- 1Department of Innate Immunity, ICMR-National Institute for Research in Reproductive Health, Mumbai, India
- 2Biosciences, College of Health and Life Sciences, Brunel University London, Uxbridge, United Kingdom
With limited therapeutic options and associated severe adverse effects, fungal infections are a serious threat to human health. Innate immune response mediated by pattern recognition proteins is integral to host defense against fungi. A soluble pattern recognition protein, Surfactant protein D (SP-D), plays an important role in immune surveillance to detect and eliminate human pathogens. SP-D exerts its immunomodulatory activity via direct interaction with several receptors on the epithelial cells lining the mucosal tracts, as well as on innate and adaptive immune cells. Being a C-type lectin, SP-D shows calcium- and sugar-dependent interactions with several glycosylated ligands present on fungal cell walls. The interactome includes cell wall polysaccharides such as 1,3-β-D-glucan, 1,6-β-D-glucan, Galactosaminogalactan Galactomannan, Glucuronoxylomannan, Mannoprotein 1, and glycosylated proteins such as gp45, gp55, major surface glycoprotein complex (gpA). Recently, binding of a recombinant fragment of human SP-D to melanin on the dormant conidia of Aspergillus fumigatus was demonstrated that was not inhibited by sugars, suggesting a likely protein-protein interaction. Interactions of the ligands on the fungal spores with the oligomeric forms of full-length SP-D resulted in formation of spore-aggregates, increased uptake by phagocytes and rapid clearance besides a direct fungicidal effect against C. albicans. Exogenous administration of SP-D showed significant therapeutic potential in murine models of allergic and invasive mycoses. Altered susceptibility of SP-D gene-deficient mice to various fungal infections emphasized relevance of SP-D as an important sentinel of anti-fungal immunity. Levels of SP-D in the serum or lung lavage were significantly altered in the murine models and patients of fungal infections and allergies. Here, we review the cell wall ligands of clinically relevant fungal pathogens and allergens that are recognized by SP-D and their impact on the host defense. Elucidation of the molecular interactions between innate immune humoral such as SP-D and fungal pathogens would facilitate the development of novel therapeutic interventions.
Introduction
Fungal infections range from asymptomatic, mild mucocutaneous infections to potentially life-threatening systemic infections. With more than a billion people with mild infections, several millions mucosal candidiasis cases and 150 million people with serious fungal diseases, fungi have a huge impact on public health (Bongomin et al., 2017). Cells of the innate immune system (e.g., dendritic cells and macrophages) bind components of fungal cell walls using surface or soluble pattern recognition receptors (PRRs). The C-type lectin receptors and the Toll-like receptors are particularly involved in anti-fungal immunity (Salazar and Brown, 2018). Surfactant protein D (SP-D), a soluble C-type lectin, recognizes several fungal ligands and inhibits their interactions with target host cells (Pandit et al., 2012; Carreto-Binaghi et al., 2016). Importantly, SP-D can activate or inhibit the pro-inflammatory signaling in the target cells via a range of activating or inhibitory receptors (Kishore et al., 2006).
Surfactant Protein D, a Soluble Collectin
The human SP-D gene is located proximal to the centromere of chromosome 10 in humans and Chromosome 14 in mouse. The primary structure of the SP-D protein comprises of four distinct regions: an N-terminal non-collagenous domain, a triple-helical collagenous region, an α-helical coiled-coil neck region, and the C-terminal carbohydrate recognition domain (CRD) (Kishore et al., 2005). This primary structural organization exists as a trimeric subunit, which can form further oligomeric units up to dodecamers. It is quite usual to visualize, under electron microscopy, fuzzy balls of SP-D proteins in lung washings.
SP-D is produced by different cell types, including type II pneumocytes, non-ciliated bronchiolar cells, submucosal gland and epithelial cells of trachea in the lung. Other sources include ductal epithelial cells in the lacrimal apparatus, mucosal and glandular/ductal epithelial cells in the gastrointestinal tract, skin, male and female genitourinary tracts (Kishore et al., 2005). Vascular endothelial cells in the heart and brain tissues also synthesize significant levels of SP-D (Sorensen et al., 2006; Schob et al., 2013). SP-D expression is considerably increased in a human corneal cell line challenged with A. fumigatus via TLR4 signaling, and corneal tissue of rats challenged with Fusarium solani (Che et al., 2012a,b; Wu et al., 2015). Such a widespread existence of SP-D in various tissues and fluids and its increased expression in response to fungal pathogens emphasizes its importance as an innate immune surveillance molecule at the mucosal barriers.
SP-D mediates immune regulation by direct interaction with multiple receptors on immune and epithelial cells, leading to altered cytokine and free radical production (Jakel et al., 2013; Sorensen, 2018) (Table 1). SP-D stimulates antigen presentation by dendritic cells but inhibits T cell proliferation. SP-D via its collagen domain, binds calreticulin/CD91 receptor complex and activates macrophages. The globular CRD domain promotes an anti-inflammatory effect through a signal inhibitory regulatory protein α (SIRPα) on macrophages (Gardai et al., 2003). Various immunomodulatory mechanisms mediated by SP-D that strengthen host defense against fungi are summarized in Figure 1.
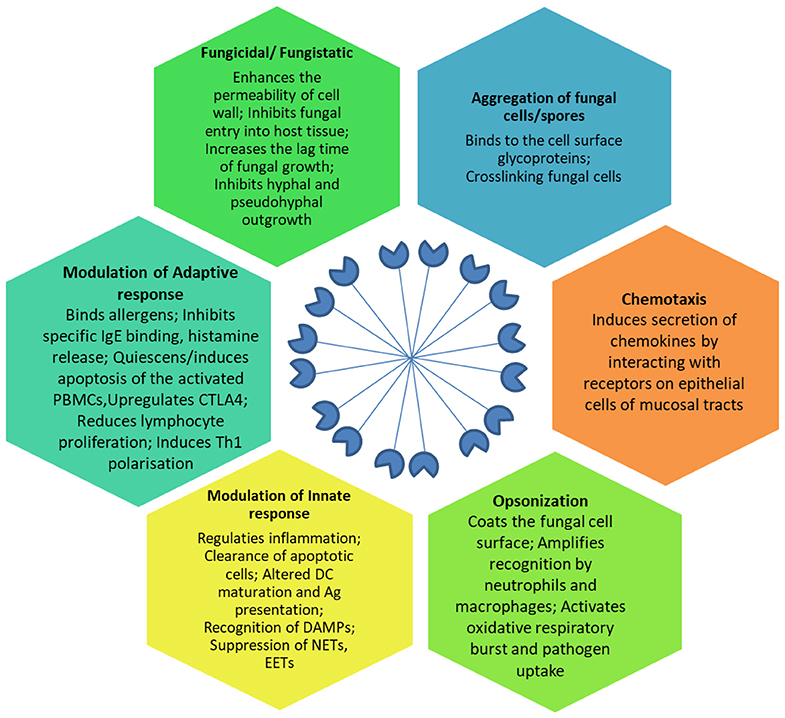
Figure 1. Effector anti-fungal mechanisms mediated by SP-D. The cartoon in the center of the figure depicts the fuzzy ball structure of SP-D formed from several dodecameric structural units. Ag, Antigen; CTLA-4, Cytotoxic T-lymphocyte Associated protein 4; DAMPs, Damage Associated Molecular Patterns; DC, Dendritic cells; EETs, Eosinophil Extracellular Traps; IgE, Immunoglobulin E antibodies; NETs, Neutrophil Extracellular Traps; PBMCs, Peripheral Blood Mononuclear Cells; Th1, T helper 1 lymphocytes.
Being a calcium-dependent lectin, SP-D binds maltose, glucose, mannose, fucose, galactose, lactose, glucosamine, and N-acetylglucosamine, and to complex carbohydrates on the surface of fungal pathogens (Pandit et al., 2012). The binding of SP-D to a variety of opportunistic fungi results in the direct inhibition of fungal growth, aggregation and enhanced phagocytosis (Pandit et al., 2012). Importantly, the downstream immune response elicited by SP-D also contributes to the reduction/elimination of fungal infection in mice (Pandit et al., 2012).
Here, we review the cell wall ligands of clinically relevant fungal pathogens and allergens that are recognized by SP-D and their impact on the host defense (Table 2). Elucidation of the interactions between SP-D and fungal ligands could lead to the development of novel therapeutic interventions for both allergic and invasive mycoses. We also provide an update on the cellular receptors of SP-D involved in immunomodulation, serum and BAL levels of SP-D, polymorphisms of SP-D, oligomeric forms of SP-D, proteolytic degradation of SP-D and a possible road map to pursue clinical applications of SP-D.
SP-D Recognizes and Strengthens Host Defense Against Aspergillus fumigatus
Aspergillus fumigatus is a prominent fungal pathogen in immunocompromised individuals, and can cause invasive aspergillosis. In the immunocompetent host, it causes allergic disorders such as allergic rhinitis, allergic sinusitis, hypersensitivity pneumonitis, and allergic bronchopulmonary Aspergillosis (ABPA).
With a series of in vitro and in vivo studies, we established that SP-D was relevant in host defense against A. fumigatus (Madan et al., 2005a). SP-D bound and agglutinated A. fumigatus conidia in a calcium-dependent manner, and enhanced uptake of opsonized conidia by the alveolar macrophages and neutrophils (Madan et al., 1997a). Rat SP-D bound the A. fumigatus conidia via CRD; EDTA, mannose, glucose, maltose, and inositol inhibited the binding (Allen et al., 2001). Human SP-D binding to conidia was unaffected by hydrophobic surfactant components. However, SP-D did not increase the association of conidia with rat alveolar macrophages. SP-D-enriched rat BAL fluid inhibited spore binding to extracellular matrix (ECM) proteins and epithelial cells (Yang et al., 2000). Pre-incubation of ECM proteins and epithelial cells with SP-D-enriched BAL fluid prevented the enhancement of spore binding induced by A. fumigatus spore diffusate. SP-D localized to A. fumigatus surface and stayed bound through the different stages of infection of Calu-3 cells (a human airway epithelial cell line) grown on an air-liquid interface (Ordonez et al., 2019). Importantly, fungal adhesion to the epithelium decreased and fungal clearance by neutrophils increased in the presence of SP-D. Human monocyte-derived macrophages phagocytosed SP-D opsonized dormant conidia more efficiently and upregulated the secretion of pro-inflammatory cytokines (Wong et al., 2018). In a murine model of immunocompromised pulmonary invasive aspergillosis, intranasal SP-D treatment rescued mice from death, concomitant with enhanced local production of protective Th1 cytokines, TNF-α and IFN-γ, and that of protective C-C chemokine, MIP-1α (Singh et al., 2009). Immunosuppressed SP-D gene-deficient mice showed an early death upon conidial challenge, a higher hyphal density and tissue injury in lungs. Treatment with SP-D, or a recombinant fragment of human SP-D composed of trimeric neck and CRD regions (rfhSP-D), reduced the mortality, concomitant with higher IFN-γ to IL-4 ratios in treated SP-D gene-deficient mice (Madan et al., 2010). SP-D gene-deficient immunocompetent mice displayed significantly reduced pro-inflammatory cytokines in the lung upon intranasal challenge with wild-type conidia or melanin ghosts (i.e., hollow melanin spheres) (Wong et al., 2018).
In mice mimicking human ABPA, intranasal treatment with native SP-D (or rfhSP-D) suppressed A. fumigatus allergen-specific IgE levels, eosinophilia, pulmonary cellular infiltration and switched the cytokine profile from a pathogenic Th2 to a protective Th1 (Madan et al., 2001). The exogenous SP-D reduced allergen-induced early airway response, bronchial hyper-responsiveness, blood eosinophilia, and Th2 cytokines in murine models of A. fumigatus induced allergic asthma possibly by reducing eotaxin levels in the lung (Erpenbeck et al., 2006). SP-D treatment reduced the allergen-induced histamine release from peripheral blood cells. A 9-fold increase in SP-D protein levels with no concomitant changes in SP-D mRNA was observed in the BALB/c mice sensitized intraperitoneally and challenged intranasally with A. fumigatus allergenic extract (Haczku et al., 2001). C57BL/6 mice have attenuated A. fumigatus-induced allergic airway hyper-responsiveness (AHR) when compared with Balb/c mice owing to a markedly increased SP-D protein expression (Atochina et al., 2003). SP-D gene-deficient mice exhibited intrinsic hypereosinophilia and several-fold increase in levels of IL-5 and IL-13, and lower of IFN-γ to IL-4 ratio in the lungs, suggesting a Th2 bias of immune response (Madan et al., 2005b). SP-D gene-deficient mice were more susceptible than wild-type mice to pulmonary hypersensitivity induced by A. fumigatus allergens. Intranasal treatment with SP-D or rfhSP-D was effective in rescuing the A. fumigatus-sensitized SP-D gene-deficient mice (Madan et al., 2005b).
SP-D Interactome on Aspergillus fumigatus: Involvement of Both CRD and Collagen Domains
Purified human SP-D bound to the allergens present in the 3-week culture filtrate of A. fumigatus as well as purified allergens, gp55 and gp45, in a carbohydrate and calcium-dependent manner but not to the deglycosylated forms of these allergens (Madan et al., 1997b). SP-D inhibited binding of the glycosylated allergens to specific IgE antibodies and induction of histamine release from sensitized basophils. The calcium-activated protein phosphatase, calcineurin, which regulates production of several cell wall molecules, such as 1, 3-β-D-glucan, seems to be a critical factor for SP-D binding to A. fumigatus hyphae (Geunes-Boyer et al., 2010). Calcineurin-deficient hyphae [generated via either deletion of the catalytic subunit calcineurin A (Delta cna A) or pharmacologic inhibition by FK506] were not recognized by SP-D. In presence of Caspofungin (which inhibits 1,3-β-D-glucan synthesis) and nikkomycin Z (which inhibits chitin synthesis), SP-D binding to the wild-type strain was enhanced. These observations suggested presence of multiple SP-D ligands, leaving little room for Aspergillus to escape immune surveillance mediated by SP-D.
To unravel the SP-D ligands present on dormant conidia, the first pathogen entity that enters the host, various conidial cell wall components were purified and examined for their interaction with SP-D via ELISA (Wong et al., 2018). SP-D significantly bound to melanin pigment (polymer of 1,8-dihydroxynaphthalene), a virulent factor that contributes to immune suppression of the host. Germinating conidia, which lacks in melanin layer, bound to SP-D via two cell-wall polysaccharides, galactomannan (GM) and galactosaminogalactan (GAG). As per the localization of melanin, GM and GAG on the conidial surface, SP-D binding was punctate on the dormant conidia, while it was uniform on germinating conidia. SP-D interacted with melanin via its collagen domain whereas its CRD region was involved in binding to GM and GAG.
SP-D Binds 1, 3 β-Glucan on Blastomyces dermatitidis and Inhibits TNF-α Production
Inhaled spores of Blastomyces dermatitidis may cause flu-like symptoms, leading to blastomycosis, an invasive and often serious fungal infection in immunocompromised patients. Bronchoalveolar lavage fluid (BALF)-treated B. dermatitidis showed presence of bound-SP-D that was significantly reduced in the presence of 1,3-β-Glucan, a cell wall component of B. dermatitidis spores. BALF as well as purified SP-D-supplemented SP-D−/− BALF reduced the TNF-α level from β-Glucan-stimulated murine alveolar macrophages. BALF, pre-incubated with B. dermatitidis or β-Glucan, lost the ability to inhibit TNF-α production (Lekkala et al., 2006).
SP-D Directly Inhibits the Growth of Candida albicans and Its Adherence to the Mucosal Epithelial Cells
Candida albicans colonizes the mucosal surfaces of humans and is a common member of the human gut and vaginal flora. Immunocompromised individuals develop fatal candidiasis. SP-D bound and agglutinated C. albicans (yeast form) in a calcium-dependent manner; this interaction was inhibited by competing sugars such as maltose or mannose. Importantly, incubation with SP-D had a fungicidal effect on C. albicans. However, SP-D inhibited phagocytosis of C. albicans by alveolar macrophages (van Rozendaal et al., 2000). SP-D strongly bound to C. albicans infecting Calu-3 cells grown on an air-liquid interface. Binding with SP-D interfered with adhesion of Candida to the epithelium and enhanced neutrophil mediated clearance of opsonized Candida (Ordonez et al., 2019). Thus, SP-D seems to facilitate C. albicans clearance by making the pathogen readily available for neutrophils away from macrophages and epithelial cells.
SP-D Binds to Culture Filtrate Antigens of Coccidioides posadasii
Coccidioidomycosis is a fungal disease caused by highly virulent, soil-fungus Coccidioides immitis or C. posadasiie. Inhalation of air-borne arthroconidia leads to initiation of the primary infection. Clinical manifestations include a severe fatal mycosis involving extra-pulmonary tissues. SP-D binds to coccidiodal culture filtrate antigens. Lungs of mice infected intranasally with a lethal dose of C. posadasii had significantly reduced levels of SP-D (Awasthi et al., 2004).
SP-D Recognizes Glucuronoxylomannan and Mannoprotein 1 of Cryptococcus neoformans Capsule
Cryptococcus neoformans causes infection as a primary human pathogen, or may cause invasive cryptococcosis as an opportunistic pathogen invading the immunocompromised hosts including AIDS patients. Inhalation of acapsular, or sparsely encapsulated cells of C. neoformans leads to development of infection with capsule as the chief virulence factor.
SP-D bound and agglutinated acapsular C. neoformans, but not the encapsulated form, in a calcium- and sugar-dependent manner (Schelenz et al., 1995). Another study reported that SP-D bound acapsular form with a higher affinity than the encapsulated cryptococci leading to their aggregation (van de Wetering et al., 2004). The cryptococcal capsular components, glucuronoxylomannan (GXM) and mannoprotein 1 (MP1), were identified as SP-D ligands; secreted GXM inhibited SP-D-mediated aggregation of acapsular C. neoformans.
SP-D binds and protects C. neoformans cells against macrophage-mediated defense mechanisms in vitro and in vivo (Geunes-Boyer et al., 2009). SP-D binding to C. neoformans was calcineurin-independent (Geunes-Boyer et al., 2010). SP-D-deficient mice infected with C. neoformans showed reduced eosinophil infiltration, IL-5, fungal burden, and increased survival than wild type control animals (Geunes-Boyer et al., 2012; Holmer et al., 2014). The evidence provided by murine models of fungal infections treated with exogenous native or recombinant human SP-D is direct and reliable. SP-D gene-deficient mice develop increased pulmonary inflammation, emphysema, and surfactant phospholipid accumulations (Botas et al., 1998; Korfhagen et al., 1998; Ikegami et al., 2000, 2005; Wert et al., 2000; Fisher et al., 2002). Further, there is a significant increase in activated lymphocytes, apoptotic alveolar macrophages as well as enlarged, lipid-laden, macrophages that release metalloproteinases and reactive oxygen species (Botas et al., 1998; Korfhagen et al., 1998; Ikegami et al., 2000, 2005; Wert et al., 2000; Fisher et al., 2002). Importantly, oxygen radical release and production of the pro-inflammatory mediators, TNF-α, IL-1, and IL-6, were increased in response to either viral or bacterial pathogens (LeVine et al., 2000, 2001, 2004). These coexisting pulmonary abnormalities of SP-D gene deficient mice complicate the interpretation of challenge models. For example, lymphocyte and macrophage activation along with the increased pro-inflammatory mediators might enhance killing and offset any increase of pathogen survival that results more directly from SP-D deficiency.
SP-D Mediated Direct Killing of Histoplasma capsulatum
Histoplasma capsulatum is an intracellular fungal pathogen that causes a self-limiting flu-like illness, and sometimes, a more serious pneumonitis or a chronic cavitary pulmonary infection. In immunosuppressed patients, the fungus can act as an opportunistic pathogen causing a progressive, disseminated disease. Though the ligand on the fungus is not known, exposure to SP-D resulted in increased H. capsulatum permeability in a dose-dependent manner, and hence, decrease in viability. However, SP-D did not aggregate H. capsulatum, or inhibit the phagocytosis of H. capsulatum and growth of macrophage-internalized H. capsulatum (McCormack et al., 2003).
SP-D Binds and Aggregates Pneumocystis carinii via Major Surface Glycoprotein Complex and β-glucans
A 3-fold increase in the total alveolar SP-D protein content was observed in the pulmonary fluid samples from Pneumocystis-infected humans and rats (Aliouat et al., 1998; Qu et al., 2001). SP-D bound to P. carinii via glucose-, mannose-, and N-acetyl-glucosamine-rich major surface glycoprotein complex (gpA), and augmented the adherence of the organisms to alveolar macrophages (O'Riordan et al., 1995). The binding was calcium-dependent and competitively inhibited by maltose > glucose > mannose > N-acetyl-glucosamine, with a higher affinity toward oligomeric forms of SP-D (Vuk-Pavlovic et al., 2001). Maximal SP-D binding was at pH 7.4, with significant inhibition at pH 4. Though SP-D induced aggregation, the phagocytosis of P. carinii was not enhanced. SP-D also bound to fungal β-glucans present on Pneumocystis' cystic forms, which are potent stimulators of TNF-α release (Vuk-Pavlovic et al., 1998).
Vuk-Pavlovic et al. generated human SP-D overexpressing transgenic mice that produced approximately 30-50-fold higher level of SP-D and noted that the mice did not had any significant differences in lung morphology and function (Vuk-Pavlovic et al., 2006). These mice were then depleted of CD4+ lymphocytes, before and during the Pneumocystis murina challenge. SP-D-overexpressing mice exhibited significantly higher fungal burden, with increased levels of TNF-α and macrophage inflammatory protein-2 as well as increased lymphocyte and eosinophil infiltration in BAL on 10 and 14th week (Allen et al., 2001). Though the study inferred increased levels of SP-D led to formation of higher aggregates of Pneumocystis, basal levels of other pattern recognition proteins or immunoregulatory molecules were not examined in the SP-D overexpressing mice.
SP-D Binds Mannoprotein and 1,6-β-D-glucan, Two Cell Wall Components OF Saccharomyces cerevisiae
S. cerevisiae, an opportunistic pathogen, causes severe infections such as fungemia, endocarditis, pneumonia, peritonitis, urinary tract infections, skin infections, and esophagitis in patients with chronic disease, cancer, and immunosuppression. SP-D bound and aggregated Saccharomyces cerevisiae cells and isolated cell walls. Cell wall mannoprotein and 1,6-β-D-glucan of yeast were SP-D ligands but SP-D failed to aggregate chitin. Pustulan [a 1,6-β-linked glucose homopolymer] inhibited SP-D binding to yeast as well as to A. fumigatus. F4/80+ cells, isolated from the BAL of SP-D gene-deficient mice, internalized considerably less zymosan particles (a glucan with repeating glucose units connected by β-1,3-glycosidic linkages) than F4/80+ WT cells (Allen et al., 2001).
SP-D Reversed the Pulmonary Neutrophilic Infiltration and TNF-α Levels Induced in 1,3-β-Glucan-Modulated Allergic Inflammation
As mentioned above, 1,3-β-glucan is an important fungal ligand recognized by SP-D. Sensitization with 1,3-β-Glucan induced pulmonary neutrophilic infiltration and increased TNF-α level in the BAL of SP-D gene-deficient mice (Fakih et al., 2015). This infiltration was significantly reversed by treatment with rfhSP-D. Thus, a high-dose of SP-D could potentially offer protection against mold-induced exacerbations of allergic asthma.
Competitive Interaction of SP-D With Other PRRs for the Common Fungal Ligands
Other PRRs such as Dectins, Surfactant Protein-A (SP-A), Mannan-Binding Lectin (MBL), Mannose Receptor (MR), Macrophage inducible calcium dependent lectin receptor (Mincle), Macrophage C-type Lectin (MCL), Complement receptor 3 (CR3), Dendritic Cell-Specific Intercellular adhesion molecule-3-Grabbing Non-integrin (DC-SIGN), Langerin, and melanin-sensing C-type lectin receptor (MelLec) bind to common fungal ligands as SP-D (Goyal et al., 2018). Another complexity in the anti-fungal host defense is brought in by the fact that SP-D binds to other PRRs such as SP-A (Kuroki et al., 1991), TLR-2, TLR-4 (Ohya et al., 2006) and DC-SIGN (Dodagatta-Marri et al., 2017). These complex interactions need further investigation in pre-clinical studies to realize the true translation potential of PRRs.
Impact of SP-D Genetic Polymorphisms and Proportion of Oligomeric Forms
Genetic polymorphisms of SP-D have been shown to be associated with an increased susceptibility to infections. Allelic variations can influence the quantity and multimerisation of SP-D produced in the serum with differential effects on its binding properties. Individuals with the Thr/Thr (Che et al., 2012b)-encoding genotype had significantly lower SP-D serum levels with predominantly the monomeric form of SP-D than individuals with the Met/Met (Che et al., 2012b) genotype with higher oligomeric forms (Leth-Larsen et al., 2005). However, there has been no study correlating SP-D polymorphisms with susceptibility to fungal infections.
SP-D Levels in Fungal Allergy and Infections
Serum levels of SP-D are altered in asthma, lung hypersensitivity and pulmonary infection caused by P. carinii during AIDS. Median SP-D in BAL was significantly decreased in the lung bacterial infection (12.17 ng/ml) compared with the control group (641 ng/ml), and was below assay limits for the majority of cystic fibrosis children (Postle et al., 1999). In asthmatics and allergics, SP-D levels increased in BAL and serum that went down following corticosteroid therapy (Cheng et al., 2000; Koopmans et al., 2004).
P. carinii pneumonia is associated with raised levels of alveolar SP-D, probably as a result of increased expression and accumulation. The synthesis and secretion of SP-D increased with acute injury and epithelial activation (Atochina et al., 2001).
SP-D levels have not been determined for other fungal infections. SP-D serum levels may be a useful and non-invasive diagnostic tool for fungal infections. The successive monitoring of serum levels of SP-A and/or SPD may predict disease activity, although it is presently unclear if these alterations are a cause or consequence of the disease.
Predisposition of Type-2 Diabetes to Fungal Infections and Serum SP-D Levels
Patients with type-2 diabetes (T2D) exhibited higher serum SP-D concentrations than control subjects (P = 0.006) (López-Cano et al., 2017). There was an inverse association between forced expiratory volume in 1 s (FEV1) and serum SP-D (r = −0.265; P = 0.029), as well as a significant positive relationship between SP-D concentration and residual volume (r = 0.293; P = 0.043). Endurance exercise training with improvement in aerobic fitness induced a significant reduction in serum SP-D levels in obese women with T2D (Rezaei et al., 2017). Significantly increased leukocyte SFTPD mRNA levels were observed in hyperglycemic gestational diabetes mellitus (GDM) patients (P < 0.05) with a significant positive association with C-reactive protein (Wojcik et al., 2016). Additionally, transcript level of SFTPD also correlated positively with fasting glycemia and insulin resistance. These reports suggest that serum levels of SP-D are increased in T2D patients in response to the increased systemic inflammation and get reduced by endurance exercise. However, none of the studies have evaluated the proportion of various oligomeric forms and functional competence of the serum SP-D for host defense in T2D patients. Importantly, T2D obese patients having respiratory tract infections had lower serum SP-D levels than those who did not have infections (p = 0.01) (Jawed et al., 2015). It is likely that in the presence of a respiratory infection, SP-D from the serum moved to the lungs to fight off the pathogen. There are no reports on the association of SP-D levels with respiratory or non-respiratory fungal infections in T2D patients.
Proteolytic Degradation of SP-D
SP-D levels and proportion of oligomeric forms could be significantly altered by proteolytic degradation. Mildly acidic pH, as might be found in endocytic compartments, may disrupt the lectin-dependent activities of SP-D (Persson et al., 1990). Importantly, SP-D was highly resistant to degradation by a wide variety of neutral proteases in vitro, and degradation products have not yet been shown to accumulate under pathological conditions in vivo (Brown-Augsburger et al., 1996). Dodecamers of recombinant rat SP-D were not degraded by human leukocyte elastase (HLE) (1 μM) or a variety of secreted mammalian neutral proteases at 37°C in the presence of physiologic calcium concentrations (Crouch, 2000). In the absence of calcium, multimeric SP-D was partially digested by 1,000-fold higher molar concentrations of HLE (50 μM) in a dose- and time-dependent manner with complete digestion happening in 24 h (Griese et al., 2003). Functional studies showed that digested SP-D had lost its calcium-dependent lectin properties, i.e., it neither bound to mannose nor agglutinated bacteria. These studies demonstrate that elastase results in the limited proteolysis of SP-D with loss of its CRD-dependent activities. HLE is present up to 19 U/ml in BAL in patients with cystic fibrosis. Results in patients with CF and high elastase activity in the BAL fluid indicated decreased SP-D in some, but not in all subjects (Griese et al., 2003). In contrast, lung diseases without significant neutrophilic inflammation in BAL fluid are not expected to exhibit degraded SP-D. This observed resistance of native SP-D to proteolytic damage might lead to enhancement of host defense functions on exogenous intranasal administration as validated by the murine model studies (Madan et al., 2001, 2005b, 2010; Singh et al., 2009). Incubation of cell-free BAL fluid with protease IV of P. aeruginosa resulted in degradation of SP-D in a time- and dose-dependent fashion; this degradation was inhibited by the trypsin-like serine protease inhibitor N-α-p-tosyl-L-lysine-chloromethyl ketone (TLCK) (Malloy et al., 2005). Degradation by protease IV led to inhibition of SP-D-mediated bacterial aggregation and uptake by macrophages.
Perspectives
Interaction of SP-D with several fungal pathogens has been established beyond doubt. SP-D binds multiple ligands of several fungi and modulates the immune response of the host geared mostly toward elimination of the pathogen, and alleviation of fungal allergies. Although the studies are limited mostly to respiratory fungal pathogens, with the presence of SP-D in digestive tract mucosa, reproductive tract mucosa, and most importantly skin, it is quite likely that SP-D may impact other fungal infections too.
Functionally competent purified recombinant forms of full length and truncated human SP-D have been evaluated extensively using in vitro, in vivo, and ex vivo systems, and are available in adequate amounts for therapeutic intervention (Madan et al., 2005a; Mahajan et al., 2013). Intranasal delivery of recombinant SP-D proteins via nebulisers or inhalers would provide the fastest delivery to the site of action, maximal efficacy up to distal airways and half-life (bypassing the proteolytic degradation by other routes of delivery) as evident from the animal model studies (Madan et al., 2001, 2005b, 2010; Singh et al., 2009). rfhSP-D would significantly and immediately reduce the pathogen load as well as restore the immune homeostasis in both allergic and invasive mycoses. In view of the serious adverse effects of available anti-fungal drugs, and a significant therapeutic efficacy and safety of rfhSP-D reported in murine models of allergic and invasive aspergillosis, rfhSP-D as an adjunct therapy may be a more effective option. However, there are no studies yet that have evaluated rfhSP-D in conjunction with standard therapies such as Amphotericin B/ azoles for invasive pulmonary aspergillosis and corticosteroids for allergic pulmonary aspergillosis. Insight into the molecular mechanisms and reproducibility of the preclinical proof-of-concept therapeutic efficacy of rfhSP-D in animal models of fungal infections, strengthen the case for pursuing clinical trials of inhalation formulations of rfhSP-D.
It is important to recognize that there are additional biological functions of SP-D that have been demonstrated already for other diseases. These include: (i) assisting elimination of several viral and bacterial pathogens (Kishore et al., 2005); (ii) enhanced apoptosis of cancer cells (Mahajan et al., 2013) and activated T lymphocytes (Pandit et al., 2016); (iii) attenuation of sepsis-induced pancreatic injury (Liu et al., 2015); (iv) dual role in vascular inflammation and pro-inflammatory disease (Colmorten et al., 2019); and (v) regulation of energy intake and inhibitor of metabolic endotoxemia (Stidsen et al., 2012). This is in addition to its recently discovered involvement in male and female fertility (Kay and Madan, 2016; Rokade et al., 2017). Therefore, it is pertinent to consider that clinical application of SP-D may impact upon more than one facet of health.
Author Contributions
TM wrote the first draft of the review. UK provided critical suggestions for the manuscript.
Conflict of Interest
The authors declare that the research was conducted in the absence of any commercial or financial relationships that could be construed as a potential conflict of interest.
Acknowledgments
The authors dedicate this article to Dr. Puranam Usha Sarma who mentored their initial research in the area of fungal immunology. TM would like to thank ICMR-National Institute for Research in Reproductive Health, Mumbai for the financial support. Editorial help by Dr Sanjeev Kumar Gupta is gratefully appreciated.
References
Aliouat, E. M., Escamilla, R., Cariven, C., Vieu, C., Mullet, C., Dei-Cas, E., et al. (1998). Surfactant changes during experimental pneumocystosis are related to Pneumocystis development. Eur. Respir. J. 11, 542–547.
Allen, M. J., Voelker, D. R., and Mason, R. J. (2001). Interactions of surfactant proteins A and D with Saccharomyces Cerevisiae and Aspergillus Fumigatus. Infect. Immun. 69, 2037–2044. doi: 10.1128/IAI.69.4.2037-2044.2001
Atochina, E. N., Beck, J. M., Scanlon, S. T., Preston, A. M., and Beers, M. F. (2001). Pneumocystis carinii pneumonia alters expression and distribution of lung collectins SP-A and SP-D. J. Lab Clin. Med. 137, 429–439. doi: 10.1067/mlc.2001.115220
Atochina, E. N., Beers, M. F., Tomer, Y., Scanlon, S. T., Russo, S. J., Panettieri, R. A. Jr, et al. (2003). Attenuated allergic airway hyperresponsiveness in C57BL/6 mice is associated with enhanced surfactant protein (SP)-D production following allergic sensitization. Respir. Res. 4, 15. doi: 10.1186/1465-9921-4-15
Awasthi, S., Magee, D. M., and Coalson, J. J. (2004). Coccidioides Posadasii infection alters the expression of pulmonary surfactant proteins (SP)-A and SP-D. Respir. Res. 5:28. doi: 10.1186/1465-9921-5-28
Barrow, A. D., Palarasah, Y., Bugatti, M., Holehouse, A. S., Byers, D. E., Holtzman, M. J., et al. (2015). OSCAR is a receptor for surfactant protein D that activates TNF-alpha release from human CCR2+ inflammatory monocytes. J. Immunol. 194, 3317–3326. doi: 10.4049/jimmunol.1402289
Bongomin, F., Gago, S., Oladele, R. O., and Denning, D. W. (2017). Global and multi-national prevalence of fungal diseases—estimate precision. J. Fungi. 3:57. doi: 10.3390/jof3040057
Botas, C., Poulain, F., Akiyama, J., Brown, C., Allen, L., Goerke, J., et al. (1998). Altered surfactant homeostasis and alveolar type II cell morphology in mice lacking surfactant protein D. Proc. Natl. Acad. Sci. U. S. A 95, 11869–11874 doi: 10.1073/pnas.95.20.11869
Brown-Augsburger, P. K. L., Hartshorn, D., Chang, K., Rust, C., Fliszar, H. G., and Welgus, E. C. (1996). Crouch. Site-directed mutagenesis of Cys-15 and Cys-20 of pulmonary surfactant protein D. J. Biol. Chem. 271, 13724–13730. doi: 10.1074/jbc.271.23.13724
Carreto-Binaghi, L. E., Aliouat, E. M., and Taylor, M. L. (2016). Surfactant proteins, SP-A and SP-D, in respiratory fungal infections: their role in the inflammatory response. Respir. Res.17:66. doi: 10.1186/s12931-016-0385-9
Che, C. Y., Jia, W. Y., Xu, Q., Li, N., Hu, L. T., Jiang, N., et al. (2012a). The roles of surfactant protein D during Aspergillus fumigatus infection in human corneal epithelial cells. Int. J. Ophthalmol. 5, 13–17. doi: 10.3980/j.issn.2222-3959.2012.01.03
Che, C. Y., Li, X. J., Jia, W. Y., Li, N., Xu, Q., Lin, J., et al. (2012b). Early expression of surfactant proteins D in fusarium solani infected rat cornea. Int. J. Ophthalmol. 5, 297–300. doi: 10.3980/j.issn.2222-3959.2012.03.09
Cheng, G., Ueda, T., Numao, T., Kuroki, Y., Nakajima, H., Fukushima, Y., et al. (2000). Increased levels of surfactant protein A and D in bronchoalveolar lavage fluids in patients with bronchial asthma. Eur. Respir. J. 16, 831–835. doi: 10.1183/09031936.00.16583100
Colmorten, K. B., Nexoe, A. B., and Sorensen, G. L. (2019). The dual role of surfactant protein-D in vascular inflammation and development of cardiovascular disease. Front. Immunol. 10:2264. doi: 10.3389/fimmu.2019.02264
Crouch, E. C. (2000). Surfactant protein-D and pulmonary host defense. Respir. Res. 1, 93–108. doi: 10.1186/rr19
Dodagatta-Marri, E., Mitchell, D. A., Pandit, H., Sonawani, A., Murugaiah, V., Idicula-Thomas, S., et al. (2017). Protein-protein interaction between surfactant protein D and DC-SIGN via C-type lectin domain can suppress HIV-1 transfer. Front. Immunol. 8:834. doi: 10.3389/fimmu.2017.00834
Erpenbeck, V. J., Ziegert, M., Cavalet-Blanco, D., Martin, C., Baelder, R., Glaab, T., et al. (2006). Surfactant protein D inhibits early airway response in Aspergillus Fumigatus-sensitized mice. Clin. Exp. Allergy. 36, 930–940. doi: 10.1111/j.1365-2222.2006.02524.x
Fakih, D., Pilecki, B., Schlosser, A., Jepsen, C. S., Thomsen, L. K., Ormhøj, M., et al. (2015). Protective effects of surfactant protein D treatment in 1,3-β-glucan-modulated allergic inflammation. Am. J. Physiol. Lung Cell Mol. Physiol. 309, L1333–L1343. doi: 10.1152/ajplung.00090.2015
Fisher, J. H., Larson, J., Cool, C., and Dow, S. W. (2002). Lymphocyte activation in the lungs of SP-D null mice. Am. J. Respir. Cell Mol. Biol. 27, 24–33. doi: 10.1165/ajrcmb.27.1.4563
Fukuzawa, T., Ishida, J., Kato, A., Ichinose, T., Ariestanti, D. M., Takahashi, T., et al. (2013). Lung surfactant levels are regulated by Ig-Hepta/GPR116 by monitoring surfactant protein D. PLoS ONE 8:e69451. doi: 10.1371/journal.pone.0069451
Gardai, S. J., Xiao, Y. Q., Dickinson, M., Nick, J. A., Voelker, D. R., Greene, K. E., et al. (2003). By binding SIRPalpha or calreticulin/CD91, lung collectins act as dual function surveillance molecules to suppress or enhance inflammation. Cell 115, 13–23. doi: 10.1016/S0092-8674(03)00758-X
Ge, M. Q., Kokalari, B., Flayer, C. H., Killingbeck, S. S., Redai, I. G., MacFarlane, A. W. IV., et al. (2016). Cutting edge: role of NK cells and surfactant protein D in dendritic cell lymph node homing: effects of ozone exposure. J. Immunol. 196, 553–557. doi: 10.4049/jimmunol.1403042
Geunes-Boyer, S., Beers, M. F., Perfect, J. R., Heitman, J., and Wright, J. R. (2012). Surfactant protein D facilitates Cryptococcus Neoformans infection. Infect. Immun. 80, 2444–2453. doi: 10.1128/IAI.05613-11
Geunes-Boyer, S., Heitman, J., Wright, J. R., and Steinbach, W. J. (2010). Surfactant protein D binding to Aspergillus Fumigatus hyphae is calcineurin-sensitive. Med. Mycol. 48, 580–588. doi: 10.3109/13693780903401682
Geunes-Boyer, S., Oliver, T. N., Janbon, G., Lodge, J. K., Heitman, J., Perfect, J. R., et al. (2009). Surfactant protein D increases phagocytosis of hypocapsular Cryptococcus Neoformans by murine macrophages and enhances fungal survival. Infect. Immun. 77, 2783–2794. doi: 10.1128/IAI.00088-09
Goyal, S., Castrillón-Betancur, J. C., Klaile, E., and Slevogt, H. (2018). The interaction of human pathogenic fungi with C-type lectin receptors. Front. Immunol. 9:1261. doi: 10.3389/fimmu.2018.01261
Griese, M., Wiesener, A., Lottspeich, F., and von Bredow, C. H. (2003). Limited proteolysis of surfactant protein D and causes a loss of its calcium-dependent lectin functions. Biochim. Biophys. Acta 1638, 157–63. doi: 10.1016/S0925-4439(03)00063-2
Haczku, A., Atochina, E. N., Tomer, Y., Chen, H., Scanlon, S. T., Russo, S., et al. (2001). Aspergillus fumigatus-induced allergic airway inflammation alters surfactant homeostasis and lung function in BALB/c mice. Am. J. Respir. Cell Mol. Biol. 25, 45–50. doi: 10.1165/ajrcmb.25.1.4391
Hasegawa, Y., Takahashi, M., Ariki, S., Asakawa, D., Tajiri, M., Wada, Y., et al. (2015). Surfactant protein D suppresses lung cancer progression by downregulation of epidermal growth factor signaling. Oncogene 34, 838–845. doi: 10.1038/onc.2014.20
Holmer, S. M., Evans, K. S., Asfaw, Y. G., Saini, D., Schell, W. A., Ledford, J. G., et al. (2014). Impact of surfactant protein D, interleukin-5, and eosinophilia on cryptococcosis. Infect. Immun. 82, 683–693. doi: 10.1128/IAI.00855-13
Ikegami, M., Na, C. L., Korfhagen, T. R., and Whitsett, J. A. (2005). Surfactant protein D influences surfactant ultrastructure and uptake by alveolar type II cells. Am. J. Physiol. Lung Cell Mol. Physiol. 288, L552–L561 doi: 10.1152/ajplung.00142.2004
Ikegami, M., Whitsett, J. A., Jobe, A., Ross, G., Fisher, J., and Korfhagen, T. (2000). Surfactant metabolism in SP-D gene-targeted mice. Am. J. Physiol. Lung Cell Mol. Physiol. 279, L468–L476. doi: 10.1152/ajplung.2000.279.3.L468
Jakel, A., Qaseem, A. S., Kishore, U., and Sim, R. B. (2013). Ligands and receptors of lung surfactant proteins SP-A and SP-D. Front. Biosci. 18, 1129–1140. doi: 10.2741/4168
Jawed, S., Saeed, M., and Parveen, N. (2015). Respiratory tract infections in diabetic and non-diabetic individuals are linked with serum surfactant protein-D. J. Pak. Med. Assoc. 65, 1210–1213.
Kay, S., and Madan, T. (2016). Fertility defects in Surfactant associated protein D knockout female mice: altered ovarian hormone profile. Mol Immunol. 71, 87–97. doi: 10.1016/j.molimm.2016.01.002
Kishore, U., Bernal, A. L., Kamran, M. F., Saxena, S., Singh, M., Sarma, P. U., et al. (2005). Surfactant proteins SP-A and SP-D in human health and disease. Arch Immunol. Ther. Exp. 53, 399–417.
Kishore, U., Greenhough, T. J., Waters, P., Shrive, A. K., Ghai, R., Kamran, M. F., et al. (2006). Surfactant proteins SP-A and SP-D: structure, function and receptors. Mol. Immunol. 43, 1293–1315. doi: 10.1016/j.molimm.2005.08.004
Koopmans, J. G., van der Zee, J. S., Krop, E. J., Lopuhaa, C. E., Jansen, H. M., and Batenburg, J. J. (2004). Serum surfactant protein D is elevated in allergic patients. Clin. Exp. Allergy 34, 1827–1833. doi: 10.1111/j.1365-2222.2004.02083.x
Korfhagen, T. R., Sheftelyevich, V., Burhans, M. S., Bruno, M. D., Ross, G. F., Wert, S. E., et al. (1998). Surfactant protein-D regulates surfactant phospholipid homeostasis in vivo. J. Biol. Chem. 273, 28438–28443 doi: 10.1074/jbc.273.43.28438
Kurimura, Y., Nishitani, C., Ariki, S., Saito, A., Hasegawa, Y., Takahashi, M., et al. (2012). Surfactant protein D inhibits adherence of uropathogenic Escherichia Coli to the bladder epithelial cells and the bacterium-induced cytotoxicity: a possible function in urinary tract. J. Biol. Chem. 287, 39578–39588. doi: 10.1074/jbc.M112.380287
Kuroki, Y., Shiratori, M., Murata, Y., and Akino, T. (1991). Surfactant protein D (SP-D) counteracts the inhibitory effect of surfactant protein A (SP-A) on phospholipid secretion by alveolar type II cells. Interaction of native SP-D with SP-A. Biochem. J. 279 (Pt 1), 115–119. doi: 10.1042/bj2790115
Lekkala, M., LeVine, A. M., Linke, M. J., Crouch, E. C., Linders, B., Brummer, E., et al. (2006). Effect of lung surfactant collectins on bronchoalveolar macrophage interaction with Blastomyces Dermatitidis: inhibition of tumor necrosis factor alpha production by surfactant protein D. Infect. Immun. 74, 4549–4556. doi: 10.1128/IAI.00243-06
Leth-Larsen, R., Garred, P., Jensenius, H., Meschi, J., Hartshorn, K., Madsen, J., et al. (2005). A common polymorphism in the SFTPD gene influences assembly, function, and concentration of surfactant protein D. J. Immunol. 174, 1532–1538. doi: 10.4049/jimmunol.174.3.1532
LeVine, A. M., Elliott, J., Whitsett, J. A., Srikiatkhachorn, A., Crouch, E., DeSilva, N., et al. (2004). Surfactant protein-d enhances phagocytosis and pulmonary clearance of respiratory syncytial virus. Am. J. Respir. Cell Mol. Biol. 31, 193–199 doi: 10.1165/rcmb.2003-0107OC
LeVine, A. M., Whitsett, J. A., Gwozdz, J. A., Richardson, T. R., Fisher, J. H., Burhans, M. S., et al. (2000). Distinct effects of surfactant protein A or D deficiency during bacterial infection on the lung. J. Immunol. 165, 3934–3940 doi: 10.4049/jimmunol.165.7.3934
LeVine, A. M., Whitsett, J. A., Hartshorn, K. L., Crouch, E. C., and Korfhagen, T. R. (2001). Surfactant protein D enhances clearance of influenza A virus from the lung in vivo. J. Immunol. 167, 5868–5873 doi: 10.4049/jimmunol.167.10.5868
Liu, C. F., Rivere, M., Huang, H. J., Puzo, G., and Wang, J. Y. (2010). Surfactant protein D inhibits mite-induced alveolar macrophage and dendritic cell activations through TLR signalling and DC-SIGN expression. Clin. Exp. Allergy 40, 111–122. doi: 10.1111/j.1365-2222.2009.03367.x
Liu, Z., Shi, Q., Liu, J., Abdel-Razek, O., Xu, Y., Cooney, R. N., et al. (2015). Innate immune molecule surfactant protein D attenuates sepsis-induced acute pancreatic injury through modulating apoptosis and NF-κB-mediated inflammation. Sci. Rep. 5:17798. doi: 10.1038/srep17798
López-Cano, C., Lecube, A., García-Ramírez, M., Muñoz, X., Sánchez, E., Seminario, A., et al. (2017). Serum surfactant protein D as a biomarker for measuring lung involvement in obese patients with type 2 diabetes. J. Clin. Endocrinol. Metab. 102, 4109–4116. doi: 10.1210/jc.2017-00913
Madan, T., Eggleton, P., Kishore, U., Strong, P., Aggrawal, S. S., Sarma, P. U., et al. (1997a). Binding of pulmonary surfactant proteins A and D to Aspergillus fumigatus conidia enhances phagocytosis and killing by human neutrophils and alveolar macrophages. Infect. Immun. 65, 3171–3179. doi: 10.1128/IAI.65.8.3171-3179.1997
Madan, T., Kaur, S., Saxena, S., Singh, M., Kishore, U., Thiel, S., et al. (2005a). Role of collectins in innate immunity against aspergillosis. Med. Mycol. 43(Suppl. 1), S155–S163. doi: 10.1080/13693780500088408
Madan, T., Kishore, U., Shah, A., Eggleton, P., Strong, P., Wang, J. Y., et al. (1997b). Lung surfactant proteins A and D can inhibit specific IgE binding to the allergens of Aspergillus Fumigatus and block allergen-induced histamine release from human basophils. Clin. Exp. Immunol. 110, 241–249. doi: 10.1111/j.1365-2249.1997.tb08323.x
Madan, T., Kishore, U., Singh, M., Strong, P., Clark, H., Hussain, E. M., et al. (2001). Surfactant proteins A and D protect mice against pulmonary hypersensitivity induced by Aspergillus Fumigatus antigens and allergens. J. Clin. Invest. 107, 467–475. doi: 10.1172/JCI10124
Madan, T., Reid, K. B., Clark, H., Singh, M., Nayak, A., Sarma, P. U., et al. (2010). Susceptibility of mice genetically deficient in SP-A or SP-D gene to invasive pulmonary aspergillosis. Mol. Immunol. 47, 1923–1930. doi: 10.1016/j.molimm.2010.02.027
Madan, T., Reid, K. B., Singh, M., Sarma, P. U., and Kishore, U. (2005b). Susceptibility of mice genetically deficient in the surfactant protein (SP)-A or SP-D gene to pulmonary hypersensitivity induced by antigens and allergens of Aspergillus Fumigatus. J. Immunol. 174, 6943–6954. doi: 10.4049/jimmunol.174.11.6943
Mahajan, L., Pandit, H., Madan, T., Gautam, P., Yadav, A. K., Warke, H., et al. (2013). Human surfactant protein D alters oxidative stress and HMGA1 expression to induce p53 apoptotic pathway in eosinophil leukemic cell line. PLoS ONE 8:e85046. doi: 10.1371/journal.pone.0085046
Malloy, J. L., Veldhuizen, R. A., Thibodeaux, B. A., O'Callaghan, R. J., and Wright, J. R. (2005). Pseudomonas aeruginosa protease IV degrades surfactant proteins and inhibits surfactant host defense and biophysical functions. Am. J. Physiol. Lung Cell Mol. Physiol. 288, L409–L418 doi: 10.1152/ajplung.00322.2004
McCormack, F. X., Gibbons, R., Ward, S. R., Kuzmenko, A., Wu, H., and Deepe, G. S Jr. (2003). Macrophage-independent fungicidal action of the pulmonary collectins. J. Biol. Chem. 278, 36250–36256. doi: 10.1074/jbc.M303086200
Nie, X., Nishitani, C., Yamazoe, M., Ariki, S., Takahashi, M., Shimizu, T., et al. (2008). Pulmonary surfactant protein D binds MD-2 through the carbohydrate recognition domain. Biochemistry 47, 12878–12885. doi: 10.1021/bi8010175
Ohya, M., Nishitani, C., Sano, H., Yamada, C., Mitsuzawa, H., Shimizu, T., et al. (2006). Human pulmonary surfactant protein D binds the extracellular domains of Toll-like receptors 2 and 4 through the carbohydrate recognition domain by a mechanism different from its binding to phosphatidylinositol and lipopolysaccharide. Biochemistry 45, 8657–8664. doi: 10.1021/bi060176z
Olde Nordkamp, M. J., van Eijk, M., Urbanus, R. T., Bont, L., Haagsman, H. P., and Meyaard, L. (2014). Leukocyte-associated Ig-like receptor-1 is a novel inhibitory receptor for surfactant protein D. J. Leukoc. Biol. 96, 105–111. doi: 10.1189/jlb.3AB0213-092RR
Ordonez, S. R., van Eijk, M., Escobar Salazar, N., de Cock, H., Veldhuizen, E. J. A., and Haagsman, H. P. (2019). Antifungal activities of surfactant protein D in an environment closely mimicking the lung lining. Mol. Immunol. 105, 260–269. doi: 10.1016/j.molimm.2018.12.003
O'Riordan, D. M., Standing, J. E., Kwon, K. Y., Chang, D., Crouch, E. C., and Limper, A. H. (1995). Surfactant protein D interacts with Pneumocystis carinii and mediates organism adherence to macrophages. J. Clin. Invest. 95, 2699–2710. doi: 10.1172/JCI117972
Pandit, H., Madhukaran, S. P., Nayak, A., and Madan, T. (2012). SP-A and SP-D in host defense against fungal infections and allergies. Front. Biosci. 4, 651–661. doi: 10.2741/e406
Pandit, H., Thakur, G., Koippallil Gopalakrishnan, A. R., Dodagatta-Marri, E., Patil, A., Kishore, U., et al. (2016). Surfactant protein D induces immune quiescence and apoptosis of mitogen-activated peripheral blood mononuclear cells. Immunobiology 221, 310–322. doi: 10.1016/j.imbio.2015.10.004
Persson, A., Chang, D., and Crouch, E. (1990). Surfactant protein D is a divalent cation-dependent carbohydrate-binding protein. J. Biol. Chem. 265, 5755–5760.
Postle, A. D., Mander, A., Reid, K. B. M., Wang, J. Y., Wright, S. M., Moustaki, M., et al. (1999). Deficient hydrophilic lung surfactant proteins A and D with normal surfactant phospholipid molecular species in cystic fibrosis. Am. J. Respir. Cell Mol. Biol. 20, 90–98. doi: 10.1165/ajrcmb.20.1.3253
Qu, J., He, L., Rong, Z., Pan, J., Chen, X., Morrison, D. C., et al. (2001). Alteration of surfactant proteins A and D in bronchoalveolar lavage fluid of Pneumocystis carinii pneumonia. Chin. Med. J. 114, 1143–1146.
Rezaei, S., Shamsi, M. M., Mahdavi, M., Jamali, A., Prestes, J., Tibana, R. A., et al. (2017). Endurance exercise training decreased serum levels of surfactant protein D and improved aerobic fitness of obese women with type-2 diabetes. Diabetol. Metab. Syndr. 9:74. doi: 10.1186/s13098-017-0273-6
Rokade, S., Kishore, U., and Madan, T. (2017). Surfactant protein D regulates murine testicular immune milieu and sperm functions. Am. J. Reprod. Immunol. 77, 1–15. doi: 10.1111/aji.12629
Salazar, F., and Brown, G. D. (2018). Antifungal innate immunity: a perspective from the last 10 years. J. Innate Immun. 10, 373–397 doi: 10.1159/000488539
Sano, H., Chiba, H., Iwaki, D., Sohma, H., Voelker, D. R., and Kuroki, Y. (2000). Surfactant proteins A and D bind CD14 by different mechanisms. J. Biol. Chem. 275, 22442–22451. doi: 10.1074/jbc.M001107200
Schelenz, S., Malhotra, R., Sim, R. B., Holmskov, U., and Bancroft, G. J. (1995). Binding of host collectins to the pathogenic yeast Cryptococcus Neoformans: human surfactant protein D acts as an agglutinin for acapsular yeast cells. Infect. Immun. 63, 3360–3366. doi: 10.1128/IAI.63.9.3360-3366.1995
Schob, S., Schicht, M., Sel, S., Stiller, D., Kekulé, A. S., Paulsen, F., et al. (2013). The detection of surfactant proteins A, B, C and D in the human brain and their regulation in cerebral infarction, autoimmune conditions and infections of the CNS. PLoS ONE 8:e74412. doi: 10.1371/journal.pone.0074412
Singh, M., Madan, T., Waters, P., Sonar, S., Singh, S. K., Kamran, M. F., et al. (2009). Therapeutic effects of recombinant forms of full-length and truncated human surfactant protein D in a murine model of invasive pulmonary aspergillosis. Mol. Immunol. 46, 2363–2369. doi: 10.1016/j.molimm.2009.03.019
Sorensen, G. L. (2018). Surfactant protein D in respiratory and non-respiratory diseases. Front. Med. 5:18. doi: 10.3389/fmed.2018.00018
Sorensen, G. L., Madsen, J., Kejling, K., Tornoe, I., Nielsen, O., Townsend, P., et al. (2006). Surfactant protein D is proatherogenic in mice. Am. J. Physiol. Heart Circ. Physiol. 290, H2286–H2294. doi: 10.1152/ajpheart.01105.2005
Stidsen, J. V., Khorooshi, R., Rahbek, M. K., Kirketerp-Møller, K. L., Hansen, P. B., Bie, P., et al. (2012). Surfactant protein D deficiency in mice is associated with hyperphagia, altered fat deposition, insulin resistance, and increased basal endotoxemia. PLoS ONE 7:e35066. doi: 10.1371/journal.pone.0035066
van de Wetering, J. K., Coenjaerts, F. E., Vaandrager, A. B., van Golde, L. M., and Batenburg, J. J. (2004). Aggregation of Cryptococcus Neoformans by surfactant protein D is inhibited by its capsular component glucuronoxylomannan. Infect. Immun. 72, 145–153. doi: 10.1128/IAI.72.1.145-153.2004
van Rozendaal, B. A., van Spriel, A. B., van De Winkel, J. G., and Haagsman, H. P. (2000). Role of pulmonary surfactant protein D in innate defense against Candida Albicans. J. Infect. Dis. 182, 917–922. doi: 10.1086/315799
von Bredow, C., Hartl, D., Schmid, K., Schabaz, F., Brack, E., Reinhardt, D., et al. (2006). Surfactant protein D regulates chemotaxis and degranulation of human eosinophils. Clin. Exp. Allergy 36, 1566–1574. doi: 10.1111/j.1365-2222.2006.02598.x
Vuk-Pavlovic, Z., Diaz-Montes, T., Standing, J. E., and Limper, A. H. (1998). Surfactant protein-D binds to cell wall b-glucans. Am. J. Respir. Crit. Care Med. 157:A236.
Vuk-Pavlovic, Z., Mo, E. K., Icenhour, C. R., Standing, J. E., Fisher, J. H., and Limper, A. H. (2006). Surfactant protein D enhances pneumocystis infection in immune-suppressed mice. Am. J. Physiol. Lung Cell. Mol. Physiol. 290, L442–L449. doi: 10.1152/ajplung.00112.2005
Vuk-Pavlovic, Z., Standing, J. E., Crouch, E. C., and Limper, A. H. (2001). Carbohydrate recognition domain of surfactant protein D mediates interactions with Pneumocystis carinii glycoprotein A. Am. J. Respir. Cell Mol. Biol. 24, 475–484. doi: 10.1165/ajrcmb.24.4.3504
Wert, S. E., Yoshida, M., LeVine, A. M., Ikegami, M., Jones, T., Ross, G. F., et al. (2000). Increased metalloproteinase activity, oxidant production, and emphysema in surfactant protein D gene-inactivated mice. Proc Natl Acad Sci U S A 97, 5972–5977. doi: 10.1073/pnas.100448997
Wojcik, M., Zieleniak, A., Zurawska-Klis, M., Cypryk, K., and Wozniak, L. A. (2016). Increased expression of immune-related genes in leukocytes of patients with diagnosed gestational diabetes mellitus (GDM). Exp. Biol. Med. 241, 457–465. doi: 10.1177/1535370215615699
Wong, S. S. W., Rani, M., Dodagatta-Marri, E., Ibrahim-Granet, O., Kishore, U., Bayry, J., et al. (2018). Fungal melanin stimulates surfactant protein D-mediated opsonization of and host immune response to Aspergillus Fumigatus spores. J. Biol. Chem. 293, 4901–4912. doi: 10.1074/jbc.M117.815852
Wu, X., Zhao, G., Lin, J., Jiang, N., Li, C., Hu, L., et al. (2015). The production mechanism and immunosuppression effect of pulmonary surfactant protein D via toll like receptor 4 signaling pathway in human corneal epithelial cells during Aspergillus Fumigatus infection. Int. Immunopharmacol. 29, 433–439. doi: 10.1016/j.intimp.2015.10.018
Yamazoe, M., Nishitani, C., Takahashi, M., Katoh, T., Ariki, S., Shimizu, T., et al. (2008). Pulmonary surfactant protein D inhibits lipopolysaccharide (LPS)-induced inflammatory cell responses by altering LPS binding to its receptors. J. Biol. Chem. 283, 35878–35888. doi: 10.1074/jbc.M807268200
Keywords: SP-D, fungi, cell wall, polysaccharides, glycoprotein, mycoses, allergy
Citation: Madan T and Kishore U (2020) Surfactant Protein D Recognizes Multiple Fungal Ligands: A Key Step to Initiate and Intensify the Anti-fungal Host Defense. Front. Cell. Infect. Microbiol. 10:229. doi: 10.3389/fcimb.2020.00229
Received: 27 January 2020; Accepted: 23 April 2020;
Published: 29 May 2020.
Edited by:
Jagadeesh Bayry, Institut National de la Santé et de la Recherche Médicale (INSERM), FranceReviewed by:
Grith Lykke Sorensen, University of Southern Denmark, DenmarkJiu-Yao Wang, National Cheng Kung University, Taiwan
Copyright © 2020 Madan and Kishore. This is an open-access article distributed under the terms of the Creative Commons Attribution License (CC BY). The use, distribution or reproduction in other forums is permitted, provided the original author(s) and the copyright owner(s) are credited and that the original publication in this journal is cited, in accordance with accepted academic practice. No use, distribution or reproduction is permitted which does not comply with these terms.
*Correspondence: Taruna Madan, dGFydW5hX20mI3gwMDA0MDtob3RtYWlsLmNvbQ==