- 1The Marshall Centre for Infectious Diseases Research and Training, School of Biomedical Sciences, The University of Western Australia, Perth, WA, Australia
- 2Wesfarmers Centre of Vaccines and Infectious Diseases, Telethon Kids Institute, The University of Western Australia, Perth, WA, Australia
- 3Telethon Kids Institute, The University of Western Australia, Perth, WA, Australia
Alloiococcus otitidis and Turicella otitidis are common bacteria of the human ear. They have frequently been isolated from the middle ear of children with otitis media (OM), though their potential role in this disease remains unclear and confounded due to their presence as commensal inhabitants of the external auditory canal. In this review, we summarize the current literature on these organisms with an emphasis on their role in OM. Much of the literature focuses on the presence and abundance of these organisms, and little work has been done to explore their activity in the middle ear. We find there is currently insufficient evidence available to determine whether these organisms are pathogens, commensals or contribute indirectly to the pathogenesis of OM. However, building on the knowledge currently available, we suggest future approaches aimed at providing stronger evidence to determine whether A. otitidis and T. otitidis are involved in the pathogenesis of OM. Such evidence will increase our understanding of the microbial risk factors contributing to OM and may lead to novel treatment approaches for severe and recurrent disease.
Introduction
Otitis media (OM) is a polymicrobial disease most common in young children, characterized by inflammation of the middle ear and the presence of fluid behind the tympanic membrane. OM is a significant health care burden, affecting over 80% of children by the age of 3 (Teele et al., 1989) at an estimated annual cost of AUD$100 to 400 million in Australia (Taylor et al., 2009). The signs and symptoms of OM have been described as a phenotypic landscape of disease (Bhutta, 2014), but are commonly divided into two broad categories; acute OM (AOM) and OM with effusion (OME). In AOM, signs of an acute infection are present with a bulging tympanic membrane and often purulent fluid in the middle ear. Episodes of AOM may recur, becoming recurrent AOM (rAOM) if 3 or more episodes are experienced within 6 months, or ≥ 4 in 12 months (Kong and Coates, 2009). In OME, there are no signs of acute infection with the fluid more commonly serous or mucoid. If the fluid persists for ≥3 months, this is diagnosed as chronic OME (COME) (Bluestone et al., 2002). The onset of AOM will often occur subsequent to a viral upper respiratory infection, and may develop into OME (Bhutta, 2014). Chronic suppurative OM (CSOM) may follow untreated rAOM or COME, where the tympanic membrane perforates and there is persistent discharge (otorrhea) from the middle ear (Kong and Coates, 2009), allowing infection from external sources. Untreated OM can result in conductive hearing loss and consequent speech and language delays (Kong and Coates, 2009).
Three major bacterial pathogens are known to be involved in OM (known as otopathogens); Streptococcus pneumoniae, non-typeable Haemophilus influenzae (NTHi), and Moraxella catarrhalis. These otopathogens are frequently detected in middle ear fluid (MEF) from both children with OME and with AOM (Bluestone et al., 1992). As colonizers of the nasopharynx, these otopathogens are thought to ascend the Eustachian tube to the middle ear, often following an episode of an upper respiratory tract viral infection (Chonmaitree et al., 2008). Respiratory viruses are capable of increasing the adherence of otopathogens to host cells, compromising Eustachian tube function, stimulating the immune system and inducing mucus production which may contribute to the otopathogens' ability to colonize the middle ear (Bakaletz, 2002). These otopathogens are capable of intracellular invasion and the formation of biofilm in the middle ear (Thornton et al., 2011); a matrix of extracellular DNA, polysaccharides and proteins. With these mechanisms, otopathogens are protected from antibiotic molecules and the host immune system and are able to later recolonise the middle ear, likely contributing to the recurrent or persistent nature of severe OM (Hall-Stoodley et al., 2006). Colonization with multiple otopathogens in the nasopharynx is more common in children with rAOM than in healthy children (Wiertsema et al., 2011). However, in some studies utilizing PCR, substantial proportions (18–31%) of children with AOM do not appear to have any evidence of the three major otopathogens in the middle ear fluid (Leskinen et al., 2004; Harimaya et al., 2006a; Kaur et al., 2010; Holder et al., 2012); suggesting other microbes are also involved in the pathogenesis of OM.
There are other bacterial species often isolated from the middle ear fluid of children with OM that are not currently considered major otopathogens. The two organisms most frequently reported are Alloiococcus otitidis and Turicella otitidis. These bacteria have primarily been observed in the middle ear fluid of children with OME (Ashhurst-Smith et al., 2007; Jervis-Bardy et al., 2015; Chan et al., 2016), including Indigenous Australian children; however, these organisms have also been identified as normal flora of the external auditory canal (Stroman et al., 2001). It has been speculated for over 25 years that these species are involved in the development of OM (Faden and Dryja, 1989; Funke et al., 1994), but there remains no substantial evidence describing their role, if any, in the disease.
In this review, we aim to summarize the current knowledge on these two species and their association with OM. We extensively review reports of their prevalence and abundance in the MEF from children with OM by culture, species-specific PCR and next generation sequencing methods, which have limitations in accurately estimating the abundance of these organisms. We explore the major issues with interpreting these organisms as otopathogens, including their natural colonization of the external ear canal and their relative absence in the nasopharynx; features not characteristic of major otopathogen species. We also evaluate the minimal evidence available from studies that have aimed to demonstrate a pathogenic role. As these organisms' involvement in OM currently remains equivocal we propose directions for future research that could firmly establish how these “controversial” organisms relate to the health of children with OM.
Alloiococcus otitidis
A. otitidis is a Gram positive coccus first reported as an unknown organism isolated from the MEF of children with OME in 1989 (Faden and Dryja, 1989). It was characterized as slow-growing, aerobic, catalase positive, and oxidase negative and was distinguishable from the phenotypically similar Aerococcus, Gemella, Enterococcus, and Micrococcus (Faden and Dryja, 1989). In 1992, the 16S rRNA sequence of the novel organism was analyzed and it was named Alloiococcus (“different coccus”) otitis (Aguirre and Collins, 1992). This nomenclature was later revised (von Graevenitz, 1993) to A. otitidis. Placed within the Carnobacteriaceae family, it is the only species in its genus and is most closely related to Dolosigranulum by 16S rRNA sequence homology (Vos et al., 2009). Dolosigranulum is a nasopharyngeal commensal of relevance to OM, and their similarity leads to misclassification in 16S rRNA gene surveys using older taxonomic databases (Lappan et al., 2018). A. otitidis strains have been characterized as resistant to trimethoprim-sulfamethoxazole and macrolides, and susceptible or intermediately resistant to penicillin and ampicillin (Bosley et al., 1995; Ashhurst-Smith et al., 2007; Marsh et al., 2012), though they are β-lactamase negative (Bosley et al., 1995).
Turicella otitidis
The first description of T. otitidis was in 1993, when it was independently determined that unidentified coryneforms from MEF specimens (primarily from children with AOM), whilst phenotypically similar to Corynebacterium afermentans, were biochemically distinct from this species (Funke et al., 1993; Simonet et al., 1993). The 16S rRNA sequences of these strains showed they were also phylogenetically distinct and the new genus and species T. otitidis was proposed (Funke et al., 1994). T. otitidis is a Gram positive bacillus; catalase positive, oxidase negative and aerobic (Funke et al., 1994). Like A. otitidis, T. otitidis is the only member of its genus and was placed in the Corynebacteriaceae family with its closest relative, Corynebacterium (Goodfellow et al., 2012); though it has recently been proposed that T. otitidis is a true member of the Corynebacterium genus (Baek et al., 2018). Like A. otitidis, T. otitidis' closest relative is also a nasopharyngeal commensal, and Corynebacterium and Dolosigranulum commonly co-occur in healthy children (Biesbroek et al., 2014; Lappan et al., 2018). T. otitidis is similarly misclassified as Corynebacterium in at least one ribosomal taxonomic database (Lappan et al., 2018). T. otitidisis sensitive to tetracyclines and amoxicillin, but is resistant to sulfamethoxazole and co-trimoxazole (Troxler et al., 2001) and some strains have developed mutations conferring macrolide resistance (Gómez-Garcés et al., 2004; Boumghar-Bourtchai et al., 2009).
Why Have These Organisms Been Implicated in Otitis Media?
Both Alloiococcus and Turicella were first isolated by culture from the MEF of children with OM, prompting suggestions of their association with the disease (Faden and Dryja, 1989; Funke et al., 1993; Simonet et al., 1993). This is the primary niche from which these organisms have since been consistently detected, though they have not been studied as extensively as the three major otopathogen species. A. otitidis has appeared more frequently than T. otitidis in the scientific literature, with 97 results for “Alloiococcus” and 44 for “Turicella” in the Scopus database as at 2019-02-07; this literature has been used for this review. This disparity is likely because A. otitidis can be detected by both culture and PCR methods, whereas there are currently no published primer pairs specific to T. otitidis. Additionally, cultured T. otitidis isolates are not often distinguished from commensal skin coryneforms (von Graevenitz and Funke, 2014). Table 1 summarizes the studies that have evaluated the prevalence of A. otitidis in MEF by either microbiological culture or targeted PCR, including the OM phenotype and extent of ear canal avoidance for each. Few studies describe the prevalence of T. otitidis (see section T. otitidis Has Been Detected in MEF by Culture, But Is Seldom Reported).
A. otitidis Can Be Difficult to Detect by Culture
A. otitidis was originally detected by microbiological culture from MEF and was thus implicated as a cause of OME. However, it has remained a challenging organism to grow, requiring extended incubation times and producing small colonies (Ashhurst-Smith et al., 2007) so it may have been overlooked as an otopathogen in early studies. At its discovery, it was successfully cultured at 37°C with 5% CO2 on blood agar for 2–5 days (Faden and Dryja, 1989). Subsequent studies apparently failed to culture the organism, with rates of 0% reported until 2007 when Ashhurst-Smith et al. (2007) successfully cultured the organism in 40% of MEF samples using a slightly lower temperature (35°C) and higher CO2 (7.5%). Only six studies since the 1989 paper have reported the prevalence of A. otitidis in MEF by culture; five cultured it from 1 to 40% of samples from children with OME or COME (Ashhurst-Smith et al., 2007; de Miguel Martínez and Ramos Macías, 2008; Khoramrooz et al., 2012; Garibpour et al., 2013; Sheikh et al., 2015) and one cultured it from 46% of qPCR-positive otorrhoea swabs from children with AOM and perforation (Marsh et al., 2012). Due to the varying culture conditions used to isolate the organism (see Table 1) and the potential for overgrowth of other organisms to mask the presence of small A. otitidis colonies, it is not clear whether the prevalence of A. otitidis by culture is comparable to that of the three major otopathogens. A clearer picture can be obtained from those studies that have detected these organisms via species-specific PCR.
A. otitidis Is Commonly Detected in MEF by PCR
In 1997, A. otitidis was incorporated as part of a multiplex PCR method with the three major otopathogens, NTHi, S. pneumonia, and M. catarrhalis (Hendolin et al., 1997). The use of PCR has substantially improved the detection rate of these four organisms in MEF specimens compared to microbiological culture, as demonstrated by studies where both methods were used (Hendolin et al., 1997, 1999; Leskinen et al., 2002; Pereira et al., 2004; Harimaya et al., 2006a; Kaur et al., 2010; Aydin et al., 2012; Khoramrooz et al., 2012; Sheikh et al., 2015; Sillanpää et al., 2016; Slinger et al., 2016). In children with OME or COME, A. otitidis has been reported by PCR in 18.5–60.5% of MEF specimens (13 studies), and in children with AOM or rAOM, 7–50% (5 studies). Two studies compared the prevalence of A. otitidis in purulent and non-purulent MEF; A. otitidis was detected in 18.4 and 22.6% of purulent fluids, and in 25.4 and 25.7% of non-purulent fluids (Holder et al., 2012, 2015). However, there were 4–8 times as many non-purulent fluids tested in these studies as purulent fluids. One study has measured the bacterial load of A. otitidis in children with AOM with perforation and demonstrated that it was present at a comparable load to H. influenzae, the most dominant otopathogen (Marsh et al., 2012); but this is the only study to have done so.
The information currently available on the prevalence of A. otitidis suggests it is more commonly associated with persistence (OME) than acute infection (AOM). The within study-comparisons of purulent and non-purulent fluid support this (Holder et al., 2012, 2015), though no other studies have made a direct comparison between phenotypes and the difference in proportions is not large. It therefore may be hypothesized that the role of A. otitidis in OM is in the perpetuation of inflammation rather than as a direct pathogenic cause of acute infection. Further studies are needed to test this hypothesis, however some caution is required as the apparent association may be due to publication bias with few reported studies having sought to detect A. otitidis in children with AOM. Importantly, the prevalence and abundance of A. otitidis in MEF is possibly overestimated due to its habitation of the external ear canal. Several of the studies in Table 1 either did not describe measures that were taken to avoid contamination with the ear canal, disinfected it only with 70% alcohol (which does not destroy DNA) or included children whose tympanic membranes had previously been breached.
Of the 16 studies summarized in Table 1 that have reported the prevalence A. otitidis, S. pneumoniae, NTHi, and M. catarrhalis by species-specific PCR, A. otitidis was detected more frequently than each of the major otopathogens in 9 of them (for two of these studies, in non-purulent fluid only). Table 2 describes those studies that have reported whether A. otitidis occurs alone or is detected together with the major otopathogens. Both scenarios are reported, with A. otitidis, present in the fluid-filled middle ear with and without the presence of the major otopathogen species. Therefore, if A. otitidis is involved in the pathogenesis of OM, it may directly cause disease or it may contribute by supporting the main otopathogens in some way.
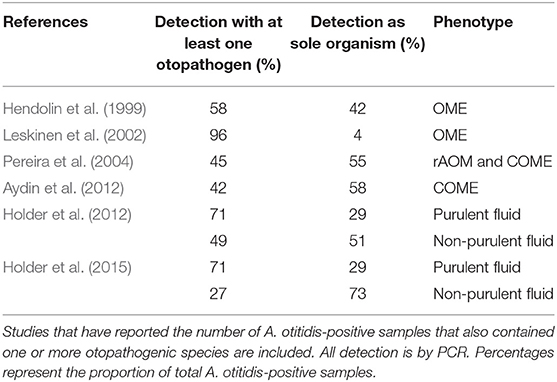
Table 2. Distribution of A. otitidis detected with at least one otopathogen species or as the sole detected organism.
T. otitidis Has Been Detected in MEF by Culture, but Is Seldom Reported
Detection of T. otitidis has been described infrequently in the literature. This is likely due to the difficulty in phenotypically distinguishing it from other coryneforms, which are often considered contaminants and do not appear to be routinely identified to species level (von Graevenitz and Funke, 2014). Additionally, no PCR primers have been described in the literature for the detection of T. otitidis, so it has only been detected by standard culture methods and next generation sequencing. Like A. otitidis, it has primarily been observed and reported in the MEF of children with OM, so both organisms may have been overlooked as otopathogens.
Since original detection of the organism in MEF in two independent studies (Funke et al., 1993; Simonet et al., 1993), very few studies have reported detection of T. otitidis by culture. Renaud et al. (1996) reported that otorrheal fluid from a child with a perforated tympanic membrane produced several colonies of T. otitidis (Renaud et al., 1996). Its prevalence appears to be low, with 7/112 (6.3%) of MEF samples in a 2004 study producing T. otitidis colonies (Gómez-Garcés et al., 2004). In 5 of these 7 samples the children had perforations and the MEF was collected from the external ear canal. One study has assessed the prevalence of T. otitidis where perforations were not present, reporting it in 10% of MEF samples from children with COME, compared to the major otopathogens at 3.3–8.3% (Holzmann et al., 2002). In this study, where T. otitidis was detected in the MEF, it was also detected in the external auditory canal; though the canal was disinfected between sampling each site. As we describe later in this review, the contribution of canal flora is challenging to tease out from the detection of both A. otitidis and T. otitidis in the MEF.
Detection of A. otitidis and T. otitidis in MEF Has Increased With Next Generation Sequencing
In recent years, several studies have utilized next generation DNA sequencing to study the whole bacterial community present in MEF specimens (Table 3). By amplifying and sequencing a segment of the 16S rRNA gene, the microbial profile (or microbiome) can be characterized, including bacteria that are challenging to isolate in culture or are not targeted by commonly-used species-specific PCRs. The advantage of this method is that these potentially overlooked organisms can not only be detected, but their relative abundance in a sample can be estimated and compared between samples. However, the major disadvantage is that only part of the gene is sequenced, providing little to no taxonomic resolution beyond the genus level (or broader, depending on the taxa and the region sequenced).
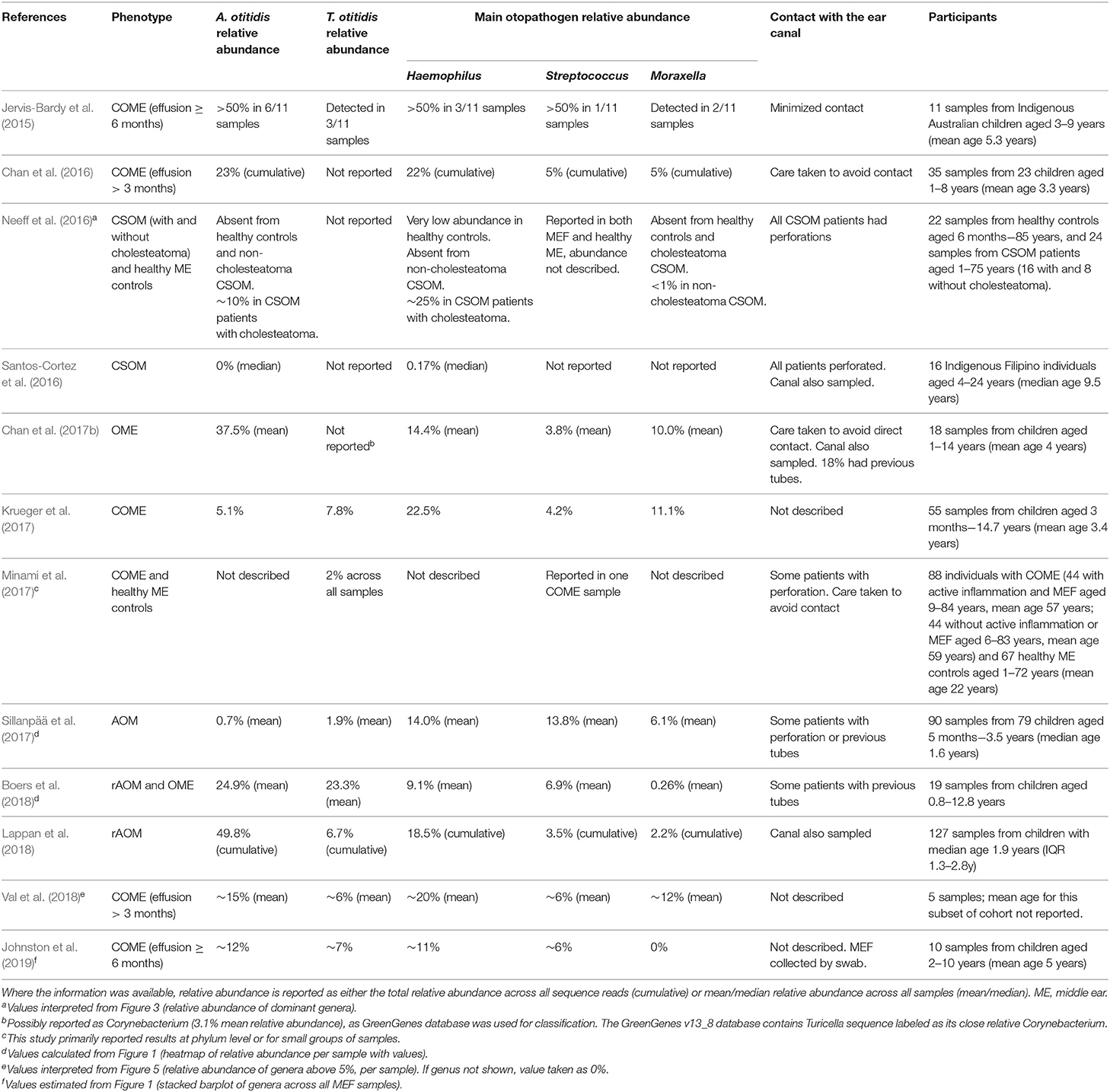
Table 3. Genus-level relative abundance of A. otitidis and T. otitidis in MEF specimens by 16S rRNA gene sequencing.
In the microbiota of MEF, Alloiococcus appears to be one of the dominant genera (Jervis-Bardy et al., 2015; Chan et al., 2016, 2017b; Boers et al., 2018; Lappan et al., 2018; Val et al., 2018; Johnston et al., 2019). Turicella is less often reported, but has been identified as a member of the MEF microbiota in 8 of the 12 MEF microbiota studies reported in Table 3 (Jervis-Bardy et al., 2015; Krueger et al., 2017; Minami et al., 2017; Sillanpää et al., 2017; Boers et al., 2018; Lappan et al., 2018; Val et al., 2018; Johnston et al., 2019). However, due to the compositional nature of microbiome data it remains difficult to interpret which organisms are more abundant than others. Microbiome composition may also vary with the efficacy of the chosen DNA extraction method and amplicon primers on different organisms, and the variation in numbers of copies of the 16S rRNA gene amongst different bacterial genera; qPCR is still a more reliable indicator of whether there are greater numbers of Alloiococcus than other organisms within the same sample.
What Does Their Presence in MEF Tell us?
A. otitidis, and to a lesser extent T. otitidis, are prevalent in MEF specimens from children with OM. A. otitidis in particular is often detected at a similar or higher frequency to the three major otopathogen species. These two organisms may have been overlooked as potential otopathogens as they can be challenging to detect, though studies of the microbiome of MEF have revealed that they are not insignificant members of the microbiota of the otitis-prone middle ear. However, the observations from the studies summarized thus far only describe their prevalence and abundance, and do not assess their potential role in the pathogenesis of OM.
Why Is the Role of These Organisms in Otitis Media Still Under Debate?
Despite their prevalence in the MEF of children with OM, the role of A. otitidis and T. otitidis as potential otopathogens remains under debate. This is mostly due to two complicating factors: their presence in the external auditory canal (EAC), where the major otopathogens are rarely observed; and their infrequency in the nasopharynx, the site where major otopathogens initially colonize before ascension via the Eustachian tube to the middle ear.
A. otitidis and T. otitidis Are Found in the External Auditory Canal
When first discovered, A. otitidis was reported not to be found in the EAC (Faden and Dryja, 1989), but both A. otitidis and T. otitidis have since been proposed as members of the normal EAC flora. Both organisms have been observed in the EACs of healthy adults and children (Stroman et al., 2001; Frank et al., 2003; Tano et al., 2008; De Baere et al., 2010), indicating that they are capable of growth in the EAC without causing disease. Given this finding, it becomes important to establish whether the detection of A. otitidis and T. otitidis in MEF is a result of contamination during sampling, or if they inhabit both sites. Currently, this is difficult to determine as only three studies, to our knowledge, have been in a position to address this question by looking for these organisms in EAC and MEF samples from the same ear in children with OM and intact tympanic membranes.
A 2002 study cultured T. otitidis from the EAC and MEF of children with OME, and found that the frequency of T. otitidis was higher in the EAC of children with OME (23%) than in healthy control children (11%) (Holzmann et al., 2002). Additionally, they noted that it was never isolated from the MEF alone; every MEF in which it was present had a positive result for the corresponding EAC sample. Because of this finding, they suggested that it resides only in the EAC, noting that many studies observing T. otitidis in MEF did not look for it in the EAC and that this was the likely source.
A recent study by Chan et al. (2017b) using 16S rRNA amplicon sequencing on samples from the EAC and MEF of children with OME did not observe T. otitidis, but reported A. otitidis as the most abundant species in both sites. They noted its high abundance in children who had previously had grommets, and suggested that the EAC may serve as a reservoir of infection for the middle ear via a tympanic membrane perforation. This study detailed their care in sampling the MEF without touching the EAC and concluded it unlikely that the abundance of A. otitidis in the MEF was the result of contamination from the EAC during sampling.
We recently took a similar approach, undertaking 16S rRNA amplicon sequencing on EAC and MEF samples from children with rAOM (Lappan et al., 2018). We observed A. otitidis and T. otitidis as dominant organisms in both sites in a cohort where the majority of children had no known current tympanic membrane perforations or previous grommets. While it is unlikely that these children had suffered tympanic membrane perforations, a perforation history was not obtained. The relative abundance of both A. otitidis and T. otitidis was significantly higher in the EAC than in the MEF. As with the Chan et al. study, care was taken to ensure the MEF was sampled without touching the EAC, however as we did not identify any bacteria unique to the EAC we could not rule out contamination of the MEF during sampling.
Based on the studies above, it is plausible that these organisms are present in both sites, but the evidence currently suggests that their primary habitat is the EAC. In support of this possibility, other studies have detected A. otitidis and T. otitidis in the EAC by culture and PCR, commonly observing a higher frequency in the EAC of children with OM than in healthy children (Gómez-Hernando et al., 1999; Durmaz et al., 2002; Holzmann et al., 2002). Tano et al. found A. otitidis more frequently in the EAC of healthy individuals (29%) than patients with OM (6%), but sampled from adults and children and did not report these results separately (Tano et al., 2008). In the EAC of healthy children and adults, Stroman et al. (2001) cultured A. otitidis as the most common streptococci/enterococci-like organism, and T. otitidis as the dominant coryneform. While most of the A. otitidis isolates were from children, it was also detected in adults and T. otitidis isolates were found across both age groups. Frank et al. (2003) undertook a similar assessment of EAC samples from adults and children using 16S rRNA cloning and sequencing. They observed A. otitidis and T. otitidis (referred to as Corynebacterium otitidis) as the most prevalent sequence types, representing 57 and 20% of all clones, respectively, and with A. otitidis common in children. A more recent study also suggested that the organisms are not specific to children, detecting A. otitidis by PCR in 83% of EAC samples from healthy young adults, where T. otitidis was also cultured from 5 of 10 randomly selected samples (De Baere et al., 2010).
It therefore seems likely that A. otitidis and T. otitidis found in MEF originate from the EAC; but if these organisms do colonize the middle ear, their mechanism of entry remains unknown. It has been hypothesized that these organisms could enter the middle ear through a perforation in the tympanic membrane (De Baere et al., 2010; Marsh et al., 2012; Chan et al., 2017b). However, there are some studies that have reported the detection of A. otitidis in children with intact tympanic membranes when care is taken to avoid contact with the EAC (Leskinen et al., 2002, 2004; de Miguel Martínez and Ramos Macías, 2008; Khoramrooz et al., 2012). It is possible that small perforations sufficient for bacterial entry may not be detected by parents or physicians; and perhaps inflammation of the middle ear increases permeability of the tympanic membrane. However, understanding their entry into the middle ear does not indicate whether they are responsible for causing disease once inside. The sparsity of the major otopathogens in the EAC compared to A. otitidis and T. otitidis (Stroman et al., 2001; Frank et al., 2003; De Baere et al., 2010; Chan et al., 2017b; Lappan et al., 2018) indicates that A. otitidis and T. otitidis either have a different pathway for involvement in OM compared to the major otopathogens, or they are not otopathogenic organisms.
They Are Infrequently Found in the Nasopharynx
A. otitidis and T. otitidis show dissimilarity to the major otopathogens not only because they are found in the EAC, but also because they are rarely found in the nasopharynx. It is commonly accepted that the major otopathogens colonize the nasopharynx before ascension to the middle ear and subsequent infection (Chonmaitree et al., 2008). The low detection rates of A. otitidis and T. otitidis in the nasopharynx are another indicator that these organisms behave differently compared to the major otopathogens.
A. otitidis has infrequently been reported in the nasopharynx, and then only by PCR. The first study to do so was Durmaz et al. (2002), where it was detected in 15% of 27 nasopharyngeal samples from children with chronic OM (Durmaz et al., 2002). It has been observed more frequently in the nasopharynx of children with a history of otitis media episodes (>3 in 6 months, >4 in 12 months, or > 4 by 2 years of age; 29.4%) than those with fewer episodes (2.6%) (Harimaya et al., 2006b). It was also observed in 9% of nasopharyngeal samples from children with AOM (Kaur et al., 2010) and in 7% of children with an upper respiratory tract infection (Tano et al., 2008). It is very rare in the nasopharynx of healthy children, seen in 0.5% in one study (Janapatla et al., 2011) whilst in others was not detected (Durmaz et al., 2002; Aydin et al., 2012). It was also found to be absent from the nasopharynx of healthy young adults (De Baere et al., 2010). Where these studies also observed A. otitidis in the MEF or ear canal, it was found more commonly in these sites than in the nasopharynx (Harimaya et al., 2006b; Tano et al., 2008; De Baere et al., 2010; Kaur et al., 2010; Aydin et al., 2012), with one exception (Durmaz et al., 2002). A. otitidis also appears to be absent, or has been detected at very low abundance, on the tonsils (Aydin et al., 2012) and adenoids (Khoramrooz et al., 2012; Jervis-Bardy et al., 2015; Chan et al., 2016) of children with OME, suggesting that these lymphatic tissues are not a source of the organism in the middle ear. To our knowledge, T. otitidis has not been reported in the nasopharynx by culture or by PCR.
Both A. otitidis and T. otitidis have occasionally been detected in the nasopharynx by 16S rRNA amplicon sequencing. However, both organisms are likely to be misclassified depending on the reference database used. A. otitidis was reported in the nasopharynx of healthy infants (Teo et al., 2015) and in sinonasal swabs from adults with chronic rhinosinusitis (Lal et al., 2017), however both studies used the GreenGenes 13_8 taxonomic database for classification. This database does not contain Dolosigranulum, a nasopharyngeal commensal, and will misclassify it as Alloiococcus. These studies may similarly have not been able to detect Turicella in the nasopharynx as the GreenGenes database will report it as Corynebacterium. Similarly, nasopharyngeal microbiome studies that aggregate taxa at broader levels will have missed these genera. In 16S rRNA studies that did not use GreenGenes, Alloiococcus, and Turicella were not reported in the nasopharynx of children with OM (Laufer et al., 2011; Pettigrew et al., 2012; Jervis-Bardy et al., 2015). Alloiococcus was present at very low relative abundance in nasopharyngeal samples from children with rAOM (0.19% across all samples) and in healthy controls (0.17%) in our own study—significantly lower abundance than in the MEF (49.8%) (Lappan et al., 2018). Turicella was similarly present at 0.03% in the nasopharynx of both groups of children, again significantly lower than in the MEF (6.7%) (Lappan et al., 2018). In the nasopharynx, both genera were present at a similar level to known contaminants in negative control samples, indicating that caution should be taken to interpret the organisms as present in the nasopharynx.
These studies indicate that if A. otitidis and T. otitidis are present in the nasopharynx of children with OM then it is only at low abundance; they appear to be even more scarce in the nasopharynx of healthy individuals. The nasopharynx is therefore unlikely to represent a reservoir for these organisms, or their presence there is transient and potentially originates from the middle ear. If they do not originate from the nasopharynx, then it is also unlikely that they follow the same pathway as the major otopathogens to the middle ear. This may be because these organisms are indeed not otopathogens, or it may be because the EAC, rather than the nasopharynx, is their predominant niche. The studies summarized so far do not provide any causal or mechanistic link between the presence of these organisms and the development of OM.
Are These Organisms Capable of Causing Disease?
In addition to understanding their mechanism for colonizing the middle ear (and indeed confirmation that they are not simply contaminants from the EAC introduced during sampling), the other aspect of A. otitidis and T. otitidis' ecology that we lack is an understanding of whether they are capable of causing disease. Very few studies have attempted to investigate the behavior and potential pathogenic activity of these two species, with all published articles that have investigated this issue focusing on A. otitidis. Evaluating their pathogenic potential is an integral part of understanding whether these organisms are causative of OM, if they contribute indirectly to pathogenesis, or whether they are aural commensals that are not involved in the pathogenesis of OM.
A. otitidis Provokes an Immune Response
There are a handful of studies that have assessed the ability of A. otitidis to elicit an immune response, as a proxy measure to indicate pathogenic potential. Initial studies assessed the production of interleukins (IL) from THP-1 cells (IL-8 and IL-12) and Hep-2, HeLa and U937 cells (IL-8) in response to A. otitidis. These studies demonstrated that viable A. otitidis was capable of stimulating IL-8 and IL-12 at similar levels to the three major otopathogens (Himi et al., 2000; Kita et al., 2000). The effect was reduced when cells were stimulated with whole killed A. otitidis and when viable cells were prevented from direct contact, but soluble proteins from A. otitidis elicited a response for both cytokines (Himi et al., 2000; Kita et al., 2000). Whole killed A. otitidis can also induce expression of CD69 (indicating activation of lymphocytes) in blood and adenoidal lymphocytes at comparable levels to the major otopathogens (Harimaya et al., 2005), but the response is lower than that induced by Staphylococcus aureus (Tarkkanen et al., 2000). Whole killed A. otitidis has also been shown to activate signaling pathways for the production of IL-8, similarly to S. pneumoniae (Harimaya et al., 2007a). Formalin-killed cells from clinical isolates of A. otitidis have induced IL-1β, IL-6, IL-8, and TNF-α on a cell line in vitro at or above levels induced by S. pneumoniae (Ashhurst-Smith et al., 2014a). An experiment with cell-free filtrates from these isolates indicated that extracellular proteins rather than peptodiglycan may be responsible for the induction (Ashhurst-Smith et al., 2014b).
The production of pro-inflammatory cytokines provides preliminary evidence that the human immune system responds to A. otitidis. However, these studies lack replication in a middle ear epithelial environment, have not involved comparisons with non-pathogenic bacteria, and are potentially strain-dependent. For example, some strains of the nasopharyngeal commensal Haemophilus haemolyticus have been shown to elicit an inflammatory response in vitro (Pickering et al., 2016). It remains unclear from these studies whether these inflammatory responses are characteristic of the response in the middle ear of children with OM, and whether they are evidence for pathogenicity.
Two further studies by Harimaya et al. have explored the immune response to A. otitidis within the middle ear. In 2007, they determined that MEF from children with A. otitidis-positive OM (in the absence of other detectable otopathogens) contained IgG, secretory IgA, IgG2, and IgM specific to A. otitidis (Harimaya et al., 2007b). In 2009, they also discovered that A. otitidis-positive MEF contained similar levels of pro-inflammatory cytokines and chemokines as S. pneumoniae-positive MEF (both in the absence of other otopathogens detectable by culture or PCR) (Harimaya et al., 2009). While these preliminary studies require replication, it is important to note that A. otitidis does appear to elicit an innate and adaptive immune response in the middle ear of children with OM.
Observations of Bacterial Activity
There is also some limited evidence for Alloiococcus having pathogenic potential based on how the organism behaves. Upon its initial discovery, Alloiococcus was reported to be found intracellularly, which the authors suggested was an indication of pathogenic capability (Faden and Dryja, 1989); though this may have been an observation of engulfment by neutrophils. Two reports of intracellular bacteria found in the middle ear mucosa described Gram positive cocci (Coates et al., 2008) and other, unidentified bacteria (Thornton et al., 2011), though no studies since have indicated the presence of intracellular Alloiococcus. In children with AOM and perforation, the Alloiococcus bacterial load in MEF was comparable to H. influenzae (Marsh et al., 2012). A. otitidis has been observed more frequently than the major otopathogens in non-purulent MEF (Holder et al., 2012, 2015) and more commonly in persistent (≥3 month duration) effusions than those of shorter duration (Leskinen et al., 2002), suggesting involvement in chronicity. It is similarly prevalent in purulent effusions (Holder et al., 2012, 2015), though its presence in the MEF of children with AOM appears to be unrelated to disease severity (Leskinen et al., 2004). A. otitidis is strictly aerobic, as its viability decreases greatly when grown anaerobically (Matsuo et al., 2011). Its presence in middle ear fluids is therefore somewhat unexpected, as the fluid-filled middle ear in OM is thought to be hypoxic (Cheeseman et al., 2011). Interestingly, the organism has recently been shown to form biofilms in broth culture, both with H. influenzae and independently as a single-species biofilm (Chan et al., 2017a). Furthermore, A. otitidis improved the growth of H. influenzae when in media without its required X and V factors and at suboptimal temperatures, suggesting its enhancement of biofilm production by otopathogens may allow A. otitidis to indirectly contribute to the pathogenesis of OM. This multispecies biofilm was also less susceptible to antimicrobial killing, and it is possible that A. otitidis' ability to also form biofilm alone may contribute to the perpetuation of inflammation and chronic OM (Chan et al., 2017a). Understanding whether A. otitidis DNA detected in MEF originates from live or dead cells, and whether residence in biofilm improves its tolerance to low oxygen conditions would be highly informative regarding its persistence in the middle ear.
For Turicella, very little has been investigated with respect to pathogenicity other than its resistance to antimicrobials. Turicella strains have shown resistance to macrolides and clindamycin (Gómez-Garcés et al., 2007; Boumghar-Bourtchai et al., 2009), though are susceptible to amoxicillin-clavulanic acid (Funke et al., 1996; Troxler et al., 2001) which is more commonly used to treat AOM (Antibiotic Expert Group, 2014). The draft genome sequence of a strain isolated from MEF of a child with OM apparently did not contain any candidate virulence factors, but no detail was given as to how these were sought (Brinkrolf et al., 2012). We therefore currently have minimal evidence for the pathogenicity of T. otitidis.
Assessment in Animal Models
To our knowledge, there has only been one published study investigating Alloiococcus in an animal model to evaluate pathogenicity, and none investigating Turicella. In 2008, Tano et al. inoculated >108 CFU/ml of Alloiococcus into the middle ear of 7 rats (a 10-fold higher concentration than required of the major otopathogens) (Tano et al., 2008). Reactions were mild; after 3 days, all rats had an amber-colored (but not purulent) effusion in the middle ear but by day 14 all ears were normal. In conjunction with their observations that Alloiococcus was found in the EAC of healthy adults and rarely in the nasopharynx of children, Tano et al. concluded that Alloiococcus is not an otopathogen. This study requires replication, though it suggests that A. otitidis is not overtly pathogenic on its own; an observation consistent with the association of A. otitidis with OME and persistent inflammation rather than AOM. However, effective animal models of AOM often make use of a viral infection to predispose the animal to AOM, as occurs in humans (Davidoss et al., 2018); so it remains possible that A. otitidis would produce or exacerbate disease in the presence of a co-infecting virus or organism. This would be congruent with the observations of coinfection summarized in Table 2, and with A. otitidis' ability to enhance the survival of NTHi in biofilm (Chan et al., 2017a).
Pathogenicity in Diseases Other Than Otitis Media
There have been isolated reports of A. otitidis and T. otitidis as causative agents of diseases seemingly unrelated to otitis media. A. otitidis has been described as the causative agent in two case reports, where it was isolated from vitreous fluid of a patient with acute-onset endopthalmitis (Marchino et al., 2013) and from blood cultures in a young adult with endocarditis (Guler et al., 2015). Both infections are usually caused by the Gram positive cocci Streptococcus and Staphylococcus (Marchino et al., 2013; Guler et al., 2015); it is possible that the cause was misidentified as these reports do not provide any detail on how the isolates were identified as A. otitidis. In the endocarditis case, the patient had chronic OME with perforation which had apparently been a problem since childhood, so it is possible that in long-term severe disease A. otitidis can opportunistically infect the bloodstream. T. otitidis has similarly had few instances of unrelated opportunistic infection. Two case reports isolated T. otitidis from the blood of an immunocompromised child (Loïez et al., 2002) and an elderly hospitalized patient (Bîrlutiu et al., 2017) with bacteraemia. In the case of the child, otitis externa was apparently present at the time and T. otitidis was also isolated from an external ear swab (Loïez et al., 2002). It has also been isolated in pure culture from a posterior auricular abscess (Reynolds et al., 2001) and a case of mastoiditis (Dana et al., 2001), both in young children. The T. otitidis strain TD1, whose genome was recently sequenced (Greninger et al., 2015), was isolated from a venous catheter. It is therefore plausible that the genomic contents of this strain are not representative of strains involved in OM.
Thus, there is weak evidence for both A. otitidis and T. otitidis to have opportunistic pathogenicity outside of the ear; these reports are infrequent and not replicated. Samples taken from in or around the ear have the potential to have been contaminated with the organisms from the EAC; outside of these few case reports these species have only been reported in the middle or external ear. It is unknown how these organisms would migrate to the blood, and it is likely that the connection between these patients' ear disease and bacteraemia is a result of sample contamination or transient bacteraemia rather than invasive infection by these organisms.
These sparse reports of pathogenic activity are in contrast to the major otopathogens, each of which can reside asymptomatically but are also responsible for a wide range of infections (Verduin et al., 2002; Bogaert et al., 2004; Van Eldere et al., 2014). Overall, there is currently minimal evidence for the pathogenicity of A. otitidis and T. otitidis as the immunological and animal studies lack a comparison to commensal organisms known not to cause OM, and the work is lacking replication.
Summary and Future Directions
What we Currently Know
Our summary of the literature available at the time of writing suggests that A. otitidis and T. otitidis are primarily organisms of the human ear. They are found abundantly in both the MEF and EAC of children with OM, but are also present in the EAC of healthy children and adults. Current evidence suggests that A. otitidis and T. otitidis in MEF may originate from the EAC as they are uncommonly found in the nasopharynx. This pattern of detection is opposite to what is commonly observed of the major otopathogens, which typically colonize the nasopharynx to ascend to the middle ear and are rarely found in the EAC. Curiously, the closest relatives of Alloiococcus and Turicella are Dolosigranulum, and Corynebacterium, respectively; both organisms being usual nasopharyngeal commensals. These organisms may have diverged to inhabit the middle ear and the nasopharynx, where they have potentially developed specialized but very different interactions with the other dominant organisms in these niches (the otopathogen genera).
The majority of available studies on A. otitidis and T. otitidis only hypothesize their role in the pathogenesis of OM by describing their prevalence and abundance, but relatively few studies set out to test these hypotheses to better define their role in disease. Thus, we have minimal information on whether or how these organisms contribute to the development of OM.
The few studies that have aimed to directly investigate pathogenic potential have tested only A. otitidis. There is evidence that it is capable of forming biofilm and supporting the survival of NTHi, stimulating release of pro-inflammatory cytokines in vitro, and stimulating the production of specific antibodies. However, these studies have compared A. otitidis only with the major otopathogens and not with known commensal species; it is possible that a commensal organism would still elicit these responses. There is still much that is unknown about the role of A. otitidis and T. otitidis in the pathogenesis of OM, and a targeted research effort is required to further characterize their function.
Designing Future Studies to Clarify Their Role in Otitis Media
Even after more than 25 years since their discovery, there is much about the behavior of A. otitidis and T. otitidis in the middle ear and EAC that remains unknown. Firstly, while there are many studies describing their prevalence in the MEF of children with OM, and some in the EAC, these studies are often confounded with the possibility of EAC flora contaminating MEF samples during collection. However, several studies have reported the presence of A. otitidis or T. otitidis in specimens from children in which tympanic membrane perforations (including previous grommets) had not occurred (Hendolin et al., 1999; Leskinen et al., 2002, 2004; de Miguel Martínez and Ramos Macías, 2008; Khoramrooz et al., 2012). This suggests three possibilities: 1) A. otitidis and T. otitidis inhabit the normal middle ear, prior to the onset of OM; 2) they enter the middle ear through undetectable, minor tympanic membrane perforations; 3) they are detectable in MEF specimens as a result of contamination from the EAC during sampling.
The first possibility is challenging to address, as the healthy middle ear is a very low biomass environment, where samples are highly prone to environmental contamination and the area is inaccessible without surgery. Some attempts have been made to survey the microbiota of the healthy middle ear, and these studies have not consistently reported the presence of A. otitidis and T. otitidis (Antonelli and Ojano-Dirain, 2013; Neeff et al., 2016; Minami et al., 2017) and some have not found evidence for the presence of bacteria in the normal middle ear at all (de Miguel Martínez and Ramos Macías, 2008; Westerberg et al., 2009; Thornton et al., 2011; Papp et al., 2016). The second possibility is also challenging, but may be explored with animal or tissue models of an inflamed tympanic membrane to test its permeability to bacteria. These will also be important studies if it is found that viable A. otitidis and T. otitidis in the MEF originate from the EAC, even in children with intact tympanic membranes. The final possibility of MEF contamination is the most straightforward to test, and these experiments are also useful for addressing the second possibility. A well-designed study aiming to isolate viable A. otitidis and T. otitidis from the two sites independently is required. This may be achieved by sampling the EAC, sterilizing it thoroughly, sampling it again, and then opening the tympanic membrane to sample the MEF with all care taken not to contact the EAC. This should be carried out in children who have never had a known tympanic membrane perforation as well as those who have, or have had tympanostomy tubes in the past. Such a study would also be well-positioned to answer the subsequent question, addressing the minor perforation hypothesis: if these organisms reside in the MEF, how do they get there? The isolates from the EAC and MEF of the same ear may be phenotypically or genetically compared to determine if the EAC is the likely origin of the isolates from the MEF. Higher similarity between EAC and MEF strains of the same patient than between strains from different patients may indicate the transferral of strains between the EAC and MEF in the absence of sampling contamination. Metagenomics is also a useful tool for answering this question if isolation of live bacteria is a challenge (which could be informative in itself). Metagenomic analysis would also allow for the characterization of other EAC community members to aid in the assessment of contamination and strain relatedness between the EAC and MEF communities. Bacterial load estimation via species-specific qPCR would also be useful to determine whether there is a larger population of A. otitidis and T. otitidis in the EAC or in the MEF, keeping in mind that there may be differences in the live and dead populations of A. otitidis and T. otitidis. Longitudinal sampling of the EAC may also indicate whether the presence or abundance of these organisms in the EAC is associated with episodes of OM. These experiments should provide a solid body of evidence to a) show whether A. otitidis and T. otitidis do inhabit the MEF and are not present due to contamination; and b) whether they enter the middle ear from the EAC, and how they do so.
The second major gap in current knowledge is that the functions these organisms perform in the EAC and MEF is entirely unknown. To begin to understand the behavior of these organisms in the middle ear, metagenomics and other new “'omics” techniques may be able to answer many of these questions. The genomes of A. otitidis and T. otitidis isolates are currently in draft stage; complete, annotated genomes will allow us to understand whether they contain genes that may allow them to cause disease. The assembly of many additional genomes will aid an understanding of their metabolic functions and strain heterogeneity Transcriptomics and proteomics analysis of MEF could directly indicate their activity in the inflamed middle ear; this could be compared to their activity in the EAC. In vitro analyses making use of middle ear epithelial cell lines could confirm the pro-inflammatory stimulation by A. otitidis, and T. otitidis could be studied in this manner as well. These experiments would also be useful to determine whether the species are capable of residing intracellularly in the middle ear mucosa, as a mechanism for immune or antibiotic evasion. Further work with animal models would also fill gaps in our knowledge of the behavior of A. otitidis and T. otitidis in the middle ear, perhaps allowing us to determine if the presence of these organisms in OM caused by the major otopathogens results in a more severe phenotype. With these models, we may determine whether these organisms can produce OM on their own, or whether the presence of otopathogens or viruses is required. It can be assessed whether the phenotype is more severe in the presence of A. otitidis or T. otitidis. Understanding what functions these organisms perform in the MEF is essential to understand whether they play a pathogenic role, by themselves or in synergism with the otopathgoens.
Conclusion
Research focusing on the development of new therapies to treat severe OM has focused on the major otopathogens; with advances in the areas of vaccinations, tackling biofilm and restoring the commensal flora of the nasopharynx. Despite these advances in prevention and treatment, OM remains a very common childhood disease that can be difficult to treat. The complex polymicrobial nature of the disease and the role dominant organisms like A. otitidis and T. otitidis may play has remained equivocal for decades. The current understanding of these organisms is limited, and there are many ways in which they can be further characterized. It is important to understand their role, if any, in the pathogenesis of OM, as it is plausible that they are supporting otopathogen growth or contributing to the persistence and recurrence of chronic OM; potentially entering the middle ear through minor tympanic membrane perforations. These organisms may be useful targets for treatment, which are perpetually overlooked as their commensal residence in the external ear canal continues to confound efforts to understand their pathogenicity. Establishing their role in the otitis-prone middle ear will allow the advancement of new therapies, or will ensure that resources are not overspent investigating commensals that are uninvolved in the disease.
Author Contributions
RL undertook the literature review and drafted the manuscript. SJ and CP provided critical feedback on the manuscript. All authors have read and approved the final manuscript.
Funding
RL was supported by an Australian Government Research Training Program Scholarship and a top-up scholarship from the Wesfarmers Centre of Vaccines and Infectious Diseases, Telethon Kids Institute.
Conflict of Interest
The authors declare that the research was conducted in the absence of any commercial or financial relationships that could be construed as a potential conflict of interest.
Acknowledgments
The authors would like to acknowledge Professor Alexander von Graevenitz, who kindly airmailed a copy of his article for inclusion in this review: (Funke et al., 1993).
References
Aguirre, M., and Collins, M. D. (1992). Phylogenetic analysis of Alloiococcus otitis gen. nov., sp. nov., an organism from human middle ear fluid. Int. J. Syst. Evol. Microbiol. 42, 79–83. doi: 10.1099/00207713-42-1-79
Antibiotic Expert Group (2014). Therapeutic Guidelines: Antibiotic. Version 15. Melbourne, VIC: Therapeutic Guidelines Limited.
Antonelli, P. J., and Ojano-Dirain, C. P. (2013). Microbial flora of cochlear implants by gene pyrosequencing: Otol. Neurotol. 34, e65–e71. doi: 10.1097/MAO.0b013e3182941101
Ashhurst-Smith, C., Hall, S. T., Burns, C. J., Stuart, J., and Blackwell, C. C. (2014a). In vitro inflammatory responses elicited by isolates of Alloiococcus otitidis obtained from children with otitis media with effusion. Innate Immun. 20, 320–326. doi: 10.1177/1753425913492181
Ashhurst-Smith, C., Hall, S. T., Burns, C. J., Stuart, J., and Blackwell, C. C. (2014b). Induction of inflammatory responses from THP-1 cells by cell-free filtrates from clinical isolates of Alloiococcus otitidis. Innate Immun. 20, 283–289. doi: 10.1177/1753425913490535
Ashhurst-Smith, C., Hall, S. T., Walker, P., Stuart, J., Hansbro, P. M., and Blackwell, C. C. (2007). Isolation of Alloiococcus otitidis from Indigenous and non-Indigenous Australian children with chronic otitis media with effusion. FEMS Immunol. Med. Microbiol. 51, 163–170. doi: 10.1111/j.1574-695X.2007.00297.x
Aydin, E., Taştan, E., Yücel, M., Aydogan, F., Karakoç, E, Arslan, N., et al. (2012). Concurrent assay for four bacterial species including Alloiococcus otitidis in middle ear, nasopharynx and tonsils of children with otitis media with effusion: a preliminary report. Clin. Exp. Otorhinolaryngol. 5, 81–85. doi: 10.3342/ceo.2012.5.2.81
Bîrlutiu, V., Mihaila, R. G., Bîrlutiu, R. M., and Cipaian, C. R. (2017). Bacteremia with Turicella otitidis in an institutionalized elderly patient with multiple hospital admissions: a case report. Biomed. Res. 28, 2196–2198. Available online at: https://www.alliedacademies.org/abstract/bacteremia-with-turicella-otitidis-in-an-institutionalized-elderly-patient-with-multiple-hospital-admissions-a-case-report-6715.html
Baek, I., Kim, M., Lee, I., Na, S. I., Goodfellow, M., and Chun, J. (2018). Phylogeny trumps chemotaxonomy: a case study involving Turicella otitidis. Front. Microbiol. 9. doi: 10.3389/fmicb.2018.00834
Bakaletz, L. O. (2002). “Otitis Media,” in Polymicrobial Diseases, eds K. Brogden and J. Guthmiller (Washington, DC: ASM Press). Available online at: https://www.ncbi.nlm.nih.gov/books/NBK2482/ (accessed August 11, 2017).
Beswick, A. J., Lawley, B., Fraise, A. P., Pahor, A. L., and Brown, N. L. (1999). Detection of Alloiococcus otitis in mixed bacterial populations from middle-ear effusions of patients with otitis media. Lancet 354, 386–389. doi: 10.1016/S0140-6736(98)09295-2
Bhutta, M. F. (2014). Epidemiology and pathogenesis of otitis media: construction of a phenotype landscape. Audiol. Neurotol. 19, 210–223. doi: 10.1159/000358549
Biesbroek, G., Tsivtsivadze, E., Sanders, E. A. M., Montijn, R., Veenhoven, R. H., Keijser, B. J. F., et al. (2014). Early respiratory microbiota composition determines bacterial succession patterns and respiratory health in children. Am. J. Respir. Crit. Care Med. 190, 1283–1292. doi: 10.1164/rccm.201407-1240OC
Bluestone, C. D., Gates, G. A., Klein, J. O., Lim, D. J., Mogi, G., Ogra, P. L., et al. (2002). Definitions, terminology, and classification of otitis media. Ann. Otol. Rhinol. Laryngol. 111:8. doi: 10.1177/00034894021110S304
Bluestone, C. D., Stephenson, J. S., and Martin, L. M. (1992). Ten-year review of otitis media pathogens. Pediatr. Infect. Dis. J. 11, S7–11. doi: 10.1097/00006454-199208001-00002
Boers, S. A., de Zeeuw, M., Jansen, R., van der Schroeff, M. P., van Rossum, A. M. C., Hays, J. P., et al. (2018). Characterization of the nasopharyngeal and middle ear microbiota in gastroesophageal reflux-prone versus gastroesophageal reflux non-prone children. Eu. J. Clin. Microbiol. Infect. Dis. 37, 851–857. doi: 10.1007/s10096-017-3178-2
Bogaert, D., de Groot, R., and Hermans, P. (2004). Streptococcus pneumoniae colonisation: the key to pneumococcal disease. Lancet Infect. Dis. 4, 144–154. doi: 10.1016/S1473-3099(04)00938-7
Bosley, G. S., Whitney, A. M., Pruckler, J. M., Moss, C. W., Daneshvar, M., Sih, T., et al. (1995). Characterization of ear fluid isolates of Alloiococcus otitidis from patients with recurrent otitis media. J. Clin. Microbiol. 33:2876. doi: 10.1128/JCM.33.11.2876-2880.1995
Boumghar-Bourtchai, L., Chardon, H., Malbruny, B., Mezghani, S., Leclercq, R., and Dhalluin, A. (2009). Resistance to macrolides by ribosomal mutation in clinical isolates of Turicella otitidis. Int. J. Antimicrob. Agents 34, 274–277. doi: 10.1016/j.ijantimicag.2009.03.023
Brinkrolf, K., Schneider, J., Knecht, M., Rückert, C., and Tauch, A. (2012). Draft genome sequence of Turicella otitidis ATCC 51513, isolated from middle ear fluid from a child with otitis media. J. Bacteriol. 194, 5968–5969. doi: 10.1128/JB.01412-12
Casey, J. R., Adlowitz, D. G., and Pichichero, M. E. (2010). New patterns in the otopathogens causing acute otitis media six to eight years after introduction of pneumococcal conjugate vaccine. Pediatr. Infect. Dis. J. 29, 304–309. doi: 10.1097/INF.0b013e3181c1bc48
Chan, C. L., Richter, K., Wormald, P.-J., Psaltis, A. J., and Vreugde, S. (2017a). Alloiococcus otitidis forms multispecies biofilm with Haemophilus influenzae: effects on antibiotic susceptibility and growth in adverse conditions. Front. Cell Infect. Microbiol. 7:344. doi: 10.3389/fcimb.2017.00344
Chan, C. L., Wabnitz, D., Bardy, J. J., Bassiouni, A., Wormald, P.-J., Vreugde, S., et al. (2016). The microbiome of otitis media with effusion. Laryngoscope 126, 2844–2851. doi: 10.1002/lary.26128
Chan, C. L., Wabnitz, D., Bassiouni, A., Wormald, P.-J., Vreugde, S., and Psaltis, A. J. (2017b). Identification of the bacterial reservoirs for the middle ear using phylogenic analysis. JAMA Otolaryngol. Head Neck Surg. 143:155. doi: 10.1001/jamaoto.2016.3105
Cheeseman, M. T., Tyrer, H. E., Williams, D., Hough, T. A., Pathak, P., Romero, M. R., et al. (2011). HIF–VEGF pathways are critical for chronic otitis media in junbo and jeff mouse mutants. PLoS Genet. 7:e1002336. doi: 10.1371/journal.pgen.1002336
Chonmaitree, T., Revai, K., Grady, J. J., Clos, A., Patel, J. A., Nair, S., et al. (2008). Viral upper respiratory tract infection and otitis media complication in young children. Clin. Infect. Dis. 46, 815–823. doi: 10.1086/528685
Coates, H., Thornton, R., Langlands, J., Filion, P., Anthony, D. K., Vijayasekaran, S., et al. (2008). The role of chronic infection in children with otitis media with effusion: evidence for intracellular persistence of bacteria. Otolaryngol. Head Neck Surg. 138, 778–781. doi: 10.1016/j.otohns.2007.02.009
Dana, A., Fader, R., and Sterken, D. (2001). Turicella otitidis mastoiditis in a healthy child. Pediatr. Infect. Dis. J. 20, 84–85. doi: 10.1097/00006454-200101000-00020
Davidoss, N. H., Varsak, Y. K., and Santa Maria, P. L. (2018). Animal Models of Acute Otitis Media – A Review with Practical Implications for Laboratory Research. Eur. Ann. Otorhinolaryngol. Head Neck Dis. 135, 183–190. doi: 10.1016/j.anorl.2017.06.013
De Baere, T., Vaneechoutte, M., Deschaght, P., Huyghe, J., and Dhooge, I. (2010). The prevalence of middle ear pathogens in the outer ear canal and the nasopharyngeal cavity of healthy young adults. Clin. Microbiol. Infect. 16, 1031–1035. doi: 10.1111/j.1469-0691.2009.02928.x
de Miguel Martínez, I., and Ramos Macías, Á. (2008). Serous otitis media in children: implication of Alloiococcus otitidis. Otol. Neurotol. 29, 526–530. doi: 10.1097/MAO.0b013e318170b5f8
Durmaz, R., Ozerol, I. H., Kalcioglu, M. T., Oncel, S., Otlu, B., Direkel, S., et al. (2002). Detection of Alloiococcus otitidis in the nasopharynx and in the outer ear canal. New Microbiol. 25, 265–268. Available online at: www.researchgate.net/publication/11351598_Detection_of_Alloiococcus_otitidis_in_the_nasopharynx_and_in_the_outer_ear_canal
Faden, H., and Dryja, D. (1989). Recovery of a unique bacterial organism in human middle ear fluid and its possible role in chronic otitis media. J. Clin. Microbiol. 27, 2488–2491. doi: 10.1128/JCM.27.11.2488-2491.1989
Frank, D. N., Spiegelman, G. B., Davis, W., Wagner, E., Lyons, E., and Pace, N. R. (2003). Culture-independent molecular analysis of microbial constituents of the healthy human outer ear. J. Clin. Microbiol. 41, 295–303. doi: 10.1128/JCM.41.1.295-303.2003
Funke, G., Pfyffer, G. E., and von Graevenitz, A. (1993). A hitherto undescribed coryneform bacterium isolated from patients with otitis media. Med. Microbiol. Lett. 2, 183–190.
Funke, G., Pünter, V., and von Graevenitz, A. (1996). Antimicrobial susceptibility patterns of some recently established coryneform bacteria. Antimicrob. Agents Chemother. 40, 2874–2878. doi: 10.1128/AAC.40.12.2874
Funke, G., Stubbs, S., Altwegg, M., Carlotti, A., and Collins, M. D. (1994). Turicella otitidis gen. nov., sp. nov., a coryneform bacterium isolated from patients with otitis media. Int. J. Syst. Bacteriol. 44, 270–273. doi: 10.1099/00207713-44-2-270
Garibpour, F., Khoramrooz, S. S., Mirsalehian, A., Emaneini, M., Jabalameli, F., Darban-Sarokhalil, D., et al. (2013). Isolation and detection of Alloiococcus otitidis in children with otitis media with effusion using culture and PCR methods. J Mazandaran Univ Med Sci. 23, 52–60. Available online at: http://jmums.mazums.ac.ir/browse.php?a_id=2175&sid=1&slc_lang=en
Gómez-Garcés, J. L., Alhambra, A., Alos, J. I., Barrera, B., and García, G. (2004). Acute and chronic otitis media and Turicella otitidis: a controversial association. Clin. Microbiol. Infect. 10, 854–857. doi: 10.1111/j.1198-743X.2004.00965.x
Gómez-Garcés, J. L., Alos, J. I., and Tamayo, J. (2007). In vitro activity of linezolid and 12 other antimicrobials against coryneform bacteria. Int. J. Antimicrob. Agents 29, 688–692. doi: 10.1016/j.ijantimicag.2006.11.032
Gómez-Hernando, C., Toro, C., Gutiérrez, M., Enríquez, A., and Baquero, M. (1999). Isolation of Alloiococcus otitidis from the external ear in children. EJCMID 18, 69–70. doi: 10.1007/s100960050230
Goodfellow, M., Kämpfer, P., Busse, H. J., Trujillo, M. E., Suzuki, K., and Ludwig, W., (eds.). (2012). Bergey's Manual of Systematic Bacteriology: Volume 5: The Actinobacteria. New York, NY: Springer New York. doi: 10.1007/978-0-387-68233-4
Greninger, A. L., Kozyreva, V., Truong, C.-L., Graves, M., and Chaturvedi, V. (2015). Draft genome sequence of Turicella otitidis TD1, isolated from a patient with bacteremia. Genome Announc. 3:e01060-15. doi: 10.1128/genomeA.01060-15
Guler, A., Sahin, M. A., Yesil, F. G., Yildizoglu, U., Demirkol, S., and Arslan, M. (2015). Chronic otitis media resulting in aortic valve replacement: a case report. J. Tehran Univ Heart Center 10:98.
Güvenç, M. G., Midilli, K., Inci, E., Kuşkucu, M., Tahamiler, R., Özergil, E., et al. (2010). Lack of Chlamydophila pneumoniae and predominance of Alloiococcus otitidis in middle ear fluids of children with otitis media with effusion. Auris Nasus Larynx 37, 269–273. doi: 10.1016/j.anl.2009.09.002
Hall-Stoodley, L., Hu, F. Z., Gieseke, A., Nistico, L., Nguyen, D., Hayes, J., et al. (2006). Direct detection of bacterial biofilms on the middle-ear mucosa of children with chronic otitis media. JAMA 296, 202–211. doi: 10.1001/jama.296.2.202
Harimaya, A., Fujii, N., and Himi, T. (2009). Preliminary study of proinflammatory cytokines and chemokines in the middle ear of acute otitis media due to Alloiococcus otitidis. Int. J. Pediatr. Otorhinolaryngol. 73, 677–680. doi: 10.1016/j.ijporl.2008.12.033
Harimaya, A., Himi, T., Fujii, N., Tarkkanen, J., Carlson, P., Ylikoski, J., et al. (2005). Induction of CD69 expression and Th1 cytokines release from human peripheral blood lymphocytes after in vitro stimulation with Alloiococcus otitidis and three middle ear pathogens. FEMS Immunol. Med. Microbiol. 43, 385–392. doi: 10.1016/j.femsim.2004.10.014
Harimaya, A., Koizumi, J.-I., Fujii, N., and Himi, T. (2007a). Interleukin-8 induction via NF-κB, p38 mitogen-activated protein kinase and extracellular signal-regulated kinase1/2 pathways in human peripheral blood mononuclear cells by Alloiococcus otitidis. Int. J. Pediatric Otorhinolaryngol. 71, 1465–1470. doi: 10.1016/j.ijporl.2007.06.003
Harimaya, A., Takada, R., Hendolin, P. H., Fujii, N., Ylikoski, J., and Himi, T. (2006a). High incidence of Alloiococcus otitidis in children with otitis media, despite treatment with antibiotics. J. Clin. Microbiol. 44, 946–949. doi: 10.1128/JCM.44.3.946-949.2006
Harimaya, A., Takada, R., Himi, T., Yokota, S., and Fujii, N. (2007b). Evidence of local antibody response against Alloiococcus otitidis in the middle ear cavity of children with otitis media. FEMS Immunol. Med. Microbiol. 49, 41–45. doi: 10.1111/j.1574-695X.2006.00166.x
Harimaya, A., Takada, R., Somekawa, Y., Fujii, N., and Himi, T. (2006b). High frequency of Alloiococcus otitidis in the nasopharynx and in the middle ear cavity of otitis-prone children. Int. J. Pediatric Otorhinolaryngol. 70, 1009–1014. doi: 10.1016/j.ijporl.2005.10.012
Hendolin, P. H., Kärkkäinen, U., Himi, T., Markkanen, A., and Ylikoski, J. (1999). High incidence of Alloiococcus otitis in otitis media with effusion. Pediatr. Infect. Dis. J. 18, 860–865. doi: 10.1097/00006454-199910000-00005
Hendolin, P. H., Markkanen, A., Ylikoski, J., and Wahlfors, J. J. (1997). Use of multiplex PCR for simultaneous detection of four bacterial species in middle ear effusions. J. Clin. Microbiol. 35, 2854–2858. doi: 10.1128/JCM.35.11.2854-2858.1997
Hendolin, P. H., Paulin, L., and Ylikoski, J. (2000). Clinically applicable multiplex PCR for four middle ear pathogens. J. Clin. Microbiol. 38, 125–132. Available online at: https://jcm.asm.org/content/38/1/125
Himi, T., Kita, H., Mitsuzawa, H., Harimaya, A., Tarkkanen, J., Hendolin, P., et al. (2000). Effect of Alloiococcus otitidis and three pathogens of otitis media in production of interleukin-12 by human monocyte cell line. FEMS Immunol. Med. Microbiol. 29, 101–106. doi: 10.1111/j.1574-695X.2000.tb01511.x
Holder, R. C., Kirse, D. J., Evans, A. K., Peters, T. R., Poehling, K. A., Swords, W. E., et al. (2012). One third of middle ear effusions from children undergoing tympanostomy tube placement had multiple bacterial pathogens. BMC Pediatr. 12:87. doi: 10.1186/1471-2431-12-87
Holder, R. C., Kirse, D. J., Evans, A. K., Whigham, A. S., Peters, T. R., Poehling, K. A., et al. (2015). Otopathogens detected in middle ear fluid obtained during tympanostomy tube insertion: contrasting purulent and non-purulent effusions. PLoS ONE 10:e0128606. doi: 10.1371/journal.pone.0128606
Holzmann, D., Funke, G., Linder, T., and Nadal, D. (2002). Turicella otitidis and Corynebacterium auris do not cause otitis media with effusion in children. Pediatr. Infect. Dis J 21, 1124–1126. doi: 10.1097/00006454-200212000-00007
Janapatla, R. P., Chang, H. J., Hsu, M. H., Hsieh, Y. C., Lin, T. Y., and Chiu, C. H. (2011). Nasopharyngeal carriage of Streptococcus pneumoniae, Haemophilus influenzae, Moraxella catarrhalis, and Alloiococcus otitidis in young children in the era of pneumococcal immunization, Taiwan. Scand. J. Infect. Dis. 43, 937–942. doi: 10.3109/00365548.2011.601754
Jervis-Bardy, J., Rogers, G. B., Morris, P. S., Smith-Vaughan, H. C., Nosworthy, E., Leong, L. E. X., et al. (2015). The microbiome of otitis media with effusion in Indigenous Australian children. Int. J. Pediatr. Otorhinolaryngol. 79, 1548–1555. doi: 10.1016/j.ijporl.2015.07.013
Johnston, J., Hoggard, M., Biswas, K., Astudillo-García, C., Radcliff, F. J., Mahadevan, M., et al. (2019). Pathogen reservoir hypothesis investigated by analyses of the adenotonsillar and middle ear microbiota. Int. J. Pediatr. Otorhinolaryngol. 118, 103–109. doi: 10.1016/j.ijporl.2018.12.030
Kalcioglu, M. T., Oncel, S., Durmaz, R., Otlu, B., Miman, M. C., and Ozturan, O. (2002). Bacterial etiology of otitis media with effusion; focusing on the high positivity of Alloiococcus otitidis. New Microbiol. 25, 31–35.
Kaur, R., Adlowitz, D. G., Casey, J. R., Zeng, M., and Pichichero, M. E. (2010). Simultaneous assay for four bacterial species including Alloiococcus otitidis using multiplex-PCR in children with culture negative acute otitis media. Pediatr. Infect. Dis. J. 29, 741–745. doi: 10.1097/INF.0b013e3181d9e639
Khoramrooz, S. S., Mirsalehian, A., Emaneini, M., Jabalameli, F., Aligholi, M., Saedi, B., et al. (2012). Frequency of Alloicoccus otitidis, Streptococcus pneumoniae, Moraxella catarrhalis and Haemophilus influenzae in children with otitis media with effusion (OME) in Iranian patients. Auris Nasus Larynx 39, 369–373. doi: 10.1016/j.anl.2011.07.002
Kita, H., Himi, T., Fujii, N., and Ylikoski, J. (2000). Interleukin-8 secretion of human epithelial and monocytic cell lines induced by middle ear pathogens. Microbiol. Immunol. 44, 511–517. doi: 10.1111/j.1348-0421.2000.tb02526.x
Kong, K., and Coates, H. L. C. (2009). Natural history, definitions, risk factors and burden of otitis media. Med. J. Aust. 191, S39–S43. doi: 10.5694/j.1326-5377.2009.tb02925.x
Krueger, A., Val, S., Pérez-losada, M., Panchapakesan, K., Devaney, J., Duah, V., et al. (2017). Relationship of the middle ear effusion microbiome to secretory mucin production in pediatric patients with chronic otitis media. Pediatr. Infect. Dis. J. 36, 635–640. doi: 10.1097/INF.0000000000001493
Lal, D., Keim, P., Delisle, J., Barker, B., Rank, M. A., Chia, N., et al. (2017). Mapping and comparing bacterial microbiota in the sinonasal cavity of healthy, allergic rhinitis, and chronic rhinosinusitis subjects. Int. Forum Allergy Rhinol. 7, 561–569. doi: 10.1002/alr.21934
Lappan, R., Imbrogno, K., Sikazwe, C., Anderson, D., Mok, D., Coates, H., et al. (2018). A microbiome case-control study of recurrent acute otitis media identified potentially protective bacterial genera. BMC Microbiol. 18:13. doi: 10.1186/s12866-018-1154-3
Laufer, A. S., Metlay, J. P., Gent, J. F., Fennie, K. P., Kong, Y., and Pettigrew, M. M. (2011). Microbial communities of the upper respiratory tract and otitis media in children. mBio 2, e00245–e00210. doi: 10.1128/mBio.00245-10
Leskinen, K., Hendolin, P., Virolainen-Julkunen, A., Ylikoski, J., and Jero, J. (2002). The clinical role of Alloiococcus otitidis in otitis media with effusion. Int. J. Pediatr. Otorhinolaryngol. 66, 41–48. doi: 10.1016/S0165-5876(02)00186-6
Leskinen, K., Hendolin, P., Virolainen-Julkunen, A., Ylikoski, J., and Jero, J. (2004). Alloiococcus otitidis in acute otitis media. Int. J. Pediatr. Otorhinolaryngol. 68, 51–56. doi: 10.1016/j.ijporl.2003.09.005
Loïez, C., Wallet, F., Fruchart, A., Husson, M.-O., and Courcol, R. J. (2002). Turicella otitidis in a bacteremic child with acute lymphoblastic leukemia. Clin. Microbiol. Infect. 8, 758–759. doi: 10.1046/j.1469-0691.2002.00474.x
Marchino, T., Vela, J. I., Bassaganyas, F., Sánchez, S., and Buil, J. A. (2013). Acute-onset endophthalmitis caused by Alloiococcus otitidis following a dexamethasone intravitreal implant. COP 4, 37–41. doi: 10.1159/000348809
Marsh, R. L., Binks, M. J., Beissbarth, J., Christensen, P., Morris, P. S., Leach, A. J., et al. (2012). Quantitative PCR of ear discharge from Indigenous Australian children with acute otitis media with perforation supports a role for Alloiococcus otitidis as a secondary pathogen. BMC Ear Nose Throat Disord. 12:11. doi: 10.1186/1472-6815-12-11
Matsuo, J., Yamaguchi, H., Harimaya, A., Fukumoto, T., Nakamura, S., Yoshida, M., et al. (2011). Impact of anaerobic and oligotrophic conditions on survival of Alloiococcus otitidis, implicated as a cause of otitis media. J. Infect. Chemother. 17, 478–482. doi: 10.1007/s10156-010-0199-5
Minami, S. B., Mutai, H., Suzuki, T., Horii, A., Oishi, N., Wasano, K., et al. (2017). Microbiomes of the normal middle ear and ears with chronic otitis media. Laryngoscope 127, E371–E377. doi: 10.1002/lary.26579
Neeff, M., Biswas, K., Hoggard, M., Taylor, M. W., and Douglas, R. (2016). Molecular microbiological profile of chronic suppurative otitis media. J. Clin. Microbiol. 54, 2538–2546. doi: 10.1128/JCM.01068-16
Papp, Z., Elgabsi, H., and Tóth, L. (2016). MALDI-TOF mass spectrometry reveals a highly complex bacterial profile of otitis media with effusion. Int. J. Pediatr. Otorhinolaryngol. 86, 189–192. doi: 10.1016/j.ijporl.2016.05.004
Pereira, M. B. R., Cantarelli, V., Pereira, D. R. R., and da Costa, S. S. (2004). High incidence of Alloiococcus otitidis in otitis media with effusion measured by multiplex PCR. Braz. J. Otorhinolaryngol. 70, 217–224. doi: 10.1590/S0034-72992004000200012
Pettigrew, M. M., Laufer, A. S., Gent, J. F., Kong, Y., Fennie, K. P., and Metlay, J. P. (2012). Upper respiratory tract microbial communities, acute otitis media pathogens, and antibiotic use in healthy and sick children. Appl. Environ. Microbiol. 78, 6262–6270. doi: 10.1128/AEM.01051-12
Pickering, J. L., Prosser, A., Corscadden, K. J., de Gier, C., Richmond, P. C., Zhang, G., et al. (2016). Haemophilus haemolyticus interaction with host cells is different to nontypeable Haemophilus influenzae and prevents NTHi association with epithelial cells. Front. Cell Infect. Microbiol. 6:50. doi: 10.3389/fcimb.2016.00050
Renaud, F. N., Grégory, A., Barreau, C., Aubel, D., and Freney, J. (1996). Identification of Turicella otitidis isolated from a patient with otorrhea associated with surgery: differentiation from Corynebacterium afermentans and Corynebacterium auris. J. Clin. Microbiol. 34, 2625–2627. doi: 10.1128/JCM.34.10.2625-2627.1996
Reynolds, S. J., Behr, M., and McDonald, J. (2001). Turicella otitidis as an unusual agent causing a posterior auricular abscess. J. Clin. Microbiol. 39, 1672–1673. doi: 10.1128/JCM.39.4.1672-1673.2001
Santos-Cortez, R. L. P., Hutchinson, D. S., Ajami, N. J., Reyes-Quintos, M. R. T., Tantoco, M. L. C., Labra, P. J., et al. (2016). Middle ear microbiome differences in indigenous Filipinos with chronic otitis media due to a duplication in the A2ML1 gene. Infect. Dis. Poverty 5:97. doi: 10.1186/s40249-016-0189-7
Sheikh, A. F., Saki, N., Roointan, M., Ranjbar, R., Yadyad, M. J., Kaydani, A., et al. (2015). Identification of Alloiococcus otitidis, Streptococcus pneumoniae, Moraxella catarrhalis and Haemophilus influenzae in children with otitis media with effusion. Jundishapur J. Microbiol. 8:e17985. doi: 10.5812/jjm.17985
Sillanpää, S., Kramna, L., Oikarinen, S., Sipilä, M., Rautiainen, M., Aittoniemi, J., et al. (2017). Next-generation sequencing combined with specific PCR assays to determine the bacterial 16S rRNA gene profiles of middle ear fluid collected from children with acute otitis media. mSphere 2:e00006–17. doi: 10.1128/mSphere.00006-17
Sillanpää, S., Oikarinen, S., Sipilä, M., Kramna, L., Rautiainen, M., Huhtala, H., et al. (2016). Moraxella catarrhalis might be more common than expected in acute otitis media in young finnish children. J. Clin. Microbiol. 54, 2373–2379. doi: 10.1128/JCM.01146-16
Simonet, M., De Briel, D., Boucot, I., Minck, R., and Veron, M. (1993). Coryneform bacteria isolated from middle ear fluid. J. Clin. Microbiol. 31, 1667–1668. doi: 10.1128/JCM.31.6.1667-1668.1993
Slinger, R., Duval, M., Langill, J., Bromwich, M., MacCormick, J., Chan, F., et al. (2016). Direct molecular detection of a broad range of bacterial and viral organisms and Streptococcus pneumoniae vaccine serotypes in children with otitis media with effusion. BMC Res. Notes 9:247. doi: 10.1186/s13104-016-2040-4
Stroman, D. W., Roland, P. S., Dohar, J., and Burt, W. (2001). Microbiology of normal external auditory canal. Laryngoscope 111, 2054–2059. doi: 10.1097/00005537-200111000-00035
Tano, K., Von Essen, R., Eriksson, P. O., and Sjöstedt, A. (2008). Alloiococcus otitidis- otitis media pathogen or normal bacterial flora? APMIS 116, 785–790. doi: 10.1111/j.1600-0463.2008.01003.x
Tarkkanen, J., Carlson, P., Himi, T., Ylikoski, J., Atshushi, H., and Mattila, P. S. (2000). Stimulation of adenoidal lymphocytes by Alloiococcus otitidis. Ann. Otol. Rhinol. Laryngol. 109, 958–964. doi: 10.1177/000348940010901010
Taylor, P. S., Faeth, I., Marks, M. K., Del Mar, C. B., Skull, S. A., Pezzullo, M. L., et al. (2009). Cost of treating otitis media in Australia. Expert Rev. Pharmacoecon. Outcomes Res. 9, 133–141. doi: 10.1586/erp.09.6
Teele, D. W., Klein, J. O., and Rosner, B. Greater Boston Otitis Media Study Group (1989). Epidemiology of otitis media during the first seven years of life in children in greater boston: a prospective, cohort study. J. Infect. Dis. 160, 83–94. doi: 10.1093/infdis/160.1.83
Teo, S. M., Mok, D., Pham, K., Kusel, M., Serralha, M., Troy, N., et al. (2015). The infant airway microbiome in health and disease impacts later asthma development. Cell Host Microbe 17, 704–715. doi: 10.1016/j.chom.2015.03.008
Thornton, R. B., Rigby, P. J., Wiertsema, S. P., Filion, P., Langlands, J., Coates, H. L., et al. (2011). Multi-species bacterial biofilm and intracellular infection in otitis media. BMC Pediatr. 11:94. doi: 10.1186/1471-2431-11-94
Troxler, R., Funke, G., Von Graevenitz, A., and Stock, I. (2001). Natural antibiotic susceptibility of recently established coryneform bacteria. Eur. J. Clin. Microbiol. Infect. Dis. 20, 315–323. doi: 10.1007/s100960100503
Val, S., Poley, M., Anna, K., Nino, G., Brown, K., Pérez-Losada, M., et al. (2018). Characterization of mucoid and serous middle ear effusions from patients with chronic otitis media: implication of different biological mechanisms? Pediatr. Res. 84, 296–305. doi: 10.1038/s41390-018-0060-6
Van Eldere, J., Slack, M. P. E., Ladhani, S., and Cripps, A. W. (2014). Non-typeable Haemophilus influenzae, an under-recognised pathogen. Lancet Infect. Dis. 14, 1281–1292. doi: 10.1016/S1473-3099(14)70734-0
Verduin, C. M., Hol, C., Fleer, A., Dijk, H., van Belkum, A., and van (2002). Moraxella catarrhalis: from emerging to established pathogen. Clin. Microbiol. Rev. 15, 125–144. doi: 10.1128/CMR.15.1.125-144.2002
von Graevenitz, A. (1993). Revised nomenclature of Alloiococcus otitis. J. Clin. Microbiol. 31:472. doi: 10.1128/JCM.31.2.472-472.1993
von Graevenitz, A., and Funke, G. (2014). Turicella otitidis and Corynebacterium auris: 20 years on. Infection 42, 1–4. doi: 10.1007/s15010-013-0488-x
Vos, P., Garrity, G., Jones, D., Krieg, N. R., Ludwig, W., Rainey, F. A., et al. (2009). Bergey's Manual of Systematic Bacteriology: Volume 3: The Firmicutes, 2nd Edn. New York, NY: Springer Science & Business Media.
Westerberg, B. D., Kozak, F. K., Thomas, E. E., Blondel-Hill, E., Brunstein, J. D., and Patrick, D. M. (2009). Is the healthy middle ear a normally sterile site? Otol. Neurotol. 30, 174–177. doi: 10.1097/MAO.0b013e31819225a0
Wiertsema, S. P., Kirkham, L.-A. S., Corscadden, K. J., Mowe, E. N., Bowman, J. M., Jacoby, P., et al. (2011). Predominance of nontypeable Haemophilus influenzae in children with otitis media following introduction of a 3 + 0 pneumococcal conjugate vaccine schedule. Vaccine 29, 5163–5170. doi: 10.1016/j.vaccine.2011.05.035
Keywords: Alloiococcus, Turicella, otitis media, middle ear, otopathogen
Citation: Lappan R, Jamieson SE and Peacock CS (2020) Reviewing the Pathogenic Potential of the Otitis-Associated Bacteria Alloiococcus otitidis and Turicella otitidis. Front. Cell. Infect. Microbiol. 10:51. doi: 10.3389/fcimb.2020.00051
Received: 29 August 2019; Accepted: 27 January 2020;
Published: 14 February 2020.
Edited by:
Regie Santos-Cortez, University of Colorado, United StatesReviewed by:
Kevin Mason, The Ohio State University, United StatesJoshua Chang Mell, Drexel University, United States
Copyright © 2020 Lappan, Jamieson and Peacock. This is an open-access article distributed under the terms of the Creative Commons Attribution License (CC BY). The use, distribution or reproduction in other forums is permitted, provided the original author(s) and the copyright owner(s) are credited and that the original publication in this journal is cited, in accordance with accepted academic practice. No use, distribution or reproduction is permitted which does not comply with these terms.
*Correspondence: Christopher S. Peacock, Y2hyaXN0b3BoZXIucGVhY29ja0B1d2EuZWR1LmF1