- Faculty of Kinesiology, Libin Cardiovascular Institute of Alberta, University of Calgary, Calgary, AB, Canada
Introduction
The capacity to convey oxygen (O2) from the atmosphere in to mitochondria essentially determines maximal aerobic metabolism in humans (1–6). The inherent constitution of the O2 transport and utilization chain is asymmetrical, not all steps have the same importance (7, 8). Intracellular biochemical mechanisms that could in theory limit O2 utilization are overbuilt in relation to the potential delivery of O2 through the circulatory system (2, 3, 9). Peak oxygen consumption (VO2peak), a hallmark of aerobic capacity elicited by incremental exercise involving more than half of total muscle mass, is mainly determined by the circulatory capacity to deliver O2 to working muscle even in the presence of compromised muscle oxidative capacity (5, 7, 8). Glaring evidence of the impact of the circulatory system on VO2peak includes conditions such as heart failure (HF), intrinsically linked with impaired cardiac output and thus limited convective O2 delivery (2, 6). VO2peak is a strong and independent predictor of survival in HF patients used to determine eligibility for cardiac transplantation (6, 10, 11). After diagnosis of HF, survival estimates do not exceed 50% at 5 years (12, 13).
Understanding the physiology of O2 delivery and thereby VO2peak in HF may facilitate the identification of target mechanisms and the advent of effective treatments. While classic empirical studies in HF patients support the primary role of impaired cardiac pumping capacity in the limitation of VO2peak (14, 15), a recent paradigm based on theoretical assumptions attribute the main importance to skeletal muscle abnormalities in O2 diffusion from capillaries in to mitochondria (16). Given the radical change of rehabilitation programs implicit in the “skeletal muscle” paradigm, herein we sought to shed light on the foundation of this relatively new tenet in the HF field. In particular, a fundamental aspect will be clarified: the measurement and calculation of O2 diffusion in skeletal muscle.
O2 Transport Assessment: de facto Measurement of O2 Diffusion in Skeletal Muscle
The transport of O2 in living organisms follows well-known physical phenomena. O2 molecules move via (i) convection, due to the bulk motion of fluids, and (ii) diffusion, spontaneously spreading out from a region of high concentration to a region of low concentration. Along the O2 cascade, convection is the mode of O2 transport between the atmosphere and the lungs, and between pulmonary capillary blood and tissue microvascular beds, respectively determined by the bulk motion of air and circulating blood. Diffusion of O2 mainly occurs from alveoli in to pulmonary capillaries, and from tissue microvascular beds in to mitochondria. With respect to the measurement of O2 transport, both convection steps (air-to-lung, blood circulation) can be measured with relatively high accuracy in humans by means of spirometers, air/blood gas analyzers, arterial/venous blood samples and indicator-dilution/ultrasound techniques (17, 18). These well-established research methods may also be used to assess lung O2 diffusion. The final O2 diffusion step in the skeletal muscle microcirculation, however, cannot yet be directly measured. The level of resolution required to capture O2 extraction in microvessels supplying active muscle fibers is beyond reach owing to technical limitations including temporal and spatial constraints (8, 19).
The possibility seemingly exists, nonetheless, to make use of partial measurements and multiple assumptions to deliver a quantitative value for skeletal muscle O2 diffusion capacity (DMO2) (20–22). Notably, in the field of HF (16), some clinical researchers are currently applying a method for estimating DMO2 conceived almost 3 decades ago (20–22). Herein, DMO2 is portrayed as the ratio of skeletal muscle O2 consumption (VO2) and O2 pressure gradient between microvessels and mitochondria (21, 23, 24).
At first sight the notion of DMO2 appears consistent, albeit a close scrutiny of the actual measurements reveals salient incongruences. Skeletal muscle O2 consumption—calculated by the product of leg blood flow (LBF) and the difference between femoral arterial and venous O2 content (16, 21)—is primarily determined by convective O2 delivery, since LBF is substantially impaired (up to −40%) in HF conforming to the reduced pumping capacity of the failing heart (6, 25). Moreover, femoral vein O2 content is close to zero in HF patients at VO2peak (2). Therefore, the first component (numerator) of the DMO2 equation, i.e., skeletal muscle VO2, is essentially a function of convective O2 delivery (LBF × arterial O2 content) a fundamental mathematical flaw for a variable claimed to represent diffusive O2 transport (Figure 1).
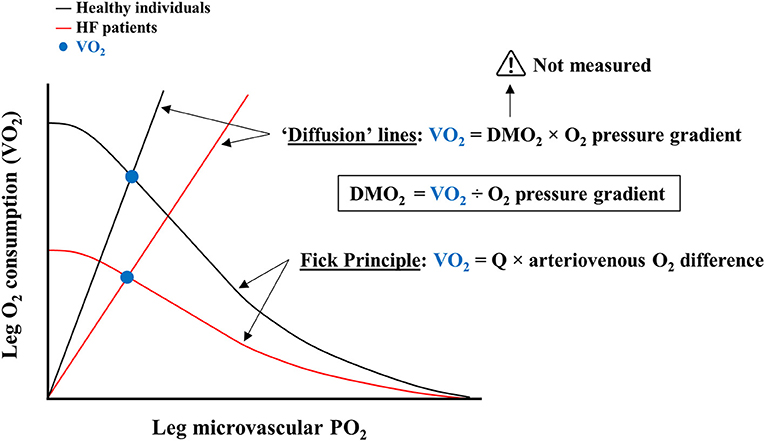
Figure 1. Common schematic illustration of convective and diffusive components of oxygen consumption (VO2) by proponents of abnormalities in skeletal muscle O2 diffusion. Leg O2 consumption is represented as a function of leg microvascular partial pressure of oxygen (PO2). Black lines depict Fick and “Diffusion” lines for healthy control individuals; red lines refer to heart failure (HF) patients. The intersection of these lines reflects the VO2 achieved (blue circles). Curved lines are derived from the established Fick Principle. Straight “Diffusion” lines are calculated by the product of skeletal muscle O2 diffusion capacity (DMO2) and O2 pressure gradient. Given that DMO2 cannot be directly measured, the “Diffusion” lines are intrinsically dependent on VO2, which is at least in part determined by cardiac output (Q), i.e., convective O2 delivery, according to the Fick Principle. Hence, “Diffusion” lines are compounded representations of convective and diffusive components of VO2.
The O2 pressure gradient between skeletal muscle microvessels and mitochondria is also estimated from femoral arterial and venous O2 content measurements, both pertaining to the macro- instead of microcirculation (16, 21). A myriad of assumptions are thus necessary to infer the postulated denominator of the DMO2 equation (20). For instance, the inherent heterogeneity of leg microvascular blood flow (26, 27), which even at VO2peak perfuses tissues (e.g., adipose tissue, bone, inactive muscle) not demanding a high VO2, is neglected (20, 21). Similarly, altered capillarization as well as anatomical and/or functional shunting within the lower limb, which may have a substantial influence in HF patients at VO2peak (28, 29), is ignored (16, 21). Taken together, the estimation of the O2 pressure gradient entails as a necessary premise that all blood flow downstream of the femoral artery perfuses active muscle fibers, in a perfect match between O2 delivery and metabolic demand, an untenable shortcoming (26–28).
Considering the actual measurements underpinning the concept of DMO2, its mathematical equation would be more accurately expressed as:
Hence, the numerator and denominator of DMO2 comprise variables reflecting convective O2 delivery, LBF and blood flow distribution, respectively. The observation of reduced DMO2 in HF patients is therefore not surprising (16, 23, 24). To conclude from these studies that mechanisms underlying skeletal muscle O2 diffusion should be primarily targeted for therapy is questionable (30). Caution should be taken in the interpretation of lower DMO2 in HF patients, which can be largely attributed to abnormalities in convective O2 delivery, let alone presenting DMO2 results as the main buttress of a new paradigm (31, 32). Further research taking advantage of technological developments in measurement accuracy and resolution of O2 dynamics in skeletal muscle will have to elucidate its role in the limitation of VO2peak in HF populations.
Author Contributions
DM and CD-C contributed to study design, interpretation, and manuscript writing.
Conflict of Interest Statement
The authors declare that the research was conducted in the absence of any commercial or financial relationships that could be construed as a potential conflict of interest.
References
1. Wagner PD. Determinants of maximal oxygen transport and utilization. Annu Rev Physiol. (1996) 58:21–50. doi: 10.1146/annurev.ph.58.030196.000321
2. Katz SD, Maskin C, Jondeau G, Cocke T, Berkowitz R, Lejemtel T. Near-maximal fractional oxygen extraction by active skeletal muscle in patients with chronic heart failure. J Appl Physiol. (2000) 88:2138–42. doi: 10.1152/jappl.2000.88.6.2138
3. Boushel R, Gnaiger E, Calbet JA, Gonzalez-Alonso J, Wright-Paradis C, Sondergaard H, et al. Muscle mitochondrial capacity exceeds maximal oxygen delivery in humans. Mitochondrion. (2011) 11:303–7. doi: 10.1016/j.mito.2010.12.006
4. Montero D, Cathomen A, Jacobs RA, Fluck D, De Leur J, Keiser S, et al. Haematological rather than skeletal muscle adaptations contribute to the increase in peak oxygen uptake induced by moderate endurance training. J Physiol. (2015) 593:4677–88. doi: 10.1113/JP270250
5. Lundby C, Montero D, Joyner M. Biology of VO2 max: looking under the physiology lamp. Acta Physiol. (2017) 220:218–28. doi: 10.1111/apha.12827
6. Montero D, Diaz-Canestro C. Determinants of exercise intolerance in heart failure with preserved ejection fraction: a systematic review and meta-analysis. Int J Cardiol. (2018) 254:224–9. doi: 10.1016/j.ijcard.2017.10.114
7. Levine BD. VO2max: what do we know, and what do we still need to know? J Physiol. (2008) 586:25–34. doi: 10.1113/jphysiol.2007.147629
8. Lundby C, Montero D. CrossTalk opposing view: diffusion limitation of O2 from microvessels into muscle does not contribute to the limitation of VO2 max. J Physiol. (2015) 593:3759–61. doi: 10.1113/JP270550
9. Calbet JA, Losa-Reyna J, Torres-Peralta R, Rasmussen P, Ponce-Gonzalez JG, Sheel AW, et al. Limitations to oxygen transport and utilization during sprint exercise in humans: evidence for a functional reserve in muscle O2 diffusing capacity. J Physiol. (2015) 593:4649–64. doi: 10.1113/JP270408
10. Mancini DM, Eisen H, Kussmaul W, Mull R, Edmunds LH Jr., Wilson JR. Value of peak exercise oxygen consumption for optimal timing of cardiac transplantation in ambulatory patients with heart failure. Circulation. (1991) 83:778–86. doi: 10.1161/01.CIR.83.3.778
11. Laukkanen JA, Kurl S, Salonen R, Rauramaa R, Salonen JT. The predictive value of cardiorespiratory fitness for cardiovascular events in men with various risk profiles: a prospective population-based cohort study. Eur Heart J. (2004) 25:1428–37. doi: 10.1016/j.ehj.2004.06.013
12. Macintyre K, Capewell S, Stewart S, Chalmers JW, Boyd J, Finlayson A, et al. Evidence of improving prognosis in heart failure: trends in case fatality in 66 547 patients hospitalized between 1986 and 1995. Circulation. (2000) 102:1126–31. doi: 10.1161/01.CIR.102.10.1126
13. Mosterd A, Cost B, Hoes AW, De Bruijne MC, Deckers JW, Hofman A, et al. The prognosis of heart failure in the general population: the Rotterdam Study. Eur Heart J. (2001) 22:1318–27. doi: 10.1053/euhj.2000.2533
14. Sullivan MJ, Knight JD, Higginbotham MB, Cobb FR. Relation between central and peripheral hemodynamics during exercise in patients with chronic heart failure. Muscle blood flow is reduced with maintenance of arterial perfusion pressure. Circulation. (1989) 80:769–81. doi: 10.1161/01.CIR.80.4.769
15. Kitzman DW, Higginbotham MB, Cobb FR, Sheikh KH, Sullivan MJ. Exercise intolerance in patients with heart failure and preserved left ventricular systolic function: failure of the Frank-Starling mechanism. J Am Coll Cardiol. (1991) 17:1065–72. doi: 10.1016/0735-1097(91)90832-T
16. Houstis NE, Eisman AS, Pappagianopoulos PP, Wooster L, Bailey CS, Wagner PD, et al. Exercise intolerance in heart failure with preserved ejection fraction: diagnosing and ranking its causes using personalized O2 pathway analysis. Circulation. (2018) 137:148–61. doi: 10.1161/CIRCULATIONAHA.117.029058
17. Gonzalez-Alonso J, Calbet JA. Reductions in systemic and skeletal muscle blood flow and oxygen delivery limit maximal aerobic capacity in humans. Circulation. (2003) 107:824–30. doi: 10.1161/01.CIR.0000049746.29175.3F
18. Dominelli PB, Foster GE, Dominelli GS, Henderson WR, Koehle MS, Mckenzie DC, et al. Exercise-induced arterial hypoxaemia and the mechanics of breathing in healthy young women. J Physiol. (2013) 591:3017–34. doi: 10.1113/jphysiol.2013.252767
19. Koga S, Rossiter HB, Heinonen I, Musch TI, Poole DC. Dynamic heterogeneity of exercising muscle blood flow and O2 utilization. Med Sci Sports Exerc. (2014) 46:860–76. doi: 10.1249/MSS.0000000000000178
20. Roca J, Agusti AG, Alonso A, Poole DC, Viegas C, Barbera JA, et al. Effects of training on muscle O2 transport at VO2max. J Appl Physiol (1985). (1992) 73, 1067–76. doi: 10.1152/jappl.1992.73.3.1067
21. Wagner PD. Gas exchange and peripheral diffusion limitation. Med Sci Sports Exerc. (1992) 24:54–8. doi: 10.1249/00005768-199201000-00010
22. Rud B, Foss O, Krustrup P, Secher NH, Hallen J. One-legged endurance training: leg blood flow and oxygen extraction during cycling exercise. Acta Physiol. (2012) 205:177–85. doi: 10.1111/j.1748-1716.2011.02383.x
23. Esposito F, Mathieu-Costello O, Shabetai R, Wagner PD, Richardson RS. Limited maximal exercise capacity in patients with chronic heart failure: partitioning the contributors. J Am Coll Cardiol. (2010) 55:1945–54. doi: 10.1016/j.jacc.2009.11.086
24. Esposito F, Reese V, Shabetai R, Wagner PD, Richardson RS. Isolated quadriceps training increases maximal exercise capacity in chronic heart failure: the role of skeletal muscle convective and diffusive oxygen transport. J Am Coll Cardiol. (2011) 58:1353–62. doi: 10.1016/j.jacc.2011.06.025
25. Lee JF, Barrett-O'keefe Z, Nelson AD, Garten RS, Ryan JJ, Nativi-Nicolau JN, et al. Impaired skeletal muscle vasodilation during exercise in heart failure with preserved ejection fraction. Int J Cardiol. (2016) 211:14–21. doi: 10.1016/j.ijcard.2016.02.139
26. Piiper J. Perfusion, diffusion and their heterogeneities limiting blood-tissue O2 transfer in muscle. Acta Physiol Scand. (2000) 168:603–7. doi: 10.1046/j.1365-201x.2000.00711.x
27. Kalliokoski KK, Oikonen V, Takala TO, Sipila H, Knuuti J, Nuutila P. Enhanced oxygen extraction and reduced flow heterogeneity in exercising muscle in endurance-trained men. Am J Physiol Endocrinol Metab. (2001) 280:E1015–1021. doi: 10.1152/ajpendo.2001.280.6.E1015
28. Wilson JR, Martin JL, Ferraro N, Weber KT. Effect of hydralazine on perfusion and metabolism in the leg during upright bicycle exercise in patients with heart failure. Circulation. (1983) 68:425–32. doi: 10.1161/01.CIR.68.2.425
29. Del Buono MG, Arena R, Borlaug BA, Carbone S, Canada JM, Kirkman DL, et al. Exercise intolerance in patients with heart failure: JACC state-of-the-art review. J Am Coll Cardiol. (2019) 73:2209–25. doi: 10.1016/j.jacc.2019.01.072
30. Fleg JL, Cooper LS, Borlaug BA, Haykowsky MJ, Kraus WE, Levine BD, et al. Exercise training as therapy for heart failure: current status and future directions. Circ Heart Fail. (2015) 8:209–20. doi: 10.1161/CIRCHEARTFAILURE.113.001420
31. Maurer MS, Schulze PC. Exercise intolerance in heart failure with preserved ejection fraction: shifting focus from the heart to peripheral skeletal muscle. J Am Coll Cardiol. (2012) 60:129–31. doi: 10.1016/j.jacc.2012.04.012
Keywords: exercise intolerance, oxygen delivery, muscle diffusion, blood flow distribution, heart failure
Citation: Montero D and Diaz-Canestro C (2019) Skeletal Muscle O2 Diffusion and the Limitation of Aerobic Capacity in Heart Failure: A Clarification. Front. Cardiovasc. Med. 6:78. doi: 10.3389/fcvm.2019.00078
Received: 09 April 2019; Accepted: 29 May 2019;
Published: 12 June 2019.
Edited by:
Chris J. Pemberton, University of Otago, New ZealandReviewed by:
Daryl Owen Schwenke, University of Otago, New ZealandRisto Kerkela, University of Oulu, Finland
Copyright © 2019 Montero and Diaz-Canestro. This is an open-access article distributed under the terms of the Creative Commons Attribution License (CC BY). The use, distribution or reproduction in other forums is permitted, provided the original author(s) and the copyright owner(s) are credited and that the original publication in this journal is cited, in accordance with accepted academic practice. No use, distribution or reproduction is permitted which does not comply with these terms.
*Correspondence: David Montero, ZGF2aWQubW9udGVyb2JhcnJpbCYjeDAwMDQwO3VjYWxnYXJ5LmNh