- 1L&T Microbiology Research Centre, Vision Research Foundation, Sankara Nethralaya, Chennai, India
- 2Department of HIV/AIDS, National Institute for Research in Tuberculosis, Chennai, India
With the success of antiretroviral therapy (ART), a dramatic decrease in viral burden and opportunistic infections and an increase in life expectancy has been observed in human immunodeficiency virus (HIV) infected individuals. However, it is now clear that HIV- infected individuals have enhanced susceptibility to non-AIDS (Acquired immunodeficiency syndrome)-related complications such as cardiovascular disease (CVD). CVDs such as atherosclerosis have become a significant cause of morbidity and mortality in individuals with HIV infection. Though studies indicate that ART itself may increase the risk to develop CVD, recent studies suggest a more important role for HIV infection in contributing to CVD independently of the traditional risk factors. Endothelial dysfunction triggered by HIV infection has been identified as a critical link between infection, inflammation/immune activation, and atherosclerosis. Considering the inability of HIV to actively replicate in endothelial cells, endothelial dysfunction depends on both HIV-encoded proteins as well as inflammatory mediators released in the microenvironment by HIV-infected cells. Indeed, the HIV proteins, gp120 (envelope glycoprotein) and Tat (transactivator of transcription), are actively secreted into the endothelial cell micro-environment during HIV infection, while Nef can be actively transferred onto endothelial cells during HIV infection. These proteins can have significant direct effects on the endothelium. These include a range of responses that contribute to endothelial dysfunction, including enhanced adhesiveness, permeability, cell proliferation, apoptosis, oxidative stress as well as activation of cytokine secretion. This review summarizes the current understanding of the interactions of HIV, specifically its proteins with endothelial cells and its implications in cardiovascular disease. We analyze recent in vitro and in vivo studies examining endothelial dysfunction in response to HIV proteins. Furthermore, we discuss the multiple mechanisms by which these viral proteins damage the vascular endothelium in HIV patients. A better understanding of the molecular mechanisms of HIV protein associated endothelial dysfunction leading to cardiovascular disease is likely to be pivotal in devising new strategies to treat and prevent cardiovascular disease in HIV-infected patients.
Introduction
The introduction of highly active antiretroviral therapy has lead to a drastic reduction in viral burden and opportunistic infections, resulting in a remarkable improvement in the life expectancy of HIV-infected individuals. However, it is now evident that HIV-infected individuals have an enhanced susceptibility to non-AIDS (Acquired Immunodeficiency syndrome)-related comorbidities such as cardio-vascular diseases (CVDs), which have emerged as prominent causes of morbidity and mortality in this population (1–9). Atherosclerotic CVD rates and risk of myocardial infarction are significantly elevated in HIV-infected individuals than the general population (1, 10, 11). Studies further indicate that both clinical cardiovascular events such as coronary heart disease (11–13), peripheral artery disease (14), as well as subclinical cardiovascular damage such as elevation of intima-media thickness (15, 16), coronary calcification (17), abnormal ankle-brachial index (18) and silent myocardial ischemia (19) are much higher in HIV-infected individuals (20).
Etiopathogenesis of Cardiovascular Disease in Patients With HIV Infection
The increased cardiovascular risk in HIV-infected individuals is attributable to a combination of multiple factors, including higher prevalence of traditional risk factors, inherent effects of the HIV infection, effects of antiretroviral therapy and the presence of other co-morbidities seen frequently in HIV-positive patients (such as hepatitis C virus and herpes family virus co-infections). Though initial studies indicated a predominance of traditional CVD risk factors (21, 22) and effect of ART (23) as major causes for CVD among HIV-positive individuals (10, 24, 25), evidence from experimental and observational studies (26, 27) in recent years have redirected attention more toward the consequences of HIV infection itself. Hsue et al demonstrated a correlation between HIV infection and premature atherosclerosis even in the absence of detectable viremia, immunodeficiency, and ART exposure, with the atherosclerosis being independent of traditional cardiovascular risk factors (28).
Among multiple pathogenic effects that contribute to atherosclerosis and ultimately CVD, HIV-induced endothelial dysfunction is now established as a major contributing factor. Higher plasma HIV RNA levels have been shown to correlate with endothelial dysfunction in HIV-infected patients (29). A transgenic mouse model expressing HIV viral proteins env, tat, nef, vpu, vpr, and rev demonstrated aortic endothelial dysfunction and increased arterial stiffness (30). HIV-infected patients had significantly impaired endothelial function, as demonstrated by reduced flow-mediated dilation, a measure of endothelial vasomotor function in comparison to the HIV-negative group (31).
Endothelial Dysfunction and Cardiovascular Disease
Endothelial dysfunction as a precursor of atherosclerosis and future cardiovascular events has been demonstrated in multiple population studies (32, 33). The development of atherosclerosis resulting from dysfunctional endothelium is highly complex and regulated by several factors. Endothelial dysfunction is characterized by decreased anti-oxidant, anti-inflammatory and anti-thrombotic properties (due to reduced NO bioavailability) and increased endothelial permeability, pro-inflammatory cytokine levels, and adhesion molecule expression. Leukocyte recruitment and adhesion represent the initial events in development of atherosclerosis. Leukocyte recruitment is mediated by several chemoattractants such as IL-6, IL-8, and MCP-1 and adherence of leukocytes to the endothelium is mediated by cell adhesion molecules (CAM). Leukocytes, especially monocytes traverse the endothelium, and migrate into the intima (34). Transmigration of leukocytes, as well as infiltration of plasma contents into the vascular wall is facilitated by an increase in endothelial permeability. These infiltrated plasma contents such as modified low-density lipoprotein (LDL), along with substances produced by infiltrated leukocytes, such as cytokines and chemokines, alter smooth muscle function and contribute to the development of atherosclerosis (35). Further, in the intima, the monocytes differentiate into macrophages, expressing receptors that facilitate lipid uptake. On lipid uptake and accumulation, macrophages transform into foam cells. These foam cells initiate atherosclerotic lesions, which are later characterized by plaque formation (34). Studies suggest that HIV impairs several of these processes that maintain vascular homeostasis, potentially leading to atherosclerosis (Table 1). Several mechanisms have been suggested to explain how HIV infection induces endothelial dysfunction leading to CVD, including direct HIV infection of endothelial cells (ECs), inflammation and effect of HIV proteins HIV proteins released in the endothelial microenvironment or directly transferred to ECs by HIV and HIV-infected cells represent critical mediators of endothelial dysfunction. This article reviews the current understanding of the mechanisms by which HIV, in particular, the different HIV proteins drive EC dysregulation, potentially leading to CVD.
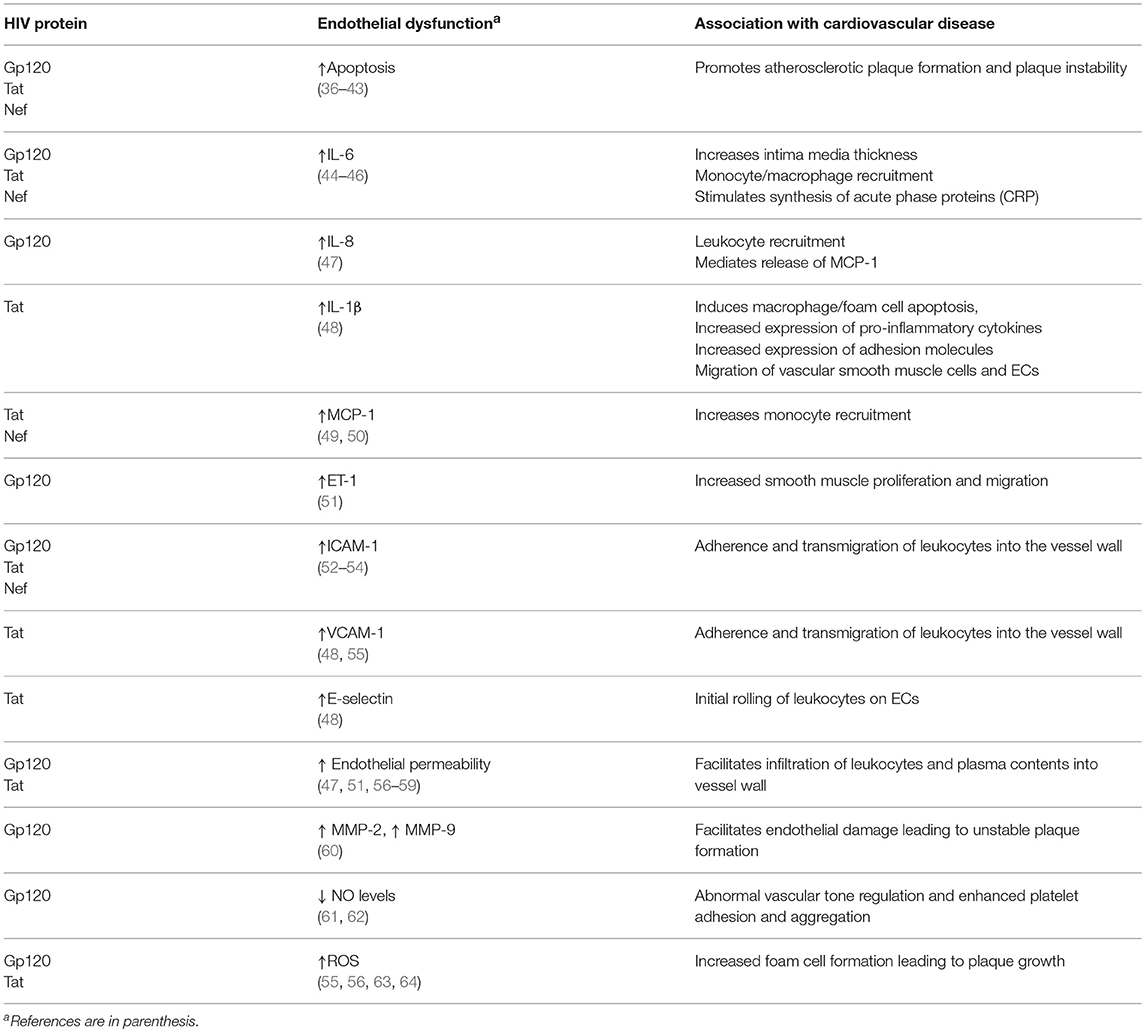
Table 1. Summary of the potential mechanisms by which HIV protein-induced endothelial dysfunction contribute directly or indirectly to the development of atherosclerosis and CVD.
HIV Encoded Proteins and Endothelial Dysfunction
HIV is a retrovirus with a glycoprotein-rich envelope surrounding a nucleocapsid. The HIV structural and regulatory/accessory proteins are designed for the virus to adapt efficiently to the human host, thereby promoting its replication and transmission. The HIV viral genome contains 9 principal genes, gag, pol, env, tat, rev, vpu, vpr, vif, and nef. The Gag-Pol precursor protein undergoes proteolytic cleavage to generate the matrix p17, capsid p24, nucleocapsids p9 and p6, reverse transcriptase, protease, and integrase, all of which are major structural components of the viral core. The Env undergoes proteolytic cleavage to generate the envelope glycoproteins gp120 and gp41. Tat and Rev are the regulatory proteins, while Vpu, Vpr, Vif, and Nef are the accessory proteins (65). Among these viral proteins, gp120, Tat and Nef play a major role in the pathogenesis of endothelial dysfunction. The experimental evidence supporting a functional role for the HIV viral proteins in the disruption of EC cell biology is outlined in the following sections.
HIV gp120
HIV envelope glycoprotein is synthesized as a precursor glycoprotein, gp160, which is then processed into an amino terminus subunit, gp120, and a carboxyl transmembrane subunit, gp41. The envelope glycoprotein, gp120 is expressed on the outer layer of the virus, as well as on the surface of infected cells. Gp120 is critical for virus infection, as the protein is necessary for binding to specific cell surface receptors on target cells and facilitating virus entry. The primary receptor for gp120 is the CD4 receptor, while the main co-receptors are CXCR4 and CCR5. Gp120 is found both in the free form in the body fluids of HIV-positive patients (66, 67) and bound form on the surface of apoptotic CD4 positive T-cells (68). In fact, gp120 has been shown in the germinal center of lymph nodes in HIV-infected individuals under ART with no detectable viral replication (69). Multiple studies have confirmed that gp120, both in soluble and surface bound form, has an important role in viral pathogenesis on diverse uninfected bystander cells (70–74), including ECs.
Gp120 is associated with apoptosis, adhesion molecule expression, pro-inflammatory cytokine production and EC permeability. Gp120 present either on viral particles, surface of infected cells, or as free soluble protein causes endothelial apoptosis predominantly by direct interaction with the co-receptor, CXCR4. Gp120 induces apoptosis in human coronary ECs (36), human umbilical vein ECs (HUVECs) (37, 38), lung microvascular ECs (LMVECs) (39), and brain microvascular ECs (BMVECs) (40, 41). EC apoptosis is an important process, initially in atherosclerotic plaque formation, and later in the progression to an advanced stage of atherosclerosis, when the plaques become vulnerable to rupture (42, 75, 76). The molecular mechanism by which gp120 exerts its endothelial toxicity, may involve caspase-3 activation (38), Bax upregulation (38), protein kinase C (PKC) activation (77) and p38 mitogen-activated protein (MAP) kinase signaling (41). Gp120 also induces an increase in reactive oxygen species (ROS), signaling oxidative stress to the ECs (56, 63). Oxidative stress induced by generation of excess reactive oxygen species is a critical process in the development of atherosclerosis (78). HIV-induced ROS likely contributes to endothelial dysfunction through direct effects on the endothelium and/or indirectly through monocytes /macrophages contacting the vessel wall. The viral glycoptotein is also able to increase endothelin-1 (ET-1) secretion (51) and promote surface expression of Endothelial monocyte activating polypeptide II (EMAPII) (79). ET-1 mediates the reduction of vascular nitric oxide production by ECs, leading to the smooth muscle proliferation and migration, which in turn leads to arterial vasoconstriction (80), whereas EMAPII is released in response to stress such as hypoxia, mechanical strain and apoptosis (81) and acts as a pro-apoptotic factor. In addition, a recent study has shown that HIV gp120 (X4 and R5) promotes EC senescence and impairs the regulation of senescence-associated microRNAs (82). Senescent ECs develop a dysfunctional phenotype acquiring pro-inflammatory, pro-oxidant, vasoconstrictor, and prothrombotic properties (83).
Gp120 is directly involved in upregulation of pro-inflammatory cytokines such as IL-6 and IL-8 in primary ECs (44). IL-6 and IL-8 play a major role in recruitment of leukocytes, especially of the monocyte/macrophage and neutrophil lineage, respectively. IL-6 can also actively promote atherogenesis, both directly by inducing vascular endothelial dysfunction, extracellular matrix degradation and indirectly by stimulating hepatocytes synthesis of acute phase proteins involved in inflammation, such as C-reactive protein (84). Gp120 also facilitates monocyte (52) and T-cell adherence (85) to the vascular endothelium through upregulation of CAMs. Among the CAMs, E-selectin is involved in the initial rolling of leukocytes on the endothelial cells, while ICAM-1 and VCAM-1 induce firm adhesion and transmigration of leukocytes across the endothelium (86). Gp120 augments expression of ICAM-1, but not VCAM-1 or E-selectin, in ECs of multiple origins, including human coronary artery, lung, brain, umbilical vein, and dermal microvascular ECs (52).
Gp120 also increases endothelial permeability by various mechanisms including cytoskeletal rearrangement (56), down-regulation of tight junction proteins (51) and PKC activation (47). An increase in endothelial permeability was observed in brain endothelial cultures of HIV gp120 transgenic mice (87), compared to non-transgenic mice. Gp120 also induces expression of the matrix metalloproteases (MMPs), MMP-2 and MMP-9, that mediate endothelial damage with the formation of an unstable atherosclerotic plaque morphology (60). Additionally, gp120 reduces the EC-derived nitric oxide (NO) synthesized by the NO synthase, thus affecting endothelium-dependent vasorelaxation and enhancing platelet adhesion and aggregation (61, 62).
HIV Tat
HIV Tat (trans-activator of transcription) is a regulatory protein encoded by the tat gene that enhances viral transcription (88). Tat has been detected in the sera of HIV patients (89), even during complete ART (cART) (90). Tat is secreted into the extracellular microenvironment by HIV-infected T-cells and monocyte/macrophages (89, 91). In the circulation, Tat is suggested to act as a proto-cytokine, modulating the functions of several cells including ECs (92). Thus, Tat is involved in the pathogenesis of several HIV-associated disease conditions ranging from pulmonary hypertension to cognitive abnormalities (36, 48, 93–95). Tat protein possesses both transcription promotion and membrane transduction properties. Tat has five discrete domains, the N-terminal, cysteine-rich, core, basic, and C-terminal domain. Tat interacts with three known receptors to trigger endothelial dysfunction. The C-terminal domain, containing an Arg-Gly-Asp (RGD) sequence, binds with high affinity to the integrins alphaVbeta1 and alphaVbeta3 receptors (96). The basic domain binds to the integrin alphaVbeta5 receptor (97) as well as the Flk-1/KDR receptor (98). Tat activates these receptors to initiate endothelial signaling pathways that affect diverse processes such as endothelial permeability (57, 58), cytokine production (59), adhesion (48), angiogenesis (99–102), and apoptosis (43).
Tat exhibits a dual function with regard to survival regulation, exhibiting either EC proliferation or apoptosis, depending on the micro-environment conditions (103). One of the prominent properties of Tat is that of a direct angiogenic factor (92). Endothelial proliferation is enhanced by factors such as FGF-2 (fibroblast growth factor) (104). Tat activates Rac1 through a signaling cascade involving RhoA, Ras, and extracellular signal-regulated kinase (ERK), which in turn, induces EC proliferation and survival (105). Tat mediates Rac1 activation through PAK-1, phosphorylates c-Jun N-terminal kinase (JNK), activates endothelial NADPH oxidase and regulates actin cytoskeletal dynamics (106). Tat has been suggested to play a role in HIV-related Kaposi sarcoma by promoting EC proliferation and tumor angiogenesis, where Tat binds specifically and activates the Flk-1/kinase insert domain receptor (Flk- 1/KDR), a VEGF-A tyrosine kinase receptor, and promotes angiogenesis (98). Contrary to its role in angiogenesis, Tat also induces the apoptosis of primary microvascular ECs via either TNF-alpha secretion or through activation of the Fas-dependent pathway (43). Fiala et al. (36) analyzed the pathogenesis of HIV-related cardiomyopathy, and found that exogenous Tat protein was capable of activating apoptosis of both ECs or cardiomyocytes. A recent report indicates that HIV Tat along with morphine induces autophagy in pulmonary ECs, suggesting a role for Tat in HIV-related pulmonary arterial hypertension in the presence of opioids (107). In addition, Tat also promotes EC senescence and dysregulation of senescence-associated microRNAs (82).
Tat stimulates the release of pro-inflammatory cytokines and induces expression of CAMs (45, 48, 55, 108, 109) in ECs of diverse origin (i.e., pulmonary artery, umbilical vein, aorta, and brain). In human vascular ECs (HUVECs), Tat stimulates the upregulation of inflammatory mediators, including IL-1β, MCP-1, VCAM-1 and E-selectin through nuclear factor-kappa B (NF-κB) (48, 108). IL-1β can induce macrophage and foam cell apoptosis, causing the release of their lipid content into the intima of the artery and contributing toward the lipid core in the plaque (110). IL-1β can also induce the expression of cytokines, adhesion molecules and the migration and mitogenesis of vascular smooth muscle and endothelial cells (111). MCP-1 is a major chemokine involved in monocyte recruitment during atherosclerosis development (35). Expression of IL-6 and MCP-1 is dependent upon the activity of the kinases, PKC (45) and cAMP-dependent protein kinase A (49). Tat stimulated ICAM-1 expression in HUVECs by suppressing miR-221/-222 via an NF-κB-dependent pathway (53), while Tat stimulated VCAM-1 expression through p38 MAP kinase and NF-kB activation (55). Upregulation of these adhesion molecules resulted in monocyte (45, 108, 112) and T-cell (113) adhesion to the endothelium. Furthermore, Matzen et al showed that Tat in combination with TNF-alpha, a cytokine increased in sera and tissues of HIV-infected patients, acts synergistically to increase the adhesion of leukocytes to ECs, suggesting that both these proteins act in co-operation to contribute to the vascular damage during HIV infection (113). Finally, Tat induces endothelial oxidative stress through activation of NADPH oxidase and through decreased antioxidant capacity. Tat-induced MAPK signaling requires upstream superoxide production by various NADPH oxidase subunits. Moreover, Tat-induced ROS activates the NF-kB pathway (55) and decreases GSH levels (64). Tat also attenuates the expression of the mitochondrial superoxide scavenger, Manganese-superoxide dismutase (Mn-SOD) (46, 114).
HIV Nef
HIV Nef is a 27-kD, n-myristoylated accessory protein that lacks enzymatic activity. It is an adaptor molecule containing multiple domains essential for interaction with host cell signaling molecules (115, 116). Nef is involved in modulation of several intracellular functions that include regulation of protein trafficking and cell signaling pathways, attenuation of antibody maturation in B cells (117), and increase in HIV infectivity (118). The presence of Nef has been shown in the endothelium of coronary and pulmonary arteries of SIV-HIV-Nef-infected macaques (50). Sowinski et al (119) demonstrated that Nef induces the formation of conduit-like nanotubes, connecting HIV-positive cells to bystander cells. Further, Wang et al. (50) showed that Nef transfer from HIV-infected cells to ECs promotes endothelial dysfunction (50, 120). Nef is also delivered to bystander cells through exosomes (121). ECs, especially those present in developing atherosclerotic plaques, would therefore be in a prime physical position to receive Nef transfer from circulating monocytes and T cells. Transgenic mice that express CD4-promoter-driven Nef develop multiple pathologies including vasospasm in the heart (122). Studies show that Rhesus macaques demonstrate pulmonary hypertension (PH)-like pulmonary vascular remodeling, when infected with chimeric SHIVnef virions, but not with SIV, indicating a role of HIV-Nef in PH, with certain Nef gene variants showing a higher propensity to develop PH (123, 124).
Similar to gp120 and Tat, Nef has been associated with several aspects of HIV-induced endothelial dysfunction. Acheampong et al. (42) showed that Nef, when expressed both extracellularly and endogenously, induces apoptosis in primary human brain microvascular ECs (HB-MVECs) by activation of caspases. A microarray analysis of apoptosis genes in Nef-transduced HB-MVECs demonstrated that the up-regulated genes belong to both mitochondrial and Fas/FasL apoptotic pathways, indicating that Nef may utilize multiple pathways to induce apoptosis in ECs. In contrast, in the context of Kaposi's sarcoma, Nef and KSHV oncogene K1 synergistically promote angiogenesis by inducing cellular miR-718 to regulate the PTEN/AKT/mTOR signaling pathway. However, in Kaposi's sarcoma, Nef in combination with KSHV oncogene K1 synergistically induces cellular miR-718 to regulate the PTEN/AKT/mTOR signaling pathway and thus promotes angiogenesis. This pathway is an important factor in aberrant neovascularization caused by KS-associated herpesvirus (KSHV) (125).
Nef-expressing T cells demonstrate enhanced adherence to ECs as observed by their impaired diapedesis and migration into the subendothelial space (126). Fan et al have shown ERK kinase-mediated ICAM-1 upregulation in vascular ECs stably expressing Nef (54). Furthermore, Nef increases endothelial MCP-1 production through activation of the NF-kB signaling pathway (50). In a recent study, Nef was shown to be involved in the alteration of EC cholesterol homeostasis through phosphorylation of Caveolin-1 (Cav-1), leading to Cav-1 redistribution and impairment of HDL-mediated cholesterol efflux in ECs (127). In addition to its direct effects on ECs, Nef activates macrophages and produces foam cells (128). The interactions of these foam cells with ECs could also contribute to EC dysfunction, and potentially facilitate the development of atherosclerosis.
Conclusion and Future Perspectives
In summary, the present review underscores the role of HIV-encoded proteins, specifically Gp120, Tat and Nef, in the pathogenesis of endothelial dysfunction, a precursor for the development of CVD (Figure 1). Our understanding of the complex interaction of traditional factors, inflammation and immune activation, cART and HIV in the progression of CVD has grown rapidly over the past decade. However, a more detailed exploration into the mechanisms of HIV-induced endothelial dysfunction is needed to formulate targeted approaches to prevent and treat HIV-related vascular diseases. Presently, large prospective studies such as REPRIEVE (NCT02344290), a randomized trial to prevent vascular events in HIV, are being carried out that will provide valuable data on the relation between inflammation, CVD and HIV infection (129). Research efforts will also need to focus on identifying HIV-specific markers that could predict the risk of developing CVD and facilitate the early detection of CVD in HIV patients. An accurate assessment of patients based on such biomarkers could be incorporated in guidelines such as the European AIDS Clinical Society guidelines (130) on the joint management and prevention of CVD in HIV patients, thereby providing vital information to guide clinicians on the most appropriate approach to prevent and treat CVD in this high-risk population.
Author Contributions
GR and DP wrote the manuscript. AA wrote and revised the manuscript.
Funding
This work was supported by the DBT-Ramalingaswami re-entry fellowship grant (BT/RLF/10/2013) from the Department of Biotechnology, Ministry of Science & Technology, Government of India to AA.
Conflict of Interest Statement
The authors declare that the research was conducted in the absence of any commercial or financial relationships that could be construed as a potential conflict of interest.
Acknowledgments
We would like to acknowledge Department of Biotechnology, New Delhi, India for financial support to GR.
References
1. Palella FJ Jr, Baker RK, Moorman AC, Chmiel JS, Wood KC, Brooks JT, et al. Mortality in the highly active antiretroviral therapy era: changing causes of death and disease in the HIV outpatient study. J Acquir Immune Defic Syndr. (2006) 43:27–34. doi: 10.1097/01.qai.0000233310.90484.16
2. Crum NF, Riffenburgh RH, Wegner S, Agan BK, Tasker SA, Spooner KM, et al. Comparisons of causes of death and mortality rates among HIV-infected persons: analysis of the pre-, early, and late HAART (highly active antiretroviral therapy) eras. J Acquir Immune Defic Syndr. (2006) 41:194–200. doi: 10.1097/01.qai.0000179459.31562.16
3. Grinspoon SK, Grunfeld C, Kotler DP, Currier JS, Lundgren JD, Dube MP, et al. State of the science conference: initiative to decrease cardiovascular risk and increase quality of care for patients living with HIV/AIDS: executive summary. Circulation (2008) 118:198–210. doi: 10.1161/CIRCULATIONAHA.107.189622
4. Kamin DS, Grinspoon SK. Cardiovascular disease in HIV-positive patients. AIDS (2005) 19:641–52. doi: 10.1097/01.aids.0000166087.08822.bc
5. Sackoff JE, Hanna DB, Pfeiffer MR, Torian LV. Causes of death among persons with AIDS in the era of highly active antiretroviral therapy: New York City. Ann Inter Med. (2006) 145:397–406. doi: 10.7326/0003-4819-145-6-200609190-00003
6. Mateen FJ, Shinohara RT, Carone M, Miller EN, McArthur JC, Jacobson LP, et al. Neurologic disorders incidence in HIV+ vs HIV- men: multicenter AIDS cohort study, 1996-2011. Neurology (2012) 79:1873–80. doi: 10.1212/WNL.0b013e318271f7b8
7. Morlat P, Roussillon C, Henard S, Salmon D, Bonnet F, Cacoub P, et al. Causes of death among HIV-infected patients in France in 2010 (national survey): trends since 2000. AIDS (2014) 28:1181–91. doi: 10.1097/QAD.0000000000000222
8. French AL, Gawel SH, Hershow R, Benning L, Hessol NA, Levine AM, et al. Trends in mortality and causes of death among women with HIV in the United States: a 10-year study. J Acquir Immune Defic Syndr. (2009) 51:399–406. doi: 10.1097/QAI.0b013e3181acb4e5
9. Mocroft A, Reiss P, Gasiorowski J, Ledergerber B, Kowalska J, Chiesi A, et al. Serious fatal and nonfatal non-AIDS-defining illnesses in Europe. J Acquir Immune Defic Syndr. (2010) 55:262–70. doi: 10.1097/QAI.0b013e3181e9be6b
10. Currier JS, Lundgren JD, Carr A, Klein D, Sabin CA, Sax PE, et al. Epidemiological evidence for cardiovascular disease in HIV-infected patients and relationship to highly active antiretroviral therapy. Circulation (2008) 118:e29–35. doi: 10.1161/CIRCULATIONAHA.107.189624
11. Triant VA, Lee H, Hadigan C, Grinspoon SK. Increased acute myocardial infarction rates and cardiovascular risk factors among patients with human immunodeficiency virus disease. J Clin Endocrinol Metabol. (2007) 92:2506–12. doi: 10.1210/jc.2006-2190
12. Islam FM, Wu J, Jansson J, Wilson DP. Relative risk of cardiovascular disease among people living with HIV: a systematic review and meta-analysis. HIV Med. (2012) 13:453–68. doi: 10.1111/j.1468-1293.2012.00996.x
13. Currier JS, Taylor A, Boyd F, Dezii CM, Kawabata H, Burtcel B, et al. Coronary heart disease in HIV-infected individuals. J Acquir Immune Defic Syndr. (2003) 33:506–12. doi: 10.1097/00126334-200308010-00012
14. Palacios R, Alonso I, Hidalgo A, Aguilar I, Sanchez MA, Valdivielso P, et al. Peripheral arterial disease in HIV patients older than 50 years of age. AIDS Res Hum Retroviruses (2008) 24:1043–6. doi: 10.1089/aid.2008.0001
15. Lorenz MW, Stephan C, Harmjanz A, Staszewski S, Buehler A, Bickel M, et al. Both long-term HIV infection and highly active antiretroviral therapy are independent risk factors for early carotid atherosclerosis. Atherosclerosis (2008) 196:720–6. doi: 10.1016/j.atherosclerosis.2006.12.022
16. Jeong SJ, Kim HW, Ku NS, Han SH, Kim CO, Choi JY, et al. Clinical factors associated with carotid plaque and intima-medial thickness in HIV-infected patients. Yonsei Med J. (2013) 54:990–8. doi: 10.3349/ymj.2013.54.4.990
17. Kingsley LA, Cuervo-Rojas J, Munoz A, Palella FJ, Post W, Witt MD, et al. Subclinical coronary atherosclerosis, HIV infection and antiretroviral therapy: multicenter AIDS cohort study. AIDS (2008) 22:1589–99. doi: 10.1097/QAD.0b013e328306a6c5
18. Periard D, Cavassini M, Taffe P, Chevalley M, Senn L, Chapuis-Taillard C, et al. High prevalence of peripheral arterial disease in HIV-infected persons. Clin Infect Dis. (2008) 46:761–7. doi: 10.1086/527564
19. Carr A, Grund B, Neuhaus J, El-Sadr WM, Grandits G, Gibert C, et al. Asymptomatic myocardial ischaemia in HIV-infected adults. AIDS (2008) 22:257–67. doi: 10.1097/QAD.0b013e3282f20a77
20. Gibellini D, Borderi M, Clo A, Morini S, Miserocchi A, Bon I, et al. HIV-related mechanisms in atherosclerosis and cardiovascular diseases. J Cardiovasc Med. (2013) 14:780–90. doi: 10.2459/JCM.0b013e3283619331
21. Dolan SE, Hadigan C, Killilea KM, Sullivan MP, Hemphill L, Lees RS, et al. Increased cardiovascular disease risk indices in HIV-infected women. J Acquir Immune Defic Syndr. (2005) 39:44–54. doi: 10.1097/01.qai.0000159323.59250.83
22. Saves M, Chene G, Ducimetiere P, Leport C, Le Moal G, Amouyel P, et al. Risk factors for coronary heart disease in patients treated for human immunodeficiency virus infection compared with the general population. Clin Infect Dis. (2003) 37:292–8. doi: 10.1086/375844
23. Group DADS, Friis-Moller N, Reiss P, Sabin CA, Weber R, Monforte A, et al. Class of antiretroviral drugs and the risk of myocardial infarction. N Engl J Med. (2007) 356:1723–35. doi: 10.1056/NEJMoa062744
24. Lo J, Abbara S, Shturman L, Soni A, Wei J, Rocha-Filho JA, et al. Increased prevalence of subclinical coronary atherosclerosis detected by coronary computed tomography angiography in HIV-infected men. AIDS (2010) 24:243–53. doi: 10.1097/QAD.0b013e328333ea9e
25. Oliviero U, Bonadies G, Apuzzi V, Foggia M, Bosso G, Nappa S, et al. Human immunodeficiency virus per se exerts atherogenic effects. Atherosclerosis (2009) 204:586–9. doi: 10.1016/j.atherosclerosis.2008.10.012
26. Lundgren JD, Strategies for Management of Antiretroviral Therapy Study G, Babiker A, El-Sadr W, Emery S, Grund B, et al. Inferior clinical outcome of the CD4+ cell count-guided antiretroviral treatment interruption strategy in the SMART study: role of CD4+ Cell counts and HIV RNA levels during follow-up. J Infect Dis. (2008) 197:1145–55. doi: 10.1086/529523
27. Armah KA, McGinnis K, Baker J, Gibert C, Butt AA, Bryant KJ, et al. HIV status, burden of comorbid disease, and biomarkers of inflammation, altered coagulation, and monocyte activation. Clin Infect Dis. (2012) 55:126–36. doi: 10.1093/cid/cis406
28. Hsue PY, Hunt PW, Schnell A, Kalapus SC, Hoh R, Ganz P, et al. Role of viral replication, antiretroviral therapy, and immunodeficiency in HIV-associated atherosclerosis. AIDS (2009) 23:1059–67. doi: 10.1097/QAD.0b013e32832b514b
29. Funderburg NT, Mayne E, Sieg SF, Asaad R, Jiang W, Kalinowska M, et al. Increased tissue factor expression on circulating monocytes in chronic HIV infection: relationship to in vivo coagulation and immune activation. Blood (2010) 115:161–7. doi: 10.1182/blood-2009-03-210179
30. Hansen L, Parker I, Sutliff RL, Platt MO, Gleason RL, Jr. Endothelial dysfunction, arterial stiffening, and intima-media thickening in large arteries from HIV-1 transgenic mice. Ann Biomed Eng. (2013) 41:682–93. doi: 10.1007/s10439-012-0702-5
31. Solages A, Vita JA, Thornton DJ, Murray J, Heeren T, Craven DE, et al. Endothelial function in HIV-infected persons. Clin Infect Dis. (2006) 42:1325–32. doi: 10.1086/503261
32. Gokce N, Keaney JF Jr, Hunter LM, Watkins MT, Nedeljkovic ZS, Menzoian JO, et al. Predictive value of noninvasively determined endothelial dysfunction for long-term cardiovascular events in patients with peripheral vascular disease. J Am College Cardiol. (2003) 41:1769–75. doi: 10.1016/S0735-1097(03)00333-4
33. Suwaidi JA, Hamasaki S, Higano ST, Nishimura RA, Holmes DR, Jr., Lerman A. Long-term follow-up of patients with mild coronary artery disease and endothelial dysfunction. Circulation (2000) 101:948–54. doi: 10.1161/01.CIR.101.9.948
35. Ramji DP, Davies TS. Cytokines in atherosclerosis: key players in all stages of disease and promising therapeutic targets. Cytokine Growth Factor Rev. (2015) 26:673–85. doi: 10.1016/j.cytogfr.2015.04.003
36. Fiala M, Popik W, Qiao JH, Lossinsky AS, Alce T, Tran K, et al. HIV-1 induces cardiomyopathyby cardiomyocyte invasion and gp120, Tat, and cytokine apoptotic signaling. Cardiovasc Toxicol. (2004) 4:97–107. doi: 10.1385/CT:4:2:097
37. Huang MB, Khan M, Garcia-Barrio M, Powell M, Bond VC. Apoptotic effects in primary human umbilical vein endothelial cell cultures caused by exposure to virion-associated and cell membrane-associated HIV-1 gp120. J Acquir Immune Defic Syndr. (2001) 27:213–21. doi: 10.1097/00126334-200107010-00001
38. Ullrich CK, Groopman JE, Ganju RK. HIV-1 gp120- and gp160-induced apoptosis in cultured endothelial cells is mediated by caspases. Blood (2000) 96:1438–42.
39. Kanmogne GD, Kennedy RC, Grammas P. Analysis of human lung endothelial cells for susceptibility to HIV type 1 infection, coreceptor expression, and cytotoxicity of gp120 protein. AIDS Res Hum Retroviruses (2001) 17:45–53. doi: 10.1089/088922201750056771
40. Kanmogne GD, Kennedy RC, Grammas P. HIV-1 gp120 proteins and gp160 peptides are toxic to brain endothelial cells and neurons: possible pathway for HIV entry into the brain and HIV-associated dementia. J Neuropathol Exp Neurol. (2002) 61:992–1000. doi: 10.1093/jnen/61.11.992
41. Khan NA, Di Cello F, Stins M, Kim KS. Gp120-mediated cytotoxicity of human brain microvascular endothelial cells is dependent on p38 mitogen-activated protein kinase activation. J Neurovirol. (2007) 13:242–51. doi: 10.1080/13550280701286531
42. Acheampong EA, Parveen Z, Muthoga LW, Kalayeh M, Mukhtar M, Pomerantz RJ. Human Immunodeficiency virus type 1 Nef potently induces apoptosis in primary human brain microvascular endothelial cells via the activation of caspases. J Virol. (2005) 79:4257–69. doi: 10.1128/JVI.79.7.4257-4269.2005
43. Park IW, Ullrich CK, Schoenberger E, Ganju RK, Groopman JE. HIV-1 Tat induces microvascular endothelial apoptosis through caspase activation. J Immunol. (2001) 167:2766–71. doi: 10.4049/jimmunol.167.5.2766
44. Yang B, Akhter S, Chaudhuri A, Kanmogne GD. HIV-1 gp120 induces cytokine expression, leukocyte adhesion, and transmigration across the blood-brain barrier: modulatory effects of STAT1 signaling. Microvasc Res. (2009) 77:212–9. doi: 10.1016/j.mvr.2008.11.003
45. Park IW, Wang JF, Groopman JE. HIV-1 Tat promotes monocyte chemoattractant protein-1 secretion followed by transmigration of monocytes. Blood (2001) 97:352–8. doi: 10.1182/blood.V97.2.352
46. Westendorp MO, Shatrov VA, Schulze-Osthoff K, Frank R, Kraft M, Los M, et al. HIV-1 Tat potentiates TNF-induced NF-kappa B activation and cytotoxicity by altering the cellular redox state. EMBO J. (1995) 14:546–54. doi: 10.1002/j.1460-2075.1995.tb07030.x
47. Kanmogne GD, Schall K, Leibhart J, Knipe B, Gendelman HE, Persidsky Y. HIV-1 gp120 compromises blood-brain barrier integrity and enhances monocyte migration across blood-brain barrier: implication for viral neuropathogenesis. J Cereb Blood Flow Metabol. (2007) 27:123–34. doi: 10.1038/sj.jcbfm.9600330
48. Cota-Gomez A, Flores NC, Cruz C, Casullo A, Aw TY, Ichikawa H, et al. The human immunodeficiency virus-1 Tat protein activates human umbilical vein endothelial cell E-selectin expression via an NF-kappa B-dependent mechanism. J Biol Chem. (2002) 277:14390–9. doi: 10.1074/jbc.M108591200
49. Zidovetzki R, Wang JL, Chen P, Jeyaseelan R, Hofman F. Human immunodeficiency virus Tat protein induces interleukin 6 mRNA expression in human brain endothelial cells via protein kinase C- and cAMP-dependent protein kinase pathways. AIDS Res Hum Retroviruses (1998) 14:825–33. doi: 10.1089/aid.1998.14.825
50. Wang T, Green LA, Gupta SK, Kim C, Wang L, Almodovar S, et al. Transfer of intracellular HIV Nef to endothelium causes endothelial dysfunction. PLoS ONE (2014) 9:e91063. doi: 10.1371/journal.pone.0091063
51. Kanmogne GD, Primeaux C, Grammas P. HIV-1 gp120 proteins alter tight junction protein expression and brain endothelial cell permeability: implications for the pathogenesis of HIV-associated dementia. J Neuropathol Exp Neurol. (2005) 64:498–505. doi: 10.1093/jnen/64.6.498
52. Ren Z, Yao Q, Chen C. HIV-1 envelope glycoprotein 120 increases intercellular adhesion molecule-1 expression by human endothelial cells. Lab Invest. (2002) 82:245–55. doi: 10.1038/labinvest.3780418
53. Duan M, Yao H, Hu G, Chen X, Lund AK, Buch S. HIV Tat induces expression of ICAM-1 in HUVECs: implications for miR-221/-222 in HIV-associated cardiomyopathy. PLoS ONE (2013) 8:e60170. doi: 10.1371/journal.pone.0060170
54. Fan Y, Liu C, Qin X, Wang Y, Han Y, Zhou Y. The role of ERK1/2 signaling pathway in Nef protein upregulation of the expression of the intercellular adhesion molecule 1 in endothelial cells. Angiology (2010) 61:669–78. doi: 10.1177/0003319710364215
55. Liu K, Chi DS, Li C, Hall HK, Milhorn DM, Krishnaswamy G. HIV-1 Tat protein-induced VCAM-1 expression in human pulmonary artery endothelial cells and its signaling. Am J Physiol Lung Cell Mol Physiol. (2005) 289:L252–60. doi: 10.1152/ajplung.00200.2004
56. Shiu C, Barbier E, Di Cello F, Choi HJ, Stins M. HIV-1 gp120 as well as alcohol affect blood-brain barrier permeability and stress fiber formation: involvement of reactive oxygen species. Alcohol Clin Exp Res. (2007) 31:130–7. doi: 10.1111/j.1530-0277.2006.00271.x
57. Andras IE, Pu H, Deli MA, Nath A, Hennig B, Toborek M. HIV-1 Tat protein alters tight junction protein expression and distribution in cultured brain endothelial cells. J Neurosci Res. (2003) 74:255–65. doi: 10.1002/jnr.10762
58. Avraham HK, Jiang S, Lee TH, Prakash O, Avraham S. HIV-1 Tat-mediated effects on focal adhesion assembly and permeability in brain microvascular endothelial cells. J Immunol. (2004) 173:6228–33. doi: 10.4049/jimmunol.173.10.6228
59. Arese M, Ferrandi C, Primo L, Camussi G, Bussolino F. HIV-1 Tat protein stimulates in vivo vascular permeability and lymphomononuclear cell recruitment. J Immunol. (2001) 166:1380–8. doi: 10.4049/jimmunol.166.2.1380
60. Louboutin JP, Reyes BA, Agrawal L, Van Bockstaele EJ, Strayer DS. HIV-1 gp120 upregulates matrix metalloproteinases and their inhibitors in a rat model of HIV encephalopathy. Euro J Neurosci. (2011) 34:2015–23. doi: 10.1111/j.1460-9568.2011.07908.x
61. Chatterjee A, Black SM, Catravas JD. Endothelial nitric oxide (NO) and its pathophysiologic regulation. Vasc Pharmacol. (2008) 49:134–40. doi: 10.1016/j.vph.2008.06.008
62. Jiang J, Fu W, Wang X, Lin PH, Yao Q, Chen C. HIV gp120 induces endothelial dysfunction in tumour necrosis factor-alpha-activated porcine and human endothelial cells. Cardiovasc Res. (2010) 87:366–74. doi: 10.1093/cvr/cvq013
63. Price TO, Uras F, Banks WA, Ercal N. A novel antioxidant N-acetylcysteine amide prevents gp120- and Tat-induced oxidative stress in brain endothelial cells. Exp Neurol. (2006) 201:193–202. doi: 10.1016/j.expneurol.2006.03.030
64. Toborek M, Lee YW, Pu H, Malecki A, Flora G, Garrido R, et al. HIV-Tat protein induces oxidative and inflammatory pathways in brain endothelium. J Neurochem. (2003) 84:169–79. doi: 10.1046/j.1471-4159.85.s2.16_7.x
65. Turner BG, Summers MF. Structural biology of HIV. J Mol Biol. (1999) 285:1–32. doi: 10.1006/jmbi.1998.2354
66. Oh SK, Cruikshank WW, Raina J, Blanchard GC, Adler WH, Walker J, et al. Identification of HIV-1 envelope glycoprotein in the serum of AIDS and ARC patients. J Acquir Immune Defic Syndr. (1992) 5:251–6.
67. Schneider J, Kaaden O, Copeland TD, Oroszlan S, Hunsmann G. Shedding and interspecies type sero-reactivity of the envelope glycopolypeptide gp120 of the human immunodeficiency virus. J General Virol. (1986) 67(Pt 11):2533–8. doi: 10.1099/0022-1317-67-11-2533
68. Twu C, Liu NQ, Popik W, Bukrinsky M, Sayre J, Roberts J, et al. Cardiomyocytes undergo apoptosis in human immunodeficiency virus cardiomyopathy through mitochondrion- and death receptor-controlled pathways. Proc Natl Acad Sci USA. (2002) 99:14386–91. doi: 10.1073/pnas.212327899
69. Popovic M, Tenner-Racz K, Pelser C, Stellbrink HJ, van Lunzen J, Lewis G, et al. Persistence of HIV-1 structural proteins and glycoproteins in lymph nodes of patients under highly active antiretroviral therapy. Proc Natl Acad Sci USA. (2005) 102:14807–12. doi: 10.1073/pnas.0506857102
70. Anand AR, Ganju RK. HIV-1 gp120-mediated apoptosis of T cells is regulated by the membrane tyrosine phosphatase CD45. J Biol Chem. (2006) 281:12289–99. doi: 10.1074/jbc.M511786200
71. Anand AR, Prasad A, Bradley RR, Deol YS, Nagaraja T, Ren X, et al. HIV-1 gp120-induced migration of dendritic cells is regulated by a novel kinase cascade involving Pyk2, p38 MAP kinase, and LSP1. Blood (2009) 114:3588–600. doi: 10.1182/blood-2009-02-206342
72. Banda NK, Bernier J, Kurahara DK, Kurrle R, Haigwood N, Sekaly RP, et al. Crosslinking CD4 by human immunodeficiency virus gp120 primes T cells for activation-induced apoptosis. J Exp Med. (1992) 176:1099–106. doi: 10.1084/jem.176.4.1099
73. Freedman BD, Liu QH, Del Corno M, Collman RG. HIV-1 gp120 chemokine receptor-mediated signaling in human macrophages. Immunol Res. (2003) 27:261–76. doi: 10.1385/IR:27:2-3:261
74. Munshi N, Balasubramanian A, Koziel M, Ganju RK, Groopman JE. Hepatitis C and human immunodeficiency virus envelope proteins cooperatively induce hepatocytic apoptosis via an innocent bystander mechanism. J Infect Dis. (2003) 188:1192–204. doi: 10.1086/378643
75. Libby P, Ridker PM, Hansson GK. Progress and challenges in translating the biology of atherosclerosis. Nature (2011) 473:317–25. doi: 10.1038/nature10146
76. Otsuka F, Finn AV, Yazdani SK, Nakano M, Kolodgie FD, Virmani R. The importance of the endothelium in atherothrombosis and coronary stenting. Nat Rev Cardiol. (2012) 9:439–53. doi: 10.1038/nrcardio.2012.64
77. Huang MB, Bond VC. Involvement of protein kinase C in HIV-1 gp120-induced apoptosis in primary endothelium. J Acquir Immune Defic Syndr. (2000) 25:375–89. doi: 10.1097/00126334-200012150-00001
78. Yang X, Li Y, Li Y, Ren X, Zhang X, Hu D, et al. Oxidative stress-mediated atherosclerosis: mechanisms and therapies. Front Physiol. (2017) 8:600. doi: 10.3389/fphys.2017.00600
79. Green LA, Yi R, Petrusca D, Wang T, Elghouche A, Gupta SK, et al. HIV envelope protein gp120-induced apoptosis in lung microvascular endothelial cells by concerted upregulation of EMAP II and its receptor, CXCR3. Am J Physiol Lung Cell Mol Physiol. (2014) 306:L372–82. doi: 10.1152/ajplung.00193.2013
80. Sandoval YH, Atef ME, Levesque LO, Li Y, Anand-Srivastava MB. Endothelin-1 signaling in vascular physiology and pathophysiology. Curr Vascu Pharmacol. (2014) 12:202–14. doi: 10.2174/1570161112666140226122054
81. Matschurat S, Knies UE, Person V, Fink L, Stoelcker B, Ebenebe C, et al. Regulation of EMAP II by hypoxia. Am J Pathol. (2003) 162:93–103. doi: 10.1016/S0002-9440(10)63801-1
82. Hijmans JG, Stockleman K, Reiakvam W, Levy MV, Brewster LM, Bammert TD, et al. Effects of HIV-1 gp120 and tat on endothelial cell sensescence and senescence-associated microRNAs. Physiol Rep. (2018) 6:e13647. doi: 10.14814/phy2.13647
83. Katsuumi G, Shimizu I, Yoshida Y, Minamino T. Vascular senescence in cardiovascular and metabolic diseases. Front Cardiovasc Med. (2018) 5:18. doi: 10.3389/fcvm.2018.00018
84. Ferrante G, Condorelli G. Interleukin-6 trans-signaling and risk of future cardiovascular events: a new avenue for atheroprotection? Cardiovasc Res. (2018). doi: 10.1093/cvr/cvy233. [Epub ahead of print].
85. Takano Y, Shimokado K, Hata Y, Yoshida M. HIV envelope protein gp120-triggered CD4+ T-cell adhesion to vascular endothelium is regulated via CD4 and CXCR4 receptors. Biochim Biophys Acta (2007) 1772:549–55. doi: 10.1016/j.bbadis.2007.01.010
86. Granger DN, Senchenkova E. Inflammation and the Microcirculation. Integrated Systems Physiology-From Cell to Function. San Rafael, CA: Morgan and Claypool Life Sciences (2010).
87. Cioni C, Annunziata P. Circulating gp120 alters the blood-brain barrier permeability in HIV-1 gp120 transgenic mice. Neurosci Lett. (2002) 330:299–301. doi: 10.1016/S0304-3940(02)00814-5
88. Debaisieux S, Rayne F, Yezid H, Beaumelle B. The ins and outs of HIV-1 Tat. Traffic (2012) 13:355–63. doi: 10.1111/j.1600-0854.2011.01286.x
89. Poggi A, Carosio R, Fenoglio D, Brenci S, Murdaca G, Setti M, et al. Migration of V delta 1 and V delta 2 T cells in response to CXCR3 and CXCR4 ligands in healthy donors and HIV-1-infected patients: competition by HIV-1 Tat. Blood (2004) 103:2205–13. doi: 10.1182/blood-2003-08-2928
90. Mediouni S, Darque A, Baillat G, Ravaux I, Dhiver C, Tissot-Dupont H, et al. Antiretroviral therapy does not block the secretion of the human immunodeficiency virus tat protein. Infect Disord Drug Targets (2012) 12:81–6. doi: 10.2174/187152612798994939
91. Chang HC, Samaniego F, Nair BC, Buonaguro L, Ensoli B. HIV-1 Tat protein exits from cells via a leaderless secretory pathway and binds to extracellular matrix-associated heparan sulfate proteoglycans through its basic region. AIDS (1997) 11:1421–31. doi: 10.1097/00002030-199712000-00006
92. Barillari G, Gendelman R, Gallo RC, Ensoli B. The Tat protein of human immunodeficiency virus type 1, a growth factor for AIDS Kaposi sarcoma and cytokine-activated vascular cells, induces adhesion of the same cell types by using integrin receptors recognizing the RGD amino acid sequence. Proc Natl Acad Sci USA. (1993) 90:7941–5. doi: 10.1073/pnas.90.17.7941
93. Carey AN, Sypek EI, Singh HD, Kaufman MJ, McLaughlin JP. Expression of HIV-Tat protein is associated with learning and memory deficits in the mouse. Behav Brain Res. (2012) 229:48–56. doi: 10.1016/j.bbr.2011.12.019
94. Ensoli F, Fiorelli V, DeCristofaro M, Santini Muratori D, Novi A, Vannelli B, et al. Inflammatory cytokines and HIV-1-associated neurodegeneration: oncostatin-M produced by mononuclear cells from HIV-1-infected individuals induces apoptosis of primary neurons. J Immunol. (1999) 162:6268–77.
95. Wang T, Jiang Z, Hou W, Li Z, Cheng S, Green LA, et al. HIV Tat protein affects circadian rhythmicity by interfering with the circadian system. HIV Med. (2014) 15:565–70. doi: 10.1111/hiv.12154
96. Mitola S, Soldi R, Zanon I, Barra L, Gutierrez MI, Berkhout B, et al. Identification of specific molecular structures of human immunodeficiency virus type 1 Tat relevant for its biological effects on vascular endothelial cells. J Virol. (2000) 74:344–53. doi: 10.1128/JVI.74.1.344-353.2000
97. Vogel BE, Lee SJ, Hildebrand A, Craig W, Pierschbacher MD, Wong-Staal F, et al. A novel integrin specificity exemplified by binding of the alpha v beta 5 integrin to the basic domain of the HIV Tat protein and vitronectin. J Cell Biol. (1993) 121:461–8. doi: 10.1083/jcb.121.2.461
98. Albini A, Soldi R, Giunciuglio D, Giraudo E, Benelli R, Primo L, et al. The angiogenesis induced by HIV-1 tat protein is mediated by the Flk-1/KDR receptor on vascular endothelial cells. Nat Med. (1996) 2:1371–5. doi: 10.1038/nm1296-1371
99. Fiorelli V, Barillari G, Toschi E, Sgadari C, Monini P, Sturzl M, et al. IFN-gamma induces endothelial cells to proliferate and to invade the extracellular matrix in response to the HIV-1 Tat protein: implications for AIDS-Kaposi's sarcoma pathogenesis. J Immunol. (1999) 162:1165–70.
100. Margheri F, D'Alessio S, Serrati S, Pucci M, Del Rosso A, Benelli R, et al. The urokinase-type plasminogen activator, its receptor and u-PA inhibitor type-1 affect in vitro growth and invasion of Kaposi's sarcoma and capillary endothelial cells: role of HIV-Tat protein. Int J Oncol. (2005) 27:223–35. doi: 10.3892/ijo.27.1.223
101. Barillari G, Sgadari C, Palladino C, Gendelman R, Caputo A, Morris CB, et al. Inflammatory cytokines synergize with the HIV-1 Tat protein to promote angiogenesis and Kaposi's sarcoma via induction of basic fibroblast growth factor and the alpha v beta 3 integrin. J Immunol. (1999) 163:1929–35.
102. Urbinati C, Mitola S, Tanghetti E, Kumar C, Waltenberger J, Ribatti D, et al. Integrin alphavbeta3 as a target for blocking HIV-1 Tat-induced endothelial cell activation in vitro and angiogenesis in vivo. Arterioscler Thromb Vasc Biol. (2005) 25:2315–20. doi: 10.1161/01.ATV.0000186182.14908.7b
103. Rusnati M, Presta M. HIV-1 Tat protein and endothelium: from protein/cell interaction to AIDS-associated pathologies. Angiogenesis (2002) 5:141–51. doi: 10.1023/A:1023892223074
104. Sgadari C, Barillari G, Palladino C, Bellino S, Taddeo B, Toschi E, et al. Fibroblast growth factor-2 and the HIV-1 Tat protein synergize in promoting Bcl-2 expression and preventing endothelial cell apoptosis: implications for the pathogenesis of AIDS-associated Kaposi's sarcoma. Int J Vasc Med. (2011) 2011:452729. doi: 10.1155/2011/452729
105. Toschi E, Bacigalupo I, Strippoli R, Chiozzini C, Cereseto A, Falchi M, et al. HIV-1 Tat regulates endothelial cell cycle progression via activation of the Ras/ERK MAPK signaling pathway. Mol Biol Cell (2006) 17:1985–94. doi: 10.1091/mbc.e05-08-0717
106. Wu RF, Ma Z, Myers DP, Terada LS. HIV-1 Tat activates dual Nox pathways leading to independent activation of ERK and JNK MAP kinases. J Biol Chem. (2007) 282:37412–9. doi: 10.1074/jbc.M704481200
107. Dalvi P, Sharma H, Chinnappan M, Sanderson M, Allen J, Zeng R, et al. Enhanced autophagy in pulmonary endothelial cells on exposure to HIV-Tat and morphine: role in HIV-related pulmonary arterial hypertension. Autophagy (2016) 12:2420–38. doi: 10.1080/15548627.2016.1238551
108. Dhawan S, Puri RK, Kumar A, Duplan H, Masson JM, Aggarwal BB. Human immunodeficiency virus-1-tat protein induces the cell surface expression of endothelial leukocyte adhesion molecule-1, vascular cell adhesion molecule-1, and intercellular adhesion molecule-1 in human endothelial cells. Blood (1997) 90:1535–44.
109. Pieper GM, Olds CL, Bub JD, Lindholm PF. Transfection of human endothelial cells with HIV-1 tat gene activates NF-kappa B and enhances monocyte adhesion. Ame J Physiol Heart Circ Physiol. (2002) 283:H2315–21. doi: 10.1152/ajpheart.00469.2002
110. Andres V, Pello OM, Silvestre-Roig C. Macrophage proliferation and apoptosis in atherosclerosis. Curr Opin Lipidol. (2012) 23:429–38. doi: 10.1097/MOL.0b013e328357a379
111. Tousoulis D, Oikonomou E, Economou EK, Crea F, Kaski JC. Inflammatory cytokines in atherosclerosis: current therapeutic approaches. Euro Heart J. (2016) 37:1723–32. doi: 10.1093/eurheartj/ehv759
112. Pu H, Tian J, Flora G, Lee YW, Nath A, Hennig B, et al. HIV-1 Tat protein upregulates inflammatory mediators and induces monocyte invasion into the brain. Mol Cell Neurosci. (2003) 24:224–37. doi: 10.1016/S1044-7431(03)00171-4
113. Matzen K, Dirkx AE, oude Egbrink MG, Speth C, Gotte M, Ascherl G, et al. HIV-1 Tat increases the adhesion of monocytes and T-cells to the endothelium in vitro and in vivo: implications for AIDS-associated vasculopathy. Virus Res. (2004) 104:145–55. doi: 10.1016/j.virusres.2004.04.001
114. Flores SC, Marecki JC, Harper KP, Bose SK, Nelson SK, McCord JM. Tat protein of human immunodeficiency virus type 1 represses expression of manganese superoxide dismutase in HeLa cells. Proc Natl Acad Sci USA. (1993) 90:7632–6. doi: 10.1073/pnas.90.16.7632
115. Kestler HW III, Ringler DJ, Mori K, Panicali DL, Sehgal PK, Daniel MD, et al. Importance of the nef gene for maintenance of high virus loads and for development of AIDS. Cell (1991) 65:651–62. doi: 10.1016/0092-8674(91)90097-I
116. Kirchhoff F, Greenough TC, Brettler DB, Sullivan JL, Desrosiers RC. Brief report: absence of intact nef sequences in a long-term survivor with nonprogressive HIV-1 infection. N Eng J Med. (1995) 332:228–32. doi: 10.1056/NEJM199501263320405
117. Qiao X, He B, Chiu A, Knowles DM, Chadburn A, Cerutti A. Human immunodeficiency virus 1 Nef suppresses CD40-dependent immunoglobulin class switching in bystander B cells. Nat Immunol. (2006) 7:302–10. doi: 10.1038/ni1302
118. Qi M, Aiken C. Nef enhances HIV-1 infectivity via association with the virus assembly complex. Virology (2008) 373:287–97. doi: 10.1016/j.virol.2007.12.001
119. Sowinski S, Jolly C, Berninghausen O, Purbhoo MA, Chauveau A, Kohler K, et al. Membrane nanotubes physically connect T cells over long distances presenting a novel route for HIV-1 transmission. Nat Cell Biol. (2008) 10:211–9. doi: 10.1038/ncb1682
120. Duffy P, Wang X, Lin PH, Yao Q, Chen C. HIV Nef protein causes endothelial dysfunction in porcine pulmonary arteries and human pulmonary artery endothelial cells. J Surg Res. (2009) 156:257–64. doi: 10.1016/j.jss.2009.02.005
121. Lenassi M, Cagney G, Liao M, Vaupotic T, Bartholomeeusen K, Cheng Y, et al. HIV Nef is secreted in exosomes and triggers apoptosis in bystander CD4+ T cells. Traffic (2010) 11:110–22. doi: 10.1111/j.1600-0854.2009.01006.x
122. Kay DG, Yue P, Hanna Z, Jothy S, Tremblay E, Jolicoeur P. Cardiac disease in transgenic mice expressing human immunodeficiency virus-1 nef in cells of the immune system. Am J Pathol. (2002) 161:321–35. doi: 10.1016/S0002-9440(10)64184-3
123. Almodovar S, Hsue PY, Morelli J, Huang L, Flores SC, Lung HIVS. Pathogenesis of HIV-associated pulmonary hypertension: potential role of HIV-1 Nef. Proc Am Thorac Soc. (2011) 8:308–12. doi: 10.1513/pats.201006-046WR
124. Almodovar S, Knight R, Allshouse AA, Roemer S, Lozupone C, McDonald D, et al. Human Immunodeficiency Virus nef signature sequences are associated with pulmonary hypertension. AIDS Res Hum Retroviruses (2012) 28:607–18. doi: 10.1089/aid.2011.0021
125. Xue M, Yao S, Hu M, Li W, Hao T, Zhou F, et al. HIV-1 Nef and KSHV oncogene K1 synergistically promote angiogenesis by inducing cellular miR-718 to regulate the PTEN/AKT/mTOR signaling pathway. Nucleic Acids Res. (2014) 42:9862–79. doi: 10.1093/nar/gku583
126. Stolp B, Imle A, Coelho FM, Hons M, Gorina R, Lyck R, et al. HIV-1 Nef interferes with T-lymphocyte circulation through confined environments in vivo. Proc Natl Acad Sci USA. (2012) 109:18541–6. doi: 10.1073/pnas.1204322109
127. Lin S, Nadeau PE, Mergia A. HIV inhibits endothelial reverse cholesterol transport through impacting subcellular Caveolin-1 trafficking. Retrovirology (2015) 12:62. doi: 10.1186/s12977-015-0188-y
128. Bukrinsky M, Sviridov D. Human immunodeficiency virus infection and macrophage cholesterol metabolism. J Leukocyte Biol. (2006) 80:1044–51. doi: 10.1189/jlb.0206113
129. Mitka M. Exploring statins to decrease HIV-related heart disease risk. JAMA (2015) 314:657–9. doi: 10.1001/jama.2015.5498
Keywords: HIV proteins, endothelial dysfunction, cardiovascular disease, gp120, Nef, Tat, atherosclerosis
Citation: Anand AR, Rachel G and Parthasarathy D (2018) HIV Proteins and Endothelial Dysfunction: Implications in Cardiovascular Disease. Front. Cardiovasc. Med. 5:185. doi: 10.3389/fcvm.2018.00185
Received: 18 September 2018; Accepted: 06 December 2018;
Published: 19 December 2018.
Edited by:
Mohamed Boutjdir, Veterans Affairs New York Harbor Healthcare System, United StatesReviewed by:
Keigi Fujiwara, University of Texas MD Anderson Cancer Center, United StatesPlinio Cirillo, University of Naples Federico II, Italy
Copyright © 2018 Anand, Rachel and Parthasarathy. This is an open-access article distributed under the terms of the Creative Commons Attribution License (CC BY). The use, distribution or reproduction in other forums is permitted, provided the original author(s) and the copyright owner(s) are credited and that the original publication in this journal is cited, in accordance with accepted academic practice. No use, distribution or reproduction is permitted which does not comply with these terms.
*Correspondence: Appakkudal R. Anand, YXJhbmFuZEBnbWFpbC5jb20=