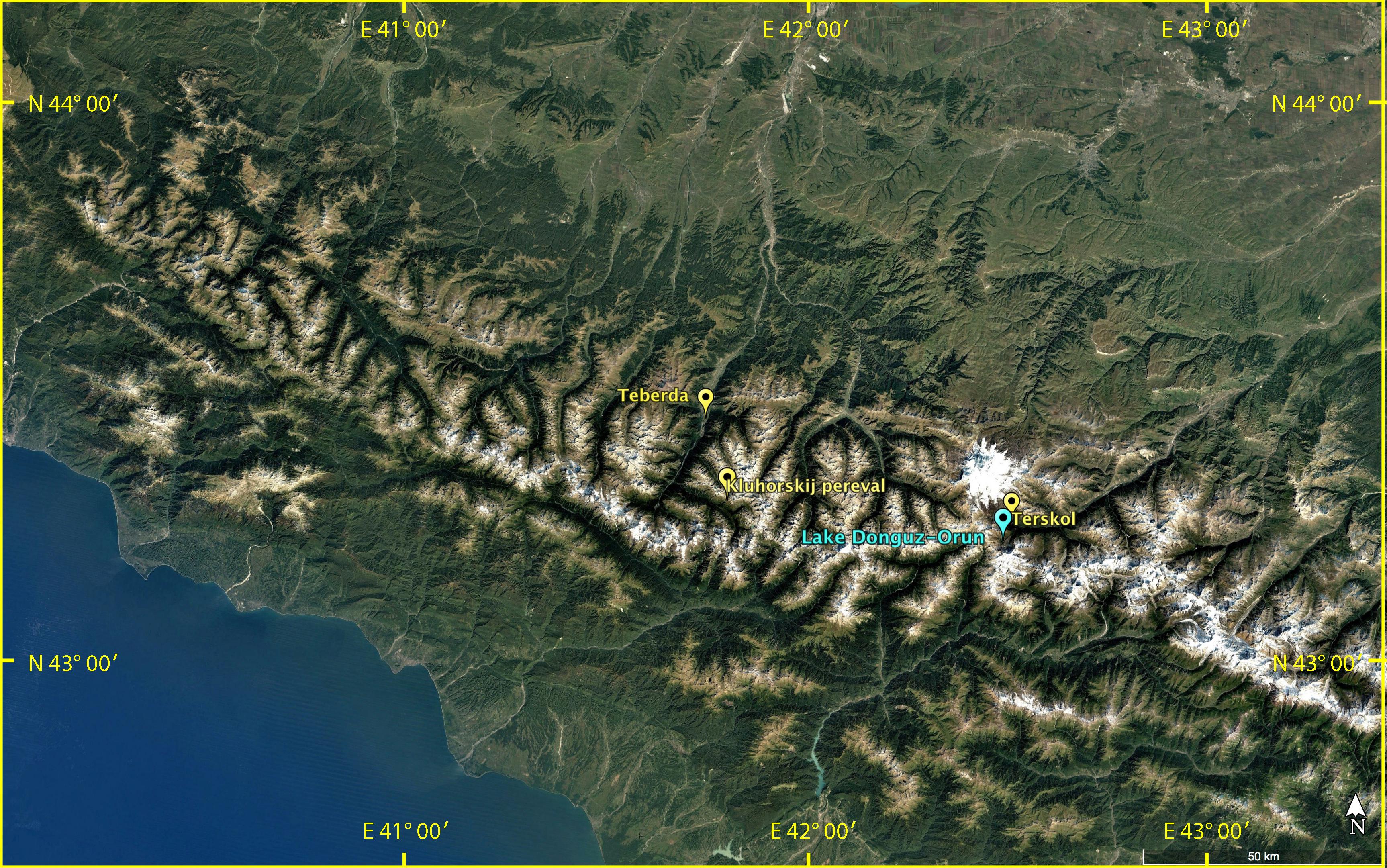
FIGURE 1. The Caucasus, location of the Lake Donguz-Orun. Blue mark shows the lake, yellow marks show the featured meteorological stations.
Lake Donguz-Orun has a complex catchment area encompassing several glaciers. The lake was formed as a result of damming by a lateral moraine of Donguz-Orun Glacier (Figure 2). The minimum age of the damming moraine is 350 years old according to the annual ring counting of the juniper growing on this surface. The Rhizocarpon geographicum sensu lato reaching here the diameter up to 105–114 mm indicates an older age (up to 700 years), although the growth rate for lichens in the Caucasus is still not yet well established (Solomina et al., 2016). The Donguz-Orun Glacier area is 2.79 km2 (GLIMS database). The glacier is quite unusual: it is fed mostly by the avalanches and its tongue is covered by a continuous cover of a surface moraine. This may be the cause of its limited retreat over the past decades compared to the other glaciers of this region. The lake is fed by several streams that flow from several small glaciers. Part of the runoff probably enters the lake via infiltration through the moraine of Donguz-Orun Glacier, although its influence can be considered negligible. In addition to the outflow from several small glaciers, the lake is also fed by the water supplied by precipitation, surface wash-off, and by the avalanche snow from the slopes. The outflow from the lake forms the Donguz-Orun River (Figure 2).
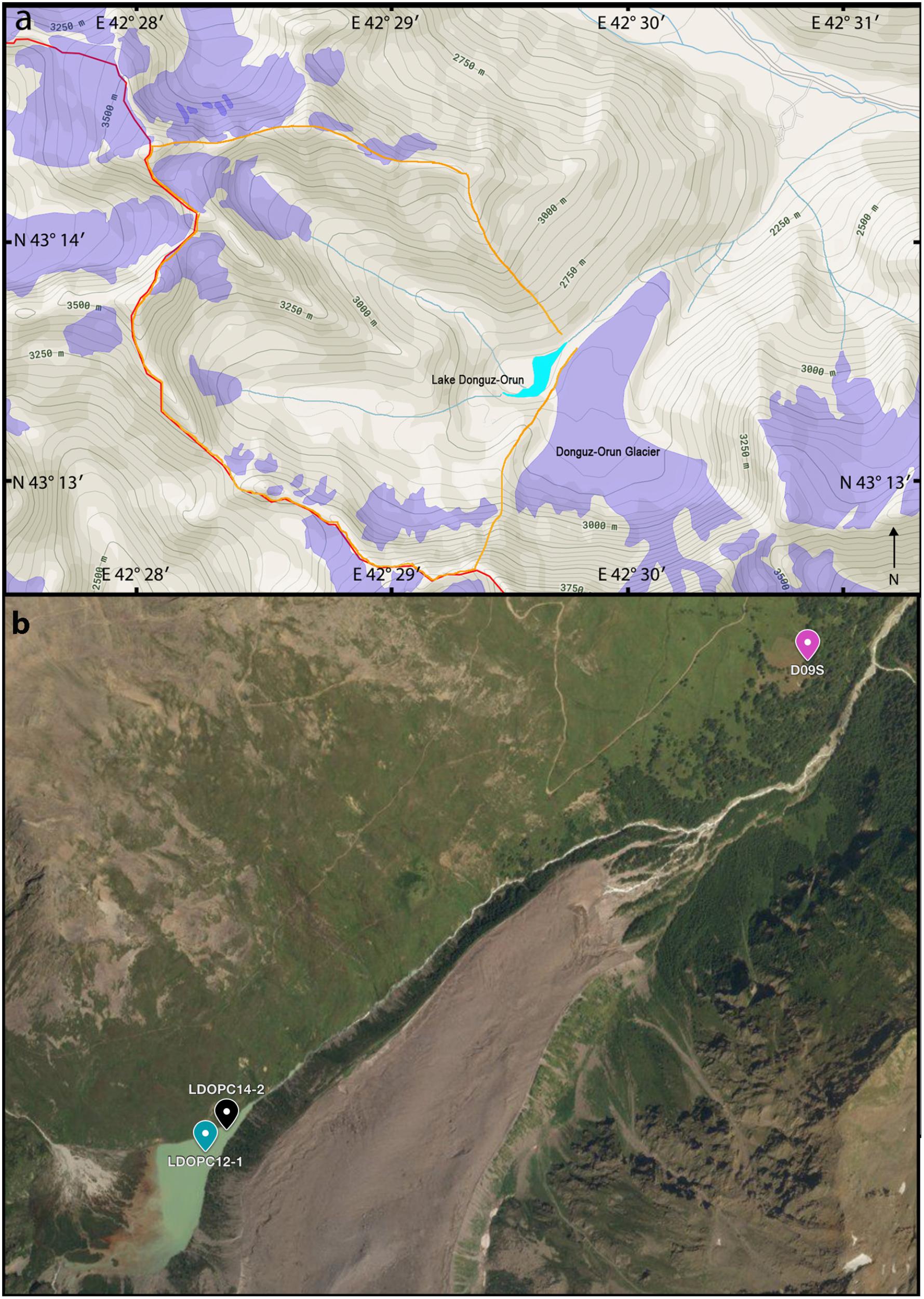
FIGURE 2. (a) Schematic layout of the lake setting. Translucent purple outlines mark glaciers according to GLIMS database; orange line indicates lake’s catchment area, red line marks administrative border; (b) Aerial view of Lake and Glacier Donguz-Orun. The pins indicate coring sites and location of the tree ring site D09S. Image courtesy: Bing/DigitalGlobe.
The Main Caucasus Ridge, situated directly south of Lake Donguz-Orun, serves a boundary between the temperate zone and the subtropics, the climate of the region is characterized by distinct seasonality in temperature. In summer, the Caucasus is dominated by subtropical high in the west and Asian depression in the east. In winter, atmospheric circulation is dominated by western extension of Siberian high in the east and Icelandic depression in the west. Influence of the Black Sea, as well as the orographic effects, contribute to the complex climatic picture of the region. The higher elevations are predominantly affected by the westerly flow (Shahgedanova, 2002).
Meteorological observations in the region have a relatively short time span. The longest at our disposal – the station of Teberda offers observations since 1927. It is situated at an altitude of 1330 m a.s.l., at the bottom of the valley of the Teberda River – in a different environment compared to the glaciolacustrine setting of Lake Donguz-Orun. The other two stations – Terskol and Kluhorskij Pereval present data starting from 1951 and 1959, respectively1 (Figure 1). The proximal meteorological station of Terskol (4 km north of Lake Donguz-Orun) is situated at 2141 m a.s.l., apparently offering the most representative source. For the observed period the temperature values recorded at Terskol station are as follows: mean annual temperature: 2.6°C; mean summer temperature (JJA): 11.4°C, mean winter temperature (DJF): -6.3°C. Long-term averages of annual temperatures exhibit a slight decline throughout the period 1951–2010, with a growth starting from 1963 to 2001 onward. Precipitation maxima occur in July–September. The yearly averaged annual precipitation value is 948 mm with monthly sums ranging from 57 mm in January to 100 mm in July. Strong interannual variability in precipitation is observed in all seasons, however, long-term changes in precipitation recorded at Terskol station are not that pronounced. Annual precipitation recorded at Teberda station reveals a consistent positive linear trend accountable for 26% of the variance.
Most of the clastic material is supplied to Lake Donguz-Orun during the season of peak glacier meltwater runoff. At the study site, peak meltwater runoff occurs in June-August (Shahgedanova et al., 2007). The catchment area of Lake Donguz-Orun consists of Precambrian and Lower Paleozoic gneiss and crystalline schist. The prevailing part of the catchment area is comprised of non-vegetated sloping rock surfaces. As a result, the majority of the material entering the lake is terrigenous clastics. Minor amounts of organic material are transported to the lake through downwash of the catchment area by precipitation and meltwater. Biological productivity in the lake is restricted due to low temperatures and bioturbation of the sediment is not observed.
Materials and Methods
Sediment Cores
The first sediment cores were obtained at Lake Donguz-Orun in the summer of 2012. A modified piston corer (Nesje, 1992) was employed for coring. The corer was mounted on an inflatable catamaran on a specially prepared platform. Coring was carried out in the central deepest part of the lake at the depth of 12 m (Figure 2). The coring process showed that the sediment penetrability is very limited, and the longest core that was taken – core LDOPC12-1 – was only 260 mm long. In 2014 additional sediment coring extracted a substantially longer core – LDOPC14-2 (580 mm long) from near the outflow at a water depth of 10.5 m (Figure 2). This paper focuses solely on the core LDOPC12-1.
Core LDOPC12-1 was cut in half along the main axis and sampled as described below. Visual inspection revealed beige-brown clays with regular laminae. The laminae range from very to less distinct (Figure 3). Very high sediment density has led to downward warping of the sediment layers along the tube.
137Cs and 210Pb Isotope Analysis
Measurements of Pb-210 and Cs-137 (Appleby and Oldfield, 1978; Chen et al., 2008) often serve as an age control for young sediments. The maximum of Cs-137 activity corresponds to the period A.D. 1961–1963 associated with nuclear weapons testing. In addition, in some regions of Europe, there is also a peak in the activity of Cs-137 associated with the Chernobyl accident of A.D. 1986 (e.g., Tylmann et al., 2013). Measurements of the activity of Cs-137 and Pb-210 were performed at the V.S. Sobolev Institute of Geology and Mineralogy (Siberian Branch of the Russian Academy of Sciences) according to the procedures described in Bobrov et al. (1999). For this purpose, samples from the upper part of the core were taken within the depth 5–120 mm at 10 mm interval (the total of eight samples).
X-ray Fluorescence Element Analysis
X-ray fluorescence analysis using synchrotron radiation (SR-XRF) was performed for the upper 160 mm of the core LDOPC12-1 at the Siberian Center for Synchrotron Radiation (INP SB RAS, Novosibirsk). The synchrotron radiation facility here has long been used for multielement analysis of sediment core samples (e.g., Darin et al., 1991, 2005, 2013; Bobrov et al., 1999; Kalugin et al., 2007). One half of the 260-mm core LDOPC12-1 was used for subsampling of a block that was subsequently used for the scanning XRF analysis. The block was sampled using a specially prepared frame made of thin aluminum with dimensions 160 mm × 20 mm × 5 mm. The analysis was done on a wet block, the following elements were measured: K, Ca, Ti, V, Cr, Mn, Fe, Ni, Cu, Zn, Ga, As, Br, Rb, Sr, Y, Zr, Nb, Mo and the ratio of Compton (incoherent) and Rayleigh (coherent) scattering data (Co/Inc) with a 200-micron resolution.
Subsequently a thin section was prepared for ultra-high resolution analysis using the following steps (described in Darin et al., 2013): (1) installation of specially constructed aluminum frames on the surface of the sediment and their extraction from the core with an undamaged layer of sediment; (2) freezing of the obtained samples in liquid nitrogen and drying in a lyophilic chamber; (3) saturation of the block of sediment with a polymer – a mixture of epoxy and acetone. In order to accelerate the process of polymerization of epoxy resin, the samples were kept in an oven at 60°C for 3–4 days. The resulting blocks 160 mm × 20 mm × 5 mm can be stored for future use. For the scanning analysis 2-mm thick sections were cut out from each block and polished. In order to test the hypothesis that the laminae represent annual laminae (i.e., varves), a scanning XRF analysis using concentrating X-ray optics at an ultra-high resolution (30 μm) was obtained for the uppermost 20 mm following the procedures described in Darin and Rakshun (2013).
The SR-XRF procedure is costly and time-consuming. For the current project only one block corresponding to the upper 160 mm of the sediment was subject to the analysis. Given that the main objectives of this work are to confirm the annual pattern of lamination and reveal the potential of the sedimentary sequence to record climate signal, that 160 mm-section serves that purpose well. Complying with both hypotheses will imply further SR-XRF scanning of the core LDOPC14-2 (580 mm long).
Results
Elemental Analysis
Figure 4 shows the results of SR-XRF analysis carried out for the upper 160 mm of the core LDOPC12-1. Synchrotron radiation XRF analysis allows for the elemental values in parts-per-million (ppm), the values were then normalized using min-max normalization following formula where x is an original value in ppm, x′ is the normalized value. In order to extract and assess elemental groupings we applied principal component analysis (PCA) to the high resolution XRF data. PCA analysis serves as an important tool for distinguishing common signals within a complex dataset. We have applied a PCA on the dataset consisting of contents of 20 elements and Co/Inc ratio scanned at 200-μm resolution for the upper 160 mm of the core LDOPC12-1. Out of all the components, the first three explain more than 60% of total elemental variability: PC-1 – 41.8%, PC-2 – 10.1%, PC-3 – 7.2%. The results imply a rather strong signal described by PC-1 component. Figure 5 shows the primary component PC-1 plotted against components PC-2 and PC-3. It is evident that the terrigenous elements – Fe, Ti, Rb, Mn, Zn – cluster along the positive direction of PC-1, Co/Inc ratio and, to a lesser extent, Br make a strong contribution toward the negative direction of PC-1. Br also reveals a substantial contribution in components 2 and 3. PCA analysis of the elemental data for the upper 160 mm suggests a primary process governing the sedimentation described by PC-1, associated with increased content of terrigenous elements/decreased content of Br and Co/Inc ratio and vice versa.
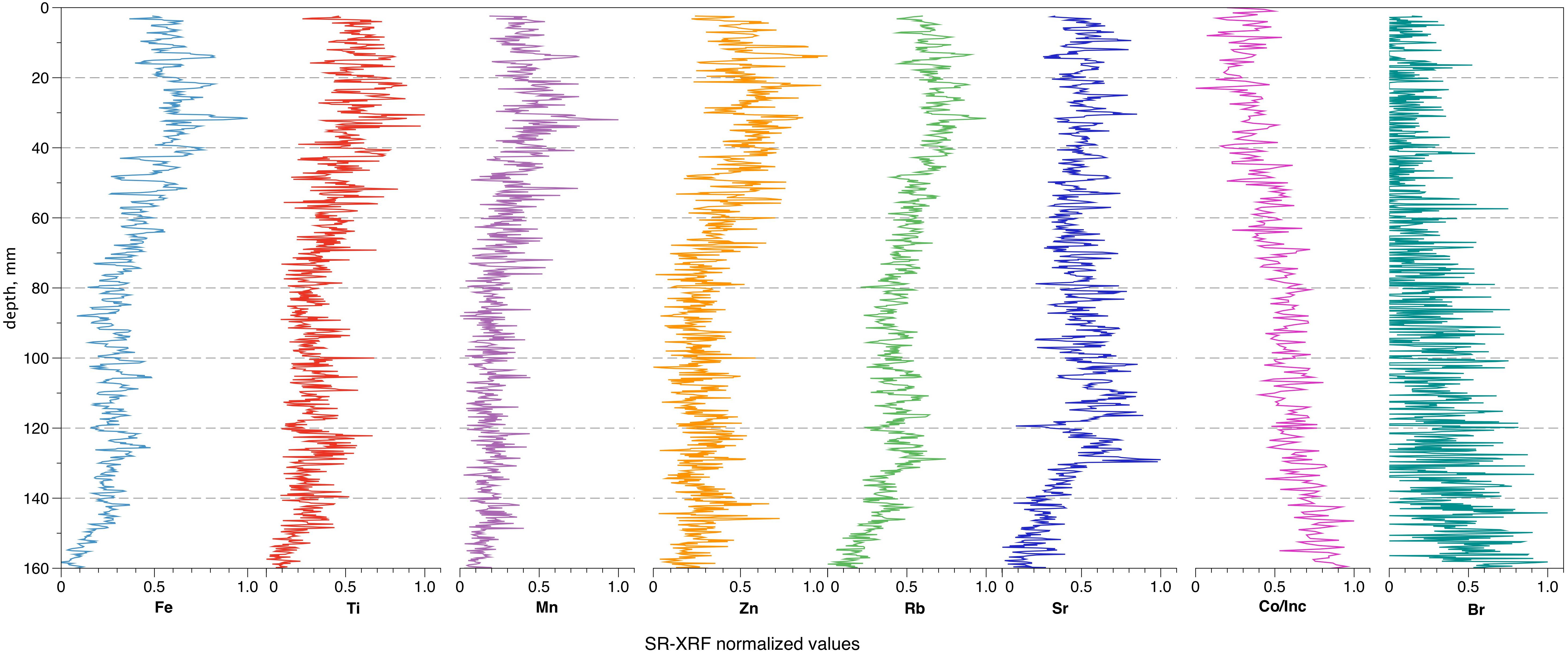
FIGURE 4. Results of the high-resolution SR-XRF scanning analysis (upper 160 mm, normalized values).
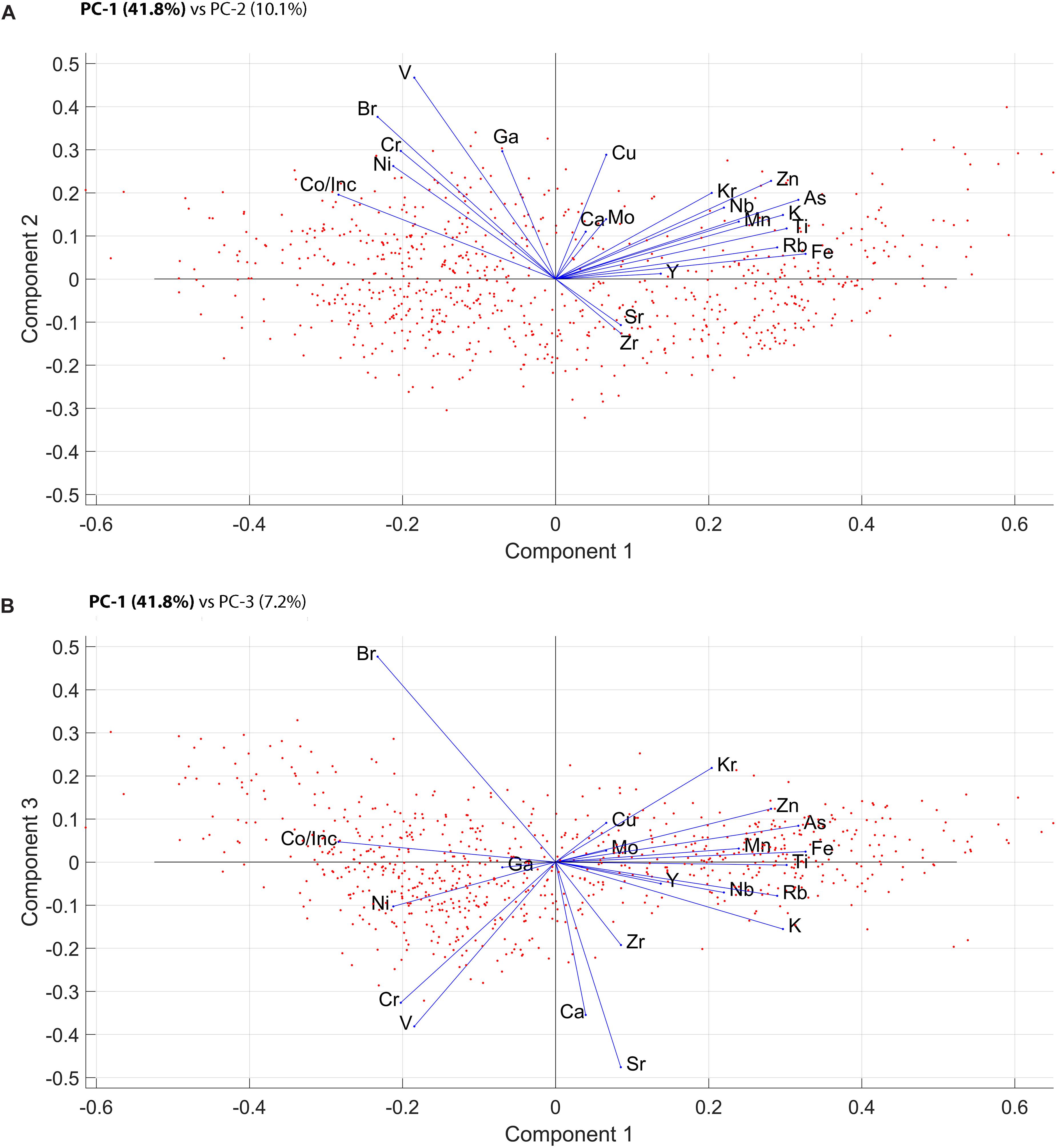
FIGURE 5. Results of PCA analysis of the sedimentary geochemical data; (A) component 1 plotted against component 2; (B) component 1 plotted against component 3.
Chronology
The first fundamental hypothesis of this project is to confirm, whether the distinctively alternating dark and light laminae constituting the bottom sediment of Lake Donguz-Orun are formed by inter-annual variations in sedimentation, i.e., represent clastic varves. In a mountainous environment like the one hosting Lake Donguz-Orun, clastic varves are common. Clastic varves often consist of a couplet – coarse-grained sediments are deposited in spring-summer period during active transport of the sediment; whereas, a fine-grained layer is deposited during winter period when the lake is ice-covered (Ojala et al., 2012; Zolitschka et al., 2015). The approach of distinguishing annual layers within a given elevated setting with very limited autochthonous productivity and bioturbation can be based solely on within-year variations in sediment grain size. The traditional approach of developing a varve-based age-depth relationship relies on independent visual varve counting and thickness measurements (Ojala et al., 2012). In case of Lake Donguz-Orun, varve counting was limited by the varying quality of laminae preservation. We undertook various attempts to directly calculate the layers on the surface of the split core visually or by means of processing the photographic image. Visual counts indicate between 70 and 100 layers for the upper 160 mm of core LDOPC12-1 (Figure 3).
In order to establish this visually based age-depth determination, we employed geochemical markers obtained by means of high-resolution scanning XRF analysis serving as high-precision markers of potential annual and sub-annual sedimentation processes. The approach of using high resolution XRF data to trace the boundaries of annual layers has been used previously in various settings. Marshall et al. (2012) report a successful counting of elemental peaks of Fe and Mn as markers of autumn siderite layers complimentary to direct varve counts. The authors also state distinct peaks of Ti, K, Rb, and Zr – the elements that have a tendency to become concentrated in particular grain size fractions (Marshall et al., 2012). Kalugin et al. (2013) found Ca/Sr and Br/Rb ratios having opposite profiles as the most stable indices for varve counting with Rb representing clastic part of the sediment, Br – organic and Ca responsible for carbonates. In this study we use changes in Rb/Sr and Zr/Rb ratios as indicators of seasonal changes in spring/summer vs. winter clastic input.
The approach of using the Rb/Sr ratio is based on different environmental mobility of the two elements (Zhisheng et al., 2001; Kalugin et al., 2005; Darin et al., 2015). The finer the sediment, the higher the surface area. Consequently, strontium – a more mobile element than rubidium – is weathered and removed from the sediment grains at a higher rate. In that case summer (flood) layers would be represented with minimums on the curve of Rb/Sr ratio, while winter layers consisting of fine-grain sediment would be characterized by maximums. Kalugin et al. (2007) in their study dedicated to the bottom sediments of Teletskoye Lake (Altai Mountains) report a significant correlation between Sr/Rb ratio and median grain size in the range from <2 to 28 μm (r = 0.68, p < 0.01) for the dataset of 390 samples confirming visual thin-section observations. Rb/Sr curve throughout the scanned 160 mm reveals prominent peaks that allow for a confident calculation. Counting of Rb/Sr maxima within the scanning distance yields the result of 88 years (see Figure 6A). Additional scanning using concentrating X-ray optics at 30 μm resolution was performed for the upper 20 mm of the core LDOPC12-1 in order to examine in detail within-year variations in grain size and support the varve hypothesis. The assumed annual cycle of sedimentation can be seen on the curve of Rb/Sr superimposed on the photographic image of the upper section of the sediment core (see Figure 6B). It can be seen that variations in Rb/Sr ratio generally follow the unapparent laminated pattern of the sediment. The resolution of 30 μm yields ∼40–50 measurements across each couplet – a number considerably exceeding standard procedures (Zolitschka et al., 2015).
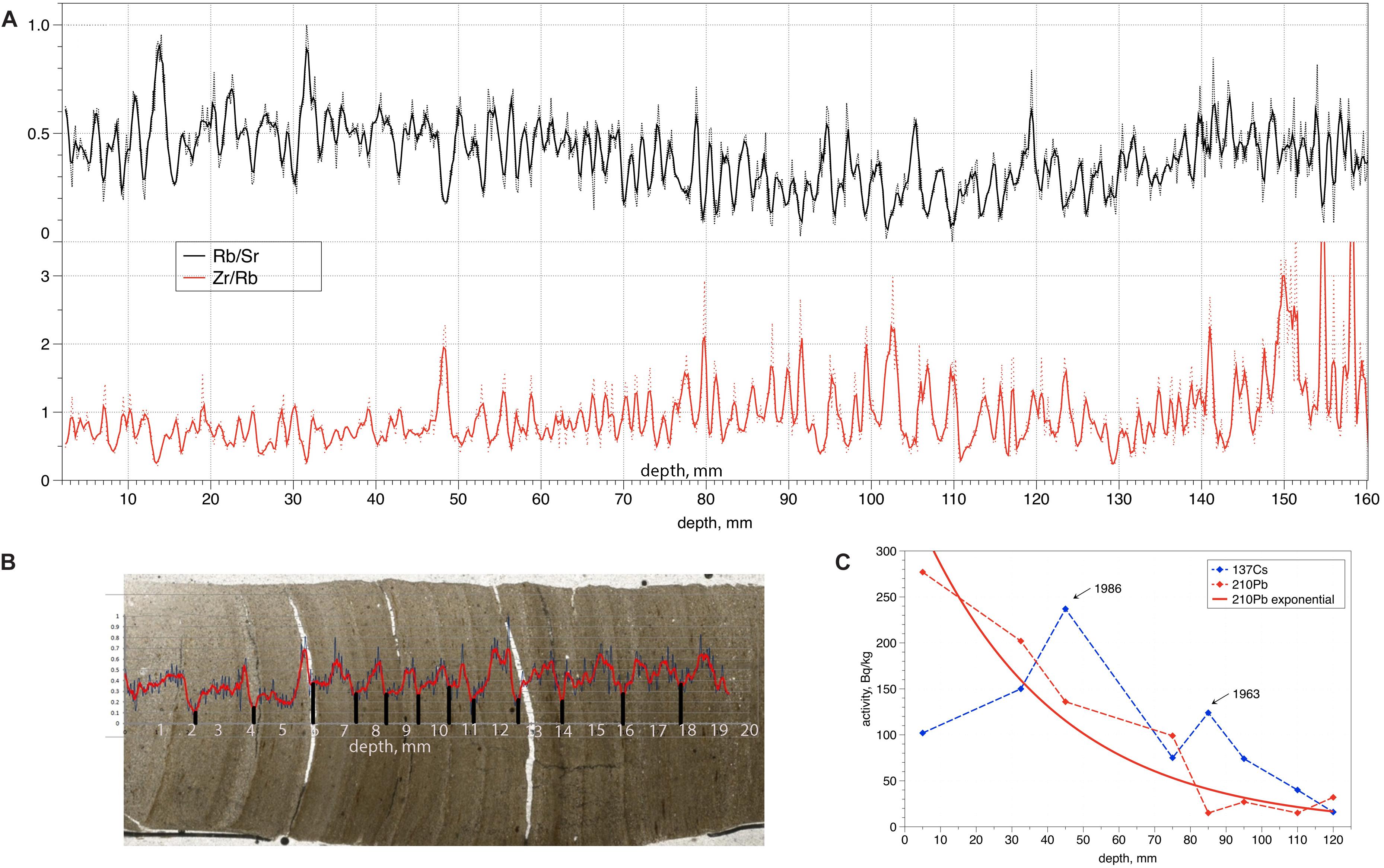
FIGURE 6. (A) Profiles of Rb/Sr and Zr/Rb ratios used as markers of annual layers. Dashed lines indicate measured data, solid lines: 3-point averaged; (B) Rb/Sr profile for the upper 20 mm obtained using concentrating X-ray optics at 30 μm resolution superimposed on the photographic image; (C) 137Cs and 210Pb dating results.
A Zr/Rb ratio was previously used as a proxy for changes in grain size for arctic and alpine sedimentary sequences (Dypvik and Harris, 2001; Kylander et al., 2011), with lower values representing fine-grained material and higher values representing coarse-grained material. Rb/Sr and Zr/Rb curves, thus, must have opposed behavior, which is clearly observed on the joint graph (Figure 6A) and supported by correlation values (r = -0.41, p < 0.01). The use of a secondary calculation method helps to eliminate additional smaller peaks apparently occurring as a result of certain flash flood events within a year. The calculation of Zr/Rb minima within the scanning distance yields the result of 87 years.
An independent age control is crucial while creating a varve-based age-depth relationship. In order to constrain the XRF-derived chronology, 137Cs and 210Pb dating were used. The 137Cs curve for the sediment of Lake Donguz-Orun has two prominent peaks that can be consistently attributed to the two widespread events of the isotope fallout (Figure 6C). If the maximum of 137Cs at 40–50 mm corresponds to 1986 and the one at 80–90 mm to 1961–1963, the sedimentation rate appears close to linear and, basing on the two peaks, yields the mean result of 1.75 mm/year.
In order to determine the sedimentation rate with the use of 210Pb isotopes, the unsupported 210Pb (210Pbuns) was calculated by subtracting supported activity (226Ra) from the total activity (Figure 6C). The constant rate of supply (CRS) model was used (Appleby, 2002). The mean sedimentation rate (calculated as 210Pb radioactive decay constant divided by the tangent to the 210Pbuns curve) yields the value of 1.69 mm/year. Table 1 shows the comparison of mean sedimentation rates acquired with the use of different methods. Shown in bold is the selected method used for creating annually resolved age-depth model.
Figure 7 shows the comparison of chronologies derived from XRF peak counting (Rb/Sr maxima and Zr/Rb minima) and isotopes (137Cs and 210Pb). It can be seen that although the number of years for the selected 160-mm depth range is practically equal for both XRF counts, there is a discrepancy of up to 6–7 years in the middle section. Unlike Zr/Rb chronology, Rb/Sr chronology is constrained by both peaks in 137Cs activity. Rb/Sr chronology also lies closer to the range of mean age-depth relations obtained through 210Pb dating. Consequently, in case of Lake Donguz-Orun sediment, Rb/Sr ratio must serve as a most reliable method of establishing annually resolved age-depth relation, and we proceed with Rb/Sr-derived chronology.
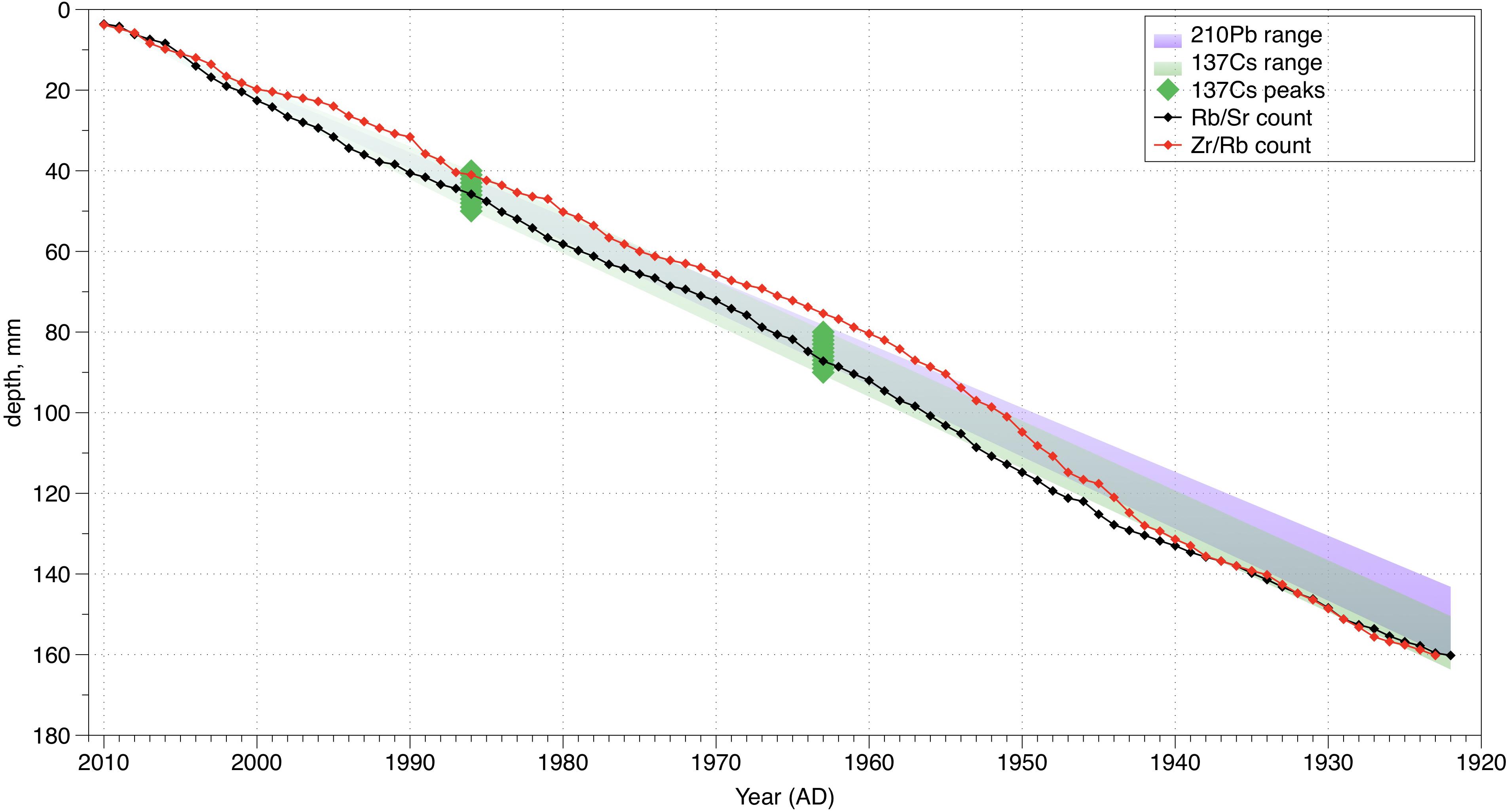
FIGURE 7. Comparison of chronologies derived from XRF peak counting (Rb/Sr maxima and Zr/Rb minima) and isotopes (137Cs and 210Pb). Black and red dots mark annual layers according to Rb/Sr and Zr/Rb counts, respectively. Green dots mark the position of the two known peaks on the 137Cs curve. Green and purple shaded areas show possible distribution of mean age-depth relations for 137Cs and 210Pb, respectively.
A crucial issue while working with piston corer-derived sediment cores is whether the uppermost laminae are preserved and if not, then how long is the deficient part. In case of a stiff sediment like the one of Lake Donguz-Orun it is unlikely that a significant part is lost, in addition, results of 137Cs content imply ∼40 cm above the layer corresponding to the year 1986. Nevertheless, the uppermost year requires confirmation in order to tie in the absolute chronology. Although the coring date is known (July 16, 2012) we cannot be sure that the uppermost layer dates back to 2011 (the layer of 2012 was not yet complete in July). In order to check the date of the upper layer and to assess the accuracy of the varve identification in general, we used the local pine tree ring width chronology from the site D09S that is situated in less than 3 km from the Lake Donguz-Orun (see Figure 2) and compared it with the assumed annual layer thickness. Varve thickness is a basic element of any varve chronology that is usually measured visually or by means of various graphic analyses. In case of Lake Donguz-Orun records, we measured annual layer thickness as a distance between neighboring peaks on the Rb/Sr curve. The comparison showed that there is a significant agreement (r = 0.4, p < 0.05) between the ring width and layer thickness if the sediment sequence starts in 2010. Figure 8 shows the comparison of the detrended series of annual layer thickness and TRW indices of the site D09S. It is evident that there is a year-by-year correspondence between series without major lags and offsets. We discuss potential reasons for this correlation in the Section “Discussion”. We therefore suppose that we can use absolutely dated tree-ring series as a basis for the cross-dating of our sediment sequence and to confirm that the uppermost preserved layer of sediments indeed was deposited in 2010. Thus, we can proceed with chorology for the years 1922–2010.
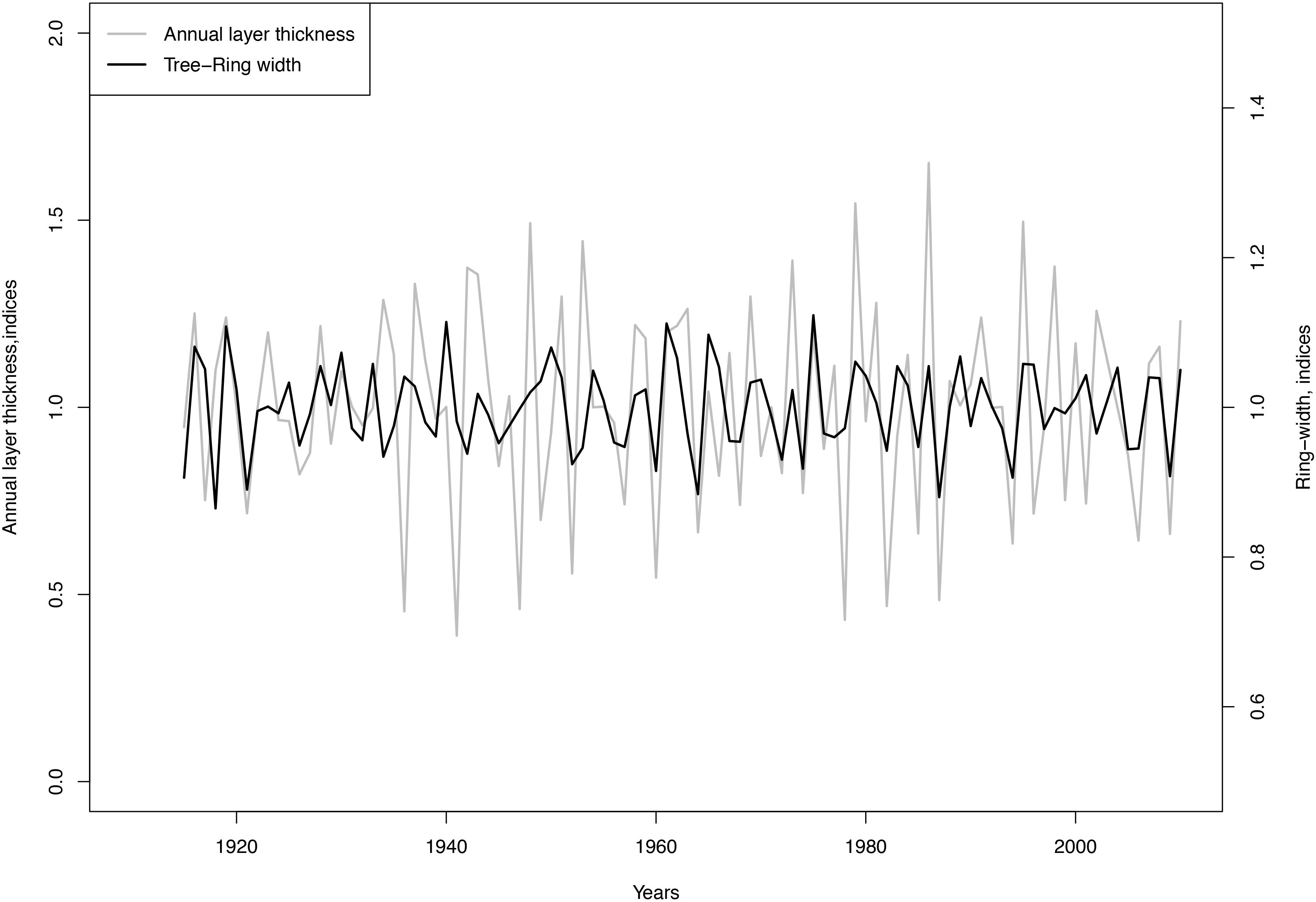
FIGURE 8. Comparison of the detrended series of annual layer thickness (calculated as distance between Rb/Sr peaks) and TRW indices of the site D09S.
Discussion
Accuracy and Completeness of the Sediment Sequence
A typical varve chronology has an accuracy of ±1–4% (Ojala et al., 2012). Although we demonstrated a good correspondence of various metrics of sedimentation (Rb/Sr, Zr/Rb, 137Cs, 210Pb) and even a correlation of varve thickness and ring width, it is evident that complete 1-year accuracy may not be achieved in our sediment series. Figure 7 shows that although the number of layers identified by two records Rb/Sr and Zr/Rb are almost equal (88 and 87 layers, respectively), there is no year-by-year correspondence in the middle of the sequences, where the offset may reach 6–7 years. The reason for these uncertainties is explained by certain subjectivity in attribution of some peaks in the records. They can either mark an annual boundary or secondary inter-seasonal peaks. Secondary peaks may occur due to significant within-year events, such as flood events or mass wasting. At the same time, certain annual layers can be omitted being represented by weak peaks on the Rb/Sr or Zr/Rb curve. The question of distinguishing annual layers from layers formed by sub-annual processes (i.e., flash floods, etc.) in the geochemically derived record remains topical.
Relation Between Sedimentary Data and Meteorological Parameters
Having established a varve chronology, we can proceed with the second fundamental assumption – whether or not the sedimentary sequence is capable of recording a climate signal. In order to do this, we compare the sedimentary data, namely annually averaged values of elements and elemental ratios with the available local annually reserved archives: temperature and precipitation (annual and seasonal) registered at the three closest meteorological stations – Terskol, Teberda, Kluhorskij Pereval; Glacier Djankuat (Elbrus Region) mass-balance measurements and tree-ring chronologies from various sites in Western and Central Caucasus.
Principal component analysis implies a consistent common signal in the geochemical sedimentary data associated with variations in concentrations of terrigenous elements on the one hand, and Br and Co/Inc ratio on the other. Basing on PCA analysis, we divide the elements into two groups: indicators of terrigenous and organic input. Annually averaged elemental values reveal a consistent linear trend throughout the 1922–2010 period: increasing for terrigenous elements (i.e., Fe: r = 0.90, p < 0.01), and decreasing for Br and Co/Inc ratio (Br: r = -0.84; p < 0.01).
The majority of terrigenous elements are transported to the lake as a result of weathering of baserock of the catchment area, and the intensity of their supply directly depends on the sediment load of the glaciofluvial streams and intensity of surface runoff. Content of terrigenous elements in Donguz-Orun sediments reveals a significant relation with annual precipitation recorded at the meteorological station of Teberda (Ti: r = 0.44, p < 0.01; Fe: r = 0.41, p < 0.01; Zn: r = 0.37, p < 0.01; Mn: r = 0.37, p < 0.01) (Figure 9). At the same time, annually averaged values of terrigenous elements reveal consistent correlation (up to r = 0.55, p < 0.01 for Ti) with summer temperature registered at the meteorological stations of Terskol and Teberda. Within a study site, increased summer temperatures result in increased glacier melt and, as a result, increased glaciofluvial sediment load. For the glacier of Djankuat (situated 20 km eastward of Lake Donguz-Orun), where uninterrupted mass balance measurements take place since 19672, it has been shown that ablation is largely dictated by variations in summer (JJA) temperatures (correlations of 0.61 and 0.77 between ablation and JJA mean and mean maximum air temperatures, respectively) (Shahgedanova et al., 2005). Sedimentary elemental data compared with Djankuat ablation directly yield lesser (up to r = 0.33, p < 0.05 for Ti), but still positive and statistically significant values. Taken together, that points to the conclusion that variations in the content of terrigenous elements in the sediment register a complex climate signal that is comprised of at least two primary components: 1 – annual precipitation that accounts for denudation and downwash of the catchment bedrock; 2 – summer temperatures that account for glacier melt and glacial meltwater sediment load.
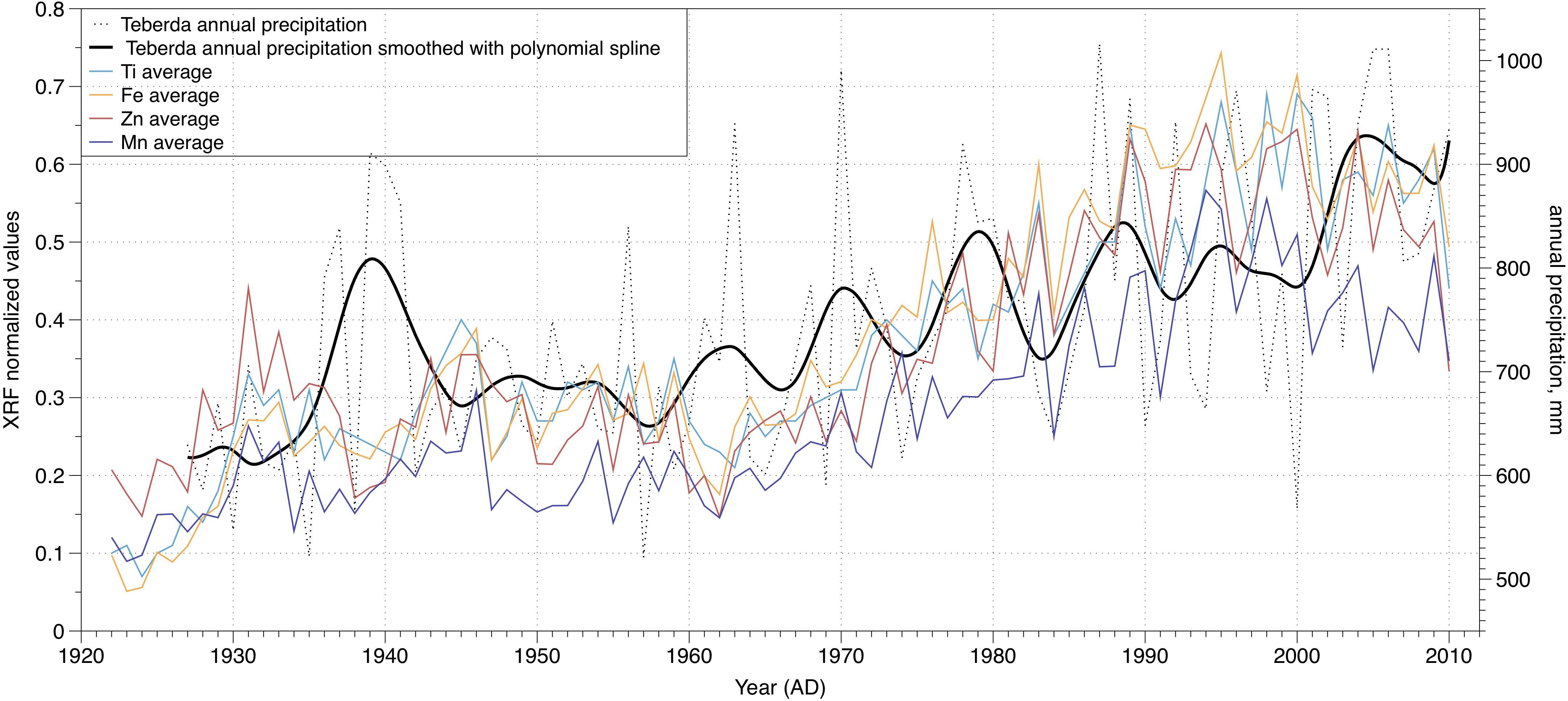
FIGURE 9. Annual precipitation recorded at the Teberda meteorological station compared with the yearly averaged values of terrigenous elements.
Bromine content in the sediment and Co/Inc ratio are often interpreted to reflect relative changes in organic content of the sediment. Davies et al. (2015) in their review dedicated to the recent progress in interpretation of XRF data in lake sediments cite a number of successful applications of these proxies at high resolution. Br makes strong covalent bonds with organic molecules (Gilfedder et al., 2011) and can be used as an indicator of both variations in the input of organic matter and/or lake primary productivity. Our previous results of studying the sediment cores of Lake Karakyol (Western Caucasus) showed that Br content in the sediment is coherent with the peak of the thermophile broad-leaved pollen in the past millennia (Solomina et al., 2014). Kalugin et al. (2007) report that Br content in the bottom sediments of Teletskoye Lake (Altai Mountains) covaries with measured total organic carbon (TOC); the authors also state a correlation between the Br content and annual temperature at the meteorological station of Barnaul during AD 1840–1996 (r = 0.41, p < 0.05). Inc/Coh is an atomic number-dependent ratio that can serve as proxy for organic carbon content due to its low atomic number (Croudace et al., 2006). Previous studies reported a pronounced covariance between Inc/Coh ratio and measured TOC (Guyard et al., 2007; Giralt et al., 2011; Liu et al., 2013).
For the sediment of Lake Donguz-Orun annually averaged values of Br and Co/Inc ratio show comprehensive positive correlation (r = 0.82, p < 0.01). Both parameters positively correlate with the annual temperature at the neighboring meteorological station of Terskol (Br: r = 0.41, p < 0.05; Co/Inc: r = 0.40, p < 0.05) (Figure 10) and negatively with annual precipitation of Teberda (Br: r = 0.39, p < 0.05; Co/Inc: r = 0.45, p < 0.05). Accordance with annual temperature may appear reasonable, considering that in case of elevated lakes like Donguz-Orun, increased temperatures can lead to increased bioproductivity in the lake. Increased precipitation, in turn, results in increased intensity of downwash from the proximal vegetated slopes. Thus, we hypothesize that variations of Br and Co/Inc ratio also register a dual climate signal comprised of both annual temperature (accountable for variations in lake’s autochthonous productivity) and precipitation (accountable for allochthonous input of organic matter).
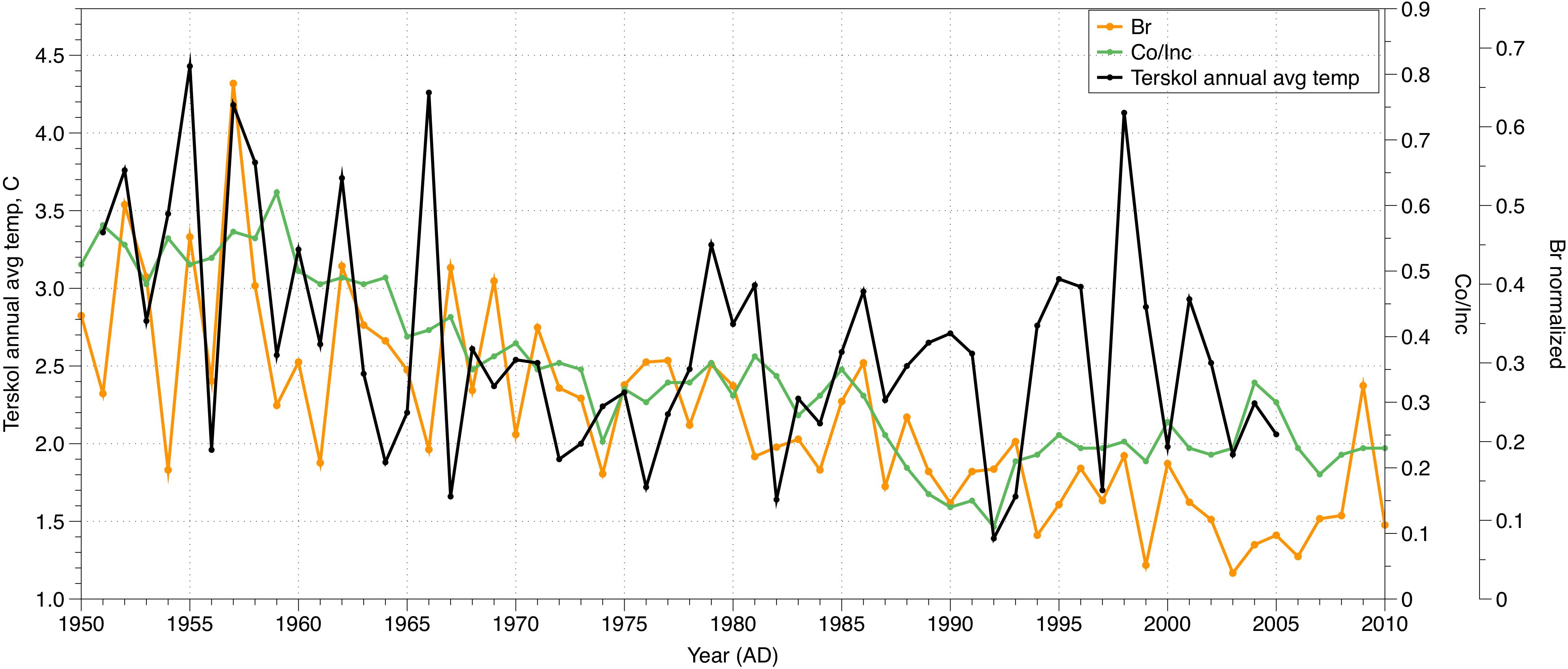
FIGURE 10. Annual average temperature recorded at the Terskol meteorological station compared with the yearly maximal values of bromine and Co/Inc ratio.
Relation Between Sedimentary Data and Tree Rings
Owing to a vast and ongoing dendrochronological research in the Caucasus we were able to compare the sedimentary elemental data with 18 Scots pine (Pinus sylvestris L.) ring width (TRW) chronologies in the Western and Central Caucasus and a regional blue intensity (BI) chronology (Dolgova, 2016). It is noteworthy that out of all the tree ring chronologies the strongest correlation with the sedimentary data is demonstrated by the ring-width chronology from the site D09S that is situated less than 3 km away from the Lake Donguz-Orun (see Figure 1).
Positive correlation of TRW indices and yearly averaged values of bromine (Br) is observed (r = 0.39, p < 0.01). At the same time, terrigenous elements – Fe, Ti, Zn, Mn – reveal a consistent negative relation with TRW indices of the site D09S with notably high correlation values (Fe: r = -0.51, p < 0.01; Ti: r = -0.48, p < 0.01; Zn: r = -0.56, p < 0.01; Mn: r = -0.55, p < 0.01). Figure 11 shows the reversed values of the three terrigenous elements – iron (Fe), titanium (Ti), and zinc (Zn) compared to TRW indices of the site D09S. The values of the elements were reversed (original normalized values were subtracted from 1) for the sake of visual clarity. The accordance of the two time series smoothed with a 5-year running mean (Ti: r = -0.65, p < 0.01; Fe: r = 0.69, p < 0.01; Zn: r = 0.75, p < 0.01; Mn: r = 0.72, p < 0.01) (Figure 11) provides an additional evidence of the adequate varve chronology.
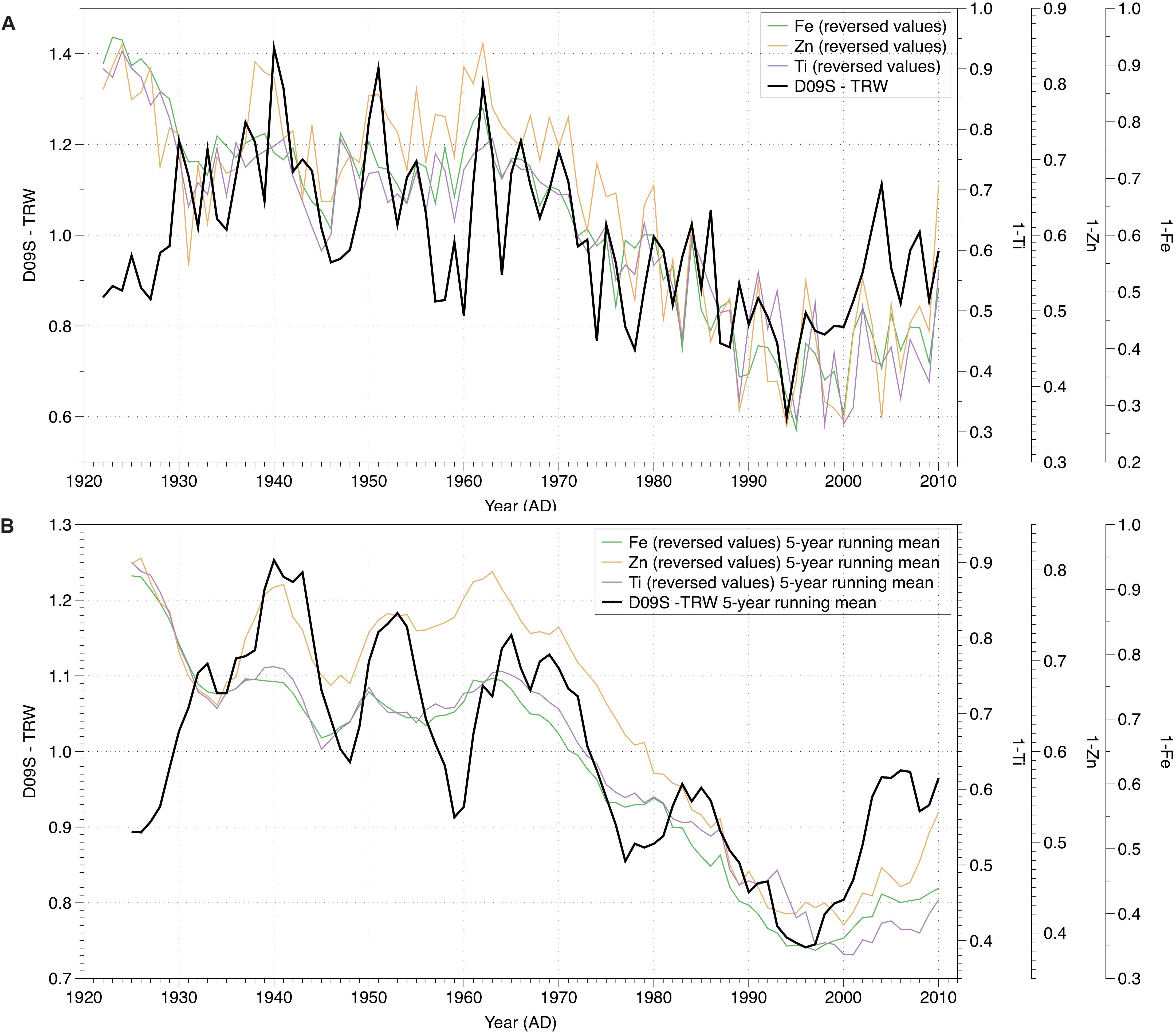
FIGURE 11. Reversed values of Fe, Zn, and Ti compared with the tree-ring width indices (site D09S): (A) yearly values; (B) values smoothed with 5-year running mean.
It is interesting, that for the period 1922–1931 the TRW and elemental data show no meaningful correlation. That may indicate (1) a shift in the sedimentation pattern around 1922, (2) a shift of the ring width climate sensitivity, (3) an error in distinguishing the annual layers in the lowermost part of the core.
Tracing Signal in Varve Thickness
In a glacier-fed lake (such as Lake Dongus-Orun) the annual layer thickness and the terrigenous composition would represent two major environmental agents: (1) the activity of the glacier, nourishing the lake; (2) the precipitation, both liquid and solid, over the whole year. The glacial component in the sediments would positively correlate with the temperature in summer, when clastic material eroded by the melting glacier is transported and deposited in the lake. Increased annual precipitation would also increase the layer thickness bringing more sediment in the lake. According to this logic, the thickest layers would correspond to the hot summers and the years with wet climate, or either of them. The same combination of climatic parameters would contribute to the abundance of terrigenous elements in the deposits due to a more intensive weathering and erosion.
It would be ideal if we could disentangle the impact of glacier activity and precipitation in the sediment sequences in order to reconstruct precipitation and summer temperature rather than recording their cumulative effect. For this purpose, Lan et al. (2017) used the first fine fraction (5–6 microns) in Lake Harnur sediments as an indicator of glacier melt, and hence, summer temperature, while the coarse fraction (20–22 microns) dominated by the runoff input was used to reconstruct precipitation variations. Unfortunately, we had no possibility to use this approach with our high resolution records, because the annual layers are too narrow for direct grain-size measurements. The only potential high resolution proxy for glacial activity in our disposal is Rb/Sr-derived annual layer thickness (Figure 12). The correlation of varve thickness with local meteorological data (annual and summer temperatures, annual, summer and winter precipitation) as well as Djankuat accumulation, ablation and mass balance, however, did not reveal statistically significant results. We also compared the varve thickness with the dates of major avalanche events that occurred during the snowiest winters in the Near-Elbrus area (Oleynikov et al., 2000) but no clear correspondence was found, as well.
According to the direct observation and dating of moraines, during the 20th–21st centuries the glaciers in the area were retreating with two short periods of re-advances or stabilization of front positions in 1920s and 1970s–1980s (Solomina et al., 2016). The varve thickness chronology neither shows any long-term trends in the period 1922–2010, nor shorter periods that can be connected to the change of glacier sizes and variation of glacier runoff. The lack of correlation of the varve thickness with the glacier activity may be partly explained by geomorphic reasons. Large deltas occupy the flat surface of the ancient fluvioglacial plain (paleolake) between the modern glaciers and the lake Donguz-Orun (Figure 2). Some glacial deposits may be trapped here, before they reach the lake. At the same time, the lack of correlation here may be explained by the short time period available for comparison.
At the same time, a positive correlation between the Rb/Sr-derived annual layer thickness and the pine ring width of the proximal site D09S was discovered (see section “Chronology”) in addition to substantial correlations between annually averaged elemental values and TRW of the same site, described in the previous section. As it was established before (Dolgova, 2016), climatic signal in ring width pine chronologies in the Caucasus is generally very weak. To explore the potential signal in the varve thickness series of the tree ring site D09S to record climate data we used traditional response function approach. The D09S ring width chronology has significant (but low) positive correlations (r = 0.22, p < 0.05) with the temperature in November–December and a negative correlation with precipitation of April (r = -0.22, p < 0.05) – the months, that are obviously not very significant for the varve deposition because the lake is covered ice-covered during this period. Hence, the response function of the D09S pine chronology is unable to help us to understand a significance of the similarity between the two records and potential climatic signal in the varve thickness series. The correlation between the two different proxies most probably exists due to the combination of certain climatic factors favorable for both proxies, which are yet to be resolved.
A longer sequence is needed to explain the observed phenomena. The new core LDOPC14-2, having the length of at least 580 mm cold yields a varve-based chronology of up to 300–350 years long, considering approximately constant sedimentation rate. Apart from regular lamination that is consistent throughout the whole core, the core LDOPC14-2 includes several atypical homogenous layers comprised of presumably larger grain-size sediment that may represent flood or avalanche layers. Deeper analysis could potentially provide reconstructions of past catastrophic events supported by the local historical archives.
Conclusion
(1) Bottom sediments of Lake Donguz-Orun consist of visually distinct laminae with limited possibilities for direct counting. The hypothesis that the laminae reflect annual changes in sediment grain size, i.e., represent clastic varves, was confirmed with the use of Rb/Sr ratio serving as a proxy for grain size variations, that was obtained by means of scanning SR-XRF. Additional scanning for the upper 20 mm obtained using concentrating X-ray optics at 30 μm resolution allowed for a detailed examination of within-year variations in grain size. The results of Rb/Sr counting were supported by 137Cs and 210Pb dating results. In a mountain setting like the one studied, Rb/Sr ratio can most probably serve as a reliable high-resolution indicator of variations in grain size.
(2) Rb/Sr maxima counting allowed for an annually resolved chronology for the years 1922–2010. Comparison with local meteorological data reveals a consistent relation of the sedimentary elemental values with temperature and precipitation. The most prominent is the coherence between the concentration Br and annual temperature and between concentrations of terrigenous elements (Fe, Ti, Mn, and Zn) and precipitation. At the same time, correlation values are not as high so that elemental variability could be dictated solely by ether meteorological parameter.
(3) Comparison with the tree ring archive across Western and Central Caucasus revealed a pronounced common behavior of sedimentary elemental data and the tree ring width of the proximal tree ring site – positive for Br and negative for terrigenous elements. Considering that both archives reveal a limited response to meteorological data that connection points to a common composite climatic signal in the two independent records and offers additional confirmation that the laminae represent annual layers. The detailed nature of that signal is yet to be resolved.
(4) Combining of the supposed annual layer thickness, measured as a distance between neighboring maxima on the Rb/Sr curve, with the tree ring width indices of the abovementioned site allowed for constraining the uppermost complete year of the sediment sequence and exclude the possibility of a longer lost top section. Cross correlation of lake sediment properties with local tree ring data appears to be a promising tool for the future research.
(5) Taken together, our data point to the conclusion that bottom sediments of Lake Donguz-Orun represent an important source of complex paleoclimatic information that can be decoded with annual to seasonal resolution. Core LDOPC14-2, that reveals regular lamination throughout 580 mm, offers such a source for estimated 300–350 years and will be considered for the future research. Updating tree-ring records and continuing work on Lake Donguz-Orun, namely coring, studying sedimentation using traps, and employing XRF and other scanning techniques on the new cores, is important to gain better understanding of the processes forming the local environmental signals that can be used for paleoreconstructions.
Author Contributions
MA was responsible for the research process concerning the bottom sediment of the Lake Donguz-Orun in the IG RAS, Moscow – starting from the field coring works to carrying out analyses and writing. AD and IK performed the pioneering studies of lake sediments using SR-XRF in the IGM SB RAS, Novosibirsk and enabled high-resolution analyses described in the paper. AG provided editing and consulting. ED is a tree-ring specialist from the IG RAS who facilitated joint sedimentary and tree-ring research. OS is an unchallenged leader of the paleoclimatic research group in the IG RAS responsible for general conception and advice.
Funding
The dendrochronological research was supported by grant #18-55-18012.
Conflict of Interest Statement
The authors declare that the research was conducted in the absence of any commercial or financial relationships that could be construed as a potential conflict of interest.
Acknowledgments
The authors thank the Russian Foundation for Basic Research (Grants #17-05-01170 À and 17-35-50134) for support. They also thank Vladimir Matskovsky, Vasily Shishkov, and Vladimir Mikhalenko for the assistance in the coring process. MA is grateful to Prof. Atle Nesje and other colleagues from the University of Bergen (Norway) for assistance, advice, and laboratory facilities during his visit. They also grateful for the help in the field work to Dr. Alexander Oleinikov and the other colleagues from the Moscow State University. They also like to express their profound gratitude to the reviewers – their efficient criticisms allowed them to better understand the research subject and thus make the paper more focused.
Footnotes
References
Appleby, P. G. (2002). “Chronostratigraphic techniques in recent sediments,” in Tracking Environmental Change Using Lake Sediments, eds M. William, Smol, and P. John (Dordrecht: Springer), 171–203.
Appleby, P. G., and Oldfield, F. (1978). The calculation of lead-210 dates assuming a constant rate of supply of unsupported 210Pb to the sediment. Catena 5, 1–8. doi: 10.1016/S0341-8162(78)80002-2
Bakke, J., Lie,Ø, Nesje, A., Dahl, S. O., and Paasche, Ø (2005). Utilizing physical sediment variability in glacier-fed lakes for continuous glacier reconstructions during the Holocene, northern Folgefonna, western Norway. Holocene 15, 161–176. doi: 10.1191/0959683605hl797rp
Bobrov, V. A., Kalugin, I. A., Klerks, J., and Stepin, A. S. (1999). Modern accumulation rate in the Lake Teletskoe based on gamma-spectrometry (137Cs). Geol. Geofiz. 40, 530–536.
Chen, H. W., Lee, T., Ku, T. L., and Das, J. P. (2008). Production ratio of nuclear fallout 137Cs/135Cs. Chin. J. Phys. 46, 560–569.
Croudace, I. W., Rindby, A., and Rothwell, R. G. (2006). “ITRAX: description and evaluation of a new multi-function X-ray core scanner,” in New Techniques in Sediment Core Analysis, Vol. 267, ed. R. G. Rothwell (London: Geological Society of London), 51–63.
Darin, A. V., Aleksandrin, M. Y., Kalugin, I. A., and Solomina, O. N. (2015). Influence of meteorological conditions on the geochemistry of modern bottom sediments exemplified by deposits of Donguz-Orun Lake, Caucasus. Dokl. Earth Sci. 463, 842–846. doi: 10.1134/S1028334X15080097
Darin, A. V., Baryshev, V. B., and Zolotarev, K. V. (1991). Scanning X-ray fluorescence microanalysis of phosphorites from the underwater mountains of the Pacific. Nucl. Instrum. Methods Phys. Res. A 308, 318–320. doi: 10.1016/0168-9002(91)90658-D
Darin, A. V., Kalugin, I. A., Maksimova, N. V., Smolyaninova, L. G., and Zolotarev, K. V. (2005). Use of a scanning XRF analysis on SR beams from VEPP-3 storage ring for research of core bottom sediments from Teletskoe Lake with the purpose of high resolution quantitative reconstruction of last millennium paleoclimate. Nucl. Instrum. Methods Phys. Res. A 543, 255–258. doi: 10.1016/j.nima.2005.01.217
Darin, A. V., Kalugin, I. A., and Rakshun, Y. V. (2013). Scanning X-ray microanalysis of bottom sediments using synchrotron radiation from the BINP VEPP-3 storage ring. Bull. Russ. Acad. Sci. 77, 182–184. doi: 10.3103/S106287381302010X
Darin, A. V., and Rakshun Ya, V. (2013). Method of measurement during determination of the elemental composition of rock samples by X-ray fluorescence analysis using synchrotron radiation from the VEPP-3 storage ring. Sci. Bull. NSTU 2, 112–118.
Davies, S. J., Lamb, H. F., and Roberts, S. J. (2015). “Micro-XRF core scanning in palaeolimnology: recent developments,” in Micro-XRF Studies of Sediment Cores, eds R. G. Rothwell and I. W. Croudace (Dordrecht: Springer), 189–226. doi: 10.1007/978-94-017-9849-5_7
Dolgova, E. (2016). June–September temperature reconstruction in the Northern Caucasus based on blue intensity data. Dendrochronologia 39, 17–23. doi: 10.1016/j.dendro.2016.03.002
Dolgova, E. A., and Solomina, O. N. (2010). First quantitative reconstruction of air temperature for the warm period in the Caucasus based on dendrochronological data. Dokl. Earth Sci. 431, 371–375. doi: 10.1134/S1028334X10030220
Dypvik, H., and Harris, N. B. (2001). Geochemical facies analysis of fine-grained siliciclastics using Th/U, Zr/Rb and (Zr + Rb)/Sr ratios. Chem. Geol. 181, 131–146. doi: 10.1016/S0009-2541(01)00278-9
Gilfedder, B. S., Petri, M., Wessels, M., and Biester, H. (2011). Bromine species fluxes from Lake Constance’s catchment, and a preliminary lake mass balance. Geochim. Cosmochim. Acta 75, 3385–3401. doi: 10.1016/j.gca.2011.03.021
Giralt, S., Rico-Herrero, M. T., Vega, J. C., and Valero-Garcés, B. L. (2011). Quantitative climate reconstruction linking meteorological, limnological and XRF core scanner datasets: the Lake Sanabria case study, NW Spain. J. Paleolimnol. 46, 487–502. doi: 10.1007/s10933-011-9509-x
Gulisashvili, V. Z., Makhatadze, L. E., and Prilipko, L. I. (1975). Rastitel’nost’Kavkaza. (Vegetation of the Caucasus). Moscow: Nauka Press.
Guyard, H., Chapron, E., St-Onge, G., Anselmetti, F. S., Arnaud, F., Magand, O., et al. (2007). High-altitude varve records of abrupt environmental changes and mining activity over the last 4000 years in the Western French Alps (Lake Bramant, Grandes Rousses Massif). Quat. Sci. Rev. 26, 2644–2660. doi: 10.1016/j.quascirev.2007.07.007
IPCC (2013). “Climate change 2013: the physical science basis,” in Contribution of Working Group I to the Fifth Assessment Report of the Intergovernmental Panel on Climate Change, (Geneva: IPCC).
Kalugin, I., Darin, A., Rogozin, D., and Tretyakov, G. (2013). Seasonal and centennial cycles of carbonate mineralisation during the past 2500 years from varved sediment in Lake Shira, South Siberia. Quat. Int. 290, 245–252. doi: 10.1016/j.quaint.2012.09.016
Kalugin, I., Daryin, A., Smolyaninova, L., Andreev, A., Diekmann, B., and Khlystov, O. (2007). 800-yr-long records of annual air temperature and precipitation over southern Siberia inferred from Teletskoye Lake sediments. Quat. Res. 67, 400–410. doi: 10.1016/j.yqres.2007.01.007
Kalugin, I., Selegei, V., Goldberg, E., and Seret, G. (2005). Rhythmic fine-grained sediment deposition in Lake Teletskoye, Altai, Siberia, in relation to regional climate change. Quat. Int. 136, 5–13. doi: 10.1016/j.quaint.2004.11.003
Karlén, W. (1976). Lacustrine sediments and tree-limit variations as indicators of Holocene climatic fluctuations in Lappland, northern Sweden. Geogr. Ann. 58, 1–34. doi: 10.1080/04353676.1976.11879921
Karlén, W. (1981). Lacustrine sediment studies: a technique to obtain a continous record of Holocene glacier variations. Geogr. Ann. 63, 273–281.
Knyazev, A. V., Savinetsky, A. B., and Gei, N. A. (1992). “History of North Osetia vegetation during the Holocene,” in Historical Ecology of Wild and Domestic Ungulates, ed. L. G. Dinesman (Moscow: Nauka Press),84–108.
Kvavadze, E. V., and Efremov, Y. V. (1996). Palynological studies of lake and lake-swamp sediments of the Holocene in the high mountains of Arkhyz (western Caucasus). Acta Palaeobot. Krakow 36, 107–120.
Kylander, M. E., Ampel, L., Wohlfarth, B., and Veres, D. (2011). High-resolution X-ray fluorescence core scanning analysis of Les Echets (France) sedimentary sequence: new insights from chemical proxies. J. Quat. Sci. 26, 109–117. doi: 10.1002/jqs.1438
Lan, J., Xu, H., Sheng, E., Yu, K., Wu, H., Zhou, K., et al. (2017). Climate changes reconstructed from a glacial lake in High Central Asia over the past two millennia. Quat. Int. 487, 43–53. doi: 10.1016/j.quaint.2017.10.035
Leemann, A., and Niessen, F. (1994). Holocene glacial activity and climatic variations in the Swiss Alps: reconstructing a continuous record from proglacial lake sediments. Holocene 4, 259–268. doi: 10.1177/095968369400400305
Leonard, E. M. (1986). Varve studies at Hector Lake, Alberta, Canada, and the relationship between glacial activity and sedimentation. Quat. Res. 25, 199–214. doi: 10.1016/0033-5894(86)90057-8
Liu, X., Colman, S. M., Brown, E. T., Minor, E. C., and Li, H. (2013). Estimation of carbonate, total organic carbon, and biogenic silica content by FTIR and XRF techniques in lacustrine sediments. J. Paleolimnol. 50, 387–398. doi: 10.1007/s10933-013-9733-7
Marshall, M., Schlolaut, G., Nakagawa, T., Lamb, H., Brauer, A., Staff, R., et al. (2012). A novel approach to varve counting using μXRF and X-radiography in combination with thin-section microscopy, applied to the Late Glacial chronology from Lake Suigetsu, Japan. Quat. Geochronol. 13, 70–80. doi: 10.1016/j.quageo.2012.06.002
Matthews, J. A., Dahl, S. O., Nesje, A., Berrisford, M. S., and Andersson, C. (2000). Holocene glacier variations in central Jotunheimen, southern Norway based on distal glaciolacustrine sediment cores. Quat. Sci. Rev. 19, 1625–1647. doi: 10.1016/S0277-3791(00)00008-1
Moiseenko, T. I., Razumovskii, L. V., Gashkina, N. A., Shevchenko, A. V., Razumovskii, V. L., Mashukov, A. S., et al. (2012). Paleoecological studies of mountain lakes. Water Resour. 39, 576–589. doi: 10.1038/srep17419
Nesje, A. (1992). A piston corer for lacustrine and marine sediments. Arct. Alp. Res. 24, 257–259. doi: 10.2307/1551667
Nesje, A., Matthews, J. A., Dahl, S. O., Berrisford, M. S., and Andersson, C. (2001). Holocene glacier fluctuations of Flatebreen and winter-precipitation changes in the Jostedalsbreen region, western Norvay, based on glaciolacustrine sediment records. Holocene 11, 267–280. doi: 10.1191/095968301669980885
Ojala, A. E. K., Francus, P., Zolitschka, B., Besonen, M., and Lamoureux, S. F. (2012). Characteristics of sedimentary varve chronologies–a review. Quat. Sci. Rev. 43, 45–60. doi: 10.1016/j.quascirev.2012.04.006
Oleynikov, A. D., Volodicheva, N. A., and Boyarshinov, A. V. (2000). Snowiness of winters and avalanche activity at the Great Caucasus during the period of instrumental observations. Materialy glyatsiologicheskikh issledovaniy. Data Glaciol. Stud. 88, 74–83.
Osborn, G., Menounos, B., Koch, J., Clague, J. J., and Vallis, V. (2007). Multi-proxy record of Holocene glacial history of the Spearhead and Fitzsimmons ranges, southern Coast Mountains, British Columbia. Quat. Sci. Rev. 26, 479–493. doi: 10.1016/j.quascirev.2006.09.003
Serebryanny, L. R., Golodkovskaya, N. A., Orlov, A. V., Maljasova, E. S., and Ilves, E. O. (1984). Glacier Fluctuations and Moraine Accumulation Processes in the High Mountain Caucasus (Kolebanija lednikov i processy morenonakoplenija na vysokogornom Kavkaze). Moscow: Nauka, 216.
Shahgedanova, M. (2002). “Climate at present and in the historical past,” in The Physical Geography of Northern Eurasia, ed. M. Shahgedanova (New York, NY: Oxford Univ. Press), 70–102.
Shahgedanova, M., Popovnin, V., Aleynikov, A., Petrakov, D., and Stokes, C. R. (2007). Long-term change, interannual and intra-seasonal variability in climate and glacier mass balance in the central Greater Caucasus, Russia. Ann. Glaciol. 46, 355–361. doi: 10.3189/172756407782871323
Shahgedanova, M., Stokes, C. R., Gurney, S. D., and Popovnin, V. (2005). Interactions between mass balance, atmospheric circulation, and recent climate change on the Djankuat Glacier, Caucasus Mountains, Russia. J. Geophys. Res. 110:D04108. doi: 10.1029/2004JD005213
Solomina, O., Bushueva, I., Dolgova, E., Jomelli, V., Alexandrin, M., Mikhalenko, V., et al. (2016). Glacier variations in the Northern Caucasus compared to climatic reconstructions over the past millennium. Glob. Planet. Chang. 140, 28–58. doi: 10.1016/j.gloplacha.2016.02.008
Solomina, O. N., Kalugin, I. A., Darin, A. V., Chepurnaya, A. A., Alexandrin, M. Y., and Kuderina, T. M. (2014). The implementation of geochemical and palynological analyses of the sediment core of Karakyol for reconstructions of climatic changes in the valley of Teberda river (Northern Caucasus) during the Late Holocene: possibilities and limitations. Vopr. Geogr. 137,234–266.
Tylmann, W., Enters, D., Kinder, M., Moska, P., Ohlendorf, C., Porêba, G., et al. (2013). Multiple dating of varved sediments from Lake Łazduny, northern Poland: toward an improved chronology for the last 150 years. Quat. Geochronol. 15, 98–107. doi: 10.1016/j.quageo.2012.10.001
Zhisheng, A., Kutzbach, J. E., Prell, W. L., and Porter, S. C. (2001). Evolution of Asian monsoons and phased uplift of the Himalaya–Tibetan plateau since Late Miocene times. Nature 411, 62–66. doi: 10.1038/35075035
Keywords: lake sediments, varves, X-ray fluorescence analysis, paleoclimatic reconstructions, high-resolution reconstruction, tree rings, Central Caucasus
Citation: Alexandrin MY, Darin AV, Kalugin IA, Dolgova EA, Grachev AM and Solomina ON (2018) Annual Sedimentary Record From Lake Donguz-Orun (Central Caucasus) Constrained by High Resolution SR-XRF Analysis and Its Potential for Climate Reconstructions. Front. Earth Sci. 6:158. doi: 10.3389/feart.2018.00158
Received: 09 April 2018; Accepted: 24 September 2018;
Published: 12 October 2018.
Edited by:
Carmen De Jong, Université de Strasbourg, FranceReviewed by:
Selvaraj Kandasamy, Xiamen University, ChinaXu Chen, China University of Geosciences, China
Lesleigh Anderson, United States Geological Survey, United States
Copyright © 2018 Alexandrin, Darin, Kalugin, Dolgova, Grachev and Solomina. This is an open-access article distributed under the terms of the Creative Commons Attribution License (CC BY). The use, distribution or reproduction in other forums is permitted, provided the original author(s) and the copyright owner(s) are credited and that the original publication in this journal is cited, in accordance with accepted academic practice. No use, distribution or reproduction is permitted which does not comply with these terms.
*Correspondence: Mikhail Y. Alexandrin, alexandrin@igras.ru