- 1Division of Infections and Molecular Biology, Department of Health Promotion, Kyushu Dental University, Kitakyushu, Japan
- 2Division of Functional Interface Engineering, Department of Biological Systems and Engineering, Kyushu Institute of Technology, Kitakyushu, Japan
Persister cells are difficult to eliminate because they are tolerant to antibiotic stress. In the present study, using artificially induced Escherichia coli persister cells, we found that reactive oxygen species (ROS) have greater effects on persister cells than on exponential cells. Thus, we examined which types of ROS could effectively eliminate persister cells and determined the mechanisms underlying the effects of these ROS. Ultraviolet (UV) light irradiation can kill persister cells, and bacterial viability is markedly increased under UV shielding. UV induces the production of ROS, which kill bacteria by moving toward the shielded area. Electron spin resonance-based analysis confirmed that hydroxyl radicals are produced by UV irradiation, although singlet oxygen is not produced. These results clearly revealed that ROS sterilizes persister cells more effectively compared to the sterilization of exponential cells (**p < 0.01). These ROS do not injure the bacterial cell wall but rather invade the cell, followed by cell killing. Additionally, the sterilization effect on persister cells was increased by exposure to oxygen plasma during UV irradiation. However, vapor conditions decreased persister cell sterilization by reducing the levels of hydroxyl radicals. We also verified the effect of ROS against bacteria in biofilms that are more resistant than planktonic cells. Although UV alone could not completely sterilize the biofilm bacteria, UV with ROS achieved complete sterilization. Our results demonstrate that persister cells strongly resist the effects of antibiotics and starvation stress but are less able to withstand exposure to ROS. It was shown that ROS does not affect the cell membrane but penetrates it and acts internally to kill persister cells. In particular, it was clarified that the hydroxy radical is an effective sterilizer to kill persister cells.
Introduction
Commonly used sterilization techniques include autoclaving, ultraviolet (UV) irradiation treatment (Mori et al., 2007), ethylene oxide gas (EOG) exposure, (Shintani, 2017), and radiation sterilization (Goldman and Pruitt, 1998). These techniques are currently used to sterilize medical instruments and food. However, each method has limitations. For example, autoclaving is time-consuming and cannot be used for all materials. In UV irradiation, it is difficult to sterilize areas of an object not exposed to UV light. The EOG method shows residual gas effects after processing, and radiation sterilization is costly and energy-intensive. Thus, a rapid sterilization method that can access all parts of an object, is not toxic, does not produce hazardous waste, is low-cost, and consumes low levels of energy is needed.
Bacteria are resistant to various external stresses such as drugs and starvation by forming “persisters.” Persister bacteria were discovered in 1942 by Hobby et al. (1942). They found that 1% of wild-type Staphylococcus aureus survived after penicillin treatment. These cells were named as “persisters” by Bigger (1944). Persister cells are widely present and the phenotype is formed by many types of both Gram-negative and Gram-positive bacteria such as Escherichia coli (Balaban et al., 2004), Pseudomonas aeruginosa (Fisher et al., 2017), Enterococcus faecalis (Abranches et al., 2009; Gaca et al., 2015), S. aureus (Corrigan et al., 2016), and Salmonella enterica serovar Typhimurium (Helaine et al., 2014; Stapels et al., 2018). Additionally, persister cells account for <0.001% of the cell population in a non-stress environment but can reach as high as 1% in stationary-phase cultures and biofilms (Lewis, 2007, 2008). Persister cells are difficult to completely eliminate because of their high tolerance to stress such as antibiotics treatment (Lewis, 2012; Wood et al., 2019). Slight persister survival allows for cell regrowth and biofilm regeneration (Spoering and Lewis, 2001). Recently, studies on newer medicines to kill persister cells have been reported. For example, it has been reported that two synthetic retinoids (CD437 and CD1530) showed anti-persister activity against a methicillin-resistant S. aureus strain (Kim et al., 2018c). Although mitomycin C (Kwan et al., 2015; Cruz-Muniz et al., 2017, 2018) and cisplatin (Chowdhury et al., 2016) also can kill persister cells, these agents have not been approved for clinical treatment (Kim and Wood, 2016). Therefore, persisters remain a problem in the medical and food production fields because they can re-grow in response to external environmental changes and cause serious infectious diseases. To achieve effective sterilization and remove persisters, new sterilization techniques are required.
In our previous study, we developed a radical vapor reactor (RVR) that continuously produces reactive oxygen species (ROS) at high concentrations from water and air (O2) which are then exposed to an object. This process is performed at ambient temperature and pressure and exhausts only water and oxygen, as the produced ROS are detoxified by a catalyst after the reaction (Matsuo et al., 2015). Therefore, the RVR has minimal environmental effects. The ROS of the RVR can be used for various applications such as to functionalize the surface of materials (Yamasaki et al., 2017) and for surface cleaning (Yamasaki et al., 2018). ROS are suitable for sterilization because of their strong oxidation activities. The sterilization effects of ROS by RVR have been reported previously (Takatsuji et al., 2017) in a study demonstrating that ROS have stronger sterilization effects than ozone toward exponential cells of E. coli (Gram-negative) and Bacillus subtilis (Gram-positive). In this present study, we effectively sterilized persister cells using the RVR. Similar to our previous report (Takatsuji et al., 2017), many studies have described sterilization using ROS (Okpara-Hofmann et al., 2005; Murray et al., 2008). However, no studies have evaluated the sterilization of persister cells using ROS externally. To determine whether ROS are effective against persister cells, we prepared rifampicin-induced E. coli persister cells as previously described (Kwan et al., 2013; Kim et al., 2018a,b; Yamasaki et al., 2020). The RVR can be used in various modes such as UV mode and O2 plasma mode. We aimed to investigate the various RVR modes to evaluate which types of ROS are effective for killing persister cells and the mechanisms underlying these effects.
Materials and Methods
Cultivation of E. coli and Persister Cell Formation
The Escherichia coli K-12 BW25113 strain (Baba et al., 2006) was grown in lysogenic broth (LB; Difco Laboratories, Detroit, MI, USA) (Bertani, 1951) containing 1% (w/v) yeast extract at 37°C. For experiments using exponential cells, the E. coli were cultured overnight in a 5-mL LB medium and then inoculated into a 25-mL fresh LB medium at a 1/100 dilution and incubated at 37°C to a turbidity of 0.8 at 600 nm (~2 h). The cells were collected by centrifugation at 3,500 × g for 2 min and washed twice with 1× phosphate-buffered saline buffer (PBS) (Dulbecco and Vogt, 1954). The cells were resuspended in 1 mL of 1× PBS. For experiments using persister cells, the cells were grown to a turbidity of 0.8 at 600 nm as described for exponential cells. Rifampicin (FUJIFILM Wako Pure Chemical Corporation, Osaka, Japan) was added at a final concentration of 100 μg/mL, and the culture was incubated at 37°C for 30 min. The antibiotic concentration was selected to be at least 10× the minimum inhibitory concentration, following a previous report (Kwan et al., 2013). The rifampicin-treated culture (5 mL) was harvested by centrifugation at 3,500 × g for 10 min and resuspended in 5 mL of LB with ampicillin [final conc. 100 μg/mL, Tokyo Chemical Industry Co., Ltd (Tokyo, Japan)] followed by incubation at 37°C for 3 h to remove non-persister cells. Next, 1 mL of the rifampicin/ampicillin-treated culture was harvested by centrifugation at 3,500 × g for 5 min. The cell pellets were washed with 1× PBS at 3,500 × g for 2 min and re-suspended in 1 mL of 1× PBS twice. This procedure for developing persister cells was performed as described previously (Kwan et al., 2013; Kim et al., 2018a), and many groups have used this approach (Song and Wood, 2020).
ROS Treatment by RVR
Under all plasma treatment conditions, the temperature in the RVR chamber was set to 40°C. This temperature is suitable for producing singlet oxygen and hydroxy radicals (Matsuo et al., 2015). The following RVR conditions are illustrated in Figures 1B–J. In O2 mode, O2 was introduced at 4 L/min into the chamber (Figure 1B). In UV mode, the sample on the sample stage was irradiated with UV light (185 and 254 nm) (Figure 1C). In UV (vapor) mode, the sample was irradiated with UV light under humid conditions (vapor) using a stainless-steel dish filled with water as a vaporizer (Figure 1D). In UV cover mode, a shielding plate was used to avoid direct irradiation of the sample (Figure 1E). In UV cover (vapor) mode, UV irradiation was shielded during humidification (Figure 1F). In O2 plasma mode, pure oxygen introduced at a constant flow rate into the RVR was plasmatized by an electric discharger (Figure 1G). In O2 plasma/UV mode, the sample was exposed to both plasmatized O2 and UV simultaneously, but UV was not directly irradiated because of the shielding plate (Figure 1H). In O2 plasma/UV (vapor) mode, under humidified conditions, the sample was exposed to both plasmatized O2 and UV simultaneously, but UV was not directly irradiated because of the shielding plate (Figure 1I). In O2 plasma/UV without (w/o) cover mode, the sample was directly irradiated with UV and plasmatized O2 (Figure 1J). Under conditions using UV light, the stainless-steel walls in the RVR chamber were covered with black board to avoid UV light reflection. To avoid nutrient activation of persister cells, M9 agar (no carbon source) (Rodriguez and Tait, 1983) was used for ROS treatment. The cells (8 μL) were plated onto M9 agar, placed on the RVR sample stage, and treated in each RVR mode. After treatment, 30 μL of 10× LB was added to the cell agar plates. These plates were incubated at 37°C overnight and colonies were counted to calculate the survival rate. In the M9 plate with 10× LB, the cells grew, and colonies could be counted as on the LB plate (Supplemental Table 1 and Supplemental Figure 1).
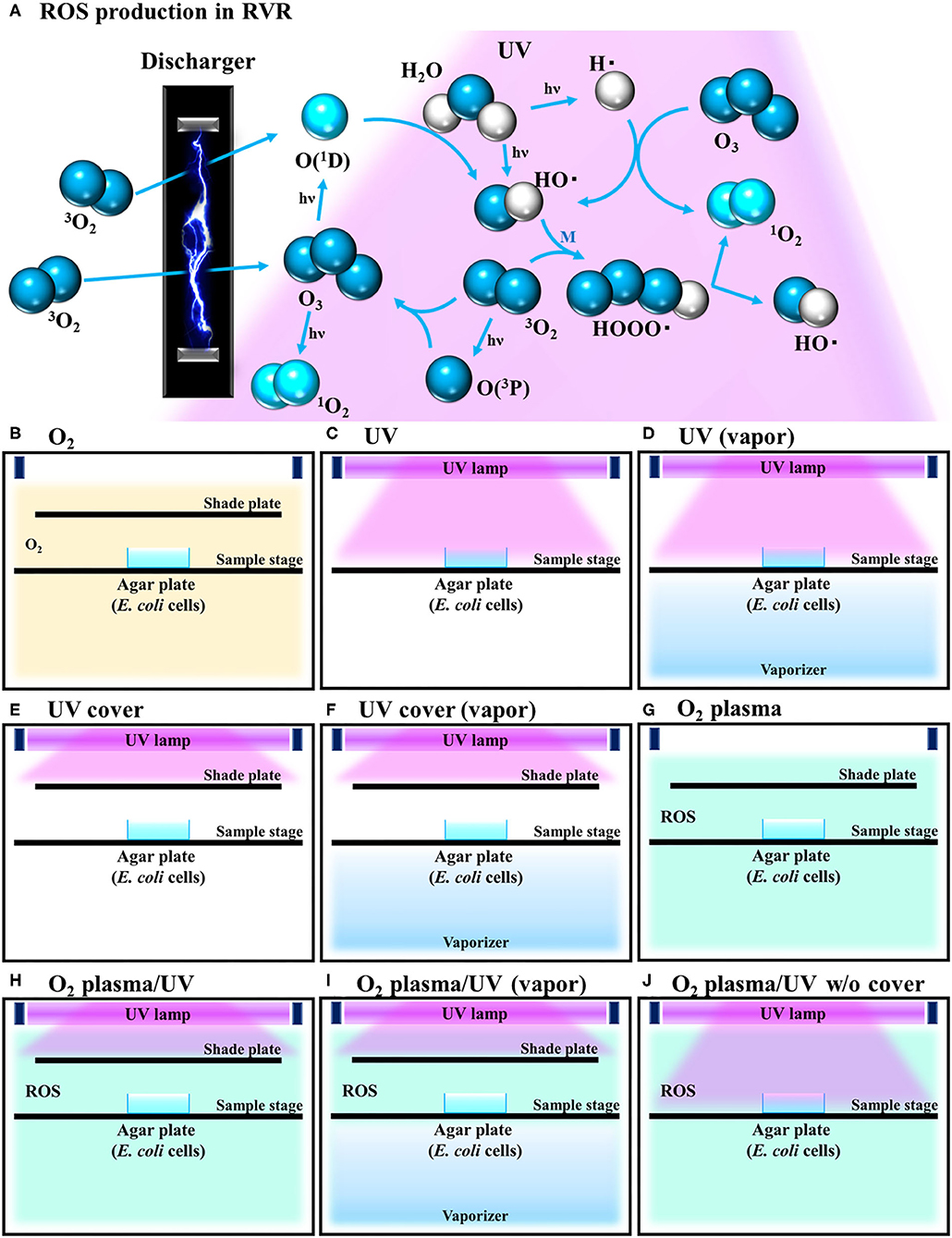
Figure 1. Schematic illustration of reactive oxygen species (ROS) production reaction under various radical vapor reactor (RVR) conditions. (A) ROS production reaction pathway. Oxygen is ozonized by discharging. Ozone is degraded to atomic oxygen and singlet oxygen by UV irradiation. Singlet oxygen reacts with H2O to produce hydroxyl radicals. HOOO• (hydrogen-trioxide radical) is produced by a reaction between hydroxyl radicals and oxygen, and then cleaved to 1O2 and HO•. M in the third part of this reaction. (B) O2 mode as a control. Inserted non-activated O2 gas. (C) UV mode. Sample on the sample stage was directly exposed only UV. (D) UV mode in vapor mode. Internal reactor created high humidity conditions by the vaporizer. (E) UV cover mode. UV was irradiated but sample plate was covered with a shade plate to avoid direct irradiation. (F) UV cover mode in vapor mode. (G) O2 plasma mode. Plasmatized 3O2 molecules by an electric-discharger were introduced into the RVR chamber. Plasmatized (activated) O2 plasma produces ROS as shown in (A). (H) O2 plasma/UV mode (O2 plasma mode with UV). (I) O2 plasma/UV in vapor mode. (J) O2 plasma/UV no cover mode.
Electron Spin Resonance Analysis
To detect singlet oxygen and hydroxyl radicals, 2,2,5,5-tetramethly-3-pyrroline-3-carboxamide [TPC, Sigma-Aldrich (St. Louis, MO, USA)] and 5,5-dimethyl-1-pyrroline N-oxide [DMPO, LABOTEC (Tokyo, Japan)] were used as spin trap reagents. Each reagent was prepared at 0.5 M in ultrapure water. Next, 1 mL of the prepared reagent solution in the 30-mm culture dish was placed on the center of the sample stage in the RVR. The solution was treated in each RVR mode for 40 or 120 s and then analyzed by electron spin resonance (ESR) spectrometry (JES-FA-100, JEOL, Tokyo, Japan) to detect ROS. The ESR conditions for detecting ROS were as follows: field sweep, 329.1–344.1 mT; field modulation frequency, 100 kHz; field modulation width, 0.05 mT; amplitude, 100; sweep time, 1 min; microwave frequency, 9,450 MHz; microwave power, 4 mW. For calculating the spin-adduct concentration, 0.5 mM to 0.03125 mM 4-hydroxy-2,2,6,6-tetramethyl piperidine-1-oxyl [TEMPOL, Sigma-Aldrich (St. Louis, MO, USA)] was used as a standard sample. The ESR spectrum of Mn2+ was used as an internal standard, and the spin concentration was determined using a digital data processor (JEOL, Tokyo, Japan). DMPO-OH and TPC-1O2 were simulated using the isotropic simulation program. Atmosphere conditions (no RVR treatment) were evaluated as a negative control.
Scanning Electron Microscopy Observation
The E. coli exponential and persister cells were prepared as described above. The cells were suspended in 1 mL 1× PBS and centrifuged twice at 3,500 × g for 2 min. The supernatant was discarded, and the pellet was re-suspended in 100 μL 1× PBS. The cell suspensions were plated onto a mixed cellulose esters membrane (Merck Millipore, Billerica, MA, USA) on an M9 agar plate. The cells were treated in each RVR mode for 10 min for complete sterilization. The cells on the membrane were prefixed with 2% glutaraldehyde fixative solution for 1 h. The fixed cell samples were washed twice with 0.1 M phosphate buffer (pH 7.4), and then treated with 50, 70, 90, 95, and 100% acetone for 15 min to dehydrate the samples. For lyophilization, acetone was substituted with t-butanol and incubated twice for 30 min at 30°C, and then frozen at −30°C. After lyophilization, platinum deposition was carried out for scanning electron microscopy (SEM) observation (S-4300, HITACHI, Tokyo, Japan).
Biofilm Removal by RVR
Escherichia coli cells were incubated overnight and diluted to a turbidity of 0.05 at 600 nm in LB. The diluted culture was distributed into a 96-well plate (300 μL/well) and incubated for 24 h at 37°C. Planktonic cells were discarded and washed with dH2O gently three times. This washing procedure was performed based on a previous biofilm assay report (Zhu et al., 2019). The plate was incubated at 37°C for 20 min to dry. Formed biofilms of E. coli were treated in each RVR mode for 10 min. Fresh LB medium was added to each well (300 μL/well) and the plate was incubated for 16 h at 37°C. Wells showing re-growth were counted. Data were analyzed using Excel (Microsoft, Redmond, WA, USA) and expressed as the means ± standard deviations. Differences between multiple groups were assessed by one-way analysis of variance (ANOVA) followed by Tukey's post-hoc test. A value of P < 0.05 was considered statistically significant.
Results
Attenuation of Bactericidal Effect by UV Shading
Our developed RVR can produce ROS at high concentrations in a continuous manner as shown in the reaction pathway in Figure 1A. This ROS production reaction pathway was developed based on previous studies (Tasaki et al., 2009; Le Picard et al., 2010; Matsumura et al., 2013; Matsuo et al., 2015; Yamasaki et al., 2017). An RVR can use several modes to combine UV, a discharger, and vaporizer (Figures 1B–J). First, the E. coli bactericidal effects in UV mode (Figure 1C), UV mode with vapor (Figure 1D), UV cover mode (Figure 1E), or UV cover mode with vapor (Figure 1F) were investigated. Direct irradiation with UV immediately killed the bacterial cells in a few seconds (Figure 2A). However, when direct UV irradiation was blocked by the cover, the bactericidal effect was greatly decreased (Figure 2A); however, E. coli died over an area of a few inches despite the shielding of UV light. We predicted that ROS were produced by UV and moved toward the sample. In each UV or UV cover mode, no differences in sterilizing effects were observed in the presence and absence of vapor (high or low humidity) (Figure 2A). Next, E. coli persister cells or exponential cells induced by rifampicin (Kwan et al., 2013) were treated in UV cover mode. As shown for the exponential cells in Figure 2A, direct irradiation with UV immediately killed all persister cells (Supplemental Figure 2). Notably, UV cover mode showed an ~10-fold higher bactericidal effect on persister cells than on exponential cells for 120 s treatment (Figure 2B). After 40 s of treatment, a 100-fold higher bactericidal effect on persister cells than on exponential cells was observed. Therefore, ROS by UV irradiation efficiently killed persister cells rather than exponential cells. This result was statistically significant (**p < 0.01). The values obtained in these experiments are shown in Supplemental Table 2. To identify the ROS produced by UV, two primary types of ROS (singlet oxygen and hydroxyl radical) that can be detected by ESR were analyzed. Each spin trap reagent was placed on the sample stage in RVR for 40 s as the negative control (NC). To detect singlet oxygen, TPC is a suitable reagent (Nakamura et al., 2011). Singlet oxygen by TPC was weakly detected and UV cover mode detected specific signal of TPC-1O2 more than the NC (Figure 3A). Hydroxyl radical by DMPO was detected in less quantity and UV cover mode showed a specific signal of DMPO-OH (1:2:2:1 quartet pattern intensity) (Nakamura et al., 2010) (Figure 3B). Both TPC-1O2 and DMPO-OH were simulated and are shown in Figure 3 (lower spectra). For each simulation, the following hyperfine coupling constants were used: aN = aH = 1.61 mT and g = 2.0061 (Matsumura et al., 2013) for TPC-1O2, and aN = 15 mT, aH = 14.7 mT, and g = 2.0057 for DMPO-OH (Bosnjakovic and Schlick, 2006). Each ROS concentration was obtained by analyzing ESR spectra and is shown in Table 1. As shown in Table 1, 4.4-fold higher levels of hydroxyl radicals were detected in UV cover mode than in the NC. Singlet oxygen levels were also 2.2-fold higher than in the NC (Table 1).
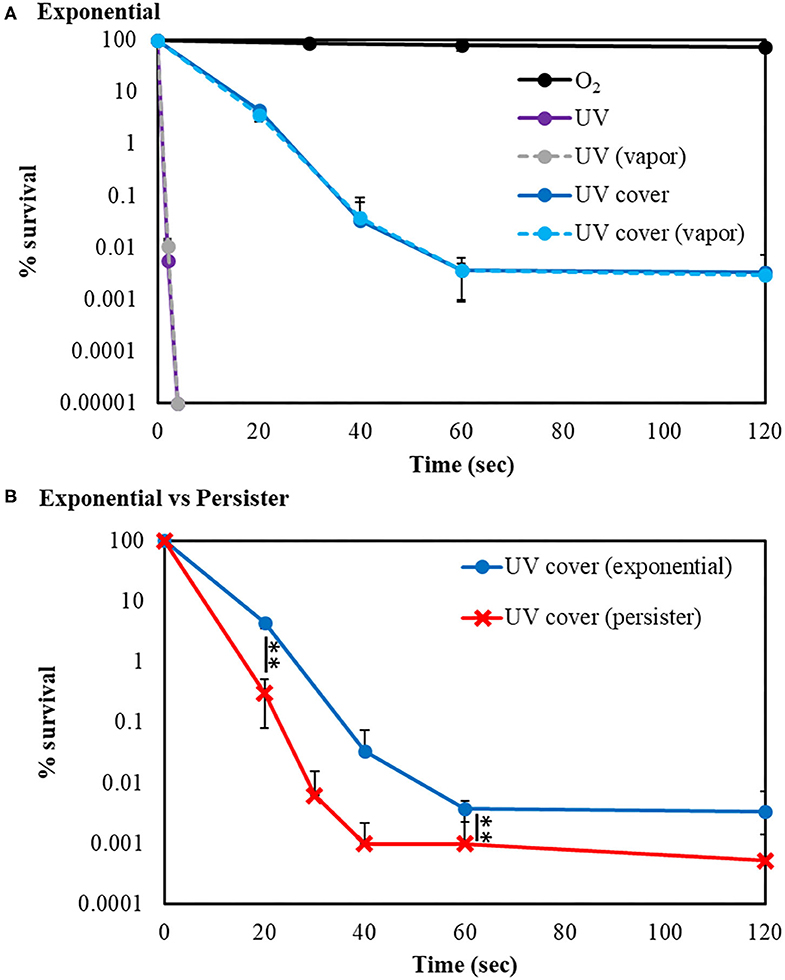
Figure 2. UV sterilization of E. coli exponential cells and persister cells with or without cover. (A) Survival rate of E. coli BW25113 exponential cells after sterilization in UV (purple) or UV cover (blue) modes. Vapor conditions using a vaporizer are indicated as broken lines (gray is UV, and light blue is UV cover). Black plots are O2 treatment as a control. (B) Comparison of E. coli BW25113 exponential cells (blue) and persister cells (red) in UV cover mode treatment. Each exponential or persister cell sample was plated on M9 agar and treated in each RVR mode. Error bars indicate S.D. of at least three experiments. Student's t-test was used to compare two groups (**P < 0.01). The values obtained in these experiments are shown in Supplemental Table 2.
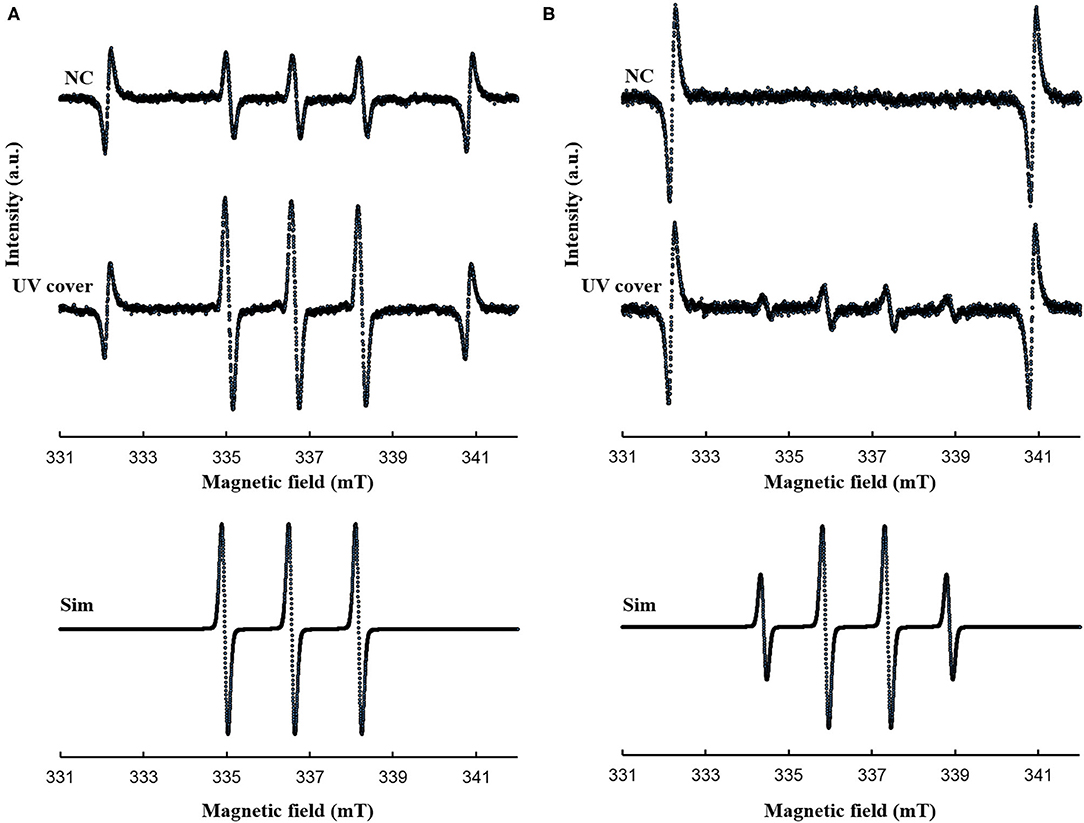
Figure 3. ESR spectra for (A) TPC-1O2 and (B) DMPO-OH. A 0.5 mM DMPO or 0.5 mM TPC solution was processed in each RVR condition (NC; negative control and UV cover mode) for 40 sec. Details of the ESR conditions are described in the methods section. Upper spectrum is NC and middle spectrum is UV cover condition. The lower spectra represent the simulated spectra of each adduct. Analyzed values are shown in Table 1. Y-axis indicates intensity (arbitrary unit).
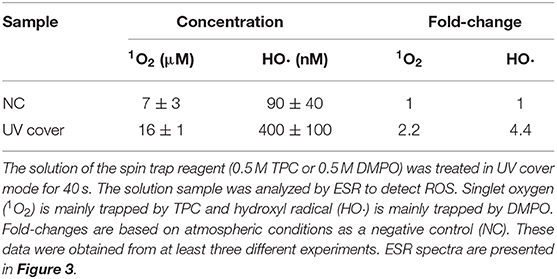
Table 1. Reactive oxygen species (ROS) analysis using electron spin resonance (ESR) after treatment in UV cover mode.
Effect of ROS on Bacterial Cell Wall
To determine how bactericidal effects by UV cover treatment affect the cell wall, the E. coli cell surface was observed by SEM after treatment. Both E. coli exponential cells and persister cells showed nearly the same regular cell wall surface (Figures 4A,B). The E. coli persister cells after treatment in UV cover mode are shown in Figure 4C. To determine the efficiency of the effects of ROS, persister cells were treated in UV cover mode for 10 min [this time is excessive, as 40 s of treatment has a 99.99% sterilization effect (Figure 2B)]. Although lethal treatment was applied, no noticeable injury was found (Figure 4C). This result clearly indicates that ROS kill bacteria without injuring the bacterial cell wall.
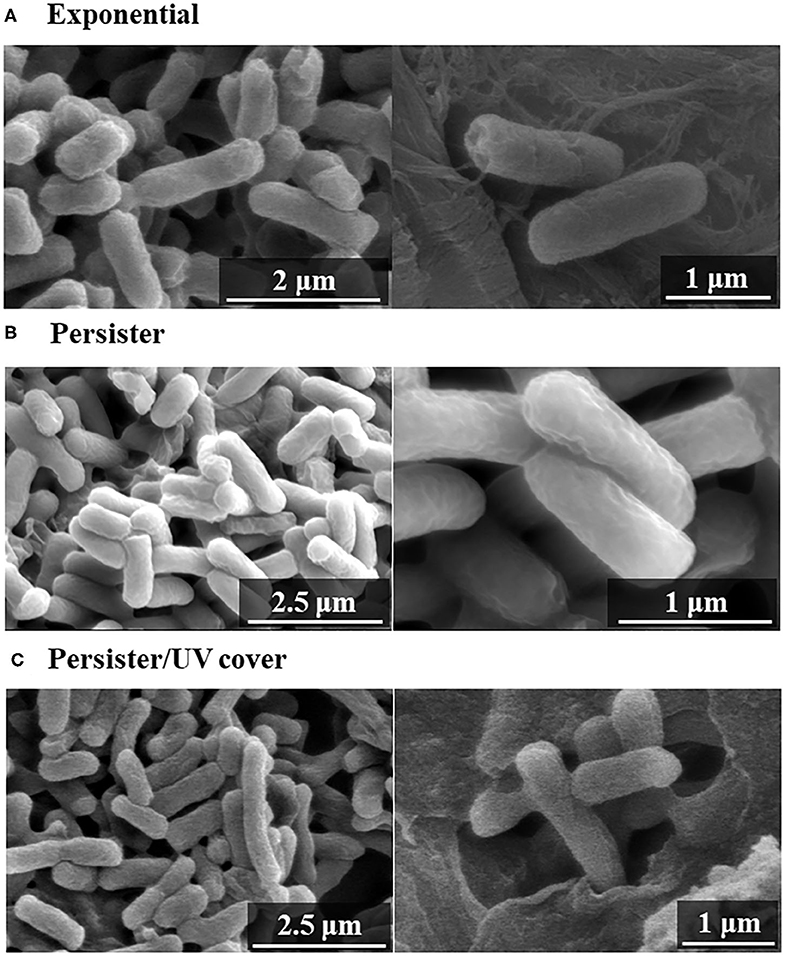
Figure 4. Scanning electron microscopy (SEM) images after UV irradiation. Bacterial cell wall observation of (A) exponential cells, (B) persister cells, and (C) persister cells treated with UV with cover for 10 min (UV cover) by SEM.
Sterilization Effect of Other RVR Modes
The bactericidal effects of other RVR modes on E. coli exponential and persister cells were investigated. The RVR can produce O2 plasma by discharging O2 gas. O2 plasma mode alone, O2 plasma/UV mode (combining O2 plasma and UV irradiation), and O2 plasma/UV with vapor in vaporizer mode were evaluated (Figures 1B,G–I). For exponential cells, O2 plasma/UV with vapor showed good bactericidal effects (Figure 5A). All cells were sterilized at O2 plasma/UV with vapor for 120 s. In contrast, O2 plasma/UV without vapor was more effective than O2 plasma/UV with vapor against persister cells (Figure 5B). The values obtained in these experiments are shown in Supplemental Table 3. Both singlet oxygen and hydroxyl radicals produced in each mode were quantified by ESR. In O2 plasma mode, the spectral intensity of ESR was almost the same as that for NC (Figure 6A). In contrast, O2 plasma/UV and O2 plasma/UV with vapor modes showed higher signal than O2 plasma mode (Figure 6A). Although the NC did not detect the hydroxyl radical, the O2 plasma mode detected and showed a specific signal (Figure 6B). The O2 plasma/UV mode showed a highly specific signal. However, the O2 plasma/UV with vapor mode decreased the DMPO-OH signal (Figure 6B). Each ROS concentration was obtained by analyzing ESR spectra and is shown in Table 2. In a comparison of O2 plasma/UV and O2 plasma/UV with vapor, the levels of singlet oxygen in both modes were very high (31–and 37-fold) but not significantly different (Table 2). However, O2 plasma/UV produced 3-fold higher hydroxy levels compared to O2 plasma/UV with vapor (Table 2). In contrast, in O2 plasma mode, hydroxyl radicals were detected; however, singlet oxygen was not detected (Table 2).
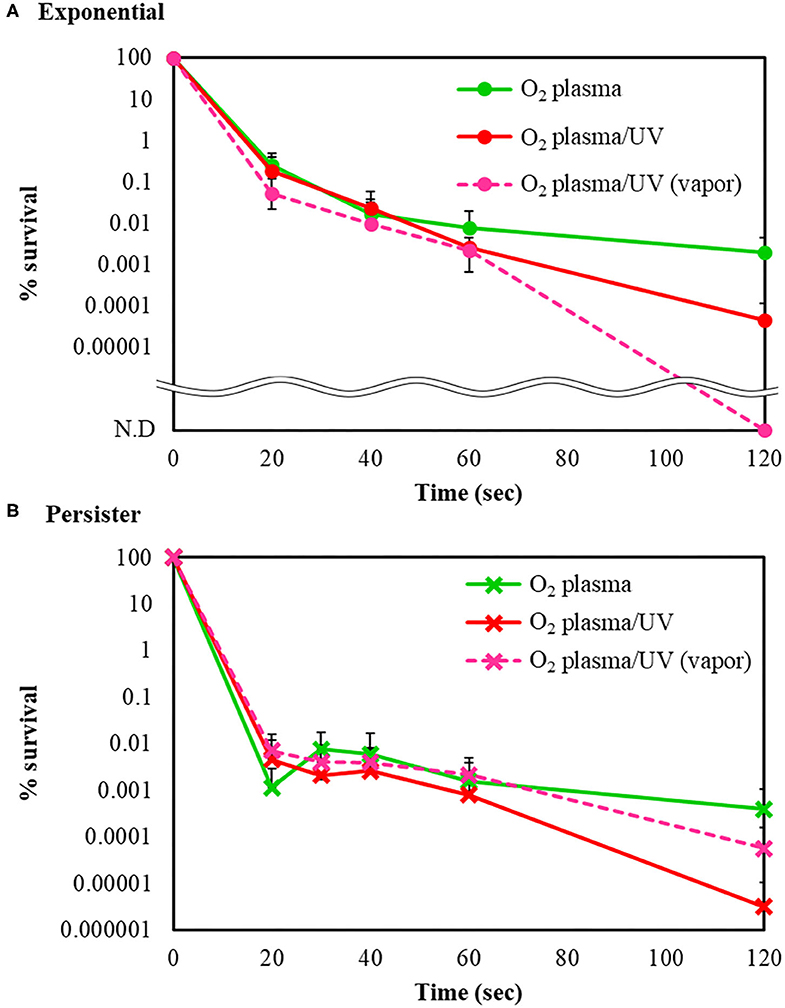
Figure 5. Bactericidal effects of other radical vapor reactor (RVR) conditions. Survival rate of (A) E. coli exponential cells and (B) E. coli persister cells after sterilization with O2 plasma (green), O2 plasma/UV (red), and O2 plasma/UV with vapor (pink, broken line) modes. Each exponential or persister cell sample was plated on M9 agar and treated in each RVR mode. Non-detectable bacterial numbers are indicated as N.D. Error bars indicate S.D. of at least three experiments. The values obtained in these experiments are shown in Supplemental Table 3.
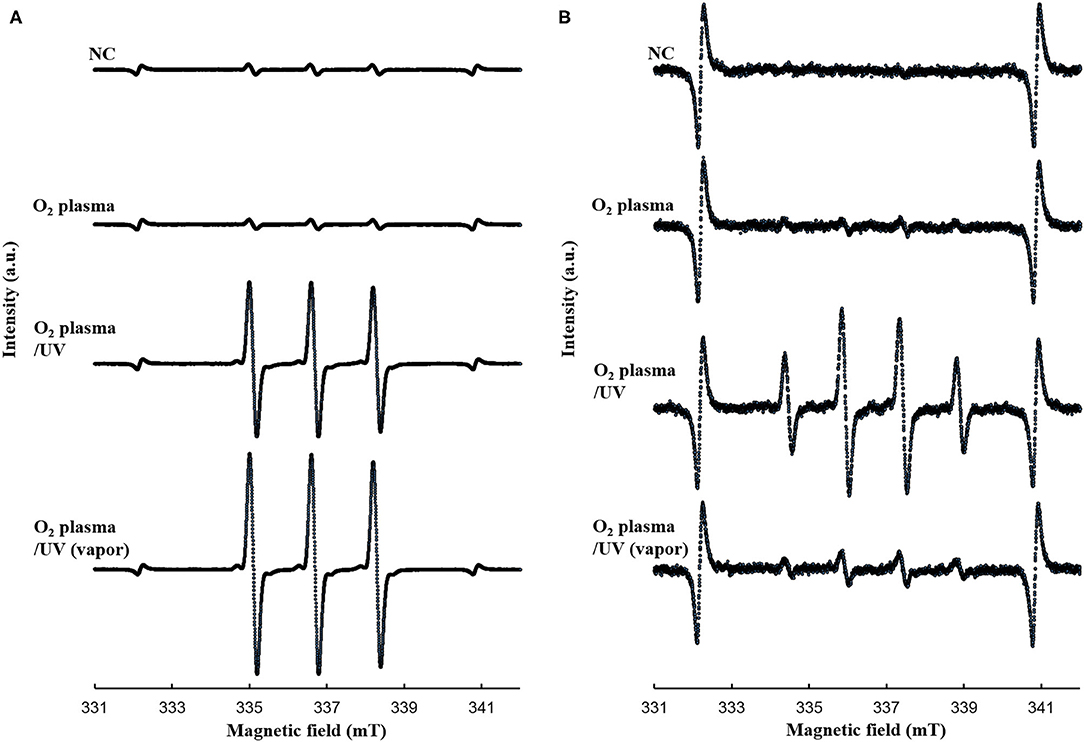
Figure 6. ESR spectra for (A) TPC-1O2 and (B) DMPO-OH. A 0.5 mM DMPO or 0.5 mM TPC solution was processed in each RVR condition [NC, O2 plasma, O2 plasma/UV, and O2 plasma/UV (vapor)] for 120 sec. Details of the ESR conditions are provided in the methods section. NC, O2 plasma, O2 plasma/UV, and O2 plasma/UV (vapor) condition are indicated. Analyzed values are shown in Table 2. Y-axis indicates intensity (arbitrary unit).
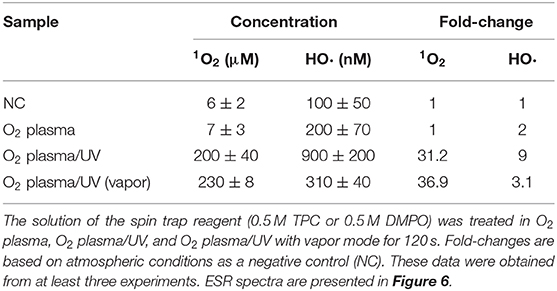
Table 2. Reactive oxygen species (ROS) analysis using electron spin resonance (ESR) in various radical vapor reactive modes.
Escherichia coli Biofilm Removal by RVR
Escherichia coli forms a biofilm during growth. Biofilm cells are generally considered as more resistant to treatment than planktonic cells (Bridier et al., 2011). Therefore, the effects of various RVR modes on E. coli-derived biofilms were examined and tested to ascertain whether the biofilms could be completely sterilized. E. coli biofilms formed on the 96-well plate were each treated with UV cover, UV, O2 plasma, O2 plasma/UV, and O2 plasma/UV without cover. Non-treatment (NT) as a control (wash alone) grew in all 96 wells (Figure 7). Hence, there was no effect of washing against biofilm bacteria. Interestingly, UV irradiation for 10 min of treatment could not completely remove the biofilm (2% survived), whereas O2 plasma/UV without a cover killed all biofilm cells (Figure 7). UV cover and O2 plasma/UV modes showed nearly the same results (19 and 16% survived, respectively). The O2 plasma mode did not effectively remove the biofilm (53% survived). The values obtained in these experiments are shown in Supplemental Table 4.
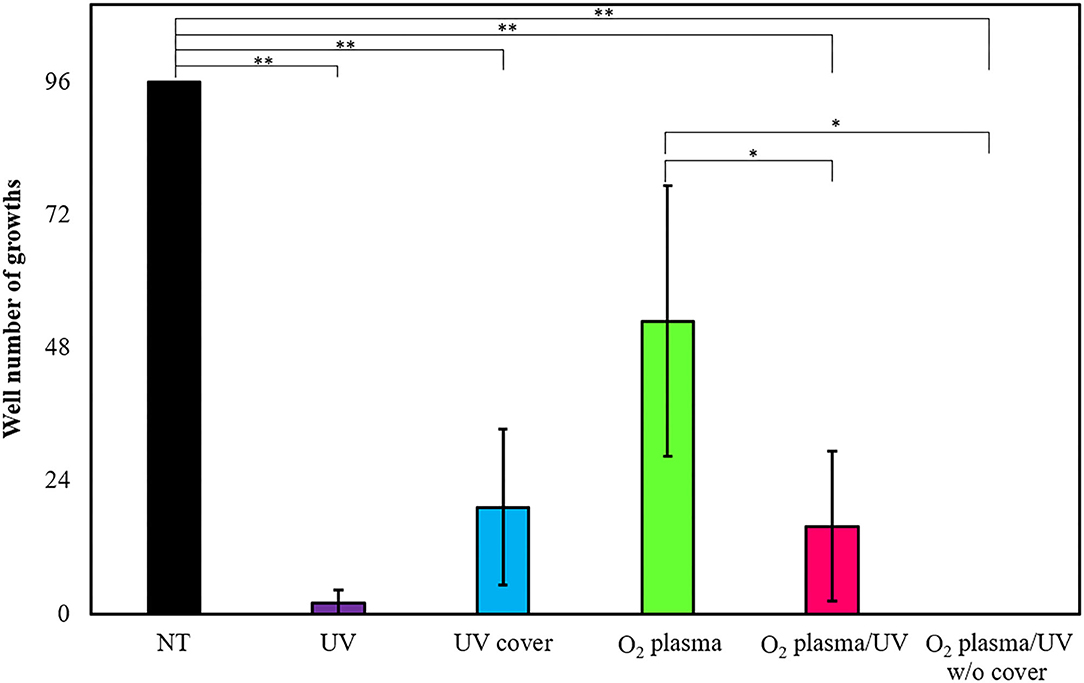
Figure 7. Biofilm extinction by radical vapor reactor (RVR) treatment. Number of wells growing cells after treatment in UV (purple), UV cover (blue), O2 plasma (green), O2 plasma/UV (pink), and O2 plasma/UV without cover mode. Formed E. coli biofilms on the 96-well plate were treated in each RVR mode for 10 min, and then LB medium was added to all wells and incubated at 37°C for 16 h. Wells which re-grew cells were counted. Non-treatment (NT) as a control is indicated at a black bar. Error bars indicate S.D. of at least three experiments. All data were analyzed using Tukey's post-hoc test after one-way analysis of variance. Significant difference of two individual groups comparison connects with a line and indicates at asterisks (*P < 0.05, **P < 0.01). The values obtained in these experiments are shown in Supplemental Table 4.
Discussion
Persister cells are highly tolerant to stress compared to cells in the exponential state. Therefore, sterilization of persister cells is difficult. In this study, we found that ROS (particularly hydroxyl radicals) effectively sterilize E. coli persister cells compared to exponential cells. Thus, this sterilization method is suitable depending on the bacterial phenotype. Thus, RVR has fewer disadvantages than EOG and radiation sterilization and shows highly effective sterilization. These results may be useful for developing effective sterilization methods for use in the public health and clinical medicine fields.
Previously, Vermeulen et al. (2008) reported the growth inhibitory effect of UV light in E. coli. When irradiated with various wavelengths of radiation, a wavelength of 265 nm was shown to be the most effective for killing of E. coli. Our study demonstrated that UV was effective for killing both E. coli exponential cells and persister cells in <5 s (Figure 2A and Supplemental Figure 2). As shown in Figure 2A, the germicidal effect was reduced when UV irradiation was blocked with a shielding plate. Therefore, this result suggests that bacteria cell survival to avoid UV light is a limitation of UV treatment. However, despite the complete inhibition of UV irradiation toward the cell sample, the levels of surviving E. coli were gradually decreased. ROS are produced by UV irradiation to oxygen and water in the atmosphere (de Jager et al., 2017; Georgiou et al., 2017). In the ESR results, hydroxyl radicals were detected at high concentrations than in the NC. Because ROS are produced by UV, bacteria are not enough but killed. E. coli persister cells were killed at ~100-fold higher levels than exponential cells by 40-s treatment in UV cover mode. Singlet oxygen and hydroxyl radicals were present at higher levels than in the NC in UV cover mode. Therefore, ROS, particularly hydroxyl radicals, have greater effects on persister cells than on exponential cells. To determine if ROS injures the bacterial cell membrane and kills the cells directly or penetrates the intracellular environment to kill the cells, E. coli exponential, persister, and persister cells after treatment in UV cover mode were observed by SEM (Figure 4). No large differences in cell shape were observed. ROS did not react with the bacterial cell wall but rather reacted intracellularly to exert cell-killing effects. Oxidizing bactericidal agents are widely used to kill pathogenic bacteria, and many studies of the characteristics and mechanisms of their action have been reported (Linley et al., 2012; Vatansever et al., 2013). In general, intracellularly produced ROS exert bactericidal action by causing DNA damage, protein denaturation, and lipid peroxide production, which negatively impact cell survival (Cabiscol et al., 2000; Dwyer et al., 2007; Hong et al., 2017; Van Acker and Coenye, 2017). Therefore, ROS produced during RVR killed the bacteria.
ROS effectively killed persister cells. The RVR can produce high levels of ROS to plasmatize O2 gas. This O2 plasma combined UV cover mode also showed sterilization effects. High humidity conditions (with vapor) were also evaluated, as ROS production may be altered by H2O. Interestingly, the opposite results were obtained for exponential cells and persister cells (Figure 5). For exponential cells, O2 plasma/UV with vapor showed greater sterilization effects than without vapor (Figure 5A). In contrast, O2 plasma/UV with vapor showed lower sterilization effects than without vapor for persister cells (Figure 5B). Comparison of the concentration of ROS under each condition revealed that singlet oxygen was produced at the same high level in both O2 plasma/UV without vapor mode and O2 plasma/UV with vapor mode, and the hydroxyl radical level was 3-fold higher in O2 plasma/UV without vapor mode than in O2 plasma/UV with vapor mode (Table 2). Therefore, high concentrations of hydroxyl radical showed strong sterilization effects toward persister cells, and high concentrations of singlet oxygen and low concentrations of hydroxyl radical showed strong sterilization effects toward exponential cells. A previous study also indicated that the vapor condition had higher sterilization effects on cells in the exponential state than the condition without vapor (Takatsuji et al., 2017). It has been reported that viable E. coli persister cells can stop the production of hydroxyl radicals (Kim et al., 2011). Thus, hydroxyl radicals strongly affect the survival of persister cells. In the absence of UV (O2 plasma mode), sterilization effects were weak toward both cell types. Because O2 plasma exposure alone did not result in high production of ROS, this method is less effective than O2 plasma/UV mode. In addition, O2 plasma/UV mode was continuously performed to completely sterilize the persister cells. All persister cells were killed after 360 s of treatment (Supplemental Figure 3).
We demonstrated the RVR sterilization effect dispersed not only E. coli but also the biofilm formed by E. coli (Figure 7). Direct UV irradiation immediately killed (<5 s) the cells on an agar plate. However, UV could not completely kill biofilm cells even after irradiation for 10 min. UV sterilization effects weaken as the distance between the UV lamp and sample increases (Bank et al., 1990). Additionally, areas not reached by UV are difficult to irradiate, and small samples are difficult to sterilize. Thus, cells deeply positioned in the biofilm on the 96-well plate may have not been sterilized and then re-grew. The poor sterilization effect of O2 plasma mode (green) is due to the low production of both singlet oxygen and hydroxyl radicals (Table 2). The UV cover mode (blue) and O2 plasma/UV (pink) mode showed better sterilization effects than O2 plasma, as the ROS concentration was higher; however, the sterilization effects were low. There is no statistical significance between UV cover mode (blue) and O2 plasma/UV mode (pink), but O2 plasma/UV showed higher effect than UV cover mode. This result also related in ROS amount. Importantly, O2 plasma/UV mode without a cover showed complete sterilization effects. This mode directly combined UV irradiation and O2 plasma exposure. The ROS produced by O2 plasma also reached deep parts of the sample, enabling complete sterilization. These results suggested that the biofilm, that is, both exponential cells and the persister, can be sterilized in a short time by using RVR.
In summary, this study revealed that hydroxyl radicals have strong bactericidal effects against persister cells, which cause intractable infection diseases. Combining UV and O2 plasma resulted in strong sterilization effects against biofilm. ROS, particularly hydroxyl radicals, effectively remove persister cells and biofilm remaining even after chemical treatment. The time required for sterilization by the RVR is much shorter than that for autoclaving sterilization. Furthermore, no hazardous waste is produced, and RVR sterilization is not costly, as it only requires oxygen gas. Thus, ROS produced by RVR may be more effective for sterilizing persisters compared to conventional sterilization methods and may be useful for performing sterilization operations in the medical field. We will use the RVR for sterilization of dental instruments. Some dental instruments cannot be autoclaved and, thus, require sterilization. For these instruments, EOG or UV sterilization methods have been used. However, the EOG method shows residual gas effects after processing and the UV method cannot completely kill bacteria. In contrast, RVR can use a wide variety of instruments and achieve complete sterilization in a short time (a few seconds). Thus, RVR may become an alternative general sterilization method.
Data Availability Statement
All datasets generated for this study are included in the article/Supplementary Material.
Author Contributions
AK, RY, TS, and YT performed the experiments. AK wrote the initial draft of the manuscript. RY assisted in the preparation of the manuscript. AK, RY, TS, YT, TH, YY, and WA designed the experiments. All authors have contributed to data collection, interpretation, critically reviewed the manuscript, read, and approved the manuscript.
Funding
This work was supported by funds derived from Grants-in-Aid for Scientific Research from the Japan Society for the Promotion of Science (No. 18K09556 and 18K09797).
Conflict of Interest
The authors declare that the research was conducted in the absence of any commercial or financial relationships that could be construed as a potential conflict of interest.
Acknowledgments
We thank Dr. Takashi Toyono for SEM technical support. We would like to thank Editage (www.editage.com) for English language editing.
Supplementary Material
The Supplementary Material for this article can be found online at: https://www.frontiersin.org/articles/10.3389/fcimb.2020.00496/full#supplementary-material
Supplemental Figure 1. Cell growth comparison on the LB plate and LB with M9 plate. Escherichia coli persister cells were diluted at 105 and plated (8 μL) onto the LB plate or M9 plate. Next, 30 μL of 10× LB was added to the M9 cell agar plates. These plates were incubated at 37°C for 1–2 days.
Supplemental Figure 2. UV effect to the persister cells. Comparison of the bactericidal effect by UV directly irradiation (UV mode) to E. coli exponential cells (blue) and persister cells (red).
Supplemental Figure 3. Complete sterilization of persister cells using O2 plasma/UV mode. Longer time treatment (360 s) in O2 plasma/UV mode of persister cells (Figure 5B).
Supplemental Table 1. Cell growth comparison on the LB plate and M9 with LB plate. Escherichia coli persister cells were diluted at 105 and plated (8 μL) onto the LB plate or M9 plate. Next, 30 μL of 10x LB was added to the M9 cell agar plates. These plates were incubated at 37°C for 1–2 days.
Supplemental Table 2. Number of survivingcells after RVR treatment. Colony forming units of E. coli after treatment in UV or UV cover mode were counted (CFU/mL). The graph of these data is shown in Figure 2.
Supplemental Table 3. Number of surviving cells after RVR treatment. Colony forming units of E. coli after treatment in O2 plasma or O2 plasma/UV mode were counted (CFU/mL). The graph of these data is shown in Figure 4.
Supplemental Table 4. Escherichia coli biofilm removal by RVR. The well number of growths after treatment in UV, UV cover, O2 plasma, O2 plasma/UV, and O2 plasma/UV without cover mode. Non-treatment (NT) is indicated as a control. The graph of these data is shown in Figure 5.
References
Abranches, J., Martinez, A. R., Kajfasz, J. K., Chavez, V., Garsin, D. A., and Lemos, J. A. (2009). The molecular alarmone (p)ppGpp mediates stress responses, vancomycin tolerance, and virulence in Enterococcus faecalis. J. Bacteriol. 191, 2248–2256. doi: 10.1128/JB.01726-08
Baba, T., Ara, T., Hasegawa, M., Takai, Y., Okumura, Y., Baba, M., et al. (2006). Construction of Escherichia coli K-12 in-frame, single-gene knockout mutants: the Keio collection. Mol. Syst. Biol. 2:2006.0008. doi: 10.1038/msb4100050
Balaban, N. Q., Merrin, J., Chait, R., Kowalik, L., and Leibler, S. (2004). Bacterial persistence as a phenotypic switch. Science 305, 1622–1625. doi: 10.1126/science.1099390
Bank, H. L., John, J., Schmehl, M. K., and Dratch, R. J. (1990). Bactericidal effectiveness of modulated UV light. Appl. Environ. Microbiol. 56, 3888–3889. doi: 10.1128/AEM.56.12.3888-3889.1990
Bertani, G. (1951). Studies on lysogenesis. I. The mode of phage liberation by lysogenic Escherichia coli. J. Bacteriol. 62, 293–300. doi: 10.1128/JB.62.3.293-300.1951
Bigger, J. W. (1944). Treatment of staphylococcal infections with penicillin by intermittent sterilisation. Lancet 244, 497–500. doi: 10.1016/S0140-6736(00)74210-3
Bosnjakovic, A., and Schlick, S. (2006). Spin trapping by 5,5-dimethylpyrroline-N-oxide in fenton media in the presence of nafion perfuorinated membranes: limitations and potential. J. Phys. Chem. B 110, 10720–10728. doi: 10.1021/jp061042y
Bridier, A., Briandet, R., Thomas, V., and Dubois-Brissonnet, F. (2011). Resistance of bacterial biofilms to disinfectants: a review. Biofouling 27, 1017–1032. doi: 10.1080/08927014.2011.626899
Cabiscol, E., Tamarit, J., and Ros, J. (2000). Oxidative stress in bacteria and protein damage by reactive oxygen species. Int. Microbiol. 3, 3–8. doi: 10.2436/im.v3i1.9235
Chowdhury, N., Wood, T. L., Martinez-Vazquez, M., Garcia-Contreras, R., and Wood, T. K. (2016). DNA-crosslinker cisplatin eradicates bacterial persister cells. Biotechnol. Bioeng. 113, 1984–1992. doi: 10.1002/bit.25963
Corrigan, R. M., Bellows, L. E., Wood, A., and Grundling, A. (2016). ppGpp negatively impacts ribosome assembly affecting growth and antimicrobial tolerance in Gram-positive bacteria. Proc. Natl. Acad. Sci. U. S. A. 113, E1710–1719. doi: 10.1073/pnas.1522179113
Cruz-Muniz, M. Y., Lopez-Jacome, L. E., Hernandez-Duran, M., Franco-Cendejas, R., Licona-Limon, P., Ramos-Balderas, J. L., et al. (2017). Repurposing the anticancer drug mitomycin C for the treatment of persistent Acinetobacter baumannii infections. Int. J. Antimicrob. Agents 49, 88–92. doi: 10.1016/j.ijantimicag.2016.08.022
Cruz-Muniz, M. Y., Lopez-Jacome, L. E., Hernandez-Duran, M., Franco-Cendejas, R., Licona-Limon, P., Ramos-Balderas, J. L., et al. (2018). Corrigendum to 'Repurposing the anticancer drug mitomycin C for the treatment of persistent Acinetobacter baumannii infections. Int. J. Antimicrob. Agents 52:868. doi: 10.1016/j.ijantimicag.2018.10.001
de Jager, T. L., Cockrell, A. E., and Du Plessis, S. S. (2017). Ultraviolet light induced generation of reactive oxygen species. Adv. Exp. Med. Biol. 996, 15–23. doi: 10.1007/978-3-319-56017-5_2
Dulbecco, R., and Vogt, M. (1954). Plaque formation and isolation of pure lines with poliomyelitis viruses. J. Exp. Med. 99, 167–182. doi: 10.1084/jem.99.2.167
Dwyer, D. J., Kohanski, M. A., Hayete, B., and Collins, J. J. (2007). Gyrase inhibitors induce an oxidative damage cellular death pathway in Escherichia coli. Mol. Syst. Biol. 3:91. doi: 10.1038/msb4100135
Fisher, R. A., Gollan, B., and Helaine, S. (2017). Persistent bacterial infections and persister cells. Nat. Rev. Microbiol. 15, 453–464. doi: 10.1038/nrmicro.2017.42
Gaca, A. O., Colomer-Winter, C., and Lemos, J. A. (2015). Many means to a common end: the intricacies of (p)ppGpp metabolism and its control of bacterial homeostasis. J. Bacteriol. 197, 1146–1156. doi: 10.1128/JB.02577-14
Georgiou, C. D., Zisimopoulos, D., Kalaitzopoulou, E., and Quinn, R. C. (2017). Radiation-driven formation of reactive oxygen species in oxychlorine-containing mars surface analogues. Astrobiology 17, 319–336. doi: 10.1089/ast.2016.1539
Goldman, M., and Pruitt, L. (1998). Comparison of the effects of gamma radiation and low temperature hydrogen peroxide gas plasma sterilization on the molecular structure, fatigue resistance, and wear behavior of UHMWPE. J. Biomed. Mater. Res. 40, 378–384. doi: 10.1002/(SICI)1097-4636(19980605)40:3<378::AID-JBM6>3.0.CO;2-C
Helaine, S., Cheverton, A. M., Watson, K. G., Faure, L. M., Matthews, S. A., and Holden, D. W. (2014). Internalization of Salmonella by macrophages induces formation of nonreplicating persisters. Science 343, 204–208. doi: 10.1126/science.1244705
Hobby, G. L., Meyer, K., and Chaffee, E. (1942). Observations on the mechanism of action of penicillin. Exp. Biol. Med. 50, 281–285. doi: 10.3181/00379727-50-13773
Hong, Y., Li, L., Luan, G., Drlica, K., and Zhao, X. (2017). Contribution of reactive oxygen species to thymineless death in Escherichia coli. Nat. Microbiol. 2, 1667–1675. doi: 10.1038/s41564-017-0037-y
Kim, J. S., Chowdhury, N., Yamasaki, R., and Wood, T. K. (2018a). Viable but non-culturable and persistence describe the same bacterial stress state. Environ. Microbiol. 20, 2038–2048. doi: 10.1111/1462-2920.14075
Kim, J. S., Heo, P., Yang, T. J., Lee, K. S., Jin, Y. S., Kim, S. K., et al. (2011). Bacterial persisters tolerate antibiotics by not producing hydroxyl radicals. Biochem. Biophys. Res. Commun. 413, 105–110. doi: 10.1016/j.bbrc.2011.08.063
Kim, J. S., and Wood, T. K. (2016). Persistent persister misperceptions. Front. Microbiol. 7:2134. doi: 10.3389/fmicb.2016.02134
Kim, J. S., Yamasaki, R., Song, S., Zhang, W., and Wood, T. K. (2018b). Single cell observations show persister cells wake based on ribosome content. Environ. Microbiol. 20, 2085–2098. doi: 10.1111/1462-2920.14093
Kim, W., Zhu, W., Hendricks, L. G., Tyne, V. D., Steele, D. A., Keohane, E. C., et al. (2018c). A new class of synthetic retinoid antibiotics effective against bacterial persisters. Nature 556, 103–107. doi: 10.1038/nature26157
Kwan, B. W., Chowdhury, N., and Wood, T. K. (2015). Combatting bacterial infections by killing persister cells with mitomycin C. Environ. Microbiol. 17, 4406–4414. doi: 10.1111/1462-2920.12873
Kwan, B. W., Valenta, J. A., Benedik, M. J., and Wood, T. K. (2013). Arrested protein synthesis increases persister-like cell formation. Antimicrob. Agents Chemother. 57, 1468–1473. doi: 10.1128/AAC.02135-12
Le Picard, S. D., Tizniti, M., Canosa, A., Sims, I. R., and Smith, I. W. (2010). The thermodynamics of the elusive HO3 radical. Science 328, 1258–1262. doi: 10.1126/science.1184459
Lewis, K. (2007). Persister cells, dormancy and infectious disease. Nat. Rev. Microbiol. 5, 48–56. doi: 10.1038/nrmicro1557
Lewis, K. (2008). Multidrug tolerance of biofilms and persister cells. Curr. Top. Microbiol. Immunol. 322, 107–131. doi: 10.1007/978-3-540-75418-3_6
Lewis, K. (2012). Persister cells: molecular mechanisms related to antibiotic tolerance. Handb. Exp. Pharmacol. 211, 121–133. doi: 10.1007/978-3-642-28951-4_8
Linley, E., Denyer, S. P., McDonnell, G., Simons, C., and Maillard, J. Y. (2012). Use of hydrogen peroxide as a biocide: new consideration of its mechanisms of biocidal action. J. Antimicrob. Chemother. 67, 1589–1596. doi: 10.1093/jac/dks129
Matsumura, Y., Iwasawa, A., Kobayashi, T., Kamachi, T., Ozawa, T., and Kohno, M. (2013). Detection of high-frequency ultrasound-induced singlet oxygen by the ESR spin-trapping method. Chem. Lett. 42, 1291–1293. doi: 10.1246/cl.130618
Matsuo, K., Takatsuji, Y., Kohno, M., Kamachi, T., Nakada, H., and Haruyama, T. (2015). Dispersed-phase Interfaces between mist water particles and oxygen plasma efficiently produce singlet oxygen 1O2 and hydroxyl radical (·OH). Electrochemistry 83, 721–724. doi: 10.5796/electrochemistry.83.721
Mori, M., Hamamoto, A., Takahashi, A., Nakano, M., Wakikawa, N., Tachibana, S., et al. (2007). Development of a new water sterilization device with a 365 nm UV-LED. Med. Biol. Eng. Comput. 45, 1237–1241. doi: 10.1007/s11517-007-0263-1
Murray, B. K., Ohmine, S., Tomer, D. P., Jensen, K. J., Johnson, F. B., Kirsi, J. J., et al. (2008). Virion disruption by ozone-mediated reactive oxygen species. J. Virol. Methods 153, 74–77. doi: 10.1016/j.jviromet.2008.06.004
Nakamura, K., Ishiyama, K., Ikai, H., Kanno, T., Sasaki, K., Niwano, Y., et al. (2011). Reevaluation of analytical methods for photogenerated singlet oxygen. J. Clin. Biochem. Nutr. 49, 87–95. doi: 10.3164/jcbn.10-125
Nakamura, K., Kanno, T., Ikai, H., Sata, E., Mokudai, T., Niwano, Y., et al. (2010). Reevaluation of quantitative ESR spin trapping analysis of hydroxyl radical by applying sonolysis of water as a model system. Bull. Chem. Soc. Jpn 83, 1037–1046. doi: 10.1246/bcsj.20100078
Okpara-Hofmann, J., Knoll, M., Durr, M., Schmitt, B., and Borneff-Lipp, M. (2005). Comparison of low-temperature hydrogen peroxide gas plasma sterilization for endoscopes using various sterrad models. J. Hosp. Infect. 59, 280–285. doi: 10.1016/j.jhin.2004.10.002
Rodriguez, R. L., and Tait, R. C. (1983). Recombinant DNA Techniques: an Introduction. Menlo Park, CA: Benjamin/Cummings Publishing.
Shintani, H. (2017). Ethylene oxide gas sterilization of medical devices. Biocontrol Sci. 22, 1–16. doi: 10.4265/bio.22.1
Song, S., and Wood, K. T. (2020). Are we really studying persister cells? Environ. Microbiol. Rep. (In press) doi: 10.1111/1758-2229.12849
Spoering, A. L., and Lewis, K. (2001). Biofilms and planktonic cells of Pseudomonas aeruginosa have similar resistance to killing by antimicrobials. J. Bacteriol. 183, 6746–6751. doi: 10.1128/JB.183.23.6746-6751.2001
Stapels, D. A. C., Hill, P. W. S., Westermann, A. J., Fisher, R. A., Thurston, T. L., Saliba, A. E., et al. (2018). Salmonella persisters undermine host immune defenses during antibiotic treatment. Science 362, 1156–1160. doi: 10.1126/science.aat7148
Takatsuji, Y., Ishikawa, S., and Haruyama, T. (2017). Efficient sterilization using reactive oxygen species generated by a radical vapor reactor. Process Biochem. 54, 140–143. doi: 10.1016/j.procbio.2017.01.002
Tasaki, T., Wada, T., Fujimoto, K., Kai, S., Ohe, K., Oshima, T., et al. (2009). Degradation of methyl orange using short-wavelength UV irradiation with oxygen microbubbles. J. Hazard. Mater. 162, 1103–1110. doi: 10.1016/j.jhazmat.2008.05.162
Van Acker, H., and Coenye, T. (2017). The role of reactive oxygen species in antibiotic-mediated killing of Bbacteria. Trends Microbiol. 25, 456–466. doi: 10.1016/j.tim.2016.12.008
Vatansever, F., de Melo, W. C., Avci, P., Vecchio, D., Sadasivam, M., Gupta, A., et al. (2013). Antimicrobial strategies centered around reactive oxygen species–bactericidal antibiotics, photodynamic therapy, and beyond. FEMS Microbiol. Rev. 37, 955–989. doi: 10.1111/1574-6976.12026
Vermeulen, N., Keeler, W. J., Nandakumar, K., and Leung, K. T. (2008). The bactericidal effect of ultraviolet and visible light on Escherichia coli. Biotechnol. Bioeng. 99, 550–556. doi: 10.1002/bit.21611
Wood, T. K., Song, S., and Yamasaki, R. (2019). Ribosome dependence of persister cell formation and resuscitation. J. Microbiol. 57, 213–219. doi: 10.1007/s12275-019-8629-2
Yamasaki, R., Song, S., Benedik, J. M., and Wood, K. T. (2020). Persister cells resuscitate using membrane sensors that activate chemotaxis, lower cAMP levels, and revive ribosomes. iScience 23:100792. doi: 10.1016/j.isci.2019.100792
Yamasaki, R., Takatsuji, Y., Morimoto, M., Ishikawa, S., Fujinami, T., and Haruyama, T. (2017). Sustainable process for functional group introduction onto HOPG by exposing ·OH and 1O2 using a radical vapor reactor (RVR) without any chemical reagents. Colloid. Surf. A Physicochem. Eng. Aspects 522, 328–334. doi: 10.1016/j.colsurfa.2017.03.013
Yamasaki, R., Takatsuji, Y., Morimoto, M., Sakakura, T., Matsuo, K., and Haruyama, T. (2018). Green surface cleaning in a radical vapor reactor to remove organic fouling on a substrate. Electrochemistry 86, 355–362. doi: 10.5796/electrochemistry.18-00036
Keywords: reactive oxygen species, persister, Escherichia coli, radical vapor reactor, biofilm
Citation: Kawano A, Yamasaki R, Sakakura T, Takatsuji Y, Haruyama T, Yoshioka Y and Ariyoshi W (2020) Reactive Oxygen Species Penetrate Persister Cell Membranes of Escherichia coli for Effective Cell Killing. Front. Cell. Infect. Microbiol. 10:496. doi: 10.3389/fcimb.2020.00496
Received: 12 September 2019; Accepted: 10 August 2020;
Published: 18 September 2020.
Edited by:
Rodnei Dennis Rossoni, São Paulo State University, BrazilReviewed by:
Michael Benedik, Texas A&M University, United StatesAnkush Prasad, Palacký University, Olomouc, Czechia
Copyright © 2020 Kawano, Yamasaki, Sakakura, Takatsuji, Haruyama, Yoshioka and Ariyoshi. This is an open-access article distributed under the terms of the Creative Commons Attribution License (CC BY). The use, distribution or reproduction in other forums is permitted, provided the original author(s) and the copyright owner(s) are credited and that the original publication in this journal is cited, in accordance with accepted academic practice. No use, distribution or reproduction is permitted which does not comply with these terms.
*Correspondence: Ryota Yamasaki, r18yamasaki@fa.kyu-dent.ac.jp