- 1Division of Cardiology, Department of Medicine, Emory Clinical Cardiovascular Research Institute, Atlanta, GA, United States
- 2Section on Cardiovascular Medicine, Center for the Prevention of Cardiovascular Disease, Wake Forest Baptist Medical Center, Winston-Salem, NC, United States
- 3Heart Disease Prevention Program, Division of Cardiology, University of California, Irvine, Irvine, CA, United States
Despite unprecedented advances in treatment of atherosclerotic cardiovascular disease, it remains the leading cause of death and disability worldwide. Treatment of major traditional risk factors, including low-density lipoprotein-cholesterol, serves as the foundation of atherosclerotic risk reduction. However, there remains a significant residual risk of cardiovascular events despite optimal risk factor management. Beyond traditional risk factors, other drivers of residual risk have come to the forefront, including inflammatory, pro-thrombotic, and metabolic pathways that contribute to recurrent events and are often unrecognized and not addressed in clinical practice. This review will explore the evidence linking these pathways to atherosclerotic cardiovascular disease and potential future therapeutic options to attenuate residual cardiovascular risk conferred by these pathways.
Introduction
The treatment of cardiovascular disease has seen unprecedented progress in the past several decades. Critical advancements have been made in the recognition and treatment of major traditional risk factors for atherosclerotic cardiovascular disease (ASCVD), among them elevated low-density lipoprotein (LDL)-cholesterol (LDL-C) levels, one of the principal drivers of atherosclerosis. An LDL-centric approach to risk reduction, namely with statins, has served as the foundation for primary and secondary prevention for decades and has led to significant improvement in cardiovascular outcomes. The development of the proprotein convertase subtilisin/kexin type 9 (PCSK9) inhibitors (PCSK9i) has driven LDL-C to levels previously not achievable by statins alone and the randomized controlled trials with PCSK9i provided further confirmation of the LDL hypothesis. Moreover, the cardiovascular outcomes trials with PCSK9i demonstrated that there was no level of achieved LDL-C that was not associated with further benefit. However, despite reduction of LDL-C levels to a mean of 30 mg/dL in the FOURIER (Further Cardiovascular Outcomes Research with PCSK9 Inhibition in Subjects with Elevated Risk) trial, the absolute risk reduction was only modestly reduced by 1.5%. In fact, the recurrent cardiovascular event rate at 3 years in those treated with a PCSK9i remained high at 9.8% (1). A similar finding was seen in ODYSSEY OUTCOMES (Evaluation of Cardiovascular Outcomes After an Acute Coronary Syndrome During Treatment with Alirocumab), in which the composite primary end point of cardiovascular death, non-fatal myocardial infarction (MI), stroke or unstable angina occurred in 9.5% of participants receiving alirocumab, as compared to 11.1% in the placebo arm, despite reductions of LDL-C levels to a mean of 40 mg/dL in the treatment group (2).
The high observed event rate despite aggressive secondary prevention efforts speaks to the concept known as “residual cardiovascular risk,” defined as any suboptimally controlled causal risk factor for ASCVD. There are myriad drivers of residual risk, including pathways that are not directly addressed through current management strategies. Although treatment of LDL-C is the cornerstone of risk reduction, there are other drivers of ASCVD and recurrent events. Although residual risk can be attributable to many factors (i.e., hypertension, tobacco use, elevated blood glucose), this review will focus on pathways beyond traditional risk factors addressed in usual clinical care (inflammatory, pro-thrombotic, and metabolic) that may play a critical role in driving recurrent cardiovascular events (Figure 1).
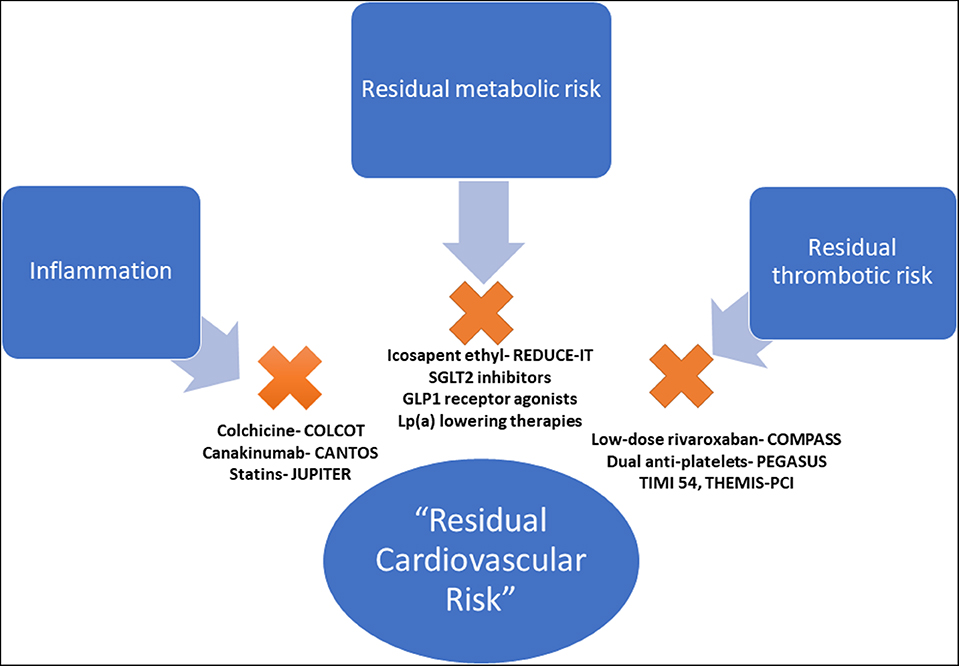
Figure 1. Pathways of residual cardiovascular risk. The pathways of residual cardiovascular risk, beyond traditional risk factors, with evidence-based therapeutic options. COLCOT, Colchicine Cardiovascular Outcomes Trial; CANTOS, Canakinumab Anti-inflammatory Thrombosis Outcomes Study; JUPITER, Justification for the Use of Statins in Prevention: an Intervention Trial Evaluating Rosuvastatin; PEGASUS TIMI 54, Prevention of Cardiovascular Events in Patients with Prior Heart Attack Using Ticagrelor Compared to Placebo on a Background of Aspirin–Thrombolysis In Myocardial Infarction 54; THEMIS-PCI, The Effect of Ticagrelor on Health Outcomes in Diabetes Mellitus Patients Intervention Study- PCI; COMPASS, Cardiovascular Outcomes for People Using Anticoagulation Strategies; REDUCE-IT, Reduction of Cardiovascular Events With EPA–Intervention; EMPA-REG, Empagliflozin, Cardiovascular Outcomes, and Mortality in Type 2 Diabetes trial; CREDENCE, Canagliflozin and Renal Events in Diabetes and Nephropathy Clinical Evaluation; DECLARE TIMI 58, Dapagliflozin Effect on Cardiovascular Events A Multicenter, Randomized, Double-Blind, Placebo-Controlled Trial to Evaluate the Effect of Dapagliflozin 10 mg Once Daily on the Incidence of Cardiovascular Death, Myocardial Infarction or Ischemic Stroke in Patients With Type 2 Diabetes; LEADER, Liraglutide Effect and Action in Diabetes: Evaluation of Cardiovascular Outcome Results.
Residual Inflammatory Risk
Beyond LDL-C lowering, statins are known to have multiple pleiotropic effects. Of note, numerous trials demonstrated consistent anti-inflammatory effects. In the JUPITER trial (Justification for the Use of Statin in Prevention: an Interventional Trial Evaluating Rosuvastatin), rosuvastatin 20 mg daily reduced median high sensitivity C-reactive protein (hsCRP) by 37% as compared to placebo (3). Interestingly, the magnitude of hsCRP reduction achieved with rosuvastatin was proportional to the reduction in cardiovascular risk. Individuals who achieved hsCRP levels <2 mg/L demonstrated a 55% reduction in the primary endpoint compared to those with hsCRP levels ≥2 mg/L (p = 0.007); however, this benefit was not independent of LDL-C lowering. Similarly, in PROVE-IT TIMI 22 (Pravastatin or Atorvastatin Evaluation and Infection Therapy-Thrombolysis in Myocardial Infarction 22), those who achieved hsCRP levels <2 mg/L sustained fewer recurrent cardiovascular events (4). Whether the benefits of statins are related to LDL-C lowering, reduction in inflammation, or a combination of these factors remains a matter of debate.
In addition to hsCRP, one biomarker of inflammation, lipoprotein-associated phospholipase A2 (Lp-PLA2), is known to increase the production of pro-inflammatory and proapoptotic mediators within atherosclerotic plaques (5–10). In observational studies, the increase in Lp-PLA2 was associated with increased risk of adverse cardiovascular outcomes (6, 11). However, when a potent inhibitor of Lp-PLA2, darapladib, was tested in a randomized controlled trial in a cohort with stable coronary heart disease, there was no benefit seen in cardiovascular outcomes (12). Importantly, 96% of patients enrolled in the trial were on statins, which themselves are known to reduce LP-PLA2 by 35% (13–15). This inhibition of Lp-PLA2 with associated reduction of inflammation and plaque stabilization may be one of the several mechanisms through which statins exert their benefit.
The direct causal role of inflammation in cardiovascular disease was not formally proven until CANTOS (Canakinumab Anti-Inflammatory Thrombosis Outcome Study) (16). CANTOS enrolled 10,061 participants with a history of myocardial infarction (MI), optimized LDL-C, and hsCRP ≥ 2 mg/L and randomized them to optimal medical therapy (OMT) plus placebo vs. OMT plus canakinumab, a fully human monoclonal antibody targeted to interleukin-1β (IL-1β). Importantly, previous studies demonstrated that canakinumab has no effect on LDL-C. For the primary efficacy end-point of non-fatal myocardial infarction (MI), non-fatal stroke, or cardiovascular death, there was benefit observed with the 150 mg dose (HR 0.85, 95% CI 0.74–0.98, p = 0.021) and 300 mg dose (0.86 95% CI 0.75–0.99, p = 0.031) of canakinumab as compared to placebo. Importantly, lowering of hsCRP to levels <2 mg/L lead to a 25% reduction in major adverse cardiovascular events (MACE) and a 31% reduction in cardiovascular death and all-cause mortality, without any effect on LDL-C. There were non-significant reductions in mortality if hsCRP levels remained above 2 mg/L. A surprising finding was the reduction in cancer mortality associated with high dose (300 mg) canakinumab compared to placebo (HR 0.49, 95% CI 0.31–0.75; p = 0.0009), lung cancer mortality (HR 0.23, 95% CI 0.10–0.54; p = 0.0002), and incident lung cancer (HR 0.33, 95% CI 0.18–0.59; p < 0.0001), lending credence to the hypothesis that inflammation also plays a central role in the evolution of malignancy (17). Regarding safety, neutropenia and death due to sepsis were more common in the treatment arm than placebo (incidence rate 0.31 vs. 0.18 events per 100 person-years; p = 0.02). The FDA did not grant canakinumab an indication for cardiovascular risk reduction.
The Cardiovascular Inflammation Trial (CIRT) also sought to investigate the role of inflammation reduction in mitigating cardiovascular disease risk. In CIRT, over 3,000 subjects with a history of MI or multivessel coronary artery disease as well as type 2 diabetes mellitus or metabolic syndrome were randomized to OMT plus placebo vs. OMT plus low dose methotrexate (15–20 mg weekly) (18). In the treatment arm, there was no effect on cardiovascular events or all-cause mortality with low-dose methotrexate as compared to placebo. Importantly the median hsCRP in this trial was 1.5 mg/L at baseline, and at 8 months following randomization, there was no impact on blood levels of hsCRP, interleukin (IL)-6, or IL-1β. Taking the findings of CANTOS and CIRT together, inhibition of the IL-1β to IL-6 to hsCRP pathway achieved by canakinumab (but not methotrexate), appears to play a role critical in ASCVD (19).
A recent trial evaluating low-dose colchicine following MI, COLCOT (Colchicine Cardiovascular Outcomes Trial), demonstrated a reduction in the primary composite outcome of cardiovascular death, cardiac arrest, non-fatal MI, stroke, or angina leading to revascularization (HR 0.77; 95% CI 0.61–0.96; p = 0.02) (20). HsCRP was only measured in a subgroup of 207 patients, with a placebo-adjusted mean percent change of −10.1% of hsCRP at 6 months in those randomized to colchicine. The broad use of anti-inflammatories for prevention of cardiovascular events is not yet widely recommended, particularly in those without elevated systemic inflammation, though colchicine may ultimately provide a low-cost intervention for secondary prevention in select patients based on the findings above.
Recently, clonal hematopoiesis of indeterminate potential (CHIP) has emerged as a risk factor for ASCVD through inflammatory pathways (21). CHIP refers to clonal expansion of hematopoietic stem cells due to acquired somatic mutations, which occurs during the aging process. Deficiency of TET2 (tet methylcytosine dioxygenase 2), one of the genes associated with CHIP, was shown to promote atherogenesis in mouse models through an IL-1β-dependent and NLRP3 inflammasome- dependent mechanism (22). CHIP is surprisingly common, occurring in up to 20% of septuagenarians, and though it rarely transforms to acute leukemia (occurring 0.5–1% per year in carriers), CHIP confers a 40% increased risk of CVD and thus has emerged as a novel cardiovascular risk factor. Intense investigation is underway to determine optimal approaches to recognition and management of this non-traditional ASCVD risk factor (23).
Based on the discussion above as well as multiple observations implicating higher rates of ASCVD in those with chronic inflammatory diseases (e.g., rheumatoid arthritis, systemic lupus erythematosus, psoriasis, human immunodeficiency virus, etc.), there is growing recognition of the importance of the link between inflammation and cardiovascular disease. An important case study of the role of inflammation in ASCVD is the Tsimane, a pre-industrial population in Bolivia. Despite elevated inflammatory markers (51% of participants had a hs-CRP above 3.0 mg/L), this population had a 5-fold lower prevalence of coronary artery calcium than an industrialized population, likely attributed to a low prevalence of traditional risk factors (24). This example emphasizes the importance of traditional risk factors in risk assessment. In the 2019 American College of Cardiology (ACC)/American Heart Association (AHA) Primary Prevention guidelines, chronic inflammatory diseases are considered risk enhancers and may be considered when quantitative risk assessment using traditional risk factors appears to underestimate risk of ASCVD. As of 2019, there are no anti-inflammatory drugs with a formal indication for use in prevention of cardiovascular events, though clearly the inflammatory pathway is an important avenue for further research in cardiovascular disease prevention.
Residual Thrombotic Risk
Anticoagulation
The routine use of antiplatelet medications in primary prevention has come under scrutiny given recent data that suggest an unfavorable risk:benefit ratio when used in those without manifest cardiovascular disease (25–28). However, in patients with established ASCVD, antiplatelet agents are an essential component of optimal medical management. Despite appropriate antiplatelet use, usually with aspirin, risk of atherothrombosis and subsequent cardiovascular events remain (29). For example, in the Antithrombotic Trialists' Collaboration, which included 16 secondary prevention randomized trials of 17,000 patients, although aspirin did reduce the risk of serious vascular events, this endpoint still occurred in 6.7% of patients, as compared with 8.2% in the placebo group (30). As a result, there is interest in identification of patients that would benefit from intensification of antithrombotic or anticoagulant therapy.
Two trials have demonstrated the utility of adding low-dose anticoagulation to antiplatelet therapy. In ATLAS ACS 2-TIMI 51 (Anti-Xa Therapy to Lower Cardiovascular Events in Addition to Standard Therapy in Subjects with Acute Coronary Syndrome- Thrombolysis in Myocardial Infarction Trial 51), the addition of low dose rivaroxaban (2.5 or 5 mg twice a day) to antiplatelet therapy after a recent cardiovascular event (with 93% of patients on dual anti-platelet therapy) demonstrated a 16% relative risk reduction in major adverse cardiovascular events (31). However, this benefit was offset by an increase in major bleeding (2.1% vs. 0.6%, p < 0.001) and intracranial bleeding.
COMPASS (Cardiovascular Outcomes for People Using Anticoagulation Strategies) demonstrated that low-dose rivaroxaban plus aspirin vs. aspirin alone led to a 24% reduction in the primary outcome and a 22% reduction in the net clinical benefit endpoint (composite of primary outcome, fatal bleeding, and symptomatic bleeding into a critical organ/area) (32). Importantly, in a subgroup analysis, the magnitude of benefit was even more pronounced in those with peripheral arterial disease, a population with increased atherosclerotic burden generally affecting multiple vascular beds, and thus at higher risk of thrombotic events (33). Given these findings low-dose rivaroxaban appears to be a promising avenue for further reduction of residual thrombotic risk.
Dual Antiplatelet Therapy
Dual antiplatelet therapy (DAPT), with aspirin and a P2Y12 inhibitor, is a mainstay of therapy up to 1 year post percutaneous coronary intervention (PCI). The P2Y12 inhibitors that are used clinically are listed in Table 1. However, the benefit of extending DAPT beyond 12 months has been less clear. PEGASUS-TIMI 54 (Prevention of Cardiovascular Events in Patients with Prior Heart Attack Using Ticagrelor Compared to Placebo on a Background of Aspirin–Thrombolysis In Myocardial Infarction 54) demonstrated that the addition of ticagrelor to aspirin resulted in a 16% reduction in MACE (34). A meta-analysis of 6 trials evaluating the efficacy of long-term DAPT in the post-ACS stable cardiovascular disease setting demonstrated similar results (35). However, the benefits of prolonged DAPT were offset by an increased risk of major (but not fatal) bleeding.
THEMIS (The Effect of Ticagrelor on Health Outcomes in Diabetes Mellitus Patients Intervention Study) and its substudy in prior percutaneous coronary intervention (PCI) or coronary bypass patients, THEMIS-PCI, was presented at the European Society of Cardiology Congress in 2019. This study sought to identify populations that would receive greater benefit from DAPT (36). In patients with stable ischemic heart disease and diabetes, the addition of ticagrelor to aspirin in diabetic patients led to a reduction in MI (2.8% with ticagrelor plus aspirin vs. 3.4% with aspirin plus placebo). An exploratory composite outcome of irreversible harm, all-cause death, MI, stroke, fatal bleeding, or intracranial hemorrhage occurred in 10.1% of the ticagrelor + aspirin group compared with 10.8% of aspirin + placebo group. There was no difference in cardiovascular death, but the incidence of TIMI major bleeding was higher in the ticagrelor + aspirin group as compared to the aspirin + placebo group (2.2% vs. 1.0%, respectively, p < 0.001). THEMIS-PCI enrolled patients with diabetes and stable CAD with a history of PCI or coronary artery bypass, and thus enriched for a population that had tolerated DAPT previously (37). In this select population, the investigators demonstrated that fewer patients in the treatment arm suffered from the composite endpoint of cardiovascular death, MI, or stroke [7.3% in ticagrelor + aspirin arm vs. 8.6% in aspirin + placebo arm; HR 0.85 (95% CI 0.74–0.97), p = 0.013]. There was statistically significant higher occurrence of TIMI major bleeding in the treatment arm [HR 2.03 (95% CI 1.48–2.76), p < 0.0001] but fatal bleeding and intracranial hemorrhage did not differ between the two groups. Interestingly, ticagrelor improved net clinical benefit in patients with prior history of PCI (e.g., those more likely to have received DAPT previously).
Residual Metabolic Risk
Lipoprotein (a)
Lipoprotein (a) (Lp[a]) is an LDL-like particle covalently bound to apolipoprotein(a) [apo(a)], a non-functional mimic of plasminogen (38, 39). Lp(a) plays an active role in vascular atherothrombosis and its plasma levels are highly heritable (40). In the INTERHEART study, an Lp(a) level >50 mg/dL was associated with an increased risk of MI (OR 1.48, 95% CI 1.32–1.67, p < 0.001) (41). A subanalysis from JUPITER demonstrated that Lp(a) was a strong predictor of residual risk in patients already on a statin (adjusted HR 1.27; 95% CI 1.01–1.59, p = 0.04), independent of LDL-C and other risk factors (42). Whether Lp(a) should be a target of therapy remains a matter of debate. While the epidemiology and genetics suggest that Lp(a) is causal for ASCVD and calcific aortic stenosis (43–46), no randomized controlled trials have been performed to answer this question. Mendelian randomization studies demonstrate lower risk of ASCVD proportional to reduction in genetically conferred Lp(a) levels (46, 47), and genome-wide association studies demonstrate that single-nucleotide polymorphisms associated with the LPA locus are associated with ASCVD (48, 49). In FOURIER, evolocumab reduced Lp(a) levels by a median of 26.9%, which was moderately correlated with change in LDL-C (r = 0.37) (50). In patients with above the median baseline Lp(a), evolocumab was associated with a 23% reduction in the risk of CHD death, MI or urgent revascularization (compared to placebo), whereas only a 7% reduction was seen in those with Lp(a) below the median (P for interaction = 0.07). Importantly, in a post-hoc analysis of phase 3 ODYSSEY trials, Lp(a) reductions were not significantly associated with MACE reductions independently of LDL-C, except at the highest baseline values of Lp(a) (≥105 nmol/L), in whom reductions of Lp(a) using alirocumab translated to significant reductions in risk beyond LDL-C lowering (51, 52). Based on the available data, the AHA/ACC guideline on blood cholesterol recommend initiating moderate- to high-intensity statin therapy in adults aged 40–75 years with a 10 year ASCVD risk of 7.5% to ≤ 20% and Lp(a) ≥100 nmol/L. Additionally, high risk patients with LDL-C ≥70 mg/dL (non-HDL-C ≥100 mg/dL) and a Lp(a) ≥100 nmol/L on maximally tolerated statin should be considered for more intensive therapies (ezetimibe and PCSK9 inhibitors) to lower LDL-C (53).
Currently, novel therapies that selectively target Lp(a) are under development. A phase 2 trial of AKCEA apo(a)-LRx, an apo(a) antisense oligonucleotide, reduced Lp(a) up to 80% (54). A phase 3 study is being planned. Additionally, a therapeutic monoclonal antibody targeting oxidized phospholipids [of which Lp(a) represents the major plasma carrier] that binds and inactivates the pro-osteogenic activity of Lp(a) demonstrated promising in vitro data (55, 56). The cardiovascular outcomes trials of these novel therapies are awaited with interest.
Triglycerides and Remnant Cholesterol
Triglycerides and triglyceride-rich lipoproteins (TGRL) have also been identified as important contributors to residual risk. TGRLs are derived from the diet (chylomicrons and their remnants) and the liver (VLDL and their remnants) and circulate in the plasma (57). Lipoprotein lipase (LPL) lines the luminal surface of capillaries and hydrolyzes the TGs within the core of these TGRLs to free fatty acids (FFA) and glycerol. As FFA are liberated, the TGRL particles are remodeled physically (become smaller by losing TG and surface phospholipids) and chemically (become relatively cholesterol enriched). These partially lipolyzed TGRL are known as remnant particles (58).
Several studies have demonstrated an epidemiologic association between TGRLs and ASCVD. Additionally, a causal role was supported by Mendelian randomization studies (59). Moreover, remnant cholesterol, calculated as total cholesterol minus HDL-C minus LDL-C, consistently demonstrates an association with cardiovascular disease and mortality (60–62). TGRLs and their remnants are thought to be atherogenic through their ability to readily penetrate the arterial wall and direct uptake by arterial wall macrophages without further modification, in contrast to the oxidative modification required by arterial macrophages to take up LDL (63). Additionally, increased oxidative stress, impairment of endothelium-dependent vasodilation, activation of inflammation, and activation of the pro-thrombotic factors are all possible mechanisms explaining the pathogenicity of TGRLs (64–68).
There has been debate about the role of targeting triglycerides to improve cardiovascular outcomes. Trials with several triglyceride lowering therapeutics demonstrated inconsistent results (69–75). However, the use of high-dose, purified eicosapentaenoic acid (EPA) in populations with elevated triglycerides suggest a role in reducing residual cardiovascular risk. In JELIS (Japan EPA Lipid Intervention Study), an open-label blinded study of supplementation with 1.8 g/day of EPA and a statin (pravastatin 10 mg or simvastatin 5 mg) vs. statin alone in a primary prevention population, the investigators demonstrated a statistically significant (p = 0.01) 19% reduction in major coronary events (76). In a sub-analysis of JELIS evaluating individuals with triglycerides >150 mg/dL and a HDL-C <40 mg/dL, EPA treatment led to a large reduction in incident coronary artery disease (HR 0.47; 95% CI 0.23–0.98; p = 0.043), highlighting the potential benefit of this therapy from a primary prevention standpoint in individuals with elevated triglycerides and low HDL-C (76). Recently, the REDUCE-IT (Reduction of Cardiovascular Events With EPA–Intervention) trial enrolled patients with established ASCVD or diabetes with other risk factors and mild-moderate hypertriglyceridemia (77). All patients were on background statin therapy and had fasting triglyceride levels of 135 to 499 mg/dL and LDL-C levels of 41 to 100 mg/dL. Subjects were randomized to 4 grams of EPA daily or a mineral oil placebo. The intervention arm exhibited a 25% relative risk reduction in the primary composite endpoint of cardiovascular death, non-fatal MI, non-fatal stroke, coronary revascularization, or unstable angina (17.2% in the EPA group vs. 22.0% in the placebo group; HR 0.75, 95% CI 0.68–0.83; p < 0.001). Interestingly, the large reduction in the primary end-point was accompanied with only a modest decrease in plasma triglyceride concentration (median 18.3%, 39 mg/dL), suggesting that there may be additional mechanisms (e.g., antithrombotic, antiarrhythmic, antioxidant, anti-inflammatory, etc.) beyond triglyceride-lowering that lead to the improvement in cardiovascular outcomes. Moreover, the reduction in ASCVD events was similar regardless of whether or not triglycerides were reduced to below 150 mg/dL, providing further evidence supporting the effects were due to factors other than reduction in triglycerides. Importantly, STRENGTH (Outcomes Study to Assess Statin Residual Risk Reduction with EpaNova in HiGh CV Risk), a trial investigating the use of a Epanova, a formulation consisting of EPA + docoseahexaenoic acid, was stopped due to futility, suggesting that EPA itself may be the important component for risk reduction, as shown in REDUCE-IT.
High-Density Lipoprotein-Cholesterol
One of the most strikingly consistent relationships in cardiovascular epidemiology relates to the association of low levels high-density lipoprotein (HDL)-cholesterol (HDL-C) and ASCVD (78–80). In the Framingham cohort, there was a graded decrease in risk for every 1 mg/dL increase in HDL-C concentration (81). Although there had been hope that raising HDL-C levels would reduce cardiovascular risk, randomized controlled trials with niacin and cholesterol ester transfer protein inhibitors, while effective at raising HDL-C, did not lead to a reduction in major adverse cardiovascular events (82–87). Additionally, Mendelian randomization studies have not supported a causal relationship between HDL-C levels and cardiovascular disease (88, 89). To confuse the matter further, very high levels of HDL-C associate with poorer outcomes in large general population studies in Canada and Denmark (90, 91). The evolution in thinking suggests that functional measures of HDL may be more clinically useful than concentration. As proof of principle, one functional measurement of HDL, cholesterol efflux capacity, demonstrated the ability to predict both prevalent and incident coronary artery disease (92–95). In the Dallas Heart Study, in 2,924 patients without known ASCVD, there was a 67% reduction in cardiovascular risk in those in the highest quartile of cholesterol efflux capacity vs. the lowest quartile (hazard ratio, 0.33; 95% CI, 0.19–0.55). Importantly, cholesterol efflux capacity exhibited a weak correlation with HDL-C level (Spearman correlation coefficient of 0.07, p < 0.05). At present, low HDL-C (and perhaps at very high levels) does serve to identify patients at risk for adverse events, though at present no pharmacological treatment targeting HDL-C has proven effective in cardiovascular reducing risk. Currently, there are ongoing investigations with apolipoprotein A-1 infusion therapies to test if raising and/or improving the function of HDL can reduce residual risk, where prior efforts in raising HDL-C concentration had failed (96).
Diabetes
For years, none of the medications used to treat diabetes demonstrated the ability to reduce cardiovascular events and/or mortality. The advent of the glucagon like peptide 1 receptor agonists (GLP-1RA) and sodium-glucose cotransporter 2 inhibitors (SGLT2i) heralded a new era in diabetes care. EMPA-REG OUTCOME (Empagliflozin, Cardiovascular Outcomes, and Mortality in Type 2 Diabetes trial) in 2015 ushered in a paradigm shift in the treatment of diabetes and cardiovascular disease (97). Since that time, there have been a number of other trials that demonstrated improvement in cardiovascular outcomes with these two classes of drugs (Table 2) (98–102). An important consideration is that the substantial benefits seen with these medications occurred in a cohort in which a majority of patients were on optimal or near optimal background therapy per current guidelines. As an example, in EMPA-REG OUTCOME, 81% of subjects were on angiotensin-converting enzyme inhibitors (ACE-i) or angiotensin receptor blockers (ARB), 77% were on statins, and 82% were on aspirin. Despite this, in the placebo arm, the primary composite outcome of cardiovascular death, non-fatal MI, or non-fatal stroke occurred in 12.1% of the group, with 5.9% dying from cardiovascular causes over the 3 year observation time. Although empagliflozin was heralded as a great success for a 14% relative risk reduction of the primary outcome and a striking 38% relative risk reduction for cardiovascular death, these reductions were still associated with a significant proportion (10.5%) of participants sustaining major adverse cardiovascular events, with nearly 4% succumbing to death from cardiovascular causes. Although these trials signal an important advancement in the care of patients with diabetes, they also serve to highlight the substantial residual risk that exists despite adherence to standard of care therapies.
Likely, there are two different mechanisms of benefit between the two classes. SLGT2i exhibit their benefit early on following initiation and the benefit is seen with heart failure hospitalizations and significant slowing of decline in renal function. The possible explanations for these observations include osmotic diuresis leading to improved cardiac hemodynamics by reduction in left ventricular preload, lowering of body weight due to calorie and fluid losses, and lowering of blood pressure (103, 104). Another proposed mechanism of cardiovascular benefit may be a shift in fuel energetics from free fatty acids to ketones, which are a preferred substrate for myocardial cells (105). This improvement in metabolic efficiency is theorized to translate to cardiovascular benefit. GLP1-RA display their mortality benefit following a matter of months to years on atherosclerotic outcomes and do not appear to have a significant impact on heart failure endpoints. The mechanism for the anti-atherogenic effect of GLP1-RA is unclear, but the reduction of blood pressure, weight loss, and avoidance of hypoglycemia associated with these medications may contribute to improved cardiovascular outcomes (106).
Importantly, SGLT2i demonstrate benefit in individuals without diabetes as well, particularly in those with heart failure and reduced ejection fraction as demonstrated in the DAPA-HF study (Study to Evaluate the Effect of Dapagliflozin on the Incidence of Worsening Heart Failure or Cardiovascular Death in Patients with Chronic Heart Failure) (107). The application of the SGLT2i in patients without diabetes but with heart failure is an exciting area of active investigation.
As stated previously, an important consideration is that these novel medications displayed additive benefit to background therapy of proven cardioprotective medications (i.e., statins, ACEi/ARB). In a meta-analysis of over 18,000 patients with diabetes treated with statins, for each mmol/L (39 mg/dL) reduction in LDL-C due to statin therapy, there was a proportional 9% reduction in mortality and a 13% reduction in vascular mortality, irrespective of prior history of cardiovascular disease (108). As such, at least a moderate-intensity statin is indicated in all individuals with diabetes aged 40–75 years of age, with a high-intensity statin indicated for those with ASCVD or at high risk for ASCVD (10 year ASCVD risk ≥ 20%) (53, 109). Similarly, ACE-i/ARBs have demonstrated consistent reduction in cardiovascular events in hypertensive patients with diabetes, particularly in those with diabetic nephropathy, and as such are indicated in this population (13, 110). There is also an updated recommendation from the American Diabetes Association for use of EPA in patients with diabetes and cardiovascular disease or with risk factors on a statin with controlled LDL-C but elevated triglycerides (135–499 mg/dL) based on the strength of evidence presented above in REDUCE-IT (77, 111). As new therapies emerge that serve to incrementally reduce risk in this high-risk population, it is critical to ensure adherence to proven preventative therapies.
Conclusion
Identification and treatment of residual cardiovascular risk is critical to optimize patient outcomes, particularly in those at risk for recurrent events despite optimal treatment of traditional risk factors. The pathways discussed in this review represent sources of residual risk for the clinician to be mindful of when personalizing risk prediction and subsequent treatment for each patient. As we reach the limits of benefit of currently available therapies, it will be important to investigate and await the results of new approaches to managing residual cardiovascular risk. In the meantime, it seems prudent to recognize emerging risk factors and adopt new therapeutics that address some of these risk factors (i.e., EPA, SGLT2i/GLP1-ra, low-dose rivaroxaban).
Author Contributions
All authors listed have made a substantial, direct and intellectual contribution to the work, and approved it for publication.
Funding
DD and PS are funded by Abraham J. & Phyllis Katz Foundation (Atlanta, GA).
Conflict of Interest
The authors declare that the research was conducted in the absence of any commercial or financial relationships that could be construed as a potential conflict of interest.
References
1. Sabatine MS, Giugliano RP, Keech AC, Honarpour N, Wiviott SD, Murphy SA, et al. Evolocumab and clinical outcomes in patients with cardiovascular disease. N Engl J Med. (2017) 376:1713–22. doi: 10.1056/NEJMoa1615664
2. Schwartz GG, Steg PG, Szarek M, Bhatt DL, Bittner VA, Diaz R, et al. Alirocumab and Cardiovascular outcomes after acute coronary syndrome. N Engl J Med. (2018) 379:2097–107. doi: 10.1056/NEJMoa1801174
3. Ridker PM, Danielson E, Fonseca FAH, Genest J, Gotto AM, Kastelein JJP, et al. Rosuvastatin to prevent vascular events in men and women with elevated C-reactive protein. N Engl J Med. (2008) 359:2195–207. doi: 10.1056/NEJMoa0807646
4. Cannon CP, Braunwald E, McCabe CH, Rader DJ, Rouleau JL, Belder R, et al. Intensive versus moderate lipid lowering with statins after acute coronary syndromes. N Engl J Med. (2004) 350:1495–504. doi: 10.1056/NEJMoa040583
5. Carpenter KLH, Dennis IF, Challis IR, Osborn DP, Macphee CH, Leake DS, et al. Inhibition of lipoprotein-associated phospholipase A2 diminishes the death-inducing effects of oxidised LDL on human monocyte-macrophages. FEBS Lett. (2001) 505:357–63. doi: 10.1016/S0014-5793(01)02840-X
6. Lp-PLA(2) Studies Collaboration, Thompson A, Gao P, Orfei L, Watson S, Di Angelantonio E, et al. Lipoprotein-associated phospholipase A(2) and risk of coronary disease, stroke, and mortality: collaborative analysis of 32 prospective studies. Lancet. (2010) 375:1536–44. doi: 10.1016/S0140-6736(10)60319-4
7. Hsieh C-C, Yen M-H, Liu H-W, Lau Y-T. Lysophosphatidylcholine induces apoptotic and non-apoptotic death in vascular smooth muscle cells: in comparison with oxidized LDL. Atherosclerosis. (2000) 151:481–91. doi: 10.1016/S0021-9150(00)00453-6
8. Macphee CH, Moores KE, Boyd HF, Dhanak D, Ife RJ, Leach CA, et al. Lipoprotein-associated phospholipase A2, platelet-activating factor acetylhydrolase, generates two bioactive products during the oxidation of low-density lipoprotein: use of a novel inhibitor. Biochem J. (1999) 338:479–87. doi: 10.1042/bj3380479
9. Shi Y, Zhang P, Zhang L, Osman H, Mohler ER, Macphee C, et al. Role of lipoprotein-associated phospholipase A2 in leukocyte activation and inflammatory responses. Atherosclerosis. (2007) 191:54–62. doi: 10.1016/j.atherosclerosis.2006.05.001
10. Takahashi M, Okazaki H, Ogata Y, Takeuchi K, Ikeda U, Shimada K. Lysophosphatidylcholine induces apoptosis in human endothelial cells through a p38-mitogen-activated protein kinase-dependent mechanism. Atherosclerosis. (2002) 161:387–94. doi: 10.1016/S0021-9150(01)00674-8
11. Sabatine MS, Morrow DA, O'Donoghue M, Jablonksi KA, Rice MM, Solomon S, et al. Prognostic utility of lipoprotein-associated phospholipase A for cardiovascular outcomes in patients with stable coronary artery disease. Arterioscler Thromb Vasc Biol. (2007) 27:2463–9. doi: 10.1161/ATVBAHA.107.151670
12. STABILITY Investigators, White HD, Held C, Stewart R, Tarka E, Brown R, et al. Darapladib for preventing ischemic events in stable coronary heart disease. N Engl J Med. (2014) 370:1702–11. doi: 10.1056/NEJMoa1315878
13. Palmer SC, Mavridis D, Navarese E, Craig JC, Tonelli M, Salanti G, et al. Comparative efficacy and safety of blood pressure-lowering agents in adults with diabetes and kidney disease: a network meta-analysis. Lancet. (2015) 385:2047–56. doi: 10.1016/S0140-6736(14)62459-4
14. Ridker PM, MacFadyen JG, Wolfert RL, Koenig W. Relationship of lipoprotein-associated phospholipase A mass and activity with incident vascular events among primary prevention patients allocated to placebo or to statin therapy: an analysis from the JUPITER trial. Clin Chem. (2012) 58:877–86. doi: 10.1373/clinchem.2011.180281
15. Group HPSC. Lipoprotein-associated phospholipase A2 activity and mass in relation to vascular disease and nonvascular mortality. J Intern Med. (2010) 268:348–58. doi: 10.1111/j.1365-2796.2010.02258.x
16. Ridker PM, Everett BM, Thuren T, MacFadyen JG, Chang WH, Ballantyne C, et al. Antiinflammatory therapy with canakinumab for atherosclerotic disease. N Engl J Med. (2017) 377:1119–31. doi: 10.1056/NEJMoa1707914
17. Ridker PM, MacFadyen JG, Thuren T, Everett BM, Libby P, Glynn RJ, et al. Effect of interleukin-1β inhibition with canakinumab on incident lung cancer in patients with atherosclerosis: exploratory results from a randomised, double-blind, placebo-controlled trial. Lancet. (2017) 390:1833–42. doi: 10.1016/S0140-6736(17)32247-X
18. Ridker PM, Everett BM, Pradhan A, MacFadyen JG, Solomon DH, Zaharris E, et al. Low-dose methotrexate for the prevention of atherosclerotic events. N Engl J Med. (2018) 380:752–62. doi: 10.1056/NEJMoa1809798
19. Aday AW, Ridker PM. Targeting residual inflammatory risk: a shifting paradigm for atherosclerotic disease. Front Cardiovasc Med. (2019) 6:16. doi: 10.3389/fcvm.2019.00016
20. Tardif J-C, Kouz S, Waters DD, Bertrand OF, Diaz R, Maggioni AP, et al. Efficacy and safety of low-dose colchicine after myocardial infarction. (2019) 381:2497–505. doi: 10.1056/NEJMoa1912388
21. Jaiswal S, Natarajan P, Silver AJ, Gibson CJ, Bick AG, Shvartz E, et al. Clonal hematopoiesis and risk of atherosclerotic cardiovascular disease. N Eng J Med. (2017) 377:111–21. doi: 10.1056/NEJMoa1701719
22. Sano S, Wang Y, Walsh K. Clonal hematopoiesis and its impact on cardiovascular disease. Circulation J. (2019) 83:2–11. doi: 10.1253/circj.CJ-18-0871
23. Libby P, Sidlow R, Lin AE, Gupta D, Jones LW, Moslehi J, et al. Clonal hematopoiesis. crossroads of aging, cardiovascular disease, and cancer: review topic of the week. J Am Coll Cardiol. (2019) 74:567–77. doi: 10.1016/j.jacc.2019.06.007
24. Kaplan H, Thompson RC, Trumble BC, Wann LS, Allam AH, Beheim B, et al. Coronary atherosclerosis in indigenous South American Tsimane: a cross-sectional cohort study. Lancet. (2017) 389:1730–9. doi: 10.1016/S0140-6736(17)30752-3
25. McNeil JJ, Wolfe R, Woods RL, Tonkin AM, Donnan GA, Nelson MR, et al. Effect of aspirin on cardiovascular events and bleeding in the healthy elderly. N Engl J Med. (2018) 379:1509–18. doi: 10.1056/NEJMoa1805819
26. ASCEND Study Collaborative Group, Bowman L, Mafham M, Wallendszus K, Stevens W, Buck G Effects of aspirin for primary prevention in persons with diabetes mellitus. N Engl J Med. (2018) 379:1529–39. doi: 10.1056/NEJMoa1804988
27. Gaziano JM, Brotons C, Coppolecchia R, Cricelli C, Darius H, Gorelick PB, et al. Use of aspirin to reduce risk of initial vascular events in patients at moderate risk of cardiovascular disease (ARRIVE): a randomised, double-blind, placebo-controlled trial. Lancet. (2018) 392:1036–46. doi: 10.1016/S0140-6736(18)31924-X
28. Zheng SL, Roddick AJ. Association of aspirin use for primary prevention with cardiovascular events and bleeding events: a systematic review and meta-analysis. JAMA. (2019) 321:277–87. doi: 10.1001/jama.2018.20578
29. Gallone G, Baldetti L, Pagnesi M, Latib A, Colombo A, Libby P, et al. Medical therapy for long-term prevention of atherothrombosis following an acute coronary syndrome. J Am Coll Cardiol. (2018) 72:2886–903. doi: 10.1016/j.jacc.2018.09.052
30. Baigent C, Blackwell L, Collins R, Emberson J, Godwin J, Peto R, et al. Aspirin in the primary and secondary prevention of vascular disease: collaborative meta-analysis of individual participant data from randomised trials. Lancet. (2009) 373:1849–60. doi: 10.1016/S0140-6736(09)60503-1
31. Mega JL, Braunwald E, Wiviott SD, Bassand J-P, Bhatt DL, Bode C, et al. Rivaroxaban in patients with a recent acute coronary syndrome. N Engl J Med. (2011) 366:9–19. doi: 10.1056/NEJMoa1112277
32. Eikelboom JW, Connolly SJ, Bosch J, Dagenais GR, Hart RG, Shestakovska O, et al. Rivaroxaban with or without aspirin in stable cardiovascular disease. N Engl J Med. (2017) 377:1319–30. doi: 10.1056/NEJMoa1709118
33. Anand SS, Bosch J, Eikelboom JW, Connolly SJ, Diaz R, Widimsky P, et al. Rivaroxaban with or without aspirin in patients with stable peripheral or carotid artery disease: an international, randomised, double-blind, placebo-controlled trial. Lancet. (2018) 391:219–29. doi: 10.1016/S0140-6736(17)32409-1
34. Bonaca MP, Bhatt DL, Cohen M, Steg PG, Storey RF, Jensen EC, et al. Long-term use of ticagrelor in patients with prior myocardial infarction. N Engl J Med. (2015) 372:1791–800. doi: 10.1056/NEJMoa1500857
35. Palmerini T, Della Riva D, Benedetto U, Bacchi Reggiani L, Feres F, Abizaid A, et al. Three, six, or twelve months of dual antiplatelet therapy after DES implantation in patients with or without acute coronary syndromes: an individual patient data pairwise and network meta-analysis of six randomized trials and 11 473 patients. Eur Heart J. (2017) 38:1034–43. doi: 10.1093/eurheartj/ehw627
36. Steg PG, Bhatt DL, Simon T, Fox K, Mehta SR, Harrington RA, et al. Ticagrelor in patients with stable coronary disease and diabetes. N Engl J Med. (2019) 381:1309–20. doi: 10.1056/NEJMoa1908077
37. Bhatt DL, Steg PG, Mehta SR, Leiter LA, Simon T, Fox K, et al. Ticagrelor in patients with diabetes and stable coronary artery disease with a history of previous percutaneous coronary intervention (THEMIS-PCI): a phase 3, placebo-controlled, randomised trial. Lancet. (2019) 394:1169–80. doi: 10.1016/S0140-6736(19)31887-2
38. Steyrer E, Durovic S, Frank S, Giessauf W, Burger A, Dieplinger H, et al. Structural integrity of low density lipoproteins is a prerequisite for Lp(a) formation in human plasma. J Clin Investig. (1994) 94:2330–40. doi: 10.1172/JCI117598
39. Seimon TA, Nadolski MJ, Liao X, Magallon J, Nguyen M, Feric NT, et al. Atherogenic lipids and lipoproteins trigger CD36-TLR2-dependent apoptosis in macrophages undergoing endoplasmic reticulum stress. Cell Metab. (2010) 12:467–82. doi: 10.1016/j.cmet.2010.09.010
40. Tsimikas S, Tsironis LD, Tselepis AD. New insights into the role of lipoprotein(a)-associated lipoprotein-associated phospholipase A2 in atherosclerosis and cardiovascular disease. Arterioscler Thromb Vasc Biol. (2007) 27:2094–9. doi: 10.1161/01.ATV.0000280571.28102.d4
41. Pare G, Caku A, McQueen M, Anand SS, Enas E, Clarke R, et al. Lipoprotein(a) levels and the risk of myocardial infarction among 7 ethnic groups. Circulation. (2019) 139:1472–82. doi: 10.1161/CIRCULATIONAHA.118.034311
42. Khera AV, Everett BM, Caulfield MP, Hantash FM, Wohlgemuth J, Ridker PM, et al. Lipoprotein(a) concentrations, rosuvastatin therapy, and residual vascular risk: an analysis from the JUPITER Trial (Justification for the Use of Statins in Prevention: an Intervention Trial Evaluating Rosuvastatin). Circulation. (2014) 129:635–42. doi: 10.1161/CIRCULATIONAHA.113.004406
43. Thanassoulis G, Campbell CY, Owens DS, Smith JG, Smith AV, Peloso GM, et al. Genetic associations with valvular calcification and aortic stenosis. N Engl J Med. (2013) 368:503–12. doi: 10.1056/NEJMoa1109034
44. Kostner GM, Avogaro P, Cazzolato G, Marth E, Bittolo-Bon G, Qunici GB. Lipoprotein Lp(a) and the risk for myocardial infarction. Atherosclerosis. (1981) 38:51–61. doi: 10.1016/0021-9150(81)90103-9
45. Kamstrup PR, Benn M, Tybjaerg-Hansen A, Nordestgaard BG. Extreme lipoprotein(a) levels and risk of myocardial infarction in the general population: the Copenhagen City Heart Study. Circulation. (2008) 117:176–84. doi: 10.1161/CIRCULATIONAHA.107.715698
46. Burgess S, Ference BA, Staley JR, Freitag DF, Mason AM, Nielsen SF, et al. Association of LPA variants with risk of coronary disease and the implications for lipoprotein(a)-lowering therapies: a mendelian randomization analysis. JAMA Cardiol. (2018) 3:619–27. doi: 10.1016/j.atherosclerosis.2018.06.895
47. Lamina C, Kronenberg F, Lp-GWAS-Consortium ft. Estimation of the required lipoprotein(a)-lowering therapeutic effect size for reduction in coronary heart disease outcomes: a mendelian randomization analysis. JAMA Cardiol. (2019) 4:575–9. doi: 10.1001/jamacardio.2019.1041
48. Nikkola E, Ko A, Alvarez M, Cantor RM, Garske K, Kim E, et al. Family-specific aggregation of lipid GWAS variants confers the susceptibility to familial hypercholesterolemia in a large Austrian family. Atherosclerosis. (2017) 264:58–66. doi: 10.1016/j.atherosclerosis.2017.07.024
49. Liu Y, Ma H, Zhu Q, Zhang B, Yan H, Li H, et al. A genome-wide association study on lipoprotein (a) levels and coronary artery disease severity in a Chinese population. J Lipid Res. (2019) 60:1440–8. doi: 10.1194/jlr.P091009
50. O'Donoghue ML, Fazio S, Giugliano RP, Stroes ESG, Kanevsky E, Gouni-Berthold I, et al. Lipoprotein(a), PCSK9 inhibition, and cardiovascular risk. Circulation. (2019) 139:1483–92. doi: 10.1161/CIRCULATIONAHA.118.037184
51. Ray KK, Vallejo-Vaz AJ, Ginsberg HN, Davidson MH, Louie MJ, Bujas-Bobanovic M, et al. Lipoprotein(a) reductions from PCSK9 inhibition and major adverse cardiovascular events: pooled analysis of alirocumab phase 3 trials. Atherosclerosis. (2019) 288:194–202. doi: 10.1016/j.atherosclerosis.2019.06.896
52. Bittner VA, Szarek M, Aylward PE, Bhatt DL, Diaz R, Edelberg JM, et al. Effect of alirocumab on lipoprotein(a) and cardiovascular risk after acute coronary syndrome. J Am Coll Cardiol. (2020) 75:133–44. doi: 10.1016/j.jacc.2019.10.057
53. Grundy SM, Stone NJ, Bailey AL, Beam C, Birtcher KK, Blumenthal RS. 2018 AHA/ACC/AACVPR/AAPA/ABC/ACPM/ADA/AGS/APhA/ASPC/NLA/PCNA guideline on the management of blood cholesterol. A report of the American College of Cardiology/American Heart Association Task Force on Clinical Practice Guidelines. J Am Coll Cardiol. (2019) 2018:25709. doi: 10.1016/j.jacc.2018.11.002
54. Tsimikas S, Karwatowska-Prokopczuk E, Gouni-Berthold I, Tardif J-C, Baum SJ, et al. Lipoprotein(a) reduction in persons with cardiovascular disease. N Engl J Med. (2020) 382:244–55. doi: 10.1056/NEJMoa1905239
55. Viney NJ, van Capelleveen JC, Geary RS, Xia S, Tami JA, Yu RZ, et al. Antisense oligonucleotides targeting apolipoprotein(a) in people with raised lipoprotein(a): two randomised, double-blind, placebo-controlled, dose-ranging trials. Lancet. (2016) 388:2239–53. doi: 10.1016/S0140-6736(16)31009-1
56. Que X, Hung MY, Yeang C, Gonen A, Prohaska TA, Sun X, et al. Oxidized phospholipids are proinflammatory and proatherogenic in hypercholesterolaemic mice. Nature. (2018) 558:301–6. doi: 10.1038/s41586-018-0198-8
57. Toth PP. Triglyceride-rich lipoproteins as a causal factor for cardiovascular disease. Vascular health and risk management. (2016) 12:171–83. doi: 10.2147/VHRM.S104369
58. Rosenson RS, Davidson MH, Hirsh BJ, Kathiresan S, Gaudet D. Genetics and causality of triglyceride-rich lipoproteins in atherosclerotic cardiovascular disease. Journal of the American College of Cardiology. (2014) 64:2525–40. doi: 10.1016/j.jacc.2014.09.042
59. Musunuru K, Kathiresan S. Surprises from genetic analyses of lipid risk factors for atherosclerosis. Circul Res. (2016) 118:579–85. doi: 10.1161/CIRCRESAHA.115.306398
60. Varbo A, Freiberg JJ, Nordestgaard BG. Extreme nonfasting remnant cholesterol vs. extreme LDL cholesterol as contributors to cardiovascular disease and all-cause mortality in 90000 individuals from the general population. Clin Chem. (2015) 61:533–43. doi: 10.1373/clinchem.2014.234146
61. Jepsen AM, Langsted A, Varbo A, Bang LE, Kamstrup PR, Nordestgaard BG. Increased remnant cholesterol explains part of residual risk of all-cause mortality in 5414 patients with ischemic heart disease. Clin Chem. (2016) 62:593–604. doi: 10.1373/clinchem.2015.253757
62. Sarwar N, Danesh J, Eiriksdottir G, Sigurdsson G, Wareham N, Bingham S, et al. Triglycerides and the risk of coronary heart disease: 10,158 incident cases among 262,525 participants in 29 Western prospective studies. Circulation. (2007) 115:450–8. doi: 10.1161/CIRCULATIONAHA.106.637793
63. Miller YI, Choi SH, Fang L, Tsimikas S. Lipoprotein modification and macrophage uptake: role of pathologic cholesterol transport in atherogenesis. Sub Cellular Biochem. (2010) 51:229–51. doi: 10.1007/978-90-481-8622-8_8
64. Vogel RA, Corretti MC, Plotnick GD. Effect of a single high-fat meal on endothelial function in healthy subjects. Am J Cardiol. (1997) 79:350–4. doi: 10.1016/S0002-9149(96)00760-6
65. Anderson RA, Evans ML, Ellis GR, Graham J, Morris K, Jackson SK, et al. The relationships between post-prandial lipaemia, endothelial function and oxidative stress in healthy individuals and patients with type 2 diabetes. Atherosclerosis. (2001) 154:475–83. doi: 10.1016/S0021-9150(00)00499-8
66. Shin HK, Kim YK, Kim KY, Lee JH, Hong KW. Remnant lipoprotein particles induce apoptosis in endothelial cells by NAD(P)H oxidase-mediated production of superoxide and cytokines via lectin-like oxidized low-density lipoprotein receptor-1 activation: prevention by cilostazol. Circulation. (2004) 109:1022–8. doi: 10.1161/01.CIR.0000117403.64398.53
67. Olufadi R, Byrne CD. Effects of VLDL and remnant particles on platelets. Pathophysiol Haemost Thromb. (2006) 35:281–91. doi: 10.1159/000093221
68. Reiner Z. Hypertriglyceridaemia and risk of coronary artery disease. Nat Rev Cardiol. (2017) 14:401–11. doi: 10.1038/nrcardio.2017.31
69. Frick MH, Elo O, Haapa K, Heinonen OP, Heinsalmi P, Helo P, et al. Helsinki Heart Study: primary-prevention trial with gemfibrozil in middle-aged men with dyslipidemia. Safety of treatment, changes in risk factors, and incidence of coronary heart disease. N Eng J Med. (1987) 317:1237–45. doi: 10.1056/NEJM198711123172001
70. Rubins HB, Robins SJ, Collins D, Fye CL, Anderson JW, Elam MB, et al. Gemfibrozil for the secondary prevention of coronary heart disease in men with low levels of high-density lipoprotein cholesterol. Veterans Affairs High-Density Lipoprotein Cholesterol Intervention Trial Study Group. N Engl J Med. (1999) 341:410–8. doi: 10.1056/NEJM199908053410604
71. Keech A, Simes RJ, Barter P, Best J, et al. Effects of long-term fenofibrate therapy on cardiovascular events in 9795 people with type 2 diabetes mellitus (the FIELD study): randomised controlled trial. Lancet. (2005) 366:1849–61. doi: 10.1016/S0140-6736(05)67667-2
72. Bezafibrate Infarction Prevention (BIP) Study Group. Secondary prevention by raising HDL cholesterol and reducing triglycerides in patients with coronary artery disease. Circulation. (2000) 102:21–7. doi: 10.1161/01.CIR.102.1.21
73. Jun M, Foote C, Lv J, Neal B, Patel A, Nicholls SJ, et al. Effects of fibrates on cardiovascular outcomes: a systematic review and meta-analysis. Lancet. (2010) 375:1875–84. doi: 10.1016/S0140-6736(10)60656-3
74. Wang D, Liu B, Tao W, Hao Z, Liu M. Fibrates for secondary prevention of cardiovascular disease and stroke. Cochrane Database Syst Rev. (2015) 2015:Cd009580. doi: 10.1002/14651858.CD009580.pub2
75. Guyton JR, Slee AE, Anderson T, Fleg JL, Goldberg RB, Kashyap ML, et al. Relationship of lipoproteins to cardiovascular events: the AIM-HIGH Trial (Atherothrombosis Intervention in Metabolic Syndrome With Low HDL/High Triglycerides and Impact on Global Health Outcomes). J Am Coll Cardiol. (2013) 62:1580–4. doi: 10.1016/j.jacc.2013.07.023
76. Yokoyama M, Origasa H, Matsuzaki M, Matsuzawa Y, Saito Y, Ishikawa Y, et al. Effects of eicosapentaenoic acid on major coronary events in hypercholesterolaemic patients (JELIS): a randomised open-label, blinded endpoint analysis. Lancet. (2007) 369:1090–8. doi: 10.1016/S0140-6736(07)60527-3
77. Bhatt DL, Steg PG, Miller M, Brinton EA, Jacobson TA, Ketchum SB, et al. Cardiovascular risk reduction with icosapent ethyl for hypertriglyceridemia. N Engl J Med. (2019) 380:11–22. doi: 10.1056/NEJMoa1812792
78. Castelli WP, Garrison RJ, Wilson PW, Abbott RD, Kalousdian S, Kannel WB. Incidence of coronary heart disease and lipoprotein cholesterol levels. The Framingham Study. JAMA. (1986) 256:2835–8. doi: 10.1001/jama.256.20.2835
79. Ridker PM, Genest J, Boekholdt SM, Libby P, Gotto AM, Nordestgaard BG, et al. HDL cholesterol and residual risk of first cardiovascular events after treatment with potent statin therapy: an analysis from the JUPITER trial. Lancet. (2010) 376:333–9. doi: 10.1016/S0140-6736(10)60713-1
80. Di Angelantonio E, Sarwar N, Perry P, Kaptoge S, Ray KK, Thompson A, et al. Major lipids, apolipoproteins, and risk of vascular disease. JAMA. (2009) 302:1993–2000. doi: 10.1001/jama.2009.1619
81. Wilson PW, Abbott RD, Castelli WP. High density lipoprotein cholesterol and mortality. The Framingham Heart Study. Arteriosclerosis. (1988) 8:737–41. doi: 10.1161/01.ATV.8.6.737
82. Boden WE, Probstfield JL, Anderson T, Chaitman BR, Desvignes-Nickens P, Koprowicz K, et al. Niacin in patients with low HDL cholesterol levels receiving intensive statin therapy. N Engl J Med. (2011) 365:2255–67. doi: 10.1056/NEJMoa1107579
83. Landray MJ, Haynes R, Hopewell JC, Parish S, Aung T, Tomson J, et al. Effects of extended-release niacin with laropiprant in high-risk patients. N Engl J Med. (2014) 371:203–12. doi: 10.1056/NEJMoa1300955
84. Lincoff AM, Nicholls SJ, Riesmeyer JS, Barter PJ, Brewer HB, Fox KAA, et al. Evacetrapib and cardiovascular outcomes in high-risk vascular disease. N Engl J Med. (2017) 376:1933–42. doi: 10.1056/NEJMoa1609581
85. Schwartz GG, Olsson AG, Abt M, Ballantyne CM, Barter PJ, Brumm J, et al. Effects of dalcetrapib in patients with a recent acute coronary syndrome. N Engl J Med. (2012) 367:2089–99. doi: 10.1056/NEJMoa1206797
86. Bowman L, Hopewell JC, Chen F, Wallendszus K, Stevens W, Collins R, et al. Effects of anacetrapib in patients with atherosclerotic vascular disease. N Engl J Med. (2017) 377:1217–27. doi: 10.1016/j.jvs.2017.11.029
87. Barter PJ, Caulfield M, Eriksson M, Grundy SM, Kastelein JJ, Komajda M, et al. Effects of torcetrapib in patients at high risk for coronary events. N Engl J Med. (2007) 357:2109–22. doi: 10.1056/NEJMoa0706628
88. Holmes MV, Asselbergs FW, Palmer TM, Drenos F, Lanktree MB, Nelson CP, et al. Mendelian randomization of blood lipids for coronary heart disease. Eur Heart J. (2015) 36:539–50. doi: 10.1093/eurheartj/eht571
89. Voight BF, Peloso GM, Orho-Melander M, Frikke-Schmidt R, Barbalic M, Jensen MK, et al. Plasma HDL cholesterol and risk of myocardial infarction: a mendelian randomisation study. Lancet. (2012) 380:572–80. doi: 10.1016/S0140-6736(12)60312-2
90. Madsen CM, Varbo A, Nordestgaard BG. Extreme high high-density lipoprotein cholesterol is paradoxically associated with high mortality in men and women: two prospective cohort studies. Eur Heart J. (2017) 38:2478–86. doi: 10.1093/eurheartj/ehx163
91. Ko DT, Alter DA, Guo H, Koh M, Lau G, Austin PC, et al. High-density lipoprotein cholesterol and cause-specific mortality in individuals without previous cardiovascular conditions: the CANHEART Study. J Am Coll Cardiol. (2016) 68:2073–83. doi: 10.1016/j.jacc.2016.08.038
92. Rohatgi A, Khera A, Berry JD, Givens EG, Ayers CR, Wedin KE, et al. HDL cholesterol efflux capacity and incident cardiovascular events. N Engl J Med. (2014) 371:2383–93. doi: 10.1056/NEJMoa1409065
93. Shea S, Stein JH, Jorgensen NW, McClelland RL, Tascau L, Shrager S, et al. Cholesterol mass efflux capacity, incident cardiovascular disease, and progression of carotid plaque. Arterioscler Thromb Vasc Biol. (2019) 39:89–96. doi: 10.1161/ATVBAHA.118.311366
94. Saleheen D, Scott R, Javad S, Zhao W, Rodrigues A, Picataggi A, et al. Association of HDL cholesterol efflux capacity with incident coronary heart disease events: a prospective case-control study. Lancet Diabetes Endocrinol. (2015) 3:507–13. doi: 10.1016/S2213-8587(15)00126-6
95. Mutharasan RK, Thaxton CS, Berry J, Daviglus ML, Yuan C, Sun J, et al. HDL efflux capacity, HDL particle size, and high-risk carotid atherosclerosis in a cohort of asymptomatic older adults: the Chicago Healthy Aging Study. J Lipid Res. (2017) 58:600–6. doi: 10.1194/jlr.P069039
96. Capodanno D, Mehran R, Gibson CM, Angiolillo DJ. CSL112, a reconstituted, infusible, plasma-derived apolipoprotein A-I: safety and tolerability profiles and implications for management in patients with myocardial infarction. Expert Opin Investig Drugs. (2018) 27:997–1005. doi: 10.1080/13543784.2018.1543399
97. Zinman B, Wanner C, Lachin JM, Fitchett D, Bluhmki E, Hantel S, et al. Empagliflozin, cardiovascular outcomes, and mortality in type 2 diabetes. N Engl J Med. (2015) 373:2117–28. doi: 10.1056/NEJMoa1504720
98. Perkovic V, Jardine MJ, Neal B, Bompoint S, Heerspink HJL, Charytan DM, et al. Canagliflozin and renal outcomes in type 2 diabetes and nephropathy. N Engl J Med. (2019) 380:2295–306. doi: 10.1056/NEJMoa1811744
99. Wiviott SD, Raz I, Bonaca MP, Mosenzon O, Kato ET, Cahn A, et al. Dapagliflozin and cardiovascular outcomes in type 2 diabetes. N Engl J Med. (2019) 380:347–57. doi: 10.1056/NEJMoa1812389
100. Marso SP, Daniels GH, Brown-Frandsen K, Kristensen P, Mann JF, Nauck MA, et al. Liraglutide and cardiovascular outcomes in type 2 diabetes. N Engl J Med. (2016) 375:311–22. doi: 10.1056/NEJMoa1603827
101. Marso SP, Bain SC, Consoli A, Eliaschewitz FG, Jodar E, Leiter LA, et al. Semaglutide and cardiovascular outcomes in patients with type 2 diabetes. N Engl J Med. (2016) 375:1834–44. doi: 10.1056/NEJMoa1607141
102. Hernandez AF, Green JB, Janmohamed S, D'Agostino RB, Granger CB, Jones NP, et al. Albiglutide and cardiovascular outcomes in patients with type 2 diabetes and cardiovascular disease (Harmony Outcomes): a double-blind, randomised placebo-controlled trial. Lancet. (2018) 392:1519–29. doi: 10.1016/S0140-6736(18)32261-X
103. Neeland IJ, McGuire DK, Chilton R, Crowe S, Lund SS, Woerle HJ, et al. Empagliflozin reduces body weight and indices of adipose distribution in patients with type 2 diabetes mellitus. Diabetes Vasc Dis Res. (2016) 13:119–26. doi: 10.1177/1479164115616901
104. Tikkanen I, Narko K, Zeller C, Green A, Salsali A, Broedl UC, et al. Empagliflozin reduces blood pressure in patients with type 2 diabetes and hypertension. Diabetes Care. (2015) 38:420–8. doi: 10.2337/dc14-1096
105. Mudaliar S, Alloju S, Henry RR. Can a shift in fuel energetics explain the beneficial cardiorenal outcomes in the EMPA-REG OUTCOME study? A unifying hypothesis. Diabetes Care. (2016) 39:1115–22. doi: 10.2337/dc16-0542
106. Shah M, Vella A. Effects of GLP-1 on appetite and weight. Rev Endocr Metab Disord. (2014) 15:181–7. doi: 10.1007/s11154-014-9289-5
107. McMurray JJV, Solomon SD, Inzucchi SE, Køber L, Kosiborod MN, Martinez FA, et al. Dapagliflozin in patients with heart failure and reduced ejection fraction. N Engl J Med. (2019) 381:1995–2008. doi: 10.1056/NEJMoa1911303
108. Kearney PM, Blackwell L, Collins R, Keech A, Simes J, Peto R, et al. Efficacy of cholesterol-lowering therapy in 18,686 people with diabetes in 14 randomised trials of statins: a meta-analysis. Lancet. (2008) 371:117–25. doi: 10.1016/S0140-6736(08)60104-X
109. Baigent C, Blackwell L, Emberson J, Holland LE, Reith C, Bhala N, et al. Efficacy and safety of more intensive lowering of LDL cholesterol: a meta-analysis of data from 170,000 participants in 26 randomised trials. Lancet. (2010) 376:1670–81. doi: 10.1016/S0140-6736(10)61350-5
110. Catala-Lopez F, Macias Saint-Gerons D, Gonzalez-Bermejo D, Rosano GM, Davis BR, Ridao M, et al. Cardiovascular and renal outcomes of renin-angiotensin system blockade in adult patients with diabetes mellitus: a systematic review with network meta-analyses. PLoS Med. (2016) 13:e1001971. doi: 10.1371/journal.pmed.1001971
Keywords: residual risk, secondary prevention, primary prevention, inflammation, cardiovascular risk factors, cardiovascular disease, atherosclerosis, metabolic syndrome
Citation: Dhindsa DS, Sandesara PB, Shapiro MD and Wong ND (2020) The Evolving Understanding and Approach to Residual Cardiovascular Risk Management. Front. Cardiovasc. Med. 7:88. doi: 10.3389/fcvm.2020.00088
Received: 16 January 2020; Accepted: 22 April 2020;
Published: 13 May 2020.
Edited by:
Hendrik Tevaearai Stahel, Bern University Hospital, SwitzerlandReviewed by:
Hack-Lyoung Kim, Seoul Metropolitan Government - Seoul National University Boramae Medical Center, South KoreaDaniel Jean Louis Hayoz, Fribourg Cantonal Hospital, Switzerland
Copyright © 2020 Dhindsa, Sandesara, Shapiro and Wong. This is an open-access article distributed under the terms of the Creative Commons Attribution License (CC BY). The use, distribution or reproduction in other forums is permitted, provided the original author(s) and the copyright owner(s) are credited and that the original publication in this journal is cited, in accordance with accepted academic practice. No use, distribution or reproduction is permitted which does not comply with these terms.
*Correspondence: Devinder S. Dhindsa, ddhinds@emory.edu