Commentary: Global prevalence and antibiotic resistance in clinical isolates of Stenotrophomonas maltophilia: a systematic review and meta-analysis
- 1Laboratorio de Investigación en Bacteriología Intestinal, Hospital Infantil de México “Federico Gómez”, Ciudad de México, Mexico
- 2Centro de Investigación en Enfermedades Infecciosas, Instituto Nacional de Enfermedades Respiratorias, Ciudad de México, Mexico
- 3Laboratorio Central de Bacteriología, Hospital Infantil de México “Federico Gómez”, Ciudad de México, Mexico
- 4Laboratorio de Neuropatología, Instituto Nacional de Neurología y Neurocirugía “Manuel Velasco Suárez”, Ciudad de México, Mexico
- 5Hospital Ángeles Morelia, Morelia, Mexico
- 6Departamento de Epidemiología, Hospital Infantil de México “Federico Gómez”, Ciudad de México, Mexico
Stenotrophomonas maltophilia, an emerging opportunistic pathogen, is widely distributed in the environment the resistance mechanisms, and virulence factors of this bacterium facilitate its dissemination in hospitals. This study aimed to characterize the molecular epidemiology of S. maltophilia strains associated with an outbreak in the Children's Hospital of México Federico Gómez (HIMFG). Twenty-one clinical S. maltophilia strains were recovered from cultures of blood and urine samples from 10 pediatric patients at the emergency department, and nine environmental S. maltophilia strains recovered from faucets in the same area were also included. Two of the 10 patients were related with health care-associated infections (HCAIs), and the other eight patients (8/10) were infected with environmental S. maltophilia strains. The outbreak was controlled by monthly disinfection of the faucets in the emergency department. Typing using pulsed-field gel electrophoresis (PFGE) showed a 52% genetic diversity with seven pulsotypes denoted P1–P7 among all S. maltophilia strains. Three pulsotypes (P2, P3, and P7) were identified among both the clinical and environmental S. maltophilia strains and associated with two type sequences (STs), namely, ST304 and ST24. Moreover, 80% (24/30) of the strains exhibited resistance mainly to tetracycline, 76.66% (23/30) to trimethoprim-sulfamethoxazole, and 23.33% (7/30) to the extended-spectrum β-lactamase (ESBL) phenotype. The main resistance genes identified by multiplex PCR were sul1 in 100% (30/30), qnr in 86.66% (26/30), and intl1 in 80% (24/30) of the samples, respectively. Furthermore, the pilU, hlylII, and rmlA genes were identified in 96.6% (29/30), 90% (27/30), and 83.33% (25/30) of the samples, respectively. Additionally, 76.66% (23/30) of the S. maltophilia strains exhibited high swimming motility, 46.66% (14/30) showed moderate biofilm formation capacity, 43.33% (13/30) displayed moderate twitching motility, and 20% (6/30) exhibited high adherence. The clinical S. maltophilia strains isolated from blood most strongly adhered to HTB-9 cells. In conclusion, the molecular epidemiology and some of the features such as resistance, and virulence genes associated with colonization patterns are pathogenic attributes that can promote S. maltophilia dissemination, persistence, and facilitate the outbreak that occurred in the HIMFG. This study supports the need for faucet disinfection as a control strategy for clinical outbreaks.
Introduction
Stenotrophomonas maltophilia is an intrinsically multidrug-resistant (MDR) gram-negative bacillus that is widely distributed in aqueous and humid habitats in the environment, including plants, animals, and water sources (Gulcan et al., 2004). S. maltophilia is recognized as an important nosocomial pathogen that causes health care-associated infections (HCAIs) through direct contact, ingestion, aspiration, aerosolization of potable water or the hands of healthcare workers (Guyot et al., 2013). This opportunistic pathogen can cause severe infections, such as bacteremia, sepsis, pneumonia, meningitis after neurosurgical procedures, endocarditis, urinary tract infection, septic arthritis, and endophthalmitis, in immunocompromised patients (Denton and Kerr, 1998; Park et al., 2008; Botana-Rial et al., 2016; Waite et al., 2016). HCAI-associated S. maltophilia strains exhibit resistance to a broad spectrum of antibiotics, including β-lactams, macrolides, fluoroquinolones, aminoglycosides, chloramphenicol (C), tetracyclines (TEs), polymyxins, and trimethoprim-sulfamethoxazole (SXT) (Wu et al., 2006; Chang et al., 2007). The intrinsic resistance of S. maltophilia strains is associated with low membrane permeability, efflux pumps, and the intrinsic beta-lactamases L1 and L2, among other drug resistance determinants that shield this microorganism (Sanchez et al., 2002; Crossman et al., 2008; Mojica et al., 2019). S. maltophilia can also acquire resistance via the acquisition of mutations or resistance genes by horizontal gene transfer (Sanchez, 2015). Among these genes, dihydropteroate synthase (sul1 and sul2), and dihydrofolate reductase (drfA) are the main mechanisms of SXT resistance in S. maltophilia (Toleman et al., 2007).
Some virulence factors have been described in S. maltophilia, including Smf1-fimbrial operon, proteases, hemolysins, exopolysaccharides (EPSs), lipopolysaccharides (LPSs), siderophores, flagella, lipases, putative virulence genes in a pathogenicity island encoding proteins from the RTX (repeats-in-toxin) family, and transmembrane secretion system components (Brooke, 2012; Adamek et al., 2014; Sanchez, 2015; Pompilio et al., 2016; Alcaraz et al., 2018; Trifonova and Strateva, 2019). A few studies have described the colonization of S. maltophilia in cultures of HEp-2 and IB3-1 cells as well as A/J, DBA/2, BALB/c, and C57BL/6 mice (De Vidipo et al., 2001; De Oliveira-Garcia et al., 2003; Pompilio et al., 2008; Lin et al., 2015). Outbreaks of S. maltophilia strains have been associated with a high clonal diversity by pulsed-field gel electrophoresis (PFGE), and these analyses included investigations of the population structure of bacteria from cystic fibrosis patients (Nicoletti et al., 2011; Vidigal et al., 2014). PFGE has been used to analyze the profiles of the environmental reservoirs of epidemic clones, mainly in damp areas (taps, water reservoirs of humidifiers, assisted ventilation equipment, aspirators, and ventilators), and their modes of transmission (Valdezate et al., 2004; Gherardi et al., 2015). Outbreaks of S. maltophilia clinical strains have been associated with intensive care units (ICUs), emergency departments, respiratory units, oncology units, and surgery wards (Brooke, 2012). In this study, the molecular epidemiology of clinical and environmental strains of outbreak-associated S. maltophilia was characterized by identifying their virulence, antibiotic resistance, twitching motility, biofilm formation capacity, and adherence profiles. Our results suggest that some of these features can promote S. maltophilia dissemination and persistence in the HIMFG.
Materials and Methods
Bacterial Strains
A collection of 21 clinical strains of S. maltophilia was obtained between December 19th, 2016, and February 13th, 2017 (Table 1). These strains were sourced from the blood and urine of pediatric patients who were accepted into the emergency department and subsequently hospitalized in different wards (surgery, internal medicine, intensive therapy unit, neurology, infectology, neurosurgery, oncology, and surgical therapy). Nine environmental strains of S. maltophilia recovered from faucets in the emergency department were also included in this study. S. maltophilia strains were routinely cultured in blood agar (5% sheep blood) overnight at 37°C and identified using the MALDI-TOF VITEK MS Microbial Identification System (bioMérieux, 376 Chemin de l'Orme, 69280 Marcy-l'Étoile, France) at the Central Clinical Laboratory of the Children's Hospital of México Federico Gómez (HIMFG) (Tatman-Otkun et al., 2005; Jacquier et al., 2011).
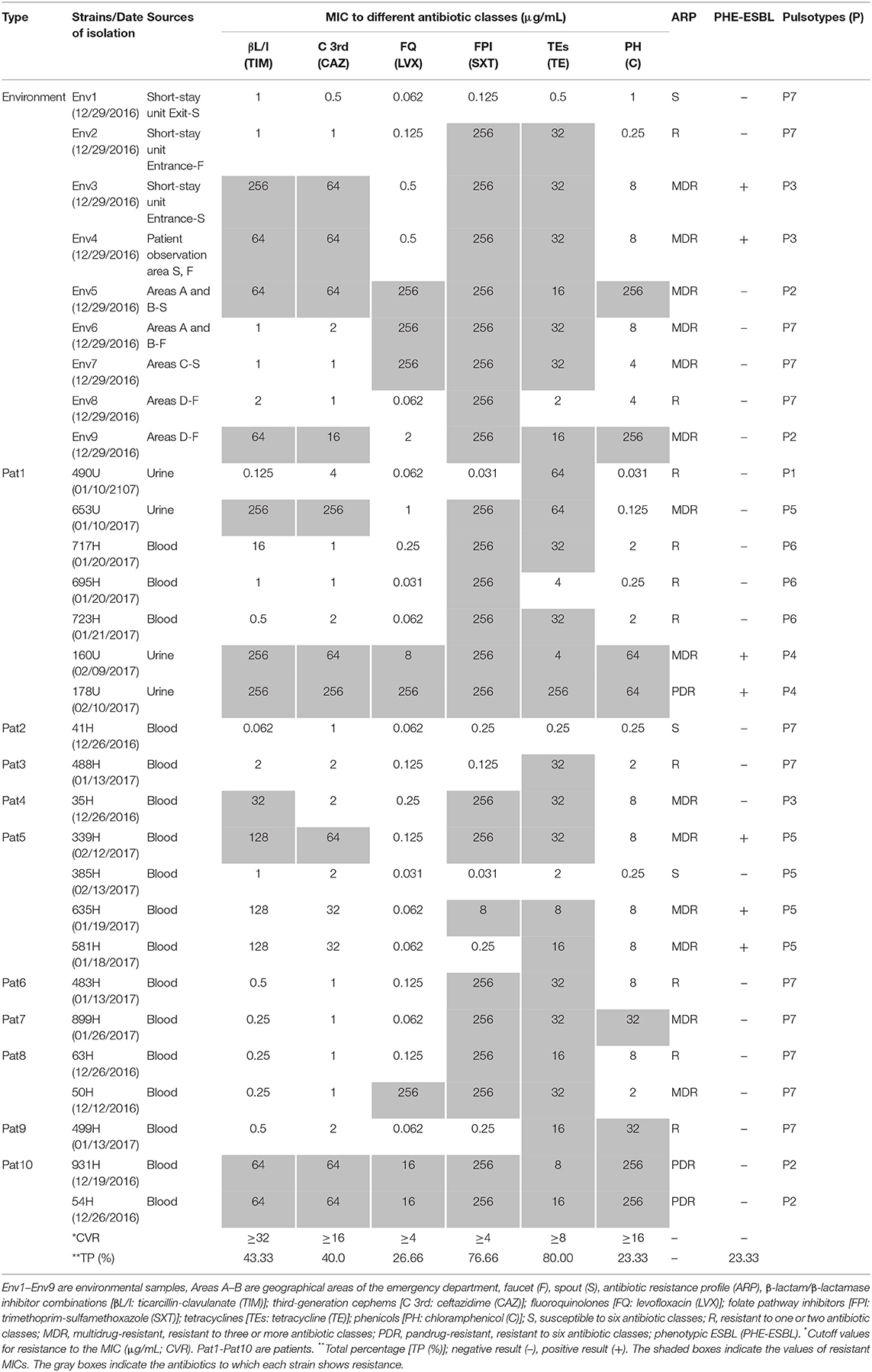
Table 1. MICs to six antibiotic classes suggested by the CLSI (2018) obtained for 21 clinical strains and nine environmental strains of S. maltophilia associated with a hospital outbreak.
Antimicrobial Susceptibility and Extended-Spectrum β-Lactamase (ESBL) Phenotypic Detection in the S. maltophilia Strains
Tests of the antimicrobial susceptibility of the S. maltophilia strains were performed according to the Clinical and Laboratory Standards Institute (CLSI, 2018). The minimum inhibitory concentration (MIC) was determined through a microdilution test in Muller-Hinton broth (NJ, USA). Several antibiotic categories were included in this study: TEs (TE) from Heritage Pharmaceuticals Inc. (Edison, NJ, USA), β-lactam/β-lactamase inhibitor combinations (ticarcillin/clavulanate, TIM) from Sigma-Aldrich (St. Louis, MO, USA), third-generation cephems (ceftazidime, CAZ) from Roselle Rd (Schaumburg, IL, USA), fluoroquinolones (levofloxacin, LVX) from MP Biomedicals (Solon, OH, USA), folate pathway inhibitors (SXT), and phenicols (C) from Roche (Basel, Switzerland). Escherichia coli ATCC 25922 and Pseudomonas aeruginosa ATCC 27853 were used as controls.
The extended-spectrum β-lactamase (ESBL) phenotypes were detected via double-disk synergy testing to evaluate the synergistic effects between clavulanic acid and β-lactam antibiotics as previously described by our research group (Ochoa et al., 2016; CLSI, 2018).
Detection of Quinolone, Sulfonamide Resistance, and Integron-Integrase Gene Classes in the S. maltophilia Strains
Genomic DNA from the S. maltophilia strains after culture in trypticase soy broth (TSB, Difco-Becton Dickinson, NJ, USA) overnight at 37°C was obtained using the Wizard® Genomic DNA Purification Kit from Promega Corporation (Madison, WI, USA). The obtained genomic DNA was used for identification of the resistance genes (sul1, sul2, and qnr) through end-point PCR and the integron classes (1, 2, and 3) by multiplex PCR (Table S1) (Ozkaya et al., 2014). The DNA products were resolved by electrophoresis and stained with 0.5 μg/mL ethidium bromide (Sigma-Aldrich, St. Louis, MO, USA; Ochoa et al., 2016).
Identification of S. maltophilia Strain Virulence Genes
DNA was extracted from S. maltophilia strains as described in Detection of quinolone, sulfonamide resistance and integron-integrase gene classes in the S. maltophilia strains. The primers used for the identification of 20 virulence genes (virB, rmlA, fliC, stmPr1, pilU, hlyIII, gspD, papD, afaD, hgbB, entA, tpsB, picN1, motA, zot, fhaB, frpC, fimH, lktD, and hcp) by multiplex PCR were designed based on the following sequences: S. maltophilia K279a, S. maltophilia R551_3, S. maltophilia SJTH1, S. maltophilia NCTC10257, S. maltophilia FDAARGOS_92, 13637, S. maltophilia WZN_1, S. maltophilia JV3, S. maltophilia CSM2, and S. maltophilia ISMMS2 (Table S2). The PCR products were resolved by electrophoresis on agarose gels and stained with 0.5 μg/mL ethidium bromide for visualization as previously described (Ochoa et al., 2016).
Biofilm and Adherence Assays of the S. maltophilia Strains
Twenty microliters (1 × 107 bacteria/mL) of S. maltophilia strains cultured overnight in TSB were added to 180 μL of TSB in each well of a 96-well plate and incubated for 24 h at 37°C. The medium was then discarded from each well, and the growing bacteria were removed by washing three times with sterile 1× phosphate-buffered saline (PBS, pH 7.3, Sigma-Aldrich). The biofilms formed by the S. maltophilia strains were fixed with 200 μL of 2% formaldehyde, stained with crystal violet for 1 h and washed three times with 1× PBS to remove excess dye. Subsequently, 200 μL of methanol was added to each well to elute the crystal violet, and the absorbance at 620 nm (OD620) was quantified using a Multiskan FC instrument from Thermo Scientific (Waltham, MA, USA). The experiments were performed in triplicate at least three times on three different days. The data are presented as the means of the averages of the results obtained from all the experiments. The values for low, moderate and high biofilm formation capacity were determined based on the OD620 of the biofilm formed by S. maltophilia ATCC 13637 according to the protocol described by Pompilio et al. (2011).
The adherence assays were performed using HTB-9 bladder cells (5637, ATCC® HTB9™; Manassas, VA, USA), which were derived from a human urinary bladder grade II carcinoma. HTB-9 bladder cells were cultured in Roswell Park Memorial Institute medium (RPMI-1640; ATCC®, Manassas, VA, USA) supplemented with 10% fetal bovine serum (ATCC®, Manassas, VA, USA). The HTB-9 cells were counted with trypan blue (ATCC®, Manassas, VA, USA) and the number of bacteria was adjusted to a multiplicity of infection (MOI) of 100:1 (1 × 105 cells/mL/well) in 24-well plates with RPMI-1640. The cell monolayers were infected with 1 × 107 bacteria/mL and incubated for 3 h at 37°C with 5% CO2. To remove non-attached bacteria, the supernatant was discarded, and the infected cell monolayers were washed three times with 1× PBS. The bacteria attached to the cell monolayers were removed after adding 1 mL of 0.1% Triton X-100 in PBS (Amresco, OH, USA), and serial dilutions were plated onto Luria-Bertani (LB) agar plates to determine the number of colony-forming units (CFU)/mL. The adherence assays were performed in triplicate three times on different days, and the CFU/mL values are expressed as the means of the averages. The environmental and clinical S. maltophilia strains were considered to adhere at low, moderate or high levels based on the adherence value (CFU/mL) of the S. maltophilia ATCC 13637 control strains, and the standard deviation of the events was recorded (De Oliveira-Garcia et al., 2003).
PFGE and MLST Analyses of the S. maltophilia Strains
S. maltophilia strains were analyzed by PFGE according to methods modified by our research group (Ochoa et al., 2016). Briefly, purified DNA was digested with 2 U of Xbal (Invitrogen, Life Technologies, Waltham, MA, USA) at 37°C for 4 h. The DNA samples were electrophoresed on a CHEF Mapper system from Bio-Rad Life Science Research (Hercules, CA, USA) for 23 h at 200 V (6 V/cm). The macro restriction products were resolved by electrophoresis on 1.2% agarose gels, stained with 0.5 μg/mL ethidium bromide for 40 min and visualized under UV light using a ChemiDoc MP® imaging system. The PFGE patterns were analyzed using NTSYS-pc software, and the clonality degree was estimated according to the criteria described by Tenover et al. (1995).
MLST of S. maltophilia strains were also performed following the protocol described by Kaiser et al. (2009), Jolley et al. (2018). The seven housekeeping genes (atpD, gapA, guaA, mutM, nuoD, ppsA, and recA) were amplified using Phusion TM High-fidelity DNA polymerase (Thermo Fisher, Waltham, MA, USA), purified and then after sequenced using the BigDye Terminator v3.1 Cycle Sequencing Kit (Applied Biosystems, Foster City, CA, USA) with an ABI 3500 Genetic Analyzer (Applied Biosystems) automatic sequencer. The allelic profiles of each gene were analyzed using Chromas v2.6.6 (http://technelysium.com.au/wp/chromas-version-history/) and BioEdit v7.0.5.3 (https://www.nucleics.com/DNA_sequencing_support/Trace_viewer_reviews/BioEdit/) software, and the ST for each unique allelic profile was designated based on information from the MLST website (https://pubmlst.org/smaltophilia/). In addition, the primer sequences for the gapA, mutM, nuoD, and ppsA housekeeping genes were manually designed. The allele sequences of these genes were obtained from the S. maltophilia MLST database (Oxford). Multiple sequence alignment was performed by identifying conserved regions. The designed MLST primers generated products of 768 bp for gapA, 592 bp for mutM, 531 bp for nuoD, and 887 bp for ppsA (Table S1).
Twitching and Swimming Motility Assays
For the assessment of twitching motility, the S. maltophilia strains were first grown on TSB at 37°C for 24 h, and 1 μL of each bacterial suspension (1.5 × 108 bacteria/mL) was then vertically inoculated into a well of a six-well plate with 5 mL of TSB and 1% agar and incubated at 37°C for 24 h. The colonies exhibiting twitching motility were then visualized by staining with Coomassie Brilliant Blue R-250 (Bio-Rad, Hercules, USA).
To assess the swimming motility, the S. maltophilia strains were grown overnight in TSB at 37°C, and 1 μL of each bacterial suspension (1.5 × 108 bacteria/mL) was vertically inoculated into a well of a six-well plate containing 5 mL of TSB and 0.3% agar and incubated at 37°C for 24 h.
The twitching and swimming motilities were evaluated by measuring the halo of growth in millimeters (mm) around the inoculation zone, and one, two and four standard deviations indicated low, moderate, and high profiles, respectively (Pompilio et al., 2011). The strains P. aeruginosa ATCC 27853, E. coli ATCC 25922, and S. maltophilia ATCC 13637 were used as controls in both experiments.
Statistical Analysis
ANOVA followed by Fisher's protected least significant difference (PLSD) was used to identify significant differences in the data, and values of p < 0.05 were considered to indicate statistical significance. The PFGE results were analyzed with NTSYS-pc software (version 2.0, Applied Biostatistics, Inc., NY, USA) based on the simple matching similarity coefficient and the unweighted pair group method using arithmetic averages (UPGMA). The clonal complexes were analyzed using PHYLOViZ software (https://online.phyloviz.net/index), which is based on the goeBURST algorithm (Francisco et al., 2009). The biofilm, adherence, twitching, and swimming motility data were analyzed with StatView for Windows software (www.statview.com) (Landau and Rabe-Hesketh, 1999). The heat maps were generated using GraphPad Prism v8.0 software (https://www.graphpad.com/). The association among the clonal, clinical, and environmental S. maltophilia strains was analyzed using ShinyCircos software (https://github.com/venyao/shinyCircos) (Yu et al., 2018).
Results
Outbreak Description
The HIMFG is a third-level hospital that sees pediatric patients, and the decision of whether to admit patients occurs in the emergency department. The HCAI rate is between 0 and 4%, and the incidence is between 0 and 4.2/1,000 patients each day in the emergency department. A total of 30 S. maltophilia strains were identified as follows: 17 from blood (December 19th, 2016–February 13th, 2017), four from urine (January 10th, 2017–February 13th, 2017), and nine from the environment (December 29th, 2016; Figure 1, Table 1). The cultures from blood and urine were recovered from 10 patients in the emergency department.
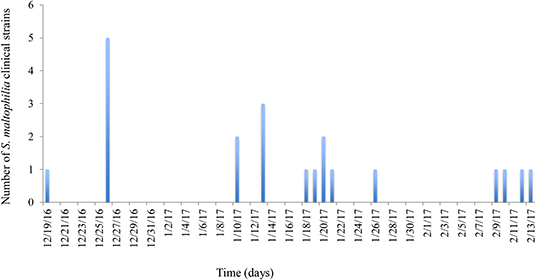
Figure 1. Graphical representation of the epidemiology of outbreak-associated S. maltophilia strains over time. The frequencies of clinical strains were determined by their persistence time during the outbreak. The first strain was identified on December 19th, 2016, and the last strain was identified on February 13th, 2017. The culture of environmental S. maltophilia strains was performed on December 26, 2016.
To study the outbreak, bacterial samples were obtained from surfaces of the nursery trolley, beds of patients (handrails and mattresses), aseptic material (single-use chlorhexidine and alcohol devices), storage drawers, water faucets, and the surrounding and interior regions of the spouts and cultured in blood culture bottles. Only the water faucets and spout cultures were positive for S. maltophilia strains. As control measures, the sampling technique was reviewed at the beginning of the outbreak, and blood cultures and hand hygiene were emphasized (De la Rosa-Zamboni et al., 2018). After S. maltophilia strains were found in the external and internal parts of the faucets, the faucets were dismantled, cleaned, disinfected with chlorine (400 ppm) and sterilized in an autoclave. A faucet disinfection policy was subsequently implemented in the emergency department, and this policy involved cleaning and disinfection every 2 weeks for the first 3 months after the outbreak and then every month thereafter, which resulted in the eradication of Stenotrophomonas infections in the emergency department.
Genetic Diversity of the S. maltophilia Strains by PFGE and MLST Analyses
The PFGE analysis revealed that the clinical and environmental strains of S. maltophilia clustered in seven pulsotypes (P1–P7) carrying between 17 and 21 DNA fragments, and the molecular weights ranged from <48.5 to >338 kb. A genetic diversity analysis of each S. maltophilia strain was performed with NTSYS-pc software to obtain a matrix of the absence/presence of the identified bands. The simple matching coefficient (Sokal and Michener, 1958) and UPGMA organization allowed the grouping of the S. maltophilia strains into seven pulsotypes, which exhibited 52% similarity among themselves (Figure 2). The cophenetic correlation coefficient obtained from the dendrogram using the Mantel test indicated the dispersion of the data and found a value of r = 0.98319. The seven pulsotypes showed the following distributions: 3.33% (1/30) of the S. maltophilia strains belonged to pulsotype P1 (strain 490U), 13.33% (4/30) belonged to pulsotype P2 (strains 54H, 931H, Env5, and Env6), 10.00% (3/30) belonged to pulsotype P3 (strains 35H, Env3, and Env4), 6.66% (2/30) belonged to pulsotype P4 (strains 160U and 178U), 16.66% (5/30) belonged to pulsotype P5 (strains 339H, 385H, 581H, 635H, and 653H), 10.00% (3/30) belonged to pulsotype P6 (strains 695H, 717H, and 723H), and 40.00% (12/30) belonged to pulsotype P7 (strains 41H, 50H, 63H, 483H, 488H, 499H, 899H, Env1, Env2, Env6, Env7, and Env8; Figure 2). The pulsotypes P2, P3, and P7 of S. maltophilia strains showed a clonal relationship with 100% similarity among themselves; however, P4, P5, and P6 were associated only with clinical S. maltophilia strains (Figure 2).
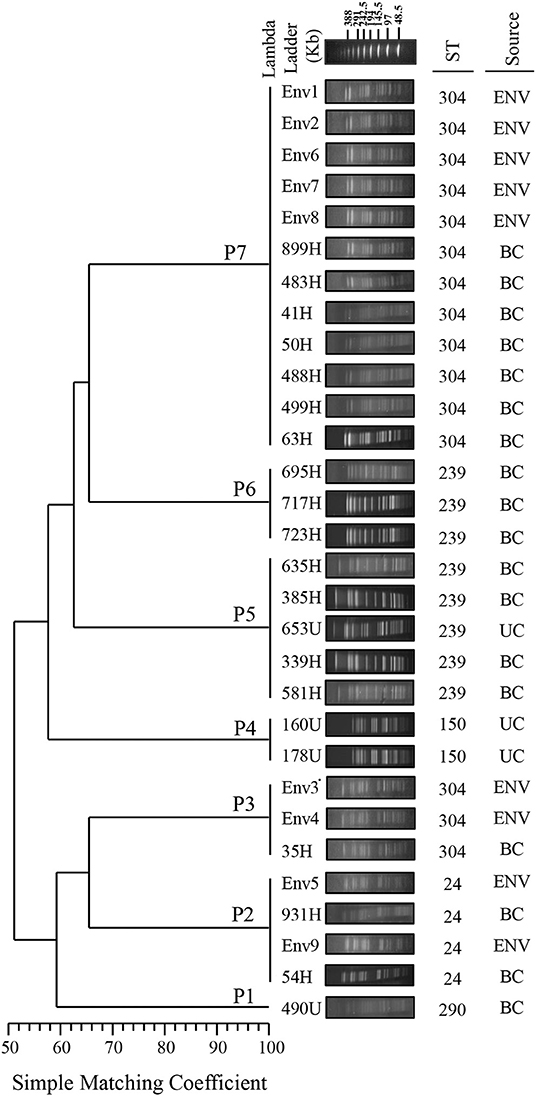
Figure 2. Dendrogram of the PFGE pulsotypes in 21 clinical strains and nine environmental strains of S. maltophilia. The diversity analysis was performed using NTSYS-pc software (version 2.0, Applied Biostatistics, Inc., NY, USA). The absence/presence matrix was evaluated based on the simple matching coefficient using the UPGMA algorithm. The dendrogram was evaluated using the cophenetic correlation coefficient obtained with the Mantel test, which indicated the dispersion of the data and showed a value of r = 0.98319. The diversity clusters and associated pulsotypes (P) are indicated, and the sequence type (ST) determined by MLST and the source are shown. Environmental (ENV), blood culture (BC) and urine culture (UC).
The MLST analysis showed that the clinical and environmental strains of S. maltophilia were grouped into five STs exhibiting the following distributions: ST304 [50.0% (15/30)], ST239 [26.66% (8/30)], ST24 [13.33% (4/30)], ST150 [6.66% (2/30)], and ST290 [3.33% (1/30); Figures 2, 3, Table S3].
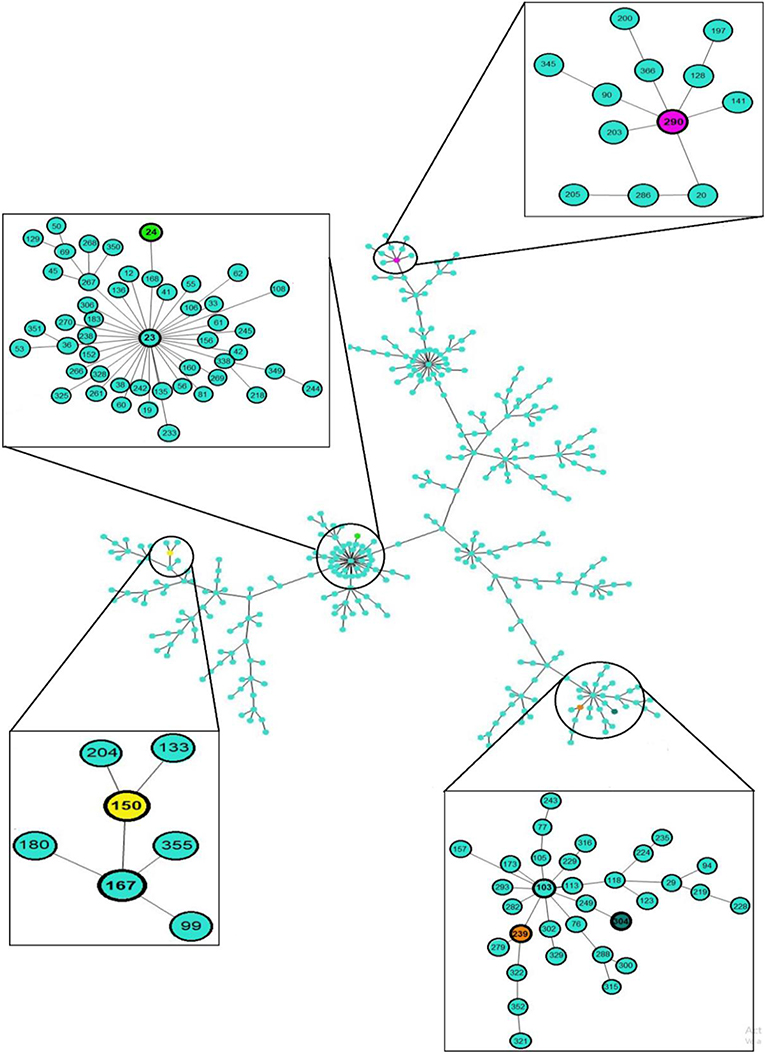
Figure 3. eBURST analysis of 30 clinical and environmental strains of S. maltophilia. The sequence type (ST) and clonal complex (CC) associated with the outbreak in the emergency department of the HIMFG are shown in boxes. The population distribution of the strains worldwide is also shown. Minimum spanning trees of the clinical and environmental strains of S. maltophilia were built using PHYLOViZ Online with the goeBURST algorithm, and this analysis considered 1,370 sequences reported in the MLST database.
The environmental S. maltophilia strains were related to two STs: ST304 [77.77% (7/9)] and ST24 [22.22% (2/9)]. The S. maltophilia strains from blood samples were associated with three STs: ST304 [47.06% (8/17)], ST239 [41.17% (7/17)], and ST24 [11.76% (2/17)]. In addition, the S. maltophilia strains from urine samples were associated with three STs: ST150 [50% (2/4)], ST239 [25% (1/4)], and ST290 [25% (1/4)]. The allelic profiles of the clinical and environmental strains of S. maltophilia are shown in Table S3. The clonal complexes (CCs) identified in the S. maltophilia strains were CC103, CC23, CC167, and CC290 (Figure 3, Table S3).
Resistance Profiles of the S. maltophilia Strains
The resistance profiles for six antibiotic categories were determined according to the CLSI (2018). S. maltophilia strains were considered MDR if they were not susceptible to at least one agent in three or more categories of antibiotics, whereas strains were considered pandrug-resistant (PDR) if they were not susceptible to all the agents in all the antibiotic categories (Magiorakos et al., 2012). Furthermore, S. maltophilia strains were considered resistant (R) if they were not susceptible to at least one agent in one to two antibiotic categories, and strains were considered susceptible if they were not resistant to all the antibiotic categories. According to the resistance profiles, 10.00% (3/30) of the S. maltophilia strains were PDR, 46.66% (14/30) were MDR, 33.33% (10/30) were R, and 10.00% (3/30) were susceptible to all the antibiotics tested. Moreover, 80.00% (24/30) of the S. maltophilia strains were resistant to TE, 76.66% (23/30) were resistant to SXT, and 23.33% (7/30) were producers of ESBL (Table 1).
Class 1, 2, and 3 integrons were identified in the following proportion: 80.00% (24/30) of the S. maltophilia strains exhibited class 1 integrons, 40.00% (12/30) exhibited class 2 integrons, and 6.66% (2/30) exhibited class three integrons. Additionally, the other resistance genes of the S. maltophilia strains included in this study were distributed as follows: 86.66% (26/30) of the strains contained the qnr gene, 100% (30/30) contained the sul1 gene, and 73.33% (22/30) contained the sul2 gene (Figure 4).
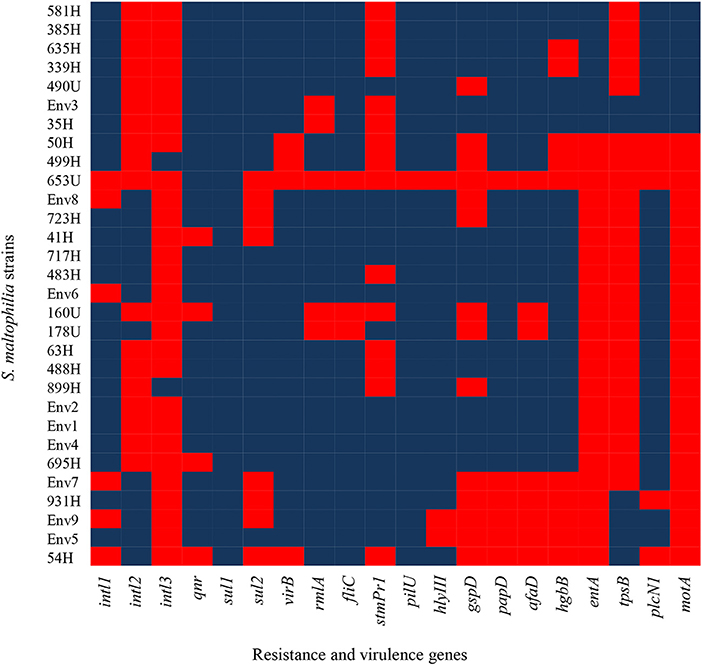
Figure 4. Heat map of putative virulence genes and resistance genes in the clinical and environmental strains of S. maltophilia. The blue and red colors indicate the presence and absence of a gene, respectively.
Frequency of Virulence Genes in the S. maltophilia Strains
The S. maltophilia strains related to virulence genes exhibited the following distribution: 86.6% (26/30) harbored the virB gene, 83.33% (25/30) harbored the rmlA gene, 90.0% (27/30) contained the fliC gene, 50.0% (15/30) contained the stmPr1 gene, 96.6% (29/30) contained the pilU gene, 90.0% (27/30) contained the hlyIII gene, 53.3% (16/30) contained the gspD gene, 80.0% (24/30) contained the papD gene, 73.33% (22/30) contained the afaD gene, 66.66% (20/30) contained the hgbB gene, 23.33% (7/30) contained the entA gene, 20.0% (6/30) contained the tpsB gene, 83.33% (25/30) contained the plcN1 gene, and 23.33% (7/30) contained the motA gene (Figure 4).
In addition, other virulence genes, such as zot, fhaB, frpC, fimH, lktD, and hcp, were not identified in the S. maltophilia strains (data not shown). By contrast, all environmental S. maltophilia strains 100% (9/9) contained the virB, fliC, pilU, plcNI, qnr, and sul1 genes, all S. maltophilia strains that originated from blood samples showed 100% (17/17) positivity for four genes (fliC, pilU, hlyIII, and sul1), and 100% (4/4) from urine samples showed positivity for the sul1 gene from urine samples (Figure 5).
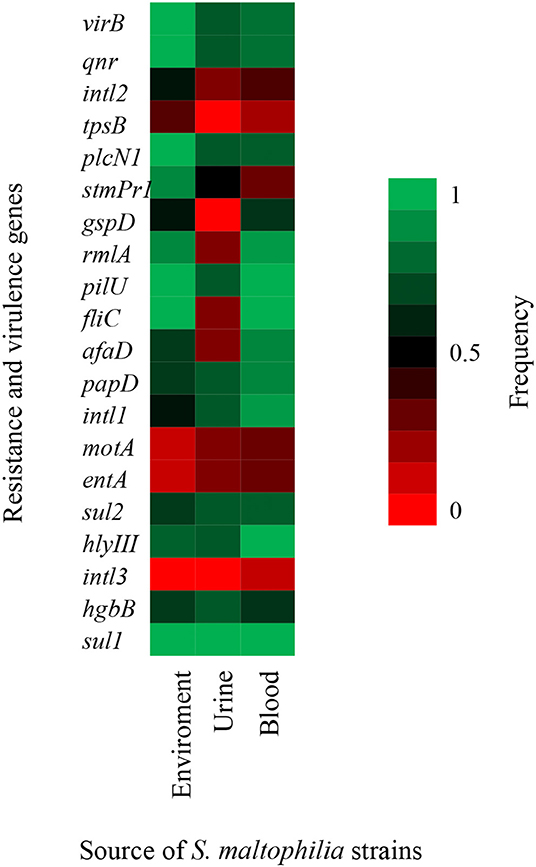
Figure 5. Heat map of putative virulence genes and resistance genes in nine strains of S. maltophilia from environmental sources, 17 strains from blood sources, and four strains from urinary sources. The percentage of strains harboring each gene is depicted by a color scale from red to green.
HTB-9 Cells Adhesion and Biofilm Formation Capacities of S. maltophilia Strains
The attachment of S. maltophilia strains to monolayers of HTB-9 cells exhibited a heterogeneous profile of adherence with different values of CFU/mL. The adherence levels ranged from 0.04 × 107 to 1.6 × 107 CFU/mL (Figure 6A) and were classified as weakly <0.9 × 107 CFU/mL), moderately (0.9–1.16 × 107 CFU/mL), or strongly (≥1.16 × 107 CFU/mL) adherent according to the mean value (1.05 × 107 CFU/mL, SD ± 1.29) obtained for the positive control (S. maltophilia ATCC 13637). The analysis of the S. maltophilia strains from environmental sources revealed that 55.5% (5/9) were weakly adherent, 44.4% (4/9) were moderately adherent, and none were strongly adherent (Figure 6A). Among the S. maltophilia clinical strains originating from blood, 41.17% (7/17) were weakly adherent, 29.41% (5/17) were moderately adherent, and 29.41% (5/17) were strongly adherent. In addition, 50.0% (2/4) of the S. maltophilia clinical strains originating from urine were weakly adherent, and the others 50% (2/4) were moderately adherent. Interestingly, the S. maltophilia clinical strains showed increased adherence compared with the S. maltophilia strains from environmental sources (Figure 6A).
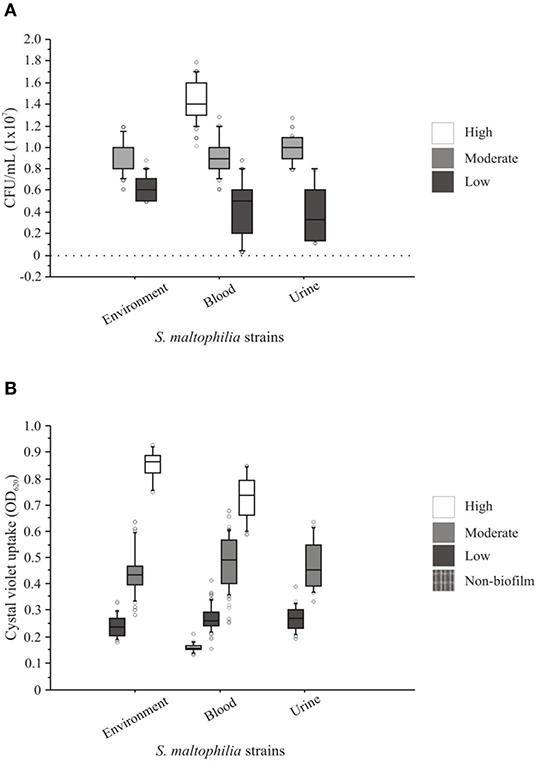
Figure 6. Adherence and biofilm patterns of clinical and environmental strains of S. maltophilia. (A) The adhesion assays were performed with human bladder HTB-9 cells. (B) The biofilm assays were based on the spectrophotometric quantification of the methanol retained by the 96-well microplate biofilm. The points outside the boxes represent the values of adhesion and biofilm formation outside the quartiles.
The S. maltophilia strains associated with the formation of biofilms showed a heterogeneous profile independent of their source of isolation. The strains were classified as follows: 10.0% (3/30) had a high biofilm-formation activity (>0.598 OD590), 46.66% (14/30) had a moderate biofilm-formation activity (>0.299 to ≤0.598 OD590), 36.66% (11/30) had a low biofilm-formation activity (>0.15 to ≤0.299 OD590), and 6.66% (2/30) did not form biofilms (≤0.15 OD590). In addition, 41.17% (7/17), 50% (2/2), and 55.55% (5/9) of the S. maltophilia strains originating from blood, urine, and the environment, respectively, showed moderate biofilm-formation activity (Figure 6B).
Twitching and Swimming Motilities of the S. maltophilia Strains
The twitching motility of the clinical and environmental strains of S. maltophilia showed heterogeneous profiles with different halo diameters: 13.33% (4/30) were classified as high (>9.0 mm/diameter), 43.33% (13/30) as moderate (>7.0 to ≤9.0 mm/diameter), 26.66% (8/30) as low (>6.0 to ≤7.0 mm/diameter), and 16.66% (5/30) did not show any twitching motility (≤6.0 mm/diameter) phenotype. Among the environmental S. maltophilia strains, 33.33% (3/9) exhibited low, 44.44% (4/9) showed moderate, and 22.22% (2/9) presented high twitching motility. In addition, 75% (3/4) of the S. maltophilia strains from urinary sources did not show any twitching phenotype. Interestingly, among the S. maltophilia strains from blood cultures, 11.76% (2/17) showed high, 52.94% (9/17) exhibited moderate, 23.52% (4/17) showed low, and 11.76% (2/7) presented no twitching motility (Figure 7).
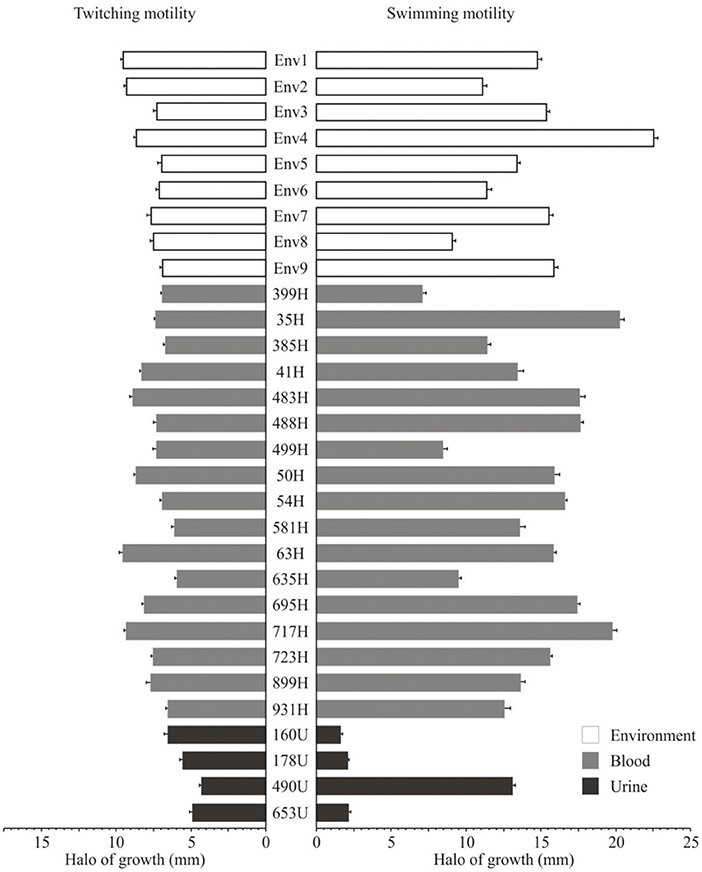
Figure 7. Twitching motility and swimming motility of the clinical and environmental strains of S. maltophilia associated with a hospital outbreak.
The swimming motility of the S. maltophilia strains showed the following distribution: 76.66% (23/30) presented high (>10.0 mm/diameter), 10.0% (3/30) exhibited moderate (>7.0 to ≤10.0 mm/diameter), and 3.33% (1/30) exhibited low motility (>6.0 to ≤7.0 mm/diameter). The environmental and blood culture strains were motile with rates of 88.88% (8/9) and 82.85% (14/17), respectively; however, 75% (3/4) of the strains from urinary sources lacked the fliC gene and showed no motile phenotype (Figure 7).
Association of S. maltophilia Strains Using Chord Graphs
The clinical data, resistance profiles, and adherence patterns and clonal relatedness of the clinical and environmental strains of S. maltophilia were visualized using chord diagram software. The analysis of these data indicated three main connections with a relationship among them (Figure 8). Environmental strains were associated with 80% (8/10) of the infected patients, and two patients (1 and 5) did not show a relationship with these strains. No association was observed between the urinary and environmental strains of S. maltophilia. The environmental strains (Env1, Env2, Env6, Env7, and Env8) showed a correlation with the blood-sourced strains in six patients, as indicated in a brown color, and the Env3 and Env4 strains were related to the blood sample from one patient. The Env5 and Env9 strains were related to two blood samples from one patient (Figure 8).
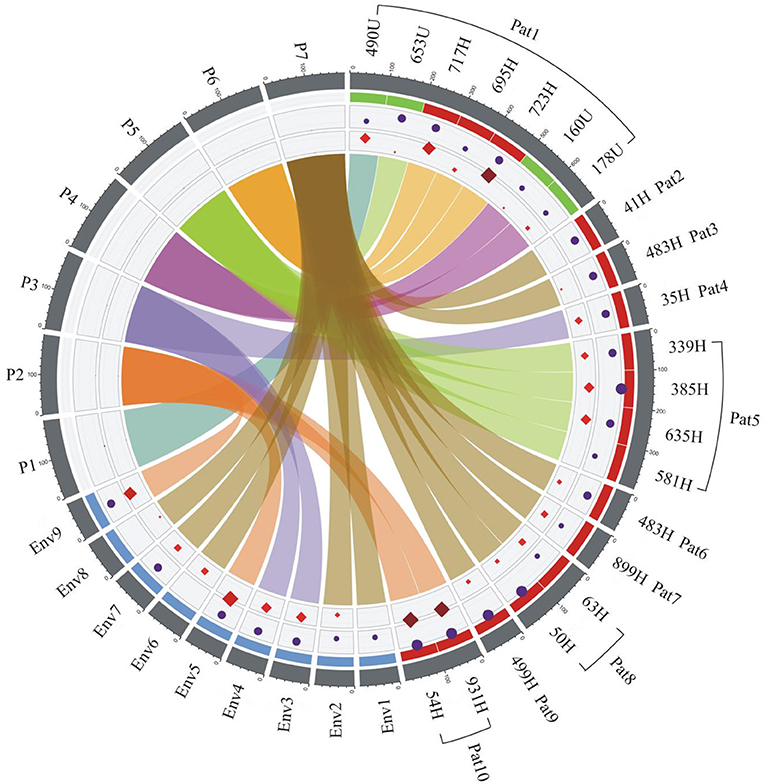
Figure 8. Epidemiological analysis of the clinical and environmental strains of S. maltophilia related to the outbreak. The associations among the source of isolation, pulsotypes (P1–P7), adherence, and resistance profiles of S. maltophilia strains were derived using ShinyCircos software. The outer ring shows the patient number (Pat1–Pat10) and source of strains: H, blood in red; U, urine in green; and Env1-9, environmental in blue. The inner rings are color coded: purple circles represent adherence, and the red diamonds illustrate the resistance profile of each strain. The size of the circles and diamonds is proportional to bacterial adherence (CFU/mL) and the number of antibiotic resistance genes, respectively. At the center, every pulsotype is associated with the clonal relatedness of the strain and is represented with a different chord color: P1 (turquoise), P2 (dark orange), P3 (blue), P4 (purple), P5 (green), P6 (light orange), and P7 (brown). The connected colors indicate the presence of a specific strain in either the patient samples or the environmental samples. The brown, purple, and dark orange colors indicate the faucet samples, and all three of these strains showed a connection with those from the patients: the strain represented in brown was associated with Pat2, 3, 6, 7, 8, and 9; the strains represented in purple were associated with Pat4; and the strains represented in dark orange were associated with Pat10. Pat1 and 5 were not associated with a faucet-sourced strain. Pat1 was associated with four different strains (turquoise, green, light orange, and purple), and one of these strains (green) was associated with Pat5. Env, environmental; Pat, patient. The number after Env or Pat indicates the number of the environmental or patient samples, which were numbered continuously.
Discussion
S. maltophilia is an aerobic, non-fermentative, gram-negative bacterium and an opportunistic pathogen that has been associated with serious infections in ICUs and nosocomial outbreaks (Gulcan et al., 2004; Guyot et al., 2013). The infection frequently occurs secondarily to the use of broad-spectrum antibiotics, prolonged hospitalization, ICU stays, mechanical ventilation, indwelling catheters and the use of equipment in contact with the respiratory tract (Pompilio et al., 2016). The HIMFG treats immunocompromised patients with diseases in specific organs, which increases the risk of opportunistic infections. In this study, we investigated an outbreak associated with S. maltophilia in the HIMFG and characterized the antibiotic resistance profiles, molecular epidemiology, and frequencies of virulence genes. S. maltophilia has been isolated from several nosocomial sources, such as blood-sampling tubes, ventilator circuits, dialysis machines, shower heads, sink traps, water faucets, and the hands of healthcare personnel (Denton and Kerr, 1998). S. maltophilia bloodstream infections are associated with the inappropriate use of antibiotics, long-term hospital stays, chronic diseases (including cancer), and immunocompromised patients (Wu et al., 2006; Chang et al., 2014).
S. maltophilia has high genetic diversity, as demonstrated with various typing methods (Gherardi et al., 2015; Pompilio et al., 2016; Alcaraz et al., 2018; Guvenir et al., 2018). However, in Mexico, 75–100% similarity has been obtained among strains collected from the same hospital source over a period of 7 years (Flores-Trevino et al., 2014). In this study, the PFGE and MLST analyses grouped the environmental strains of S. maltophilia into three pulsotypes and two STs. P3 (Env3 and Env4) and P7 (Env1, Env2, Env6, Env7, and Env8) were associated with ST304, whereas P2 (Env5 and Env9) was associated with ST24. These results indicate that PFGE remains the gold standard for DNA fingerprinting and exhibits substantial power for genetic and epidemiological discrimination in the evaluation of hospital outbreaks (Gherardi et al., 2015). However, MLST enabled identification of the clonal groups associated with the strain STs, which facilitates their monitoring worldwide. In addition, MLST provides information about the population structure as well as the long-term global and evolution history (Gherardi et al., 2015). For instance in our study, the S. maltophilia strains isolated from blood samples were associated with five pulsotypes and three STs. P2 was related to ST24, P3 was associated with ST304, P7 was related to ST304, P5 was related to ST239, and P6 (strains 695H, 717H, and 723H) was associated with ST239. The S. maltophilia strains isolated from urine samples were mainly associated with P4 and ST150. The clinical S. maltophilia strains were highly related and distributed as follows: P1 was related to ST290, P4 was related to ST150, P5 was related to ST239, and P6 was related to ST239. Furthermore, these clusters did not show a clonal relationship with S. maltophilia strains from environmental sources, which suggested an additional source of infection.
Interestingly, 47.61% (10/21) of the clinical S. maltophilia strains were clonally related to environmental strains obtained from faucets and spouts. Our data suggested that the environmental strains collected from faucets were identical to the clinical strains obtained from eight patients, which suggests a partial source of the outbreak. The HIMFG has as a policy of hand washing before taking any sample or performing any invasive procedure as well as before entering the emergency department (De la Rosa-Zamboni et al., 2018). A previous outbreak of S. maltophilia strains isolated from blood was associated with cross-contamination from the hands of healthcare workers (Park et al., 2008). The findings found in this study support the hypothesis that contamination by health workers is a probable source of infection and transmission among patients (De la Rosa-Zamboni et al., 2018). During the outbreak, the results obtained after the implementation of faucet disinfection and sterilization as a control measure indicated that cross-contamination is important for strain transmission in the emergency department.
Seven S. maltophilia strains with different infection processes were isolated from one patient. These strains were grouped into four pulsotypes (P1, P4, P5, and P6) and three STs (290, 150, and 239), which indicated the existence of a mixed infection in this patient. The urine-sourced S. maltophilia strain 490U included in P1 showed no relationship with other strains, which indicated that this strain might be found in different environmental sources possibly of community origin (Chang et al., 2014). The urine-sourced S. maltophilia strain 653U was grouped into P5 with four strains that were obtained from the blood of two other pediatric patients, which suggested the existence of cross-colonization in the patients with the same strains.
S. maltophilia strains have been associated with outbreaks in ICUs due to their MDR profiles, which enhance cross-nosocomial colonization (Gales et al., 2001; Sanchez, 2015). The MDR profiles of the S. maltophilia strains exhibit resistance to SXT and TE, which are recommended as the agents of choice for the treatment of this species (Flores-Trevino et al., 2014; Hu et al., 2016; Brooke et al., 2017). Interestingly, three S. maltophilia clinical strains (54H, 931H, and 178U) presented a PDR profile, which might be characteristic of circulation in hospital environments. In addition, multidrug resistance and resistance to stress are characteristics that facilitate the competence of S. maltophilia in areas with intensive microbial activity, such as the nosocomial environment (An and Berg, 2018). The sul and dfrA genes, class 1 integrons and mobile genetic elements contribute to SXT resistance (Chang et al., 2007; Hu et al., 2011). Moreover, resistance to SXT is associated with quaternary ammonium compounds (QACs); thus, the qacEΔ1 gene is related to SXT resistance. Therefore, the use of disinfectants containing QACs might increase the risk of SXT resistance in hospital settings and natural environments (Gaze et al., 2005). Unfortunately, only the sul1 and the sul2 genes were included in this study. Further studies are needed to detect other genes, such as aqEΔ1-sul1, dfrA12, dfrA17, and dfrA27, which are involved in the wide and rapid dissemination of resistance genes in bacteria in other countries (Hu et al., 2016). It is important to comment that the integrase gene classes 1, 2, and 3 were used as indicators of the presence of integrons in this study. However, additional sequencing studies of the integron variable region are necessary for identification of the cassette genes associated with resistance in S. maltophilia strains. Interestingly, the qnr gene that encodes resistance to quinolones was the most important characteristic of environmental S. maltophilia strains, which suggests that multidrug resistance favors competition in ecological niches of several microorganisms (Malekan et al., 2017). The presence of intl1 is indicative of the class 1 integron and was found in a high percentage of S. maltophilia strains originating from blood samples, which indicates that it could serve as a mechanism for facilitating the dissemination of resistance at the hospital level (Malekan et al., 2017).
The virulence genes of S. maltophilia strains from clinical and environmental sources have been described; however, the functions of other virulence genes have not yet been described, and these genes are considered putative virulence genes (Adamek et al., 2014). In this study, the gene profiles of the S. maltophilia strains from environmental and clinical sources showed high frequencies of virulence genes involved in swimming motility (≥80%), secretion systems (0–50%), toxins (20–60%), and siderophores (20%). The pilU (pili type 4) and fliC (flagellin) genes in the environmental and blood-sourced strains of S. maltophilia suggest an association with biofilm formation to facilitate their persistence in faucets. The virB gene (TSS4) was strongly associated with environmental strains, which suggests that the participation of secretion systems is essential for environmental prevalence. In addition, hemolysin III is a toxin that facilitates strain survival in the bloodstream (Adamek et al., 2014).
S. maltophilia strains have the ability to adhere to and form biofilms on medical devices, such as prosthetic devices and blood and urinary catheters, and have been associated with hospital outbreaks. Biofilms provide protection against antibiotics and immune system defenses (De Oliveira-Garcia et al., 2003). In this study, S. maltophilia strains from environmental and clinical sources showed inherent profiles of biofilm formation, which indicates that the biofilm-formation capacity is essential for bacterial persistence regardless of the colonization sites; indeed, only two blood-sourced strains did not form biofilms (Pompilio et al., 2008, 2011). The flagella of S. maltophilia strains are structural appendages that contribute to the appearance of hospital outbreaks (De Oliveira-Garcia et al., 2002). Our assessment of the swimming motility and the finding that 90% of the S. maltophilia strains that harbored the fliC gene, including the environmental and clinical strains, showed a heterogeneous profile reflecting a high swimming motility, which indicated that the flagella are required for the colonization of these strains in the host. Under these same conditions, 56.66% of all the S. maltophilia strains (43.33% of the blood-sourced strains and 13.33% of the urine-sourced strains) showed twitching motility. The strains from environmental and blood sources harbored the pilU gene, which is associated with the presence of the type 4 pili in S. maltophilia.
Adherence to epithelial cells is the initial step in the colonization or invasion of different epithelial cells, such as those in the respiratory and urinary tracts, injured skin and wounds, by S. maltophilia. Our results showed that the blood-sourced strains exhibited increased adherence to human bladder HTB-9 cells compared with the strains from environmental and urinary sources. The ability to adhere to plastic venous catheters and survive within intravenous solutions can contribute to the pathogenesis of bloodline-associated infections (De Oliveira-Garcia et al., 2003). Additionally, adherence to epithelial cells is related to the presence of fimbriae or pili that directly mediate the binding of bacteria to their target host cells or indirectly mediate binding by the formation of cross-junctions between cells (De Oliveira-Garcia et al., 2003). Thus, the typed genotypes of the S. maltophilia strains isolated from blood samples indicated the presence of a high percentage of genes associated with colonization, such as papD, afaD, pilU, and fliC, which is in agreement with the literature. The finding that the S. maltophilia strains of blood origin adhered to HTB-9 cells indicates their participation in the permanence and dissemination of strains in the hospital environment.
Finally, the outbreak was controlled through the routine disinfection of faucets and spouts. The policies for the routine or terminal disinfection of a clinical ward do not usually include disinfection of the internal faucet parts (Rutala and Weber, 2008; Chou, 2014). In an emergency department, water splashes generated between spouts and faucets can contaminate the internal part of faucets that harbor environmental bacteria with the ability to form biofilms, which is favored by several virulence factors. The emergency department of the HIMFG requires renovation and structural optimization, including the installation of sinks with adequate dimensions, to avoid bacterial persistence in areas at a high risk of contamination, and these measures are ongoing. In conclusion, the outbreak associated with S. maltophilia in the HIMFG was partially related to faucet contamination in the emergency department. Other pulsotypes and STs (150, 239, and 290) were not found in the environmental strains of S. maltophilia, which suggests other infection sources. Their clonality, selected virulence genes, and resistance mechanisms are attributes associated with the success of infection and persistence by these strains in the hospital and facilitated the outbreak. Finally, these findings support the need for the implementation of disinfection policies that include the disinfection of faucets as a necessary strategy for the control of outbreaks in clinical areas.
Data Availability Statement
All datasets generated for this study are included in the article/Supplementary Material.
Ethics Statement
The projects with the registration numbers HIM/2019/067, HIM/2018/045FF SSA.1503, HIM/2018/049FF SSA.1504, and HIM/2016/099 SSA.1329 were submitted, reviewed, and approved by the Research Committee (Dr. Juan Garduño Espinosa, Research Director), Ethics Committee (Dr. Luis Jasso Gutiérrez, President of the Research Ethics Committee), and Biosecurity Committee (Dr. Marcela Salazar García, Leader of Biomedical Research Service and Research Biosecurity Committee) of the Children's Hospital of México Federico Gómez (HIMFG). The clinical S. maltophilia strains included in this study were obtained from blood and urine samples using routine clinical diagnostic procedures. Signed informed consent was provided at the time of sampling in the Central Clinical Laboratory of the Hospital. The informed consent form mentions that the sample might be used for research purposes according to the following reference: General Health Law chap. IV. Art. 80, 81, 82, and 83. NOM-007-SSA3-2011. México.
Author Contributions
SO and JX-C designed and conceived the experiments, wrote and reviewed the manuscript. SO, GE-V, VC-D, and JM-R performed the experiments. SO, SZ-V, VL-P, AC-C, MM-P, IP-O, DR-Z, and JX-C analyzed the data. SO, SZ-V, AC-C, DR-Z, MH, CO, IF-H, and JX-C contributed reagents/materials/analytical tools.
Funding
This work was supported by Public Federal Funds grants (HIM/2019/067, HIM/2018/045FF SSA. 1503, HIM/2018/049FF SSA. 1504, and HIM/2016/099 SSA. 1329) from the HIMFG. This work was also supported by the Consejo Nacional de Ciencia y Tecnología (CONACYT) at Attention to National Problems under account number 1764.
Conflict of Interest
The authors declare that the research was conducted in the absence of any commercial or financial relationships that could be construed as a potential conflict of interest.
Acknowledgments
We thank QBP Marco Antonio Flores Oropeza for providing technical assistance.
Supplementary Material
The Supplementary Material for this article can be found online at: https://www.frontiersin.org/articles/10.3389/fcimb.2020.00050/full#supplementary-material
References
Adamek, M., Linke, B., and Schwartz, T. (2014). Virulence genes in clinical and environmental Stenotrophomas maltophilia isolates: a genome sequencing and gene expression approach. Microb. Pathog. 67–68, 20–30. doi: 10.1016/j.micpath.2014.02.001
Alcaraz, E., Garcia, C., Papalia, M., Vay, C., Friedman, L., and Passerini de Rossi, B. (2018). Stenotrophomonas maltophilia isolated from patients exposed to invasive devices in a university hospital in Argentina: molecular typing, susceptibility and detection of potential virulence factors. J. Med. Microbiol. 67, 992–1002. doi: 10.1099/jmm.0.000764
An, S. Q., and Berg, G. (2018). Stenotrophomonas maltophilia. Trends Microbiol. 26, 637–638. doi: 10.1016/j.tim.2018.04.006
Botana-Rial, M., Leiro-Fernandez, V., Nunez-Delgado, M., Alvarez-Fernandez, M., Otero-Fernandez, S., Bello-Rodriguez, H., et al. (2016). A pseudo-outbreak of Pseudomonas putida and Stenotrophomonas maltophilia in a bronchoscopy unit. Respiration 92, 274–278. doi: 10.1159/000449137
Brooke, J. S. (2012). Stenotrophomonas maltophilia: an emerging global opportunistic pathogen. Clin. Microbiol. Rev. 25, 2–41. doi: 10.1128/CMR.00019-11
Brooke, J. S., Di Bonaventura, G., Berg, G., and Martinez, J. L. (2017). Editorial: a multidisciplinary look at Stenotrophomonas maltophilia: an emerging multi-drug-resistant global opportunistic pathogen. Front. Microbiol. 8:1511. doi: 10.3389/fmicb.2017.01511
Chang, L. L., Lin, H. H., Chang, C. Y., and Lu, P. L. (2007). Increased incidence of class 1 integrons in trimethoprim/sulfamethoxazole-resistant clinical isolates of Stenotrophomonas maltophilia. J. Antimicrob. Chemother. 59, 1038–1039. doi: 10.1093/jac/dkm034
Chang, Y. T., Lin, C. Y., Lu, P. L., Lai, C. C., Chen, T. C., Chen, C. Y., et al. (2014). Stenotrophomonas maltophilia bloodstream infection: comparison between community-onset and hospital-acquired infections. J. Microbiol. Immunol. Infect. 47, 28–35. doi: 10.1016/j.jmii.2012.08.014
Chou, T. (2014). Environmental Services. Association for Professionals in Infection. Control and Epidemiology (APIC). Available online at: https://apic.org/resources/topic-specific-infection-prevention/environmental-services/ (accessed September 23, 2019).
CLSI (2018). Performance Standards for Antimicrobial Susceptibility Testing. CLSI Supplement M100. Wayne, PA: Clinical and Laboratory Standards Institute.
Crossman, L. C., Gould, V. C., Dow, J. M., Vernikos, G. S., Okazaki, A., Sebaihia, M., et al. (2008). The complete genome, comparative and functional analysis of Stenotrophomonas maltophilia reveals an organism heavily shielded by drug resistance determinants. Genome Biol. 9:R74. doi: 10.1186/gb-2008-9-4-r74
De la Rosa-Zamboni, D., Ochoa, S. A., Laris-Gonzalez, A., Cruz-Cordova, A., Escalona-Venegas, G., Perez-Avendano, G., et al. (2018). Everybody hands-on to avoid ESKAPE: effect of sustained hand hygiene compliance on healthcare-associated infections and multidrug resistance in a paediatric hospital. J. Med. Microbiol. 67, 1761–1771. doi: 10.1099/jmm.0.000863
De Oliveira-Garcia, D., Dall'Agnol, M., Rosales, M., Azzuz, A. C., Alcantara, N., Martinez, M. B., et al. (2003). Fimbriae and adherence of Stenotrophomonas maltophilia to epithelial cells and to abiotic surfaces. Cell. Microbiol. 5, 625–636. doi: 10.1046/j.1462-5822.2003.00306.x
De Oliveira-Garcia, D., Dall'Agnol, M., Rosales, M., Azzuz, A. C., Martinez, M. B., and Giron, J. A. (2002). Characterization of flagella produced by clinical strains of Stenotrophomonas maltophilia. Emerg. Infect. Dis. 8, 918–923. doi: 10.3201/eid0809.010535
De Vidipo, L. A., De Marques, E. A., Puchelle, E., and Plotkowski, M. C. (2001). Stenotrophomonas maltophilia interaction with human epithelial respiratory cells in vitro. Microbiol. Immunol. 45, 563–569. doi: 10.1111/j.1348-0421.2001.tb01287.x
Denton, M., and Kerr, K. G. (1998). Microbiological and clinical aspects of infection associated with Stenotrophomonas maltophilia. Clin. Microbiol. Rev. 11, 57–80. doi: 10.1128/CMR.11.1.57
Flores-Trevino, S., Gutierrez-Ferman, J. L., Morfin-Otero, R., Rodriguez-Noriega, E., Estrada-Rivadeneyra, D., Rivas-Morales, C., et al. (2014). Stenotrophomonas maltophilia in Mexico: antimicrobial resistance, biofilm formation and clonal diversity. J. Med. Microbiol. 63, 1524–1530. doi: 10.1099/jmm.0.074385-0
Francisco, A. P., Bugalho, M., Ramirez, M., and Carrico, J. A. (2009). Global optimal eBURST analysis of multilocus typing data using a graphic matroid approach. BMC Bioinform. 10:152. doi: 10.1186/1471-2105-10-152
Gales, A. C., Jones, R. N., Forward, K. R., Linares, J., Sader, H. S., and Verhoef, J. (2001). Emerging importance of multidrug-resistant Acinetobacter species and Stenotrophomonas maltophilia as pathogens in seriously ill patients: geographic patterns, epidemiological features, and trends in the SENTRY antimicrobial surveillance program (1997-1999). Clin. Infect. Dis. 32(Suppl. 2), S104–S113. doi: 10.1086/320183
Gaze, W. H., Abdouslam, N., Hawkey, P. M., and Wellington, E. M. (2005). Incidence of class 1 integrons in a quaternary ammonium compound-polluted environment. Antimicrob. Agents Chemother. 49, 1802–1807. doi: 10.1128/AAC.49.5.1802-1807.2005
Gherardi, G., Creti, R., Pompilio, A., and Di Bonaventura, G. (2015). An overview of various typing methods for clinical epidemiology of the emerging pathogen Stenotrophomonas maltophilia. Diagn. Microbiol. Infect. Dis. 81, 219–226. doi: 10.1016/j.diagmicrobio.2014.11.005
Gulcan, H., Kuzucu, C., and Durmaz, R. (2004). Nosocomial Stenotrophomonas maltophilia cross-infection: three cases in newborns. Am. J. Infect. Control 32, 365–368. doi: 10.1016/j.ajic.2004.07.003
Guvenir, M., Otlu, B., Tunc, E., Aktas, E., and Suer, K. (2018). High genetic diversity among Stenotrophomonas maltophilia isolates from single hospital: nosocomial outbreaks or genotypic profile changes during subcultures. Malays. J. Med. Sci. 25, 40–49. doi: 10.21315/mjms2018.25.2.5
Guyot, A., Turton, J. F., and Garner, D. (2013). Outbreak of Stenotrophomonas maltophilia on an intensive care unit. J. Hosp. Infect. 85, 303–307. doi: 10.1016/j.jhin.2013.09.007
Hu, L. F., Chang, X., Ye, Y., Wang, Z. X., Shao, Y. B., Shi, W., et al. (2011). Stenotrophomonas maltophilia resistance to trimethoprim/sulfamethoxazole mediated by acquisition of sul and dfrA genes in a plasmid-mediated class 1 integron. Int. J. Antimicrob. Agents 37, 230–234. doi: 10.1016/j.ijantimicag.2010.10.025
Hu, L. F., Chen, G. S., Kong, Q. X., Gao, L. P., Chen, X., Ye, Y., et al. (2016). Increase in the prevalence of resistance determinants to trimethoprim/sulfamethoxazole in clinical Stenotrophomonas maltophilia isolates in China. PLoS ONE 11:e0157693. doi: 10.1371/journal.pone.0157693
Jacquier, H., Carbonnelle, E., Corvec, S., Illiaquer, M., Le Monnier, A., Bille, E., et al. (2011). Revisited distribution of nonfermenting gram-negative bacilli clinical isolates. Eur. J. Clin. Microbiol. Infect. Dis. 30, 1579–1586. doi: 10.1007/s10096-011-1263-5
Jolley, K. A., Bray, J. E., and Maiden, M. C. J. (2018). Open-access bacterial population genomics: BIGSdb software, the PubMLST.org website and their applications. Wellcome Open Res. 3:124. doi: 10.12688/wellcomeopenres.14826.1
Kaiser, S., Biehler, K., and Jonas, D. (2009). A Stenotrophomonas maltophilia multilocus sequence typing scheme for inferring population structure. J. Bacteriol. 191, 2934–43. doi: 10.1128/JB.00892-08
Landau, S., and Rabe-Hesketh, S. (1999). Software review: StatView for windows, version 5.0. Stat. Methods Med. Res. 8, 337–341. doi: 10.1177/096228029900800411
Lin, Y. T., Huang, Y. W., Chen, S. J., Chang, C. W., and Yang, T. C. (2015). The SmeYZ efflux pump of Stenotrophomonas maltophilia contributes to drug resistance, virulence-related characteristics, and virulence in mice. Antimicrob. Agents Chemother. 59, 4067–4073. doi: 10.1128/AAC.00372-15
Magiorakos, A. P., Srinivasan, A., Carey, R. B., Carmeli, Y., Falagas, M. E., Giske, C. G., et al. (2012). Multidrug-resistant, extensively drug-resistant and pandrug-resistant bacteria: an international expert proposal for interim standard definitions for acquired resistance. Clin. Microbiol. Infect. 18, 268–281. doi: 10.1111/j.1469-0691.2011.03570.x
Malekan, M., Tabaraie, B., Akhoundtabar, L., Afrough, P., and Behrouzi, A. (2017). Distribution of class I integron and smqnr resistance gene among Stenotrophomonas maltophilia isolated from clinical samples in Iran. Avicenna J. Med. Biotechnol. 9, 138–141.
Mojica, M. F., Rutter, J. D., Taracila, M., Abriata, L. A., Fouts, D. E., Papp-Wallace, K. M., et al. (2019). Population structure, molecular epidemiology, and beta-lactamase diversity among Stenotrophomonas maltophilia isolates in the United States. MBio 10, e00405–00419. doi: 10.1128/mBio.00405-19
Nicoletti, M., Iacobino, A., Prosseda, G., Fiscarelli, E., Zarrilli, R., De Carolis, E., et al. (2011). Stenotrophomonas maltophilia strains from cystic fibrosis patients: genomic variability and molecular characterization of some virulence determinants. Int. J. Med. Microbiol. 301, 34–43. doi: 10.1016/j.ijmm.2010.07.003
Ochoa, S. A., Cruz-Cordova, A., Luna-Pineda, V. M., Reyes-Grajeda, J. P., Cazares-Dominguez, V., Escalona, G., et al. (2016). Multidrug- and extensively drug-resistant uropathogenic escherichia coli clinical strains: phylogenetic groups widely associated with integrons maintain high genetic diversity. Front. Microbiol. 7:2042. doi: 10.3389/fmicb.2016.02042
Ozkaya, E., Aydin, F., Bayramoglu, G., Buruk, C. K., and Sandalli, C. (2014). Investigation of integrons, sul1-2 and dfr genes in trimethoprim-sulfametoxazole-resistant Stenotrophomonas maltophilia strains isolated from clinical samples. Mikrobiyol. Bul. 48, 201–212. doi: 10.5578/mb.7262
Park, Y. S., Kim, S. Y., Park, S. Y., Kang, J. H., Lee, H. S., Seo, Y. H., et al. (2008). Pseudooutbreak of Stenotrophomonas maltophilia bacteremia in a general ward. Am. J. Infect. Control 36, 29–32. doi: 10.1016/j.ajic.2006.12.013
Pompilio, A., Crocetta, V., Ghosh, D., Chakrabarti, M., Gherardi, G., Vitali, L. A., et al. (2016). Stenotrophomonas maltophilia phenotypic and genotypic diversity during a 10-year colonization in the lungs of a cystic fibrosis patient. Front. Microbiol. 7:1551. doi: 10.3389/fmicb.2016.01551
Pompilio, A., Piccolomini, R., Picciani, C., D'Antonio, D., Savini, V., and Di Bonaventura, G. (2008). Factors associated with adherence to and biofilm formation on polystyrene by Stenotrophomonas maltophilia: the role of cell surface hydrophobicity and motility. FEMS Microbiol. Lett. 287, 41–47. doi: 10.1111/j.1574-6968.2008.01292.x
Pompilio, A., Pomponio, S., Crocetta, V., Gherardi, G., Verginelli, F., Fiscarelli, E., et al. (2011). Phenotypic and genotypic characterization of Stenotrophomonas maltophilia isolates from patients with cystic fibrosis: genome diversity, biofilm formation, and virulence. BMC Microbiol. 11:159. doi: 10.1186/1471-2180-11-159
Rutala, W. A., and Weber, D. J. (2008). Guideline for Disinfection and Sterilization in Healthcare Facilities. 1-163. Available online at: https://www.cdc.gov/~infectioncontrol/pdf/guidelines/disinfection-guidelines-H.pdf (accessed September 23, 2019).
Sanchez, M. B. (2015). Antibiotic resistance in the opportunistic pathogen Stenotrophomonas maltophilia. Front. Microbiol. 6:658. doi: 10.3389/fmicb.2015.00658
Sanchez, P., Alonso, A., and Martinez, J. L. (2002). Cloning and characterization of SmeT, a repressor of the Stenotrophomonas maltophilia multidrug efflux pump SmeDEF. Antimicrob. Agents Chemother. 46, 3386–3393. doi: 10.1128/AAC.46.11.3386-3393.2002
Sokal, R. R., and Michener, A. (1958). A statistical method for evaluating systematic relationships. Univ. Kan. Sci. Bull. 38, 1409–1438.
Tatman-Otkun, M., Gurcan, S., Ozer, B., Aydoslu, B., and Bukavaz, S. (2005). The antimicrobial susceptibility of Stenotrophomonas maltophilia isolates using three different methods and their genetic relatedness. BMC Microbiol. 5:24. doi: 10.1186/1471-2180-5-24
Tenover, F. C., Arbeit, R. D., Goering, R. V., Mickelsen, P. A., Murray, B. E., Persing, D. H., et al. (1995). Interpreting chromosomal DNA restriction patterns produced by pulsed-field gel electrophoresis: criteria for bacterial strain typing. J. Clin. Microbiol. 33, 2233–2239. doi: 10.1128/JCM.33.9.2233-2239.1995
Toleman, M. A., Bennett, P. M., Bennett, D. M., Jones, R. N., and Walsh, T. R. (2007). Global emergence of trimethoprim/sulfamethoxazole resistance in Stenotrophomonas maltophilia mediated by acquisition of sul genes. Emerg. Infect. Dis. 13, 559–565. doi: 10.3201/eid1304.061378
Trifonova, A., and Strateva, T. (2019). Stenotrophomonas maltophilia- a low-grade pathogen with numerous virulence factors. Infect. Dis. 51, 168–178. doi: 10.1080/23744235.2018.1531145
Valdezate, S., Vindel, A., Martin-Davila, P., Del Saz, B. S., Baquero, F., and Canton, R. (2004). High genetic diversity among Stenotrophomonas maltophilia strains despite their originating at a single hospital. J. Clin. Microbiol. 42, 693–699. doi: 10.1128/JCM.42.2.693-699.2003
Vidigal, P. G., Dittmer, S., Steinmann, E., Buer, J., Rath, P. M., and Steinmann, J. (2014). Adaptation of Stenotrophomonas maltophilia in cystic fibrosis: molecular diversity, mutation frequency and antibiotic resistance. Int. J. Med. Microbiol. 304, 613–619. doi: 10.1016/j.ijmm.2014.04.002
Waite, T. D., Georgiou, A., Abrishami, M., and Beck, C. R. (2016). Pseudo-outbreaks of Stenotrophomonas maltophilia on an intensive care unit in England. J. Hosp. Infect. 92, 392–396. doi: 10.1016/j.jhin.2015.12.014
Wu, P. S., Lu, C. Y., Chang, L. Y., Hsueh, P. R., Lee, P. I., Chen, J. M., et al. (2006). Stenotrophomonas maltophilia bacteremia in pediatric patients–a 10-year analysis. J. Microbiol. Immunol. Infect. 39, 144–149.
Keywords: outbreak, S. maltophilia, molecular typing, virulence, resistance
Citation: Cruz-Córdova A, Mancilla-Rojano J, Luna-Pineda VM, Escalona-Venegas G, Cázares-Domínguez V, Ormsby C, Franco-Hernández I, Zavala-Vega S, Hernández MA, Medina-Pelcastre M, Parra-Ortega I, Rosa-Zamboni DDl, Ochoa SA and Xicohtencatl-Cortes J (2020) Molecular Epidemiology, Antibiotic Resistance, and Virulence Traits of Stenotrophomonas maltophilia Strains Associated With an Outbreak in a Mexican Tertiary Care Hospital. Front. Cell. Infect. Microbiol. 10:50. doi: 10.3389/fcimb.2020.00050
Received: 26 September 2019; Accepted: 27 January 2020;
Published: 18 February 2020.
Edited by:
Jean-ralph Zahar, Hôpital Avicenne, FranceReviewed by:
Krisztina M. Papp-Wallace, Louis Stokes Cleveland VA Medical Center, United StatesCarlos Juan, Instituto de Investigación Sanitaria de Palma (IdISPa), Spain
Copyright © 2020 Cruz-Córdova, Mancilla-Rojano, Luna-Pineda, Escalona-Venegas, Cázares-Domínguez, Ormsby, Franco-Hernández, Zavala-Vega, Hernández, Medina-Pelcastre, Parra-Ortega, Rosa-Zamboni, Ochoa and Xicohtencatl-Cortes. This is an open-access article distributed under the terms of the Creative Commons Attribution License (CC BY). The use, distribution or reproduction in other forums is permitted, provided the original author(s) and the copyright owner(s) are credited and that the original publication in this journal is cited, in accordance with accepted academic practice. No use, distribution or reproduction is permitted which does not comply with these terms.
*Correspondence: Daniela De la Rosa-Zamboni, rzdaniela@hotmail.com; Sara A. Ochoa, saraariadnah@hotmail.com
Juan Xicohtencatl-Cortes, juanxico@yahoo.com