- 1College of Animal Science and Veterinary Medicine, Henan Agricultural University, Zhengzhou, China
- 2International Joint Research Laboratory for Zoonotic Diseases of Henan, Zhengzhou, China
- 3Scientific Research Experiment Center & Laboratory Animal Center, Henan University of Chinese Medicine, Zhengzhou, China
Anaplasma capra is an emerging zoonotic tick-borne pathogen with a broad host range, including many mammals. Dogs have close physical interactions with humans and regular contact with the external environment. Moreover, they have been previously reported to be hosts of Anaplasma phagocytophilum, A. platys, A. ovis, and A. bovis. To confirm whether dogs are also hosts of A. capra, pathogen DNA was extracted from blood samples of 521 dogs, followed by PCR amplification of the citrate synthase (gltA) gene, heat shock protein (groEL) gene, and major surface protein 4 (msp4) gene of the A. capra. A total of 12.1% (63/521) of blood samples were shown to be A. capra-positive by PCR screening. No significant differences were observed between genders (P = 0.578) or types (P = 0.154) of dogs with A. capra infections. However, significantly higher A. capra infections occurred in dogs with regular contact with vegetation (P = 0.002), those aged over 10 years (P = 0.040), and during the summer season (P = 0.006). Phylogenetic analysis based on gltA, groEL, and msp4 sequences demonstrated that the isolates obtained in this study were clustered within the A. capra clade, and were distinct from other Anaplasma species. In conclusion, dogs were shown to be a host of the human pathogenic A. capra. Considering the affinity between dogs and humans and the zoonotic tick-borne nature of A. capra, dogs should be carefully monitored for the presence of A. capra.
Introduction
Vector-borne diseases are major causes of morbidity and mortality in dogs and are potentially of great public health importance because of their zoonotic nature and the role of pets as reservoirs (Xu et al., 2015; Cui et al., 2017; Maggi and Krämer, 2019). In the last few years, the number and range of species kept as companion animals have risen, and they are maintaining increasingly close interactions with humans in industrialized societies (Cito et al., 2016). Although the phenomenon of all companion animals, especially dogs and cats, sharing the same environment as humans is long-standing (Fang et al., 2015; Cui et al., 2017), urbanization has affected the emergence and increasing incidence of tick-borne diseases (TBD) (Fang et al., 2015). Together, these changes in human activity and the increased contact between humans, their pets, and nature have contributed to the rising abundance of tick exposure (Uspensky, 2014; Fang et al., 2015). Dogs are particularly unusual companion animals because of their outdoor nature and close relationship with humans. They can therefore act as good sentinels for human tick-borne infections, suggesting that their role as hosts of ticks and tick-borne pathogens should be monitored (Hornok et al., 2013; Vlahakis et al., 2018).
Anaplasma species are zoonotic pathogens with tick vectors and mammalian reservoir hosts (Li et al., 2015). To date, three Anaplasma species have been identified as human pathogens: A. phagocytophilum, A. ovis, and A. capra (Chen et al., 1994; Chochlakis et al., 2010; Li et al., 2015). A. phagocytophilum was first confirmed as the causative agent of human granulocytic anaplasmosis (HGA) in the USA in 1994 (Chen et al., 1994). In China, the first suspected human case was described in Anhui in 2006 (Zhang et al., 2008). The severity of HGA ranges from an asymptomatic infection to a mild or severe febrile illness with multiple organ failure or even death (Li et al., 2015). Besides humans, hosts of A. phagocytophilum include domestic and wild animals such as cattle, sheep, goats, horses, dogs, hares, yaks, and rodents (Fang et al., 2015). An A. ovis variant was first identified in a patient with fever, hepatosplenomegaly, and lymphadenopathy in Cyprus in 2007 (Chochlakis et al., 2010). Hosts of this pathogen include domestic goats, sheep, deer, wild boar, and domestic dogs (Yabsley et al., 2005; Aktas et al., 2010; Pereira et al., 2016; Cui et al., 2017; Wei et al., 2017). A. capra is a novel Anaplasma species so-called because it was originally found in asymptomatic goats; soon after, it was identified in 28 patients in Heilongjiang, China (Beyer and Carlyon, 2015; Li et al., 2015; Yang et al., 2018). Clinical features of A. capra infection in humans include the acute onset of fever, headache, and malaise, but it is very difficult to distinguish from other acute febrile illnesses, thus leading to misdiagnosis (Li et al., 2015). Subsequent reports have shown that A. capra is widely distributed throughout China (Yang et al., 2018). It appears to have a broad host range and genetic diversity, with other mammalian hosts including goats, sheep, cattle, deer, serows, takins, and Reeves' muntjacs worldwide (Sato et al., 2009; Sun et al., 2015; Yang et al., 2017, 2018; Peng et al., 2018; Seo et al., 2018).
As one of the main mammalian hosts of Anaplasma species, dogs have been shown to carry A. phagocytophilum, A. platys, A. ovis, and A. bovis pathogens in recent years in China (Zhang et al., 2012; Li et al., 2014; Cui et al., 2017). However, there are no reports about dogs as hosts of A. capra worldwide. To provide further information about the host range, clinical symptoms, and risk factors of A. capra infections, the detection of this pathogen was carried out in dogs in Henan Province, China.
Materials and Methods
Sample Collection
During 2013–2018, blood sample collection from dogs was carried out at six sampling sites in Zhengzhou city, Henan Province, central China. These included three pet hospitals (Pet clinic 1, Pet clinic 2, and Pet clinic 3) and three rescue centers for stray dogs (Stray dog rescue center 1, Stray dog rescue center 2, and Stray dog rescue center 3). Blood samples from pet clinics were obtained during outpatient testing, and detailed medical records were also collected. Blood samples from stray dog rescue centers were collected from a random proportion (5–10%) of dogs with the assistance of an experienced veterinarian. All blood samples were collected from the anterior tibial vein of the dogs with the help of a pet doctor or local veterinarian.
A total of 521 EDTA-K2 whole blood samples from different types of dogs were collected. Information about pet dogs, including age, gender, and clinical features, was obtained from sampling records, descriptions of chief complaints, and veterinarian diagnoses. However, few documents about stray dogs were available, and a review of their clinical symptoms suggested that they were healthy.
DNA Extraction
Pathogen genomic DNA was extracted from 200 μL blood samples using a Blood DNA Kit (Omega Biotek Inc., Norcross, GA, USA) according to the manufacturer's protocol. The quantity and quality of the extracted DNA were evaluated using a NanoDrop™ 2000 spectrophotometer (Thermo Fisher Scientific, Waltham, MA), and it was stored at −20°C before use.
PCR Amplification
DNA samples were screened for the presence of A. capra by PCR amplification of the citrate synthase gene (gltA), heat shock protein gene (groEL), and major surface protein 4 gene (msp4) using previously described primers and PCR conditions (Table 1). Each DNA sample was screened for all three A. capra genes, and the successful amplification of any one of the three genes was taken to indicate positive infection. Each PCR reaction was conducted at least twice using nuclease-free water as a negative control, and DNA extracted from sheep infected with A. capra (GenBank accession nos. MG879297, MH174929, and MH174932) was used as a positive control. PCR reactions were performed in an ABI 2720 thermal cycler (Life Technologies Holdings Pte Ltd., Singapore). The products were examined by agarose gel electrophoresis and visualized after staining with GelRed (Biotium Inc., Hayward, CA).
Sequencing and Phylogenetic Analysis
Positive PCR products were purified using Montage PCR filters (Millipore, Bedford, MA) and sequenced using a BigDye Terminator v 3.1 cycle sequencing kit (Applied Biosystems, Foster City, CA) on an ABI 3730 DNA analyzer (Applied Biosystems). Nucleotide sequences were confirmed by bidirectional sequencing and by sequencing a new PCR product if necessary. They were then compared with reference sequences downloaded from the National Center for Biotechnology Information (https://www.ncbi.nlm.nih.gov/) using ClustalX 2.1 (http://www.clustal.org/) to determine new variant strains of A. capra.
Phylogenetic trees were conducted by Bayesian inference (BI) and Monte Carlo Markov Chain methods in MrBayes v 3.2.6 (http://mrbayes.sourceforge.net/). FigTree v 1.4.4 (http://tree.bio.ed.ac.uk/software/figtree/) was used to visualize and edit the maximum clade credibility tree generated by these analyses. Posterior probability values were estimated based on 1,000,000 generations with four simultaneous tree building chains, with trees being saved every 100th generation. A 50% majority rule consensus tree for each analysis was constructed based on the final 75% of trees generated by BI.
Sequences similarity were further analyzed by DNAStar Laser-gene program (DNAStar Inc., Madison, WI, USA) to evaluated the homology of the sequences obtained in the present with the sequences downloaded from the National Center for Biotechnology Information (https://www.ncbi.nlm.nih.gov/) (Figure S2).
Statistical Analysis
Variations in A. capra infection of dogs at different locations, seasons, age groups, genders, clinical signs, and use of vermifuge were analyzed by Fisher's exact test with SPSS (version 22.0) software. Differences were considered statistically significant if P < 0.05. Odds ratios (ORs) and their 95% confidence intervals (95% CIs) were estimated to explore the strength of the association between A. capra-positivity and the conditions tested. Then, the significant different correlation factors were used to process the canonical correspondence analysis by canoco5 (http://www.canoco5.com) (Figure S1).
Nucleotide Sequence Accession Numbers
The representative sequences obtained in this study have been submitted and deposited in the GenBank database with the following accession numbers: gltA (MK838608 and MK838609), groEL (MK862099), and msp4 (MK838605– MK838607).
Ethics Statement
This study was carried out in accordance with the Chinese Laboratory Animal Administration Act (1988) after it was reviewed and its protocol was approved by the Research Ethics Committee of Henan Agricultural University. Appropriate permission was gained from the dog owners before the collection of blood specimens.
Results
Infection of Dogs With A. capra
A total of 63 of the 521 dog blood samples were A. capra-positive by PCR screening (12.1%, 95% CI: 9.3–14.9). The infection rates of A. capra in pet dogs and stray dogs were 12.9% (59/458, 95% CI: 9.8–16.0) and 6.3% (4/63, 95% CI: 0.2–12.5), respectively, There were no significant differences in the infection ratio of A. capra in pet dogs and stray dogs (P = 0.154) (Table 2).
Distribution of A. capra Infections
A. capra infections in dogs with different types of samples were analyzed (Table 2). A. capra infections were found in five of the six sampling sites, at rates ranging from 0–18.5%, with significant differences among sites (P = 0.002). Additionally, higher infection rates were observed in dogs older than 10 years (33.3%, 95% CI: 6.3–60.4), and lower rates were seen in dogs aged 3–6 months compared with other age groups (6.5%, 95% CI: 1.4–11.5) (P = 0.040). As expected, the highest infections were documented in the summer months (20.4%, 95% CI: 13.7–27.1) (P = 0.006), and in dogs with anemic symptoms such as pale mucous membranes (P = 0.019). However, there were no differences in infection rates between dogs of different genders (P = 0.578), nor in those with influenza-like illness symptoms including fever, cough, malaise, and depression (P = 0.370), nor in those administered vermifuge in the last month (P = 0.651).
Sequences and Phylogenetic Analysis
Of the 63 A. capra-positive specimens, 26 were PCR-positive based on gltA, 17 based on groEL, and 61 based on msp4. Sequence analysis showed that gltA sequences were divided into two distinct sequence types: one (including 20 isolate sequences, MK838608) shared 100% identity with A. capra isolates from China, with previous hosts including humans (KM206274), goats (KM869310), sheep (KM869280), and ticks (MG940871 and MH029895). The others (including six isolates sequences, MK838609) showed 99.7% identity with A. capra isolates from humans (KM206274) (Figure S2A). Phylogenetic analysis based on gltA sequences revealed that the two types were in the same Anaplasma species clade (Figure 1). These isolates were closely related to A. capra, but distinct from other known Anaplasma species (Figure 1).
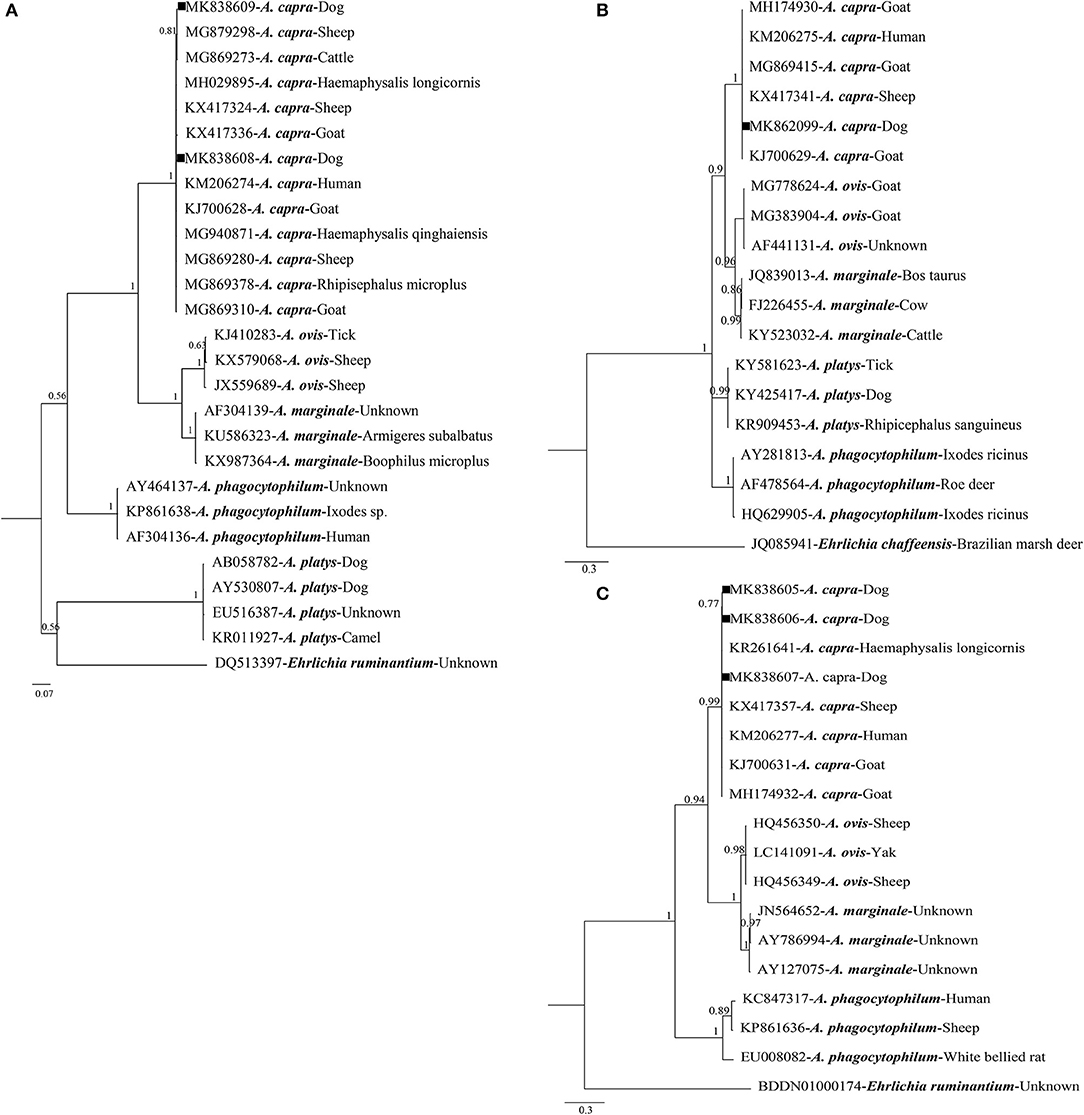
Figure 1. Bayesian phylogenetic analysis of A. capra based on gltA (A), groEL (B), and msp4 (C) sequences. Significant posterior probabilities are indicated at branches. Sample names include GenBank accession numbers followed by Anaplasma spp. Ehrlichia ruminantium and Ehrlichia chaffeensis were used as outgroups. The sequences identified in this study are marked by squares.
Sequence analysis revealed that groEL gene sequences obtained in this study (MK862099) were 100% identical to each other. Findings showed that the sequence had two single nucleotide polymorphisms (SNPs; G→ A and T→ A substitutions at positions 445 and 827, respectively) compared with the human isolate sequence (KM206275), and shared 99.9% identity with sequences isolated from sheep (KX417341), goats (KJ700629), and ticks (KX987393) (Figure S2B). Phylogenetic analysis based on groEL gene sequences demonstrated that the isolates were clustered within the A. capra clade, but distinct from other well-defined Anaplasma species (Figure 1).
For msp4 gene sequences, we acquired three different sequences (MK838605–MK838607) from dogs in the present study. One (KM838607) shared 100% identity with those of A. capra isolates from humans (KM206277), and the others had one (T→ C at position 320) or two (C→ T and T→ C substitutions at positions 246 and 320, respectively) SNPs compared with the human sequence (KM206277), and shared 99.7–99.8% similarity with sequences of Anaplasma spp. from humans (KM206277), goats (KJ700631), sheep (KX417357), and ticks (KR261641) (Figure S2C). Phylogenetic analysis based on msp4 sequences demonstrated that the isolates were clustered within the A. capra clade, but distinct from other well-defined Anaplasma species (Figure 1).
Discussion
The natural infection cycle of Anaplasma species is dependent upon the presence of tick vectors and infected vertebrate reservoir hosts (de la Fuente et al., 2016). Anaplasma species are transmitted by ixodid ticks transstadially rather than transovarially, so reservoir hosts play a crucial role in the maintenance and spread of these pathogens (Yang et al., 2018). Currently, a number of vertebrate animals are considered competent hosts for Anaplasma, including humans, cattle, sheep, goats, dogs, horses, and deer (Stuen et al., 2013). Moreover, dogs have been reported as hosts of A. phagocytophilum, A. platys, A. ovis, and A. bovis (Zhang et al., 2012; Li et al., 2014; Cui et al., 2017).
Host and environmental factors are thought to play an important role in the epidemiology of TBD in dogs (Abd Rani et al., 2011). Furthermore, high-quality vegetative cover may have increased the abundance and diversity of ticks (Fang et al., 2015). A previous study showed that TBD were less likely to infect dogs from refuges and animals fed nutritious diets, even after adjusting for the presence of ticks, than free-roaming strays (Abd Rani et al., 2011). However, we found no difference in the natural infection rate of A. capra between pet and stray dogs (P = 0.154).
The novel tick-transmitted zoonotic A. capra was identified in dogs from five of six sampling sites in the present study, with a total infection rate of 12.1% (63/521). Likewise, A. ovis, A. bovis, and A. phagocytophilum have been identified in dogs in Henan with prevalences of 6.2%, 4.1%, and 0.4%, respectively (Cui et al., 2017), suggesting that Anaplasma species are common pathogens of dogs in Henan. We detected the highest infection rate in Pet clinic 1 (P = 0.002), which is located in a suburban area of the city surrounded by rich vegetation. Similar findings were previously observed for the tick-borne fever with thrombocytopenia syndrome which was also significantly associated with vegetation-rich regions (Liu et al., 2014; Fang et al., 2015).
We observed the highest A. capra infection rate in dogs older than 10 years of age. Similarly, other canine vector-borne disease pathogens such as A. phagocytophilum and B. burgdorferi were detected at higher infection rates in Korean dogs aged over 2 years (Lim et al., 2010), while no significant difference in A. platys infection was observed among various groups of dogs (Kamani et al., 2013). Dogs aged over 10 years, so-called elderly dogs, may have a poor physical condition and be more easily infected by pathogens. Furthermore, A. capra infection may be chronic and persistent infection, so the potential role of elderly dogs as carriers of A. capra should be noted. We observed no difference in infection rate between dogs of different genders, which is consistent with previous reports about tick-borne pathogens (Lim et al., 2010; Hornok et al., 2013; Peng et al., 2018).
Our study also revealed a high prevalence of A. capra infection during the summer season. Similar results were reported for Anaplasma species such as A. bovis, A. ovis, and A. capra in sheep, goats, and cattle (Belkahia et al., 2017; Seo et al., 2018). All Anaplasma species are transmitted to their natural hosts by ticks, and the warm summer season offers favorable conditions for tick distribution (Belkahia et al., 2017). Thus far, A. capra has also been detected in ticks such as Ixodes persulcatus, Haemaphysalis longicornis, and Haemaphysalis qinghaiensis in many places in China (Peng et al., 2018). From spring to autumn, ticks are found throughout China and demonstrate extended periods of activity; therefore with rising tick numbers, the risks of host infection with tick-borne pathogens also increase (Chvostáč et al., 2018; Jaimes-Dueñez et al., 2018).
Clinical features of human infection by A. capra include an influenza-like illness such as fever, headache, malaise, and chills (Li et al., 2015). In the present study, we found no difference in A. capra infections between dogs with and without influenza-like illness symptoms. However, a significant difference was detected between dogs with and without symptoms of anemia such as pale mucous membranes. However, Li and colleagues (Li et al., 2015) did not describe morulae or other forms of A. capra in peripheral blood smears, but instead found that A capra was more closely related to species that infect mammalian erythrocytes; thus, they anticipated intracellular A capra infection in mammalian erythrocytes. The correlation of anemia with A. capra infection should nonetheless be verified in a future study, while the present study describes the clinical features of A. capra infection in dogs for the first time.
Phylogenetic analysis of A. capra based on gltA, groEL, and msp4 showed that the isolate sequences obtained in the present study, as well as those previously isolated from sheep, ticks, goats, and humans, formed an independent clade clearly distinct from other members of Anaplasma species (Li et al., 2015; Yang et al., 2017; Guo et al., 2018; Peng et al., 2018; Figure 1). For gltA, one sequence (MK838608) obtained in this study shared 100% identity with an A. capra isolate (KM206274) from humans, while the other sequence (MK838609) shared 99.7% identity with the same isolates from humans (Li et al., 2015). For groEL, the sequence acquired from dogs (MK862099) shared 99.9% identity with the sequence from humans (KM206275), and three different msp4 sequences obtained in the present study shared 99.7–100% similarity with sequences of Anaplasma species from humans (KM206277) (Li et al., 2015). Phylogenetic analysis indicated that A. capra identified in this study was highly similar to sequences obtained from humans (KM206274, KM206275, and KM206277), in view of the affinity between dogs and humans, indicating that a high level of attention should be paid to A. capra infection in dogs for public health reasons.
A large proportion of A. capra sequences (20/26) obtained from dogs in this study showed 100% identity with isolates from ticks, sheep, goats, and humans (Li et al., 2015; Yang et al., 2017; Guo et al., 2018; Peng et al., 2018). Furthermore, many domestic animals like dogs may serve as a suitable reservoir or a dead end host of Anaplasma species in urban areas (Schorn et al., 2011). Companion animals are becoming increasingly popular in China, and the transport of dogs to other areas adds to the spread of pathogens (Stuen et al., 2013). Considering dogs as hosts both of Anaplasma species and ticks, together with the growing affinity between humans and dogs, we should not ignore their important role in spreading A. capra infection in areas where tick vectors are abundant.
Conclusion
The present study documents dogs as a new host for A. capra for the first time. It is noteworthy that older dogs are more readily infected by A. capra. The risk factor for infection is predicted to increase with rising numbers of ticks in hot seasons and with increasing contact between dogs and ticks. Hence, we advise that dog owners prevent their pets from entering woods, copses, mountainsides, and grass close to rivers during peak tick seasons to limit vector contact. Further research should also evaluate whether dogs are competent reservoirs of A. capra.
Data Availability Statement
The datasets generated for this study can be found in the GenBank database with the following accession numbers: gltA (MK838608 and MK838609), groEL (MK862099), and msp4 (MK838605–MK838607).
Ethics Statement
The animal study was reviewed and approved by Chinese Laboratory Animal Administration Act (1988); Research Ethics Committee of Henan Agricultural University. Written informed consent was obtained from the owners for the participation of their animals in this study.
Author Contributions
CN conceived the study. CN and LZ designed the experiments. KS, YY, QC, KW, YZ, and YP performed the experiments. KS, DL, YC, and FY performed data analysis. KS, JL, and CN wrote the manuscript. All authors approved the final version of the manuscript.
Funding
This work was supported by the Earmarked Fund for China Modern Agro-industry Technology Research System (nycytx-38) and the National Key R&D Program of China (2018YFD0502100).
Conflict of Interest
The authors declare that the research was conducted in the absence of any commercial or financial relationships that could be construed as a potential conflict of interest.
Acknowledgments
We thank Sarah Williams, Ph.D., from Liwen Bianji, Edanz Group China (www.liwenbianji.cn), for editing the English text of a draft of this manuscript.
Supplementary Material
The Supplementary Material for this article can be found online at: https://www.frontiersin.org/articles/10.3389/fcimb.2019.00394/full#supplementary-material
Figure S1. Results of Canonical correspondence Analysis (CCA) between the prevalence of A. capra and several variables.
Figure S2. The homology analysis of A. capra based on gltA (A), groEL (B), and msp4 (C) sequences.
References
Abd Rani, P. A., Irwin, P. J., Coleman, G. T., Gatne, M., and Traub, R. J. (2011). A survey of canine tick-borne diseases in India. Parasit. Vectors. 4:141. doi: 10.1186/1756-3305-4-141
Aktas, M., Altay, K., and Dumanli, N. (2010). Molecular detection and identification of Anaplasma and Ehrlichia species in cattle from Turkey. Ticks Tick Borne Dis. 2, 62–65. doi: 10.1016/j.ttbdis.2010.11.002
Belkahia, H., Ben Said, M., El Mabrouk, N., Saidani, M., Cherni, C., Ben Hassen, M., et al. (2017). Seasonal dynamics, spatial distribution and genetic analysis of Anaplasma species infecting small ruminants from Northern Tunisia. Infect. Genet. Evol. 54, 66–73. doi: 10.1016/j.meegid.2017.06.016
Beyer, A. R., and Carlyon, J. A. (2015). Of goats and men: rethinking anaplasmoses as zoonotic infections. Lancet Infect. Dis. 15, 619–620. doi: 10.1016/S1473-3099(15)70097-6
Chen, S. M., Dumler, J. S., Bakken, J. S., and Walker, D. H. (1994). Identification of a granulocytotropic Ehrlichia species as the etiologic agent of human disease. J. Clin. Microbiol. 32, 589–595.
Chochlakis, D., Ioannou, I., Tselentis, Y., and Psaroulaki, A. (2010). Human anaplasmosis and Anaplasma ovis variant. Emerging Infect. Dis. 16, 1031–1032. doi: 10.3201/eid1606.090175
Chvostáč, M., Špitalská, E., Václav, R., Vaculová, T., Minichová, L., and Derdáková, M. (2018). Patterns in the prevalence and diversity of tick-borne Borrelia burgdorferi Sensu Lato, Anaplasma phagocytophilum and Rickettsia spp. in an Urban Temperate Forest in South Western Slovakia. Int. J. Environ. Res. Public Health. 15:E994. doi: 10.3390/ijerph15050994
Cito, F., Rijks, J., Rantsios, A. T., Cunningham, A. A., Baneth, G., Guardabassi, L., et al. (2016). Prioritization of companion animal transmissible diseases for policy intervention in Europe. J. Comp. Pathol. 155, S18–26. doi: 10.1016/j.jcpa.2015.01.007
Cui, Y., Yan, Y., Wang, X., Cao, S., Zhang, Y., Jian, F., et al. (2017). First molecular evidence of mixed infections of Anaplasma species in dogs in Henan, China. Ticks Tick Borne Dis. 8, 283–289. doi: 10.1016/j.ttbdis.2016.12.001
de la Fuente, J., Estrada-Peña, A., Cabezas-Cruz, A., and Kocan, K. M. (2016). Anaplasma phagocytophilum uses common strategies for infection of ticks and vertebrate hosts. Trends Microbiol. 24, 173–180. doi: 10.1016/j.tim.2015.12.001
Fang, L. Q., Liu, K., Li, X. L., Liang, S., Yang, Y., Yao, H. W., et al. (2015). Emerging tick-borne infections in mainland China: an increasing public health threat. Lancet Infect. Dis. 15, 1467–1479. doi: 10.1016/S1473-3099(15)00177-2
Guo, W. P., Huang, B., Zhao, Q., Xu, G., Liu, B., Wang, Y. H., et al. (2018). Human-pathogenic Anaplasma spp., and Rickettsia spp. in animals in Xi'an, China. PLoS Negl. Trop. Dis. 12:e0006916. doi: 10.1371/journal.pntd.0006916
Hornok, S., Dénes, B., Meli, M. L., Tánczos, B., Fekete, L., Gyuranecz, M., et al. (2013). Non-pet dogs as sentinels and potential synanthropic reservoirs of tick-borne and zoonotic bacteria. Vet Microbiol. 167, 700–703. doi: 10.1016/j.vetmic.2013.08.011
Jaimes-Dueñez, J., Triana-Chávez, O., and Mejía-Jaramillo, A. M. (2018). Genetic, host and environmental factors associated with a high prevalence of Anaplasma marginale. Ticks Tick Borne Dis. 9, 1286–1295. doi: 10.1016/j.ttbdis.2018.05.009
Kamani, J., Baneth, G., Mumcuoglu, K. Y., Waziri, N. E., Eyal, O., Guthmann, Y., et al. (2013). Molecular detection and characterization of tick-borne pathogens in dogs and ticks from Nigeria. PLoS Negl. Trop. Dis. 7:e2108. doi: 10.1371/journal.pntd.0002108
Li, H., Zheng, Y. C., Ma, L., Jia, N., Jiang, B. G., Jiang, R. R., et al. (2015). Human infection with a novel tick-borne Anaplasma species in China: a surveillance study. Lancet Infect. Dis. 15, 663–670. doi: 10.1016/S1473-3099(15)70051-4
Li, H. T., Sun, L. S., Chen, Z. M., Hu, J. S., Ye, C. D., Jia, K., et al. (2014). Detection of Anaplasma platys in dogs using real-time loop-mediated isothermal amplification. Vet J. 199, 468–470. doi: 10.1016/j.tvjl.2013.11.012
Lim, S., Irwin, P. J., Lee, S., Oh, M., Ahn, K., Myung, B., et al. (2010). Comparison of selected canine vector-borne diseases between urban animal shelter and rural hunting dogs in Korea. Parasit. Vectors. 3:32. doi: 10.1186/1756-3305-3-32
Liu, K., Cui, N., Fang, L. Q., Wang, B. J., Lu, Q. B., Peng, W., et al. (2014). Epidemiologic features and environmental risk factors of severe fever with thrombocytopenia syndrome, Xinyang, China. PLoS Negl. Trop. Dis. 8:e2820. doi: 10.1371/journal.pntd.0002820
Maggi, R. G., and Krämer, F. (2019). A review on the occurrence of companion vector-borne diseases in pet animals in Latin America. Parasit. Vectors. 12, 145. doi: 10.1186/s13071-019-3407-x
Peng, Y., Wang, K., Zhao, S., Yan, Y., Wang, H., Jing, J., et al. (2018). Detection and phylogenetic characterization of Anaplasma capra: an emerging pathogen in sheep and goats in China. Front. Cell. Infect. Microbiol. 8:283. doi: 10.3389/fcimb.2018.00283
Pereira, A., Parreira, R., Nunes, M., Casadinho, A., Vieira, M. L., Campino, L., et al. (2016). Molecular detection of tick-borne bacteria and protozoa in cervids and wild boars from Portugal. Parasit. Vectors. 9:251. doi: 10.1186/s13071-016-1535-0
Sato, M., Nishizawa, I., Fujihara, M., Nishimura, T., Matsubara, K., and Harasawa, R. (2009). Phylogenetic analysis of the 16S rRNA gene of Anaplasma species detected from Japanese serows (Capricornis crispus). J. Vet. Med. Sci. 71, 1677–1679. doi: 10.1292/jvms.001677
Schorn, S., Pfister, K., Reulen, H., Mahling, M., Manitz, J., Thiel, C., et al. (2011). Prevalence of Anaplasma phagocytophilum in Ixodes ricinus in Bavarian public parks, Germany. Ticks Tick Borne Dis. 2, 196–203. doi: 10.1016/j.ttbdis.2011.09.009
Seo, M. G., Ouh, I. O., Lee, H., Geraldino, P. J. L., Rhee, M. H., Kwon, O. D., et al. (2018). Differential identification of Anaplasma in cattle and potential of cattle to serve as reservoirs of Anaplasma capra, an emerging tick-borne zoonotic pathogen. Vet. Microbiol. 226, 15–22. doi: 10.1016/j.vetmic.2018.10.008
Stuen, S., Granquist, E. G., and Silaghi, C. (2013). Anaplasma phagocytophilum—a widespread multi-host pathogen with highly adaptive strategies. Front. Cell. Infect. Microbiol. 3:31. doi: 10.3389/fcimb.2013.00031
Sun, X. F., Zhao, L., Wen, H. L., Luo, L. M., and Yu, X. J. (2015). Anaplasma species in China. Lancet Infect. Dis. 15, 1263–1264. doi: 10.1016/S1473-3099(15)00377-1
Uspensky, I. (2014). Tick pests and vectors (Acari: Ixodoidea) in European towns: introduction, persistence and management. Ticks Tick Borne Dis. 5, 41–47. doi: 10.1016/j.ttbdis.2013.07.011
Vlahakis, P. A., Chitanga, S., Simuunza, M. C., Simulundu, E., Qiu, Y., Changula, K., et al. (2018). Molecular detection and characterization of zoonotic Anaplasma species in domestic dogs in Lusaka, Zambia. Ticks Tick Borne Dis. 9, 39–43. doi: 10.1016/j.ttbdis.2017.10.010
Wei, R., Liu, H. B., Jongejan, F., Jiang, B. G., Chang, Q. C., Fu, X., et al. (2017). Cultivation of Anaplasma ovis in the HL-60 human promyelocytic leukemia cell line. Emerg. Microbes Infect. 6:e83. doi: 10.1038/emi.2017.70
Xu, D., Zhang, J., Shi, Z., Song, C., Zheng, X., Zhang, Y., et al. (2015). Molecular detection of vector-borne agents in dogs from ten provinces of China. Parasit. Vectors. 8:501. doi: 10.1186/s13071-015-1120-y
Yabsley, M. J., Davidson, W. R., Stallknecht, D. E., Varela, A. S., Swift, P. K., Devos, J. C. Jr., et al. (2005). Evidence of tick-borne organisms in mule deer (Odocoileus hemionus) from the western United States. Vector Borne Zoonotic Dis. 5, 351–362. doi: 10.1089/vbz.2005.5.351
Yang, J., Liu, Z., Niu, Q., Liu, J., Han, R., Guan, G., et al. (2017). A novel zoonotic Anaplasma species is prevalent in small ruminants: potential public health implications. Parasit. Vectors. 10:264. doi: 10.1186/s13071-017-2182-9
Yang, J., Liu, Z., Niu, Q., Mukhtar, M. U., Guan, G., Liu, G., et al. (2018). A novel genotype of “Anaplasma capra” in wildlife and its phylogenetic relationship with the human genotypes. Emerg. Microbes Infect. 7:210. doi: 10.1038/s41426-018-0212-0
Zhang, L., Liu, H., Xu, B., Lu, Q., Li, L., Chang, L., et al. (2012). Anaplasma phagocytophilum infection in domestic animals in ten provinces/cities of China. Am. J. Trop. Med. Hyg. 87, 185–189. doi: 10.4269/ajtmh.2012.12-0005
Keywords: Anaplasma capra, dogs, hosts, tick-borne, zoonotic
Citation: Shi K, Li J, Yan Y, Chen Q, Wang K, Zhou Y, Li D, Chen Y, Yu F, Peng Y, Zhang L and Ning C (2019) Dogs as New Hosts for the Emerging Zoonotic Pathogen Anaplasma capra in China. Front. Cell. Infect. Microbiol. 9:394. doi: 10.3389/fcimb.2019.00394
Received: 19 July 2019; Accepted: 04 November 2019;
Published: 26 November 2019.
Edited by:
Yasuko Rikihisa, The Ohio State University, United StatesReviewed by:
Weipeng Zhang, Ocean University of China, ChinaNick Wheelhouse, Edinburgh Napier University, United Kingdom
Tais Berelli Saito, University of Texas Medical Branch at Galveston, United States
Copyright © 2019 Shi, Li, Yan, Chen, Wang, Zhou, Li, Chen, Yu, Peng, Zhang and Ning. This is an open-access article distributed under the terms of the Creative Commons Attribution License (CC BY). The use, distribution or reproduction in other forums is permitted, provided the original author(s) and the copyright owner(s) are credited and that the original publication in this journal is cited, in accordance with accepted academic practice. No use, distribution or reproduction is permitted which does not comply with these terms.
*Correspondence: Changshen Ning, nnl1986@163.com
†These authors have contributed equally to this work