- 1Department of Neuroscience, Imaging and Clinical Sciences, “G. D'Annunzio” University, Chieti, Italy
- 2Department of Clinical Sciences, Lund University, Clinical Research Center, Malmö, Sweden
- 3Casa di Cura Villa Serena, Città Sant'Angelo, Pescara, Italy
- 4Hypertension and Related Diseases Center, AOU-University of Sassari, Sassari, Italy
- 5Cardiac Intensive Care and Interventional Cardiology Unit, Santo Spirito Hospital, Pescara, Italy
- 6William Harvey Research Institute, NIHR Barts Biomedical Research Centre, Queen Mary University of London, London, United Kingdom
- 7Barts Heart Centre, St. Bartholomew's Hospital, Barts Health NHS Trust, London, United Kingdom
- 8Department of Life, Health and Environmental Science, University of L'Aquila, L'Aquila, Italy
The burden of pregnancy-related heart disease has dramatically increased over the last decades due to the increasing age at first pregnancy and higher prevalence of cardiovascular risk factors such as diabetes, hypertension, and obesity. Pregnancy is associated with physiological changes in the cardiovascular system, including hemodynamic, metabolic, and hormonal adaptations to meet the increased metabolic demands of the mother and fetus. It has been postulated that pregnancy may act as a cardiovascular stress test to identify women at high risk for heart disease, where the inability to adequately adapt to the physiologic stress of pregnancy may reveal the presence of genetic susceptibility to cardiovascular disease or accelerate the phenotypic expression of both inherited and acquired heart diseases, such as peripartum cardiomyopathy (PPCM). PPCM is a rare and incompletely understood clinical condition. Despite recent advances in the understanding of its pathogenesis, PPCM is not attributable to a well-defined pathological mechanism, and therefore, its diagnosis still relies on the exclusion of overlapping dilated phenotypes. Cardiac imaging plays a key role in any peripartum woman with signs and symptoms of heart failure in establishing the diagnosis, ruling out life-threatening complications, guiding therapy and conveying prognostic information. Echocardiography represents the first-line imaging technique, given its robust diagnostic yield and its favorable cost-effectiveness. Cardiovascular magnetic resonance is a biologically safe high-throughput modality that allows accurate morpho-functional assessment of the cardiovascular system in addition to the unique asset of myocardial tissue characterization as a pivotal piece of information in the pathophysiological puzzle of PPCM. In this review, we will highlight current evidence on the role of multimodality imaging in the differential diagnosis, prognostic assessment, and understanding of the pathophysiological basis of PPCM.
Key Points
- Peripartum cardiomyopathy is a rare but potentially fatal disease requiring prompt identification and treatment.
- Cardiac imaging plays a pivotal role for the diagnosis, risk stratification, and follow-up of peripartum cardiomyopathy and related complications.
- Cardiovascular magnetic resonance is a high-throughput imaging modality providing relevant information for clinical decision-making and understanding of the pathophysiology underlying peripartum cardiomyopathy.
Introduction
Cardiovascular diseases (CVDs) represent the main cause of maternal morbidity and mortality during or early after pregnancy in western countries (1–3). This remains an unacceptable price to pay for motherhood. After the initial description of heart failure (HF) development during pregnancy, the term “peripartum cardiomyopathy” (PPCM) was firstly introduced by Demakis et al. about 50 years ago (4). Since then, our knowledge of the pathophysiological framework of PPCM, although still incomplete, has noticeably increased, and substantial progress has been made toward improved diagnosis and treatment of this elusive disease.
According to the international guidelines (5–8), PPCM is defined by symptomatic left ventricular (LV) systolic dysfunction, with LV ejection fraction (LVEF) usually <45%, with or without LV enlargement, developing during the last month of pregnancy or in the first 5 months after delivery, abortion, or miscarriage in women without previously known heart disease. This definition entails two important requirements: firstly, the assessment of LV systolic dysfunction with cardiac imaging; secondly, the ascertainment of pre-existing maternal CVD.
PPCM is a rare disease with a generally accepted incidence of nearly 1 in 1,000–4,000 live births in western countries (9). However, the incidence is highly variable across different geographical areas (10), likely reflecting specific genetic susceptibility to different environmental influences. Currently, the incidence of PPCM is rising also in western countries, where it represents a non-negligible cause of pregnancy-associated HF and maternal death (11). At present, there is no recognized cause for PPCM, so that the diagnosis still relies on the exclusion of other specific conditions (5). Several hypotheses have been discussed (autoimmune, myocarditis, malnutrition, genetic altered prolactin formation), with familiar forms having been reported. Recently, a vasculo-hormonal hypothesis has been proposed where multiple signaling pathways may be deregulated in late gestation, causing angiogenic imbalance eventually leading to cardiac dysfunction in genetically predisposed individuals (10, 12–14). According to this hypothesis, the prolactin inhibitor bromocriptine shows promise in the treatment of PPCM (15); however, despite early promising results, specific biomarkers and therapeutic targets are lacking (16, 17).
Along with patients' medical history, physical examination, electrocardiogram (ECG), and B-type natriuretic peptide assessment (18), cardiac imaging plays a key role for the clinical evaluation of peripartum women with symptoms and signs of HF (Figure 1, Table 1). Echocardiography is the first-line diagnostic imaging modality given its wide availability, biological safety, and robust diagnostic yield in HF patients (6, 19). Noticeably, comprehensive cardiopulmonary ultrasound examination allows biventricular systolic function assessment, early detection of subclinical hemodynamic derangements, monitoring of extravascular lung water and left atrial pressure, and prompt identification of complications such as thrombosis. Cardiovascular magnetic resonance (CMR) without administration of gadolinium-based contrast agents is a second-tier imaging modality that can be safely performed during pregnancy (20). CMR outperforms echocardiography (i) in the assessment of cardiac function, flow, and volumes, (ii) in the identification of intracardiac thrombi, and (iii) in detecting and monitoring signs of acute myocardial inflammation (Figures 2–4).
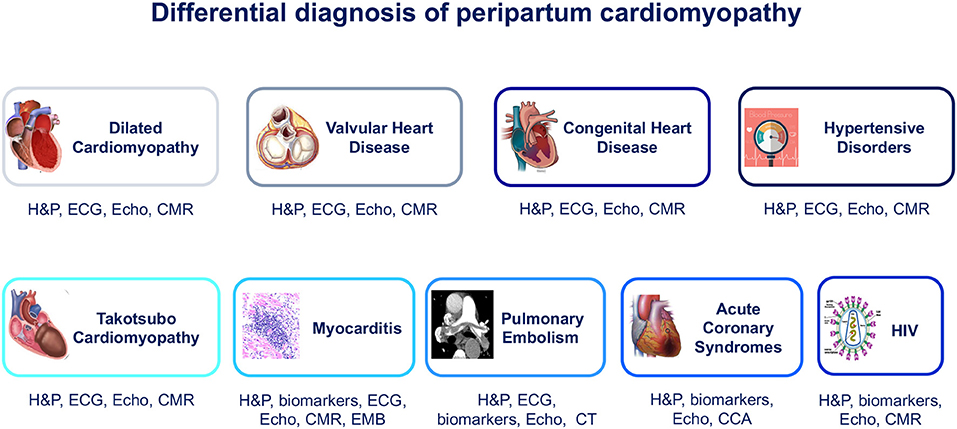
Figure 1. Differential diagnosis of peripartum cardiomyopathy. CCA, conventional coronary angiography; CMR, cardiac magnetic resonance; CT, computed tomography; ECG, electrocardiogram; EMB, endomyocardial biopsy; H&P, history and physical examination; HIV, human immunodeficiency virus; VHD, valvular heart disease.
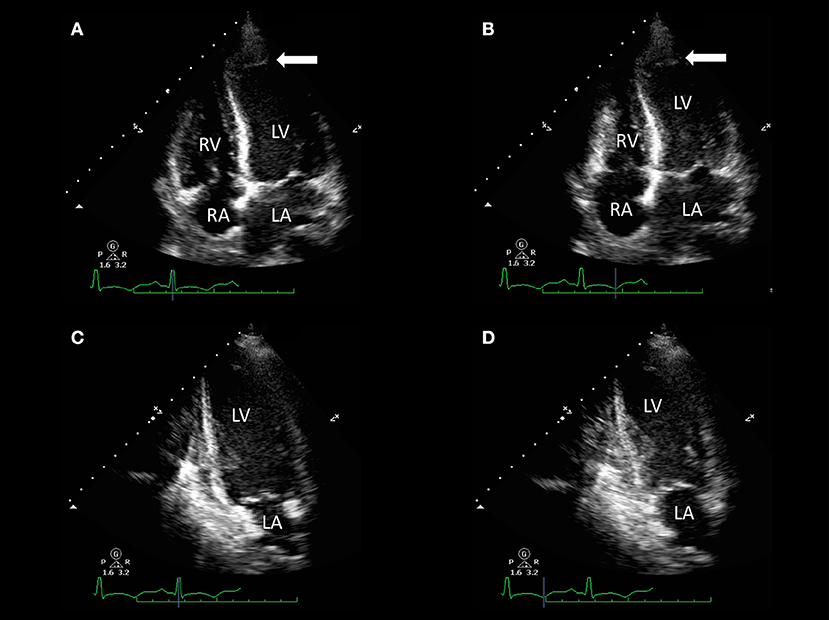
Figure 2. Transthoracic echocardiography of a 32-year-old woman with acute dyspnea 1 day after delivery. Apical four-chamber view [end-diastolic (A) and end-systolic (B) frames] and two-chamber view [end-diastolic (C) and end-systolic (D) frames] show akinesia of apical segments of the left ventricle (LV) and mild-to-moderate LV systolic impairment [LV ejection fraction (LVEF) 45%] with evidence of apical LV thrombus (arrows). Resting right ventricle (RV) function was preserved (fractional area change 48%).
Review Methodology
Authors performed a narrative review and searched Medline from January 1966 through August 2019 for potentially relevant articles using the following search terms: (peripartum cardiomyopathy OR pregnancy) AND (echocardiography OR cardiovascular magnetic resonance OR computed tomography OR nuclear OR cardiovascular imaging). References of selected papers were screened, searching for other potentially relevant publications. Websites, including escardio.org and ResearchGate, as well as reference lists of all identified articles, including hand-searching of reviews and previous meta-analyses, were also appraised for additional relevant studies. Four authors screened a list of potentially relevant articles based on title, abstract, and full-text review. A selection of the most relevant papers was finally included in the review according to authors' opinion.
Echocardiography
Pregnancy produces prominent cardiovascular adaptations that become particularly evident in the last trimester and peripartum period. These changes include increase of LV mass, dilation of cardiac chambers, increase in cardiac output, and elevation of LV filling pressure due to fluid volume overload (6, 22). For its wide availability and low cost, echocardiography, also with recently introduced three-dimensional (3-D), tissue velocity imaging and myocardial strain analysis (23), is the most commonly used imaging method for cardiac chamber quantification (24). It provides real-time information on atrioventricular size and function, valvular apparatus, and other cardiac structures and forms the first-line imaging modality for the evaluation of pregnant women with suspected CVDs (6). Echocardiography plays a key role in the differential diagnosis between PPCM and other pregnancy-related cardiac diseases such as preeclampsia (25), in which LV systolic function is mostly unaffected, valvular heart diseases (VHDs), and congenital heart diseases (CHDs), and enables long-term monitoring of cardiac function (26) (Table 2).
Although LV systolic dysfunction is observed in the vast majority of PPCM patients, as reduced LVEF is a major criterion for the diagnosis (5), several studies reported unexplained HF with preserved LVEF in peripartum women, suggesting the possibility of progressive myocardial disease development across different clinical stages (27–29) and raising the challenge for early diagnosis.
Strain has been validated as an important echocardiographic parameter with diagnostic and prognostic utility in various cardiac conditions. In this context, myocardial strain analysis would be the ideal tool for unmasking early myocardial impairment associated with various degrees of myocardial inflammation, oxidative stress, and fibrosis eventually driving the transition to overt HF. Accordingly, in a single-center experience, global longitudinal strain (GLS) was significantly lower in PPCM patients compared with healthy peripartum women regardless of LVEF values (28). This holds major promise in tracking early remodeling in PPCM and identifying a window of opportunity for preventive measures.
LV thrombosis is a common finding in PPCM, due to the coexistence of multiple predisposing factors. Although CMR has been proven to be superior to both transthoracic and transesophageal echocardiography in the detection of LV thrombi (30), echocardiographic evaluation of PPCM patients should always include a systematic assessment for this life-threatening complication. The use of 3-D techniques provides further morphological information compared to standard 2-D echocardiography and may be of additional benefit in the detection of intracardiac thrombi (31–33). The use of contrast echocardiography may improve the detection of thrombosis through the identification of an LV filling defect (34). Nevertheless, CMR remains more sensitive than echocardiography for the detection of intracardiac thrombus, especially in the case of mural or smaller thrombi (35, 36). Compared with late gadolinium enhancement (LGE) CMR as the reference standard, echocardiography has been shown to have a sensitivity of 24–37% when contrast is not used, with a higher sensitivity of <65% when contrast is used (36).
Despite PPCM-related outcome appearing to be favorable compared with other cardiomyopathies (37), with most patients showing full recovery within 6 months, current evidence for long-term outcome is based mostly on single-center studies or small registries (38). Echocardiography conveys important prognostic information useful for risk stratification (Table 3), and several echocardiographic parameters have been associated with worse outcomes or risk of relapse in subsequent pregnancies.
The results of the Investigations of Pregnancy-Associated Cardiomyopathy (IPAC) study, a prospective multicenter study on 100 PPCM patients, highlighted that baseline LVEF <30% and LV dilatation with a left ventricular end-diastolic diameter (LVEDD) >60 mm were strongly associated with lower rates of both 12-month event-free survival and LV functional recovery (39). Similar findings have been reproduced in other studies, although the effect of racial and ethnic differences requires future research (40–43).
PPCM is associated with risk of recurrence during subsequent pregnancies and requires adequate counseling (38). Several studies demonstrated that persistent LV systolic impairment is a strong predictor of poor cardiovascular outcome in this setting (44). In a retrospective study of 44 women undergoing a subsequent pregnancy, the risk of adverse maternal or fetal outcomes was substantially higher in those with persistent LV systolic dysfunction (45). Larger single-center and multicenter prospective studies (46, 47) confirmed the prognostic impact of LVEF on the outcome of subsequent pregnancies, where partial recovery of LV function (LVEF <50%) was a harbinger of higher mortality. Despite full recovery of resting LVEF, the residual risk of recurrent HF has been documented and linked to the persistence of subclinical systolic dysfunction. This has been shown in a cohort of 29 women with previous PPCM presenting with lower tissue Doppler velocities and GLS of both the LV and right ventricle (RV) despite full LVEF recovery 12 months after the index event (48).
The role of pharmacological stress testing during pregnancy is currently limited, with conflicting data on the prognostic value of LV contractile reserve assessment by dobutamine stress echocardiography (DSE). In a series of seven patients, the presence of inotropic contractile reserve—defined as the improvement of wall motion score in ≥2 segments—was a better predictor than resting LVEF of LV functional recovery during follow-up (49). Conversely, in another cohort of nine patients, resting LVEF was the sole predictor of LV functional recovery at follow-up (50). Small sample sizes, different DSE protocols, and clinical characteristics of the two patient populations may explain the conflicting results. Further larger prospective studies are needed to clarify the role of DSE for risk stratification and prognostic assessment of PPCM.
RV involvement identifies a PPCM phenotype with worse outcome. Echocardiography is a cost-effective alternative to CMR for RV function assessment in PPCM patients, particularly in those countries where PPCM is relatively more frequent and with limited economic resources (51). In the large IPAC cohort (52), the presence of RV systolic dysfunction was associated with persistent LV systolic impairment and poor outcome, and among different RV functional parameters, fractional area change (FAC) was the sole independent predictor of clinical outcome. The prognostic significance of baseline RV dysfunction was confirmed in a retrospective cohort study analysis of 53 patients (43) where moderate-to-severe RV systolic dysfunction was associated with 12-month higher risk of left ventricular assist device (LVAD) implantation, heart transplantation, or death. This evidence would suggest routine RV functional evaluation with FAC calculation in order to classify PPCM patients at higher risk of hemodynamic instability that may benefit from early advanced HF therapy. Bromocriptine treatment seems to be a promising option also in the context of biventricular dysfunction, although further placebo-controlled trials are needed (53).
Echocardiography is useful for counseling purposes and for risk stratification of PPCM women undergoing a subsequent pregnancy, individuating those that may benefit more from strict follow-up and timely treatment, also in the case of apparent full recovery of global LV systolic function (Table 2 and Figure 2).
Cardiovascular Magnetic Resonance
In the last decade, CMR has emerged as the gold standard technique for cardiac chamber quantification and flow assessment, but the key advantage of CMR is its unique ability to provide information about the tissue composition by myocardial tissue characterization with high spatial resolution, excellent accuracy, and reproducibility (54). Accordingly, CMR is the best alternative imaging modality in patients with non-diagnostic or doubtful echo, but also an indispensable diagnostic tool in the workup of dilated cardiomyopathy (DCM). CMR has demonstrated its usefulness in the evaluation of physiological and pathological maternal cardiovascular adaptations to pregnancy (55), with reference values for ventricular volumes and tissue composition in healthy pregnancies and postpartum states having been published (21, 56). CMR imaging is considered safe during pregnancy, as magnetic energy has been shown not to be harmful for the developing fetus. Conversely, the effect of gadolinium-based contrast agent administration on the fetus is not well-established, so that its administration during pregnancy preferably should be avoided, especially during the first trimester (57, 58). Nevertheless, gadolinium-based contrast agents are considered safe during breastfeeding (59). LGE imaging allows identification of myocardial scarring (60). Despite the absence of a specific PPCM scar pattern, CMR may be useful for exclusion of other diseases such as myocarditis or Takotsubo syndrome that may have substantial phenotypic overlap with PPCM (61). Morpho-functional assessment of the RV is way more accurate by CMR and may be of prognostic value in PPCM. CMR therefore should be considered, in addition to ultrasound assessment when RV involvement is suspected, in the workup of alternative diagnosis—such as myocarditis or LV thrombosis—or in the case of poor-quality ultrasound images. However, regrettably, CMR remains underused for diagnosis and risk stratification of PPCM in clinical practice (Table 2).
The first case of CMR assessment of PPCM was described in 2008 (62). Authors reported the presence of LV systolic dysfunction with non-ischemic LGE and borderline myocarditis at endomyocardial biopsy, in keeping with the hypothesis of an inflammatory process playing in the background. CMR examination was performed at 2 and 10 months after symptom onset with evidence of significant LGE reduction at follow-up. Another study found that the presence of LGE was an independent predictor of persistently reduced LV systolic function at follow-up (63).
In another case series, CMR could identify neither LGE nor signs of myocardial edema in the entire study cohort. However, it should be acknowledged that CMR was performed beyond 2 weeks from HF onset in most patients, and nearly 40% of the study population presented with pregnancy-induced hypertension, hence not entirely meeting PPCM diagnostic criteria (64).
Supporting the hypothesis of the time dependence of detection of tissue composition alteration, both diffusely increased T2 and early gadolinium enhancement ratios (markers of myocardial edema) have been documented at the initial evaluation of women with PPCM (65). LGE was not present in the acute phase, but it has been reported during follow-up in a woman with persistently elevated T2 ratio. The presence of both LGE and high T2 ratio was also associated with persistently impaired LV function.
In a series of 10 patients with established PPCM, CMR revealed LGE with non-ischemic distribution in 40% of cases. LGE was associated with higher risk of HF hospitalization and HF exacerbation during subsequent pregnancies (66). An elevated T2 ratio was detected in one of the four patients that underwent examination in the acute phase, with complete resolution at 4 months and in the absence of residual LGE.
In a larger CMR study enrolling 34 PPCM women, most patients presented with LVEF <35%, LV dilatation, and regional wall motion abnormalities (67). The observed prevalence of non-ischemic LGE and myocardial edema in this population was 70 and 25%, respectively, which was significantly higher compared with other non-ischemic cardiomyopathies. Interestingly, areas of pathological tissue composition matched regional wall motion abnormalities and were mainly located at the basal and mid-anteroseptal LV segments, in keeping with a reverse-Takotsubo pattern. About one-third of patients showed impaired RV systolic function, which was a harbinger of reduced chance of functional recovery. In another prospective multicenter study (60), the prevalence of LGE was quite low, likely because of the different time interval between symptom onset and CMR between studies.
CMR is also useful in the detection of intracardiac thrombosis. LV thrombosis is a serious complication of PPCM with potentially devastating consequences. The reported prevalence of LV thrombosis is highly variable, ranging between 10 and 30% (9). PPCM confers a higher risk of systemic thromboembolism compared to other cardiomyopathies (68) due to prolonged immobility, postpartum hypercoagulability, and reduced LV function. CMR is more accurate than transthoracic echocardiography for the identification of LV thrombus (30, 35), allowing early treatment and adequate follow-up. In a Danish study aimed at comparing the long-term CMR findings among PPCM, preeclampsia, and uncomplicated pregnancies (69), it has been shown that the prevalence of LGE was quite low and the rate of delayed functional recovery was noticeably high in PPCM, raising the issue of optimal timing for ICD implantation in primary prevention. Furthermore, CMR documented persistent diastolic dysfunction in PPCM compared with preeclampsia.
Current evidence suggests that the utility of phenotyping PPCM by CMR imaging is manifold and should be aimed at establishing a correct diagnosis, improving risk stratification, detecting thrombotic complications, and planning appropriate follow-up (Figures 3, 4). Furthermore, tissue characterization by CMR might be helpful to clarify the complex and multifactorial pathogenesis of PPCM distinguishing specific disease processes in PPCM and related phenocopies (70).
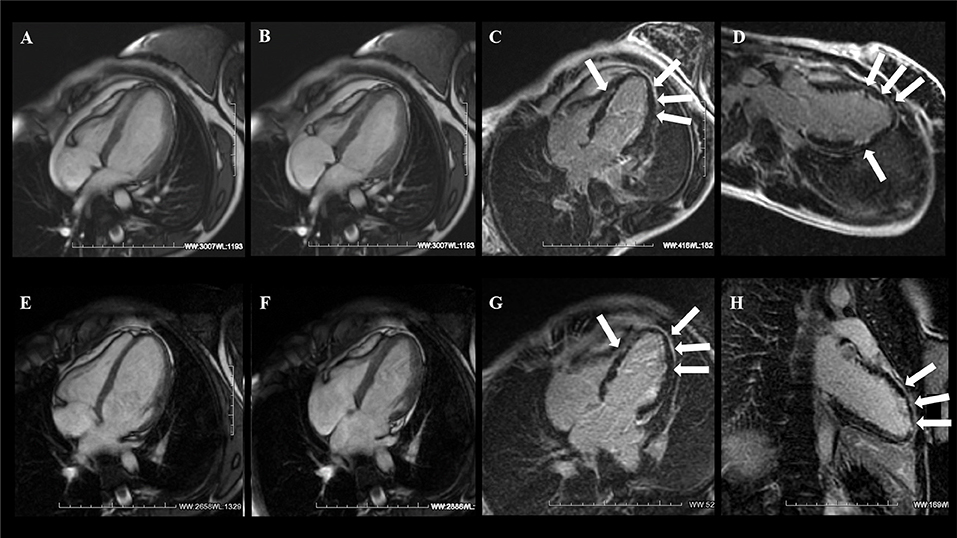
Figure 3. A 21-year-old woman with sudden onset of dyspnea 1 week after delivery and without prior medical history. CMR examination with cine balanced steady-state free precession (bSSFP) sequence four-chamber view [end-dyastole (A) and end-systole (B)] showed severely reduced LV systolic function without RV involvement. (C,D) T1-weighted inversion recovery gradient echo (T1w-IR-GRE) sequence acquired 10 min after gadolinium administration showed diffuse subendocardial late gadolinium enhancement (LGE) of the LV (arrows). At 6 months' follow-up, there was evidence of LV functional recovery (E,F) with diffuse LGE persistence (G,H). Courtesy of Dr. Giovanni Donato Aquaro, Fondazione Toscana Gabriele Monasterio, Pisa, Italy.
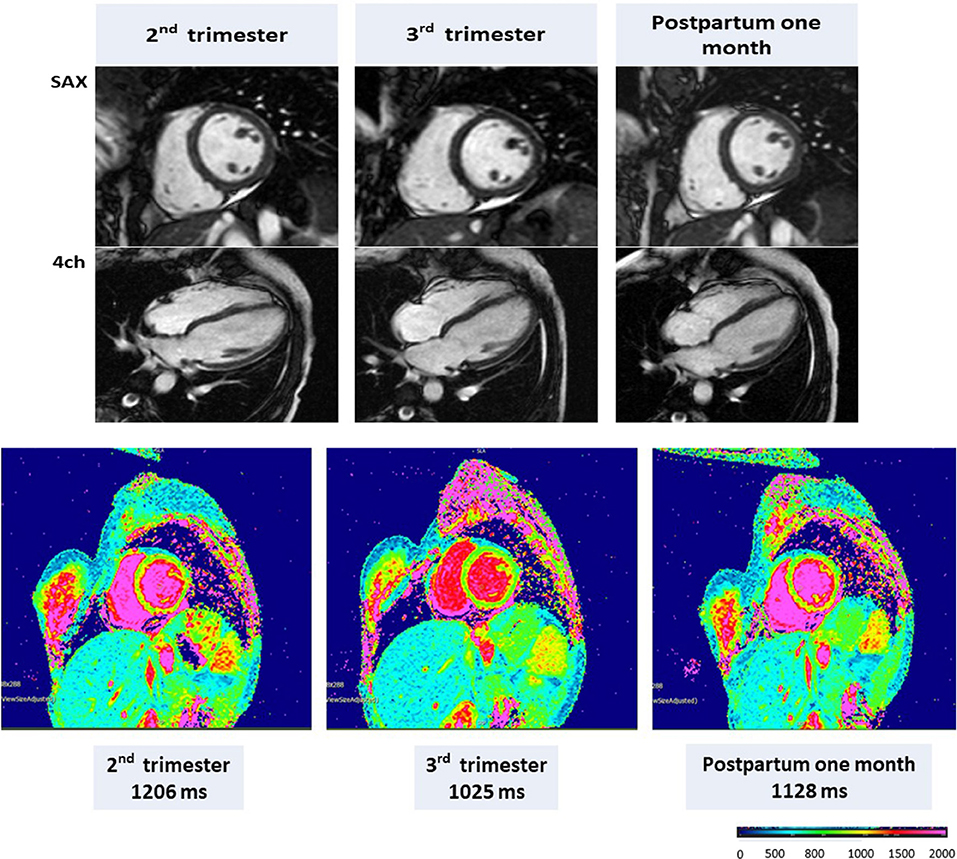
Figure 4. CMR in a representative pregnant woman with end-diastolic volume (EDV), end-systolic volume (ESV), ejection fraction (EF), and left ventricular mass (LVM), respectively, of 132 mL, 69 mL, 48.0%, and 106 g during the second trimester; 136 mL, 60 mL, 56%, and 95 g during the third trimester; and 112 mL, 57 mL, 54%, and 76 g at 1 month postpartum. Native T1 mapping (1.5 T) using MOLLI with a native T1 of 1,206 ms in the second trimester, 1,025 ms in the third trimester, and 1,128 ms at 1 month postpartum. Adapted from Nii et al. (21).
Cardiac Imaging Modalities With Ionizing Radiation
Ionizing radiation exposure during pregnancy may be harmful for both mother and fetus. In pregnant or lactating women, the risk profile of radiation exposure is similar to that of non-pregnant women, with the exception of breast tissue. Given pregnancy-induced proliferation, it has been postulated that breast tissue of such patients is more sensitive to radiation, although the entity of this effect remains unclear (71). The consequences of fetal radiation exposure include spontaneous abortion, teratogenesis, and carcinogenesis. The effect depends on both gestational age and absorbed dose. While there is no minimum threshold below which there is no increased cancer risk, a fetal absorbed dose of <50 mGy is actually considered safe in terms of abortive and teratogen effects (72, 73). With the adoption of dose-reduction protocols, such a threshold is usually not exceeded in most radiographic procedures unless repeated examinations are necessary (74). Currently used iodinated contrast agents are able to cross the placenta, but no teratogenic effects have been reported (75). Such drugs should be used when they may contribute to diagnosis and benefits outweigh the risks (74).
Non-ionizing imaging methods should be preferred whenever possible, and if radiographic or nuclear techniques are necessary, the risk/benefit profile must be carefully evaluated on an individual basis without exposing patients to potentially life-threatening consequences of delayed diagnosis.
Chest x-ray represents the initial radiographic imaging method in the evaluation of the vast majority of pregnant women with HF when other radiation-free techniques are unable to clarify symptoms (6). Both maternal and fetal absorbed radiation doses are very low and without clinical consequence.
The role of other radiographic and nuclear imaging methods in PPCM is limited, and these techniques are usually not required. However, computed tomography (CT), ventilation/perfusion (V/Q), scan or invasive coronary angiography (ICA) may be necessary in order to establish a differential diagnosis between ppCM and other diseases with acute HF presentation, such as pulmonary embolism (PE), amniotic fluid embolism, and acute coronary syndromes (ACSs).
PE is a well-known complication of pregnancy-associated hemodynamic and metabolic changes and represents a relevant cause of maternal death (76, 77). It may manifest with acute dyspnea and in severe cases with cardiogenic shock. In the case of elevated clinical suspicion and negative lower-limb Doppler ultrasound results, CT-pulmonary angiography (CTPA) is usually recommended, with generally low fetal radiation dose. A perfusion lung scan leads to similar or even lower fetal radiation exposure and a decreased maternal breast absorbed dose and may therefore be preferred to CTPA especially in the presence of normal findings in chest x-ray examination, when the ventilation portion of the V/Q scan may be eliminated with similar diagnostic accuracy to CTPA (70, 78, 79). However, the best management of PE during pregnancy is still being debated (80). Non-contrast CMR using bright blood techniques such as balanced steady-state free precession sequences may be also considered as a viable alternative approach for ruling out PE in selected cases (81–83).
Although pregnancy is associated with an increased risk of ACS compared with age-matched non-pregnant women, ACSs are relatively uncommon during pregnancy (84). In addition to coronary atherosclerosis, other non-atherosclerotic etiologies, particularly spontaneous coronary artery dissection (SCAD), represent a relevant cause of ACS in peripartum patients according to age and risk factors (85, 86). Pregnancy-related ACS is most common during the third trimester, although clinical presentation is the same as in the non-pregnant population, and management in pregnancy is similar to that in the general population, including revascularization techniques (6).
In the setting of suspected chronic coronary syndrome related to obstructive coronary artery disease (CAD), the choice of the optimal non-invasive diagnostic test should be guided by pre-test probability (PTP) and patient characteristics (87). Coronary CT angiography (CTA) is the preferred initial test in patients with low clinical likelihood of CAD and may be considered also in pregnant patients as an alternative to ICA. Although pharmacological stress echocardiography and stress CMR (i.e., adenosine, regadenoson, or dypiridamole) would represent the functional test of choice in the vast majority of symptomatic patients with intermediate PTP of CAD, they are not recommended during pregnancy, as vasodilators may lead to maternal hypotension and placental hypoperfusion. After delivery, should a myocardial perfusion test be necessary, nuclear medicine imaging [single-photon emission computed tomography (SPECT)/positron emission tomography (PET)] can be considered, especially with reduced radiotracer doses. A temporary interruption of breastfeeding, depending on the half-lives of the radiotracers, is advisable whether or not performed during lactation (74). ICA may be required to establish a definite diagnosis and start appropriate treatment in the case of typical angina and/or evidence of myocardial ischemia.
Gaps in Knowledge and Future Perspectives
A correct diagnosis of PPCM is key to start early treatment, plan appropriate follow-up, and provide adequate counseling. Recent data support the use of specific drug therapy with the prolactin inhibitor bromocriptine, although most studies are small-sized and with short-term follow-up. Future studies are needed to clarify the clinical importance and prognostic relevance of subclinical cardiac dysfunction at different stages of the disease by means of advanced echo modules including tissue Doppler imaging, speckle strain analysis, 3-D, contrast, and stress echocardiography. Further, 3-D echocardiography holds promise toward early detection of subclinical RV dysfunction and improved sensitivity for imaging of intracardiac thrombosis. Finally, the prognostic value of echocardiographic parameters other than LVEF or LV size requires further evaluation (Tables 2, 3).
Although LGE is a robust outcome predictor in several non-ischemic cardiomyopathies, available evidence for PPCM highlighted conflicting results on scar prevalence in the absence of any specific scar pattern. This might be secondary to the small number of subjects enrolled, the heterogeneity of study design, and the poorly defined pathophysiology underlying PPCM that may be different across specific patient subgroups. Advanced tissue characterization by native and post-contrast parametric mapping and MR fingerprinting may provide valuable information about specific disease process underlying PPCM and should be the objective of future investigations (Table 3). Given the relative rarity of PPCM, prospective multicenter registries would be helpful to identify novel prognostic markers in PPCM, including CMR markers of fibrosis, genetic markers, and biomarkers, and to clarify the role of multimodality cardiac imaging in the assessment of PPCM. In this way, novel and effective strategies for risk stratification will help counseling for future pregnancies, tailored decisions on HF prevention or treatment may be substantially improved, and potential targets for disease-modifying therapeutic intervention using existing or novel drug therapy may be eventually demonstrated.
Author Contributions
FR: conceptualization, literature review, and writing—original draft. CD, EV, LC, CM, CP, FI, SGab, and AD'A: literature review and writing—original draft. MK, NA, MC, GR, ED, and SP: review and editing. SGal: conceptualization, project administration, and supervision.
Funding
SP acknowledges support from the National Institute for Health Research Barts Biomedical Research Centre.
Conflict of Interest
The authors declare that the research was conducted in the absence of any commercial or financial relationships that could be construed as a potential conflict of interest.
Acknowledgments
The authors would like to thank Dr. Giovanni Donato Aquaro for his contribution.
References
1. Creanga AA, Syverson C, Seed K, Callaghan WM. Pregnancy-related mortality in the United States, 2011–2013. Obstet Gynecol. (2017) 130:366–73. doi: 10.1097/AOG.0000000000002114
2. Cantwell R, Clutton-Brock T, Cooper G, Dawson A, Drife J, Garrod D, et al. Saving Mothers' Lives: reviewing maternal deaths to make motherhood safer: 2006–2008. The Eighth Report of the Confidential Enquiries into Maternal Deaths in the United Kingdom. BJOG. (2011) 118 (Suppl. 1):1–203. doi: 10.1111/j.1471-0528.2010.02847.x
3. Khan KS, Wojdyla D, Say L, Gulmezoglu AM, Van Look PF. WHO analysis of causes of maternal death: a systematic review. Lancet. (2006) 367:1066–74. doi: 10.1016/S0140-6736(06)68397-9
4. Demakis JG, Rahimtoola SH. Peripartum cardiomyopathy. Circulation. (1971) 44:964–8. doi: 10.1161/01.CIR.44.5.964
5. Sliwa K, Hilfiker-Kleiner D, Petrie MC, Mebazaa A, Pieske B, Buchmann E, et al. Current state of knowledge on aetiology, diagnosis, management, and therapy of peripartum cardiomyopathy: a position statement from the Heart Failure Association of the European Society of Cardiology Working Group on peripartum cardiomyopathy. Eur J Heart Fail. (2010) 12:767–78. doi: 10.1093/eurjhf/hfq120
6. Regitz-Zagrosek V, Roos-Hesselink JW, Bauersachs J, Blomstrom-Lundqvist C, Cifkova R, De Bonis M, et al. 2018 ESC Guidelines for the management of cardiovascular diseases during pregnancy. Eur Heart J. (2018) 39:3165–241. doi: 10.1093/eurheartj/ehy340
7. Yancy CW, Jessup M, Bozkurt B, Butler J, Casey DE Jr, Drazner MH, et al. (2013) ACCF/AHA guideline for the management of heart failure: a report of the American College of Cardiology Foundation/American Heart Association Task Force on Practice Guidelines. J Am Coll Cardiol. (2013) 62:e147–239. doi: 10.1161/CIR.0b013e31829e8776
8. Bauersachs J, Konig T, van der Meer P, Petrie MC, Hilfiker-Kleiner D, Mbakwem A, et al. Pathophysiology, diagnosis and management of peripartum cardiomyopathy: a position statement from the Heart Failure Association of the European Society of Cardiology Study Group on peripartum cardiomyopathy. Eur J Heart Fail. (2019) 21:827–43. doi: 10.1002/ejhf.1493
9. Kolte D, Khera S, Aronow WS, Palaniswamy C, Mujib M, Ahn C, et al. Temporal trends in incidence and outcomes of peripartum cardiomyopathy in the United States: a nationwide population-based study. J Am Heart Assoc. (2014) 3:e001056. doi: 10.1161/JAHA.114.001056
10. Arany Z, Elkayam U. Peripartum cardiomyopathy. Circulation. (2016) 133:1397–409. doi: 10.1161/CIRCULATIONAHA.115.020491
11. Main EK, McCain CL, Morton CH, Holtby S, Lawton ES. Pregnancy-related mortality in California: causes, characteristics, and improvement opportunities. Obstet Gynecol. (2015) 125:938–47. doi: 10.1097/AOG.0000000000000746
12. Patten IS, Rana S, Shahul S, Rowe GC, Jang C, Liu L, et al. Cardiac angiogenic imbalance leads to peripartum cardiomyopathy. Nature. (2012) 485:333–8. doi: 10.1038/nature11040
13. Bajou K, Herkenne S, Thijssen VL, D'Amico S, Nguyen NQ, Bouche A, et al. PAI-1 mediates the antiangiogenic and profibrinolytic effects of 16K prolactin. Nat Med. (2014) 20:741–7. doi: 10.1038/nm.3552
14. van Spaendonck-Zwarts KY, Posafalvi A, van den Berg MP, Hilfiker-Kleiner D, Bollen IA, Sliwa K, et al. Titin gene mutations are common in families with both peripartum cardiomyopathy and dilated cardiomyopathy. Eur Heart J. (2014) 35:2165–73. doi: 10.1093/eurheartj/ehu050
15. Sliwa K, Blauwet L, Tibazarwa K, Libhaber E, Smedema JP, Becker A, et al. Evaluation of bromocriptine in the treatment of acute severe peripartum cardiomyopathy: a proof-of-concept pilot study. Circulation. (2010) 121:1465–73. doi: 10.1161/CIRCULATIONAHA.109.901496
16. Halkein J, Tabruyn SP, Ricke-Hoch M, Haghikia A, Nguyen NQ, Scherr M, et al. MicroRNA-146a is a therapeutic target and biomarker for peripartum cardiomyopathy. J Clin Invest. (2013) 123:2143–54. doi: 10.1172/JCI64365
17. Hilfiker-Kleiner D, Haghikia A, Nonhoff J, Bauersachs J. Peripartum cardiomyopathy: current management and future perspectives. Eur Heart J. (2015) 36:1090–7. doi: 10.1093/eurheartj/ehv009
18. Honigberg MC, Elkayam U, Rajagopalan N, Modi K, Briller JE, Drazner MH, et al. Electrocardiographic findings in peripartum cardiomyopathy. Clin Cardiol. (2019) 42:524–9. doi: 10.1002/clc.23171
19. Ricci F, Aquilani R, Radico F, Bianco F, Dipace GG, Miniero E, et al. Role and importance of ultrasound lung comets in acute cardiac care. Eur Heart J Acute Cardiovasc Care. (2015) 4:103–12. doi: 10.1177/2048872614553166
20. Chen MM, Coakley FV, Kaimal A, Laros RK Jr. Guidelines for computed tomography and magnetic resonance imaging use during pregnancy and lactation. Obstet Gynecol. (2008) 112(2 Pt 1):333–40. doi: 10.1097/AOG.0b013e318180a505
21. Nii M, Ishida M, Dohi K, Tanaka H, Kondo E, Ito M, et al. Myocardial tissue characterization and strain analysis in healthy pregnant women using cardiovascular magnetic resonance native T1 mapping and feature tracking technique. J Cardiovasc Magn Reson. (2018) 20:52. doi: 10.1186/s12968-018-0476-5
22. Khan SG, Melikian N, Mushemi-Blake S, Dennes W, Jouhra F, Monaghan M, et al. Physiological reduction in left ventricular contractile function in healthy postpartum women: potential overlap with peripartum cardiomyopathy. PLoS ONE. (2016) 11:e0147074. doi: 10.1371/journal.pone.0147074
23. Reisner SA, Lysyansky P, Agmon Y, Mutlak D, Lessick J, Friedman Z. Global longitudinal strain: a novel index of left ventricular systolic function. J Am Soc Echocardiogr. (2004) 17:630–3. doi: 10.1016/j.echo.2004.02.011
24. Lang RM, Badano LP, Mor-Avi V, Afilalo J, Armstrong A, Ernande L, et al. Recommendations for cardiac chamber quantification by echocardiography in adults: an update from the American Society of Echocardiography and the European Association of Cardiovascular Imaging. J Am Soc Echocardiogr. (2015) 28:1–39 e14. doi: 10.1016/j.echo.2014.10.003
25. Dennis AT, Castro JM. Echocardiographic differences between preeclampsia and peripartum cardiomyopathy. Int J Obstet Anesth. (2014) 23:260–6. doi: 10.1016/j.ijoa.2014.05.002
26. Shim WJ. Role of echocardiography in the management of cardiac disease in women. J Cardiovasc Ultrasound. (2014) 22:173–9. doi: 10.4250/jcu.2014.22.4.173
27. Afonso L, Arora NP, Mahajan N, Kottam A, Ballapuram K, Toosi M, et al. Comparison of patients with peripartum heart failure and normal (≥55%) versus low (<45%) left ventricular ejection fractions. Am J Cardiol. (2014) 114:290–3. doi: 10.1016/j.amjcard.2014.04.037
28. Briasoulis A, Mocanu M, Marinescu K, Qaqi O, Palla M, Telila T, et al. Longitudinal systolic strain profiles and outcomes in peripartum cardiomyopathy. Echocardiography. (2016) 33:1354–60. doi: 10.1111/echo.13277
29. Aoyama D, Hamatani Y, Kamiya C, Ohta-Ogo K, Amaki M, Kawakami S, et al. Peripartum serial echocardiographic findings in a patient with life-threatening peripartum cardiomyopathy. Intern Med. (2018) 57:3105–9. doi: 10.2169/internalmedicine.0748-17
30. Srichai MB, Junor C, Rodriguez LL, Stillman AE, Grimm RA, Lieber ML, et al. Clinical, imaging, and pathological characteristics of left ventricular thrombus: a comparison of contrast-enhanced magnetic resonance imaging, transthoracic echocardiography, and transesophageal echocardiography with surgical or pathological validation. Am Heart J. (2006) 152:75–84. doi: 10.1016/j.ahj.2005.08.021
31. Duncan K, Nanda NC, Foster WA, Mehmood F, Patel V, Singh A. Incremental value of live/real time three-dimensional transthoracic echocardiography in the assessment of left ventricular thrombi. Echocardiography. (2006) 23:68–72. doi: 10.1111/j.1540-8175.2006.00176.x
32. Kharwar RB, Chandra S, Dwivedi SK, Saran RK. A pedunculated left ventricular thrombus in a women with peripartum cardiomyopathy: evaluation by three dimensional echocardiography. J Cardiovasc Ultrasound. (2014) 22:139–43. doi: 10.4250/jcu.2014.22.3.139
33. Sinha A, Nanda NC, Khanna D, Dod HS, Vengala S, Mehmood F, et al. Morphological assessment of left ventricular thrombus by live three-dimensional transthoracic echocardiography. Echocardiography. (2004) 21:649–55. doi: 10.1111/j.0742-2822.2004.04062.x
34. Abdelmoneim SS, Pellikka PA, Mulvagh SL. Contrast echocardiography for assessment of left ventricular thrombi. J Ultrasound Med. (2014) 33:1337–44. doi: 10.7863/ultra.33.8.1337
35. Weinsaft JW, Kim RJ, Ross M, Krauser D, Manoushagian S, LaBounty TM, et al. Contrast-enhanced anatomic imaging as compared to contrast-enhanced tissue characterization for detection of left ventricular thrombus. JACC Cardiovasc Imaging. (2009) 2:969–79. doi: 10.1016/j.jcmg.2009.03.017
36. Velangi PS, Choo C, Chen KA, Kazmirczak F, Nijjar PS, Farzaneh-Far A, et al. Long-term embolic outcomes after detection of left ventricular thrombus by late gadolinium enhancement cardiovascular magnetic resonance imaging: a matched cohort study. Circ Cardiovasc Imaging. (2019) 12:e009723. doi: 10.1161/CIRCIMAGING.119.009723
37. Felker GM, Thompson RE, Hare JM, Hruban RH, Clemetson DE, Howard DL, et al. Underlying causes and long-term survival in patients with initially unexplained cardiomyopathy. N Engl J Med. (2000) 342:1077–84. doi: 10.1056/NEJM200004133421502
38. Sliwa K, Petrie MC, Hilfiker-Kleiner D, Mebazaa A, Jackson A, Johnson MR, et al. Long-term prognosis, subsequent pregnancy, contraception and overall management of peripartum cardiomyopathy: practical guidance paper from the Heart Failure Association of the European Society of Cardiology Study Group on Peripartum Cardiomyopathy. Eur J Heart Fail. (2018) 20:951–62. doi: 10.1002/ejhf.1178
39. McNamara DM, Elkayam U, Alharethi R, Damp J, Hsich E, Ewald G, et al. Clinical Outcomes for Peripartum Cardiomyopathy in North America: Results of the IPAC Study (Investigations of Pregnancy-Associated Cardiomyopathy). J Am Coll Cardiol. (2015) 66:905–14. doi: 10.1016/j.jacc.2015.06.1309
40. Chapa JB, Heiberger HB, Weinert L, Decara J, Lang RM, Hibbard JU. Prognostic value of echocardiography in peripartum cardiomyopathy. Obstet Gynecol. (2005) 105:1303–8. doi: 10.1097/01.AOG.0000161382.30233.ba
41. Biteker M, Ilhan E, Biteker G, Duman D, Bozkurt B. Delayed recovery in peripartum cardiomyopathy: an indication for long-term follow-up and sustained therapy. Eur J Heart Fail. (2012) 14:895–901. doi: 10.1093/eurjhf/hfs070
42. Li W, Li H, Long Y. Clinical Characteristics and long-term predictors of persistent left ventricular systolic dysfunction in peripartum cardiomyopathy. Can J Cardiol. (2016) 32:362–8. doi: 10.1016/j.cjca.2015.07.733
43. Peters A, Caroline M, Zhao H, Baldwin MR, Forfia PR, Tsai EJ. Initial right ventricular dysfunction severity identifies severe peripartum cardiomyopathy phenotype with worse early and overall outcomes: A 24-year cohort study. J Am Heart Assoc. (2018) 7:e008378. doi: 10.1161/JAHA.117.008378
44. Elkayam U. Risk of subsequent pregnancy in women with a history of peripartum cardiomyopathy. J Am Coll Cardiol. (2014) 64:1629–36. doi: 10.1016/j.jacc.2014.07.961
45. Elkayam U, Tummala PP, Rao K, Akhter MW, Karaalp IS, Wani OR, et al. Maternal and fetal outcomes of subsequent pregnancies in women with peripartum cardiomyopathy. N Engl J Med. (2001) 344:1567–71. doi: 10.1056/NEJM200105243442101
46. Fett JD, Fristoe KL, Welsh SN. Risk of heart failure relapse in subsequent pregnancy among peripartum cardiomyopathy mothers. Int J Gynaecol Obstet. (2010) 109:34–6. doi: 10.1016/j.ijgo.2009.10.011
47. Hilfiker-Kleiner D, Haghikia A, Masuko D, Nonhoff J, Held D, Libhaber E, et al. Outcome of subsequent pregnancies in patients with a history of peripartum cardiomyopathy. Eur J Heart Fail. (2017) 19:1723–8. doi: 10.1002/ejhf.808
48. Goland S, Weinstein JM, Zalik A, Kuperstein R, Zilberman L, Shimoni S, et al. Angiogenic imbalance and residual myocardial injury in recovered peripartum cardiomyopathy patients. Circ Heart Fail. (2016) 9:e003349. doi: 10.1161/CIRCHEARTFAILURE.116.003349
49. Dorbala S, Brozena S, Zeb S, Galatro K, Homel P, Ren JF, et al. Risk stratification of women with peripartum cardiomyopathy at initial presentation: a dobutamine stress echocardiography study. J Am Soc Echocardiogr. (2005) 18:45–8. doi: 10.1016/j.echo.2004.08.027
50. Barbosa MM, Freire CM, Nascimento BR, Rochitte CE, Silva MC, Siqueira MH, et al. Rest left ventricular function and contractile reserve by dobutamine stress echocardiography in peripartum cardiomyopathy. Rev Port Cardiol. (2012) 31:287–93. doi: 10.1016/j.repc.2012.02.002
51. Karaye KM, Lindmark K, Henein M. Right ventricular systolic dysfunction and remodelling in Nigerians with peripartum cardiomyopathy: a longitudinal study. BMC Cardiovasc Disord. (2016) 16:27. doi: 10.1186/s12872-016-0204-8
52. Blauwet LA, Delgado-Montero A, Ryo K, Marek JJ, Alharethi R, Mather PJ, et al. Right ventricular function in peripartum cardiomyopathy at presentation is associated with subsequent left ventricular recovery and clinical outcomes. Circ Heart Fail. (2016) 9:e002756. doi: 10.1161/CIRCHEARTFAILURE.115.002756
53. Haghikia A, Schwab J, Vogel-Claussen J, Berliner D, Pfeffer T, Konig T, et al. Bromocriptine treatment in patients with peripartum cardiomyopathy and right ventricular dysfunction. Clin Res Cardiol. (2019) 108:290–7. doi: 10.1007/s00392-018-1355-7
54. Petersen SE, Aung N, Sanghvi MM, Zemrak F, Fung K, Paiva JM, et al. Reference ranges for cardiac structure and function using cardiovascular magnetic resonance (CMR) in Caucasians from the UK Biobank population cohort. J Cardiovasc Magn Reson. (2017) 19:18. doi: 10.1186/s12968-017-0327-9
55. Romagano M, Louis-Jacques A, Quinones J, Freudenberger R, Fleming L, Smulian J, et al. Is there a role for cardiac magnetic resonance imaging during pregnancy? J Mater Fetal Neonatal Med. (2018) 33:1–6. doi: 10.1080/14767058.2018.1497598
56. Ducas RA, Elliott JE, Melnyk SF, Premecz S, daSilva M, Cleverley K, et al. Cardiovascular magnetic resonance in pregnancy: insights from the cardiac hemodynamic imaging and remodeling in pregnancy (CHIRP) study. J Cardiovasc Magn Reson. (2014) 16:1. doi: 10.1186/1532-429X-16-1
57. Kanal E, Barkovich AJ, Bell C, Borgstede JP, Bradley WG Jr, Froelich JW, et al. ACR guidance document for safe MR practices: 2007. Am J Roentgenol. (2007) 188:1447–74. doi: 10.2214/AJR.06.1616
58. Webb JA, Thomsen HS, Morcos SK, Members of Contrast Media Safety Committee of European Society of Urogenital R. The use of iodinated and gadolinium contrast media during pregnancy and lactation. Eur Radiol. (2005) 15:1234–40. doi: 10.1007/s00330-004-2583-y
59. Rofsky NM, Weinreb JC, Litt AW. Quantitative analysis of gadopentetate dimeglumine excreted in breast milk. J Magn Reson Imaging. (1993) 3:131–2. doi: 10.1002/jmri.1880030122
60. Schelbert EB, Elkayam U, Cooper LT, Givertz MM, Alexis JD, Briller J, et al. Myocardial damage detected by late gadolinium enhancement cardiac magnetic resonance is uncommon in peripartum cardiomyopathy. J Am Heart Assoc. (2017) 6:e005472. doi: 10.1161/JAHA.117.005472
61. Bozkurt B, Colvin M, Cook J, Cooper LT, Deswal A, Fonarow GC, et al. Current diagnostic and treatment strategies for specific dilated cardiomyopathies: a scientific statement from the American Heart Association. Circulation. (2016) 134:e579–46. doi: 10.1161/CIR.0000000000000455
62. Kawano H, Tsuneto A, Koide Y, Tasaki H, Sueyoshi E, Sakamoto I, et al. Magnetic resonance imaging in a patient with peripartum cardiomyopathy. Intern Med. (2008) 47:97–102. doi: 10.2169/internalmedicine.47.0316
63. Marmursztejn J, Vignaux O, Goffinet F, Cabanes L, Duboc D. Delayed-enhanced cardiac magnetic resonance imaging features in peripartum cardiomyopathy. Int J Cardiol. (2009) 137:e63–4. doi: 10.1016/j.ijcard.2009.04.028
64. Mouquet F, Lions C, de Groote P, Bouabdallaoui N, Willoteaux S, Dagorn J, et al. Characterisation of peripartum cardiomyopathy by cardiac magnetic resonance imaging. Eur Radiol. (2008) 18:2765–9. doi: 10.1007/s00330-008-1067-x
65. Renz DM, Rottgen R, Habedank D, Wagner M, Bottcher J, Pfeil A, et al. New insights into peripartum cardiomyopathy using cardiac magnetic resonance imaging. Rofo. (2011) 183:834–41. doi: 10.1055/s-0031-1281600
66. Arora NP, Mohamad T, Mahajan N, Danrad R, Kottam A, Li T, et al. Cardiac magnetic resonance imaging in peripartum cardiomyopathy. Am J Med Sci. (2014) 347:112–7. doi: 10.1097/MAJ.0b013e31828155e3
67. Haghikia A, Rontgen P, Vogel-Claussen J, Schwab J, Westenfeld R, Ehlermann P, et al. Prognostic implication of right ventricular involvement in peripartum cardiomyopathy: a cardiovascular magnetic resonance study. ESC Heart Fail. (2015) 2:139–49. doi: 10.1002/ehf2.12059
68. Elkayam U. Clinical characteristics of peripartum cardiomyopathy in the United States: diagnosis, prognosis, and management. J Am College Cardiol. (2011) 58:659–70. doi: 10.1016/j.jacc.2011.03.047
69. Ersboll AS, Bojer AS, Hauge MG, Johansen M, Damm P, Gustafsson F, et al. Long-term cardiac function after peripartum cardiomyopathy and preeclampsia: a danish nationwide, clinical follow-up study using maximal exercise testing and cardiac magnetic resonance imaging. J Am Heart Assoc. (2018) 7:e008991. doi: 10.1161/JAHA.118.008991
70. Baruteau AE, Leurent G, Martins RP, Thebault C, Treguer F, Leclercq C, et al. Peripartum cardiomyopathy in the era of cardiac magnetic resonance imaging: first results and perspectives. Int J Cardiol. (2010) 144:143–5. doi: 10.1016/j.ijcard.2008.12.153
71. Hurwitz LM, Reiman RE, Yoshizumi TT, Goodman PC, Toncheva G, Nguyen G, et al. Radiation dose from contemporary cardiothoracic multidetector CT protocols with an anthropomorphic female phantom: implications for cancer induction. Radiology. (2007) 245:742–50. doi: 10.1148/radiol.2453062046
72. McCollough CH, Schueler BA, Atwell TD, Braun NN, Regner DM, Brown DL, et al. Radiation exposure and pregnancy: when should we be concerned? Radiographics. (2007) 27:909–18. doi: 10.1148/rg.274065149
73. Wieseler KM, Bhargava P, Kanal KM, Vaidya S, Stewart BK, Dighe MK. Imaging in pregnant patients: examination appropriateness. Radiographics. (2010) 30:1215–29. doi: 10.1148/rg.305105034
74. Tirada N, Dreizin D, Khati NJ, Akin EA, Zeman RK. Imaging pregnant and lactating patients. Radiographics. (2015) 35:1751–65. doi: 10.1148/rg.2015150031
75. Pierce T, Hovnanian M, Hedgire S, Ghoshhajra B. Imaging of Cardiovascular Disease in Pregnancy and the Peripartum Period. Curr Treat Opt Cardiovasc Med. (2017) 19:94. doi: 10.1007/s11936-017-0593-8
76. Sullivan EA, Ford JB, Chambers G, Slaytor EK. Maternal mortality in Australia, 1973–1996. Aus N Z J Obstet Gynaecol. (2004) 44:452–7; discussion 377. doi: 10.1111/j.1479-828X.2004.00313.x
77. Abele JT, Sunner P. The clinical utility of a diagnostic imaging algorithm incorporating low-dose perfusion scans in the evaluation of pregnant patients with clinically suspected pulmonary embolism. Clin Nuclear Med. (2013) 38:29–32. doi: 10.1097/RLU.0b013e31827088f6
78. Konstantinides SV, Torbicki A, Agnelli G, Danchin N, Fitzmaurice D, Galie N, et al. 2014 ESC guidelines on the diagnosis and management of acute pulmonary embolism. Eur Heart J. (2014) 35:3033–69. doi: 10.1093/eurheartj/ehu283
79. Ridge CA, McDermott S, Freyne BJ, Brennan DJ, Collins CD, Skehan SJ. Pulmonary embolism in pregnancy: comparison of pulmonary CT angiography and lung scintigraphy. Am J Roentgenol. (2009) 193:1223–7. doi: 10.2214/AJR.09.2360
80. Konstantinides SV, Barco S, Lankeit M, Meyer G. Management of pulmonary embolism: an update. J Am College Cardiol. (2016) 67:976–90. doi: 10.1016/j.jacc.2015.11.061
81. Mantini C, Mastrodicasa D, Bianco F, Bucciarelli V, Scarano M, Mannetta G, et al. Prevalence and clinical relevance of extracardiac findings in cardiovascular magnetic resonance imaging. J Thorac Imaging. (2019) 34:48–55. doi: 10.1097/RTI.0000000000000360
82. Kluge A, Luboldt W, Bachmann G. Acute pulmonary embolism to the subsegmental level: diagnostic accuracy of three MRI techniques compared with 16-MDCT. Am J Roentgenol. (2006) 187:W7–14. doi: 10.2214/AJR.04.1814
83. Konstantinides SV, Meyer G, Becattini C, Bueno H, Geersing G-J, Harjola V-P, et al. 2019 ESC Guidelines for the diagnosis and management of acute pulmonary embolism developed in collaboration with the European Respiratory Society (ERS). Eur Respir J. (2019) 54:1901647. doi: 10.1183/13993003.01647-2019
84. Bush N, Nelson-Piercy C, Spark P, Kurinczuk JJ, Brocklehurst P, Knight M, et al. Myocardial infarction in pregnancy and postpartum in the UK. Eur J Prevent Cardiol. (2013) 20:12–20. doi: 10.1177/1741826711432117
85. Roth A, Elkayam U. Acute myocardial infarction associated with pregnancy. J Am College Cardiol. (2008) 52:171–80. doi: 10.1016/j.jacc.2008.03.049
86. Elkayam U, Jalnapurkar S, Barakkat MN, Khatri N, Kealey AJ, Mehra A, et al. Pregnancy-associated acute myocardial infarction: a review of contemporary experience in 150 cases between 2006 and 2011. Circulation. (2014) 129:1695–702. doi: 10.1161/CIRCULATIONAHA.113.002054
87. Knuuti J, Wijns W, Saraste A, Capodanno D, Barbato E, Funck-Brentano C, et al. 2019 ESC Guidelines for the diagnosis and management of chronic coronary syndromes: the task force for the diagnosis and management of chronic coronary syndromes of the European Society of Cardiology (ESC). Eur Heart J. (2020) 41:407–77. doi: 10.1093/eurheartj/ehz425
Keywords: peripartum cardiomyopathy, cardiac magnetic resonance, pregnancy, heart failure, tissue characterization, echocardiography
Citation: Ricci F, De Innocentiis C, Verrengia E, Ceriello L, Mantini C, Pietrangelo C, Irsuti F, Gabriele S, D'Alleva A, Khanji MY, Aung N, Renda G, Cameli M, Petersen SE, Di Cesare E and Gallina S (2020) The Role of Multimodality Cardiovascular Imaging in Peripartum Cardiomyopathy. Front. Cardiovasc. Med. 7:4. doi: 10.3389/fcvm.2020.00004
Received: 26 September 2019; Accepted: 13 January 2020;
Published: 18 February 2020.
Edited by:
Savvas Loizos, Henry Dunant Hospital, GreeceReviewed by:
Chiara Cirillo, King's College London, United KingdomCarla Sousa, São João University Hospital Center, Portugal
Copyright © 2020 Ricci, De Innocentiis, Verrengia, Ceriello, Mantini, Pietrangelo, Irsuti, Gabriele, D'Alleva, Khanji, Aung, Renda, Cameli, Petersen, Di Cesare and Gallina. This is an open-access article distributed under the terms of the Creative Commons Attribution License (CC BY). The use, distribution or reproduction in other forums is permitted, provided the original author(s) and the copyright owner(s) are credited and that the original publication in this journal is cited, in accordance with accepted academic practice. No use, distribution or reproduction is permitted which does not comply with these terms.
*Correspondence: Fabrizio Ricci, fabrizio.ricci@unich.it