- 1Center for Global Infectious Disease Research, Seattle Children's Research Institute, Seattle, WA, United States
- 2Department of Medicine, University of California, San Diego, La Jolla, CA, United States
- 3Department of Biology, Georgetown University, Washington, DC, United States
Parasitic diseases cause significant morbidity and mortality in the developing and underdeveloped countries. No efficacious vaccines are available against most parasitic diseases and there is a critical need for developing novel vaccine strategies for care. IL-21 is a pleiotropic cytokine whose functions in protection and immunopathology during parasitic diseases have been explored in limited ways. IL-21 and its cognate receptor, IL-21R, are highly expressed in parasitized organs of infected humans as well in murine models of the human parasitic diseases. Prior studies have indicated the ability of the IL-21/IL-21R signaling axis to regulate the effector functions (e.g., cytokine production) of T cell subsets by enhancing the expression of T-bet and STAT4 in human T cells, resulting in an augmented production of IFN-γ. Mice deficient for either IL-21 (Il21−/−) or IL-21R (Il21r−/−) showed significantly reduced inflammatory responses following parasitic infections as compared with their WT counterparts. Targeting the IL-21/IL-21R signaling axis may provide a novel approach for the development of new therapeutic agents for the prevention of parasite-induced immunopathology and tissue destruction.
Introduction
Parasitic diseases pose a major public threat to the world's population, causing immense morbidity and mortality, especially in underdeveloped and developing countries (Mackey et al., 2014; Torgerson et al., 2014, 2015). Parasites colonize and replicate in a wide range of human tissues and clinical symptoms can range from a completely asymptomatic profile to severe disease profiles that can be either transient or chronic (Solaymani-Mohammadi and Singer, 2010). In immunocompetent individuals, most parasitic infections are generally considered self-limited, indicating the development and the maintenance of protective immune mechanisms against invading parasites (Okhuysen, 2001; Meamar et al., 2006; Saporito et al., 2013; Lanocha-Arendarczyk et al., 2018). However, multiple studies have suggested that host immune responses elicited during parasitic infections can mediate immunopathology and are responsible for many of the symptoms commonly observed during parasitic diseases (Phillips and Fox, 1984; Pesce et al., 2006; Babaei et al., 2016; Ivanova et al., 2019; Weaver et al., 2019). These immunopathological changes may include parasite-induced morphological, functional, physiological, and structural alterations in parasitized tissues/cells, rendering infected individuals susceptible to organ dysfunction as well as the development of severe forms of clinical disease (Solaymani-Mohammadi and Singer, 2013; Taniguchi et al., 2015; Gorosito Serran et al., 2017; Ma'ayeh et al., 2018).
Increasing evidence suggests that immune responses meant to eliminate or restrict the invading tissue-residing parasites can drive organ fibrosis and the subsequent organ dysfunction (Pesce et al., 2006; Nair et al., 2011; Adams et al., 2014; Sripa et al., 2018; Ferreira et al., 2019). For example, continuous and chronic infection episodes of the liver in humans infected with the blood fluke, Schistosoma mansoni, or cystic hydatidosis caused by the larval stage of Echinococcus granulosus can eventually lead to severe forms of liver fibrosis (Zhang C. et al., 2016; Labsi et al., 2018; Yong et al., 2019). It has been suggested that TH1 and TH2 immune responses have opposing functions during human schistosomiasis; TH2 polarization, mainly in response to Schistosoma egg antigens, promotes liver fibrosis during human schistosomiasis, whereas a TH1-biased immune response inhibits the development of liver fibrosis during schistosomiasis (Mentink-Kane and Wynn, 2004; Wynn, 2004; Wynn et al., 2004). Increased levels of TH1-associated cytokines, such as TNF-α and IFN-γ, are observed in the blood and tissues in different clinical presentations of malaria, suggesting pivotal roles played by these cytokines in protection against malaria in both rodent and human malaria (Hernandez-Valladares et al., 2006; Poovassery et al., 2009; Korner et al., 2010; Fauconnier et al., 2012). However, it is well-established that enhanced levels of TNF-α are associated with a greater risk of the development of severe malaria in humans and mice deficient for this cytokine were resistant to the development of cerebral malaria (CM) following Plasmodium berghei infection (Rudin et al., 1997; Perera et al., 2013; Dunst et al., 2017). Further analyses have indicated that proinflammatory cytokines, such as TNF-α, can regulate the expression of chemokine/chemokine receptors and other adhesion molecules by endothelial cells of the brain and they can enhance the trafficking and the homing of inflammatory leukocytes to the brain, leading to an exacerbated disease profile (Pober and Sessa, 2007; Miu et al., 2008).
Due to a variety of factors, including elaborate mechanisms of antigenic variation and immune evasion used by many parasites, there have been no effective vaccines approved for humans against parasitic diseases. This lack of efficacious vaccines for most parasitic infections in humans coupled with the increasing emergence of drug-resistant parasite strains requires the continuous development of new therapeutic approaches. A comprehensive understanding of the host-parasite interactions as well as the cytokine crosstalk following parasitic infections, including identification of the mechanisms by which protective and deleterious immune responses are regulated, can help design novel strategies for fighting parasitic infections and reducing the associated morbidity and mortality.
IL-21/IL-21R Signaling Pathway
IL-21 is a member of the common gamma chain (γc) family of cytokines and is expressed by multiple immune cell types (Spolski and Leonard, 2008), with activated CD4+ T cells, including T follicular helper (TFH) cells and natural killer (NK) T cells, being the major sources of this cytokine (Spolski and Leonard, 2010a; Crotty, 2011; Linterman et al., 2011). The induction of IL-21 in activated CD4+ T cells is mediated by c-Maf in vitro (Hiramatsu et al., 2010; Kroenke et al., 2012) and in vivo (Bauquet et al., 2009), whereas the expression of c-Maf in CD4+ T cells is regulated by IL-6 (Hiramatsu et al., 2010) or IL-27 (Pot et al., 2009).
The biological functions of IL-21 are mediated by binding to its corresponding receptor, IL-21R. IL-21R is expressed by a wide range of immune cells, including T and B cells, NK cells, DCs and macrophages as well as non-immune cells, including epithelial cells and keratinocytes (Distler et al., 2005; Caruso et al., 2007; Crotty, 2011). The ubiquitous expression of the IL-21R may explain the broad biological functions of IL-21 on the cells of hemopoietic and non-hemopoietic origins. The IL-21/IL-21R signaling activates the Janus kinase (JAK1/3)-signal transducer and activator of transcription (STAT) signaling pathway (Spolski and Leonard, 2008). Accordingly, the phosphorylated STAT proteins are dimerized and translocated into the nucleus, where they bind to interferon (IFN)-γ-activated sequence (GAS) elements and initiate a gene transcription profile (i.e., Gzma, Gzmb, Il10, Eomes, Rorgt) (Spolski and Leonard, 2014). IL-21 exerts its regulatory functions on the target cells predominantly via the activation of STAT3 but it also recruits STAT1 and STAT5 (Spolski and Leonard, 2008; Wan et al., 2013). The cascade of signaling events downstream of the IL-21-induced activation of STAT3 is well-characterized, however, what is still unclear is how the activation of STAT1 by IL-21 regulates the expression of the downstream IL-21 target genes (Wan et al., 2013). Interestingly, the IL-21-induced activation of STAT1 leads to the augmented expression of Tbx21 and Ifng genes (Wan et al., 2015).
TFH cells are considered one of the major sources of the IL-21 production (Spolski and Leonard, 2008, 2010a,b). These cells are a specialized subset of CD4+ T cells that can promote T cell-dependent humoral immune responses (Zotos et al., 2010; Rankin et al., 2011; Achour et al., 2017). Multiple signaling pathways contribute to the differentiation and the development of TFH cells, among which IL-6 and the inducible T-cell costimulator (ICOS) ligand or ICOSL (CD275) have been shown to be important in the early differentiation of these cells in the mouse (Vinuesa et al., 2016). TFH cells are identified by several surface markers, including CXCR5, ICOS, PD-1 and, in addition to IL-21, these cells canonically secret C-X-C motif chemokine 13 (CXCL13) and IL-4 (Crotty, 2011; Liang et al., 2011; Linterman et al., 2011; Kroenke et al., 2012). CXCL13 is expressed predominantly by non-TFH cell sources (i.e., stromal cells) in mice, whereas TFH cells are the major source of this molecule in humans (Pitzalis et al., 2014; Crotty, 2019). The transcription factor, B-cell lymphoma 6 (Bcl6) is required for the differentiation of TFH cells (Nurieva et al., 2009; Hollister et al., 2013), however, other transcription factors have been shown to regulate the differentiation of TFH cells via the induction of Bcl6 (Reviewed in Crotty, 2019).
Germinal centers (GCs) are specialized locations in the secondary lymphoid tissues within which B cells proliferation, antibody somatic hypermutation and the affinity maturation (i.e., generation and selection of B cells with high affinity antibody secretion) occur (Zotos et al., 2010; Mesin et al., 2016). Severe impairment in B cells response to antigen of protein origin, a significant reduction in plasma cell formation in both the spleen and the bone marrow were observed in mice lacking IL-21 or its cognate receptor, IL-21R, Il21−/−, and Il21r−/−mice, respectively (Zotos et al., 2010). It has been proposed that the IL-21/IL-21R signaling regulates the Bcl6 expression by B cells and the TFH-derived IL-21 promotes the transition of the peri-follicular pre-GC B cell to the intrafollicular phase (Linterman et al., 2010; Gonzalez et al., 2018). In addition to its role in the regulation of GC responses, earlier reports have identified IL-21/IL-21R signaling as a switch factor for the secretion of all IgG subclasses, especially IgG1 and IgG3, as well as IgA by human splenic or peripheral naïve CD19+ B cells (Pene et al., 2004; Avery et al., 2008a). The B cell-intrinsic signaling via IL-21/IL-21R axis and the downstream STAT3 signaling have been accounted for the generation and the development of long-lived antibody responses (Avery et al., 2008b; Berglund et al., 2013). Consistent with these findings, mice lacking the IL-21R (Il21r−/−) failed to expand T cell-dependent, antigen-specific memory B cells and plasma cells (Rankin et al., 2011). Altogether, these observations demonstrate diverse biological functions for the IL-21/IL-21R axis in B cell-mediated immunity, spanning from the generation and the development of GCs to B cell proliferation, the antibody affinity maturation and the generation and the expansion of memory B cells.
IL-21 can also regulate the effector function (e.g., cytokine production) of T cell subsets alone or in synergy with other cytokines (Strengell et al., 2002). IL-21 exhibits synergy with IL-15 for the enhancement of the T-bet expression and with IL-12 for induction of STAT4-dependent DNA binding in NK cells and T cells and the subsequent augmentation of TH1-polarized immune response, as evidenced by enhanced IFN-γ production (Strengell et al., 2002). IL-21 also showed synergy with IFN-γ to induce the optimal expression of a certain set of signature interferon-stimulated genes (ISGs) in humans or mice (Figure 1) (Wan et al., 2015; Solaymani-Mohammadi and Berzofsky, 2019). Interestingly, this effect on ISG expression was independent of STAT-3. These findings exemplify the breadth and the complexity of the IL-21/IL-21R signaling axis across species and in diverse biological settings (Strengell et al., 2002).
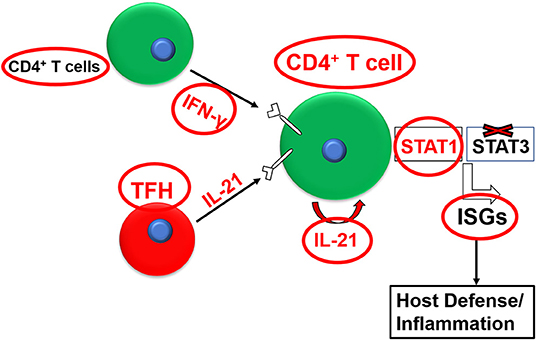
Figure 1. Synergy between the IL-21/IL-21R and the IFN-γ/IFNγR pathways for the optimal expression of interferon-stimulated genes (ISGs).
Since its discovery there has been an increasing body of evidence suggesting a variety of roles played by the IL-21/IL-21R signaling axis in the regulation of immunity and inflammation in several infectious and non-infectious settings (Ozaki et al., 2000; Sarra et al., 2010; Cupi et al., 2014; Wang et al., 2016). In recent years, the IL-21/IL-21R signaling axis has been implicated in the regulation of immunity and the pathogenesis in several bacterial, viral, fungal, and parasitic infections (Moretto and Khan, 2016; Solaymani-Mohammadi and Berzofsky, 2019; Spolski et al., 2019). The cytokine, or its cognate receptor, has been shown to be upregulated following infection with several protozoan and helminth parasites (Frohlich et al., 2007; Moretto et al., 2017). It has been suggested that the roles played by the IL-21/IL-21R signaling axis is context-dependent during microbial infections, including parasitic infections (Zhang Y. et al., 2016).
While the IL-21/IL-21R pathway has been shown to be important in protection against several microbial pathogens, the IL-21/IL-21R signaling axis has been linked to excessive inflammation and associated pathogenesis observed during infectious and non-infectious inflammatory conditions (Stolfi et al., 2011; Tortola et al., 2019). Consistent with this notion, dysregulated IL-21 expression is associated with multiple inflammatory conditions, including Crohn's disease, celiac disease and arthritis (Stolfi et al., 2011; Gensous et al., 2018; Wang et al., 2018). IL-21 is highly produced in human subjects with Crohn's disease and the blockade of the IL-21/IL-21R signaling axis in these patients resulted in reduced production of IFN-γ by mucosal lymphocytes (Monteleone et al., 2005). As such, the lack of an intact IL-21/IL-21R signaling axis in mice (Il21r−/−) resulted in attenuated inflammatory responses and reduced colitis in a murine model of human IBD (Wang et al., 2016). The activation of STAT3 in target cells has been proposed as a mechanism by which the IL-21/IL-21R signaling promotes tissue inflammation (Stolfi et al., 2011). These findings suggest that IL-21/IL-21R axis could be part of a positive feedback loop that amplifies an inflammatory response in the gut (Monteleone et al., 2005; Fina et al., 2008).
Despite the tissue distribution and the omnipresence of IL-21 target cells in tissues in which protozoan and helminth parasites reside, the role of the IL-21/IL-21R signaling pathway in protection against and/or the promotion of inflammation during parasitic infections is not fully understood. In recent years, however, it has been observed that IL-21 is induced in the periphery and mucosal tissues of humans and mice after infection with some parasites (Stumhofer et al., 2013; Carpio et al., 2015; Perez-Mazliah et al., 2015; Cai et al., 2016; Ryg-Cornejo et al., 2016; Moretto et al., 2017; Inoue et al., 2018; Wikenheiser et al., 2018) (Table 1). However, the exact mechanisms by which this signaling pathway regulates host immunity during parasitic infections are still unclear. Existing evidence suggests that the IL-21/IL-21R axis could play important roles in the development and the maintenance of anti-parasitic immunity and that targeting this signaling axis may provide an alternative approach for the development of new therapeutic agents for the prevention of parasite-induced immunopathology and tissue damage. Here, we review the roles of IL-21 and its receptor during protozoan and helminth infections in humans and in murine hosts.
Protozoan Parasites
Blood-Dwelling Apicomplexa
Plasmodium spp.
IL-21 is induced during human infections with Plasmodium falciparum (Mewono et al., 2008, 2009) and Plasmodium vivax (Figueiredo et al., 2017), as well as following vaccination against P. falciparum (Moncunill et al., 2017). In one of the first reports on IL-21 induction in malaria, serum levels of IL-21, total parasite-specific IgG, and levels of IgG subclasses were measured in 73 Gabonese children positive for P. falciparum (Mewono et al., 2008). Positive correlations were observed between IL-21 plasma levels and P. falciparum-specific anti-erythrocyte-binding antigen-175 (EBA-175)-peptide 4, and IgG1 and IgG3 subclasses. EBA-175 peptide 4, a 42-mer peptide (1062–1103), is a vaccine candidate with a molecular weight of 175-kD that binds to a sialic acid-containing ligand expressed on the surface of erythrocytes and has been shown to be critical in parasite invasion of erythrocytes (Camus and Hadley, 1985). Recombinant antibodies generated against this protein inhibited the binding of merozoites to human RBCs in vitro (Sim, 1990; Sim et al., 1990). Interestingly, the study by Mewono et al. (2008) demonstrated that the plasma levels of IL-21 also positively correlated with the hemoglobin levels as well as the parasitemia, as a marker of the disease severity, in younger children with high parasite burdens. This study, however, did not investigate a potential correlation between the serum levels of IL-21 and the clinical phenotype.
In contrast to these findings, an independent clinical study performed to investigate the pro- and anti-inflammatory cytokine signature profiles in a distinct Gabonese population found no significantly elevated IL-21 levels in the sera of children infected with P. falciparum as compared with naive controls (Oyegue-Liabagui et al., 2017). This study demonstrated a reverse correlation between the serum IL-21 and the severity of anemia. Uninfected controls were enrolled from children with a febrile syndrome or a history of fever 48 h prior to the admission. Elevated levels of IL-21 in uninfected controls could be due to non-P. falciparum induction of IL-21 production and may, in part, explain why no significant differences were observed between the levels of IL-21 in the sera of P. falciparum-infected human subjects as compared with uninfected controls. As such, the lack of significant elevation in plasma IL-21 between P. falciparum-infected as compared with uninfected control groups could be partially explained by the inclusion of uninfected controls concurrently infected with other parasitic or non-parasitic pathogens commonly found in areas where human P. falciparum infection is endemic (Dejon-Agobe et al., 2018; M'Bondoukwe et al., 2018). These two clinical studies did not measure levels of the P. falciparum-induced IL-21 production in human subjects with P. falciparum infections as well as in uninfected controls (Mewono et al., 2008; Oyegue-Liabagui et al., 2017). This lack of IL-21 production specificity may render some of the results of these two studies inconclusive.
A follow-up study by Mewono et al. (2009) demonstrated that IL-21 was induced following the stimulation of peripheral blood mononuclear cells (PBMCs) isolated from P. falciparum-experienced human subjects as compared with P. falciparum-naïve controls in response to two malaria vaccine candidates, the P. falciparum glutamate-rich protein (PfGLURP) or the P. falciparum merozoite surface protein 3 (PfMSP-3) (Mewono et al., 2009). Interestingly, PBMCs isolated from the venous blood of both P. falciparum-experienced and P. falciparum-naïve controls used in a recall assay secreted IL-21 in response to either PfGLURP or PfMSP-3, with human subjects exposed to P. falciparum producing significantly higher levels of P. falciparum peptide-induced IL-21 (Mewono et al., 2009).
PfGLURP or PfMSP-3 are expressed by all developmental stages of P. falciparum (pre-erythrocytic and erythrocytic stages) and on the surface of P. falciparum merozoites, respectively (Jordan et al., 2009; Kaur et al., 2017). These two P. falciparum-derived proteins are considered highly immunogenic and have been considered as vaccine candidates alone or in combination as fused proteins (e.g., GMZ2, a fusion of PfGLURP and PfMSP3) in clinical settings (Oeuvray et al., 1994a,b; Roussilhon et al., 2007; Belard et al., 2011). The PfGLURP- or PfMSP-3-elicited IL-21 production by PBMCs derived from P. falciparum-experienced human subjects may suggest potential roles for this cytokine to mediate immunity to P. falciparum infections and may further provide a surrogate biomarker to measure the efficacy of malaria vaccines. These finding were further augmented by the observations that RTS, S/AS01E, a vaccine consisting of sequences of the P. falciparum circumsporozoite (CS) protein (RTS,S) and the hepatitis B surface antigen (HBsAg) induced the intracellular expression of IL-21 by antigen-specific CD4+ T cells in pediatric subjects enrolled in a phase III clinical trial before and 1 month after initial vaccination (Moncunill et al., 2017).
IL-21 expression during human malaria has been mostly studied in the context of malaria infections caused by P. falciparum (Moncunill et al., 2017). However, further clinical studies demonstrated that uncomplicated P. vivax malaria infection in a Brazilian population induced significantly higher levels of serum IL-21 production and also promoted the expansion of TFH cells, defined as PD-1+ICOS+CXCR5+CD45RO+CD3+CD4+ cells, in these patients (Figueiredo et al., 2017). This study also showed the propensity of P. vivax-induced TFH cells to secret IL-21 and to induce immunoglobulin production (IgG1 and IgG3) by B cells upon being co-cultured with naïve B cells (Figueiredo et al., 2017).
IL-21 is expressed in several murine malaria models, including P. berghei (Ryg-Cornejo et al., 2016; Inoue et al., 2018), Plasmodium chabaudi (Perez-Mazliah et al., 2015; Sebina et al., 2017) and Plasmodium yoelii (Wikenheiser et al., 2018). Genetic disruption of the IL-21/IL-21R singling axis compromised P. chabaudi-specific IgG responses and resulted in the impairment of memory B cell generation. The IL-21/IL-21R axis disruption also led to impaired resolution of parasitemia in the chronic phase of infection (Perez-Mazliah et al., 2015). It also has been suggested that IL-21 promotes the humoral immune response against P. chabaudi chabaudi, as evidenced by the lack of the extrafollicular plasmablast development within the first week following the infection (Sebina et al., 2017). Mice deficient in either IL-21 (Il21−/−) or IL-21R (Il21r−/−) were susceptible to rechallenge infections with homologous murine malaria parasites (Perez-Mazliah et al., 2015). Studies using the mixed bone marrow chimeric mice also indicated that T-cell-derived (i.e., TFH cells) IL-21 interacted with the IL-21R expressed on B cells for the effective control of parasitemia during P. chabaudi infection, highlighting the requirement of T-cell/B cell crosstalk for the development and the maintenance of a robust and protective anti-malaria immune response in the mouse (Perez-Mazliah et al., 2015).
Interestingly, IL-21 and IFN-γ were co-expressed by TFH cells generated during the erythrocytic phase of P. chabaudi infection in mice (Perez-Mazliah et al., 2015). The IFN-γ-expressing T effector cells generated following P. chabaudi were predominantly positive for CXCR5, a marker for TFH cells, and acquired the ability to express IL-21 and IFN-γ, later in the course of infection and in the memory phase of the immune response (Carpio et al., 2015). Generally, the regulation of the classical CXCR5hiPD-1hi GC TFH cell subset requires Bcl6, a master transcription factor critical for the differentiation of TFH cells. However, the regulation of the CXCR5+IL-21+IFN-γ+ subset was independent of Bcl6 during infection of P. chabaudi in the mouse (Carpio et al., 2015).
Murine P. berghei ANKA infection as well as immunization with irradiated P. berghei ANKA (iPbA) induced IL-21-expressing TFH cells (Ryg-Cornejo et al., 2016). CXCR5intPD-1int TFH cells were the primary source of IL-21 expression early during the course of infection, whereas TFH cells with high expression of CXCR5 and PD-1 (CXCR5highPD-1high) also expressed IL-21 during the resolution phase of the infection (Ryg-Cornejo et al., 2016). Interestingly, infection with P. berghei, but not vaccination with iPbA, could remodel the microstructure of the splenic GC and results in impaired TFH cells differentiation (Ryg-Cornejo et al., 2016).
In addition to TFH cells, other immune cell types, including γδ T-cells and a TFH-like CD4+ T cell population expressing the surface molecule, NK1.1, and TFH cell markers simultaneously, have also been shown to express IL-21, as well as IFN-γ, during the early phase of infection with P. berghei and P. yoelii, respectively (Inoue et al., 2018; Wikenheiser et al., 2018). The importance of the IL-21 expression by non-conventional TFH cells was reinforced by the observations that mice lacking γδ T-cells were incompetent to control P. berghei infection and that the lack of ability to control infection correlated with the findings that γδ T-cell-deficient mice had impaired levels of P. berghei-specific antibodies, decreased numbers of TFH cells and fewer GC B cell toward the later course of infection as compared with their C57BL/6 counterparts (Inoue et al., 2018). Blockade of the IL-21R using the IL-21R-Fc led to a reduction in TFH cells and GC B cell numbers toward the later phase of infection, suggesting the importance of the IL-21 expressed by γδ T-cell during the early phase of infection to promote the development of TFH cells as well as the GC B cells during the later course of infection (Inoue et al., 2018). Altogether, these findings exemplify the requirement of the IL-21/IL-21R signaling axis for development of the GC reaction, the promotion of high-affinity parasite-specific antibody repertoire generation by B cells, and the regulation of the antibody class switching in these cells during experimental murine malaria infections (Perez-Mazliah et al., 2015).
Babesia sp.
The evidence supporting potential protective roles of the IL-21/IL-21R signaling axis during experimental murine babesiosis is limited (Djokic et al., 2018; Yi et al., 2018). No significant differences were observed in the plasma levels of IL-5, IL-17A, and IL-21 in Babesia microti-infected C3H/HeJ mice as compared with uninfected controls (Djokic et al., 2018). A TH1-mediated immunity, as opposed to a TH17-biased immune response, was critical for the clearance of B. microti in susceptible C3H/HeJ mice (Djokic et al., 2018). Conversely, B. microti was shown to elicit a strong immune response in C57BL/6 mice characterized by the induction of a GC reaction accompanied by the promotion of TFH cells generation and increased levels of the T cell effector cytokines, including IL-21 and IFN-γ (Yi et al., 2018). This study also showed that TFH cells expanded upon B. microti infection. However, the specific expression of IL-21 by TFH cells, as one of the major expressors of IL-21, was not determined following B. microti infection in the mouse (Yi et al., 2018). The differences in host strain susceptibility to B. microti infection, age discrepancies and the dose of inoculum could account for inconsistent findings observed between these two studies. Collectively, the lack of experimental evidence demonstrating a direct and protective role played by the IL-21/IL-21R signaling axis precludes a definite conclusion as to how this signaling axis regulates host immunity during experimental babesiosis and may warrant further future studies.
Tissue-Dwelling Apicomplexa
Toxoplasma gondii
It has been suggested that experimental toxoplasmosis elicits a strong TFH cell response and that IL-21 plays important roles in the regulation of T cell responses in an encephalitis susceptible mouse model of the disease (Moretto et al., 2017). Nevertheless, the survival rate of Il21r−/− mice infected with T. gondii was comparable with their wild-type counterparts, despite the significant impairment of IgG1 in the serum of Il21r−/− mice (Ozaki et al., 2002).
To further dissect the roles of IL-21 in defining humoral immunity and its roles in the maintenance of a balance between protective and pathogenic immune response during acute T. gondii infection, it was demonstrated that a larger proportion of IL-21-expressing CD4+ and CD8+ T cells were found in the brain of wild-type mice chronically infected with T. gondii (Stumhofer et al., 2013). As such, the requirement of IL-21 for the control of the T. gondii chronic infection was also confirmed by increased parasite burdens in the brains of chronically infected Il21−/− mice as compared with their wild-type counterparts (Stumhofer et al., 2013). Interestingly, Il21−/− mice showed no immune-mediated pathology during the acute phase of infection and IgG production was impaired in these mice as compared with wild-type controls and the impairment in IgG production in Il21−/− mice correlated with diminished numbers of GC B cells (Stumhofer et al., 2013).
It has previously been shown that IL-21 acts synergistically with several cytokines, including IL-2 (Battaglia et al., 2013), IL-15 (Kishida et al., 2003; Strengell et al., 2003; Zeng et al., 2005; Li et al., 2014), and IL-18 (Lusty et al., 2017) to exert some of its biological functions. To explore the efficacy of a DNA vaccine (pVAX/TgMIC8) encoding the T. gondii microneme protein 8 (MIC8), mice were immunized in the absence or the presence of eukaryotic plasmids expressing murine IL-15 (pVAX/mIL-15), IL-21 (pVAX/mIL-21), or both (pVAX/mIL-21/mIL-15). Mice immunized with pVAX/TgMIC8 alone or combined with pVAX/mIL-15 and, to a lesser extent IL-21 (pVAX/mIL-21), mounted strong humoral TH1 immune profiles (Li et al., 2014). Interestingly, mice co-administered with a combination of pVAX/TgMIC8 and plasmids expressing both murine IL-15 and IL-21 (pVAX/mIL-21/mIL-15) elicited the strongest immune profiles as compared with controls, leading to a significant increase in survival and decreased numbers of tissue cysts in the brain (Li et al., 2014). Similarly, vaccination with the T. gondii calcium-dependent protein kinase 1 (TgCDPK1) combined with plasmids expressing both murine IL-15 and IL-21 (pVAX/mIL-21/mIL-15) significantly increased survival time and led to reduced cyst numbers in the brain of mice receiving a combined pVAX-CDPK1/pVAX/mIL-21/mIL-15 vaccine regimen as compared with those mice receiving either pVAX-CDPK1 or pVAX/mIL-21/mIL-15 alone (Chen et al., 2014). These findings may demonstrate a synergy between IL-21 and IL-15 for the optimal expression of parasite-specific humoral and cellular immune responses following vaccine administration. IL-21, in collaboration with IFN-γ, has also been found to be required for the optimal expression of several interferon-stimulated genes, including multiple MHC class molecules and chemokine/chemokine receptors (Solaymani-Mohammadi and Berzofsky, 2019). Altogether, these observations further suggest that the inclusion of IL-21, in combination with other cytokines, as an adjuvant may lead to the optimal expression of molecules required for T cell-antigen-presenting cell (APC) interactions and may enhance the efficacy of the vaccines against T. gondii infection.
Blood-Dwelling Kinetoplastids
Trypanosoma cruzi
TH17-mediated immune responses have been mostly studied in the context of extracellular bacterial and fungal infections (Curtis and Way, 2009; Zelante et al., 2009; Sellge et al., 2010; Das and Khader, 2017). However, it has been demonstrated that T. cruzi infection elicits a strong proinflammatory cytokine profile characterized by increased expression of IFN-γ, TNF-α, and IL-17 (Silva et al., 1995; Michailowsky et al., 2001; Miyazaki et al., 2010). The TH17 response is necessary for acute resistance to T. cruzi and the transcription factor T-bet regulates T. cruzi-specific TH17 responses (Guo et al., 2009). Consistent with the protective roles of TH17 during T. cruzi infection, TH17 cells provide help to CD8+ T cells in an IL-21-dependent manner (Cai et al., 2016). Interestingly, Rag−/− mice reconstituted with Il21r−/− CD8+ T cells had consistently higher parasitemia levels as compared with those Rag−/− mice receiving wild-type CD8+ T cells and mice in the former group died before day 20 p.i. (Cai et al., 2016). These findings suggested a protective role for IL-21 in the TH17−mediated resistance via providing help to CD8+ T cells during experimental T. cruzi infection in murine hosts.
The serum levels of several cytokines, including IL-21, positively correlated with disease severity in patients with chronic Chagas disease caused by T. cruzi (Natale et al., 2018). Consistently, lower levels of IL-21 production were observed in the sera of human subjects with less severe cardiac dysfunction as compared with those presented with an advanced cardiac dysfunction profile (Natale et al., 2018). These observations may suggest a potential role for the IL-21/IL-21R axis in the promotion of pathology in chronically-infected human subjects. However, the mechanisms by which the IL-21/IL-21R signaling pathway may mediate the pathogenesis of acute and chronic T. cruzi infections still remain unclear and may warrant further investigations.
Tissue-Dwelling Kinetoplastids
Leishmania spp.
IL-21 transcripts are detectable following cutaneous leishmaniasis caused by Leishmania panamensis, Leishmania major, and Leishmania braziliensis infections as well as in humans and mice infected with Leishmania donovani, the causative agent of visceral leishmaniasis (Espitia et al., 2010; Bollig et al., 2012; Costa et al., 2015; Kong et al., 2017; Khatonier et al., 2018). IL-21 expression significantly and positively correlated with expression of IFN-γ and IL-27, but not FoxP3 or TGF-β, in human subjects with L. braziliensis cutaneous lesions (Costa et al., 2015). CD3+ T cells were the major source of IL-21 expression during visceral leishmaniasis in humans (Ansari et al., 2011). Further, recombinant human IL-21 could augment antigen-specific IL-10 production in a whole-blood assay (Ansari et al., 2011). However, an earlier report failed to establish a connection between the lack of IL-21R signaling and impaired TH1-biased immunity against L. major infection (Frohlich et al., 2007). In this study, Il21r−/− mice mounted a strong TH1 immune response against L. major infection as evidenced by a higher percentage of antigen-specific IFN-γ-producing CD4+ T cells and these mice had comparable footpad swellings as compared with C57BL/6 controls (Frohlich et al., 2007). These findings suggest that the IL-21/IL-21R signaling axis was not required for host defense against L. major and that the absence of IL-21R signaling was not critical for the development of a TH1-biased response against this intracellular protozoan parasite.
Host strain-specific susceptibility or resistant to infection is considered an important determinant of disease outcome in parasitic diseases (Araujo et al., 1976; Ruebush and Hanson, 1979; Ishii and Sano, 1989; Duleu et al., 2004). Varied susceptibility of C57BL/6 (resistant) and Balb/c (susceptible) strains to L. major, for example, represents a classical dichotomy as to how host's genetic background can contribute to disease outcome following leishmanial infections (Lohoff et al., 1989; Scott, 1991; Sommer et al., 1998; Barbi et al., 2008). Several mechanisms have been proposed as to why some mouse strains are more susceptible to a given parasite while others do not (von Stebut et al., 1998; Rosas et al., 2005; Nigg et al., 2007). These may include discrepancies in the magnitude of a particular immune pathway and/or the differential expression of immune compartments and/or immune effector molecules between mouse strains (von Stebut et al., 2000; Von Stebut et al., 2003; Hurdayal et al., 2013; Sassi et al., 2015). Analysis of the GC B cell response in the draining lymph nodes (dLNs) of C3HeB/FeJ and C57BL/6 mice (which develop healing and non-healing lesions, respectively) in a L. major/Leishmania amazonensis model of co-infection revealed that the inability of C57BL/6 mice to resolve leishmanial lesions correlated with reduced numbers of GC B cells and memory B cells and fewer parasite-specific antibody-producing cells as compared with the C3HeB/FeJ strain (Gibson-Corley et al., 2012). In this study, the differences in the local production of IL-21 or different expression levels of IL-21R did not explain the distinct clinical profiles observed between the two strains, since the two strains had comparable local production and expression of IL-21 and IL-21R, respectively (Gibson-Corley et al., 2012). These findings may suggest that varied clinical profiles in the mouse strains developing healing (C3HeB/FeJ) vs. non-healing (C57BL/6) lesions can be mainly due to the mouse strain-dependent discrepancies in intrinsic TFH cell or B cell functional properties.
It is well-established that the IFN-regulatory factor (IRF) family of transcription factors are key players in TH differentiation; whereas IRF1 is crucial for TH1 differentiation (Lohoff et al., 1997; Lohoff and Mak, 2005), IRF4 is required for TH2 and TH17 cell differentiation (Brustle et al., 2007; Huber et al., 2008). Mice deficient for IRF4 (Irf4−/−) were characterized by their failure to form GCs in the draining popliteal lymph nodes in response to chronic L. major infection as well as their inability of dLN-derived TFH cells to optimally express and secrete IL-21 at both the mRNA and protein levels, respectively (Bollig et al., 2012). These observations may suggest an important role played by IRF4 in TFH cells-derived IL-21 expression in response to a chronic, intracellular parasitic pathogen.
Helminth Parasites
Trematodes
Schistosomes
Several studies have demonstrated that IL-21 is induced at both the mRNA and protein levels during natural infections with several species of schistosomes in humans and in murine models of schistosomiasis (Milner et al., 2010; Bourke et al., 2013; Zhang Y. et al., 2016), as well as following vaccination with schistosome-derived peptides (Zhang et al., 2013, 2018; Bourke et al., 2014; Fairfax et al., 2015). Ileal lamina propria lymphocytes-(LPLs) and axillary lymph node-derived T cells and non-T cell subsets from uninfected rhesus macaques (Macaca mulatta) secreted high levels of IL-21 in a recall assay in response to S. mansoni (Sm) egg antigen (SmEA) and worm antigen (SmWA) preparations ex vivo (Torben et al., 2017). Dendritic cell-specific adhesion molecule-3-grabbing non-integrin (DC-SIGN, CD209) controls the differentiation of TFH cells via IL-27 expression during infections with S. mansoni and the liver fluke, Fasciola hepatica (Gringhuis et al., 2014). Interestingly, the transcription factor, IFN-stimulated gene factor 3 (ISGF3) induces the expression of the IL-27 subunit p28 by primed DCs, and IL-27 produced by DCs is required for the differentiation of TFH cells and the subsequent IL-21 expression by TFH cells during infections with trematodes, including S. mansoni and F. hepatica (Gringhuis et al., 2014).
In contrast to potential roles played by the IL-21/IL-21R signaling axis in protective immunity during infection with trematodes, earlier studies demonstrated that the IL-21/IL-21R signaling pathway promotes the induction of tissue damage following Schistosoma spp. infections (Pesce et al., 2006). While parasite burdens were comparable between wild-type mice and mice lacking a functional IL-21/IL-21R signaling axis (Il21r−/−), the lack of a functional IL-21/IL-21R signaling pathway in Il21r−/− mice infected with S. mansoni led to diminished TH2-dependent immunopathology in those mice, as evidenced by attenuated formation of granulomatous inflammation and liver fibrosis (Pesce et al., 2006). Mechanistically, the impaired S. mansoni-induced granulomatous formation in Il21r−/− mice correlated with a significant impairment in both the tissue expression and the function of TH2 cytokines, including IL-4 and IL-13, required for the fibrotic tissue responses during S. mansoni infection (Pesce et al., 2006). Furthermore, IL-21 treatment of the bone marrow-derived macrophages (BMDM) promotes the expression of cognate receptors for IL-4 and IL-13 (IL-4Rα and IL-13Rα1) in macrophages, indicating that the augmentation of alternate macrophage activation can occur in an IL-21/IL-21R axis-dependent manner (Pesce et al., 2006).
It is known that the ICOS/ICOSL axis contributes to the T cell differentiation, T cell activation and its optimal functions (Hutloff et al., 1999; Dong et al., 2001). Mice deficient in either a functional ICOS or ICOSL (Icos−/− and Icosl−/− mice, respectively) had diminished levels of serum antibodies, defective antibody class switching and impaired GC formation and TH17 cell development (Dong et al., 2001; Nurieva et al., 2003). Consistent with the requirement of the ICOS/ICOSL signaling pathway in the development and the function of TH17 cells, Icosl−/− mice infected with Schistosoma japonicum showed decreased expression of TH17 cytokines, including IL-21 (Wang et al., 2015). The impaired TH17 cytokine expression in Icosl−/− mice correlated with attenuated liver granulomatous inflammation around parasite eggs and the significant reduction in hepatic fibrosis in those mice as compared with wild-type controls (Wang et al., 2015).
Interestingly, IL-21 does not directly promote the production of the Schistosoma egg-induced IL-17 production by antigen-specific CD4+ T cells. Antibody neutralization of IL-21 in a DC-CD4+ T cells co-culture setting, in which DCs are pulsed with egg antigens and co-cultured with naïve CD4+ T cells, did not impair the antigen-specific IL-17 production by antigen-specific CD4+ T cells (Shainheit et al., 2008). This is consistent with studies demonstrating that the lack of the IL-21/IL-21R signaling axis does not impair the secretion of IL-17, as well as IFN-γ, following colonic inflammation (Wang et al., 2016; Solaymani-Mohammadi and Berzofsky, 2019). It is likely that the IL-21 promotion of tissue inflammation and immunopathology during schistosomiasis occurs via other signaling pathways independent of the IL-17/STAT3 signaling axis, most likely through STAT1 or STAT5 signaling.
The increased levels of IL-21 in human subjects with schistosomiasis as compared with healthy controls (Milner et al., 2010) and the fact that mice deficient for the IL-21/IL-21R signaling pathway have significantly reduced parasite-induced tissue fibrosis suggest that this axis could play important roles in parasite-induced pathogenesis. Targeting this signaling axis may provide an alternative approach for the development of new therapeutic targets for the prevention of fibrotic reactions that develop during human infections with different species of Schistosoma.
Cestodes
Cystic Echinococcosis Caused by Echinococcus granulosus
In a clinical study, the roles played by TFH cells and the cytokines they secrete, including IL-4 and IL-21, during different stages of human cystic echinococcosis (CE) were investigated in patients with active, transitional or inactive disease (Zhang et al., 2015). The findings of this study demonstrated that the percentage of circulating TFH cells, defined as CCR7loPD-1hiCXCR5+CD4+, increased in the PBMCs of patients with active and transitional disease as did the serum levels of IL-21 and IL-4 in those patients as compared with healthy controls (Zhang et al., 2015). The expression of IL-21 and IL-4 by PMBCs isolated from patients with CE significantly increased in response to the hydatid fluid as compared with PMBCs from healthy volunteers (Zhang et al., 2015). Interestingly, CE patients with inactive disease neither had increased percentages of circulating TFH cells nor did they show increased levels of IL-21 and IL-4 as compared with healthy controls. These observations suggest that TFH cells may be involved in host immunity or immunopathology associated with CE in humans via the expression of canonical cytokines expressed by these cells. However, the causality, the direction of any causality and the potential mechanisms underlying the differences in the TFH cells and cytokine profiles between human subjects with active, transitional or inactive CE still require further investigation.
Nematodes
Tissue- and Lumen-Dwelling Nematodes
Limited evidence suggests that TH17 cytokines, including IL-17A, IL-17F, IL-21, and IL-23, are elevated in human subjects with lymphatic filariasis as well as in animal models of human filariasis and this cytokine signature is associated with helminth-induced immunopathology in chronic lymphatic filariasis (Babu et al., 2009; Anuradha et al., 2014; Ritter et al., 2018).
The roles played by the IL-21/IL-21R signaling axis in the maintenance of intestinal immune homeostasis in the context of intestinal helminthiasis is not fully-understood. IL-21 was detectable following infection of mice with a natural murine intestinal nematode, Heligmosomoides polygyrus (Espitia et al., 2010; Ariyaratne et al., 2018). The expression of IL-21 in another murine model of intestinal nematodiasis, caused by the mouse pinworm, Aspiculuris tetraptera, was tissue-dependent and significantly higher levels of IL-21 transcripts were measured in the Peyer's patches (PP), and to a lesser extent in the spleens and in the mesenteric lymph nodes, of wild-type mice infected with A. tetraptera (Gomez-Samblas et al., 2018). Mice deficient for a functional IL-21/IL-21R axis failed to elicit anti-helminth-specific IgG1 responses and subsequently were unable to clear helminth infection as compared with their wild-type counterparts (King et al., 2010). The IL-21/IL-21R axis was important for driving the differentiation of H. polygyrus-specific CD138+ plasma cells following intestinal infection (King et al., 2010). Despite the observations that Il21rα−/− had impaired differentiation of CD138+ plasma cells 2 weeks post-infection, this study did not find evidence demonstrating any roles for IL-21R signaling in GC formation, isotype class switching or TH2 differentiation (King et al., 2010). In contrast, it appears that the IL-21/IL-21R signaling axis also played critical roles in the development of TH2 immune response following infection with the intestinal luminal nematodes, Nippostrongylus brasiliensis and H. polygyrus (Frohlich et al., 2007). Mice deficient for the IL-21R (Il21r−/−) showed reduced accumulation of immune subsets in the intestine, including lymphocytes, and reduced sizes and numbers of intestinal granuloma following helminth infections, suggesting a critical requirement of the IL-21/IL-21R axis for mounting TH2 responses (Frohlich et al., 2007). These findings may also suggest a role for this signaling pathway in the initiation and the promotion of inflammation and tissue immunopathology during intestinal inflammatory condition, as observed in other models of colonic infections (Solaymani-Mohammadi and Berzofsky, 2019).
In another line of research, it was found that both IL-21 and IFN-γ can drive the expression of T-bet transcripts (Tbx21) and the intranuclear expression of T-bet protein in follicular B cells in the presence of TLR7 or TLR9 agonists in vitro (Naradikian et al., 2016). This study also demonstrated that the IFN-γ-induced expression of T-bet was independent of either IL-4 or IL-21, since IFN-γ alone was sufficient for driving the expression of T-bet in follicular B cells (Naradikian et al., 2016). In contrast, the stimulation of follicular B cells with IL-21 led to the expression of CD11c, whereas IFN-γ did not alter CD11c expression by those cells. Considering that the IL-21/IL-21R signaling axis signals mainly via STAT3, but also STAT-1 and STAT5, it is likely that the expression of CD11c is mediated by the IL-21-induced activation of STAT3 or STAT5, whereas the IL-21- or IFN-γ-induced expression of T-bet may occur mainly via the STAT1 pathway, a pathway via which both cytokines exert parts or all of their biological functions. Studies in a TH2-biased murine model of infection with H. polygyrus further confirmed that the two main cytokines secreted by TFH cells, IL-21 and IL-4, can cross-regulate the expression of T-bet and CD11c in the absence of an intact IFN-γ/IFN-γR signaling axis (Naradikian et al., 2016).
Conclusion
To conclude, we and others already have shown that, in synergy with IFN-γ, the IL-21/IL-21R axis is required for the optimal expression of ISGs, including several chemokines and chemokine receptors, as well MHC class molecules required for protection against parasitic infections, in a STAT3-independent, most likely STAT1-dependent, manner in humans or in mice (Wan et al., 2015; Solaymani-Mohammadi and Berzofsky, 2019). Based on this, the use of recombinant human IL-21 in vaccine regimens in clinical trials (for example targeting P. falciparum infections) may provide novel and unique vaccine strategies to elicit an effective anti-malarial immune response and warranting a better protective outcome. Most studies investigating the roles played by the IL-21/IL-21R axis in parasitic infections, whether protective or detrimental, stemmed from reports in animal models. However, studies in animal models require careful interpretation and cannot be simply extrapolated to humans since animal immune responses, including murine hosts, are not always equivalent to those observed in humans. For instance, studies have utilized P. berghei, a rodent malaria parasite, to analyze the extent to which the IL-21/IL-21R axis contributes to host immunity during CM in the murine hosts as a surrogate for the human disease (Inoue et al., 2018). Multiple studies, however, have highlighted significant differences as to how P. berghei induces CM in mice and that the murine infection with P. berghei does not recapitulate all aspects of the human disease (reviewed in Riley et al., 2010; Stevenson et al., 2010). Unveiling the IL-21/IL-21R crosstalk with other cytokine networks and their downstream genes will provide insight into the development of novel therapeutic targets for the parasite-induced immunopathology and the control of parasitic infections.
Author Contributions
SS-M conceived the study, contributed hypotheses, and wrote the manuscript. LE and SS critically read the manuscript and provided feedback.
Funding
This work was supported by the NIH/NIAID grant R15AI109591 (SS) and the NIH/NIDDK grant DK120515 (LE).
Conflict of Interest
The authors declare that the research was conducted in the absence of any commercial or financial relationships that could be construed as a potential conflict of interest.
Acknowledgments
We thank Dr. Stefan Kappe, Center for Global Infectious Disease Research, Seattle Children's Research Institute, Seattle, for his helpful comments and discussion.
References
Achour, A., Simon, Q., Mohr, A., Seite, J. F., Youinou, P., Bendaoud, B., et al. (2017). Human regulatory B cells control the TFH cell response. J. Allergy Clin. Immunol. 140, 215–222. doi: 10.1016/j.jaci.2016.09.042
Adams, P. N., Aldridge, A., Vukman, K. V., Donnelly, S., and O'Neill, S. M. (2014). Fasciola hepatica tegumental antigens indirectly induce an M2 macrophage-like phenotype in vivo. Prasite Immunol. 36, 531–539. doi: 10.1111/pim.12127
Ansari, N. A., Kumar, R., Gautam, S., Nylen, S., Singh, O. P., Sundar, S., et al. (2011). IL-27 and IL-21 are associated with T cell IL-10 responses in human visceral leishmaniasis. J. Immunol. 186, 3977–3985. doi: 10.4049/jimmunol.1003588
Anuradha, R., George, P. J., Kumaran, P., Nutman, T. B., and Babu, S. (2014). Interleukin-10- and transforming growth factor beta-independent regulation of CD8(+) T cells expressing type 1 and type 2 cytokines in human lymphatic filariasis. Clin. Vaccine Immunol. 21, 1620–1627. doi: 10.1128/CVI.00598-14
Araujo, F. G., Williams, D. M., Grumet, F. C., and Remington, J. S. (1976). Strain-dependent differences in murine susceptibility to toxoplasma. Infect. Immun. 13, 1528–1530.
Ariyaratne, A., Szabo, E. K., Bowron, J., and Finney, C. A. M. (2018). Increases in helminth-induced IL-21 protein levels disappear upon sample freezing. Cytokine 108, 179–181. doi: 10.1016/j.cyto.2018.04.007
Avery, D. T., Bryant, V. L., Ma, C. S., de Waal Malefyt, R., and Tangye, S. G. (2008a). IL-21-induced isotype switching to IgG and IgA by human naive B cells is differentially regulated by IL-4. J. Immunol. 181, 1767–1779. doi: 10.4049/jimmunol.181.3.1767
Avery, D. T., Ma, C. S., Bryant, V. L., Santner-Nanan, B., Nanan, R., Wong, M., et al. (2008b). STAT3 is required for IL-21-induced secretion of IgE from human naive B cells. Blood 112, 1784–1793. doi: 10.1182/blood-2008-02-142745
Babaei, Z., Malihi, N., Zia-Ali, N., Sharifi, I., Mohammadi, M. A., Kagnoff, M. F., et al. (2016). Adaptive immune response in symptomatic and asymptomatic enteric protozoal infection: evidence for a determining role of parasite genetic heterogeneity in host immunity to human giardiasis. Microbes. Infect. 18, 687–695. doi: 10.1016/j.micinf.2016.06.009
Babu, S., Bhat, S. Q., Pavan Kumar, N., Lipira, A. B., Kumar, S., Karthik, C., et al. (2009). Filarial lymphedema is characterized by antigen-specific Th1 and th17 proinflammatory responses and a lack of regulatory T cells. PLoS Negl. Trop. Dis. 3:e420. doi: 10.1371/journal.pntd.0000420
Barbi, J., Brombacher, F., and Satoskar, A. R. (2008). T cells from Leishmania major-susceptible BALB/c mice have a defect in efficiently up-regulating CXCR3 upon activation. J. Immunol. 181, 4613–4620. doi: 10.4049/jimmunol.181.7.4613
Battaglia, A., Buzzonetti, A., Baranello, C., Fanelli, M., Fossati, M., Catzola, V., et al. (2013). Interleukin-21 (IL-21) synergizes with IL-2 to enhance T-cell receptor-induced human T-cell proliferation and counteracts IL-2/transforming growth factor-beta-induced regulatory T-cell development. Immunology 139, 109–120. doi: 10.1111/imm.12061
Bauquet, A. T., Jin, H., Paterson, A. M., Mitsdoerffer, M., Ho, I. C., Sharpe, A. H., et al. (2009). The costimulatory molecule ICOS regulates the expression of c-Maf and IL-21 in the development of follicular T helper cells and TH-17 cells. Nat. Immunol. 10, 167–175. doi: 10.1038/ni.1690
Belard, S., Issifou, S., Hounkpatin, A. B., Schaumburg, F., Ngoa, U. A., Esen, M., et al. (2011). A randomized controlled phase Ib trial of the malaria vaccine candidate GMZ2 in African children. PLoS ONE 6:e22525. doi: 10.1371/journal.pone.0022525
Berglund, L. J., Avery, D. T., Ma, C. S., Moens, L., Deenick, E. K., Bustamante, J., et al. (2013). IL-21 signalling via STAT3 primes human naive B cells to respond to IL-2 to enhance their differentiation into plasmablasts. Blood 122, 3940–3950. doi: 10.1182/blood-2013-06-506865
Bollig, N., Brustle, A., Kellner, K., Ackermann, W., Abass, E., Raifer, H., et al. (2012). Transcription factor IRF4 determines germinal center formation through follicular T-helper cell differentiation. Proc. Natl. Acad. Sci. U.S.A. 109, 8664–8669. doi: 10.1073/pnas.1205834109
Bourke, C. D., Nausch, N., Rujeni, N., Appleby, L. J., Mitchell, K. M., Midzi, N., et al. (2013). Integrated analysis of innate, Th1, Th2, Th17, and regulatory cytokines identifies changes in immune polarisation following treatment of human schistosomiasis. J. Infect. Dis. 208, 159–169. doi: 10.1093/infdis/jis524
Bourke, C. D., Nausch, N., Rujeni, N., Appleby, L. J., Trottein, F., Midzi, N., et al. (2014). Cytokine responses to the anti-schistosome vaccine candidate antigen glutathione-S-transferase vary with host age and are boosted by praziquantel treatment. PLoS Negl. Trop. Dis. 8:e2846. doi: 10.1371/journal.pntd.0002846
Brustle, A., Heink, S., Huber, M., Rosenplanter, C., Stadelmann, C., Yu, P., et al. (2007). The development of inflammatory T(H)-17 cells requires interferon-regulatory factor 4. Nat. Immunol. 8, 958–966. doi: 10.1038/ni1500
Cai, C. W., Blase, J. R., Zhang, X., Eickhoff, C. S., and Hoft, D. F. (2016). Th17 Cells Are More Protective Than Th1 Cells Against the Intracellular Parasite Trypanosoma cruzi. PLoS Pathog. 12:e1005902. doi: 10.1371/journal.ppat.1005902
Camus, D., and Hadley, T. J. (1985). A Plasmodium falciparum antigen that binds to host erythrocytes and merozoites. Science 230, 553–556. doi: 10.1126/science.3901257
Carpio, V. H., Opata, M. M., Montanez, M. E., Banerjee, P. P., Dent, A. L., and Stephens, R. (2015). IFN-gamma and IL-21 double producing T cells are Bcl6-independent and survive into the memory phase in Plasmodium chabaudi infection. PLoS ONE 10:e0144654. doi: 10.1371/journal.pone.0144654
Caruso, R., Fina, D., Peluso, I., Fantini, M. C., Tosti, C., Del Vecchio Blanco, G., et al. (2007). IL-21 is highly produced in Helicobacter pylori-infected gastric mucosa and promotes gelatinases synthesis. J. Immunol. 178, 5957–5965. doi: 10.4049/jimmunol.178.9.5957
Chen, J., Li, Z. Y., Huang, S. Y., Petersen, E., Song, H. Q., Zhou, D. H., et al. (2014). Protective efficacy of Toxoplasma gondii calcium-dependent protein kinase 1 (TgCDPK1) adjuvated with recombinant IL-15 and IL-21 against experimental toxoplasmosis in mice. BMC Infect. Dis. 14:487. doi: 10.1186/1471-2334-14-487
Costa, D. L., Cardoso, T. M., Queiroz, A., Milanezi, C. M., Bacellar, O., Carvalho, E. M., et al. (2015). Tr-1-like CD4+CD25-CD127-/lowFOXP3- cells are the main source of interleukin 10 in patients with cutaneous leishmaniasis due to Leishmania braziliensis. J. Infect. Dis. 211, 708–718. doi: 10.1093/infdis/jiu406
Crotty, S. (2011). Follicular helper CD4 T cells (TFH). Annu. Rev. Immunol. 29, 621–663. doi: 10.1146/annurev-immunol-031210-101400
Crotty, S. (2019). T follicular helper cell biology: a decade of discovery and diseases. Immunity 50, 1132–1148. doi: 10.1016/j.immuni.2019.04.011
Cupi, M. L., Sarra, M., Marafini, I., Monteleone, I., Franze, E., Ortenzi, A., et al. (2014). Plasma cells in the mucosa of patients with inflammatory bowel disease produce granzyme B and possess cytotoxic activities. J. Immunol. 192, 6083–6091. doi: 10.4049/jimmunol.1302238
Curtis, M. M., and Way, S. S. (2009). Interleukin-17 in host defence against bacterial, mycobacterial and fungal pathogens. Immunology 126, 177–185. doi: 10.1111/j.1365-2567.2008.03017.x
Das, S., and Khader, S. (2017). Yin and yang of interleukin-17 in host immunity to infection. F1000Res 6:741. doi: 10.12688/f1000research.10862.1
Dejon-Agobe, J. C., Zinsou, J. F., Honkpehedji, Y. J., Ateba-Ngoa, U., Edoa, J. R., Adegbite, B. R., et al. (2018). Schistosoma haematobium effects on Plasmodium falciparum infection modified by soil-transmitted helminths in school-age children living in rural areas of Gabon. PLoS Negl. Trop. Dis. 12:e0006663. doi: 10.1371/journal.pntd.0006663
Distler, J. H., Jungel, A., Kowal-Bielecka, O., Michel, B. A., Gay, R. E., Sprott, H., et al. (2005). Expression of interleukin-21 receptor in epidermis from patients with systemic sclerosis. Arthritis Rheum. 52, 856–864. doi: 10.1002/art.20883
Djokic, V., Akoolo, L., and Parveen, N. (2018). Babesia microti Infection changes host spleen architecture and is cleared by a Th1 immune response. Front. Microbiol. 9:85. doi: 10.3389/fmicb.2018.00085
Dong, C., Juedes, A. E., Temann, U. A., Shresta, S., Allison, J. P., Ruddle, N. H., et al. (2001). ICOS co-stimulatory receptor is essential for T-cell activation and function. Nature 409, 97–101. doi: 10.1038/35051100
Duleu, S., Vincendeau, P., Courtois, P., Semballa, S., Lagroye, I., Daulouede, S., et al. (2004). Mouse strain susceptibility to trypanosome infection: an arginase-dependent effect. J. Immunol. 172, 6298–6303. doi: 10.4049/jimmunol.172.10.6298
Dunst, J., Kamena, F., and Matuschewski, K. (2017). Cytokines and chemokines in cerebral malaria pathogenesis. Front. Cell. Infect. Microbiol. 7:324. doi: 10.3389/fcimb.2017.00324
Espitia, C. M., Zhao, W., Saldarriaga, O., Osorio, Y., Harrison, L. M., Cappello, M., et al. (2010). Duplex real-time reverse transcriptase PCR to determine cytokine mRNA expression in a hamster model of New World cutaneous leishmaniasis. BMC Immunol. 11:31. doi: 10.1186/1471-2172-11-31
Fairfax, K. C., Everts, B., Amiel, E., Smith, A. M., Schramm, G., Haas, H., et al. (2015). IL-4-secreting secondary T follicular helper (Tfh) cells arise from memory T cells, not persisting Tfh cells, through a B cell-dependent mechanism. J. Immunol. 194, 2999–3010. doi: 10.4049/jimmunol.1401225
Fauconnier, M., Palomo, J., Bourigault, M. L., Meme, S., Szeremeta, F., Beloeil, J. C., et al. (2012). IL-12Rbeta2 is essential for the development of experimental cerebral malaria. J. Immunol. 188, 1905–1914. doi: 10.4049/jimmunol.1101978
Ferreira, R. R., Abreu, R. D. S., Vilar-Pereira, G., Degrave, W., Meuser-Batista, M., Ferreira, N. V. C., et al. (2019). TGF-beta inhibitor therapy decreases fibrosis and stimulates cardiac improvement in a pre-clinical study of chronic Chagas' heart disease. PLoS Negl. Trop. Dis. 13:e0007602. doi: 10.1371/journal.pntd.0007602
Figueiredo, M. M., Costa, P. A. C., Diniz, S. Q., Henriques, P. M., Kano, F. S., Tada, M. S., et al. (2017). T follicular helper cells regulate the activation of B lymphocytes and antibody production during Plasmodium vivax infection. PLoS Pathog. 13:e1006484. doi: 10.1371/journal.ppat.1006484
Fina, D., Sarra, M., Caruso, R., Del Vecchio Blanco, G., Pallone, F., MacDonald, T. T., et al. (2008). Interleukin 21 contributes to the mucosal T helper cell type 1 response in coeliac disease. Gut 57, 887–892. doi: 10.1136/gut.2007.129882
Frohlich, A., Marsland, B. J., Sonderegger, I., Kurrer, M., Hodge, M. R., Harris, N. L., et al. (2007). IL-21 receptor signaling is integral to the development of Th2 effector responses in vivo. Blood 109, 2023–2031. doi: 10.1182/blood-2006-05-021600
Gensous, N., Charrier, M., Duluc, D., Contin-Bordes, C., Truchetet, M. E., Lazaro, E., et al. (2018). T follicular helper cells in autoimmune disorders. Front. Immunol. 9:1637. doi: 10.3389/fimmu.2018.01637
Gibson-Corley, K. N., Boggiatto, P. M., Bockenstedt, M. M., Petersen, C. A., Waldschmidt, T. J., and Jones, D. E. (2012). Promotion of a functional B cell germinal center response after Leishmania species co-infection is associated with lesion resolution. Am. J. Pathol. 180, 2009–2017. doi: 10.1016/j.ajpath.2012.01.012
Gomez-Samblas, M., Bernal, D., Bolado-Ortiz, A., Vilchez, S., Bolas-Fernandez, F., Espino, A. M., et al. (2018). Intraperitoneal administration of the anti-IL-23 antibody prevents the establishment of intestinal nematodes in mice. Sci. Rep. 8:7787. doi: 10.1038/s41598-018-26194-x
Gonzalez, D. G., Cote, C. M., Patel, J. R., Smith, C. B., Zhang, Y., Nickerson, K. M., et al. (2018). Nonredundant roles of IL-21 and IL-4 in the phased initiation of germinal center B cells and subsequent self-renewal transitions. J. Immunol. 201, 3569–3579. doi: 10.4049/jimmunol.1500497
Gorosito Serran, M., Tosello Boari, J., Fiocca Vernengo, F., Beccaria, C. G., Ramello, M. C., Bermejo, D. A., et al. (2017). Unconventional pro-inflammatory CD4(+) T cell response in B cell-deficient mice infected with Trypanosoma cruzi. Front. Immunol. 8:1548. doi: 10.3389/fimmu.2017.01548
Gringhuis, S. I., Kaptein, T. M., Wevers, B. A., van der Vlist, M., Klaver, E. J., van Die, I., et al. (2014). Fucose-based PAMPs prime dendritic cells for follicular T helper cell polarization via DC-SIGN-dependent IL-27 production. Nat. Commun. 5:5074. doi: 10.1038/ncomms6074
Guo, S., Cobb, D., and Smeltz, R. B. (2009). T-bet inhibits the in vivo differentiation of parasite-specific CD4+ Th17 cells in a T cell-intrinsic manner. J. Immunol. 182, 6179–6186. doi: 10.4049/jimmunol.0803821
Hernandez-Valladares, M., Naessens, J., Musoke, A. J., Sekikawa, K., Rihet, P., Ole-Moiyoi, O. K., et al. (2006). Pathology of Tnf-deficient mice infected with Plasmodium chabaudi adami 408XZ. Exp. Parasitol. 114, 271–278. doi: 10.1016/j.exppara.2006.04.003
Hiramatsu, Y., Suto, A., Kashiwakuma, D., Kanari, H., Kagami, S., Ikeda, K., et al. (2010). c-Maf activates the promoter and enhancer of the IL-21 gene, and TGF-beta inhibits c-Maf-induced IL-21 production in CD4+ T cells. J. Leukoc. Biol. 87, 703–712. doi: 10.1189/jlb.0909639
Hollister, K., Kusam, S., Wu, H., Clegg, N., Mondal, A., Sawant, D. V., et al. (2013). Insights into the role of Bcl6 in follicular Th cells using a new conditional mutant mouse model. J. Immunol. 191, 3705–3711. doi: 10.4049/jimmunol.1300378
Huber, M., Brustle, A., Reinhard, K., Guralnik, A., Walter, G., Mahiny, A., et al. (2008). IRF4 is essential for IL-21-mediated induction, amplification, and stabilization of the Th17 phenotype. Proc. Natl. Acad. Sci.U.S.A. 105, 20846–20851. doi: 10.1073/pnas.0809077106
Hurdayal, R., Nieuwenhuizen, N. E., Revaz-Breton, M., Smith, L., Hoving, J. C., Parihar, S. P., et al. (2013). Deletion of IL-4 receptor alpha on dendritic cells renders BALB/c mice hypersusceptible to Leishmania major infection. PLoS Pathog. 9:e1003699. doi: 10.1371/journal.ppat.1003699
Hutloff, A., Dittrich, A. M., Beier, K. C., Eljaschewitsch, B., Kraft, R., Anagnostopoulos, I., et al. (1999). ICOS is an inducible T-cell co-stimulator structurally and functionally related to CD28. Nature 397, 263–266. doi: 10.1038/16717
Inoue, S. I., Niikura, M., Asahi, H., Kawakami, Y., and Kobayashi, F. (2018). gammadelta T cells modulate humoral immunity against Plasmodium berghei infection. Immunology 155, 519–532. doi: 10.1111/imm.12997
Ishii, A. I., and Sano, M. (1989). Strain-dependent differences in susceptibility of mice to experimental Angiostrongylus costaricensis infection. J. Helminthol. 63, 302–306. doi: 10.1017/S0022149X00009196
Ivanova, D. L., Denton, S. L., Fettel, K. D., Sondgeroth, K. S., Munoz Gutierrez, J., Bangoura, B., et al. (2019). Innate lymphoid cells in protection, pathology, and adaptive immunity during apicomplexan infection. Front. Immunol. 10:196. doi: 10.3389/fimmu.2019.00196
Jordan, S. J., Branch, O. H., Castro, J. C., Oster, R. A., and Rayner, J. C. (2009). Genetic diversity of the malaria vaccine candidate Plasmodium falciparum merozoite surface protein-3 in a hypoendemic transmission environment. Am. J. Trop. Med. Hyg. 80, 479–486. doi: 10.4269/ajtmh.2009.80.479
Kaur, H., Sehgal, R., Goyal, K., Makkar, N., Yadav, R., Bharti, P. K., et al. (2017). Genetic diversity of Plasmodium falciparum merozoite surface protein-1 (block 2), glutamate-rich protein and sexual stage antigen Pfs25 from Chandigarh, North India. Trop. Med. Int. Health. 22, 1590–1598. doi: 10.1111/tmi.12990
Khatonier, R., Khan, A. M., Sarmah, P., and Ahmed, G. U. (2018). Role of IL-21 in host pathogenesis in experimental visceral leishmaniasis. J. Parasit. Dis. 42, 500–504. doi: 10.1007/s12639-018-1025-8
King, I. L., Mohrs, K., and Mohrs, M. (2010). A nonredundant role for IL-21 receptor signaling in plasma cell differentiation and protective type 2 immunity against gastrointestinal helminth infection. J. Immunol. 185, 6138–6145. doi: 10.4049/jimmunol.1001703
Kishida, T., Asada, H., Itokawa, Y., Cui, F. D., Shin-Ya, M., Gojo, S., et al. (2003). Interleukin (IL)-21 and IL-15 genetic transfer synergistically augments therapeutic antitumor immunity and promotes regression of metastatic lymphoma. Mol. Ther. 8, 552–558. doi: 10.1016/S1525-0016(03)00222-3
Kong, F., Saldarriaga, O. A., Spratt, H., Osorio, E. Y., Travi, B. L., Luxon, B. A., et al. (2017). Transcriptional profiling in experimental visceral leishmaniasis reveals a broad splenic inflammatory environment that conditions macrophages toward a disease-promoting phenotype. PLoS Pathog. 13:e1006165. doi: 10.1371/journal.ppat.1006165
Korner, H., McMorran, B., Schluter, D., and Fromm, P. (2010). The role of TNF in parasitic diseases: still more questions than answers. Int. J. Parasitol. 40, 879–888. doi: 10.1016/j.ijpara.2010.03.011
Kroenke, M. A., Eto, D., Locci, M., Cho, M., Davidson, T., Haddad, E. K., et al. (2012). Bcl6 and Maf cooperate to instruct human follicular helper CD4 T cell differentiation. J. Immunol. 188, 3734–3744. doi: 10.4049/jimmunol.1103246
Labsi, M., Soufli, I., Khelifi, L., Amir, Z. C., and Touil-Boukoffa, C. (2018). In vivo treatment with IL-17A attenuates hydatid cyst growth and liver fibrogenesis in an experimental model of echinococcosis. Acta Trop. 181, 6–10. doi: 10.1016/j.actatropica.2018.01.014
Lanocha-Arendarczyk, N., Kolasa-Wolosiuk, A., Wojciechowska-Koszko, I., Kot, K., Roszkowska, P., Krasnodebska-Szponder, B., et al. (2018). Changes in the immune system in experimental acanthamoebiasis in immunocompetent and immunosuppressed hosts. Parasit. Vectors 11:517. doi: 10.1186/s13071-018-3108-x
Li, Z. Y., Chen, J., Petersen, E., Zhou, D. H., Huang, S. Y., Song, H. Q., et al. (2014). Synergy of mIL-21 and mIL-15 in enhancing DNA vaccine efficacy against acute and chronic Toxoplasma gondii infection in mice. Vaccine 32, 3058–3065. doi: 10.1016/j.vaccine.2014.03.042
Liang, H. E., Reinhardt, R. L., Bando, J. K., Sullivan, B. M., Ho, I. C., and Locksley, R. M. (2011). Divergent expression patterns of IL-4 and IL-13 define unique functions in allergic immunity. Nat. Immunol. 13, 58–66. doi: 10.1038/ni.2182
Linterman, M. A., Beaton, L., Yu, D., Ramiscal, R. R., Srivastava, M., Hogan, J. J., et al. (2010). IL-21 acts directly on B cells to regulate Bcl-6 expression and germinal center responses. J. Exp. Med. 207, 353–363. doi: 10.1084/jem.20091738
Linterman, M. A., Pierson, W., Lee, S. K., Kallies, A., Kawamoto, S., Rayner, T. F., et al. (2011). Foxp3+ follicular regulatory T cells control the germinal center response. Nat. Med. 17, 975–982. doi: 10.1038/nm.2425
Lohoff, M., Ferrick, D., Mittrucker, H. W., Duncan, G. S., Bischof, S., Rollinghoff, M., et al. (1997). Interferon regulatory factor-1 is required for a T helper 1 immune response in vivo. Immunity 6, 681–689. doi: 10.1016/S1074-7613(00)80444-6
Lohoff, M., and Mak, T. W. (2005). Roles of interferon-regulatory factors in T-helper-cell differentiation. Nat. Rev. Immunol. 5, 125–135. doi: 10.1038/nri1552
Lohoff, M., Sommer, F., Solbach, W., and Rollinghoff, M. (1989). Coexistence of antigen-specific TH1 and TH2 cells in genetically susceptible BALB/c mice infected with Leishmania major. Immunobiology 179, 412–21. doi: 10.1016/S0171-2985(89)80045-2
Lusty, E., Poznanski, S. M., Kwofie, K., Mandur, T. S., Lee, D. A., Richards, C. D., et al. (2017). IL-18/IL-15/IL-12 synergy induces elevated and prolonged IFN-gamma production by ex vivo expanded NK cells which is not due to enhanced STAT4 activation. Mol. Immunol. 88, 138–147. doi: 10.1016/j.molimm.2017.06.025
Ma'ayeh, S. Y., Knorr, L., Skold, K., Garnham, A., Ansell, B. R. E., Jex, A. R., et al. (2018). Responses of the differentiated intestinal epithelial cell line Caco-2 to Infection with the Giardia intestinalis GS isolate. Front. Cell. Infect. Microbiol. 8:244. doi: 10.3389/fcimb.2018.00297
Mackey, T. K., Liang, B. A., Cuomo, R., Hafen, R., Brouwer, K. C., and Lee, D. E. (2014). Emerging and reemerging neglected tropical diseases: a review of key characteristics, risk factors, and the policy and innovation environment. Clin. Microbiol. Rev. 27, 949–979. doi: 10.1128/CMR.00045-14
M'Bondoukwe, N. P., Kendjo, E., Mawili-Mboumba, D. P., Koumba Lengongo, J. V., Offouga Mbouoronde, C., Nkoghe, D., et al. (2018). Prevalence of and risk factors for malaria, filariasis, and intestinal parasites as single infections or co-infections in different settlements of Gabon, Central Africa. Infect. Dis. Poverty 7:6. doi: 10.1186/s40249-017-0381-4
Meamar, A. R., Rezaian, M., Rezaie, S., Mohraz, M., Kia, E. B., Houpt, E. R., et al. (2006). Cryptosporidium parvum bovine genotype oocysts in the respiratory samples of an AIDS patient: efficacy of treatment with a combination of azithromycin and paromomycin. Parasitol. Res. 98, 593–595. doi: 10.1007/s00436-005-0097-4
Mentink-Kane, M. M., and Wynn, T. A. (2004). Opposing roles for IL-13 and IL-13 receptor alpha 2 in health and disease. Immunol. Rev. 202, 191–202. doi: 10.1111/j.0105-2896.2004.00210.x
Mesin, L., Ersching, J., and Victora, G. D. (2016). Germinal center B cell dynamics. Immunity 45, 471–482. doi: 10.1016/j.immuni.2016.09.001
Mewono, L., Agnandji, S. T., Matondo Maya, D. W., Mouima, A. M., Iroungou, B. A., Issifou, S., et al. (2009). Malaria antigen-mediated enhancement of interleukin-21 responses of peripheral blood mononuclear cells in African adults. Exp. Parasitol. 122, 37–40. doi: 10.1016/j.exppara.2009.01.007
Mewono, L., Matondo Maya, D. W., Matsiegui, P. B., Agnandji, S. T., Kendjo, E., Barondi, F., et al. (2008). Interleukin-21 is associated with IgG1 and IgG3 antibodies to erythrocyte-binding antigen-175 peptide 4 of Plasmodium falciparum in Gabonese children with acute falciparum malaria. Eur. Cytokine Netw. 19, 30–36. doi: 10.1684/ecn.2008.0114.
Michailowsky, V., Silva, N. M., Rocha, C. D., Vieira, L. Q., Lannes-Vieira, J., and Gazzinelli, R. T. (2001). Pivotal role of interleukin-12 and interferon-gamma axis in controlling tissue parasitism and inflammation in the heart and central nervous system during Trypanosoma cruzi infection. Am. J. Pathol. 159, 1723–1733. doi: 10.1016/S0002-9440(10)63019-2
Milner, T., Reilly, L., Nausch, N., Midzi, N., Mduluza, T., Maizels, R., et al. (2010). Circulating cytokine levels and antibody responses to human Schistosoma haematobium: IL-5 and IL-10 levels depend upon age and infection status. Parasite Immunol. 32, 710–21. doi: 10.1111/j.1365-3024.2010.01235.x
Miu, J., Mitchell, A. J., Muller, M., Carter, S. L., Manders, P. M., McQuillan, J. A., et al. (2008). Chemokine gene expression during fatal murine cerebral malaria and protection due to CXCR3 deficiency. J. Immunol. 180, 1217–1230. doi: 10.4049/jimmunol.180.2.1217
Miyazaki, Y., Hamano, S., Wang, S., Shimanoe, Y., Iwakura, Y., and Yoshida, H. (2010). IL-17 is necessary for host protection against acute-phase Trypanosoma cruzi infection. J. Immunol. 185, 1150–1157. doi: 10.4049/jimmunol.0900047
Moncunill, G., De Rosa, S. C., Ayestaran, A., Nhabomba, A. J., Mpina, M., Cohen, K. W., et al. (2017). RTS,S/AS01E malaria vaccine induces memory and polyfunctional T cell responses in a pediatric african phase III trial. Front. Immunol. 8:1008. doi: 10.3389/fimmu.2017.01008
Monteleone, G., Monteleone, I., Fina, D., Vavassori, P., Del Vecchio Blanco, G., Caruso, R., et al. (2005). Interleukin-21 enhances T-helper cell type I signaling and interferon-gamma production in Crohn's disease. Gastroenterology 128, 687–694. doi: 10.1053/j.gastro.2004.12.042
Moretto, M. M., Hwang, S., and Khan, I. A. (2017). Downregulated IL-21 response and T follicular helper cell exhaustion correlate with compromised CD8 T cell immunity during chronic toxoplasmosis. Front. Immunol. 8:1436. doi: 10.3389/fimmu.2017.01436
Moretto, M. M., and Khan, I. A. (2016). IL-21 Is Important for Induction of KLRG1+ effector CD8 T cells during acute intracellular infection. J. Immunol. 196, 375–384. doi: 10.4049/jimmunol.1501258
Nair, S. S., Bommana, A., Pakala, S. B., Ohshiro, K., Lyon, A. J., Suttiprapa, S., et al. (2011). Inflammatory response to liver fluke Opisthorchis viverrini in mice depends on host master coregulator MTA1, a marker for parasite-induced cholangiocarcinoma in humans. Hepatology 54, 1388–1397. doi: 10.1002/hep.24518
Naradikian, M. S., Myles, A., Beiting, D. P., Roberts, K. J., Dawson, L., Herati, R. S., et al. (2016). Cutting edge: IL-4, IL-21, and IFN-gamma interact to govern T-bet and CD11c expression in TLR-activated B cells. J. Immunol. 197, 1023–1028. doi: 10.4049/jimmunol.1600522
Natale, M. A., Cesar, G., Alvarez, M. G., Castro Eiro, M. D., Lococo, B., Bertocchi, G., et al. (2018). Trypanosoma cruzi-specific IFN-gamma-producing cells in chronic Chagas disease associate with a functional IL-7/IL-7R axis. PLoS Negl. Trop. Dis. 12:e0006998. doi: 10.1371/journal.pntd.0006998
Nigg, A. P., Zahn, S., Ruckerl, D., Holscher, C., Yoshimoto, T., Ehrchen, J. M., et al. (2007). Dendritic cell-derived IL-12p40 homodimer contributes to susceptibility in cutaneous leishmaniasis in BALB/c mice. J. Immunol. 178, 7251–7258. doi: 10.4049/jimmunol.178.11.7251
Nurieva, R. I., Chung, Y., Martinez, G. J., Yang, X. O., Tanaka, S., Matskevitch, T. D., et al. (2009). Bcl6 mediates the development of T follicular helper cells. Science 325, 1001–1005. doi: 10.1126/science.1176676
Nurieva, R. I., Treuting, P., Duong, J., Flavell, R. A., and Dong, C. (2003). Inducible costimulator is essential for collagen-induced arthritis. J. Clin. Invest. 111, 701–706. doi: 10.1172/JCI17321
Oeuvray, C., Bouharoun-Tayoun, H., Gras-Masse, H., Bottius, E., Kaidoh, T., Aikawa, M., et al. (1994b). Merozoite surface protein-3: a malaria protein inducing antibodies that promote Plasmodium falciparum killing by cooperation with blood monocytes. Blood 84, 1594–1602. doi: 10.1182/blood.V84.5.1594.1594
Oeuvray, C., Bouharoun-Tayoun, H., Grass-Masse, H., Lepers, J. P., Ralamboranto, L., Tartar, A., et al. (1994a). A novel merozoite surface antigen of Plasmodium falciparum (MSP-3) identified by cellular-antibody cooperative mechanism antigenicity and biological activity of antibodies. Mem. Inst. Oswaldo Cruz. 89 (Suppl. 2), 77–80. doi: 10.1590/S0074-02761994000600018
Okhuysen, P. C. (2001). Traveler's diarrhea due to intestinal protozoa. Clin. Infect. Dis. 33, 110–114. doi: 10.1086/320894
Oyegue-Liabagui, S. L., Bouopda-Tuedom, A. G., Kouna, L. C., Maghendji-Nzondo, S., Nzoughe, H., Tchitoula-Makaya, N., et al. (2017). Pro- and anti-inflammatory cytokines in children with malaria in Franceville, Gabon. Am. J. Clin. Exp. Immunol. 6, 9–20.
Ozaki, K., Kikly, K., Michalovich, D., Young, P. R., and Leonard, W. J. (2000). Cloning of a type I cytokine receptor most related to the IL-2 receptor beta chain. Proc. Natl. Acad. Sci. U.S.A. 97, 11439–11444. doi: 10.1073/pnas.200360997
Ozaki, K., Spolski, R., Feng, C. G., Qi, C. F., Cheng, J., Sher, A., et al. (2002). A critical role for IL-21 in regulating immunoglobulin production. Science 298, 1630–1634. doi: 10.1126/science.1077002
Pene, J., Gauchat, J. F., Lecart, S., Drouet, E., Guglielmi, P., Boulay, V., et al. (2004). Cutting edge: IL-21 is a switch factor for the production of IgG1 and IgG3 by human B cells. J. Immunol. 172, 5154–5157. doi: 10.4049/jimmunol.172.9.5154
Perera, M. K., Herath, N. P., Pathirana, S. L., Phone-Kyaw, M., Alles, H. K., Mendis, K. N., et al. (2013). Association of high plasma TNF-alpha levels and TNF-alpha/IL-10 ratios with TNF2 allele in severe P. falciparum malaria patients in Sri Lanka. Pathog. Glob. Health 107, 21–29. doi: 10.1179/2047773212Y.0000000069
Perez-Mazliah, D., Ng, D. H., Freitas do Rosario, A. P., McLaughlin, S., Mastelic-Gavillet, B., Sodenkamp, J., et al. (2015). Disruption of IL-21 signaling affects T cell-B cell interactions and abrogates protective humoral immunity to malaria. PLoS Pathog. 11:e1004715. doi: 10.1371/journal.ppat.1004715
Pesce, J., Kaviratne, M., Ramalingam, T. R., Thompson, R. W., Urban, J. F. Jr., Cheever, A. W., et al. (2006). The IL-21 receptor augments Th2 effector function and alternative macrophage activation. J. Clin. Invest. 116, 2044–2055. doi: 10.1172/JCI27727
Phillips, S. M., and Fox, E. G. (1984). Immunopathology of parasitic diseases: a conceptual approach. Contemp. Top. Immunobiol. 12, 421–461. doi: 10.1007/978-1-4684-4571-8_11
Pitzalis, C., Jones, G. W., Bombardieri, M., and Jones, S. A. (2014). Ectopic lymphoid-like structures in infection, cancer and autoimmunity. Nat. Rev. Immunol. 14, 447–462. doi: 10.1038/nri3700
Pober, J. S., and Sessa, W. C. (2007). Evolving functions of endothelial cells in inflammation. Nat. Rev. Immunol. 7, 803–815. doi: 10.1038/nri2171
Poovassery, J. S., Sarr, D., Smith, G., Nagy, T., and Moore, J. M. (2009). Malaria-induced murine pregnancy failure: distinct roles for IFN-gamma and TNF. J. Immunol. 183, 5342–5349. doi: 10.4049/jimmunol.0901669
Pot, C., Jin, H., Awasthi, A., Liu, S. M., Lai, C. Y., Madan, R., et al. (2009). Cutting edge: IL-27 induces the transcription factor c-Maf, cytokine IL-21, and the costimulatory receptor ICOS that coordinately act together to promote differentiation of IL-10-producing Tr1 cells. J. Immunol. 183, 797–801. doi: 10.4049/jimmunol.0901233
Rankin, A. L., MacLeod, H., Keegan, S., Andreyeva, T., Lowe, L., Bloom, L., et al. (2011). IL-21 receptor is critical for the development of memory B cell responses. J. Immunol. 186, 667–674. doi: 10.4049/jimmunol.0903207
Riley, E. M., Couper, K. N., Helmby, H., Hafalla, J. C., de Souza, J. B., Langhorne, J., et al. (2010). Neuropathogenesis of human and murine malaria. Trends Parasitol. 26, 277–278. doi: 10.1016/j.pt.2010.03.002
Ritter, M., Krupp, V., Wiszniewsky, K., Wiszniewsky, A., Katawa, G., Tamadaho, R. S. E., et al. (2018). Absence of IL-17A in Litomosoides sigmodontis-infected mice influences worm development and drives elevated filarial-specific IFN-gamma. Parasitol. Res. 117, 2665–2675. doi: 10.1007/s00436-018-5959-7
Rosas, L. E., Barbi, J., Lu, B., Fujiwara, Y., Gerard, C., Sanders, V. M., et al. (2005). CXCR3-/- mice mount an efficient Th1 response but fail to control Leishmania major infection. Eur. J. Immunol. 35, 515–523. doi: 10.1002/eji.200425422
Roussilhon, C., Oeuvray, C., Muller-Graf, C., Tall, A., Rogier, C., Trape, J. F., et al. (2007). Long-term clinical protection from falciparum malaria is strongly associated with IgG3 antibodies to merozoite surface protein 3. PLoS Med. 4:e320. doi: 10.1371/journal.pmed.0040320
Rudin, W., Eugster, H. P., Bordmann, G., Bonato, J., Muller, M., Yamage, M., et al. (1997). Resistance to cerebral malaria in tumor necrosis factor-alpha/beta-deficient mice is associated with a reduction of intercellular adhesion molecule-1 up-regulation and T helper type 1 response. Am. J. Pathol. 150, 257–266.
Ruebush, M. J., and Hanson, W. L. (1979). Susceptibility of five strains of mice to Babesia microti of human origin. J. Parasitol. 65, 430–433. doi: 10.2307/3280290
Ryg-Cornejo, V., Ioannidis, L. J., Ly, A., Chiu, C. Y., Tellier, J., Hill, D. L., et al. (2016). Severe malaria infections impair germinal center responses by inhibiting T follicular helper cell differentiation. Cell Rep. 14, 68–81. doi: 10.1016/j.celrep.2015.12.006
Saporito, L., Giammanco, G. M., De Grazia, S., and Colomba, C. (2013). Visceral leishmaniasis: host-parasite interactions and clinical presentation in the immunocompetent and in the immunocompromised host. Int. J. Infect. Dis. 17, e572–e576. doi: 10.1016/j.ijid.2012.12.024
Sarra, M., Monteleone, I., Stolfi, C., Fantini, M. C., Sileri, P., Sica, G., et al. (2010). Interferon-gamma-expressing cells are a major source of interleukin-21 in inflammatory bowel diseases. Inflamm. Bowel Dis. 16, 1332–1339. doi: 10.1002/ibd.21238
Sassi, A., Kaak, O., and Ben Ammar Elgaied, A. (2015). Identification of immunodominant Leishmania major antigenic markers of the early C57BL/6 and BALB/c mice infection stages. Parasite Immunol. 37, 544–552. doi: 10.1111/pim.12223
Scott, P. (1991). IFN-gamma modulates the early development of Th1 and Th2 responses in a murine model of cutaneous leishmaniasis. J. Immunol. 147, 3149–3155.
Sebina, I., Fogg, L. G., James, K. R., Soon, M. S. F., Akter, J., Thomas, B. S., et al. (2017). IL-6 promotes CD4(+) T-cell and B-cell activation during Plasmodium infection. Parasite Immunol. 39:e12455. doi: 10.1111/pim.12455
Sellge, G., Magalhaes, J. G., Konradt, C., Fritz, J. H., Salgado-Pabon, W., Eberl, G., et al. (2010). Th17 cells are the dominant T cell subtype primed by Shigella flexneri mediating protective immunity. J. Immunol. 184, 2076–2085. doi: 10.4049/jimmunol.0900978
Shainheit, M. G., Smith, P. M., Bazzone, L. E., Wang, A. C., Rutitzky, L. I., and Stadecker, M. J. (2008). Dendritic cell IL-23 and IL-1 production in response to schistosome eggs induces Th17 cells in a mouse strain prone to severe immunopathology. J. Immunol. 181, 8559–8567. doi: 10.4049/jimmunol.181.12.8559
Silva, J. S., Vespa, G. N., Cardoso, M. A., Aliberti, J. C., and Cunha, F. Q. (1995). Tumor necrosis factor alpha mediates resistance to Trypanosoma cruzi infection in mice by inducing nitric oxide production in infected gamma interferon-activated macrophages. Infect. Immun. 63, 4862–4867.
Sim, B. K. (1990). Sequence conservation of a functional domain of erythrocyte binding antigen 175 in Plasmodium falciparum. Mol. Biochem. Parasitol. 41, 293–295. doi: 10.1016/0166-6851(90)90193-P
Sim, B. K., Orlandi, P. A., Haynes, J. D., Klotz, F. W., Carter, J. M., Camus, D., et al. (1990). Primary structure of the 175K Plasmodium falciparum erythrocyte binding antigen and identification of a peptide which elicits antibodies that inhibit malaria merozoite invasion. J. Cell Biol. 111(5 Pt 1), 1877–1884. doi: 10.1083/jcb.111.5.1877
Solaymani-Mohammadi, S., and Berzofsky, J. A. (2019). Interleukin 21 collaborates with interferon-gamma for the optimal expression of interferon-stimulated genes and enhances protection against enteric microbial infection. PLoS Pathog. 15:e1007614. doi: 10.1371/journal.ppat.1007614
Solaymani-Mohammadi, S., and Singer, S. M. (2010). Giardia duodenalis: the double-edged sword of immune responses in giardiasis. Exp. Parasitol. 126, 292–297. doi: 10.1016/j.exppara.2010.06.014
Solaymani-Mohammadi, S., and Singer, S. M. (2013). Regulation of intestinal epithelial cell cytoskeletal remodeling by cellular immunity following gut infection. Mucosal Immunol. 6, 369–378. doi: 10.1038/mi.2012.80
Sommer, F., Meixner, M., Mannherz, M., Ogilvie, A. L., Rollinghoff, M., and Lohoff, M. (1998). Analysis of cytokine patterns produced by individual CD4+ lymph node cells during experimental murine leishmaniasis in resistant and susceptible mice. Int. Immunol. 10, 1853–1861. doi: 10.1093/intimm/10.12.1853
Spolski, R., and Leonard, W. J. (2008). Interleukin-21: basic biology and implications for cancer and autoimmunity. Annu. Rev. Immunol. 26, 57–79. doi: 10.1146/annurev.immunol.26.021607.090316
Spolski, R., and Leonard, W. J. (2010a). IL-21 is an immune activator that also mediates suppression via IL-10. Crit. Rev. Immunol. 30, 559–570. doi: 10.1615/CritRevImmunol.v30.i6.50
Spolski, R., and Leonard, W. J. (2010b). IL-21 and T follicular helper cells. Int. Immunol. 22, 7–12. doi: 10.1093/intimm/dxp112
Spolski, R., and Leonard, W. J. (2014). Interleukin-21: a double-edged sword with therapeutic potential. Nat. Rev. Drug Discov. 13, 379–395. doi: 10.1038/nrd4296
Spolski, R., West, E. E., Li, P., Veenbergen, S., Yung, S., Kazemian, M., et al. (2019). IL-21/type I interferon interplay regulates neutrophil-dependent innate immune responses to Staphylococcus aureus. Elife 8:e45501. doi: 10.7554/eLife.45501
Sripa, B., Jumnainsong, A., Tangkawattana, S., and Haswell, M. R. (2018). Immune response to Opisthorchis viverrini infection and its role in pathology. Adv. Parasitol. 102, 73–95. doi: 10.1016/bs.apar.2018.08.003
Stevenson, M. M., Gros, P., Olivier, M., Fortin, A., and Serghides, L. (2010). Cerebral malaria: human versus mouse studies. Trends Parasitol. 26, 274–275. doi: 10.1016/j.pt.2010.03.008
Stolfi, C., Rizzo, A., Franze, E., Rotondi, A., Fantini, M. C., Sarra, M., et al. (2011). Involvement of interleukin-21 in the regulation of colitis-associated colon cancer. J. Exp. Med. 208, 2279–2290. doi: 10.1084/jem.20111106
Strengell, M., Matikainen, S., Siren, J., Lehtonen, A., Foster, D., Julkunen, I., et al. (2003). IL-21 in synergy with IL-15 or IL-18 enhances IFN-gamma production in human NK and T cells. J. Immunol. 170, 5464–5469. doi: 10.4049/jimmunol.170.11.5464
Strengell, M., Sareneva, T., Foster, D., Julkunen, I., and Matikainen, S. (2002). IL-21 up-regulates the expression of genes associated with innate immunity and Th1 response. J. Immunol. 169, 3600–3605. doi: 10.4049/jimmunol.169.7.3600
Stumhofer, J. S., Silver, J. S., and Hunter, C. A. (2013). IL-21 is required for optimal antibody production and T cell responses during chronic Toxoplasma gondii infection. PLoS ONE 8:e62889. doi: 10.1371/journal.pone.0062889
Taniguchi, T., Miyauchi, E., Nakamura, S., Hirai, M., Suzue, K., Imai, T., et al. (2015). Plasmodium berghei ANKA causes intestinal malaria associated with dysbiosis. Sci. Rep. 5:15699. doi: 10.1038/srep15699
Torben, W., Molehin, A. J., Blair, R. V., Kenway, C., Shiro, F., Roslyn, D., et al. (2017). The self-curing phenomenon of schistosome infection in rhesus macaques: insight from in vitro studies. Ann. N. Y. Acad. Sci. 1408, 79–89. doi: 10.1111/nyas.13565
Torgerson, P. R., de Silva, N. R., Fevre, E. M., Kasuga, F., Rokni, M. B., Zhou, X. N., et al. (2014). The global burden of foodborne parasitic diseases: an update. Trends Parasitol. 30, 20–26. doi: 10.1016/j.pt.2013.11.002
Torgerson, P. R., Devleesschauwer, B., Praet, N., Speybroeck, N., Willingham, A. L., Kasuga, F., et al. (2015). World Health Organization estimates of the global and regional disease burden of 11 foodborne parasitic diseases, 2010: a data synthesis. PLoS Med. 12:e1001920. doi: 10.1371/journal.pmed.1001920
Tortola, L., Pawelski, H., Sonar, S. S., Ampenberger, F., Kurrer, M., and Kopf, M. (2019). IL-21 promotes allergic airway inflammation by driving apoptosis of FoxP3(+) regulatory T cells. J. Allergy Clin. Immunol. 143, 2178–2189.e5. doi: 10.1016/j.jaci.2018.11.047
Vinuesa, C. G., Linterman, M. A., Yu, D., and MacLennan, I. C. (2016). Follicular helper T cells. Annu. Rev. Immunol. 34, 335–368. doi: 10.1146/annurev-immunol-041015-055605
von Stebut, E., Belkaid, Y., Jakob, T., Sacks, D. L., and Udey, M. C. (1998). Uptake of Leishmania major amastigotes results in activation and interleukin 12 release from murine skin-derived dendritic cells: implications for the initiation of anti-Leishmania immunity. J. Exp. Med. 188, 1547–1552. doi: 10.1084/jem.188.8.1547
von Stebut, E., Belkaid, Y., Nguyen, B. V., Cushing, M., Sacks, D. L., and Udey, M. C. (2000). Leishmania major-infected murine langerhans cell-like dendritic cells from susceptible mice release IL-12 after infection and vaccinate against experimental cutaneous Leishmaniasis. Eur. J. Immunol. 30, 3498–3506. doi: 10.1002/1521-4141(2000012)30:12<3498::AID-IMMU3498>3.0.CO;2-6
Von Stebut, E., Ehrchen, J. M., Belkaid, Y., Kostka, S. L., Molle, K., Knop, J., et al. (2003). Interleukin 1alpha promotes Th1 differentiation and inhibits disease progression in Leishmania major-susceptible BALB/c mice. J. Exp. Med. 198, 191–199. doi: 10.1084/jem.20030159
Wan, C. K., Andraski, A. B., Spolski, R., Li, P., Kazemian, M., Oh, J., et al. (2015). Opposing roles of STAT1 and STAT3 in IL-21 function in CD4+ T cells. Proc. Natl. Acad. Sci. U.S.A. 112, 9394–9399. doi: 10.1073/pnas.1511711112
Wan, C. K., Oh, J., Li, P., West, E. E., Wong, E. A., Andraski, A. B., et al. (2013). The cytokines IL-21 and GM-CSF have opposing regulatory roles in the apoptosis of conventional dendritic cells. Immunity 38, 514–527. doi: 10.1016/j.immuni.2013.02.011
Wang, B., Liang, S., Wang, Y., Zhu, X. Q., Gong, W., Zhang, H. Q., et al. (2015). Th17 down-regulation is involved in reduced progression of schistosomiasis fibrosis in ICOSL KO mice. PLoS Negl. Trop. Dis. 9:e0003434. doi: 10.1371/journal.pntd.0003434
Wang, S., Wang, J., Kumar, V., Karnell, J. L., Naiman, B., Gross, P. S., et al. (2018). IL-21 drives expansion and plasma cell differentiation of autoreactive CD11c(hi)T-bet(+) B cells in SLE. Nat. Commun. 9, 1758. doi: 10.1038/s41467-018-03750-7
Wang, Y., Jiang, X., Zhu, J., Dan, Y., Zhang, X., Wang, X., et al. (2016). IL-21/IL-21R signaling suppresses intestinal inflammation induced by DSS through regulation of Th responses in lamina propria in mice. Sci. Rep. 6:31881. doi: 10.1038/srep31881
Weaver, J. D., Hoffman, V. J., Roffe, E., and Murphy, P. M. (2019). Low-level parasite Persistence drives vasculitis and myositis in skeletal muscle of mice chronically infected with Trypanosoma cruzi. Infect. Immun. 87:e00081-19. doi: 10.1128/IAI.00081-19
Wikenheiser, D. J., Brown, S. L., Lee, J., and Stumhofer, J. S. (2018). NK1.1 expression defines a population of CD4(+) effector T cells displaying Th1 and Tfh Cell properties that support early antibody production during Plasmodium yoelii infection. Front. Immunol. 9:2277. doi: 10.3389/fimmu.2018.02277
Wynn, T. A. (2004). Fibrotic disease and the T(H)1/T(H)2 paradigm. Nat. Rev. Immunol. 4, 583–594. doi: 10.1038/nri1412
Wynn, T. A., Thompson, R. W., Cheever, A. W., and Mentink-Kane, M. M. (2004). Immunopathogenesis of schistosomiasis. Immunol. Rev. 201, 156–167. doi: 10.1111/j.0105-2896.2004.00176.x
Yi, W., Bao, W., Rodriguez, M., Liu, Y., Singh, M., Ramlall, V., et al. (2018). Robust adaptive immune response against Babesia microti infection marked by low parasitemia in a murine model of sickle cell disease. Blood Adv. 2, 3462–3478. doi: 10.1182/bloodadvances.2018026468
Yong, L., Tang, Y., Ren, C., Liu, M., Shen, J., and Hou, X. (2019). B1 cells protect against Schistosoma japonicum-induced liver inflammation and fibrosis by controlling monocyte infiltration. PLoS Negl. Trop. Dis. 13:e0007474. doi: 10.1371/journal.pntd.0007474
Zelante, T., De Luca, A., D'Angelo, C., Moretti, S., and Romani, L. (2009). IL-17/Th17 in anti-fungal immunity: what's new? Eur. J. Immunol. 39, 645–648. doi: 10.1002/eji.200839102
Zeng, R., Spolski, R., Finkelstein, S. E., Oh, S., Kovanen, P. E., Hinrichs, C. S., et al. (2005). Synergy of IL-21 and IL-15 in regulating CD8+ T cell expansion and function. J. Exp. Med. 201, 139–148. doi: 10.1084/jem.20041057
Zhang, C., Wang, L., Ali, T., Li, L., Bi, X., Wang, J., et al. (2016). Hydatid cyst fluid promotes peri-cystic fibrosis in cystic echinococcosis by suppressing miR-19 expression. Parasit. Vectors 9:278. doi: 10.1186/s13071-016-1562-x
Zhang, F., Pang, N., Zhu, Y., Zhou, D., Zhao, H., Hu, J., et al. (2015). CCR7(lo)PD-1(hi) CXCR5(+) CD4(+) T cells are positively correlated with levels of IL-21 in active and transitional cystic echinococcosis patients. BMC Infect. Dis. 15:457. doi: 10.1186/s12879-015-1156-9
Zhang, J., Gao, W., Guo, Q., Huang, B., Wang, B., Xia, G., et al. (2013). Helminth protein vaccine induced follicular T helper cell for enhancement of humoral immunity against Schistosoma japonicum. Biomed. Res. Int. 2013:798164. doi: 10.1155/2013/798164
Zhang, W., Ahmad, G., Molehin, A. J., Torben, W., Le, L., Kim, E., et al. (2018). Schistosoma mansoni antigen Sm-p80: prophylactic efficacy using TLR4 agonist vaccine adjuvant glucopyranosyl lipid A-Alum in murine and non-human primate models. J. Investig. Med. 66, 1124–1132. doi: 10.1136/jim-2018-000786
Zhang, Y., Wang, Y., Jiang, Y., Pan, W., Liu, H., Yin, J., et al. (2016). T follicular helper cells in patients with acute schistosomiasis. Parasit. Vectors 9:321. doi: 10.1186/s13071-016-1602-6
Keywords: interleukin (IL)-21, parasite, inflammation, immunity, cytokine, signaling
Citation: Solaymani-Mohammadi S, Eckmann L and Singer SM (2019) Interleukin (IL)-21 in Inflammation and Immunity During Parasitic Diseases. Front. Cell. Infect. Microbiol. 9:401. doi: 10.3389/fcimb.2019.00401
Received: 20 August 2019; Accepted: 11 November 2019;
Published: 04 December 2019.
Edited by:
Tiago W. P. Mineo, Federal University of Uberlandia, BrazilReviewed by:
Maria Del Consuelo Gomez, National Polytechnic Institute, MexicoDiego Luis Costa, National Institute of Allergy and Infectious Diseases (NIAID), United States
Copyright © 2019 Solaymani-Mohammadi, Eckmann and Singer. This is an open-access article distributed under the terms of the Creative Commons Attribution License (CC BY). The use, distribution or reproduction in other forums is permitted, provided the original author(s) and the copyright owner(s) are credited and that the original publication in this journal is cited, in accordance with accepted academic practice. No use, distribution or reproduction is permitted which does not comply with these terms.
*Correspondence: Shahram Solaymani-Mohammadi, c2hhaHJhbS5zb2xheW1hbmktbW9oYW1tYWRpJiN4MDAwNDA7c2VhdHRsZWNoaWxkcmVucy5vcmc=