- 1Departamento de Ciências Biológicas, Universidade Estadual de Santa Cruz, Ilhéus, Brazil
- 2Departamento de Microbiologia, ICB – Universidade Federal de Minas Gerais, Belo Horizonte, Brazil
- 3Departamento de Genética, Ecologia e Evolução – ICB, Universidade Federal de Minas Gerais, Belo Horizonte, Brazil
- 4Programa de Pós-Graduação em Genética, Universidade Federal Do Paraná, Curitiba, Brazil
Biological control agents (BCA) are an alternative to chemical pesticides and an emerging strategy to safely eliminate plant pathogens. Trichoderma spp. are the most common fungi used as BCAs. They produce spores that are released into the air and can potentially interact with immune system of mammals. We previously showed that Trichoderma affects expression of genes encoding pattern recognition receptors (PRRs) and cytokines in mice. PRRs are involved in the recognition of microorganisms and can lead to pro-tumoral signaling. Here, we evaluated if mice injected with low doses of murine melanoma exhibited increased development of lung tumor when treated with conidia of T. stromaticum. Mice treated with T. stromaticum and inoculated with B16-F10 melanoma cells exhibited significant increase in tumor uptake (p = 0.006) and increased number of visible nodules in the lungs (p = 0.015). We also analyzed mRNA expression levels of genes encoding PRRs in lung of mice exposed to T. stromaticum and demonstrated that mice treated with T. stromaticum conidia exhibited lower expression levels of Clec7a and increased expression of Tlr4 (toll like receptor 4) compared to non-treated controls. The expression levels of Clec7a and Tlr2 were increased in mice treated with T. stromaticum and inoculated with murine melanoma compared to controls only inoculated with melanoma. Our results demonstrate that intranasal exposition to T. stromaticum increases tumor in the B16-F10 model, which may raise concerns regarding the safety of its use in agriculture.
Introduction
Biological control agents (BCAs) are an alternative to chemical pesticides for enhanced agricultural production (Verma et al., 2007). BCAs are composed of antagonistic microorganisms such as fungi or bacteria, which act against plant pathogens by lowering density of the phytopathogens in the field (Chet and Inbar, 1994; Syed Ab Rahman et al., 2018).
Trichoderma spp. are the most common antagonistic microorganism used as BCAs, comprising 60% of BCA used worldwide (Verma et al., 2007). The species T. harzianum, T. koningii, T. longibrachiatum, T. asperelloides and T. stromaticum are widely used worldwide. In Brazil, T. stromaticum is extensively applied to eliminate the witches' broom disease (Moniliophthora perniciosa) in Cacao (Theobroma cacao) plantations (Medeiros et al., 2010) under the comercial name Tricovab®. Although most Trichoderma fungi are non-pathogenic, they may represent potential risk to human health and cause opportunistic infections, particularly in immunocompromised and immunosuppressed individuals (Pomella et al., 2007; Lagrange-Xélota et al., 2008; Eduard, 2009; Sautour et al., 2018). T. longibrachiatum is the most commonly associated with opportunistic and invasive mycosis in humans, including peritonitis, lung and disseminated infections (Druzhinina et al., 2008).
Our research group has been focusing on the interaction between these fungi and the mammal immune system. We have previously demonstrated that exposition to conidia (asexually produced spores) of T. asperelloides and T. stromaticum by different routes can downregulate the immune system of mice. More specifically, we have shown their impact in peripheral blood, including inhibition of phagocytic ability in macrophages and changes in mRNA expression of genes encoding cytokines (Alves-Filho et al., 2011; dos Santos et al., 2017). In addition, we demonstrated that exposition to the conidia decreased the expression of genes encoding the pattern recognition receptors (PRRs) dectin-1, dectin-2, TLR (toll-like receptor) 2 and TLR4, which are critical for microbial recognition and pro-inflammatory response (Alves-Filho et al., 2011; dos Santos et al., 2017).
The effectiveness of the immune system is essential to control tumor growth, avoid the spread of cancer cells and the occurrence of metastasis (Erpenbeck et al., 2010; Eruslanov et al., 2014; Carpinteiro et al., 2015; Swierczak et al., 2015; Dasgupta et al., 2017). When tumor cells initially colonize the lung, a pro-inflammatory response induces differentiation of classically activated macrophages (M1) and type 1 T helper (Th1) cells, as well as activate T CD8+ response to control tumor growth (Altorki et al., 2019). However, immunosuppressed individuals are uncapable of building a robust immune response against tumor and other diseases, and exhibit higher risks of developing opportunistic infections and tumor progression (Carbone et al., 2014; Dropulic and Lederman, 2016; Manyam et al., 2017).
Several studies have demonstrated that toll-like receptors and others PRRs can affect tumor development and progression (Lowe et al., 2010; Zhang et al., 2011; Chiba et al., 2014; Dajon et al., 2019). Moreover, there is poor understanding of the real impact of exposition to BCAs for human health and their impact on the expression of genes encoding PRRs. We hypothesized that exposition to T. stromaticum can increase tumor growth and metastases and this could be partially explained by its impact on PPRs. We investigated the effect of intranasal exposition to T. stromaticum conidia in tumor development using a mouse model of pulmonary metastasis. Our results impressively show that T. stromaticum conidia significantly increase the risk of lung cancer in mice injected with metastatic melanoma cells.
Materials and Methods
Ethics Statement
C57BL/6 female mice (9–12 weeks) were acquired from Universidade Federal de Minas Gerais Animal Research Facility, maintained in specific pathogen-free conditions, with 12 h light/dark cycles receiving water and food ad libitum. The experiments with animals were conducted according to institutional guidelines for animal ethics and were approved by institutional animal care and use committees of both Universidade Estadual de Santa Cruz and Universidade Federal de Minas Gerais, under approval numbers 020/18 and 285/2018, respectively.
Culture of Trichoderma stromaticum Conidia and Inoculation
Trichoderma stromaticum was obtained from Tricovab® (Ceplac, Brazil accession #Ts3550), a biofungicide developed by the Brazilian government and commercially available for the control of Moniliophthora pernicious in cocoa plantations (Pomella et al., 2007). Trichoderma stromaticum was cultivated on potato dextrose agar (PDA) in Petri dishes at 28°C in the dark until observation of conidia (7–15 days). After sporulation, conidia were collected using 3 mL of sterile phosphate buffered saline 1 × (PBS) and the suspension of conidia was washed three times with PBS at 2200 × g for 10 min at 12°C. The spore concentration was calculated by using Neubauer chamber. For animal inoculation 1 × 105 conidia were suspended in 20 μL of PBS and inoculated by intranasal route (i.n.) once per week.
Tumor Cell Culture and Inoculation
B16-F10 cells (murine melanoma, ATCC CRL-6475) were cultured in Dulbecco's modified eagle's medium—DMEM (Gibco) supplemented with 10% of fetal bovine serum (Gibco), penicillin (100 U/mL), streptomycin (10 mg/mL), and L-glutamine (2 mM/mL) and cultured in a humidified incubator at 37°C with 5% of CO2 saturation. For the experimental metastasis model, B16-F10 cells (5 × 104 cells) were suspended in 100 μL of PBS and injected intravenously (i.v.) into the lateral tail vein. The same cell preparation was used in all experiments.
Experimental Design
The animals were divided into experimental groups with 5 to 6 mice per group. A first group of mice were intranasally treated only with T. stromaticum conidia (1 × 105 conidia) once per week starting at day 0 for 4 weeks. A second group was injected only with a low dose of B16-F10 tumor cells (5 × 104 cells) intravenously at day 9. For comparison, the control group did not receive any treatment.
To evaluate if a previous or posterior exposition to T. stromaticum conidia can be a risk factor and impact tumor development, two different protocols were designed. In protocol 1, a group of mice were treated with conidia (1 × 105 conidia) once per week starting at day 0 during 4 weeks, and injected with B16-F10 cells (5 × 104 cells) at day 9; for comparison a control group was treated only with PBS once per week (instead of conidia) and injected with B16-F10 cells at day 9. In protocol 2, a group of mice were injected with B16-F10 cells (5 × 104 cells) at day 9 and treated with conidia (1 x 105 conidia) once per week starting at day 10 during 3 weeks; for comparison a control group was injected with B16-F10 cells at day 9 and treated only with PBS one per week (instead of conidia).
The body weight was assessed every 2 days, from day 0 to day 24. At day 24 (15 days post tumor cell inoculation), all mice were euthanized with ketamine and xylazine (100 and 10 mg/kg) by intraperitoneal route (i.p.). The lungs were harvested and data from lung weight, lung-to-body weight ratio, tumor uptake were collected, and the number of visible metastatic nodules were counted. The right lung was used for histological analysis and the left lung was used for RNA extraction.
Lung Histopathology
After euthanasia, the right lungs were collected, fixed in 10% buffered formalin, paraffin-embedded, then sectioned at 4 μm slices and stained with hematoxylin and eosin (H&E) for histopathological assessment. For tumor analyses we established a micrometastatic score as described below. The total micrometastatic score is the sum of the number of micrometastasis range from 0 to 3. The severity score is: none = 0; one to four = 1; five to eight = 2; more than eight = 3. The analysis was performed on three random fragments of each collected right lung.
qRT-PCR
Total RNA from tissue was extracted using TRIzol® reagent method (Life technologies, USA Molecular Research Center, Inc). Subsequently, RNA quantification was performed using Nanodrop® ND-2000 (Thermo Scientific-USA) and two micrograms of total RNA were retro-transcribed with ImProm-II™ Reverse Transcriptase kit (Promega) and Oligo(dT)20 primers (IDT). qPCR was performed on QuantStudio3 Real-Time PCR System and QuantStudio™ Design & Analysis Software (Applied Biosystems, Life Technologies, EUA) using GoTaq® qPCR Master Mix System (Promega). Each cDNA sample was analyzed in duplicates with a total reaction volume of 10 μL, with 2 μL of cDNA samples as template (20 ng), 5 μL de Master Mix (2X) and 0.4 μL of each primer (200 ηM). The reactions were under the following conditions: 95°C for 10 min, 40 cycles of 95°C for 15 s, and 60°C for 60 s.
Primers sequences for genes Rn18s, Clec7a, Tlr2 and Tlr4 used in this study are given in Supplementary Table 1. All primers were validated previously (dos Santos et al., 2017). Expression values were normalized to the expression of Rn18s, encoding 18S ribosomal RNA, by the comparative method according to formula 2−ΔΔCt; ΔCt corresponds to the Ct value of the target gene subtracted from the Ct of the endogenous gene, and ΔΔCt corresponds to the ΔCt value of each condition subtracted from the control group ΔCt median (Livak and Schmittgen, 2001).
Data Analysis
Statistical analysis was performed using GraphPad Prism Software version 7.0. The Kolmogorov–Smirnov test was used to test the normal distribution of the variables in all experiments before statistical analysis. The body weight change was analyzed by two-way ANOVA, followed by Bonferroni's post-test. The analysis between two groups was assessed using Mann-Whitney test for visible nodules and micrometastatic score, chi-square test for tumor uptake and Student's t-test for other analysis. Data are presented as mean ± standard error of mean (SEM) and values of p < 0.05 were considered for statistical significance.
Results
Conidia of T. stromaticum Significantly Increase Lung Tumor Uptake and Development
A group of mice was initially exposed intranasally to inoculum of T. stromaticum and a second group was injected with a low dose of B16-F10 cells (Figure 1A, experimental design). No difference was observed in body weight kinetics and lung-to-body weight ratio compared control group (Figures 1B,C).
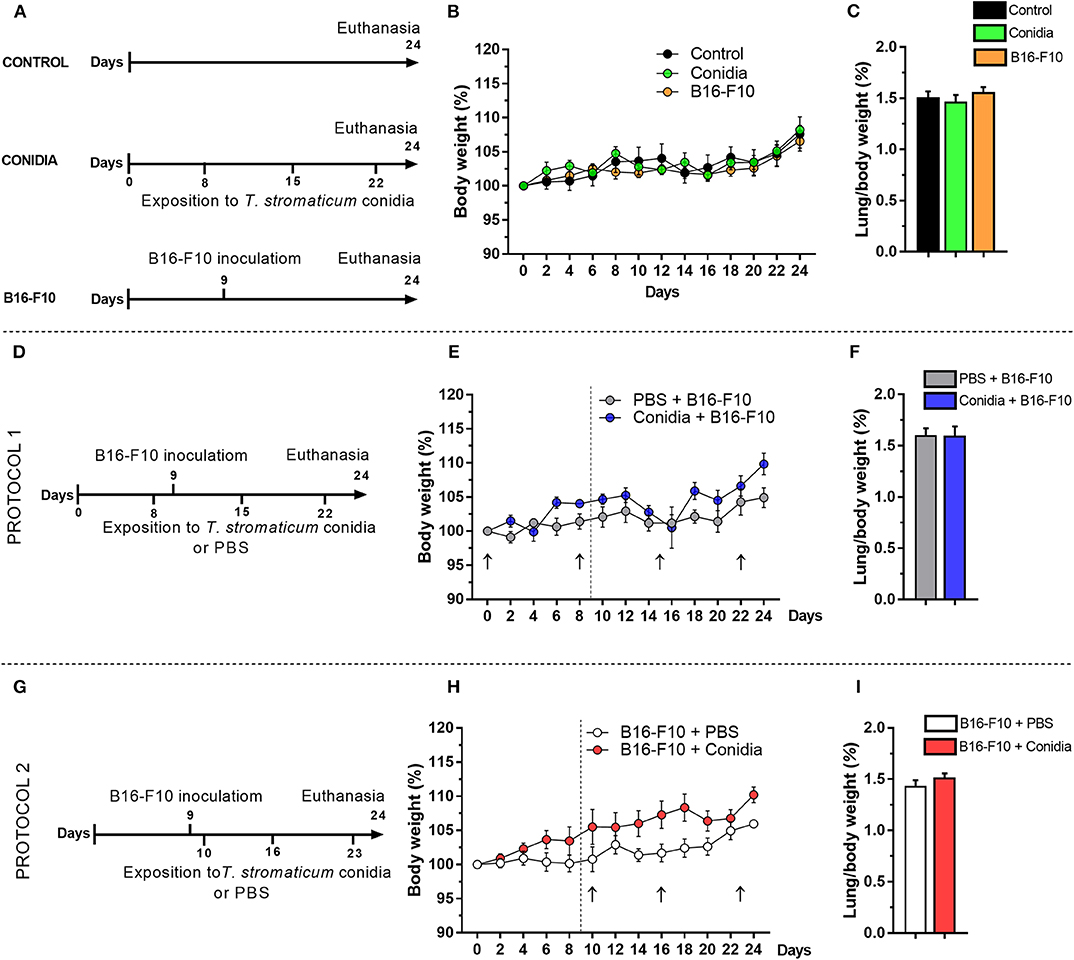
Figure 1. Effect of T. stromaticum conidia in mice from B16-F10 melanoma model. (A) Experimental design from groups conidia, B16-F10 and a control group (n = 6 mice per group); numbers in the horizontal bar indicate the days of inoculation of conidia (below) and days of tumor cell inoculation (above); (B) body weight kinetics; and (C) lung-to-body weight ratio. (D) Experimental design from Protocol 1 (n = 5–6 mice per group); (E) body weight kinetics; black arrow indicates the days of exposition to T. stromaticum conidia and dashed line the day of tumor cells inoculation; (F) lung-to-body weight ratio. (G) Experimental design from Protocol 2 (n = 5–6 mice per group); (H) body weight kinetics; black arrow indicates the days of exposition to T. stromaticum conidia and dashed line the day of tumor cells inoculation; (I) lung-to-body weight ratio. The body weight was compared between groups using Two-way ANOVA, followed by Bonferroni's post-test and Student's t-test for lung weight and lung-to-body weight ratio analysis. Data are presented as mean ± SEM. Value of p < 0.05 was considered for statistical significance.
We used the melanoma metastatic model B16-F10 to evaluate the impact of the intranasal exposition to T. stromaticum conidia on the development of murine lung cancer. Considering that humans may be occupationally exposed to conidia of BCAs before or after tumor development, we tested two different protocols in mice, being the exposition to conidia before tumor cells inoculation in protocol 1 (Figure 1D, experimental design) and after tumor cells inoculation in protocol 2 (Figure 1G, experimental design). Significant difference was not observed in body weight kinetics (Figures 1E,H) and lung-to-body weight ratio in both protocols (Figures 1F,I).
The data show that mice treated only with conidia did not exhibit macroscopic alterations in the lung compared to control. In contrast, macroscopically tumor uptake was observed by the presence of multifocal dark pigmented small areas in pleural surface of the lung of mice treated with conidia before or after B16-F10 cells injection (Figure 2A). In group B16-F10, only one animal developed lung tumor at day 15 after inoculation of melanoma cells (1/6, 16.6%; Figure 2B) with reduced visible nodules in pleural lung surface (Figure 2C). In contrast, lung tumor uptake was observed in 6/6 (100%) and 5/6 (83%) of the mice in groups treated with conidia before or after injection of B16-F10 cells, respectively (protocol 1, p = 0.006; protocol 2, p = 0.006; Figure 2B). For comparison, in mice treated with PBS, only 1/5 (20%) and 0/5 (0%) of the implanted tumors metastasized and colonized the lungs to form visible tumor until 15 days post inoculation of B16-F10 cells in protocol 1 and 2, respectively (Figure 2B). No differences were observed between the groups injected with tumor cells (B16-F10, PBS+B16-F10 from protocol 1 and B16-F10+PBS from protocol 2; Supplementary Figure S1).
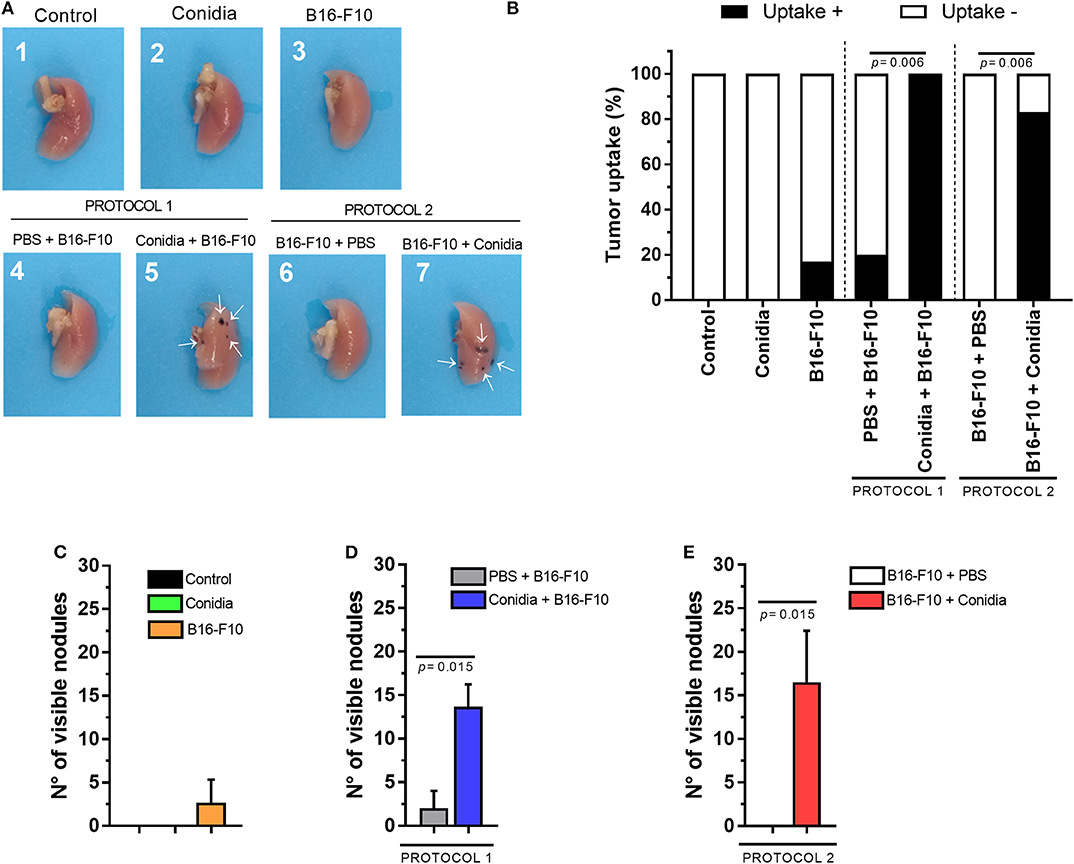
Figure 2. Conidia of T. stromaticum significantly increase lung tumor uptake and development. (A) Illustrative macroscopic image of the right lungs from mice in control group (1); inoculated only with conidia (2); only with B16-F10 (3); with PBS and B16-F10 (4); inoculated with conidia and B16-F10 cells (5); inoculated with B16-F10 cells and PBS (6); or inoculated with B16-F10 cells and conidia (7). White arrows (→) indicate the presence of tumor nodules. (B) Tumor uptake determined by the presence of tumor in the lung. Number of visible nodules count on lung surface from (C) mice treated only with conidia, only with B16-F10 cells and in control groups; in (D) Protocol 1 and (E) Protocol 2. The tumor uptake was assessed using Chi-square test and Mann-Whitney test for visible nodules. Data are presented as mean ± SEM (n = 5–6 mice per group). Value of p < 0.05 were considered for statistical significance.
We observed increased number of visible nodules in the lungs of mice from group treated with conidia before injection of B16-F10 cells (13.67 ± 2.56) compared to control (2 ± 2) in protocol 1 (p = 0.015, Figure 2D). In protocol 2, the increased count in visible nodules was also observed from 0 ± 0 to 16.5 ± 9.95 nodules in the lung of mice treated with conidia compared to control (p = 0.015; Figure 2E). We also compared tumor uptake and visible nodules count from mice in both protocols. This analysis revealed no difference between protocols, indicating that prior or posterior exposure to T. stromaticum conidia impact tumor development equally (Supplementary Figure S2). Data from tumor in experimental mouse model are presented in Supplementary Table 2.
Histopathological Characterization of Lung Tissue in B16-F10 Melanoma Model
Mice treated only with melanoma cells exhibited low micrometastatic score (Figure 3A). We observed no difference in the micrometastatic score between lung of mice treated with conidia in protocol 1 (p = 0.245, Figure 3B) and in protocol 2 (p = 0.061, Figure 3C) compared to control. No differences between the controls or between protocols 1 and 2 for micrometastatic score were observed (Supplementary Figure S3).
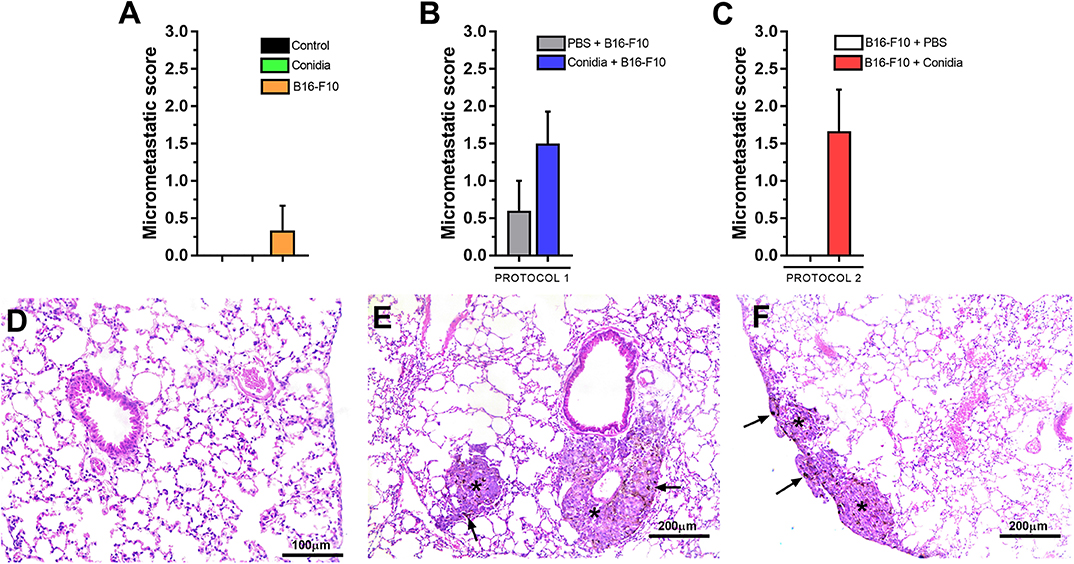
Figure 3. Histopathological analysis of mice in experimental model. (A) Micrometastatic score from mice treated with conidia, B16-F10 and control; (B) in protocol 1 and (C) protocol 2. (D) Photomicrograph of lung from mice challenged only with conidia. (E) Representative photomicrograph of early pulmonary micrometastasis in the subpleural and (F) periarteriolar area of the lung parenchyma from mice challenged with conidia and B16-F10. Asterisk (*) indicate micrometastatic foci and black arrow (→) indicate the presence of melanin in micrometastatic foci. Paraformaldehyde-fixed, paraffin-embedded sections were stained with hematoxylin & eosin (H&E). The micrometastatic score was assessed by Mann-Whitney test. Data are presented as mean ± SEM (n = 5–6 mice per group). Value of p < 0.05 were considered for statistical significance.
We observed presence of tumor cell embolism in small vessels in mice with micrometastasis. Although no inflammatory infiltrate or endothelial reactivity was present, we observed presence of rare macrophages and neutrophils around micrometastatic foci. Representative photomicrographs of lung tissue from mice treated only with conidia, with absence of inflammatory infiltrate or histological alteration in lung parenchyma are shown in Figure 3D. Early pulmonary micrometastasis was found in the subpleural (Figure 3E) and periarteriolar (Figure 3F) areas of the lung parenchyma in mice treated with conidia and B16-F10 cells.
Expression Levels of Pattern Recognition Receptors in Lung Are Affected by T. stromaticum Conidia
To test the expression of genes for PRRs in our model, we analyzed expression of genes encoding dectin-1 (Clec7a) and toll-like receptors (Tlr2, Tlr4) in lung tissue of mice exposed to T. stromaticum conidia in comparison to those not exposed. Mice treated only with T. stromaticum conidia presented lower mRNA expression levels of Clec7a (5.1-fold, p = 0.002) and increased expression of Tlr4 (3-fold, p = 0.048; Figure 4A). In contrast, we observed no differences for Tlr2 (Figure 4A).
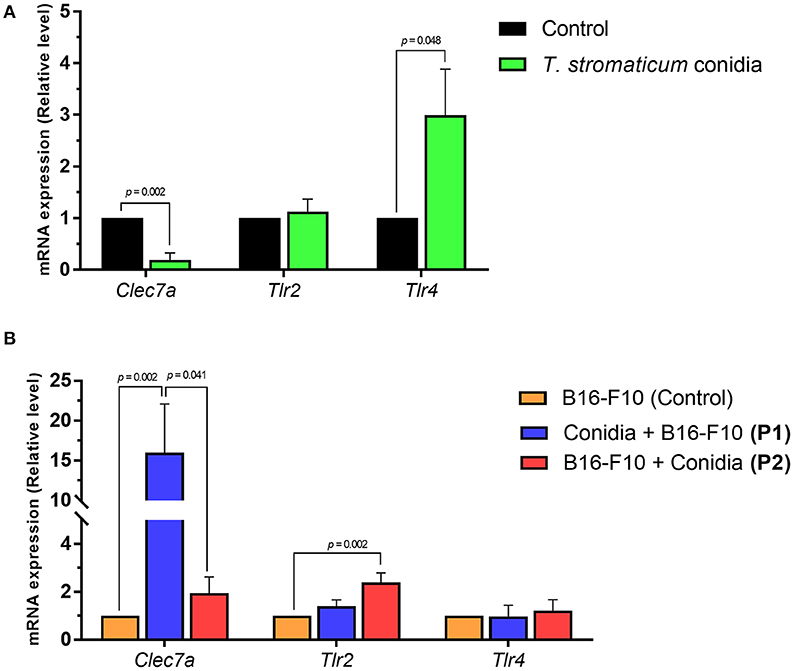
Figure 4. Expression levels of pattern recognition receptors. Abundance of mRNA transcripts of genes Clec7a, Tlr2 and Tlr4 from lungs tissue of mice. (A) Control and treated with T. stromaticum conidia. (B) Mice from protocols 1 (conidia + B16-F10) and protocol 2 (B16-F10 + Conidia) compared to control group only treated with B16-F10. Analyzed with Mann–Whitney test. Data are presented as mean ± SEM (n = 5–6 mice per group). Value of p < 0.05 were considered for statistical significance.
We observed higher Clec7a mRNA expression levels in mice from protocol 1 compared B16-F10 (control) group (16-fold, p = 0.002), but no significant difference was observed for mice treated in protocol 2 compared to control (Figure 4B). Mice from protocol 2 exhibted increased mRNA levels of Tlr2 compared to control group (2.4-fold, p = 0.002; Figure 4B). No differences were observed for Tlr4 between the compared groups.
Discussion
Previous studies have shown that chemical pesticides represent environmental risk and cause deleterious consequences for neurodevelopmental toxicity, immunotoxicity, respiratory diseases, and different types of cancer (Sanborn et al., 2002; Bonner et al., 2016; Mostafalou and Abdollahi, 2017; Daam et al., 2019). In contrast, BCAs are regarded as a safer alternative (Wang et al., 2004; Syed Ab Rahman et al., 2018) and have been successfully used worldwide (Pomella et al., 2007; Verma et al., 2007). However, the fact that the microorganisms used as BCAs may also interact with organisms other than those targeted for the biocontrol points to a potential risk, especially for those individuals who may be chronically exposed. So far, studies to understand the consequences of using BCAs to human health are scarce.
Here, we used the wild type mouse strain C57BL/6 to provide better understanding of the impact of exposition to T. stromaticum conidia in tumor development in the well-known murine B16 melanoma model (Overwijk and Restifo, 2000; Hwang et al., 2013; Foerster et al., 2018). Our results show that intranasal exposition to conidia of T. stromaticum increases tumor in our animal model. Disturbance of the mammalian respiratory system as well as occurrence of opportunistic infections caused by BCAs have been previously described, including for Trichoderma (Loeppky et al., 1983; Halpin et al., 1994; Madsen et al., 2007; Sautour et al., 2018). Human infections by Trichoderma were described in patients with pulmonary cancer, pericarditis, peritonitis, keratitis, leukemia, endocarditis, and others (Jaakkola et al., 2002; Kredics et al., 2003; Lagrange-Xélota et al., 2008; Tascini et al., 2016; Recio et al., 2019). In many cases, complications of these infections caused death (Jacobs et al., 1992; Kredics et al., 2003; Sandoval-Denis et al., 2014). Because many Trichoderma species are cosmopolitan soil-borne, agriculture is considered a potential source of emerging human infection by Trichoderma in immunocompromised and immunosuppressed individuals (Sandoval-Denis et al., 2014; Hatvani et al., 2019). However, our study is the first to demonstrate the implications of Trichoderma in cancer.
We observed that mice inoculated with a low dose of metastatic cells and treated intranasally with T. stromaticum conidia exhibited increased tumor uptake and increased number of visible lung tumors compared to controls only treated with metastatic cells, with presence of micrometastatic foci in lung tissue. In both protocols, mice exhibited increased risk of developing lung cancer when exposed to T. stromaticum conidia. Although there are reports of Trichoderma infections in cancer patients (Lagrange-Xélota et al., 2008; Sautour et al., 2018), there are no studies associating these infections with prognosis and/or course of disease. Different from chemical pesticides, for which type and duration of exposition are directly related to the risk for human health and the risk is lower for non-occupational exposure (Sabarwal et al., 2018), these assumptions cannot be extended to BCA due to the scarcity of studies and lack of direct demonstration.
In our study, we applied intranasally 105 conidia in mice to evaluate their effect on lung tumor, however, the normal exposure levels to fungal conidia depends on the environment. Indoors, the levels rarely exceed 104 conidia/m3, while outdoors, the levels are normally below to 105 conidia/m3. In contrast, the levels in workplaces are normally higher and easily exceed 106 conidia/m3 (Eduard, 2009). A previous study with immunosuppressed mice showed that exposition to higher amounts of T. longibrachiatum conidia (107 CFU/animal) caused death in all treated animals, in contrast to lower exposition levels (104 or 105 CFU/animal) (Paredes et al., 2016). In contrast, we only studied immunocompetent mice and applied a low dose of T. stromaticum conidia (105 conidia/animal). When treated only with T. stromaticum, all animals in our study did not show any alterations in body weight or lung-to-body weight ratio after 24 days, and also did not exhibit any histopathological alteration in the lungs. Similarly, in mice that were only treated with the low dose of melanoma, only 1 out 6 developed lung cancer. Interestingly, the concomitant presence of T. stromaticum conidia and B16-F10 cells significantly increased the development of lung cancer (6 out 6). Our results cannot reveal the mechanisms that explain why T. stromaticum increases tumor in individuals injected with a low dose of murine melanoma. It is also not clear if inhaled conidia will impact tumor as strongly as intranasal injection. However, our data suggest that presence of T. stromaticum results in a poorer immune response against cancer cells in mice challenged with the melanoma injection.
To date, only few studies analyzed the interaction between these fungi and mammalian immune system cells (Alves-Filho et al., 2011; Dos Santos et al., 201; Konstantinovas et al., 2017). Results from our group demonstrated that Trichoderma spp. reduces the mRNA expression of genes encoding toll-like receptor and c-type lectin receptor (CLR) in mice (Alves-Filho et al., 2011; dos Santos et al., 2017). TLR and CLR recognize microorganisms and tumor cells; they are also expressed on malignant cells, and their stimulation and/or inhibition are implicated in tumor development and metastasis (Chiba et al., 2014; Dajon et al., 2017, 2019). To provide insights of how Trichoderma may affect cancer, we investigated the gene expression levels of pattern recognition receptors involved in anti-fungal and anti-tumor immune responses in lung of the mice used in our experiments.
Analyzing RNA isolated from lung, we show that mRNA levels of Clec7a was 5.1 times lower in mice exposed to T. stromaticum conidia. Clec7a encodes the receptor dectin-1, which is expressed on the surface of dendritic cells and macrophages. Dectin-1 recognizes N-glycan structures from tumor cells, such as in B16 lineage, and activates IRF5 (interferon regulatory factor 5) pathway, among others, to activate natural killer cells to effectively eliminate neoplastic cells (Chiba et al., 2014). Our results also show that Clec7a levels were 16 times higher in mice treated with both melanoma and T. stromaticum in comparison to treated with only melanoma. It has been demonstrated that dectin-1 signaling in macrophages was associated with pancreatic carcinoma and peritumoral immune-tolerance by suppression of T cell immunogenicity (Daley et al., 2017). The altered expression of Clec7a in lung tissue of mice treated with T. stromaticum conidia allows us to speculate that dectin-1 may be involved in the increased tumor uptake and increased number of visible nodules in our model through mechanisms that are still not clear. A more modest increase (2.4-fold) was observed for Tlr2 in protocol 2. It was reported that lung cancer patients exhibit higher serum levels of TLR (Zhang et al., 2020). In mice, carcinoma induces activation of myeloid cells via TLR2 to stimulate lung metastasis with expression of TNF-α (Kim et al., 2009). TLR2 can also activate the transcription factor Stat3, and trigger immunosuppressive response in B16-bearing mice (Yang et al., 2009).
In conclusion, our results demonstrate that intranasal exposition to T. stromaticum conidia increases the development of lung cancer in mice injected with murine melanoma, which could be related to altered expression of genes encoding PRRs. It is important to notice that intranasal injection of conidia is not the way that humans and other mammals are naturally exposed to BCAs, and our results do not allow the conclusion that inhaling T. stromaticum conidia from the air will cause cancer. However, despite the fact that our observations do not imply that the use of this BCA is not safe, its association with increased tumor in our animal model cannot be ignored. It is not clear whether or not T. stromaticum could disturb immune responses in high doses and/or increase the incidence of tumor in immunocompetent or those chronically exposed individuals. Therefore, further studies are necessary in order to decipher the mechanisms of interaction between these BCA and immune cells, as well to evaluate their possible implications in human health.
Data Availability Statement
All datasets generated for this study are included in the article/Supplementary Material.
Ethics Statement
The animal study was reviewed and approved by both the Universidade Estadual de Santa Cruz and Universidade Federal de Minas Gerais Institutional Animal Care and Use Committee, under protocol numbers 020/18 and 285/2018, respectively.
Author Contributions
US and JSa conceived and designed the study. US, MC, GF, and AD conducted in vivo experiments. US and FO performed cell culture. US, BS, and JSi conducted histopathological preparation and analysis. US, FO, AD, and LC performed qRT-PCR. US, MC, AD, JSi, DA, JSa, and LC analyzed data. JSa, DS, and AD provided reagents. US, DA, MC, JSa, AD, and JSi drafted the manuscript. All authors contributed to manuscript revision, read and approval the submitted version.
Funding
This work was supported by the following Brazilian financing institutions: Fundação de Amparo à Pesquisa do Estado da Bahia (FAPESB) by Grants No. RED0011/2012 and RED008/2014, Coordenação de Aperfeiçoamento de Pessoal de Nível Superior (CAPES) and Fundação de Amparo à Pesquisa do Estado de Minas Gerais (FAPEMIG), Conselho Nacional de Desenvolvimento Científico e Tecnológico (CNPq), Grant No. 423045/2018-0.
Conflict of Interest
The authors declare that the research was conducted in the absence of any commercial or financial relationships that could be construed as a potential conflict of interest.
Acknowledgments
US and FO thank the scholarship granted by Coordenação de Aperfeiçoamento de Pessoal de Nível Superior (CAPES). Special thanks to Acácia Lippi for support.
Supplementary Material
The Supplementary Material for this article can be found online at: https://www.frontiersin.org/articles/10.3389/fcimb.2020.00252/full#supplementary-material
References
Altorki, N. K., Markowitz, G. J., Gao, D., Port, J. L., Saxena, A., Stiles, B., et al. (2019). The lung microenvironment: an important regulator of tumour growth and metastasis. Nat. Rev. Cancer 19, 9–31. doi: 10.1038/s41568-018-0081-9
Alves-Filho, E. R., Maioli, T. U., Fária, A. M. C., Noronha, F. S. M., Silva, N. M., Costa, M. G. C., et al. (2011). The biocontrol fungus trichoderma stromaticum downregulates respiratory burst and nitric oxide in phagocytes and IFN-gamma and IL-10. J. Toxicol. Environ. Health A 74, 943–958. doi: 10.1080/15287394.2011.573747
Bonner, M. R., Hines, C. J., Sandler, D. P., Lynch, C. F., Thomas, K., Hoppin, J. A., et al. (2016). Occupational exposure to pesticides and the incidence of lung cancer in the agricultural health study. Environ. Health Perspect. 125, 544–551. doi: 10.1289/EHP456
Carbone, A., Vaccher, E., Gloghini, A., Pantanowitz, L., Abayomi, A., De Paoli, P., et al. (2014). Diagnosis and management of lymphomas and other cancers in HIV-infected patients. Nat. Rev. Clin. Oncol. 11, 223–238. doi: 10.1038/nrclinonc.2014.31
Carpinteiro, A., Becker, K. A., Japtok, L., Hessler, G., Keitsch, S., Pozgajova, M., et al. (2015). Regulation of hematogenous tumor metastasis by acid sphingomyelinase. EMBO Mol. Med. 7, 714–734. doi: 10.15252/emmm.201404571
Chet, I., and Inbar, J. (1994). Biological control of fungal pathogens. Appl. Biochem. Biotechnol. 48, 37–43. doi: 10.1007/BF02825358
Chiba, S., Ikushima, H., Ueki, H., Yanai, H., Kimura, Y., Hangai, S., et al. (2014). Recognition of tumor cells by dectin-1 orchestrates innate immune cells for anti-tumor responses. Elife 3:e04177. doi: 10.7554/eLife.04177
Daam, M. A., Chelinho, S., Niemeyer, J. C., Owojori, O. J., De Silva, P. M. C. S., Sousa, J. P., et al. (2019). Environmental risk assessment of pesticides in tropical terrestrial ecosystems: test procedures, current status and future perspectives. Ecotoxicol. Environ. Saf. 181, 534–547. doi: 10.1016/j.ecoenv.2019.06.038
Dajon, M., Iribarren, K., and Cremer, I. (2017). Toll-like receptor stimulation in cancer: a pro- and anti-tumor double-edged sword. Immunobiology 222, 89–100. doi: 10.1016/j.imbio.2016.06.009
Dajon, M., Iribarren, K., Petitprez, F., Marmier, S., Lupo, A., Gillard, M., et al. (2019). Toll like receptor 7 expressed by malignant cells promotes tumor progression and metastasis through the recruitment of myeloid derived suppressor cells. Oncoimmunology 8:e1505174. doi: 10.1080/2162402X.2018.1505174
Daley, D., Mani, V. R., Mohan, N., Akkad, N., Ochi, A., Heindel, D. W., et al. (2017). Dectin 1 activation on macrophages by galectin 9 promotes pancreatic carcinoma and peritumoral immune tolerance. Nat. Med. 23, 556–567. doi: 10.1038/nm.4314
Dasgupta, A., Lim, A. R., and Ghajar, C. M. (2017). Circulating and disseminated tumor cells: harbingers or initiators of metastasis? Mol. Oncol. 11, 40–61. doi: 10.1002/1878-0261.12022
dos Santos, A. G., Mendes, É. A., de Oliveira, R. P., Faria, A. M. C., de Sousa, A. O., Pirovani, C. P., et al. (2017). Trichoderma asperelloides spores downregulate dectin1/2 and TLR2 receptors of mice macrophages and decrease candida parapsilosis phagocytosis independent of the M1/M2 polarization. Front. Microbiol. 8:1681. doi: 10.3389/fmicb.2017.01681
Dropulic, L. K., and Lederman, H. M. (2016). Overview of infections in the immunocompromised host. Microbiol. Spectr. 4, 1–43. doi: 10.1128/microbiolspec.DMIH2-0026-2016
Druzhinina, I. S., Komon-Zelazowska, M., Kredics, L., Hatvani, L., Antal, Z., Belayneh, T., et al. (2008). Alternative reproductive strategies of Hypocracea orientalis and genetically close but clonal Trichoderma longibrachiatum, both capable of causing invasive mycosis of humans. Microbiology 154, 3447–3459. doi: 10.1099/mic.0.2008/021196-0
Eduard, W. (2009). Fungal spores : a critical review of the toxicological and epidemiological evidence as a basis for occupational exposure limit setting. Clin. Rev. Toxicol. 39,799–864. doi: 10.3109/10408440903307333
Erpenbeck, L., Nieswandt, B., Schön, M., Pozgajova, M., and Schön, M. P. (2010). Inhibition of platelet GPIbα and promotion of melanoma metastasis. J. Invest. Dermatol. 130, 576–586. doi: 10.1038/jid.2009.278
Eruslanov, E. B., Bhojnagarwala, P. S., Quatromoni, J. G., Stephen, T. L., Ranganathan, A., Deshpande, C., et al. (2014). Tumor-associated neutrophils stimulate T cell responses in eraly-stage human lung cancer. J. Clin. Invest. 124, 5466–5480. doi: 10.1172/JCI77053
Foerster, F., Boegel, S., Heck, R., Pickert, G., Rüssel, N., Rosigkeit, S., et al. (2018). Enhanced protection of C57 BL/6 vs Balb/c mice to melanoma liver metastasis is mediated by NK cells. Oncoimmunology 7:e1409929. doi: 10.1080/2162402X.2017.1409929
Halpin, D. M. G., Graneek, B. J., Turner-Warwick, M., and Taylor, A. J. N. (1994). Extrinsic allergic alveolitis and asthma in a sawmill worker: case report and review of the literature. Occup. Environ. Med. 51, 160–164. doi: 10.1136/oem.51.3.160
Hatvani, L., Homa, M., Chenthamara, K., Cai, F., Kocsubé, S., Atanasova, L., et al. (2019). Agricultural systems as potential sources of emerging human mycoses caused by Trichoderma: a successful, common phylotype of Trichoderma longibrachiatum in the frontline. FEMS Microbiol Lett. 366:fnz246. doi: 10.1093/femsle/fnz246
Hwang, S. H., Lee, B. H., Kim, H. J., Cho, H. J., Shin, H. C., Im, K. S., et al. (2013). Suppression of metastasis of intravenously-inoculated B16/F10 melanoma cells by the novel ginseng-derived ingredient, gintonin: involvement of autotaxin inhibition. Int. J. Oncol. 42, 317–326. doi: 10.3892/ijo.2012.1709
Jaakkola, M. S., Laitinen, S., Piipari, R., Uitti, J., Nordman, H., Haapala, A-M., et al. (2002). Immunoglobulin G antibodies against indoor dampness-related microbes and adult-onset asthma: a population-based incident case-control study. Clin. Exp. Immunol. 129, 107–112. doi: 10.1046/j.1365-2249.2002.01884.x
Jacobs, F., Byl, B., Bourgeois, N., Coremans-Pelseneer, J., Florquin, S., Depré, G., et al. (1992). Trichoderma viride infection in a liver transplant recipient. Mycoses, 35, 301–303. doi: 10.1111/j.1439-0507.1992.tb00880.x
Kim, S., Takahashi, H., Lin, W., Descargues, P., Grivennikov, S., Kim, Y., et al. (2009). Carcinoma-produced factors activate myeloid cells through TLR2 to stimulate metastasis. Nature 457, 102–106. doi: 10.1038/nature07623
Konstantinovas, C., de Oliveira Mendes, T. A., Vannier-Santos, M. A., and Lima-Santos, J. (2017). Modulation of human immune response by fungal biocontrol agents. Front. Microbiol. 8:39. doi: 10.3389/fmicb.2017.00039
Kredics, L., Antal, Z., Dóczi, I., Mangzinger, L., Kevei, F., and Nagy, E. (2003). Clinical importance of the genus Trichoderma - a review. Acta Microbiol. Immunol. Hung. 50, 105–117. doi: 10.1556/AMicr.50.2003.2-3.1
Lagrange-Xélota, M., Schlemmera, F., Galliena, S., Lacroixb, C., and Molinaa, J. M. (2008). Trichoderma fungaemia in a neutropenic patient with pulmonary cancer and human immunodeficiency virus infection. Clin. Microbiol. Infect. 14, 1190–1192. doi: 10.1111/j.1469-0691.2008.02111.x
Livak, K. J., and Schmittgen, T. D. (2001). Analysis of relative gene expression data using real-time quantitative PCR and the 2(-Delta Delta C(T)) method. Methods 25, 402–408. doi: 10.1006/meth.2001.1262
Loeppky, C. B., Sprouse, R. F., Carlson, J. V., and Everett, E. D. (1983). Trichoderma viride peritonitis. South Med. J. 76, 798–799. doi: 10.1097/00007611-198306000-00029
Lowe, E. L., Crother, T. R., Rabizadeh, S., Hu, B., Wang, H., Chen, S., et al. (2010). Toll-like receptor 2 signaling protects mice from tumor development in a mouse model of colitis-induced cancer. PLoS ONE 5:e13027. doi: 10.1371/journal.pone.0013027
Madsen, A. M., Hansen, V. M., Meyling, N. V., and Eilenberg, J. (2007). Human exposure to airborne fungi from genera used as biocontrol agents in plant production. Ann. Agric. Environ. Med. 14, 5–24.
Manyam, B. V., Garsa, A. A., Chin, R. I., Reddy, C. A., Gastman, B., Thorstad, W., et al. (2017). A multi-institutional comparison of outcomes of immunosuppressed and immunocompetent patients treated with surgery and radiation therapy for cutaneous squamous cell carcinoma of the head and neck. Cancer 123, 2054–2060. doi: 10.1002/cncr.30601
Medeiros, F. H. V., Pomella, A. W. V., de Souza, J. T., Niella, G. R., Valle, R., Bateman, R. P., et al. (2010). A novel, integrated method for management of witches' broom disease in Cacao in Bahia, Brazil. Crop Prot. 29, 704–711. doi: 10.1016/j.cropro.2010.02.006
Mostafalou, S., and Abdollahi, M. (2017). Pesticides: an update of human exposure and toxicity. Arch. Toxicol. 91, 549–599. doi: 10.1007/s00204-016-1849-x
Overwijk, W. W., and Restifo, N. P. (2000). B16 as a mouse model for human melanoma. Curr. Protoc. Immunol. 39, 20.1.1–20.1.29. doi: 10.1002/0471142735.im2001s39
Paredes, K., Capilla, J., Mayayo, E., and Guarro, J. (2016). Virulence and experimental treatment of Trichoderma longibrachiatum, a fungus refractory of treatment. Antimicrobial. Agents Chemother. 60, 5029–5032. doi: 10.1128/AAC.00373-16
Pomella, A. W. V., De Souza, J. T., Niella, G. R., Bateman, R., Hebbar, P., Loguercio, L. L., et al. (2007). “The use of Trichoderma stromaticum in the management of witche's broom disease of cacao in Bahia State, Brazil,” in Biolofical Control: A Global Perspective – Case Studies from Around the World, eds C. Vinvent, M. Gottel, and G. Lazarovits (Wallingford: CABI Publishing), 210–7. doi: 10.1079/9781845932657.0210
Recio, R., Meléndez-Carmona, M. Á., Martín-Higuera, M. C., Pérez, V., López, E., López-Medrano, F., et al. (2019). Trichoderma longibrachiatum: na ununsual pathogen of fungal pericarditis. Clin. Microbiol. Infect. 25, 586–587. doi: 10.1016/j.cmi.2019.02.006
Sabarwal, A., Kumar, K., and Singh, R. P. (2018). Hazardous effects of chemical pesticides on human health–cancer and other associated disorders. Environ. Toxicol. Pharmacol. 63, 103–114. doi: 10.1016/j.etap.2018.08.018
Sanborn, M. D., Cole, D., Abelsohn, A., and Weir, E. (2002). Identifying and managing adverse environmental health effects: 4. pesticides. CMAJ 166, 1431–1436.
Sandoval-Denis, M., Sutton, D. A., Cano-Lira, J., Gené, J., Fothergill, A. W., Wiederhold, N. P., et al. (2014). Phylogenic of the clinically relevant species of the emerging fungus trichoderma and their antifungal susceptibilities. J. Clin. Microbiol. 52, 2112–2125. doi: 10.1128/JCM.00429-14
Sautour, M., Chrétien, M. L., Valot, S., Lafon, I., Basmaciyan, L., Legouge, C., et al. (2018). First case of proven invasive pulmonary infection due to Trichoderma longibrachiatum in a neutropenic patient with acute leukemia. J. Mycol. Med. 28, 659–662. doi: 10.1016/j.mycmed.2018.10.001
Swierczak, A., Mouchemore, K. A., Hamilton, J. A., and Anderson, R. L. (2015). Neutrophils: important contributors to tumor progression and metastasis. Cancer Metastasis Rev. 34, 735–751. doi: 10.1007/s10555-015-9594-9
Syed Ab Rahman, S. F., Singh, E., Pieterse, C. M. J., and Schenk, P. M. (2018). Emerging microbial biocontrol strategies for plant pathogens. Plant Sci. 267, 102–111. doi: 10.1016/j.plantsci.2017.11.012
Tascini, C., Cardinali, F., Barletta, V., Di Paolo, A., Leonildi, A., Zucchelli, G., et al. (2016). First case of Trichoderma longibrachiatum CIED (Cardiac Implantable Electronic Device)-associated endocarditis in a non-immunocompromised host: biofilm removal and diagnostic problems in the light of the current literature. Mycopathologia. 181, 297–303. doi: 10.1007/s11046-015-9961-7
Verma, M., Brar, S. K., Tyagi, R. D., Surampalli, R. Y., and Valéro, J. R. (2007). Antagonistic fungi, trichoderma spp.: panoply of biological control. Biochem. Eng. J. 37, 1–20. doi: 10.1016/j.bej.2007.05.012
Wang, C., Fan, M., Li, Z., and Butt, T. M. (2004). Molecular monitoring and evaluation of the application of the insect-pathogenic fungus beauveria bassiana in southeast China. J. Appl. Microbiol. 96, 861–870. doi: 10.1111/j.1365-2672.2004.02215.x
Yang, H.-Z., Cui, B., Liu, H.-Z., Mi, S., Yan, J., Yan, H.-M., et al. (2009). Blocking TLR2 activity attenuates pulmonary metastases of tumor. PLoS ONE 4:e6520. doi: 10.1371/journal.pone.0006520
Zhang, M., Zhou, Y., and Zhang, Y. (2020). High expression of TLR2 in the serum of patients with tuberculosis and lung cancer, and can promote the progression of lung cancer. Math. Biosci. Eng. 17, 1959–1972. doi: 10.3934/mbe.2020104
Keywords: biological control, Trichoderma, cancer, metastasis, patter recognition receptor, Tricovab
Citation: Santos UR, Costa MC, Freitas GJC, Oliveira FS, Santos BR, Silva JF, Santos DA, Dias AAM, Carvalho LD, Augusto DG and Santos JL (2020) Exposition to Biological Control Agent Trichoderma stromaticum Increases the Development of Cancer in Mice Injected With Murine Melanoma. Front. Cell. Infect. Microbiol. 10:252. doi: 10.3389/fcimb.2020.00252
Received: 11 January 2020; Accepted: 30 April 2020;
Published: 29 May 2020.
Edited by:
Jens Staal, Ghent University, BelgiumReviewed by:
Santiago Gutierrez, Universidad de León, SpainLea Atanasova, University of Natural Resources and Life Sciences Vienna, Austria
Copyright © 2020 Santos, Costa, de Freitas, de Oliveira, Santos, Silva, Santos, Dias, de Carvalho, Augusto and dos Santos. This is an open-access article distributed under the terms of the Creative Commons Attribution License (CC BY). The use, distribution or reproduction in other forums is permitted, provided the original author(s) and the copyright owner(s) are credited and that the original publication in this journal is cited, in accordance with accepted academic practice. No use, distribution or reproduction is permitted which does not comply with these terms.
*Correspondence: Jane L. dos Santos, jlsantos@uesc.br
†Present address: Danillo G. Augusto, Department of Neurology, University of California, San Francisco, San Francisco, CA, United States