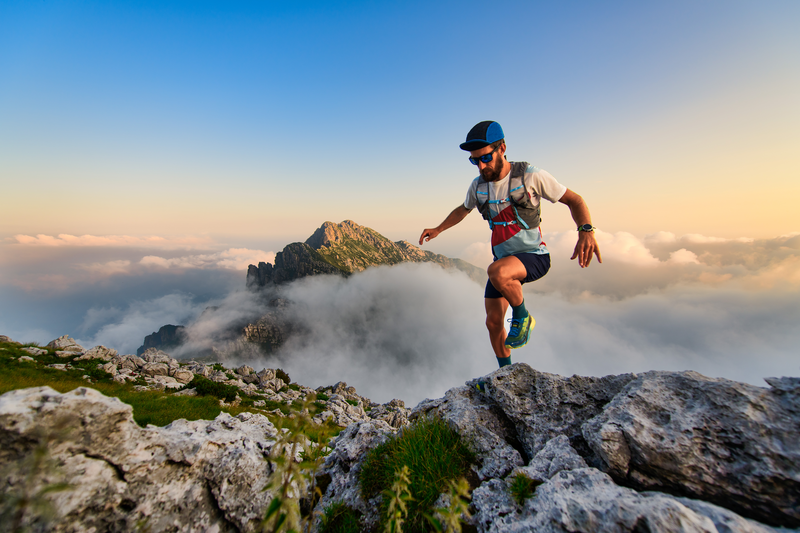
95% of researchers rate our articles as excellent or good
Learn more about the work of our research integrity team to safeguard the quality of each article we publish.
Find out more
ORIGINAL RESEARCH article
Front. Cell. Infect. Microbiol. , 16 September 2020
Sec. Bacteria and Host
Volume 10 - 2020 | https://doi.org/10.3389/fcimb.2020.00447
Enterobacteria species are common causes of hospital-acquired infections, which are associated with high morbidity and mortality rates. Immunocompromised patients such as solid organ transplant (SOT) recipients are especially at risk because they are frequently exposed to antibiotics in the course of their treatments. In this work, we used a collection of 106 Escherichia coli, 78 Klebsiella pneumoniae, 25 Enterobacter spp., and 24 Citrobacter spp. multidrug resistant strains isolated from transplant patients (hepatic, renal or renal/pancreatic) in order to examine their ability to adhere in vitro to HT-29 human colon cells, and to determine if some adhesive characteristics are associated with prevalence and persistence of these strains. A total of 33 E. coli (31%), 21 K. pneumoniae (27%), 7 Enterobacter spp. (28%), and 5 Citrobacter spp. (21%), adhered to the colon epithelial cells. Two main adherence patterns were observed in the four species analyzed, diffuse adherence, and aggregative adherence. Under transmission electronic microscopy (TEM), most bacteria lacked visible fimbria on their surface, despite their strong adherence to epithelial cells. None of the strains studied was able to induce any cytotoxic effect on HT-29 cells although some of them strongly colonizing both cells and glass coverslips at high density. Some of the strains failed to adhere to the epithelial cells but adhered strongly to the cover-slide, which shows that microscopy studies are mandatory to elucidate the adherence of bacteria to epithelial cells in vitro, and that quantitative assays using colony forming unit (CFUs) counting need to be supplemented with pictures to determine definitively if a bacterial strain adheres or not to animal cells in vitro. We report here, for the first time, the aggregative adherence pattern of two multidrug resistant (MDR) Citrobacter freundii strains isolated from human patients; importantly, biofilm formation in Citrobacter is totally dependent on the temperature; strong biofilms were formed at room temperature (RT) but not at 37°C, which can play an important role in the colonization of hospital surfaces. In conclusion, our results show that there is a great variety of adhesion phenotypes in multidrug-resistant strains that colonize transplanted patients.
The Enterobacterales family is a heterogeneous group of Gram-negative bacteria, colonizing the intestinal tract of humans and animals. These bacteria are opportunistic pathogens, commonly isolated from nosocomial lung, urinary tract, intraabdominal, and bloodstream infections, and many of them in intensive care units (ICUs) (Cervera et al., 2012; Hollyer and Ison, 2018).
The precise mechanism by which these bacteria pass from the intestinal tract of patients to the environment to cause hospital outbreaks is poorly known, although several factors such as adherence to human intestinal cells and the formation of biofilms could be key in this process.
Colonization of the epithelium is the first step in enterobacterial pathogenesis and this ability has been demonstrated using different cell lines in vitro. For diarrhoeagenic E. coli, the ability to adhere to human epithelial cells is expressed as specific patterns. When these bacteria adhere to the cells as tight clusters, the adherence is called aggregative adherence (AA), and bacteria form aggregates between the cells and the surface (usually coverslips). When these bacteria adhere diffusely to the cell surface, the pattern is called diffuse adherence; and when bacteria adhere in small clusters to the cells the adherence pattern is called localized. The AA phenotype is well-known in enteroaggregative E. coli (EAEC) (Nataro et al., 1992), but was also demonstrated in K. pneumoniae (Favre-Bonte et al., 1995; Livrelli et al., 1996), and non-human strains of Citrobacter freundii (Bai et al., 2012). Citrobacter spp. and Enterobacter spp. are increasingly being recognized as causative agents of nosocomial infections and are an important reservoir of antimicrobial resistance determinants (Chavda et al., 2016; Majewski et al., 2017; Liu et al., 2018; Yang et al., 2018; Annavajhala et al., 2019). While Klebsiella and E. coli are versatile pathogens, less is known about the adhesion, virulence, and pathogenicity of Enterobacter and Citrobacter because these genera of bacteria are very heterogeneous. All of these bacteria are increasingly the cause of nosocomial infections in immunocompromised patients such as solid organ transplant (SOT) recipients (Kumar and Ison, 2019). These patients are especially at risk of developing infections by multidrug resistant (MDR) bacteria, since among other risk factors, they are frequently exposed to antibiotics in healthcare settings.
Knowing the adherence properties of these species will help us to understand bacterial persistence and dissemination from medical devices and hospital settings, and to improve decolonization protocols in patients colonized with these pathogens.
In this work, we collected strains of K. pneumoniae, E. coli, Enterobacter spp., and Citrobacter spp. from different SOT patients in order to examine their ability to adhere in vitro to HT-29 human colon cells, and to discuss if some adhesive characteristics could associated with prevalence and persistence of these strains. As we report here the aggregative adherence pattern of two MDR Citrobacter freundii strains isolated from human patients, we have further investigated their adherence characteristics by performing biofilm formation assays.
The human prospective cohort study was conducted between August 2014 and April 2018 in seven University Hospitals from five Spanish regions. This national project (the ENTHERE Study) focused on the study of intestinal colonization and infections by multidrug resistant Enterobacterales (MDRE) in patients with kidney, liver and kidney/pancreas transplants (Ramos-Vivas et al., 2019).
The study was performed in accordance with the Declaration of Helsinki. The protocol was reviewed and approved by the Institutional Ethics Committee at all participating hospitals. The participating hospitals were: Hospital Universitario Marqués de Valdecilla (Santander), Coordinating Center; Hospital de Cruces (Bilbao); Hospital Clinic Universitari (Barcelona); Hospital Gregorio Marañón (Madrid); Hospital Universitario 12 de Octubre (Madrid); Hospital Reina Sofía (Córdoba); and Hospital Universitario Ramón y Cajal (Madrid). Informed consent was obtained from each patient, according to local standards.
Over a period of 33 months, from October 2014 to June 2017, a total of 243 MDRE isolates defined as AmpC-hyperproducers and/or extended-spectrum β-lactamases (ESBLs) or carbapenemase producers, were included in this study. MDRE were obtained from 108 patients with kidney or liver transplant or both kidney and pancreatic transplant from the ENTHERE Study. Among these, 106 were E. coli, 78 were K. pneumoniae, 25 were Enterobacter spp., 23 were C. freundii and 1 was C. braakii. As described previously (Ramos-Vivas et al., 2019), bacteria were isolated from rectal swabs (in the 48 h prior to transplant and weekly samples till 4–6 weeks after transplantation) and directly inoculated onto chromID® ESBL and chromID® CARBA chromogenic agar plates (bioMérieux, Marcy L'Etoile, France). Colonies were subcultured on blood agar and MacConkey agar and preliminary identification and antimicrobial susceptibility was performed using the Vitek 2 system (bioMérieux, France). Identification was confirmed by MALDI-TOF using the Vitek MS (bioMérieux) system, in accordance with the manufacturer's instructions. Stock cultures were frozen at −80°C with 20% (vol/vol) glycerol.
As a control for specific adherence experiments, one strain of Corynebacterium striatum was used. As a control for bacterial cytotoxicity, Serratia liquefaciens strain HUMV-3250 was used (Remuzgo-Martinez et al., 2013).
HT-29 (ATCC® HTB-38™) human colon cells were cultured in McCoy's 5a medium (Gibco) and placed in 24-well tissue culture plates containing round glass coverslips. All strains were cultured overnight in 10 ml of Luria broth at 37°C with moderate shaking (175 rpm). Bacterial suspensions were washed in phosphate-buffered saline (PBS) and adjusted to approximately 5 × 109 CFU ml−1. HT-29 cells were infected with bacteria at a multiplicity of infection (MOI, bacterium:eukaryotic cell ratio) of ~100:1. The infected plates were centrifuged for 4 min at 200 × g prior to incubation to promote adherence of bacteria to cells and to synchronize infections. Infected monolayers were then incubated at 37°C with 5% CO2 for 180 min. For Citrobacter strains, experiments were also conducted with McCoy's 5a medium containing 1% mannose. After infection, cells were washed four times and fixed with cold paraformaldehyde (3.2% in PBS) for 20 min at room temperature (RT). Then, cells were permeabilized with Triton X-100 (0.1% in PBS) for 5 min at room temperature and washed five times with PBS. Atto-488 phalloidin (Sigma), which binds polymerized F-actin, was used to identify actin filaments and fibers. After cytoskeleton staining, coverslips were mounted on glass slides with Fluoroshield mounting medium containing DAPI (Sigma Aldrich) to stain double-stranded DNA. All preparations were examined with a Nikon A1R confocal scanning laser microscope equipped with 403 nm and 488 nm lasers and with a differential interference contrast (DIC) filter. Images were captured at random with a ×40 Plan-Fluor 1.3 NA (Numerical Aperture), ×60 Plan-Apo 1.4 NA, or ×100 Apo-TIRF 1.49 NA objectives, and processed using the NIS-Elements 3.2 software. All immunofluorescence experiments for each strain were repeated at least 2 times and photographed by two different observers.
Selected strains (two adherent and two non-adherent) for each species were examined by TEM after growth at 37°C in Luria broth. Bacteria were applied to Formvar-coated grids and were air dried. The cells were then negatively stained with 1% phosphotungstic acid in distilled water for 5 s and were examined with a JEM-1011 transmission electron microscope (JEOL) operating at 80 kV and equipped with an Orius SC1000 charge-coupled device (CCD) camera (Gatan).
Biofilm formation of Citrobacter strains was estimated in 24-well polystyrene plates (Corning, Costar) as previously described (Remuzgo-Martinez et al., 2015; Ramos-Vivas et al., 2019). Briefly, plates were incubated for 48 h at 37°C and at 25°C, in static. Planktonic cells were removed and wells containing biofilms were rinsed three times with distilled water and the remaining adherent bacteria were stained with 2 ml/well of crystal violet (CV). CV was extracted by acetic acid (33%) and the amount of dye was determined at 620 nm using a microplate reader (Multiskan FC; Thermo Fisher). In each experiment, results were corrected for background staining by subtracting the value for crystal violet bound to uninoculated controls. The assays were performed 4 times for each isolate and the mean ± SD was reported. Citrobacter strains were classified as non-adherent, moderate or strong biofilm producers using the following criteria: OD ≤ 0.05, non-biofilm producer; OD > 0.05–0.1, weak biofilm producer; OD > 0.1 strong biofilm producer.
Biofilm architecture of selected Citrobacter strains was studied in 4-well μ-slides (Ibidi, Martinsried, Germany) as previously described (Remuzgo-Martinez et al., 2015). Briefly, the slides were placed inclined (~45°) into an incubator to form a liquid-air interface and after 48 h at 37 or 25°C, unfixed planktonic cells were removed by rinsing with saline (0.85% NaCl), and bacterial viability within biofilms was determined by using the BacLight LIVE/DEAD bacterial viability kit (Molecular Probes Inc.). A series of optical sections was obtained with a Nikon A1R confocal microscope; the excitation wavelengths were 488 nm (green) and 561 nm (red), and 500–550 nm and 570–620 nm emission filters were used, respectively. Images at the liquid-air interface were captured at random with a 20 × Plan Apo (NA, 0.75) objective. Reconstructions of confocal sections and quantitative measurements were performed using NIS-Elements software, version 3.2.
Standard PCR was used to amplify several genes encoding extended-spectrum β-lactamases (blaTEM, blaSHV, and blaCTXM) and carbapenemases (blaKPC, blaVIM, blaIMP, blaNDM, and blaOXA−48) as previously described (Ramos-Vivas et al., 2019). PCR multiplex plasmid-mediated AmpC (blaCIT, blaFOX, blaMOX, blaDHA, blaACC, and blaEBC) was performed as described elsewhere (Perez-Perez and Hanson, 2002).
All data from biofilm assays were derived from four independent experiments. Statistical analysis of the data was carried out with Student's paired two-tailed t-test. P < 0.05 was considered statistically significant.
Adhesion to human colon cells was considered positive if cells presented more than 1 bacteria per cell, after examining at least a quarter of the surface of two different coverslides and/or counting at least 100 eukaryotic cells. As shown in Table 1, a total of 33 E. coli (31%), 21 K. pneumoniae (27%), 7 Enterobacter spp. (28%), and 5 Citrobacter spp. (21%), adhered to the colon epithelial cells. Most strains, 73 E. coli (69%), 57 K. pneumoniae (73%), 19 Citrobacter spp. (79%), and 18 Enterobacter spp. (72%) did not adhere to this cell type (Supplementary Table 1). We observed two main patterns of adhesion, aggregative and diffuse, which are present in the four bacterial genera studied. Of these, 8 E. coli (7.5%), 4 K. pneumoniae (5%), 3 Enterobacter spp. (12%), and 2 Citrobacter spp. (8.3%) adhered to the colon epithelial cells in the so-called aggregative pattern, where large clusters of bacteria covered the cell surface or were located in large groups at the edge of the cellular cytoskeleton (Figure 1). DAPI staining helped us to demonstrate the presence of clusters of bacteria, since some strains do not differentiate well when they are on the cells (Figure 1d,d′). The other main pattern observed in most adherent strains was the diffuse adherence pattern, where the bacteria are randomly and individually attached to the edge of the cytoskeleton or over the cells (Figure 2).
Figure 1. Representative images of aggregative adherence to HT-29 cells by E. coli strain 185 (a), Enterobacter spp. strain 131 (b), Citrobacter freundii strain 202 (c), and Klebsiella pneumoniae strain 209 (d). Arrows in (d) indicate some clusters of bacteria. (d′) Shows a detail of the DAPI channel from the boxed area in (d). Cells were stained with Atto-488 phalloidin (green) and DAPI (blue). Scale bars: (a,b,d) 10 μm; (c) 5 μm. Original magnification: (a,b,d) ×600; (c) ×400.
Figure 2. Representative images of diffuse and localized adherence to HT-29 cells by E. coli strain 186 (a), Enterobacter spp. strain 136 (b), Citrobacter freundii strain 152 (c), and Klebsiella pneumoniae strain 195 (d). (a′) shows the DAPI channel from (a); where arrows indicate some attached bacteria. (c′) shows a detail of the boxed area in (c) and arrows indicate individual attached bacteria. In (d), a central area of the HT-29 cells is shown with the DAPI channel in the boxed area. Arrows indicate individual attached bacteria at the edge of the cells and open arrows in the boxed areas indicate some clusters of bacteria on the cell surface. Cells were stained with Atto-488 phalloidin (green) and DAPI (blue). Scale bars: (a,b) 10 μm; (c) 5 μm. Original magnification: (a,b,d) ×600; (c) ×400.
The so-called localized adherence pattern, was hard to see in our assays so, the adherence patterns in this work for all strains were divided into only diffuse or aggregative. The localized adherence was clearly demonstrated using a Corynebacterium strain as a positive control, which is able to adhere specifically and in a localized fashion to HT-29 cells (Supplementary Figure 1a).
Some strains adhered strongly to the glass coverslips. This adhesion to the inert surface seems to be very common for many isolates and should be taken into account or considered when carrying out quantitative studies on adherence to eukaryotic cells in vitro (Figure 3).
Figure 3. Representative images of negative adherence to HT-29 cells. (a) E. coli strain 143; (b) Enterobacter spp. strain 147; (c,c′) K. pneumoniae strain 25. Scale bars: (a,b) 10 μm; (c) 5 μm. Original magnification: (a,b) ×600; (c,c′) ×400.
Interestingly, two strains, E. coli 154 and K. pneumoniae 135 appeared to form pockets on the surface of the cells, similar to actin pedestal formation (Figure 4). However, these bacteria are not intracellular, because they are not covered by the actin cytoskeleton. In fact, by confocal microscopy we have not detected any obvious signs that any of the strains were in an intracellular position. The study of the possible invasion or intracellular behavior of bacteria is beyond the objectives of this work.
Figure 4. Representative images of E. coli and K. pneumoniae strains remodeling the actin cytoskeleton on the surface of HT-29 cells. Cells were stained with Atto-488 phalloidin (green) and DAPI (blue). Arrows indicate some bacteria forming pockets on the surface of HT-29 cells. (a–a′′) Maximum intensity projection of a 3D confocal image showing E. coli strain 154; (b,b′) K. pneumoniae strain 135. Scale bars: 10 μm; Original magnification: (a–a′′) ×600; (b,b′) ×400.
HT-29 look healthy after 3 h post-infection in all strains as similar to uninfected cells used as control, with the cytoskeleton well-attached to the glass coverslide and the nuclei well-stained with DAPI, with no signs of cytotoxicity. As a positive control for cytotoxicity, S. liquefaciens strain HUMV-3250 was used; after 90 min of infection with this bacterium, the actin cytoskeleton staining revealed that the cells start to detach and the nuclei get smaller (Supplementary Figure 1b). Control for negative adherence (to cells or to coverslips), and control cells (no infection) are shown in Supplementary Figures 1c,d.
The ability of Citrobacter strains to form biofilms was quantified by crystal violet (CV) staining after 48 h at 37 or 25°C. All the biofilms were found at the liquid-air interface and were strongly influenced by temperature (Figure 5). Four representative isolates were selected for confocal microscopy analysis. Morphology of biofilms was found to be homogeneous but these strains showed weak or strong biofilm formation depending on temperature (Supplementary Figure 2).
Figure 5. Quantification of biofilm formation. Biofilm formation by Citrobacter strains after growth at 37 or 25°C. Quantification of biofilm formation was performed after crystal violet extraction and measurement (OD620). Values are presented as the mean ± standard deviation (SD) of four independent experiments. *p < 0.05; **p < 0.01, and ***p < 0.001.
Representative TEM images of selected strains are shown in Figure 6. We have chosen a diffuse adherent and a localized adherent strain of each species to study its surface by TEM. Strains of E. coli, and Citrobacter (diffuse or aggregative adherence) grown overnight on Luria broth showed a smooth surface. In contrast, piliated cells appeared clearly in diffuse adherent Enterobacter spp. and K. pneumoniae strains.
Figure 6. TEM. Examples of transmission electron micrographs of negatively stained bacteria grown overnight in Luria medium at 37°C. (a) E. coli 46 (aggregative adherence); (b) Enterobacter 155 (aggregative adherence); (c) K. pneumoniae 25 (diffuse adherence); (d) C. freundii 207 (aggregative adherence); (e) Enterobacter 121 (diffuse adherence); (f) K. pneumoniae 135 (diffuse adherence). Note the detachment of pili from the bacterial surface of Enterobacter 121. Scale bars indicate: (a) 1 μm; (b) 0.5 μm; (c) 1 μm; (d) 1 μm; (e) 2 μm; (f) 2 μm. Magnification: (a) ×40,000; (b) ×80,000; (c,d) ×60,000; (e,f) ×25,000.
All Citrobacter isolates were clonally unrelated according to PFGE analysis (unpublished results). All strains tested except number 10 and 21 (CTXM-group 9), and strain 14 (CTXM-group 1) were positive for the overproduction of intrinsic chromosomal AmpC β-lactamases (Supplementary Table 1).
Since adherence of bacteria to cells is considered an essential step in bacterial pathogenesis, adherence to host cells in vitro provides a useful tool to investigate the interactions between pathogens and the human epithelium that occurs during infections.
Moreover, the study of colonization in SOT recipients by MDR enterobacteria may help to understand the role in infections caused by these colonizing microorganisms, considering that those patients are frequently exposed to antibiotics.
In this way, the HT-29 cell line is a very suitable model to study adherence by enteric pathogens, because these cells share morphological and functional features with normal colon cells (Gagnon et al., 2013). Therefore, the use of human colon cells can help us understand the colonization of the human intestine by these opportunistic pathogens. In this study, the adherence ability varied extensively among the strains used, and was independent of the type of transplant.
Phenotypic heterogeneity in adhesion was obvious in all species. We have not carried out quantitative studies of CFUs counts because most of the strains of the four species used in this study adhered poorly to HT-29 cells, but many of these adhered strongly to the glass surface. In the in vitro quantitative adherence experiments, CFU numbers were commonly used to classify bacteria as adherent or not adherent to human cells, assuming that all colony forming units obtained on bacteriological media after lysis of cell-infected cultures came from cell-attached bacteria. We note that many of these quantitative experiments lack microscopy. If microscopy is not used to check if the bacteria are really attached to the cells, it cannot really be said that the bacteria that are being used in these host-pathogen interaction studies were really adherent (Lazaro-Diez et al., 2016). Besides not using microscopy to observe if the bacteria are really attached to the cells or to the substrate, a positive control of adhesion is not commonly used; for example, with a bacterium that only adheres to the cells and not to the surface where these cells are growing. This means that microscopy photographs should be mandatory to evaluate the adherence of bacteria to cells, and that large microscope fields need to be showed to really verify bacterial adherence. Our positive control (C. striatum) demonstrated higher levels of specific adherence to these cells, and not to the substrate (glass) further validating our model.
We observed two different adhesion patterns, aggregative, and diffuse. The so-called localized adherence pattern, reported elsewhere for some strains of E. coli, K. pneumoniae, and Enterobacter spp. (Livrelli et al., 1996; Scaletsky et al., 1999; Mange et al., 2006) where small clusters are attached to the cells, were hard to see in our assays so, the adherence patterns in this work for all strains were divided only into diffuse or aggregative. Our results showing adherence heterogeneity are not new, because strains of the genera Klebsiella, Escherichia, and Enterobacter use different patterns to bind epithelial cells (Livrelli et al., 1996; Lopes et al., 2005; Mange et al., 2006; Krzyminska et al., 2010; Alcantar-Curiel et al., 2013; Kalita et al., 2014). However, there is a paucity of research using human colon cells to study the adherence of enterobacteria. Using colon cells is interesting because they can give us an idea of the potential of these strains to remain in the intestine of colonized patients. Only 17 strains (8 E. coli, 4 K. pneumoniae, 3 Enterobacter cloacae, and 2 Citrobacter freundii) adhered to the colon epithelial cells in the so-called aggregative pattern. The other main adherence pattern was observed in 49 strains (where bacteria are randomly attached to the edge of the cytoskeleton or over the cells).
Some strains of K. pneumoniae and E. coli appear to form pockets, similar to actin pedestal formation but generating structures toward the inside of the cell, in the inverse sense to the actin pedestals exhibited by Enterohemorhagic E. coli (EHEC) (Battle et al., 2014; Mcwilliams and Torres, 2014); these structures generated toward the inside of the eukaryotic cell merit further study, because in silico analysis of the so far sequenced K. pneumoniae genomes does not identify any T3SS components, although they are present in EHEC, EPEC, and Citrobacter rodentium (Kelly et al., 2006; Cano et al., 2009; Gaytan et al., 2016; Marcoleta et al., 2018).
Overall, the pattern of adherence is not related to the type of transplant. Also, when we have observed the relationship between adhesion and the presence of fimbriae on the surface of bacteria, we have not found any correlation. Some aggregative strains do not have visible fimbriae and others do, both in Klebsiella and Enterobacter.
We report for the first time the aggregative adherence pattern of two multidrug resistant (MDR) C. freundii strains isolated from human patients. Both strains produce a large biofilm when grown at 25°C. Other authors have found a strain of C. freundii isolated from goat that has a similar pattern of adherence, and that was also toxic to HEp-2 cells (Bai et al., 2012), indicating that this type of adherence may play a role in the pathogenicity of the bacterium. Adherence of the strains was not inhibited by the addition of 1% mannose. Furthermore, the aggregative strains did not show any visible fimbriae on their surface, as observed by transmission electron microscopy although, as in some Enterobacterales, the expression of fimbria could be regulated by temperature (Dorman and Ni Bhriain, 1992; Padilla et al., 2009; Hinthong et al., 2015) which could be involved in the formation of biofilms at room temperature but not at 37°C. Our Citrobacter strains were not cytotoxic, but both were multi-resistant, carrying a CTX-M extended-spectrum β-lactamase and hyperproduction of chromosomal AmpC, which should be taken into account when studying the biology of these pathogens in hospitals.
Cytotoxicity was reported in Citrobacter strains when HEp-2 cells (human larynx carcinoma) were used (Liu et al., 2018). Perhaps the difference in the results is that colon cells resist the presence of enterobacteria better, although the differences could also be explained by the different methods used to quantify cytotoxicity.
In this work, the percentage of strains of Citrobacter producing biofilm at 37°C was much lower than E. coli or Klebsiella strains and was similar to Enterobacter strains (Ramos-Vivas et al., 2019). In fact, the rate of biofilm formation by Enterobacter strains previously reported was very low (Ramos-Vivas et al., 2019). However, most Citrobacter strains produce strong biofilms after growth at room temperature, which can favor its persistence in the hospital environment. This finding could suggest that these strains can use a different strategy to persist in the host and to cause disease.
We also studied 6 strains that caused infections after transplantation. Of these, 3 showed a diffuse adherence pattern (E. coli 199, E. cloacae 121, and K. pneumoniae 163) and 3 were non-adherent (K. pneumoniae strains 27, 169, and 172). No strain with an aggregative pattern caused infection in transplant patients. Overall, these results indicate that adherence to colon cells by this large collection of strains is puzzling, but some strains that have caused infection and others that have an oriented phenotype to establish themselves in the cell's cytoskeleton deserve further investigation.
In this report, we have demonstrated that Enterobacterales strains display a high degree of phenotypic variability, which reinforces the relevance of monitoring the potential adverse impact of MDRE species in immunocompromised patients such as SOT recipients. Moreover, highly adherent strains can persist in the host in high numbers and can act as a reservoir of antimicrobial resistance genes so, the understanding of the interplay between those strains and the host could help us to design new strategies or treatments against them. Furthermore, these data, and recent concerning findings on MDR Citrobacter clinical isolates (Majewski et al., 2017; Venditti et al., 2017; Yang et al., 2018), call for further efforts to study the significance of Citrobacter in hospital settings.
All datasets presented in this study are included in the article/Supplementary Material.
The studies involving human participants were reviewed and approved by the study was performed in accordance with the Declaration of Helsinki. The protocol was reviewed and approved by the Institutional Ethics Committee at all participating hospitals. The participating hospitals were: Hospital Universitario Marqués de Valdecilla (Santander), Coordinating Center; Hospital de Cruces (Bilbao); Hospital Clinic Universitari (Barcelona); Hospital Gregorio Marañón (Madrid); Hospital Universitario 12 de Octubre (Madrid); Hospital Reina Sofía (Córdoba); and Hospital Universitario Ramón y Cajal (Madrid). Informed consent was obtained from each patient, according to local standards. Informed consent was obtained from each patient. The patients/participants provided their written informed consent to participate in this study.
JR-V, IC-G, MF-M, CG-R, and MF conceived and designed the experiments and analyzed the data. JR-V, IC-G, MF-M, CG-R, and JB performed the experiments. JR-V and MF wrote the paper. All authors have contributed strains, read, and approved the final manuscript.
This research was supported by Plan Nacional de I+D+i and Instituto de Salud Carlos III (Fondo de Investigaciones Sanitarias PI13/01191 to MF and PI16/01103 to JR-V), Subdirección General de Redes y Centros de Investigación Cooperativa, Spanish Ministry of Economy and Competitiveness, Spanish Network for Research in Infectious Diseases (REIPI RD16/0016/0007, RD16/0016/0010, RD16/0016/0012, RD16/0016/0011, RD16/0016/0008, and RD16/0016/0002) co-financed by the European Development Regional Fund A way to achieve Europe ERDF, and Ministerio de Ciencia e Innovación, Acciones de dinamización ≪Redes de Investigación≫ RED2018-102469-T.
The authors declare that the research was conducted in the absence of any commercial or financial relationships that could be construed as a potential conflict of interest.
The authors thank Dr. Fidel Madrazo (Electron Microscopy Unit, Technology Support Services, IDIVAL) for helping with confocal microscopy.
Carlos Armiñanzas3, Francisco Arnaiz de las Revillas3, Jorge Calvo2, Fernando Casafont-Morencos15, Emilio Fábrega15, Concepción Fariñas-Álvarez16, Mónica Gozalo2, Emilio Rodrigo17, Juan Carlos Ruiz San Millán17, Carlos Salas 1,2, Pilar Martin Dávila5, Patricia Ruiz Garbajosa18, Ana Sánchez-Díaz18, Adolfo Martínez19, Asunción Moreno7, Marta Bodro7, Maria Fernanda Solano7, María José Blanco8, Miguel Montejo8, Caroline Agnelli Bento10, Emilia Cercenado10, Mansilla, Marina Machado10, María Olmedo10, Cristina Rincón Sanz10, Teresa Vicente Rangel10, María Luisa Rodríguez Ferrero20, Sara Rodríguez Fernández10, María Luisa Rodríguez-Ferrero20, Luis Alberto Sánchez Cámara20, Elena Resino11, Fernando Rodríguez13, Julian Torre-Cisneros14. 1,3Infectious Diseases Service. Hospital Universitario Marqués de Valdecilla, Instituto de Investigación Valdecilla-IDIVAL, University of Cantabria. 1,2Service of Microbiology, Hospital Universitario Marqués de Valdecilla, Santander, Spain. 5Infectious Diseases Department, Hospital Universitario Ramón y Cajal, Madrid, Spain. 7Infectious Diseases Service, Hospital Clínic-IDIBAPS, Universidad de Barcelona, Barcelona, Spain. 8Infectiuos Diseases Unit, Hospital Universitario de Cruces, Barakaldo, Spain. 10Clinical Microbiology and Infectious Diseases, Hospital General Universitario Gregorio Marañon, Madrid, Spain. 11Infectious Diseases Unit, Hospital Universitario 12 de Octubre, Madrid, Spain.13 Microbiology Unit, Hospital Universitario Reina Sofía, Córdoba, Spain. Maimonides Biomedical Research Institute of Cordoba (IMIBIC), Córdoba, Spain. Department of Microbiology, Universidad de Córdoba, Córdoba, Spain. 14Infectious Diseases Unit, Hospital Universitario Reina Sofía, Córdoba, Spain. 15 Liver Unit, Hospital Universitario Marqués de Valdecilla Santander, Spain. 16Nephrology Department, Hospital Universitario Marqués de Valdecilla, Santander, Spain. 17 Quality Unit, Hospital Universitario Marqués de Valdecilla, Santander, Spain. 18Service of Microbiology, Hospital Universitario Ramón y Cajal, Madrid, Spain. 19Transplant Coordination, Hospital Universitario Ramón y Cajal, Madrid, Spain. 20Department of Nephrology, Hospital General Universitario Gregorio Marañon, Madrid, Spain.
The Supplementary Material for this article can be found online at: https://www.frontiersin.org/articles/10.3389/fcimb.2020.00447/full#supplementary-material
Supplementary Figure 1. C. striatum was used to determine specific adherence to HT-29 cells and as a control for localized adherence to HT-29 cells. Cells were infected for 3 h. C. striatum and the bacteria stained with a polyclonal antibody are shown in pink. The image in (b) was intentionally overexpose to show DAPI staining nuclei and bacteria. (c) Shows a non-adherent strain (neither to the cells nor the coverslips) and one control slide with cells alone (d). Arrow indicates a single bacterium to account for the assay. Magnification (a,c,d) ×400; (b) ×600. Scale bars indicate: (a,c,d) 10 μm; (b) 5 μm.
Supplementary Figure 2. CLSM of biofilm formation by selected Citrobacter strains at different temperatures. Representative examples of CLSM images of selected strains after biofilm formation. Bacteria were stained with the BacLight LIVE/DEAD viability kit. Live cells fluoresce in green with Syto 9 dye and dead cells are stained red with propidium iodide. Original magnification: ×200.
Supplementary Table 1. Strains that did not show adherence to colon cells. Strains that caused infection are highlighted in bold.
Alcantar-Curiel, M. D., Blackburn, D., Saldana, Z., Gayosso-Vazquez, C., Iovine, N. M., De La Cruz, M. A., et al. (2013). Multi-functional analysis of Klebsiella pneumoniae fimbrial types in adherence and biofilm formation. Virulence 4, 129–138. doi: 10.4161/viru.22974
Annavajhala, M. K., Gomez-Simmonds, A., and Uhlemann, A. C. (2019). Multidrug-resistant Enterobacter cloacae complex emerging as a global, diversifying threat. Front. Microbiol. 10:44. doi: 10.3389/fmicb.2019.00044
Bai, L., Xia, S., Lan, R., Liu, L., Ye, C., Wang, Y., et al. (2012). Isolation and characterization of cytotoxic, aggregative Citrobacter freundii. PLoS ONE 7:e33054. doi: 10.1371/journal.pone.0033054
Battle, S. E., Brady, M. J., Vanaja, S. K., Leong, J. M., and Hecht, G. A. (2014). Actin pedestal formation by enterohemorrhagic Escherichia coli enhances bacterial host cell attachment and concomitant type III translocation. Infect. Immun. 82, 3713–3722. doi: 10.1128/IAI.01523-13
Cano, V., Moranta, D., Llobet-Brossa, E., Bengoechea, J. A., and Garmendia, J. (2009). Klebsiella pneumoniae triggers a cytotoxic effect on airway epithelial cells. BMC Microbiol. 9:156. doi: 10.1186/1471-2180-9-156
Cervera, C., Linares, L., Bou, G., and Moreno, A. (2012). Multidrug-resistant bacterial infection in solid organ transplant recipients. Enferm. Infecc. Microbiol. Clin. 30(Suppl. 2), 40–48. doi: 10.1016/S0213-005X(12)70081-2
Chavda, K. D., Chen, L., Fouts, D. E., Sutton, G., Brinkac, L., Jenkins, S. G., et al. (2016). Comprehensive genome analysis of carbapenemase-producing Enterobacter spp.: new insights into phylogeny, population structure, and resistance mechanisms. MBio 7:e02093-16. doi: 10.1128/mBio.02093-16
Dorman, C. J., and Ni Bhriain, N. (1992). Thermal regulation of fimA, the Escherichia coli gene coding for the type 1 fimbrial subunit protein. FEMS Microbiol. Lett. 78, 125–130. doi: 10.1111/j.1574-6968.1992.tb05554.x
Favre-Bonte, S., Darfeuille-Michaud, A., and Forestier, C. (1995). Aggregative adherence of Klebsiella pneumoniae to human intestine-407 cells. Infect. Immun. 63, 1318–1328. doi: 10.1128/IAI.63.4.1318-1328.1995
Gagnon, M., Zihler Berner, A., Chervet, N., Chassard, C., and Lacroix, C. (2013). Comparison of the Caco-2, HT-29 and the mucus-secreting HT29-MTX intestinal cell models to investigate Salmonella adhesion and invasion. J. Microbiol. Methods 94, 274–279. doi: 10.1016/j.mimet.2013.06.027
Gaytan, M. O., Martinez-Santos, V. I., Soto, E., and Gonzalez-Pedrajo, B. (2016). Type three secretion system in attaching and effacing pathogens. Front. Cell. Infect. Microbiol. 6:129. doi: 10.3389/fcimb.2016.00129
Hinthong, W., Indrawattana, N., Pitaksajjakul, P., Pipattanaboon, C., Kongngoen, T., Tharnpoophasiam, P., et al. (2015). Effect of temperature on fimbrial gene expression and adherence of enteroaggregative Escherichia coli. Int. J. Environ. Res. Public Health 12, 8631–8643. doi: 10.3390/ijerph120808631
Hollyer, I., and Ison, M. G. (2018). The challenge of urinary tract infections in renal transplant recipients. Transpl. Infect. Dis. 20:e12828. doi: 10.1111/tid.12828
Kalita, A., Hu, J., and Torres, A. G. (2014). Recent advances in adherence and invasion of pathogenic Escherichia coli. Curr. Opin. Infect. Dis. 27, 459–464. doi: 10.1097/QCO.0000000000000092
Kelly, M., Hart, E., Mundy, R., Marches, O., Wiles, S., Badea, L., et al. (2006). Essential role of the type III secretion system effector NleB in colonization of mice by Citrobacter rodentium. Infect. Immun. 74, 2328–2337. doi: 10.1128/IAI.74.4.2328-2337.2006
Krzyminska, S., Koczura, R., Mokracka, J., Puton, T., and Kaznowski, A. (2010). Isolates of the Enterobacter cloacae complex induce apoptosis of human intestinal epithelial cells. Microb. Pathog. 49, 83–89. doi: 10.1016/j.micpath.2010.04.003
Kumar, R., and Ison, M. G. (2019). Opportunistic infections in transplant patients. Infect. Dis. Clin. North Am. 33, 1143–1157. doi: 10.1016/j.idc.2019.05.008
Lazaro-Diez, M., Navascues-Lejarza, T., Remuzgo-Martinez, S., Navas, J., Icardo, J. M., Acosta, F., et al. (2016). Acinetobacter baumannii and A. pittii clinical isolates lack adherence and cytotoxicity to lung epithelial cells in vitro. Microbes Infect. 18, 559–564. doi: 10.1016/j.micinf.2016.05.002
Liu, L., Chen, D., Lan, R., Hao, S., Jin, W., Sun, H., et al. (2018). Genetic diversity, multidrug resistance, and virulence of Citrobacter freundii from diarrheal patients and healthy individuals. Front. Cell. Infect. Microbiol. 8:233. doi: 10.3389/fcimb.2018.00233
Livrelli, V., De Champs, C., Di Martino, P., Darfeuille-Michaud, A., Forestier, C., and Joly, B. (1996). Adhesive properties and antibiotic resistance of Klebsiella, Enterobacter, and Serratia clinical isolates involved in nosocomial infections. J. Clin. Microbiol. 34, 1963–1969. doi: 10.1128/JCM.34.8.1963-1969.1996
Lopes, L. M., Fabbricotti, S. H., Ferreira, A. J., Kato, M. A., Michalski, J., and Scaletsky, I. C. (2005). Heterogeneity among strains of diffusely adherent Escherichia coli isolated in Brazil. J. Clin. Microbiol. 43, 1968–1972. doi: 10.1128/JCM.43.4.1968-1972.2005
Majewski, P., Wieczorek, P., Łapuć, I., Ojdana, D., Sieńko, A., Sacha, P., et al. (2017). Emergence of a multidrug-resistant Citrobacter freundii ST8 harboring an unusual VIM-4 gene cassette in Poland. Int. J. Infect. Dis. 61, 70–73. doi: 10.1016/j.ijid.2017.05.016
Mange, J. P., Stephan, R., Borel, N., Wild, P., Kim, K. S., Pospischil, A., et al. (2006). Adhesive properties of Enterobacter sakazakii to human epithelial and brain microvascular endothelial cells. BMC Microbiol. 6:58. doi: 10.1186/1471-2180-6-58
Marcoleta, A. E., Varas, M. A., Ortiz-Severin, J., Vasquez, L., Berrios-Pasten, C., Sabag, A. V., et al. (2018). Evaluating different virulence traits of Klebsiella pneumoniae using Dictyostelium discoideum and zebrafish larvae as host models. Front. Cell. Infect. Microbiol. 8:30. doi: 10.3389/fcimb.2018.00030
Mcwilliams, B. D., and Torres, A. G. (2014). EHEC adhesins. Microbiol. Spectr. 2LEHEC00032013. doi: 10.1128/microbiolspec.EHEC-0003-2013
Nataro, J. P., Deng, Y., Maneval, D. R., German, A. L., Martin, W. C., and Levine, M. M. (1992). Aggregative adherence fimbriae I of enteroaggregative Escherichia coli mediate adherence to HEp-2 cells and hemagglutination of human erythrocytes. Infect. Immun. 60, 2297–2304. doi: 10.1128/IAI.60.6.2297-2304.1992
Padilla, D., Acosta, F., Garcia, J. A., Real, F., and Vivas, J. R. (2009). Temperature influences the expression of fimbriae and flagella in Hafnia alvei strains: an immunofluorescence study. Arch. Microbiol. 191, 191–198. doi: 10.1007/s00203-008-0442-y
Perez-Perez, F. J., and Hanson, N. D. (2002). Detection of plasmid-mediated AmpC beta-lactamase genes in clinical isolates by using multiplex PCR. J. Clin. Microbiol. 40, 2153–2162. doi: 10.1128/JCM.40.6.2153-2162.2002
Ramos-Vivas, J., Chapartegui-Gonzalez, I., Fernandez-Martinez, M., Gonzalez-Rico, C., Fortun, J., Escudero, R., et al. (2019). Biofilm formation by multidrug resistant Enterobacteriaceae strains isolated from solid organ transplant recipients. Sci. Rep. 9:8928. doi: 10.1038/s41598-019-45060-y
Remuzgo-Martinez, S., Aranzamendi-Zaldunbide, M., Pilares-Ortega, L., Icardo, J. M., Acosta, F., Martinez-Martinez, L., et al. (2013). Interaction of macrophages with a cytotoxic Serratia liquefaciens human isolate. Microbes Infect. 15, 480–490. doi: 10.1016/j.micinf.2013.03.004
Remuzgo-Martinez, S., Lazaro-Diez, M., Mayer, C., Aranzamendi-Zaldumbide, M., Padilla, D., Calvo, J., et al. (2015). Biofilm formation and quorum-sensing-molecule production by clinical isolates of Serratia liquefaciens. Appl. Environ. Microbiol. 81, 3306–3315. doi: 10.1128/AEM.00088-15
Scaletsky, I. C., Pedroso, M. Z., Oliva, C. A., Carvalho, R. L., Morais, M. B., and Fagundes-Neto, U. (1999). A localized adherence-like pattern as a second pattern of adherence of classic enteropathogenic Escherichia coli to HEp-2 cells that is associated with infantile diarrhea. Infect. Immun. 67, 3410–3415. doi: 10.1128/IAI.67.7.3410-3415.1999
Venditti, C., Fortini, D., Villa, L., Vulcano, A., D'arezzo, S., Capone, A., et al. (2017). Circulation of blaKPC-3-carrying IncX3 plasmids among Citrobacter freundii isolates in an Italian Hospital. Antimicrob. Agents Chemother. 61:e00505-17. doi: 10.1128/AAC.00505-17
Keywords: Enterobacterales, Citrobacter, epithelial cells, virulence factors, bacterial adherence, transplant recipient
Citation: Ramos-Vivas J, Chapartegui-González I, Fernández-Martínez M, González-Rico C, Barrett J, Fortún J, Escudero R, Marco F, Linares L, Nieto J, Aranzamendi M, Muñoz P, Valerio M, Aguado JM, Chaves F, Gracia-Ahufinger I, Paez-Vega A, Martínez-Martínez L and Fariñas MC (2020) Adherence to Human Colon Cells by Multidrug Resistant Enterobacterales Strains Isolated From Solid Organ Transplant Recipients With a Focus on Citrobacter freundii. Front. Cell. Infect. Microbiol. 10:447. doi: 10.3389/fcimb.2020.00447
Received: 08 May 2020; Accepted: 21 July 2020;
Published: 16 September 2020.
Edited by:
Dongsheng Zhou, Beijing Institute of Microbiology and Epidemiology, ChinaReviewed by:
Olivier Disson, Institut Pasteur, FranceCopyright © 2020 Ramos-Vivas, Chapartegui-González, Fernández-Martínez, González-Rico, Barrett, Fortún, Escudero, Marco, Linares, Nieto, Aranzamendi, Muñoz, Valerio, Aguado, Chaves, Gracia-Ahufinger, Paez-Vega, Martínez-Martínez and Fariñas. This is an open-access article distributed under the terms of the Creative Commons Attribution License (CC BY). The use, distribution or reproduction in other forums is permitted, provided the original author(s) and the copyright owner(s) are credited and that the original publication in this journal is cited, in accordance with accepted academic practice. No use, distribution or reproduction is permitted which does not comply with these terms.
*Correspondence: José Ramos-Vivas, anZpdmFzQGlkaXZhbC5vcmc=; María Carmen Fariñas, bWNhcm1lbi5mYXJpbmFzQHNjc2FsdWQuZXM=
Disclaimer: All claims expressed in this article are solely those of the authors and do not necessarily represent those of their affiliated organizations, or those of the publisher, the editors and the reviewers. Any product that may be evaluated in this article or claim that may be made by its manufacturer is not guaranteed or endorsed by the publisher.
Research integrity at Frontiers
Learn more about the work of our research integrity team to safeguard the quality of each article we publish.