- 1Department of Clinical Veterinary Medicine, College of Veterinary Medicine, China Agricultural University, Beijing, China
- 2Department of Production Animal Health, Faculty of Veterinary Medicine, University of Calgary, Calgary, AB, Canada
- 3Department of Veterinary Clinical and Diagnostic Sciences, Faculty of Veterinary Medicine, University of Calgary, Calgary, AB, Canada
Prototheca bovis (formerly P. zopfii genotype-II) is an opportunistic, achlorophyllous alga that causes mastitis in cows and skin disease in cats and dogs, as well as cutaneous lesions in both immunocompetent and immunosuppressed humans. Antifungal medications are commonly ineffective. This study aimed to investigate innate immune responses contributed by cathelicidins to P. bovis in the mammary gland using a mastitis model in mice deficient in the sole murine cathelicidin (Camp). We determined P. bovis caused acute mastitis in mice and induced Camp gene transcription. Whereas, Camp−/− and Camp+/+ littermates had similar local algae burden, Camp+/+ mice produced more pro-inflammatory cytokines, TNF-α, and Cxcl-1. Likewise, Camp+/+ bone marrow-derived macrophages were more responsive to P. bovis, producing more TNF-α and Cxcl-1. Human cathelicidin (LL-37) exhibited a different effect against P. bovis; it had direct algicidal activity against P. bovis and lowered TNF-α, Cxcl-1, and IL-1β production in both cultured murine macrophages and mammary epithelial cells exposed to the pathogenic algae. In conclusion, cathelicidins were involved in protothecosis pathogenesis, with unique roles among the diverse peptide family. Whereas, endogenous cathelicidin (Camp) was key in mammary gland innate defense against P. bovis, human LL-37 had algicidal and immunomodulatory functions.
Introduction
Prototheca species are unicellular achlorophyllous algae, 3–30 μm in diameter, that lack a specific glucosamine cell wall or chloroplasts (Baudelet et al., 2017). Their reproduction is asexual, with endospores being released from sporangia (Jagielski and Lagneau, 2007). Prototheca spp. are widely distributed in the environment, particularly in organic matter with a high moisture content (Scaccabarozzi et al., 2008). Prototheca spp. are also pathogenic and provoke a variety of maladies in animals. In dogs and cats, infection with Prototheca spp. causes either cutaneous lesions or systemic disease with hemorrhagic enteritis and progressive retinal degradation (Huth et al., 2015; Carfora et al., 2017). In humans, Prototheca spp. causes rare chronic skin or articular infections (Seok et al., 2013) or disseminated infections mostly in immunodeficient patients (Tello-Zavala et al., 2013).
Among Prototheca species, P. bovis has been proposed as a major cause of chronic mastitis in cattle. Traditional taxonomic studies on Prototheca species identified two genotypes (GT): P. zopfii GT- I and P. bovis (formerly P. zopfii GT-II) (Roesler et al., 2006). P. bovis was isolated from the milk of cows with mastitis and identified as the causative agent of bovine mastitis (Jagielski et al., 2012, 2019a,b,c,d; Bozzo et al., 2014). Prototheca zopfii GT-I is commonly isolated from the environment and occasionally causes granulomatous lesions in experimentally infected bovine udders (Ito et al., 2011) and protothecosis in humans (Hirose et al., 2018). Clinical signs of protothecal mastitis in cattle can include fever, pain, edema, anorexia, and lethargy, although it is most commonly subclinical, causing decreased milk production (Wawron et al., 2013). Bovine mastitis caused by P. bovis is refractory to most therapeutic agents (Jagielski et al., 2012) and has a very low rate of spontaneous remission (Janosi et al., 2001). Virulence factors, including intracellular heat shock proteins (Hsp70) and proteins that augment peroxisome metabolism to resist harsh environments (e.g., macrophage phagolysosomes) are mostly present in pathogenic P. bovis (Irrgang et al., 2015; Zeng et al., 2019). From an epidemiological perspective, cattle infected with Prototheca spp. are a common source of Prototheca spp. for humans, with immunocompromised farmers at highest risk (Lass-Florl and Mayr, 2007). Since Prototheca spp. may survive chlorination by forming biofilms (Kwiecinski, 2015), and be returned to the environment via sewage effluent and household waste (Wirth et al., 1999), cows with mastitis caused by P. bovis are a risk factor in epidemiology of protothecosis (Chao et al., 2002).
Little is known about innate mammary gland defenses during P. bovis infection. However, cultured mammary epithelial cells from cattle and mice undergo oxidative stress and apoptosis when exposed to P. bovis (Shahid et al., 2016, 2017a,b). Moreover, bovine mammary epithelial cells respond to P. bovis infection with upregulated expression of Toll Like Receptors (TLRs)−2 and−4, pro-inflammatory cytokines (TNF-α, IL-1β, IL-8) and β-defensin-5 (Deng et al., 2016). To further understand innate mammary defenses in protothecosis, we focused on cathelicidins. Cathelicidins are cationic amphipathic peptides with an N-terminal domain containing a signal peptide, a well-conserved central cathelin domain, and a variable C-terminal domain (Schauber et al., 2003). The C- terminal domain is cleaved to produce the peptide with antimicrobial and antifungal activity (Ordonez et al., 2014). In humans, a single cathelicidin gene (cathelicidin antimicrobial peptide, Camp) yields this C-terminal active peptide termed leucine-leucine with 37 amino acid residues (LL-37) (Agerberth et al., 1995). In mice, the functional homologous peptide is cathelicidin-related-antimicrobial-peptide (Cramp) encoded by the gene Camp (Nizet et al., 2001). Cathelicidins are abundantly expressed in mammalian cells, mainly neutrophils and epithelial cells and thus, are present in various organs/tissues, including skin, eyes, mouth, lungs, intestine, and mammary gland (Zanetti, 2005; Cobo et al., 2012, 2017). Cathelicidins have been involved in various host responses against bacterial and parasitic intracellular pathogens through activation of cytokines/chemokine secretion (Boucher et al., 2018; Cirone et al., 2019; Marin et al., 2019). In sheep and murine mammary glands and in human milk, cathelicidins also have antimicrobial and anti-inflammatory effects (Murakami et al., 2005; Addis et al., 2013). Moreover, cathelicidins increased in bovine mammary epithelial cells exposed to a main mastitis pathogen, Staphylococcus aureus (Ibeagha-Awemu et al., 2010). Thus, we hypothesized that cathelicidins produced by the mammary gland contribute to host innate defense against P. bovis infection. Using genetically mutant mice that lack cathelicidin (Camp−/−) and cultured murine macrophages and mammary epithelial cells, we demonstrated that endogenous cathelicidins regulate mammary gland inflammation and macrophage activity against P. bovis, whereas exogenous human cathelicidins (LL-37) downregulated epithelial and macrophage responses and had algicidal activities.
Materials and Methods
Ethics Statement
Animal experiments were conducted in accordance with Canadian Guidelines for Animal Welfare (CGAW) and the University of Calgary Animal Care Committee (Animal Protocol AC16-0061).
Prototheca bovis
A Prototheca spp. was isolated from the milk of a cow with clinical mastitis at the College of Veterinary Medicine, China Agricultural University, Beijing, China (Gao et al., 2012). This Prototheca spp. was characterized as P. bovis by several methods. First, we characterized its cellular fatty acid pattern to confirm that P. bovis had, as expected, more eicosadienoic acid (C20: 2) compared to the reference P. zopfii GT-I (Roesler et al., 2006). Second, we determined 18S rDNA sequences using genotype-specific PCR (Roesler et al., 2006; Moller et al., 2007). For this, P. bovis was purified from a single colony of P. bovis isolated from Sabouraud dextrose agar (Sigma-Aldrich) and amplified in Sabouraud in dextrose broth (Sigma-Aldrich) (1 mL) (DP302, TIANamp DNA, Tiangen). DNA was quantified (NanoDrop ND-1000 Spectrophotometer, Thermo Fisher Scientific) and stored at −20°C. The Prototheca (450 bp) fragment internal amplification control was detected using Proto18-4f (GACATGGCGAGGATTGACAGA) and Proto18-4r (AGCACACCCAATCGGTAGGA) sequences. The P. bovis specific amplicon (165 bp) was detected with primers Proto18-4f (GACATGGCGAGGATTGACAGA) and PZGT-II/r (GTCGGCGGGGCAAAAGC) (Roesler et al., 2006; Moller et al., 2007).
The P. bovis genotype was further confirmed by restriction fragment length polymorphism (RFLP) analysis targeting the cytb gene fragment (599–668 bp) (Jagielski et al., 2018). For this, a PCR mix (25 μL) containing cytb-F1 (5′ GyGTwGAACAyATTATGAGAG-3′) and cytb-R2 (5′-wACCCATAArAArTACCATTCwGG-3′) primers (10 μM each primer), DNA template (1 μL), and 2x EasyTaq PCR supermix (TransGen Biotech, AS111-11; 12.5 μL) was amplified under specific conditions (2 min at 95°C, followed by 35 cycles of 30 s at 95°C, 30 s at 50°C, and 30 s at 72°C, with final extension of 5 min at 72°C). The PCR products depicted a 644 base pair (bp) product compatible with P. zopfii as visualized by agarose gel electrophoresis (1%, wt/vol) and stained with ethidium bromide (Supplementary Figure 1A). The amplified cytb gene products (644-bp) were digested by RsaI and TaiI digesting enzymes (FastDigest Enzymes, Thermo Fisher Scientific). The total mixture (30 μL) containing 10x restriction enzyme buffer (3 μL), PCR product (10 μL), enzymes (1.5 μL each) and PCR water (16.5 μL) was digested by RsaI (5 min at 37°C) followed by TaiI (5 min at 65°C). The restriction products visualized on 4% agarose gels, stained with ethidium bromide, and exposed to UV light showed DNA fragments of 200 and 450 bp after RSaI/TaiI digestion, compatible with P. bovis (Supplementary Figure 1B). Taken together, we confirmed a P. bovis genotype in the isolate clinically recovered from a case of mastitis in cows.
For experimentation, P. bovis was streaked on Sabouraud dextrose agar (S3181, Sigma-Aldrich) (37°C for up to 48 h) to produce typical single creamy-white, yeast-like colonies and replicated in Sabouraud dextrose broth (S3306, Sigma-Aldrich) (37°C for up to 72 h) (Gao et al., 2012).
Experimental Induction of Murine Mastitis by Prototheca bovis
C57BL/6 (6–8 wk. old) lactating female wild-type Camp+/+ and cathelicidin-null Camp−/− C57BL/6 mice (B6.129X1-Camptm1Rlg/J; The Jackson Laboratory) were housed in a specific pathogen-free environment with ad libitum access to feed and water (University of Calgary). In these Camp−/− mice, there is deletion of exons 3 and 4 of the cathelicidin gene Camp; whereas some gene portions could be detected, they do not produce functional cathelicidin peptides (Nizet et al., 2001). Camp−/− and Camp+/+ mice were infected intramammary (10–14 days after parturition) with either P. bovis (50 μL containing 1 × 105 colony forming units (CFU)/mL) or an equal volume of phosphate buffered saline (PBS) (control) in the left 4th and right 4th mammary glands (L4 and R4) (n: 4 per group). Mice were euthanized by carbon dioxide followed by cervical dislocation at 4 days post-infection when they had indications of clinical mastitis. This termination point was chosen based on preliminary studies where mice become lethargic and before humane end points associated with longer intervals.
Immediately after euthanasia, all mammary glands were excised, weighed, and collected as follows: A portion was incubated into Trizol (Invitrogen) for gene quantification and another fixed in formalin (10%) solution, embedded in paraffin wax, sectioning (5 μm) and stained with hematoxylin and eosin (H&E) or toluidine blue for histological examination (Seok et al., 2013). Prototheca organisms were identified by specific staining, using periodic acid-Schiff (PAS) and Grocott methenamine silver (GMS).
Identification of Macrophages and Neutrophils in Murine Mammary Glands
Fixed mammary gland sections were deparaffinized and dehydrated, permeabilized with PBS/Triton X-100 (0.25%, v/v) (PBS-T) buffer with donkey serum (1%; 017-000-121, Jackson ImmunoResearch) [room temperature (RT), 10 min]. Slides were blocked with PBS-T containing donkey serum [10% (v/v)] and bovine serum albumin (BSA) (1% (v/v); CA97061-416, Sigma-Aldrich) (RT, 2 h). After washing with PBS, sections were incubated with primary antibodies against murine F4/80 (macrophages) (4316835, BD Pharmingen) and Ly-6G (neutrophils) antigens (127609, Biolegend) (1:1,000 in PBS-T plus 1% BSA) for 16 h at 4°C. Following washing with PBS-T, slides were incubated with secondary antibodies (Alexa Fluor® 488-conjugated affinipure goat anti-rat IgG, 135205, Jackson Immune Research) (1:1,000 in PBS-T plus 1% BSA) (RT, 1 h), washed again with PBS-T and incubated with DAPI (4′, 6-diamidino-2- phenylindole) (Invitrogen) (RT, 20 min). Slides were examined with an immunofluorescence microscope (Axio Imager M2, Zeiss).
Determination of Prototheca bovis in Infected Mammary Tissue
Prototheca infection in mammary tissues was assessed by histological counting of Prototheca sporangia and Prototheca culture. For counting, sporongia of Prototheca spp. (7–30 μM in diameter) were counted in 10 images of the mammary gland per mouse, individually and randomly captured at 40x magnifications. For culturing, the entire mammary gland was homogenized in sterile tubes containing 1 mL of 0.025% Triton X-100 in PBS. 10-fold homogenate dilutions were spread onto duplicate plates of Sabouraud dextrose agar (Sigma-Aldrich). Number of colonies, typically with a granular serrated shape, grayish white and with a central protrusion, was determined after 2–3 days of incubation (Gogoi-Tiwari et al., 2017).
P. bovis Infection in Cultured Murine Bone Marrow-Derived Macrophages and Immortalized Mammary Epithelial Cells and Macrophages
Bone marrow-derived macrophages (BMDMs) were collected from femurs of Camp−/− and Camp+/+ mice and plated into RPMI containing 10% fetal bovine serum (Hyclone), macrophage colony stimulating factor (M-CSF, Peprotech) or granulocyte-macrophage colony-stimulating factor (GM-CSF, Peprotech) (10 ng/mL) and antibiotic mixture (penicillin-streptomycin, 1%, P4333, Sigma-Aldrich). BMDMs were cultivated for 7–10 days in 12 well plates and challenged with P. bovis (1 × 105 CFU/mL re-suspended in RPMI) (37°C with 5% CO2) for up to 8 h. Challenge doses of P. bovis were chosen based on previous in vitro experiments with bovine mammary epithelial cells infected with P. bovis (Shahid et al., 2017b) and a murine model of P. bovis mastitis (Shahid, personal communication).
Mammary epithelial and macrophage responses, relevant during mastitis (Lam et al., 2009; Xiao et al., 2015), were modeled using the murine mammary epithelial cell line (HC11), a prolactin responsive clone COMMA-1D derived from mammary tissue of BALB/c mice in mis-pregnancy (Katz and Streuli, 2007); and a murine phagocytic monocyte J774A.1 (ATCC® TIB-67™) derived from BALB/c mice, conventionally used as a macrophage model. To assess the role of exogenous cathelicidin in protothecosis, murine macrophages and mammary epithelial cells were challenged with P. bovis (1 × 105 CFU/mL resuspended in DMEM/F12) ± synthetic human cathelicidin LL-37 amide (H-Leu-Leu-Gly-Asp-Phe-Phe-Arg-Lys-Ser-Lys-Glu-Lys-Ile-Gly-Lys-Glu-Phe-Lys-Arg-Ile-Val-Gln-Arg-Ile-Lys-Asp-Phe-Leu-Arg-Asn-Leu-Val-Pro-Arg-Thr-Glu-Ser-NH2 trifluoroacetate salt) (10 μg/mL; H-6224; Bachem) for up to 8 h (37°C with 5% CO2).
Transcriptional Expression of TNF-α, IL-1β and Cxcl-1, and Secretion of Cxcl-1 and TNF-α in Mammary Tissues and Cultured Cells
Gene mRNA transcription was quantified by quantitative real-time polymerase chain reaction (RT qPCR). Total RNA from murine mammary glands, BMDMs, mammary epithelial cells and macrophages was extracted with Trizol reagent (Invitrogen) and converted into cDNA by reverse transcription (101414-098, VWR). The RNA and cDNA quality was evaluated by the A260/A280 absorbance ratio (NanoVue Spectrophotometer, GE Healthcare Bio-Sciences) (Bustin et al., 2009). The pre-designed primers (RT2 qPCR Primer Assay, Qiagen) were specific for murine tumor necrosis factor alpha (TNF-α) (PPM03113G), interleukin 1 beta (IL-1β) (PPM03109F), C-X-C motif chemokine ligand 1 (Cxcl-1) (PPM03058C), cathelicidin (Camp) (PPM25023A), defensin-4 (PPM29171A) and housekeeping gene glyceraldehyde-3-phosphate dehydrogenase (GAPDH) (forward 5′ AAATGGTGAAGGTCGGTGTG and reverse 3′ TGAAGGGGTCGTTGATGG). All primers were verified for specificity and efficiency (>95%) to ensure amplification of a single product of the correct size, as indicated in MIQE guidelines (Bustin et al., 2009). The total reaction mixture (10 μL) included 2 μL of cDNA, 0.5 μM of forward and reverse primers and 1x SsoAdvanced Universal SYBR Green Supermix (BioRad) (CFX-96 real-time PCR system). All reactions were performed in triplicate. Target gene mRNA values were corrected relative to the normaliser, GAPDH. Data were analyzed using the 2−ΔΔCT method and reported as mean fold change of target transcript levels in challenged vs. uninfected control groups.
Secreted Cxcl-1 and TNF-α Protein Quantification
TNF-α and Cxcl-1 proteins produced and secreted by the mammary gland were quantified by respective ELISAs (DY453-05 and DY410-05, R&D Systems) in supernatants collected from the whole murine mammary gland homogenized with 1 mL of 0.025% Triton X-100 in PBS and centrifuged (20,000 g; 10 min).
Direct Algicidal Activity of Cathelicidin Against P. bovis
For killing assays, P. bovis grown into Sabouraud in dextrose broth (Sigma-Aldrich) (37°C, 2 days on a rotary shaker) was poured into 96 wells plates (1 × 105 CFU/mL in logarithmic growth phase) and simultaneously incubated with LL-37 peptides (H-6224; Bachem) (1 and 2 μM diluted in distilled water) (37°C, up to 1 day). Final Prototheca spp. concentration was determined by CFU/mL and plotted after conversion to logarithm (log10) using a standard curve. P. bovis and media only were used as controls.
Statistical Analyses
Normality was assessed using D'Agostino & Pearson omnibus normality or Shapiro-Wilk (Royston) tests. Analytical data represented as histograms were recorded as mean values with bars representing standard errors of the mean (SEM) from a minimum of two independent experiments, with data obtained in triplicate, unless otherwise stated. All statistical comparisons were performed using one-way analysis of variance (ANOVA) with a post hoc Bonferroni correction for multiple group comparisons (Graph Pad Prism, 5.0). A p- value was assigned to each experimental group with reference to a control group. A p-value of <0.05 was considered significant.
Results
P. bovis-Challenged Camp−/− Mice Developed More Acute Mastitis With Predominantly Histiocytic Infiltration Compared With Camp+/+ Mice
Effects of endogenous cathelicidins in mastitis were studied in lactating Camp+/+ and Camp−/− mice intramammary infected with P. bovis isolated from a bovine clinical mastitis case. Both Camp+/+ and Camp−/− infected mice developed clinical mastitis (painful, red, and edematous mammary glands) 4 days post-infection, with onset of depression and lethargy. Microscopically, uninfected control mice had normal mammary gland architecture whereas both Camp+/+ and Camp−/− infected mice developed severe acute mastitis (Figure 1A). Mammary epithelial lobules, interstitial fibrovascular tissue and interlobular adipose tissue in both Camp+/+ and Camp−/− infected mice had infiltration of a mixed population of inflammatory cells, including lymphocytes, plasma cells, neutrophils, macrophages (Figures 1A,B) and rare mast cells (Figure 1C). More macrophages infiltrated the mammary in P. bovis infected Camp−/− mice (p < 0.05, Figure 1D). Neutrophils were numerous in P. bovis infected mice, but there was no difference between Camp−/− and Camp+/+ mice (p > 0.05, Figure 1E).
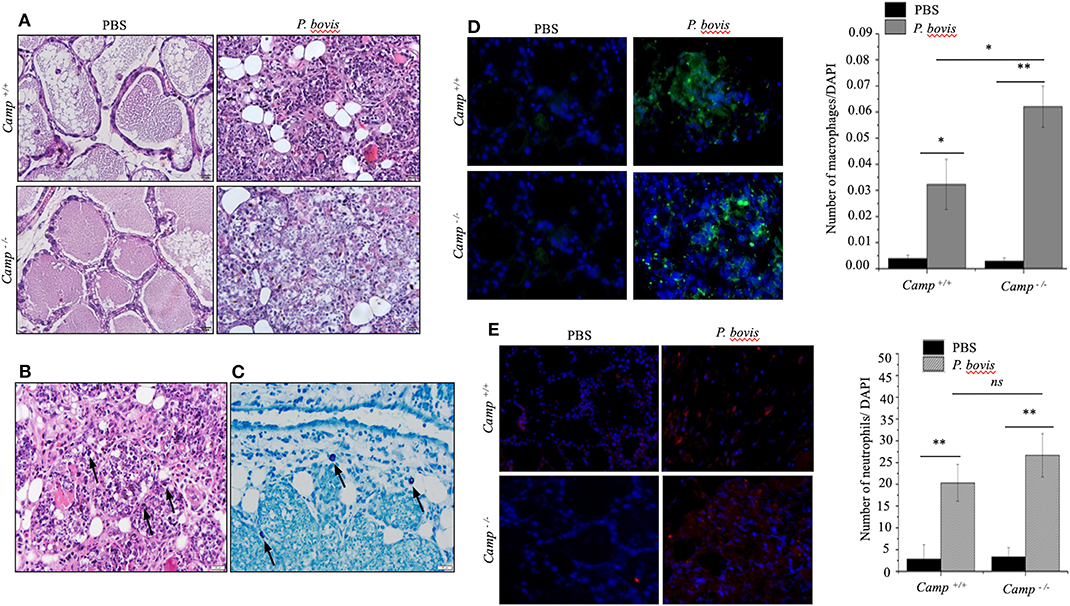
Figure 1. Prototheca bovis induced acute mastitis with higher leukocyte infiltration in Camp−/− mice. (A,B) Camp+/+ and Camp−/− mice were challenged intramammary with P. bovis or PBS (control). Mammary glands obtained 4 d post-infection were stained with hematoxylin and eosin. (B) Mammary gland from P. bovis infected Camp+/+ mouse. Note mammary epithelial lobules, interstitial fibrovascular tissue and interlobular adipose tissue infiltrated by a mixed population of inflammatory cells that includes lymphocytes, plasma cells, neutrophils, macrophages, and rare mast cells. Prototheca organisms (black arrows) are oval, approximately 10 μm in length and surrounded by a clear halo. Scale bar = 20 μm. (C) Mammary gland of an infected Camp+/+ mouse stained by toluidine blue for identification of rare mast cells (black arrows) in addition to the numerous mixed inflammatory cells shown in (B). Mast cell cytoplasmic granules highlighted by toluidine blue stain. Scale bar = 20 μm. (D,E) Mammary gland from P. bovis infected Camp+/+ and Camp−/− mice immunostained with anti-macrophage (F4/80) and anti-neutrophil (Ly6G) antibodies. Histograms represent the average number of cells per field (a minimum of 10 randomly chosen microscopic fields, x40 objective magnification). Data are shown as means ± SEM (n = 4/group). *p < 0.05, **p < 0.01 (one-way ANOVA post hoc Bonferroni correction for multiple group comparison) was considered significant.
Mammary infection with P. bovis was confirmed microscopically in Camp+/+ and Camp−/− mice 4 days post-infection. Using PAS and GMS staining, round to oval sporangia with internal divisions compatible with Prototheca spp. were visible both free within alveolar lumen and throughout the mammary gland interstitium (Figure 2A). The grade of infection with P. bovis did not differ between Camp+/+ and Camp−/− mice at 4 days post-infection, as determined by histological counting of sporangia and Prototheca spp. culture (Figure 2B).
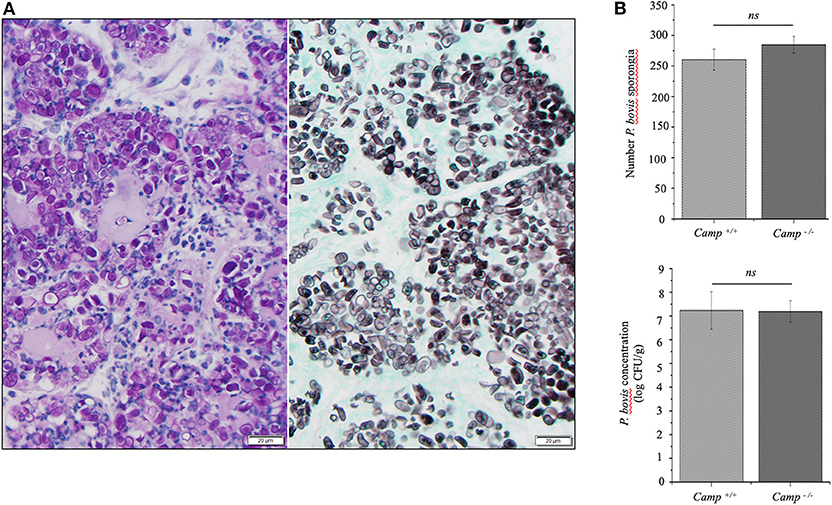
Figure 2. Prototheca bovis was present at similar levels in the mammary glands of Camp+/+ and Camp−/− mice. (A) Mammary gland from a Camp+/+ mouse infected with P. bovis (4 d post-infection). Abundance of Prototheca organisms within tissue sections was revealed with special stains. Prototheca are stained magenta by periodic acid-Schiff (PAS) stain (left image) and black by Grocott methenamine silver (GMS) stain (right image). Scale bar = 20 μm. (B) P. bovis was present in mammary glands at similar levels in Camp+/+ and Camp−/− mice, as determined by counting of sporangia per field (minimum of 10 randomly chosen microscopic fields, x40 objective magnification) and culturing 10-fold diluted homogenates on Sabouraud dextrose agar. Data are means ± SEM (n = 4/group). Ns, not significant.
The general process of mastitis is characterized by upregulated synthesis of certain pro-inflammatory cytokines, such as TNF-α and IL-1β (Bannerman et al., 2004). P. bovis induced mRNA synthesis and protein secretion of TNF-α in mammary glands from both Camp+/+ and Camp−/− mice infected with P. bovis, with the highest concentration in Camp+/+ mice (p < 0.05, Figures 3A,B).
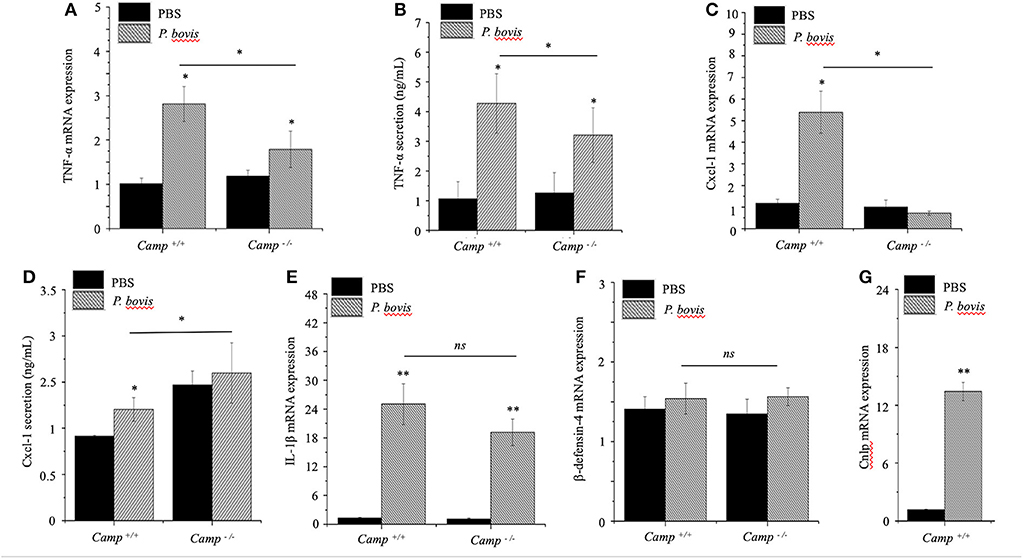
Figure 3. Lesser synthesis of pro-inflammatory cytokines in Camp−/− mice infected with Prototheca bovis. Camp+/+ and Camp−/− were intramammary challenged with P. bovis or PBS (control). Transcriptomic expression and protein secretion of pro-inflammatory genes in the mammary gland including (A,B) TNF-α, (C,D) Cxcl-1, (E) IL-1β, (F) β-defensin-4, and (G) Camp were determined at 4 d post-infection. mRNA synthesis was quantified using RT qPCR and protein secretion was determined in cell supernatant by ELISA. Data are means ± SEM (n = 4/group). *p < 0.05, **p < 0.01 (one-way ANOVA post hoc Bonferroni correction) was considered significant.
Cxcl-1 chemoattracts leukocytes to sites of infection (De Filippo et al., 2013); recruitment of neutrophils and monocytes/macrophages from blood to milk compartments is a critical defense mechanism in bovine mastitis (Zhang et al., 2012). In our study, Cxcl-1 mRNA was upregulated in P. bovis infected mammary glands of Camp+/+ relative to Camp−/− mice (p < 0.05, Figure 3C). Secretion of Cxcl-1 increased in mammary glands from both Camp+/+ and Camp−/− infected mice relative to non-infected controls but, the highest level was in Camp−/− infected mammary glands (p < 0.05, Figure 3D). Transcriptional IL-1β gene levels were increased after P. bovis challenge, but not different between Camp+/+ and Camp−/− infected mammary glands (p > 0.05, Figure 3E). Transcriptomic gene expression of host defense peptides in mammary tissue revealed that β-defensin-4 was not increased after P. bovis infection in mammary glands of Camp−/− and Camp+/+ mice (p > 0.05, Figure 3F), whereas Camp mRNA was increased in infected mammary glands of Camp+/+ mice compared to uninfected Camp+/+ mice (p < 0.05, Figure 3G). No Camp gene expression was detected in Camp−/− mice (data not shown). Taken together, P. bovis provoked acute mastitis in experimentally challenged mice. Furthermore, endogenous production of cathelicidins, induced by the algae, was associated with increased synthesis of pro-inflammatory TNF-α and Cxcl-1.
Bone Marrow-Derived Macrophages Deficient in Cathelicidin (Camp−/−) Had Impaired Pro-inflammatory Cytokine Response to P. bovis Infection
Macrophages prevailed in mastitis induced by P. bovis in mice (Figure 1D). Furthermore, when exposed to inflammatory stimuli (e.g., Prototheca spp), macrophages secrete a vast array of pro-inflammatory cytokines (Arango Duque and Descoteaux, 2014). To determine if cathelicidins modulate macrophage function in protothecal mastitis, BMDMs from Camp+/+ and Camp−/− mice were challenged with P. bovis. P. bovis induced early (2 h) TNF-α and IL-1β transcription in BMDMs, with higher levels in Camp+/+ BMDMs (p < 0.05) (Figures 4A,B). Such TNF-α and IL-1β overexpression lasted for at least 8 h post-infection (Figures 4A,B). Prototheca bovis also induced early (2 h) increased Cxcl-1 mRNA expression in Camp+/+ BMDMs compared to Camp−/− BMDMs (p < 0.05, Figure 4C). Prototheca bovis did not induce β-defensin-4 mRNA (p > 0.05, Figure 4D) whereas P. bovis increased Camp gene expression in Camp+/+ BMDMs with an expected non-expression in Camp−/− BMDMs (p < 0.05, Figure 4E). Thus, endogenous cathelicidin contributed to production of pro-inflammatory cytokines in macrophages exposed to P. bovis.
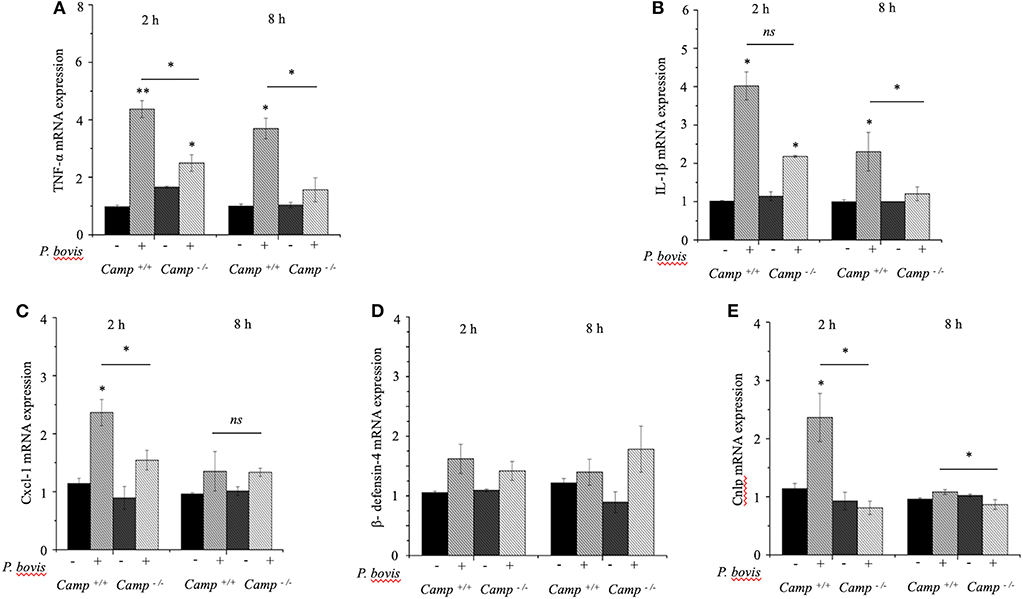
Figure 4. Reduced production of pro-inflammatory cytokines in macrophages from Camp−/− exposed to Prototheca bovis. (A) Primary bone marrow-derived macrophages were isolated from Camp+/+ and Camp−/− mice and challenged with P. bovis. (A,B) Transcriptomic expression of (A) TNF-α, (B) IL-1β, (C) Cxcl-1, (D) β-defensin-4, and (E) Camp was determined at 2 and 8 h post-infection. mRNA synthesis was quantified using RT qPCR. Data are means ± SEM (n = 3 independent experiments done in triplicate). *p < 0.05, **p < 0.01 (one-way ANOVA post hoc Bonferroni correction) was considered significant. Ns, not significant.
Human Cathelicidin LL-37 Inhibited Growth of P. bovis and Decreased Pro-inflammatory Responses in Infected Macrophages and Mammary Epithelial Cells
To mechanistically explore the role of cathelicidin in P. bovis mastitis, we studied whether exogenous cathelicidin exert algicidal or immunomodulatory effects in key cellular components in mastitis: macrophages and mammary epithelium. In regard to direct killing, synthetic human cathelicidin LL-37 (1 and 2 μM) decreased in vitro growth of P. bovis up to 8 h, with some inhibitory activity still present at 24 h (p < 0.05, Figure 5). In reference to the immunomodulatory role of LL-37, P. bovis upregulated mRNA expression of TNF-α, Cxcl-1, and IL-1β in murine phagocytic J774.A1 cells (at 2 and 8 h post-infection), whereas co-stimulation with LL-37 decreased concentrations of TNF-α, Cxcl-1, and IL-1β mRNA expression (p < 0.05, Figures 6A–C). Next, the immunoregulatory role of LL-37 was assessed in murine mammary epithelial cells (HC11) capable of producing milk casein protein. Challenge of HC11 cells with P. bovis induced early (2 h) TNF-α, Cxcl-1 and IL-1β gene expression (p < 0.05, Figures 6D–F), whereas co-stimulation with LL-37 lowered these mRNA TNF-α, Cxcl-1, and IL-1β responses. Addition of LL-37 to the mammary epithelium stimulated transcriptional expression of IL-1β and TNF-α at a later point (8 h post-infection) (p < 0.05, Figures 6D–F). Thus, human cathelicidin LL-37 reduced synthesis of pro-inflammatory cytokines in murine macrophages and mammary epithelium, whereas it had some direct killing effects on P. bovis.
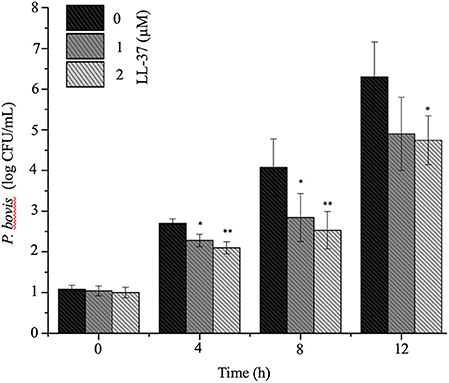
Figure 5. Synthetic human cathelicidin LL-37 inhibited in vitro Prototheca bovis. Prototheca bovis (1 × 105 CFU/mL in logarithmic growth phase) was incubated with synthetic human cathelicidin LL-37 peptides (up to 2 μM for up 12 h at 37°C) into Sabouraud dextrose broth and algae concentration were determined using a standard curve, with only P. bovis and expressed as log CFU/mL. Histogram of remaining P. bovis after peptide treatment. Data are shown as means ± SEM (n = 3 independent experiments done in triplicate). *p < 0.05, **p < 0.01 (one-way ANOVA post hoc Bonferroni correction) was considered significant.
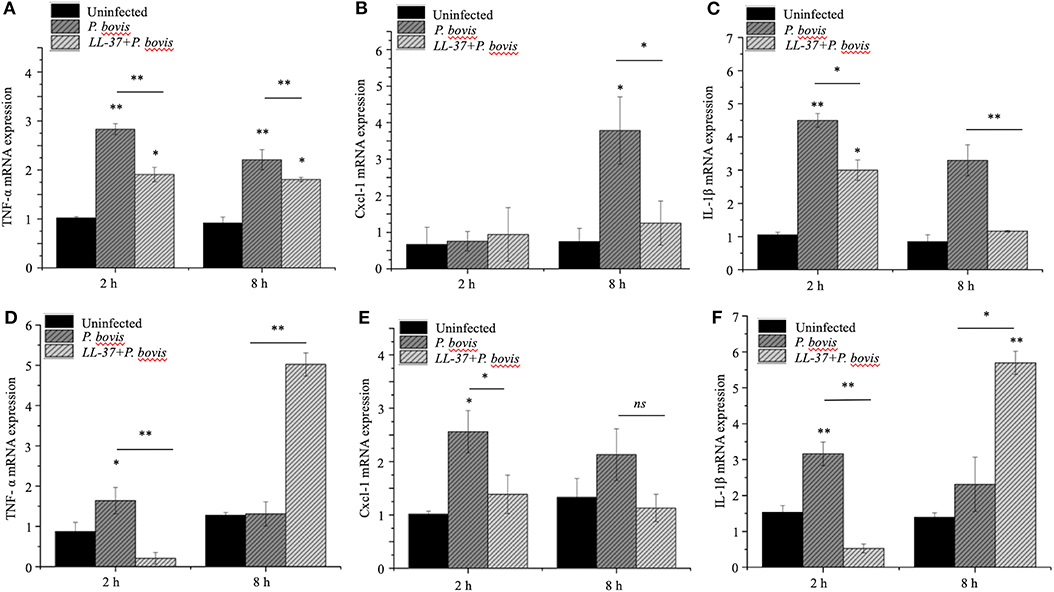
Figure 6. Synthetic human cathelicidin LL-37 mitigated production of pro-inflammatory cytokines in murine macrophages and mammary epithelia infected with Prototheca bovis. Murine phagocytic (J774.A1) macrophages (A–C) and mammary epithelial (HC-11) cells (D–F) were challenged with P. bovis (1 × 105 cfu/mL) ± synthetic human cathelicidin LL-37 (2 μM) (37°C with 5% CO2). Transcriptomic expression of (A,D) TNF-α, (B,E) Cxcl-1 and (C,F) IL-1β were determined at 2 and 8 h post-infection. mRNA synthesis was quantified using RT qPCR. Data are shown as means ± SEM (n = 3 independent experiments done in triplicate). *p < 0.05, **p < 0.01 (one-way ANOVA post hoc Bonferroni correction) was considered significant. Ns, not significant.
Discussion
Although P. bovis is a major cause of insidious and chronic (>30 days) mastitis in cattle (Moller et al., 2007; Wawron et al., 2013; Bozzo et al., 2014; Jagielski et al., 2019b), the udder innate immune response to pathogenic Prototheca spp. remains poorly understood. In this study, P. bovis triggered severe acute mastitis in mice and naturally occurring cathelicidins aided in establishing local inflammation by promoting synthesis of pro-inflammatory cytokines. Two main lineages of Prototheca spp. have been relevant in public health: a dominant species typically associated with dairy cattle, namely P. ciferrii (formerly P. zopfii GT-I), P. blaschkeae, and P. bovis (formerly P. zopfii GT-II) and others human-associated Prototheca spp. (i.e., P. wickerhamii, P. cutis, P. miyajii) (Jagielski et al., 2019a). In this study, we used a Prototheca spp. identified as P. bovis following a taxonomic approach commonly accepted for Prototheca (Roesler et al., 2006) and a cytb-based genotyping used for unambiguous Prototheca spp. identification (Jagielski et al., 2018) based on the protothecal phylogeny (Jagielski et al., 2019a).
Although Camp−/− mice had increased infiltration of macrophages in infected mammary glands, they also produced less local expression and secretion of TNF-α and Cxcl-1. Moreover, BMDMs isolated from Camp−/− mice had lower in vitro synthesis of pro-inflammatory TNF-α, IL-1β, and Cxcl-1 in response to P. bovis. That Cxcl-1 secretion in infected Camp−/− mice ended up higher than in wild type mice denoted a lack of association between Cxcl-1 mRNA and protein kinetics. Such differences in cytokine mRNA/protein expressions are usually due to post-transcriptional mechanisms (Greenbaum et al., 2003) or perhaps, Cxcl-1 gene transcription is augmented only at early points by cathelicidins or the Cxcl-1 protein half-life is longer compared with mRNA. Alternatively, increased Cxcl-1 in infected Camp−/− mice may correspond to an overwhelming and generalized inflammation, higher than any cathelicidin effect in the wild type. Overall, the observed role of endogenous cathelicidin promoting Cxcl-1 in protothecal mastitis could be critical in attracting leukocytes early to sites of infection and retain macrophages into the udder during the innate immune response against pathogenic algae. In agreement, murine cathelicidin Cramp attracted in vitro T-cells, macrophages, neutrophils, eosinophils and mast cells via an FPRL-1 receptor (Yang et al., 2000; Kurosaka et al., 2005; Tjabringa et al., 2006) whereas LL-37 chemoattracted leukocytes via G-protein coupled receptors (Vandamme et al., 2012). Cathelicidins could also chemoattract neutrophils indirectly, by inducing chemokines. In this regard, cathelicidins induced CXCL8 transcription alone and in synergy with TNFα though Src family kinase pathways downstream of P2X7R in keratinocytes (Nijnik et al., 2012; Chen et al., 2013) and gingival fibroblasts (Montreekachon et al., 2011) and in an EGFR-dependent manner in skin (Wu et al., 2010) and airway epithelial cells (Tjabringa et al., 2006). Whereas, the influx of leukocytes in the mammary gland during P. bovis infection could be functionally regulated by cathelicidin, other cathelicidin-independent chemoattractive effects cannot be disregarded. For instance, cell-surface glycoproteins involved in cell-cell interaction, such as CD44, which mediates specific adhesion of bovine blood polymorphonuclears to mammary epithelial cells (Gonen et al., 2008), could initiate recruitment of inflammatory cells during mastitis.
Our study demonstrated that a cathelicidin can exert an immunosuppressive effect in another species; in particular, human LL-37 mitigated production of pro-inflammatory TNF-α, IL-1β, and Cxcl-1 in murine macrophages and mammary epithelial cells challenged with P. bovis. This immunomodulatory role of human cathelicidin LL-37 was not surprising, as LL-37 reduced secretion of IL-1β, IL-6, IL-8, and TNF-α in human and murine neutrophils exposed to heat-inactivated Pseudomonas aeruginosa or Staphylococcus aureus (Alalwani et al., 2010). Likewise, human or murine macrophage-like cells had reduced expression of TNF-α and nitric oxide (NO) in the presence of LL-37 when stimulated with bacterial lipooligosaccharide or LPS (Scott et al., 2002; Zughaier et al., 2005). Mechanistically, LL-37 could directly bind LPS in macrophages, thereby suppressing IL-6, IL-1β, and TNF-α synthesis (Nagaoka et al., 2001; Tomasinsig et al., 2010).
In terms of algicidal effects, there was no differences between Camp+/+ and Camp−/− infected mice in P. bovis burden in mammary glands at an early point of infection (4 days). In contrast, human cathelicidin LL-37 in vitro directly reduced number of P. bovis in a dose-dependent manner. These killing properties of cathelicidins, LL-37 in this case, are related with the cationic peptide capacity to bind and disrupt negatively charged microbial membranes, leading to cell death due to destabilization of plasma membranes and efflux of ATP and proteins (Tsai et al., 2014). Cathelicidins can also cross membranes and disrupt intracellular processes, including RNA and DNA synthesis and protein degradation (Mansour et al., 2014). Although the role of cathelicidins in fungal/algal infectious is not fully explored, there is increasing evidence of protective effects. Human LL-37 has anti-fungus and algae activity against Candida albicans, Malassezia furfur, Trichophyton rubrum and Trichophyton. mentagrophytes (Lopez-Garcia et al., 2006; Tomasinsig et al., 2012; Tsai et al., 2014). Cattle have a vast repertoire of cathelicidins, with at least 8 naturally occurring (Young-Speirs et al., 2018) and direct killing effects on P. bovis observed in cathelicidins from cattle origin. Bovine myeloid antimicrobial peptide (BMAP)-28 displayed quick anti-Prototheca activity (<1 h), with extensive surface blebbing and release of intracellular material due to cell permeabilization (Tomasinsig et al., 2012) whereas other bovine host defense peptides (bactericin 5 and lingual anticrobial peptide; LAP) also had anti Prototheca activity, albeit through non-lytic mechanisms (Tomasinsig et al., 2012). Conversely, a different susceptibility of P. bovis to cathelicidins of diverse origins agrees with studies in bacteria. Human LL-37 and murine Cramp had dissimilar minimum inhibitory concentrations (MICs) against Citrobacter rodentium, Pseudomonas aeruginosa, Streptococcus pyogenes, Staphylococcus aureus, Escherichia coli, Salmonella spp. and Helicobacter pylori (Iimura et al., 2005; Coorens et al., 2017; Marin et al., 2019). In addition, endogenous Cramp had a pro-inflammatory role in murine mastitis induced by P. bovis, whereas in contrast, LL-37 reduced synthesis of pro-inflammatory cytokines in macrophages and mammary epithelia. Although human LL-37 and murine Cramp are considered homologous based on broadly similar and interspecies functions (antimicrobial and immunomodulatory) (Barlow et al., 2011) and structural properties (α-helical and net charge of +6), they actually share <70% sequence identity in their active peptide (Nizet et al., 2001; Tomasinsig and Zanetti, 2005). This may explain singular activities for each peptide and indeed, human LL-37 binded dsRNA and activated TLR3 signaling in early endosomes but murine Cramp did not (Singh et al., 2013). Likewise, LL-37 was more effective in reducing S. aureus proliferation in vitro when compared to Cramp (Coorens et al., 2017). In our study, murine cathelicidin Cramp may not have killing properties against P. bovis or perhaps acts later on the pathogen burden, when immune defenses are developed and more Cramp is available. Alternatively, Cramp may have indirect antimicrobial effects, promoting neutrophil killing by increasing production of reactive oxygen species (ROS) and enhancing phagocytic activity (Alalwani et al., 2010). In all cases, cathelicidin LL 37 appeared to be a good model of cathelicidins and potential target molecule for developing therapeutics, although comparative studies with various cathelicidins and their derivatives may offer distinctive immunomodulatory advantages.
Mast cells in mammary glands infected with P. bovis were an unexpected finding. Mast cells are immune effectors that degranulate pro-inflammatory granules rich in histamine and heparin upon activation. LL-37 stimulated degranulation of mast cells (Niyonsaba et al., 2010) through MAP kinases p38 and ERK phosphorylation, increasing vascular permeability in skin (Chen et al., 2006). Thus, such release of histamine, proteoglycans, serotonin and serine proteases from degranulated mast cells, likely regulated by cathelicidin, may be important in pathogenesis of P. bovis and other mastitis pathogens (e.g., Staphyloccocus aureus, Pseudomonas) by augmenting inflammation (e.g., increasing permeability of capillaries to permit leukocyte extravasation).
In summary, pathogenesis of P. bovis, a globally ubiquitous and environmental (grass, water, trees) algae (Rösler et al., 2003) capable of infecting various vertebrate hosts (Seok et al., 2013; Capra et al., 2014; Carfora et al., 2017) remains poorly understood. Therapeutic control for human and animal protothecosis is on demand. Whereas, a human cathelicidin (LL-37) could kill P. bovis, endogenous cathelicidin (Cramp) contributed to the inflammatory response during P. bovis-induced mastitis, likely recruiting early leukocytes, thereby aiding pathogen control. Whereas, we focused on early steps in protothecal mastitis, cathelicidins could have other effects through later stages of inflammation due to known interactions with many cell types, including endothelial cells (Koczulla et al., 2003), epithelial cells (Tjabringa et al., 2003), mast cells (Niyonsaba et al., 2001) and macrophages (Scott et al., 2002). Cathelicidins were pleitrophic, either preventing (Kirikae et al., 1998) or augmenting inflammatory reactions, e.g., skin with rosacea (Yamasaki et al., 2007). Therefore, this study represented a proof-of-concept regarding the role of innate immune responses contributed by cathelicidins in protothecal mastitis; therefore, we propose LL-37 has potential as a therapeutic compound for controlling pathogenic algae. More studies are needed to decipher the kinetics of myeloid and non-myeloid cells and cytokine milieu that regulate specific functions of cathelicidins in mastitis.
Data Availability Statement
The datasets generated for this study are available on request to the corresponding author.
Ethics Statement
The animal study was reviewed and approved by Animal experiments were conducted in accordance with Canadian Guidelines for Animal Welfare (CGAW) and the University of Calgary Animal Care Committee.
Author Contributions
MS, PC, and JG performed the in vitro and in vivo experiments. CK conducted the histopathologic analysis. MS and JG performed the analysis of data and prepared the figures. MS, BH, HB, and EC conceived this research, designed the experiments, and wrote the manuscript. All authors reviewed the manuscript.
Funding
This study was financially supported by the National Natural Science Foundation of China (31572587, 31772813, and 31850410474), the High-end Foreign Experts Recruitment Program (GDT20171100013), the National Dairy Industry and Technology System (CARS-37-02A) from HB and; the Natural Sciences and Engineering Research Council (NSERC) Discovery Grant (RGPAS-2017- 507827), the Eyes High International Collaborative Grant for Young Researchers (10014539) (University of Calgary) and Dairy Research Cluster 3 (1049122) from EC.
Conflict of Interest
The authors declare that the research was conducted in the absence of any commercial or financial relationships that could be construed as a potential conflict of interest.
Acknowledgments
We thank Dr. John Kastelic for editing the manuscript.
Supplementary Material
The Supplementary Material for this article can be found online at: https://www.frontiersin.org/articles/10.3389/fcimb.2020.00031/full#supplementary-material
Supplementary Figure 1. Cytochrome b (cytb) PCR and PCR-RFLP for identification of the Prototheca bovis. The Prototheca spp. isolate recovered from the milk of a cow with clinical mastitis and used in our murine mastitis model was genotyped by cytb PCR and PCR-RFLP in comparison with a P. ciferri reference strain isolated from the enviroment. (A) Cytb gene PCR results depicting a 644 bp PCR product for P. ciferri strain (Lane 1) and the P. bovis isolated from the milk of a cow with clinical mastitis and used in our murine model of mastitis (Lane 2). DNA ladder: molecular-weight size marker. (B) Cytb PCR-RFLP results showing the P. ciferri given 300 and 350 bp DNA fragments (from the 644 bp PCR product) after TaiI digestion (Lane 1) and the P. bovis isolated from the milk of a cow with clinical mastitis given DNA fragments of 200 and 450 bp (from the 644 bp PCR product) after digestion with TaiI, compatible with P. bovis (Lane 2). DNA ladder: molecular-weight size marker.
References
Addis, M. F., Pisanu, S., Marogna, G., Cubeddu, T., Pagnozzi, D., Cacciotto, C., et al. (2013). Production and release of antimicrobial and immune defense proteins by mammary epithelial cells following Streptococcus uberis infection of sheep. Infect. Immun. 81, 3182–3197. doi: 10.1128/IAI.00291-13
Agerberth, B., Gunne, H., Odeberg, J., Kogner, P., Boman, H. G., and Gudmundsson, G. H. (1995). FALL-39, a putative human peptide antibiotic, is cysteine-free and expressed in bone marrow and testis. Proc. Natl. Acad. Sci. U.S.A. 92, 195–199. doi: 10.1073/pnas.92.1.195
Alalwani, S. M., Sierigk, J., Herr, C., Pinkenburg, O., Gallo, R., Vogelmeier, C., et al. (2010). The antimicrobial peptide LL-37 modulates the inflammatory and host defense response of human neutrophils. Eur. J. Immunol. 40, 1118–1126. doi: 10.1002/eji.200939275
Arango Duque, G., and Descoteaux, A. (2014). Macrophage cytokines: involvement in immunity and infectious diseases. Front. Immunol. 5, 491. doi: 10.3389/fimmu.2014.00491
Bannerman, D. D., Paape, M. J., Lee, J. W., Zhao, X., Hope, J. C., and Rainard, P. (2004). Escherichia coli and Staphylococcus aureus elicit differential innate immune responses following intramammary infection. Clin. Diagn. Lab. Immunol. 11, 463–472. doi: 10.1128/CDLI.11.3.463-472.2004
Barlow, P. G., Svoboda, P., Mackellar, A., Nash, A. A., York, I. A., Pohl, J., et al. (2011). Antiviral activity and increased host defense against influenza infection elicited by the human cathelicidin LL-37. PLoS ONE 6:e25333. doi: 10.1371/journal.pone.0025333
Baudelet, P. H., Ricochon, G., Linder, M., and Muniglia, L. (2017). A new insight into cell walls of Chlorophyta. Algal Res. 25, 333–371. doi: 10.1016/j.algal.2017.04.008
Boucher, E., Marin, M., Holani, R., Young-Speirs, M., Moore, D. M., and Cobo, E. R. (2018). Characteristic pro-inflammatory cytokines and host defence cathelicidin peptide produced by human monocyte-derived macrophages infected with Neospora caninum. Parasitology 145, 871–884. doi: 10.1017/S0031182017002104
Bozzo, G., Bonerba, E., Di Pinto, A., Bolzoni, G., Ceci, E., Mottola, A., et al. (2014). Occurrence of Prototheca spp. in cow milk samples. New Microbiol. 37, 459–464.
Bustin, S. A., Benes, V., Garson, J. A., Hellemans, J., Huggett, J., Kubista, M., et al. (2009). The MIQE guidelines: minimum information for publication of quantitative real-time PCR experiments. Clin. Chem. 55, 611–622. doi: 10.1373/clinchem.2008.112797
Capra, E., Cremonesi, P., Cortimiglia, C., Bignoli, G., Ricchi, M., Moroni, P., et al. (2014). Simultaneous identification by multiplex PCR of major Prototheca spp. isolated from bovine and buffalo intramammary infection and bulk tank. Lett. Appl. Microbiol. 59, 642–647. doi: 10.1111/lam.12326
Carfora, V., Noris, G., Caprioli, A., Iurescia, M., Stravino, F., and Franco, A. (2017). Evidence of a Prototheca zopfii genotype 2 disseminated infection in a dog with cutaneous lesions. Mycopathologia 182, 603–608. doi: 10.1007/s11046-016-0108-2
Chao, S. C., Hsu, M. M., and Lee, J. Y. (2002). Cutaneous protothecosis: report of five cases. Br. J. Dermatol. 146, 688–693. doi: 10.1046/j.1365-2133.2002.04609.x
Chen, X., Niyonsaba, F., Ushio, H., Nagaoka, I., Ikeda, S., Okumura, K., et al. (2006). Human cathelicidin LL-37 increases vascular permeability in the skin via mast cell activation, and phosphorylates MAP kinases p38 and ERK in mast cells. J. Dermatol. Sci. 43, 63–66. doi: 10.1016/j.jdermsci.2006.03.001
Chen, X., Takai, T., Xie, Y., Niyonsaba, F., Okumura, K., and Ogawa, H. (2013). Human antimicrobial peptide LL-37 modulates proinflammatory responses induced by cytokine milieus and double-stranded RNA in human keratinocytes. Biochem. Biophysic. Res. Commun. 433, 532–537. doi: 10.1016/j.bbrc.2013.03.024
Cirone, K. M., Lahiri, P., Holani, R., Tan, Y. L., Arrazuria, R., De Buck, J., et al. (2019). Synthetic cathelicidin LL-37 reduces Mycobacterium avium subsp. paratuberculosis internalization and pro-inflammatory cytokines in macrophages. Cell Tissue Res. 379, 207–217. doi: 10.1007/s00441-019-03098-4
Cobo, E. R., He, C., Hirata, K., Hwang, G., Tran, U., Eckmann, L., et al. (2012). Entamoeba histolytica induces intestinal cathelicidins but is resistant to cathelicidin-mediated killing. Infect. Immun. 80, 143–149. doi: 10.1128/IAI.05029-11
Cobo, E. R., Kissoon-Singh, V., Moreau, F., Holani, R., and Chadee, K. (2017). MUC2 mucin and butyrate contribute to the synthesis of the antimicrobial peptide cathelicidin in response to Entamoeba histolytica- and dextran sodium sulfate-induced colitis. Infect. Immun. 85, e00905–16. doi: 10.1128/IAI.00905-16
Coorens, M., Scheenstra, M. R., Veldhuizen, E. J., and Haagsman, H. P. (2017). Interspecies cathelicidin comparison reveals divergence in antimicrobial activity, TLR modulation, chemokine induction and regulation of phagocytosis. Sci. Rep. 7:40874. doi: 10.1038/srep40874
De Filippo, K., Dudeck, A., Hasenberg, M., Nye, E., van Rooijen, N., Hartmann, K., et al. (2013). Mast cell and macrophage chemokines CXCL1/CXCL2 control the early stage of neutrophil recruitment during tissue inflammation. Blood 121, 4930–4937. doi: 10.1182/blood-2013-02-486217
Deng, Z., Shahid, M., Zhang, L., Gao, J., Gu, X., Zhang, S., et al. (2016). An investigation of the innate immune response in bovine mammary epithelial cells challenged by Prototheca zopfii. Mycopathologia 181, 823–832. doi: 10.1007/s11046-016-0053-0
Gao, J., Zhang, H., He, J., He, Y., Li, S., Hou, R., et al. (2012). Characterization of Prototheca zopfii associated with outbreak of bovine clinical mastitis in herd of Beijing, China. Mycopathologia 173, 275–281. doi: 10.1007/s11046-011-9510-y
Gogoi-Tiwari, J., Williams, V., Waryah, C. B., Costantino, P., Al-Salami, H., Mathavan, S., et al. (2017). Mammary gland pathology subsequent to acute infection with strong versus weak biofilm forming Staphylococcus aureus bovine mastitis isolates: a pilot study using non-invasive mouse mastitis model. PLoS ONE 12:e0170668. doi: 10.1371/journal.pone.0170668
Gonen, E., Nedvetzki, S., Naor, D., and Shpigel, N. Y. (2008). CD44 is highly expressed on milk neutrophils in bovine mastitis and plays a role in their adhesion to matrix and mammary epithelium. Vet. Res. 39:29. doi: 10.1051/vetres:2008005
Greenbaum, D., Colangelo, C., Williams, K., and Gerstein, M. (2003). Comparing protein abundance and mRNA expression levels on a genomic scale. Genome Biol. 4:117. doi: 10.1186/gb-2003-4-9-117
Hirose, N., Hua, Z., Kato, Y., Zhang, Q., Li, R., Nishimura, K., et al. (2018). Molecular characterization of Prototheca strains isolated in China revealed the first cases of protothecosis associated with Prototheca zopfii genotype 1. Med. Mycol. 56, 279–287. doi: 10.1093/mmy/myx039
Huth, N., Wenkel, R. F., Roschanski, N., Rosler, U., Plagge, L., and Schoniger, S. (2015). Prototheca zopfii genotype 2-induced nasal dermatitis in a cat. J. Comp. Pathol. 152, 287–290. doi: 10.1016/j.jcpa.2015.02.001
Ibeagha-Awemu, E. M., Ibeagha, A. E., Messier, S., and Zhao, X. (2010). Proteomics, genomics, and pathway analyses of Escherichia coli and Staphylococcus aureus infected milk whey reveal molecular pathways and networks involved in mastitis. J. Proteome Res. 9, 4604–4619. doi: 10.1021/pr100336e
Iimura, M., Gallo, R. L., Hase, K., Miyamoto, Y., Eckmann, L., and Kagnoff, M. F. (2005). Cathelicidin mediates innate intestinal defense against colonization with epithelial adherent bacterial pathogens. J. Immunol. 174, 4901–4907. doi: 10.4049/jimmunol.174.8.4901
Irrgang, A., Murugaiyan, J., Weise, C., Azab, W., and Roesler, U. (2015). Well-known surface and extracellular antigens of pathogenic microorganisms among the immunodominant proteins of the infectious microalgae Prototheca zopfii. Front. Cell. Infect. Microbiol. 5:67. doi: 10.3389/fcimb.2015.00067
Ito, T., Kano, R., Sobukawa, H., Ogawa, J., Honda, Y., Hosoi, Y., et al. (2011). Experimental infection of bovine mammary gland with Prototheca zopfii genotype 1. J. Vet. Med. Sci. 73, 117–119. doi: 10.1292/jvms.10-0242
Jagielski, T., Bakula, Z., Gawor, J., Maciszewski, K., Kusber, W. H., and Dylag, M. (2019a). The genus Prototheca (Trebouxiophyceae, Chlorophyta) revisited: implications from molecular taxonomic studies. Algal Res. 43:101639. doi: 10.1016/j.algal.2019.101639
Jagielski, T., Buzzini, P., Lassa, H., Malinowski, E., Branda, E., Turchetti, B., et al. (2012). Multicentre Etest evaluation of in vitro activity of conventional antifungal drugs against European bovine mastitis Prototheca spp. isolates. J. Antimicrob. Chemother. 67, 1945–1947. doi: 10.1093/jac/dks134
Jagielski, T., Gawor, J., Bakula, Z., Decewicz, P., Maciszewski, K., and Karnkowska, A. (2018). cytb as a new genetic marker for differentiation of Prototheca species. J. Clin. Microbiol. 56, e00584–18. doi: 10.1128/JCM.00584-18
Jagielski, T., Krukowski, H., Bochniarz, M., Piech, T., Roeske, K., Bakula, Z., et al. (2019b). Prevalence of Prototheca spp. on dairy farms in Poland - a cross-country study. Microb. Biotechnol. 12, 556–566. doi: 10.1111/1751-7915.13394
Jagielski, T., and Lagneau, P. E. (2007). Protothecosis. A pseudofungal infection. J. Mycologie Medicale 17, 261–270. doi: 10.1016/j.mycmed.2007.08.003
Jagielski, T., Roeske, K., Bakula, Z., Piech, T., Wlazlo, L., Bochniarz, M., et al. (2019c). Corrigendum to “A survey on the incidence of Prototheca mastitis in dairy herds in Lublin province, Poland” (J. Dairy Sci. 102:619-628). J. Dairy Sci. 102:8586. doi: 10.3168/jds.2019-102-9-8586
Jagielski, T., Roeske, K., Bakula, Z., Piech, T., Wlazlo, L., Bochniarz, M., et al. (2019d). A survey on the incidence of Prototheca mastitis in dairy herds in Lublin province, Poland. J. Dairy Sci. 102, 619–628. doi: 10.3168/jds.2018-15495
Janosi, S., Ratz, F., Szigeti, G., Kulcsar, M., Kerenyi, J., Lauko, T., et al. (2001). Review of the microbiological, pathological, and clinical aspects of bovine mastitis caused by the alga Prototheca zopfii. Vet. Q. 23, 58–61. doi: 10.1080/01652176.2001.9695082
Katz, E., and Streuli, C. H. (2007). The extracellular matrix as an adhesion checkpoint for mammary epithelial function. Int. J. Biochem. Cell Biol. 39, 715–726. doi: 10.1016/j.biocel.2006.11.004
Kirikae, T., Hirata, M., Yamasu, H., Kirikae, F., Tamura, H., Kayama, F., et al. (1998). Protective effects of a human 18-kilodalton cationic antimicrobial protein (CAP18)-derived peptide against murine endotoxemia. Infect. Immun. 66, 1861–1868. doi: 10.1128/IAI.66.5.1861-1868.1998
Koczulla, R., von Degenfeld, G., Kupatt, C., Krotz, F., Zahler, S., Gloe, T., et al. (2003). An angiogenic role for the human peptide antibiotic LL-37/hCAP-18. J. Clin. Invest. 111, 1665–1672. doi: 10.1172/JCI17545
Kurosaka, K., Chen, Q., Yarovinsky, F., Oppenheim, J. J., and Yang, D. (2005). Mouse cathelin-related antimicrobial peptide chemoattracts leukocytes using formyl peptide receptor-like 1/mouse formyl peptide receptor-like 2 as the receptor and acts as an immune adjuvant. J. Immunol. 174, 6257–6265. doi: 10.4049/jimmunol.174.10.6257
Kwiecinski, J. (2015). Biofilm formation by pathogenic Prototheca algae. Lett. Appl. Microbiol. 61, 511–517. doi: 10.1111/lam.12497
Lam, J., Herant, M., Dembo, M., and Heinrich, V. (2009). Baseline mechanical characterization of J774 macrophages. Biophys. J. 96, 248–254. doi: 10.1529/biophysj.108.139154
Lass-Florl, C., and Mayr, A. (2007). Human protothecosis. Clin. Microbiol. Rev. 20, 230–242. doi: 10.1128/CMR.00032-06
Lopez-Garcia, B., Lee, P. H. A., and Gallo, R. L. (2006). Expression and potential function of cathelicidin antimicrobial peptides in dermatophytosis and tinea versicolor. J. Antimicrob. Chemother. 57, 877–882. doi: 10.1093/jac/dkl078
Mansour, S. C., Pena, O. M., and Hancock, R. E. W. (2014). Host defense peptides: front-line immunomodulators. Trends Immunol. 35, 443–450. doi: 10.1016/j.it.2014.07.004
Marin, M., Holani, R., Blyth, G. A. D., Drouin, D., Odeon, A., and Cobo, E. R. (2019). Human cathelicidin improves colonic epithelial defenses against Salmonella typhimurium by modulating bacterial invasion, TLR4 and pro-inflammatory cytokines. Cell Tissue Res. 376, 433–442. doi: 10.1007/s00441-018-02984-7
Moller, A., Truyen, U., and Roesler, U. (2007). Prototheca zopfii genotype 2: the causative agent of bovine protothecal mastitis? Vet. Microbiol. 120, 370–374. doi: 10.1016/j.vetmic.2006.10.039
Montreekachon, P., Chotjumlong, P., Bolscher, J. G. M., Nazmi, K., Reutrakul, V., and Krisanaprakornkit, S. (2011). Involvement of P2X(7) purinergic receptor and MEK1/2 in interleukin-8 up-regulation by LL-37 in human gingival fibroblasts. J. Periodontal Res. 46, 327–337. doi: 10.1111/j.1600-0765.2011.01346.x
Murakami, M., Dorschner, R. A., Stern, L. J., Lin, K. H., and Gallo, R. L. (2005). Expression and secretion of cathelicidin antimicrobial peptides in murine mammary glands and human milk. Pediatr. Res. 57, 10–15. doi: 10.1203/01.PDR.0000148068.32201.50
Nagaoka, I., Hirota, S., Niyonsaba, F., Hirata, M., Adachi, Y., Tamura, H., et al. (2001). Cathelicidin family of antibacterial peptides CAP18 and CAP11 inhibit the expression of TNF-α by blocking the binding of LPS to CD14(+) cells. J. Immunol. 167, 3329–3338. doi: 10.4049/jimmunol.167.6.3329
Nijnik, A., Pistolic, J., Filewod, N. C., and Hancock, R. E. (2012). Signaling pathways mediating chemokine induction in keratinocytes by cathelicidin LL-37 and flagellin. J. Innate. Immun. 4, 377–386. doi: 10.1159/000335901
Niyonsaba, F., Someya, A., Hirata, M., Ogawa, H., and Nagaoka, I. (2001). Evaluation of the effects of peptide antibiotics human beta-defensins-1/-2 and LL-37 on histamine release and prostaglandin D(2) production from mast cells. Eur. J. Immunol. 31, 1066–1075. doi: 10.1002/1521-4141(200104)31:4<1066::AID-IMMU1066>3.0.CO;2-#
Niyonsaba, F., Ushio, H., Hara, M., Yokoi, H., Tominaga, M., Takamori, K., et al. (2010). Antimicrobial peptides human beta-defensins and cathelicidin LL-37 induce the secretion of a pruritogenic cytokine IL-31 by human mast cells. J. Immunol. 184, 3526–3534. doi: 10.4049/jimmunol.0900712
Nizet, V., Ohtake, T., Lauth, X., Trowbridge, J., Rudisill, J., Dorschner, R. A., et al. (2001). Innate antimicrobial peptide protects the skin from invasive bacterial infection. Nature 414, 454–457. doi: 10.1038/35106587
Ordonez, S. R., Amarullah, I. H., Wubbolts, R. W., Veldhuizen, E. J., and Haagsman, H. P. (2014). Fungicidal mechanisms of cathelicidins LL-37 and CATH-2 revealed by live-cell imaging. Antimicrob. Agents Chemother. 58, 2240–2248. doi: 10.1128/AAC.01670-13
Roesler, U., Moller, A., Hensel, A., Baumann, D., and Truyen, U. (2006). Diversity within the current algal species Prototheca zopfii: A proposal for two Prototheca zopfii genotypes and description of a novel species, Prototheca blaschkeae sp. nov. Int. J. Syst. Evol. Microbiol. 56, 1419–1425. doi: 10.1099/ijs.0.63892-0
Rösler, U., Scholz, H., and Hensel, A. (2003). Emended phenotypic characterization of Prototheca zopfii: a proposal for three biotypes and standards for their identification. Int. J. Syst. Evol. Microbiol. 53, 1195–1199. doi: 10.1099/ijs.0.02556-0
Scaccabarozzi, L., Turchetti, B., Buzzini, P., Pisoni, G., Bertocchi, L., Arrigoni, N., et al. (2008). Short communication: Isolation of Prototheca species strains from environmental sources in dairy herds. J. Dairy Sci. 91, 3474–3477. doi: 10.3168/jds.2008-1115
Schauber, J., Svanholm, C., Termen, S., Iffland, K., Menzel, T., Scheppach, W., et al. (2003). Expression of the cathelicidin LL-37 is modulated by short chain fatty acids in colonocytes: relevance of signalling pathways. Gut 52, 735–741. doi: 10.1136/gut.52.5.735
Scott, M. G., Davidson, D. J., Gold, M. R., Bowdish, D., and Hancock, R. E. W. (2002). The human antimicrobial peptide LL-37 is a multifunctional modulator of innate immune responses. J. Immunol. 169, 3883–3891. doi: 10.4049/jimmunol.169.7.3883
Seok, J. Y., Lee, Y., Lee, H., Yi, S. Y., Oh, H. E., and Song, J. S. (2013). Human cutaneous protothecosis: report of a case and literature review. Korean J. Pathol. 47, 575–578. doi: 10.4132/KoreanJPathol.2013.47.6.575
Shahid, M., Ali, T., Zhang, L., Hou, R., Zhang, S., Ding, L., et al. (2016). Characterization of Prototheca zopfii genotypes isolated from cases of bovine mastitis and cow barns in China. Mycopathologia 181, 185–195. doi: 10.1007/s11046-015-9951-9
Shahid, M., Gao, J., Zhou, Y., Liu, G., Ali, T., Deng, Y., et al. (2017a). Prototheca zopfii isolated from bovine mastitis induced oxidative stress and apoptosis in bovine mammary epithelial cells. Oncotarget 8, 31938–31947. doi: 10.18632/oncotarget.16653
Shahid, M., Wang, J., Gu, X., Chen, W., Ali, T., Gao, J., et al. (2017b). Prototheca zopfii induced ultrastructural features associated with apoptosis in bovine mammary epithelial cells. Front. Cell. Infect. Microbiol. 7:299. doi: 10.3389/fcimb.2017.00299
Singh, D., Qi, R., Jordan, J. L., San Mateo, L., and Kao, C. C. (2013). The human antimicrobial peptide LL-37, but not the mouse ortholog, mCRAMP, can stimulate signaling by poly(I:C) through a FPRL1-dependent pathway. J. Biol. Chem. 288, 8258–8268. doi: 10.1074/jbc.M112.440883
Tello-Zavala, M. C., Mercado-Lara, A., Gomez-Hernandez, N., and Recio-Martinez, V. (2013). Disseminated protothecosis in a Mexican child. Pediatr. Infect. Dis. J. 32, e476–477. doi: 10.1097/INF.0b013e3182a2cfb2
Tjabringa, G. S., Aarbiou, J., Ninaber, D. K., Drijfhout, J. W., Sorensen, O. E., Borregaard, N., et al. (2003). The antimicrobial peptide LL-37 activates innate immunity at the airway epithelial surface by transactivation of the epidermal growth factor receptor. J. Immunol. 171, 6690–6696. doi: 10.4049/jimmunol.171.12.6690
Tjabringa, G. S., Ninaber, D. K., Drijfhout, J. W., Rabe, K. F., and Hiemstra, P. S. (2006). Human cathelicidin LL-37 is a chemoattractant for eosinophils and neutrophils that acts via formyl-peptide receptors. Int. Arch. Allergy Immunol. 140, 103–112. doi: 10.1159/000092305
Tomasinsig, L., De Conti, G., Skerlavaj, B., Piccinini, R., Mazzilli, M., D'Este, F., et al. (2010). Broad-spectrum activity against bacterial mastitis pathogens and activation of mammary epithelial cells support a protective role of neutrophil cathelicidins in bovine mastitis. Infect. Immun. 78, 1781–1788. doi: 10.1128/IAI.01090-09
Tomasinsig, L., Skerlavaj, B., Scarsini, M., Guida, F., Piccinini, R., Tossi, A., et al. (2012). Comparative activity and mechanism of action of three types of bovine antimicrobial peptides against pathogenic Prototheca spp. J. Pept. Sci. 18, 105–113. doi: 10.1002/psc.1422
Tomasinsig, L., and Zanetti, M. (2005). The cathelicidins–structure, function and evolution. Curr. Protein Pept. Sci. 6, 23–34. doi: 10.2174/1389203053027520
Tsai, P. W., Cheng, Y. L., Hsieh, W. P., and Lan, C. Y. (2014). Responses of Candida albicans to the human antimicrobial peptide LL-37. J. Microbiol. 52, 581–589. doi: 10.1007/s12275-014-3630-2
Vandamme, D., Landuyt, B., Luyten, W., and Schoofs, L. (2012). A comprehensive summary of LL-37, the factotum human cathelicidin peptide. Cell. Immunol. 280, 22–35. doi: 10.1016/j.cellimm.2012.11.009
Wawron, W., Bochniarz, M., Piech, T., Lopuszynski, W., and Wysocki, J. (2013). Outbreak of protothecal mastitis in a herd of dairy cows in Poland. Bull. Vet. Inst. Pulawy 57, 335–339. doi: 10.2478/bvip-2013-0058
Wirth, F. A., Passalacqua, J. A., and Kao, G. (1999). Disseminated cutaneous protothecosis in an immunocompromised host: a case report and literature review. Cutis 63, 185–188.
Wu, W. K. K., Wong, C. C. M., Li, Z. J., Zhang, L., Ren, S. X., and Cho, C. H. (2010). Cathelicidins in inflammation and tissue repair: potential therapeutic applications for gastrointestinal disorders. Acta Pharmacol. Sin. 31, 1118–1122. doi: 10.1038/aps.2010.117
Xiao, H. B., Wang, C. R., Liu, Z. K., and Wang, J. Y. (2015). LPS induces pro-inflammatory response in mastitis mice and mammary epithelial cells: possible involvement of NF-κB signaling and OPN. Pathol. Biol. 63, 11–16. doi: 10.1016/j.patbio.2014.10.005
Yamasaki, K., Di, N. A., Bardan, A., Murakami, M., Ohtake, T., Coda, A., et al. (2007). Increased serine protease activity and cathelicidin promotes skin inflammation in rosacea. Nat. Med. 13, 975–980. doi: 10.1038/nm1616
Yang, D., Chen, Q., Chertov, O., and Oppenheim, J. J. (2000). Human neutrophil defensins selectively chemoattract naive T and immature dendritic cells. J. Leukoc. Biol. 68, 9–14. doi: 10.1189/jlb.68.1.9
Young-Speirs, M., Drouin, D., Cavalcante, P. A., Barkema, H. W., and Cobo, E. R. (2018). Host defense cathelicidins in cattle: types, production, bioactive functions and potential therapeutic and diagnostic applications. Int. J. Antimicrob. Agents 51, 813–821. doi: 10.1016/j.ijantimicag.2018.02.006
Zanetti, M. (2005). The role of cathelicidins in the innate host defenses of mammals. Curr. Issues Mol. Biol. 7, 179–196.
Zeng, X., Kudinha, T., Kong, F., and Zhang, Q. Q. (2019). Comparative genome and transcriptome study of the gene expression difference between pathogenic and environmental strains of Prototheca zopfii. Front. Microbiol. 10:443. doi: 10.3389/fmicb.2019.00443
Zhang, C. L., Wang, Y., Chen, H., Fang, X., and Gu, C. (2012). The chemokine receptor 1 gene polymorphism and its association with somatic cell score and milk production traits in dairy cattle. Anim. Sci. Papers Rep. 30, 25–33.
Keywords: Cramp, LL-37, Prototheca bovis, mastitis, murine model
Citation: Shahid M, Cavalcante PA, Knight CG, Barkema HW, Han B, Gao J and Cobo ER (2020) Murine and Human Cathelicidins Contribute Differently to Hallmarks of Mastitis Induced by Pathogenic Prototheca bovis Algae. Front. Cell. Infect. Microbiol. 10:31. doi: 10.3389/fcimb.2020.00031
Received: 23 July 2019; Accepted: 17 January 2020;
Published: 07 February 2020.
Edited by:
Luis-Miguel Ortega-Mora, Complutense University of Madrid, SpainReviewed by:
Matteo Ricchi, Experimental Zooprophylactic Institute of Lombardy and Emilia Romagna (IZSLER), ItalyYipeng Wang, Soochow University, China
Tomasz Jagielski, University of Warsaw, Poland
Copyright © 2020 Shahid, Cavalcante, Knight, Barkema, Han, Gao and Cobo. This is an open-access article distributed under the terms of the Creative Commons Attribution License (CC BY). The use, distribution or reproduction in other forums is permitted, provided the original author(s) and the copyright owner(s) are credited and that the original publication in this journal is cited, in accordance with accepted academic practice. No use, distribution or reproduction is permitted which does not comply with these terms.
*Correspondence: Eduardo R. Cobo, ZWNvYm8mI3gwMDA0MDt1Y2FsZ2FyeS5jYQ==