- 1Department of Prosthetic Dentistry, Center for Dental and Oral Medicine, University Medical Center Hamburg-Eppendorf, Hamburg, Germany
- 2Department of General and Interventional Cardiology, University Heart Center Hamburg (UHZ), University Medical Center Hamburg-Eppendorf, Hamburg, Germany
- 3Deutsches Zentrum für Herz-Kreislauf-Forschung (DZHK), Partner Site Hamburg/Lübeck/Kiel, Hamburg, Germany
- 4Center of Dento-Maxillo-Facial Medicine, Department of Periodontology and Synoptic Dentistry, Charité – Universitätsmedizin Berlin, corporate member of Freie Universität Berlin, Humboldt-Universität zu Berlin, and Berlin Institute of Health, Berlin, Germany
- 5Institute for Cardiogenetics, University of Lübeck, Lübeck, Germany
- 6University Heart Center Lübeck, Lübeck, Germany
Periodontitis (PD) is a common gingival infectious disease caused by an over-aggressive inflammatory reaction to dysbiosis of the oral microbiome. The disease induces a profound systemic inflammatory host response, that triggers endothelial dysfunction and pro-thrombosis and thus may aggravate atherosclerotic vascular disease and its clinical complications. Recently, a risk haplotype at the ANRIL/CDKN2B-AS1 locus on chromosome 9p21.3, that is not only associated with coronary artery disease / myocardial infarction (CAD/MI) but also with PD, could be identified by genome-wide association studies. The locus encodes ANRIL - a long non-coding RNA (lncRNA) which, like other lncRNAs, regulates genome methylation via interacting with specific DNA sequences and proteins, such as DNA methyltranferases and polycomb proteins, thereby affecting expression of multiple genes by cis and trans mechanisms. Here, we describe ANRIL regulated genes and metabolic pathways and discuss implications of the findings for target identification of drugs with potentially anti-inflammatory activity in general.
Introduction
Periodontitis (PD) is an inflammatory disease that involves the osseous, connective, and epithelial, tissues surrounding the teeth (1). Bacteria attached to the teeth along the gingival margin form a biofilm, which may trigger an immune response in the adjacent gingival tissue. If the biofilm is not removed and persists, it can induce gingivitis characterized by swelling, redness and bleeding (2). If the bacterial biofilm and the accompanying inflammatory reaction migrate apically along the root surface and penetrate into the tooth supporting structures the gingival inflammation becomes PD (3), which exists in two forms, chronic periodontitis (CP) and a more severe, early onset form called aggressive periodontitis (AgP) (4). In the US almost 50% of adults aged 30 years or above have CP, including 30% with moderate and 8.5% with severe PD (5). Compared with CP, AgP is less frequent (prevalence: <0.1%). PD is a complex inflammatory disease, which is influenced considerably by interactions between environmental, lifestyle and genetic factors. Some individuals develop PD at young age, although they have similar lifestyle habits and environmental context compared to individuals who do not develop the disease. Therefore, it is considered that early-age of disease onset often indicates a genetic predisposition (6). The genetic susceptibility to PD has been examined extensively by GWAS (7–10) and seven common variants were identified, three of which met the genome-wide significance thresholds. Of the latter three, one (GLT6D1, glycosyltransferase 6 domain containing 1) is specific for AgP, whereas the other two (SIGLEC5, sialic acid binding Ig like lectin 5; DEFA1A3, defensin alpha 1/alpha 3) are associated with both AgP and CP (8, 10, 11). However, to date no associations that met the genome-wide significance threshold for common and rare alleles could be identified for CP alone. It is considered that these not signnificant findings are caused by the small sample sizes that were employed. Yet, some loci give suggestive evidence for association with PD. This evidence is based on independent replication in samples of the same disease phenotype with sufficient statistical power, independent validation of the associations in samples of different disease manifestations, like AgP and CP, and independent identification through different unbiased systematic approaches. According to these criteria, the following loci in addition to GLT6D1, SIGLEC5 and DEFA1A3 may currently be considered to be associated with CP and/or AgP: ANRIL (antisense noncoding RNA in the INK4 locus), NPY (neuropeptide Y), PF4 (platelet factor 4), PLG (plasminogen), VAMP3 (vesicle associated membrane protein 3) (10, 12–20).
Results obtained from longitudinal epidemiological studies support that CAD and CP are associated with each other (21), although the causative relationship between CAD and CP has remained ambiguous (22). Interestingly, variants at ANRIL, PLG and VAMP3 were reported to be associated with periodontal phenotypes and also with CAD [recently reviewed in ref. (23)]. Of these, ANRIL is the most significant risk locus of CAD and the association of ANRIL with PD was replicated repeatedly. In this narrative review, we summarize recent publications on the impact of this locus on chronic inflammation and to discuss potential approaches and strategies to identify new drug targets related to anti-inflammatory therapies in general.
The Chr.9p21.3 Risk Region Is Shared Between Periodontitis and CAD/MI and Affects Gene Expression of Multiple Genes in Different Cell Types
The 9p21.3 risk haplotype at ANRIL/CDKN2B-AS1 had initially been identified by GWAS of CAD (24), and was shortly later identified by Schaefer et al. as one of the first genetic risk factors of AgP (17, 25–27) [see (Table 1) for a comparison of the association statistics of the relevant 9p21.3 lead SNPs related to AgP and coronary heart disease].

Table 1. Summary of the Chromosome 9p21.3 Locus Associated with Coronary Artery Disease and Periodontitis.
The core risk haplotype of ~50 kb, that is shared between CAD/MI and PD encodes the 3’end of a long ncRNA called “antisense non-coding RNA in the INK4 locus (ANRIL)” (also designated CDKN2BAS) (17, 25). Its sequence is oriented antisense relative to cyclin-dependent kinase inhibitor 2B (CDKN2B), which is located adjacent to the core CAD/PD region. Together with CDKN2A, which is located further upstream of ANRIL, this region harbors a hotspot for multiple complex human diseases and traits (28). Adjacent is a tightly linked locus for diabetes (29) which is neither associated with CAD (29) nor PD (17).
Given the extended region of high linkage disequilibrium at the 9p21.3 locus and the large number of transcriptional regulatory elements that are present in the CAD risk region, it is currently not entirely clear whether the risk of CAD and PD is mediated solely by ANRIL or whether its neighbors, CDKN2B and CDKN2A - two well-known tumor suppressor genes involved in cell cycle arrest and malignant transformation in certain cancers (30) - contribute to the mechanism. Knockout mice lacking CDKN2B do not only develop a cancer-related phenotype but also advanced aneurysms, accelerated smooth muscle cell apoptosis and medial arterial thinning (31), suggesting a potential involvement of CDKN2B not only in cancer but also in vascular disease. CAD risk SNP rs1537373 affects CDKN2B expression in human coronary artery smooth muscle cells, aorta and the mammary artery (32), and CDKN2B has been shown to regulate inflammatory cytokine production and the clearance of smooth muscle cell-derived apoptotic bodies during atherosclerosis (33). Miller et al. (32) recently investigated the role of SNP rs1537373 in the expression of ANRIL. This variant resides in a large haplotype block of linked variants including the highly replicated CAD SNP, rs4977574 and the CAD and PD lead SNP rs1333049 (17, 34). Although rs1537373 does not affect a known transcription factor binding motif, it is located at a site of accessible chromatin. Allele-specific transcription factor binding and histone H3 lysine 27 acetylation around rs1537373 indicated that the native chromatin structure may be affected by the genotype, which was consistent with the observed cis eQTL affecting CDKN2B rather than ANRIL in aortic tissues (32). It appears noteworthy in this context that SNP rs1537373 was earlier demonstrated to be also strongly associated with coronary artery calcification (35). If bone marrow lacking murine Cdkn2a was transplanted to the atherosclerosis prone Ldlr(-/-) mouse model, the Cdkn2a-deficient recipients exhibited accelerated atherosclerosis, a higher number of pro-inflammatory monocytes, and increased monocyte/macrophage proliferation compared to controls (36). Thus besides CDKN2B, also CDKN2A has some plausibility for being involved in the pathogenesis of vascular inflammation [see the review by Hannou et al. (37) for further information].
The location of the core risk haplotype of CAD/MI and PD at the 3’end of ANRIL implies that the encoded long ncRNA is a prime functional candidate involved in the risk mediating mechanism(s). ANRIL is a lowly expressed gene consisting of 20 exons whose transcripts could be detected in a wide variety of cell-types and tissues, including smooth muscle cells, endothelial cells, and cells of the immune system that are known to be involved in atherogenesis (29, 38, 39). Originally, two splice variants were demonstrated in normal human testis and signals using PCR with primers derived from exons 14–16 were also obtained in a range of other tissues (40). Subsequently, many additional splice variants could be identified in various cell-types (38, 41, 42). ANRIL is subject to a complex pathway of alternative splicing which may differ from tissue to tissue and which may be influenced by the presence of SNPs interfering with the function of splice signals.
ANRIL expression was reported to be tightly linked to the ANRIL genotype due to disruption of an inhibitory STAT1 binding site in risk allele carriers (43), which would be expected to impair the IFNγ signaling response. However, results published by Almontashiri et al. argued against an involvement of IFNγ in the mechanism underlying the association of the 9p21.3 genotype with CAD risk (44). The CAD risk allele of SNP rs564398, which is one of the SNPs most strongly correlated with ANRIL expression, was predicted to disrupt a Ras Responsive Element Binding protein (RREB) 1 binding site in the 9p21.3 locus (45, 46). RREB may be involved in up-regulating CDKN2B in a Ras-dependent manner by down-regulating ANRIL. Besides stimulating VSMC senescence, Ras has also been implicated to contribute to atherogenesis by affecting vascular inflammation (47). The local functional influence of variants in the 9p21.3 region on gene expression has been examined by many other studies in a variety of tissues and cells (41, 45, 48–52). The results confirmed that the CAD risk variants in the 9p21.3 region are strongly associated with ANRIL expression and also with expression of the adjacent loci (CDKN2A, CDKN2B), albeit much more moderately. However, there is some inconsistency concerning the direction of the effect. Earlier studies suggested associations between CAD risk variants and lower ANRIL expression in vascular smooth muscle cells, whole blood cells and purified peripheral blood T-cells (49, 53, 54). In contrast, the study by Holdt et al. (51), in which specifically the long ANRIL transcript (ENST00000428597) was measured, demonstrated that the CAD risk haplotype was associated with higher ANRIL expression in whole blood cells and peripheral blood mononuclear cells. Also Zhao et al. found higher expression of this transcript in transformed beta-lymphocytes collected from genotyped donors who carried the CAD risk variant rs7865618 (55). In the latter study, all CAD risk variants assayed in the study were associated with the same directions of the effects.
In addition to the linear form of ANRIL, there also exists a circular ANRIL RNA form (38). Recently, Holdt et al. (56) showed that circular ANRIL may be athero-protective by regulating rRNA maturation. In their model, pescadillo homologue 1 (PES1, a 60S-preribosomal assembly factor) binds to circular ANRIL, which impairs ribosome biogenesis and exonuclease-mediated pre-rRNA processing. The resulting nucleolar stress induces activation of p53, which triggers apoptosis and inhibits proliferation, thereby preventing the accumulation of vascular smooth muscle cells and foam cells at the sites of the atherosclerotic lesion. The balance between atherogenic linear and athero-protective circular ANRIL may be critical for the impact of ANRIL on disease progression. Conversely, a recently published study came to the opposite conclusion, namely that circular ANRIL may be pro-atherogenic (57). In this study, circular antisense ANRIL was used to investigate the inflammatory response of vascular endothelial cells in vivo in a rat model of coronary atherosclerosis which was established by injecting rats on a high fat diet with vitamin D3 (57). Circular antisense ANRIL lowered circular ANRIL in vascular endothelial cells along with the levels of several pro-atherogenic markers (serum cholesterol, triglycerides, LDL, IL-1, IL-6, MMP-9, CRP, cANRIL, Bax, caspase-3) and the rates of endothelial cell apoptosis, while HDL levels and bcl-2 expression were increased. In contrast, induction of circular ANRIL expression promoted atherosclerosis by increasing pro-inflammatory properties in vascular endothelial cells and by raising serum lipid and pro-inflammatory cytokine levels. These results were consistent with the hypothesis, that inhibiting circular ANRIL expression would be anti-inflammatory and would reduce vascular endothelial cell apoptosis, which in turn would protect against atherosclerosis in this animal model.
In earlier studies, it could be demonstrated that the epigenetic silencer polycomb repressive complexes 1 and 2 (PRC1 and PRC2) and PRC-associated activating proteins RYBP and YY1 can bind to ANRIL (58, 59), suggesting that ANRIL may be able to modulate epigenetic regulation of target gene expression in cis and trans. It could be demonstrated in vitro by inducible knock-down approaches in T-Rex 293 HEK cells that silencing of two proximal ANRIL transcripts altered expression of ADIPOR1, VAMP3 and TMEM258 (60) (see Table 2 for a list of genes regulated by ANRIL). ADIPOR1 is a high-affinity receptor for globular adiponectin, which is involved, amongst others, in PPARα (peroxisome proliferator activated receptor alpha) and AMPK (AMP-activated protein kinase) signaling (62). PPARα activation could prevent experimentally induced bone-loss in animal studies (63). AMPK and PPARα act as key regulators of glucose and fatty acid metabolism in the liver. Adiponectin levels are inversely correlated with BMI, body fat and severity of CAD (64). Globular adiponectin also increases insulin sensitivity by stimulating cellular glucose uptake via increasing recruitment of glucose transporter 4 (GLUT4) to the plasma membrane and inducing GLUT4 expression (65). Besides these metabolic roles, adiponectin also has anti-inflammatory activity by activating tissue inhibitors of metalloproteinases, IL-10, and by suppressing lipopolysaccharide-activated TNF (tumor necrosis factor) expression and phagocytic activity (66, 67). The effect of ANRIL on VAMP3 expression (Table 2) may be important, because VAMP3 belongs to the VAMP/synaptobrevin family involved in phagocytosis and trafficking of TNF-α-containing secretory vesicles to the cell surface required for TNF-α secretion (68).
Genome-wide cis and trans effects of the variants in the 9p21.3 region on gene expression were recently studied by Zhao et al. (55), who employed the SNP-set (Sequence) Kernel Association Test [SKAT, (69)] on genotyped transformed beta-lymphocytes collected from 801 participants from the Genetic Epidemiology Network of Arteriopathy (GENOA) study. The results demonstrated a significant association between the CAD and PD risk variants in the region with the expression of the long linear ANRIL transcript containing the coding information of all 20 exons except exon 13. In addition to this cis-regulatory effect, several trans eQTLs could also be identified (Table 2). The affected genes were DUT (Deoxyuridine Triphosphatase also known as UTPase), EIF1AY (Eukaryotic Translation Initiation Factor 1A, Y-Linked), CASP14 (Caspase 14), ABCA1 (ATP-binding cassette transporter A1), and DHRS9 (Dehydrogenase/Reductase 9) (Table 2) (55).
The DUT gene product is an essential enzyme of nucleotide metabolism, which is required for the hydrolysis of dUTP into dUMP and inorganic pyrophosphate. The enzyme plays an important role in controlling the relative cellular levels of dUTP/dTTP (70). Lack or inhibition of dUTPase result in elevated levels of uracil in the DNA, which triggers DNA repair and may induce the formation of DNA double strand breaks, somatic mutations, and apoptosis (71).
CASP14 is involved in cell apoptosis and is over-expressed in skin, the oral epithelium, bone, heart, and epithelial tumors (72). EIF1AY encodes a translation initiation factor which seems to be required for maximal rate of protein biosynthesis (73) and DHRS9 is involved in retinol and steroid metabolism (74). ABCA1 plays a well-known role in atherosclerosis (75); but its contribution to PD is unclear. It was proposed that LPS from P. gingivalis, which is the most important pathogen involved in PD, may suppress ABCA1 expression during periodontitis via miRNA-mediated mechanisms (76). To further investigate the potential biological implications of the trans-effected genes, Zhao et al. (55) performed gene enrichment analysis on basis of the KEGG Pathway databank. The enriched pathways included “retinol metabolism”, “TGF-β signaling”, and “N-glycan biosynthesis”. Retinol metabolism was at the top of the list of enriched pathways, in which LRAT (lecithin retinol acyltransferase), ADH1 (alcohol dehydrogenase 1), DHRS9, DHRS4L2 (dehydrogenase/reductase 9 and 4 like 2), and CYP26B1 (cytochrome P450 retinoid metabolizing protein) were significantly associated. The importance of TGF-β signaling in the pathogenesis of PD is well-known, since anti-TGF-β antibodies can inhibit the recruitment of leukocytes and the destruction of cartilage and bone at the periodontal lesion sites during periodontitis (77). Another reported downstream target regulated by ANRIL is CARD8 (caspase recruitment domain-containing protein 8) (Table 2) (61). The CARD8 SNP rs2043211 is significantly associated with ischemic stroke; but its involvement in PD is unclear. The CARD8 gene product is a component of the inflammasome together with other proteins. ANRIL is induced by pro-inflammatory factors, such as TNFα and IFN-γ, via activation of NF-κB (Figure 1) (78). The transcription factor Yin yang 1 (YY1) can bind to ANRIL and the ANRIL-YY1 complex interacts with the promoter of IL6/8 to activate IL6 and IL8 expression, two cytokines with well established roles in CAD/MI and PD.
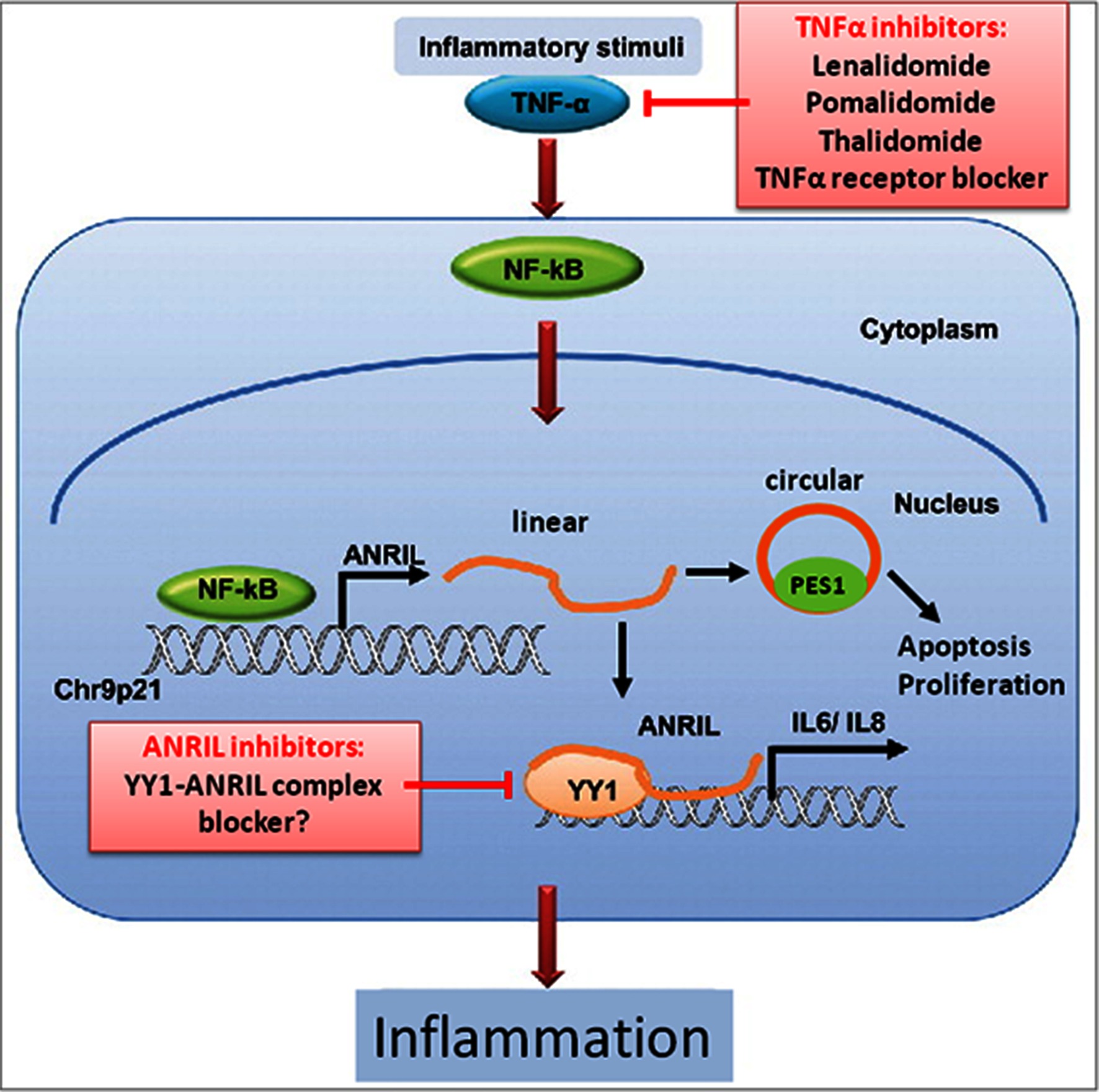
Figure 1. Hypothetical roles of linear and circular ANRIL lncRNA in regulating inflammation and cell survival in human vascular endothelial cells and potential drug targets. TNF-α triggers NF-κB activation, which induces ANRIL transcription (66). Linear ANRIL can be converted to circular ANRIL (38). Linear ANRIL interacts with the transcription factor yin yang-1 (YY1) to form a functional complex that binds to and regulates expression of target genes such as IL-6/8. Circular ANRIL interacts with pescadillo homologue 1 (PES1) to form a complex with the pre-ribosomal assembly complex, that impairs ribosome biogenesis, leading to activation of p53 and a subsequent increase in apoptosis and decrease in the proliferative rate (41). This pathway may promote atheroprotection by eliminating over-proliferating cells in atherosclerotic plaques. Neither TNFα nor NF-κB antagonists do seem suitable for wide-spread use in anti-inflammatory therapies of PD or CAD, because of their serious side effects. Since ANRIL is located downstream of TNFα and NF-κB, ANRIL or its downstream targets may be better suited as drug targets to inhibit the pro-inflammatory activities linked to this signaling pathway [modified according to ref. (78)].
Taken together, these findings seem to suggest that ANRIL exerts its effects through epigenetic regulation of a great variety of target genes. The common theme seems to be its involvement in expression regulation of genes that play important roles in inflammation, immunity, cell apoptosis and survival, cell proliferation, and metabolism. Many of the reported trans regulated genes clearly have plausible roles in CAD and PD as well. Nevertheless, at this stage, we find it premature to formulate a unifying theory that would be consistent with at least the majority of the findings. Most concerning is the apparent complete lack of replication of trans regulated genes between the published studies. The reasons for this striking inconsistency may have something to do with the diversity of the experimental approaches and cell-types that have been employed to date. The genome-wide approaches may lack sufficient power to detect some of the differentially expressed genes identified by targeted strategies (55). Antisense approaches are difficult to control due to the complex cell-type specific alternative splicing pathways (38, 41, 42) and findings coming from rodent animal models may not be relevant for humans, since rodent and human ANRIL are evolutionary not well conserved and they differ structurally substantially from each other (79).
Implications of the Chr.9p21.3 ANRIL Locus for Drug Target Identification
Zhou et al. (78) showed that ANRIL expression is up-regulated via the TNFα/NF-κB signaling pathway under inflammatory stress conditions (Figure 1). Since endothelial cell-specific inhibition of NF-κB protects mice from atherosclerosis (80), and since ANRIL is a downstream target of TNFα/NF-κB signaling, targeting TNFα or NF-κB may theoretically be considered to be athero-protective via inhibiting ANRIL-YY1-mediated IL-6/8 production. Several TNFα receptor antagonists (mostly antibodies) have been tested for safety and efficiency for modulating pro-inflammatory cytokine release in the treatment of rheumatoid arthritis (81). However, clinical trials have shown that these receptor antagonists are associated with increased risks of malignancies and serious infections (81). Since ANRIL is located downstream of TNFα and NF-κB, it may be better suited as drug target. However, given the important role of ANRIL transcripts in controlling cell growth, its expression is likely precisely regulated. Possibly, putative drug targeting options may come to mind from a better understanding of the precise downstream effects of the linear and circular ANRIL lncRNAs on expression of genes involved in chronic inflammatory pathways, suggesting that such work has potential to identify new drug targets for anti-inflammatory intervention.
Author Contributions
GA and US had the initial idea of writing a review and proposed the topic. Moreover, they conducted extensive literature search and created the first draft of the manuscript. TZ, GH, AS and MM integrated the different information and also wrote and submited the manuscript.
Funding
No third party funds were used for this work.
Conflict of Interest Statement
The authors declare that the research was conducted in the absence of any commercial or financial relationships that could be construed as a potential conflict of interest.
Acknowledgments
GA, TZ, and GH are employed at and receive salaries from the University Medical Center Hamburg-Eppendorf, US is employed at the University Medical Center Hamburg-Eppendorf and receives his salary from a grant provided by the Else Kröner-Fresenius Foundation, AS and MM are employed at and receive a salary from the Charité - Universitätsmedizin Berlin.
References
1. Kinane DF, Loe H, Theilade E, Jensen SB, Kinane DF, Loe H et al Pathogenesis of Periodontitis. In: Lindhe J, Lang NP, Karring T, editors. Clinical Periodontology and Implant Dentistry. Oxford: Blackwell Munksgaard (2008). p. 256–69.
2. Loe H, Theilade E, Jensen SB. Experimental gingivitis in man. J Periodontol (1965) 36:177–87. doi: 10.1902/jop.1965.36.3.177
4. Kinane DF. Berglundh: Pathogenesis of Periodontitis. In: Lindhe J, Lang NP, Karring T, editors. Clinical Periodontology and Implant Dentistry. Oxford: Blackwell Munksgaard (2008).
5. Thornton-Evans G, Eke P, Wei L, Palmer A, Moeti R, Hutchins S, et al. Periodontitis among adults aged ≥30 years - United States, 2009-2010. MMWR supplements (2013) 62(3):129–35.
6. Vieira AR, Albandar JM. Role of genetic factors in the pathogenesis of aggressive periodontitis. Periodontol. 2000 (2014) 65(1):92–106. doi: 10.1111/prd.12021
7. Divaris K, Monda KL, North KE, Olshan AF, Reynolds LM, Hsueh WC, et al. Exploring the genetic basis of chronic periodontitis: a genome-wide association study. Hum Mol Genet (2013) 22(11):2312–24. doi: 10.1093/hmg/ddt065
8. Schaefer AS, Richter GM, Nothnagel M, Manke T, Dommisch H, Jacobs G, et al. A genome-wide association study identifies GLT6D1 as a susceptibility locus for periodontitis. Hum Mol Genet (2010) 19(3):553–62. doi: 10.1093/hmg/ddp508
9. Teumer A, Holtfreter B, Völker U, Petersmann A, Nauck M, Biffar R, et al. Genome-wide association study of chronic periodontitis in a general German population. J Clin Periodontol (2013) 40(11):977–85. doi: 10.1111/jcpe.12154
10. Munz M, Willenborg C, Richter GM, Jockel-Schneider Y, Graetz C, Staufenbiel I, et al. A genome-wide association study identifies nucleotide variants at SIGLEC5 and DEFA1A3 as risk loci for periodontitis. Hum Mol Genet (2017) 26(13):2577–88. doi: 10.1093/hmg/ddx151
11. Hashim NT, Linden GJ, Ibrahim ME, Gismalla BG, Lundy FT, Hughes FJ, et al. Replication of the association of GLT6D1 with aggressive periodontitis in a Sudanese population. J Clin Periodontol (2015) 42(4):319–24. doi: 10.1111/jcpe.12375
12. Freitag-Wolf S, Dommisch H, Graetz C, Jockel-Schneider Y, Harks I, Staufenbiel I, et al. Genome-wide exploration identifies sex-specific genetic effects of alleles upstream NPY to increase the risk of severe periodontitis in men. J Clin Periodontol (2014) 41(12):1115–21. doi: 10.1111/jcpe.12317
13. Bochenek G, Häsler R, El Mokhtari NE, König IR, Loos BG, Jepsen S, et al. The large non-coding RNA ANRIL, which is associated with atherosclerosis, periodontitis and several forms of cancer, regulates ADIPOR1, VAMP3 and C11ORF10. Hum Mol Genet (2013) 22(22):4516–27. doi: 10.1093/hmg/ddt299
14. Divaris K, Monda KL, North KE, Olshan AF, Lange EM, Moss K, et al. Genome-wide association study of periodontal pathogen colonization. J Dent Res (2012) 91(7 Suppl):S21–8. doi: 10.1177/0022034512447951
15. Munz M, Chen H, Jockel-Schneider Y, Adam K, Hoffman P, Berger K, et al. A haplotype block downstream of plasminogen is associated with chronic and aggressive periodontitis. J Clin Periodontol (2017) 44(10):962–70. doi: 10.1111/jcpe.12749
16. Schaefer AS, Bochenek G, Jochens A, Ellinghaus D, Dommisch H, Güzeldemir-Akçakanat E, et al. Genetic evidence for PLASMINOGEN as a shared genetic risk factor of coronary artery disease and periodontitis. Circ Cardiovasc Genet (2015) 8(1):159-67–167. doi: 10.1161/CIRCGENETICS.114.000554
17. Schaefer AS, Richter GM, Groessner-Schreiber B, Noack B, Nothnagel M, El Mokhtari NE, et al. Identification of a shared genetic susceptibility locus for coronary heart disease and periodontitis. PLoS Genet (2009) 5(2):e1000378. doi: 10.1371/journal.pgen.1000378
18. Ernst FD, Uhr K, Teumer A, Fanghänel J, Schulz S, Noack B, et al. Replication of the association of chromosomal region 9p21.3 with generalized aggressive periodontitis (gAgP) using an independent case-control cohort. BMC Med Genet (2010) 11(1):119. doi: 10.1186/1471-2350-11-119
19. Shusterman A, Munz M, Richter G, Jepsen S, Lieb W, Krone B, et al. The PF4/PPBP/CXCL5 gene cluster is associated with periodontitis. J Dent Res (2017) 96(8):945–52. doi: 10.1177/0022034517706311
20. Schaefer AS, Bochenek G, Jochens A, Ellinghaus D, Dommisch H, Güzeldemir-Akçakanat E, et al. Genetic evidence for PLASMINOGEN as a shared genetic risk factor of coronary artery disease and periodontitis. Circ Cardiovasc Genet (2015) 8(1):159–67. doi: 10.1161/CIRCGENETICS.114.000554
21. Dietrich T, Jimenez M, Krall Kaye EA, Vokonas PS, Garcia RI. Age-dependent associations between chronic periodontitis/edentulism and risk of coronary heart disease. Circulation (2008) 117(13):1668–74. doi: 10.1161/CIRCULATIONAHA.107.711507
22. Lockhart PB, Bolger AF, Papapanou PN, Osinbowale O, Trevisan M, Levison ME, et al. Periodontal disease and atherosclerotic vascular disease: does the evidence support an independent association?: a scientific statement from the American Heart Association. Circulation (2012) 125(20):2520–44. doi: 10.1161/CIR.0b013e31825719f3
23. Aarabi G, Zeller T, Seedorf H, Reissmann DR, Heydecke G, Schaefer AS, et al. Genetic susceptibility contributing to periodontal and cardiovascular disease. J Dent Res (2017) 96(6):610–7. doi: 10.1177/0022034517699786
24. Mcpherson R, Pertsemlidis A, Kavaslar N, Stewart A, Roberts R, Cox DR, et al. A common allele on chromosome 9 associated with coronary heart disease. Science (2007) 316(5830):1488–91. doi: 10.1126/science.1142447
25. Ernst FD, Uhr K, Teumer A, Fanghänel J, Schulz S, Noack B, et al. Replication of the association of chromosomal region 9p21.3 with generalized aggressive periodontitis (gAgP) using an independent case-control cohort. BMC Med Genet (2010) 11:119. doi: 10.1186/1471-2350-11-119
26. Schaefer AS, Richter GM, Dommisch H, Reinartz M, Nothnagel M, Noack B, et al. CDKN2BAS is associated with periodontitis in different European populations and is activated by bacterial infection. J Med Genet (2011) 48(1):38–47. doi: 10.1136/jmg.2010.078998
27. Schaefer AS, Bochenek G, Manke T, Nothnagel M, Graetz C, Thien A, et al. Validation of reported genetic risk factors for periodontitis in a large-scale replication study. J Clin Periodontol (2013) 40(6):563–72. doi: 10.1111/jcpe.12092
28. Pasmant E, Sabbagh A, Vidaud M, Bièche I. ANRIL, a long, noncoding RNA, is an unexpected major hotspot in GWAS. Faseb J (2011) 25(2):444–8. doi: 10.1096/fj.10-172452
29. Broadbent HM, Peden JF, Lorkowski S, Goel A, Ongen H, Green F, et al. Susceptibility to coronary artery disease and diabetes is encoded by distinct, tightly linked SNPs in the ANRIL locus on chromosome 9p. Hum Mol Genet (2008) 17(6):806–14. doi: 10.1093/hmg/ddm352
30. Boström J, Meyer-Puttlitz B, Wolter M, Blaschke B, Weber RG, Lichter P, et al. Alterations of the tumor suppressor genes CDKN2A (p16(INK4a)), p14(ARF), CDKN2B (p15(INK4b)), and CDKN2C (p18(INK4c)) in atypical and anaplastic meningiomas. Am J Pathol (2001) 159(2):661–9. doi: 10.1016/S0002-9440(10)61737-3
31. Leeper NJ, Raiesdana A, Kojima Y, Kundu RK, Cheng H, Maegdefessel L, et al. Loss of CDKN2B promotes p53-dependent smooth muscle cell apoptosis and aneurysm formation. Arterioscler Thromb Vasc Biol (2013) 33(1):e1–e10. doi: 10.1161/ATVBAHA.112.300399
32. Miller CL, Pjanic M, Wang T, Nguyen T, Cohain A, Lee JD, et al. Integrative functional genomics identifies regulatory mechanisms at coronary artery disease loci. Nat Commun (2016) 7:12092. doi: 10.1038/ncomms12092
33. Kojima Y, Downing K, Kundu R, Miller C, Dewey F, Lancero H, et al. Cyclin-dependent kinase inhibitor 2B regulates efferocytosis and atherosclerosis. J Clin Invest (2014) 124(3):1083–97. doi: 10.1172/JCI70391
34. Samani NJ, Erdmann J, Hall AS, Hengstenberg C, Mangino M, Mayer B, et al. Genomewide association analysis of coronary artery disease. N Engl J Med (2007) 357(5):443–53. doi: 10.1056/NEJMoa072366
35. Pechlivanis S, Mühleisen TW, Möhlenkamp S, Schadendorf D, Erbel R, Jöckel KH, et al. Risk loci for coronary artery calcification replicated at 9p21 and 6q24 in the Heinz Nixdorf Recall Study. BMC Med Genet (2013) 14:23. doi: 10.1186/1471-2350-14-23
36. Kuo CL, Murphy AJ, Sayers S, Li R, Yvan-Charvet L, Davis JZ, et al. Cdkn2a is an atherosclerosis modifier locus that regulates monocyte/macrophage proliferation. Arterioscler Thromb Vasc Biol (2011) 31(11):2483–92. doi: 10.1161/ATVBAHA.111.234492
37. Hannou SA, Wouters K, Paumelle R, Staels B. Functional genomics of the CDKN2A/B locus in cardiovascular and metabolic disease: what have we learned from GWASs? Trends Endocrinol Metab (2015) 26(4):176–84. doi: 10.1016/j.tem.2015.01.008
38. Burd CE, Jeck WR, Liu Y, Sanoff HK, Wang Z, Sharpless NE. Expression of linear and novel circular forms of an INK4/ARF-associated non-coding RNA correlates with atherosclerosis risk. PLoS Genet (2010) 6(12):e1001233. doi: 10.1371/journal.pgen.1001233
39. Holdt LM, Beutner F, Scholz M, Gielen S, Gäbel G, Bergert H, et al. ANRIL expression is associated with atherosclerosis risk at chromosome 9p21. Arterioscler Thromb Vasc Biol (2010) 30(3):620–7. doi: 10.1161/ATVBAHA.109.196832
40. Pasmant E, Laurendeau I, Héron D, Vidaud M, Vidaud D, Bièche I. Characterization of a germ-line deletion, including the entire INK4/ARF locus, in a melanoma-neural system tumor family: identification of ANRIL, an antisense noncoding RNA whose expression coclusters with ARF. Cancer Res (2007) 67(8):3963–9. doi: 10.1158/0008-5472.CAN-06-2004
41. Folkersen L, Kyriakou T, Goel A, Peden J, Mälarstig A, Paulsson-Berne G, et al. Relationship between CAD risk genotype in the chromosome 9p21 locus and gene expression. Identification of eight new ANRIL splice variants. PLoS ONE (2009) 4(11):e7677. doi: 10.1371/journal.pone.0007677
42. Holdt LM, Hoffmann S, Sass K, Langenberger D, Scholz M, Krohn K, et al. Alu elements in ANRIL non-coding RNA at chromosome 9p21 modulate atherogenic cell functions through trans-regulation of gene networks. PLoS Genet (2013) 9(7):e1003588. doi: 10.1371/journal.pgen.1003588
43. Harismendy O, Notani D, Song X, Rahim NG, Tanasa B, Heintzman N, et al. 9p21 DNA variants associated with coronary artery disease impair interferon-γ signalling response. Nature (2011) 470(7333):264–8. doi: 10.1038/nature09753
44. Almontashiri NA, Fan M, Cheng BL, Chen HH, Roberts R, Stewart AF. Interferon-γ activates expression of p15 and p16 regardless of 9p21.3 coronary artery disease risk genotype. J Am Coll Cardiol (2013) 61(2):143–7. doi: 10.1016/j.jacc.2012.08.1020
45. Congrains A, Kamide K, Oguro R, Yasuda O, Miyata K, Yamamoto E, et al. Genetic variants at the 9p21 locus contribute to atherosclerosis through modulation of ANRIL and CDKN2A/B. Atherosclerosis (2012) 220(2):449–55. doi: 10.1016/j.atherosclerosis.2011.11.017
46. Gschwendtner A, Bevan S, Cole JW, Plourde A, Matarin M, Ross-Adams H, et al. Sequence variants on chromosome 9p21.3 confer risk for atherosclerotic stroke. Ann Neurol (2009) 65(5):531–9. doi: 10.1002/ana.21590
47. Minamino T, Yoshida T, Tateno K, Miyauchi H, Zou Y, Toko H, et al. Ras induces vascular smooth muscle cell senescence and inflammation in human atherosclerosis. Circulation (2003) 108(18):2264–9. doi: 10.1161/01.CIR.0000093274.82929.22
48. Jarinova O, Stewart AF, Roberts R, Wells G, Lau P, Naing T, et al. Functional analysis of the chromosome 9p21.3 coronary artery disease risk locus. Arterioscler Thromb Vasc Biol (2009) 29(10):1671–7. doi: 10.1161/ATVBAHA.109.189522
49. Liu Y, Sanoff HK, Cho H, Burd CE, Torrice C, Mohlke KL, et al. INK4/ARF transcript expression is associated with chromosome 9p21 variants linked to atherosclerosis. PLoS ONE (2009) 4(4):e5027. doi: 10.1371/journal.pone.0005027
50. Deloukas P, Kanoni S, Willenborg C, Farrall M, Assimes TL, Thompson JR, et al. Large-scale association analysis identifies new risk loci for coronary artery disease. Nat Genet (2013) 45(1):25–33. doi: 10.1038/ng.2480
51. Holdt LM, Beutner F, Scholz M, Gielen S, Gäbel G, Bergert H, et al. ANRIL expression is associated with atherosclerosis risk at chromosome 9p21. Arterioscler Thromb Vasc Biol (2010) 30(3):620–7. doi: 10.1161/ATVBAHA.109.196832
52. Congrains A, Kamide K, Katsuya T, Yasuda O, Oguro R, Yamamoto K, et al. CVD-associated non-coding RNA, ANRIL, modulates expression of atherogenic pathways in VSMC. Biochem Biophys Res Commun (2012) 419(4):612–6. doi: 10.1016/j.bbrc.2012.02.050
53. Cunnington MS, Santibanez Koref M, Mayosi BM, Burn J, Keavney B. Chromosome 9p21 SNPs associated with multiple disease phenotypes correlate with ANRIL expression. PLoS Genet (2010) 6(4):e1000899. doi: 10.1371/journal.pgen.1000899
54. Motterle A, Pu X, Wood H, Xiao Q, Gor S, Ng FL, et al. Functional analyses of coronary artery disease associated variation on chromosome 9p21 in vascular smooth muscle cells. Hum Mol Genet (2012) 21(18):4021–9. doi: 10.1093/hmg/dds224
55. Zhao W, Smith JA, Mao G, Fornage M, Peyser PA, Sun YV, et al. The cis and trans effects of the risk variants of coronary artery disease in the Chr9p21 region. BMC Med Genomics (2015) 8:21. doi: 10.1186/s12920-015-0094-0
56. Holdt LM, Stahringer A, Sass K, Pichler G, Kulak NA, Wilfert W, et al. Circular non-coding RNA ANRIL modulates ribosomal RNA maturation and atherosclerosis in humans. Nat Commun (2016) 7:12429. doi: 10.1038/ncomms12429
57. Song CL, Wang JP, Xue X, Liu N, Zhang XH, Zhao Z, et al. Effect of circular ANRIL on the inflammatory response of vascular endothelial cells in a rat model of coronary atherosclerosis. Cell Physiol Biochem (2017) 42(3):1202–12. doi: 10.1159/000478918
58. Kotake Y, Nakagawa T, Kitagawa K, Suzuki S, Liu N, Kitagawa M, et al. Long non-coding RNA ANRIL is required for the PRC2 recruitment to and silencing of p15(INK4B) tumor suppressor gene. Oncogene (2011) 30(16):1956–62. doi: 10.1038/onc.2010.568
59. González I, Busturia A. High levels of dRYBP induce apoptosis in Drosophila imaginal cells through the activation of reaper and the requirement of trithorax, dredd and dFADD. Cell Res (2009) 19(6):747–57. doi: 10.1038/cr.2009.29
60. Bochenek G, Häsler R, El Mokhtari NE, König IR, Loos BG, Jepsen S, et al. The large non-coding RNA ANRIL, which is associated with atherosclerosis, periodontitis and several forms of cancer, regulates ADIPOR1, VAMP3 and C11ORF10. Hum Mol Genet (2013) 22(22):4516–27. doi: 10.1093/hmg/ddt299
61. Bai Y, Nie S, Jiang G, Zhou Y, Zhou M, Zhao Y, et al. Regulation of CARD8 expression by ANRIL and association of CARD8 single nucleotide polymorphism rs2043211 (p.C10X) with ischemic stroke. Stroke (2014) 45(2):383–8. doi: 10.1161/STROKEAHA.113.003393
62. Yamauchi T, Nio Y, Maki T, Kobayashi M, Takazawa T, Iwabu M, et al. Targeted disruption of AdipoR1 and AdipoR2 causes abrogation of adiponectin binding and metabolic actions. Nat Med (2007) 13(3):332–9. doi: 10.1038/nm1557
63. Stunes AK, Westbroek I, Gustafsson BI, Fossmark R, Waarsing JH, Eriksen EF, et al. The peroxisome proliferator-activated receptor (PPAR) alpha agonist fenofibrate maintains bone mass, while the PPAR gamma agonist pioglitazone exaggerates bone loss, in ovariectomized rats. BMC Endocr Disord (2011) 11:11. doi: 10.1186/1472-6823-11-11
64. Hara K, Yamauchi T, Imai Y, Manabe I, Nagai R, Kadowaki T. Reduced adiponectin level is associated with severity of coronary artery disease. Int Heart J (2007) 48(2):149–53. doi: 10.1536/ihj.48.149
65. Ceddia RB, Somwar R, Maida A, Fang X, Bikopoulos G, Sweeney G. Globular adiponectin increases GLUT4 translocation and glucose uptake but reduces glycogen synthesis in rat skeletal muscle cells. Diabetologia (2005) 48(1):132–9. doi: 10.1007/s00125-004-1609-y
66. Kumada M, Kihara S, Ouchi N, Kobayashi H, Okamoto Y, Ohashi K, et al. Adiponectin specifically increased tissue inhibitor of metalloproteinase-1 through interleukin-10 expression in human macrophages. Circulation (2004) 109(17):2046–9. doi: 10.1161/01.CIR.0000127953.98131.ED
67. Yokota T, Oritani K, Takahashi I, Ishikawa J, Matsuyama A, Ouchi N, et al. Adiponectin, a new member of the family of soluble defense collagens, negatively regulates the growth of myelomonocytic progenitors and the functions of macrophages. Blood (2000) 96(5):1723–32.
68. Murray RZ, Kay JG, Sangermani DG, Stow JL. A role for the phagosome in cytokine secretion. Science (2005) 310(5753):1492–5. doi: 10.1126/science.1120225
69. Wu MC, Lee S, Cai T, Li Y, Boehnke M, Lin X. Rare-variant association testing for sequencing data with the sequence kernel association test. Am J Hum Genet (2011) 89(1):82–93. doi: 10.1016/j.ajhg.2011.05.029
70. Vértessy BG, Tóth J. Keeping uracil out of DNA: physiological role, structure and catalytic mechanism of dUTPases. Acc Chem Res (2009) 42(1):97–106. doi: 10.1021/ar800114w
71. Wilson PM, Labonte MJ, Lenz HJ, Mack PC, Ladner RD. Inhibition of dUTPase induces synthetic lethality with thymidylate synthase-targeted therapies in non-small cell lung cancer. Mol Cancer Ther (2012) 11(3):616–28. doi: 10.1158/1535-7163.MCT-11-0781
72. Krajewska M, Kim H, Shin E, Kennedy S, Duffy MJ, Wong YF, et al. Tumor-associated alterations in caspase-14 expression in epithelial malignancies. Clin Cancer Res (2005) 11(15):5462–71. doi: 10.1158/1078-0432.CCR-04-2527
73. Jackson RJ, Hellen CU, Pestova TV. The mechanism of eukaryotic translation initiation and principles of its regulation. Nat Rev Mol Cell Biol (2010) 11(2):113–27. doi: 10.1038/nrm2838
74. Soref CM, di YP, Hayden L, Zhao YH, Satre MA, Wu R. Characterization of a novel airway epithelial cell-specific short chain alcohol dehydrogenase/reductase gene whose expression is up-regulated by retinoids and is involved in the metabolism of retinol. J Biol Chem (2001) 276(26):24194–202. doi: 10.1074/jbc.M100332200
75. Cohen JC, Kiss RS, Pertsemlidis A, Marcel YL, Mcpherson R, Hobbs HH. Multiple rare alleles contribute to low plasma levels of HDL cholesterol. Science (2004) 305(5685):869–72. doi: 10.1126/science.1099870
76. Olsen I, Singhrao SK, Osmundsen H. Periodontitis, pathogenesis andprogression: miRNA-mediated cellular responses to. J Oral Microbiol (2017) 9(1):1333396.
77. Wahl SM, Allen JB, Costa GL, Wong HL, Dasch JR. Reversal of acute and chronic synovial inflammation by anti-transforming growth factor beta. J Exp Med (1993) 177(1):225–30. doi: 10.1084/jem.177.1.225
78. Zhou X, Han X, Wittfeldt A, Sun J, Liu C, Wang X, et al. Long non-coding RNA ANRIL regulates inflammatory responses as a novel component of NF-κB pathway. RNA Biol (2016) 13(1):98–108. doi: 10.1080/15476286.2015.1122164
79. He S, Gu W, Li Y, Zhu H. ANRIL/CDKN2B-AS shows two-stage clade-specific evolution and becomes conserved after transposon insertions in simians. BMC Evol Biol (2013) 13:247. doi: 10.1186/1471-2148-13-247
80. Gareus R, Kotsaki E, Xanthoulea S, van der Made I, Gijbels MJ, Kardakaris R, et al. Endothelial cell-specific NF-kappaB inhibition protects mice from atherosclerosis. Cell Metab (2008) 8(5):372–83. doi: 10.1016/j.cmet.2008.08.016
81. Bongartz T, Sutton AJ, Sweeting MJ, Buchan I, Matteson EL, Montori V. Anti-TNF antibody therapy in rheumatoid arthritis and the risk of serious infections and malignancies: systematic review and meta-analysis of rare harmful effects in randomized controlled trials. JAMA (2006) 295(19):2275–85. doi: 10.1001/jama.295.19.2275
Keywords: periodontitis, inflammation, ANRIL, 9p21.3, drug target, anti-inflammatory agents, coronary artery disease, CDKN2B-AS1
Citation: Aarabi G, Zeller T, Heydecke G, Munz M, Schäfer A and Seedorf U (2018). Roles of the Chr.9p21.3 ANRIL Locus in Regulating Inflammation and Implications for Anti-Inflammatory Drug Target Identification. Front. Cardiovasc. Med. 5:47. doi: 10.3389/fcvm.2018.00047
Received: 08 March 2018; Accepted: 01 May 2018;
Published: 18 May 2018
Reviewed by:
Tom Robert Webb, University of Leicester, United KingdomYuqi Zhao, University of California, Los Angeles, United States
Copyright © 2018 Aarabi, Zeller, Heydecke, Munz, Schäfer and Seedorf. This is an open-access article distributed under the terms of the Creative Commons Attribution License (CC BY). The use, distribution or reproduction in other forums is permitted, provided the original author(s) and the copyright owner are credited and that the original publication in this journal is cited, in accordance with accepted academic practice. No use, distribution or reproduction is permitted which does not comply with these terms.
*Correspondence: Udo Seedorf, u.seedorf@uke.de