- 1Cell Culture & Immunology Laboratory, Department of Immunochemistry of Glycoconjugates, Center for Glycomics, Institute of Chemistry, Slovak Academy of Sciences, Bratislava, Slovakia
- 2Laboratory of Glycoconjugate Chemistry, N.D. Zelinsky Institute of Organic Chemistry, Russian Academy of Sciences, Moscow, Russia
The incidence and prevalence of serious fungal infections is rising, especially in immunosuppressed individuals. Moreover, co-administration of antibiotics and immunosuppressants has driven the emergence of new multidrug-resistant pathogens. The significant increase of multidrug-resistant pathogens, together with their ability to form biofilms, is associated with morbidity and mortality. Research on novel synthetically prepared immunomodulators as potential antifungal immunotherapeutics is of serious interest. Our study demonstrated the immunobiological activity of synthetically prepared biotinylated mannooligosaccharides mimicking Candida antigenic factors using RAW264.7 macrophages. Macrophage exposure to the set of eight structurally different mannooligosaccharides induced a release of Th1, Th2, Th17, and Treg cytokine signature patterns. The observed immune responses were tightly associated with structure, dose, exposure time, and selected signature cytokines. The viability/cytotoxicity of the mannooligosaccharide formulas was assessed based on cell proliferation. The structure-based immunomodulatory activity of the formulas was evaluated with respect to the length, branching and conformation of the various formulas. Glycoconjugate formulas with terminal β-mannosyl-units tended to be more potent in terms of Candida relevant cytokines IL-12 p70, IL-17, GM-CSF, IL-6, and TNFα induction and cell proliferation, and this tendency was associated with structural differences between the studied glycoconjugate formulas. The eight tested mannooligosaccharide conjugates can be considered potential in vitro immunomodulative agents suitable for in vitro Candida diagnostics or prospectively for subcellular anti-Candida vaccine design.
Introduction
Most Candida species, including the facultative pathogenic strains, belong to the normal commensal mycobiota of immunocompetent individuals. The factors affecting the candidosis are diverse, including the prolonged antifungal treatment in long-term care, immunosuppression associated with anticancer therapy and transplantation of solid organ or bone marrow, immunosuppressive states as diabetes mellitus and HIV, use of vascular devices and hospitalization at intensive care units (Richter et al., 2005; Angiolella et al., 2008; Adiguzel et al., 2010; Cortés and Corrales, 2018). Next, immunocompromised persons with genetic immune system defects are at high risk for mucocutaneous and invasive fungal infections (Vinh, 2011; Cunha and Carvalho, 2012; Pichard et al., 2015; Beenhouwer, 2018). Approximately 17 different Candida species are known etiological agents of human infections; more than 90% of systemic infections are caused by Candida albicans (C. albicans), Candida glabrata (C. glabrata), Candida parapsilosis (C. parapsilosis), Candida tropicalis (C. tropicalis), and Candida krusei (C. krusei) (Pfaller et al., 2002). The new multidrug-resistant species Candida auris (C. auris) was recently isolated (Sears and Schwartz, 2017; Forsberg et al., 2019). CD4+-derived T-cell subpopulations Th1, Th2, and Th17 contribute to anti-Candida cellular immune protection. The protective anticandidal Th1 response requires the activity of various cytokines, such as interferon gamma (IFN-γ), transforming growth factor beta (TGF-β), interleukin 6 (IL-6), tumor necrosis factor alpha (TNFα), and IL-12. The induction of the protective antifungal Th1 immune response is inhibited by Th2 cytokines, such as IL-4 and IL-10 (Ito, 2011; Netea et al., 2015; Richardson and Moyes, 2015; Gow et al., 2017). In early infection, neutralization of Th1 cytokines, mainly IFN-γ and IL-12, leads predominately to the onset of Th2 rather than Th1 responses. Th2-type responses are frequently associated with susceptibility to recurrent or persistent infection and fungal allergy. TNFα, IL-1β, IL-6, IL-8, and colony-stimulating factors (CSFs) are among the major proinflammatory cytokines associated with the interaction of immune-competent cells with Candida cells. TNFα is thought to be essential in the primary control of disseminated infection caused by C. albicans. Although IL-1 shares common properties with TNFα, both IL-1β and IL-6, acting mainly through recruitment of polymorphonuclear neutrophils (PMNs), presumably are not as essential as TNFα in the innate antifungal response. IL-12 is recognized as essential to induce the protective Th1 response to the fungus, simultaneously blocking the Th2 response. The crucial role of the Th17 subset has been associated with anti-Candida effectiveness, especially the mucosal immune response (Romani, 2003; Rizzetto et al., 2010; van de Veerdonk and Netea, 2010). Proinflammatory cytokines, such as IL-12, IL-15, and TNFα, have been studied as candidate adjuvants in preclinical trials based on their ability to upregulate the antifungal Th1 response (Ashman and Papadimitriou, 1995; Romani, 2011; Pikman and Ben-Ami, 2012; Naglik, 2014).
Fungal cell wall antigenically active polysaccharides, such as N-linked and O-linked α- and β-mannans, chitin, α- and β-glucans, galactomannan, galactosaminogalactan, glucuronoxylomannan, and some others, are essential immunogens that play crucial roles during host-fungus interactive communication. Cell-wall components act as pathogen-associated molecular patterns (PAMPs), recognized by the immune system through pattern recognition receptors (PRRs) such as TLR2, TLR4, dectin-2, dectin-1, Mincle, DC-SIGN, or galectin-3, on the surfaces of epithelia and myeloid cells (Netea et al., 2006, 2008, 2015; Moyes and Naglik, 2011; Perez-Garcia et al., 2011; Romani, 2011; Cunha and Carvalho, 2012; Salek-Ardakani et al., 2012; Hall and Gow, 2013; Moyes et al., 2015; Zheng et al., 2015; Gow et al., 2017; Snarr et al., 2017).
Generally, specific PAMP–PRR interactions activate the inflammatory response by triggering interleukins and growth factors cell release and phagocytosis. O-linked mannans are recognized via TL4 receptor (Netea et al., 2006), α- linked N- mannans are sensed through mannose receptor, dectin-2, Mincle, and DC-SIGN (Harris et al., 2009; McKenzie et al., 2010), and the specific receptor for β-mannan is galectin-3 (Jouault et al., 2006; Linden et al., 2013). Chitin cooperates with the mannose receptor and induces TLR9 and NOD-2dependentIL-10 release (Wagener et al., 2014; Erwig and Gow, 2016). Recently, it has been demonstrated that chitin particles of small size stimulated IL-17, IL-12, IL-23, IL-10, and TNF-α in macrophages via a MyD88- and TLR2-dependent pathway (Da Silva et al., 2008, 2009). Additionally, Dectin-1 receptor on macrophages and TLR-2 recognizes β-1,3-glucan (Brown and Gordon, 2001; Brown et al., 2002, 2003; Brown, 2006). Dectin-1 uses Syk kinase and the CARD9 to stimulate IL-10, TLR2 via the MyD88 is required for the production of IL-12p40 (Dennehy et al., 2008; Netea et al., 2008), and both pathways collaborate in TNF- stimulation. Moreover, dectin-1 and galectin-3 interact synergistically to improve the outcome of host immune response to C. albicans (Gantner et al., 2003; Taylor et al., 2007; Esteban et al., 2011).
The antigenic factors of mannan from medically relevant Candida species have been characterized and their chemical structures determined in several studies (Nishikawa et al., 1982; Suzuki and Fukazawa, 1982; Shibata et al., 1995; Fukazawa et al., 1997; Suzuki, 1997). The antigenic determinants of cell wall polysaccharides and oligosaccharides from medically important yeasts have been studied for their serological specificity and biological activity (Fukazawa et al., 1997). The investigation of species-specific antigenic factor variations of Candida mannan and oligomannosyl structures is essential to evaluate the structure-activity relationship, since mannan structure and epitope availability intensely affect its immunobiological behavior (Trinel et al., 1992; Fukazawa et al., 1997; Suzuki, 1997; Shibata et al., 2007). The particular structure of mannan, comprising an α-1,6-mannoside backbone and side chains with α/β-1,2-mannoside or α/β-1,3-mannoside moieties of variable lengths, varies for different Candida species and is dependent on the expression of a complex network of mannan biosynthesis, trafficking, and cell wall remodeling genes (Shibata et al., 2012). Different growth conditions are likely to modulate the activation of cell wall signaling cascades, expression of cell wall biosynthesis genes, and alterations in mannan composition (Ernst and Pla, 2011; Lowman et al., 2011). The role of mannosylation in fungal biology and virulence has been studied using C. albicans mutants; the suitability of these mutants for exploring the significance of specific mannan epitopes on cell function, pathogenesis and immune recognition has been proposed (Hall and Gow, 2013; Hall et al., 2013; West et al., 2013). Several studies have attempted to design and develop an anti-Candida vaccine based on cell wall-derived structures (Ito, 2011; Richardson and Moyes, 2015; Tso et al., 2018; Piccione et al., 2019). The immunogenic polysaccharide cell wall structures applied in experimental vaccine models include 65 kDa mannoproteins (Sandini et al., 2007), β-1,3-glucan (Torosantucci et al., 2005), and β-1,2- mannosides (Han et al., 1999; Cutler, 2005). These model structures were effective in humoral antibody-mediated antifungal protection. Several monoclonal antibodies were protective in preclinical studies: anti- β-1,3-glucan mAb2G8 (Torosantucci et al., 2005), anti-mannoprotein mAb C7 (Moragues et al., 2003), anti-idiotypic antibodies (Magliani et al., 2004), anti-mannan mAb (Han et al., 1999; Cutler, 2005), and anti-glycosyl mAb (Kavishwar and Shukla, 2006). These antibodies efficiently appeared as candidacidal (Moragues et al., 2003; Magliani et al., 2004; Kavishwar and Shukla, 2006), growth inhibitory, or they neutralized heat shock protein 90 (Hsp90) (Torosantucci et al., 2005). Moreover, mannan conjugated in certain vaccine formulas has already been included in clinical trials (Apostolopoulos et al., 2006; Pashov et al., 2011).
Mannan has also been studied as a promising bioactive material for drug nanocarrier systems and vaccine adjuvant formulations (Tang et al., 2009). Moreover, nanoliposomes with orthogonally bound mannan represent a platform for the development of targeted drug delivery systems and self-adjuvanted carriers for construction of recombinant vaccines (Bartheldyova et al., 2019). Concerning the design of anti-fungal vaccination therapy, apart from Candida cell wall moieties, potential new anti-Candida drugs have targeted the growth and virulence factors of C. albicans, including core signaling components of the high-osmolarity glycerol (HOG) and target of rapamycin (TOR) signaling pathways (Li et al., 2015), as well as various immunomodulators, e.g., colony-stimulating factors and proinflammatory cytokines (Pikman and Ben-Ami, 2012).
Natural Candida mannan is a complex polysaccharide structure containing linear and branched fragments composed of α- and β-mannose units, as seen in Figure 1 (Klis et al., 2001), with the carbohydrate sequences represented according to symbol carbohydrate nomenclature (Varki et al., 1999). Thus, the use of such a heterogenic structure is problematic for the assessment of the biological role of its distinct fragments. However, the application of synthetic mannooligosaccharide derivatives, related to the structures of selected antigenic factors of Candida mannan, creates the opportunity to assess the biological roles of each antigenic factor.
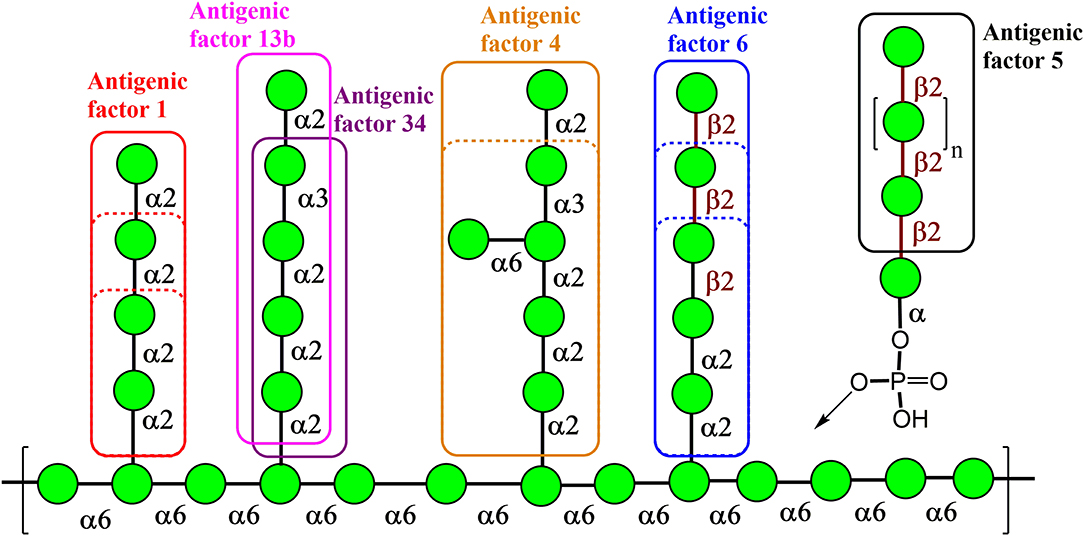
Figure 1. Key structural elements of the cell wall mannan from the fungus Candida albicans representing its antigenic factors. The carbohydrate sequences are represented according to symbol carbohydrate nomenclature (Varki et al., 1999).
Our work investigated the immunomodulation properties of antigenically active distinct parts of C. albicans mannan by using a series of structurally related synthetic mannooligosaccharides.
Materials and Methods
Synthesis of Biotinylated Oligomannosides 1–8
Mannooligosaccharide conjugate formulas 1 (Krylov et al., 2018a), 2 (Krylov et al., 2018a,b), 3 (Karelin et al., 2007), 4 (Karelin et al., 2010), and 5–8 (Karelin et al., 2016) were prepared by the biotinylation of parent ligands according to previously described biotinylation protocols (Figure 2) (Tsvetkov et al., 2012).
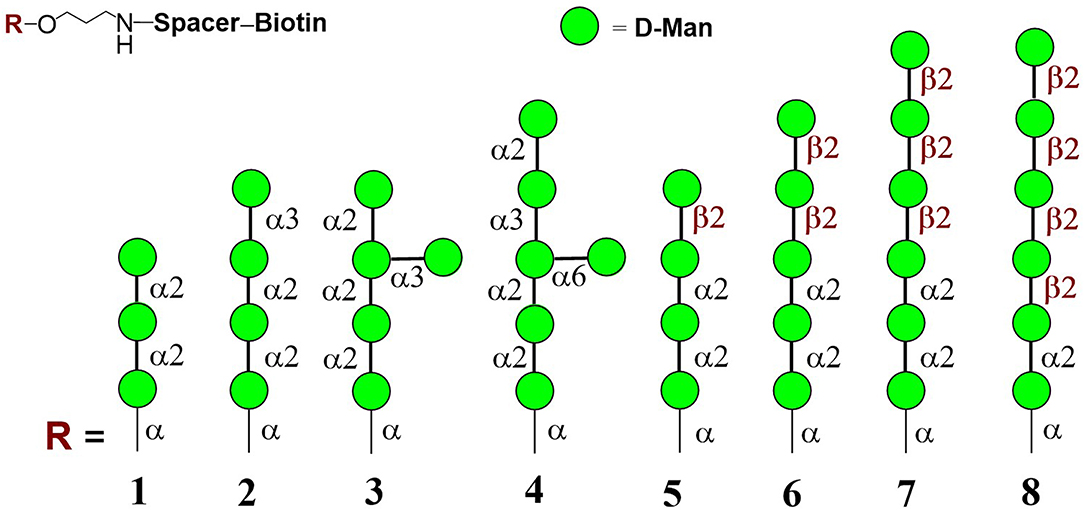
Figure 2. Structures of studied biotinylated mannooligosaccharides 1–8 (Argunov et al., 2019).
Isolation of Natural Cellular Mannan and Preparation of FITC-Labeled Mannan
The yeast strain Candida albicans CCY 29-3-100 (serotype A) (CCY Culture Collection of Yeasts, Institute of Chemistry, Center for Glycomics, Slovak Academy of Sciences, Bratislava, Slovakia) was used to isolate and purify cellular mannan from fresh biomass. Mannan was extracted by autoclaving in 0.2 mol/l NaCl (120°C, 700 kPa) for 10 min and purified using precipitation with Fehling reagent according to a previously described method (Peat et al., 1961).
For the preparation of FITC-labeled mannan, C. albicans CCY 29-3-100 mannan (54 mg) was suspended in 1.00 mL of dimethyl sulfoxide and 2.0 μL of pyridine was added. The suspension was heated in a thermoblock at 95°C until the mannan dissolved (3 h). Then, 20 mg of isothiocyanatofluorescein (FITC) was added and heated for another 2 h at 95°C. The reaction was quenched by addition of 10 mL of water, and the result was dialyzed using cellulose membrane tube (cut-off = 14,000, Sigma) against 0.05 % NaHCO3 (1 × 0.9 L, 4 h stirred) and deionized water [8 × 0.9 L, 4 h on stirrer or 12 h in the refrigerator (5°C)] and then lyophilized (FreeZone 18 Liter Console Freeze Dry System, Labconco Corporation, Kansas City, USA).
Preparation of Stock Solutions of Natural Cellular Mannan and Synthetically Prepared Mannooligosaccharides
Stock solutions and different dilutions of natural cellular mannan and glycoconjugate formulas 1–8 were prepared aseptically using pre-sterilized disposable plastic wares and sterile, apyrogenic aqua pro injectione (Fresenius Kabi Italia S.r.l., Verona, Italy). All solutions were prepared in a laminar flow hood and sterilized using a 0.2-μm filter (Q-Max®Syringe filter, Frisenette ApS, Knebel, Denmark) before exposure. The laminar flow cabinet was sterilized with 70% ethanol p.a. and UV for 30 min prior to each experiment. The stock solutions were assayed with EndoLISA® ELISA-based Endotoxin Detection Assay (Hyglos, Bernried am Starnberger See, Germany) and evaluated using the Cytation 5 Imager Multi-Mode Reader (BioTek, Winooski, USA) to ascertain endotoxin-free exposure conditions.
Cell Maintenance and Culture, Cell Exposure
The murine macrophage-like RAW 264.7 cell line was selected in the present study because this cell model has been frequently used in in vitro studies on phagocytosis, cytokine production, and to evaluate potential bioactive substances to predict their effect in vivo.
RAW 264.7 (ATCC®TIB-71TM, ATCC, Manassas, USA) cells were cultured in complete Dulbecco's Modified Eagle Medium for 24 h and 48 h, at 37°C under 5% CO2 atmosphere and 90–100% relative humidity until ~80% confluence. Viability of cells was determined by Trypan Blue dye exclusion method using a TC20TM automated cell counter (Bio-Rad Laboratories, Inc., Hercules, USA). The starting inoculum of 1 × 105 cells/mL/well (98.3% of viable cells) was seeded in a 24-well cell culture plate (Sigma-Aldrich, St. Louis USA) and exposed to 10 and 100 μg per well of glycoconjugates for 24 and 48 h. Cell mitogens Concanavalin A (Con A; 10 μg/mL, Sigma-Aldrich), phytohemagglutinin (PHA; 10 μg/mL, Sigma-Aldrich), pokeweed mitogen (PWM, 1 μg/mL, Sigma-Aldrich), and lipopolysaccharide (LPS; 1 μg/mL, Sigma-Aldrich) were used as positive controls. The cell culture media were separated and stored at −20°C until further use. Cell morphology and viability were assayed before ELISA and evaluation of cytotoxicity. The interaction of FITC-labeled Candida mannan (100 μg/mL) and RAW 264.7 macrophage cells (1 × 105 cells/mL) was evaluated using either light and fluorescence microscopy (AxioVision Imager A.1, magnification 630x; Zeiss, Wetzlar, Germany) or confocal imaging (Axio Observer LSM 880 employing an Airyscan Plan-Apochromat 63x/1.4 oil DIC M27 optical lens and Zen 2 software) with application of 3D Z-stack imaging (Zeiss).
Cell Proliferation and Cytotoxicity
The influence of glycoconjugates on RAW 264.7 cell proliferation and cytotoxicity was evaluated using the cell proliferation assay ViaLightTM plus kit (Lonza, Rockland, ME, USA) according to the manufacturer's recommendations. Cellular ATP was determined with luciferase-based luminescence quantification. The intensity of emitted light was measured using the Cytation 5 Cell Imaging Multi-Mode Reader (BioTek Instruments, Inc.). Light emission was recorded continuously for 1 s and peak values were evaluated and expressed as relative light units (RLU). The values of unexposed cells were considered the baseline. The proliferation index was calculated as the ratio between the stimulated cells (glycoconjugate formula-treated cells) and the baseline proliferation of unexposed cells. Thus, the proliferation index of the negative control, i.e., unexposed cells, was equal to one.
Determination of Interleukins and Growth Factors
The levels of interleukins and growth factors in cell culture supernates induced by exposure with glycoconjugate formulas 1–8 were assayed according to the manufacturer's instructions with Platinum ELISAs® (eBioscience, Thermo Fisher Scientific, Waltham, USA): Mouse IL-12 p70 (MDD 4 pg/mL), Mouse granulocyte-macrophage colony-stimulating factor (GM-CSF;MDD 2 pg/mL), Mouse IL-17 (MDD 1.6 pg/mL), and Mouse IL-6 (MDD 6.5 pg/mL), and Instant ELISAs® (eBioscience): Mouse tumor necrosis factor (TNF)-α (MDD 4 pg/mL), and Mouse IL-10 (MDD 5.28 pg/mL).
To compare the effect of different glycoconjugates on RAW 264.7 macrophage interleukins and growth factors, analyses were performed on raw cytokine concentration data and cytokine concentration data normalized to viable cell counts of untreated control RAW 264.7 cells. The raw concentrations of cytokines determined by ELISA were divided by the RLU [ATP detection systems to quantify viable cells, ViaLightTM plus kit (Lonza, USA)] of living cells in a corresponding sample and multiplied by the RLU of untreated control RAW 264.7 cells.
Statistical Analysis
The experimental results were expressed as mean values ± SD. Normality of data distribution was established according to the Shapiro–Wilk test at the 0.05 level of significance. Statistical comparisons were performed by one-way ANOVA and post-hoc Bonferroni tests. Pearson's correlation coefficient was used to compare the strength of the relationship between immunobiological variables. Results were significant when the differences equaled or exceeded the 95% confidence level (P < 0.05). Statistics were performed using ORIGIN 7.5 PRO software (OriginLab Corporation, Northampton, USA).
Results
Modern chemical methods enable regio- and stereoselective assembling of linear and branched structures similar to C. albicans mannan (Collot et al., 2009; Karelin et al., 2017; Krylov et al., 2017). Here we report the results of our investigation into the structure-driven immunomodulating properties of synthetically prepared mannooligosaccharides in RAW264.7 macrophages using a synthetically prepared panel of biotinylated mannooligosaccharides, formulas 1–8 (Figure 2). These oligomannosides represented antigenic factor 1 (formula 1), factor 34 (formula 2), factor 4 (formula 4), and factor 6 (formulas 5–8) of C. albicans mannan. Side chains related to formula 3 were also found in C. albicans mannan (Kogan et al., 1988), but their antigenic specificity is not yet clear.
As natural Candida mannan (section Isolation of natural cellular mannan and preparation of FITC-labeled mannan) was utilized in all experiments as a comparative substance, evaluation of its interaction with RAW 264.7 cells was essential. Fluorescently labeled natural mannan was used to visualize the cell interaction and endocytotosis of Candida mannan by the murine macrophage RAW 264.7 cells. Evaluation of the interaction was performed with light and fluorescence microscopy (Figure 3A) and 3D Z-stack imaging (Figure 3B). The patterns documented the ingestion of mannan and its inclusion into subcellular compartments.
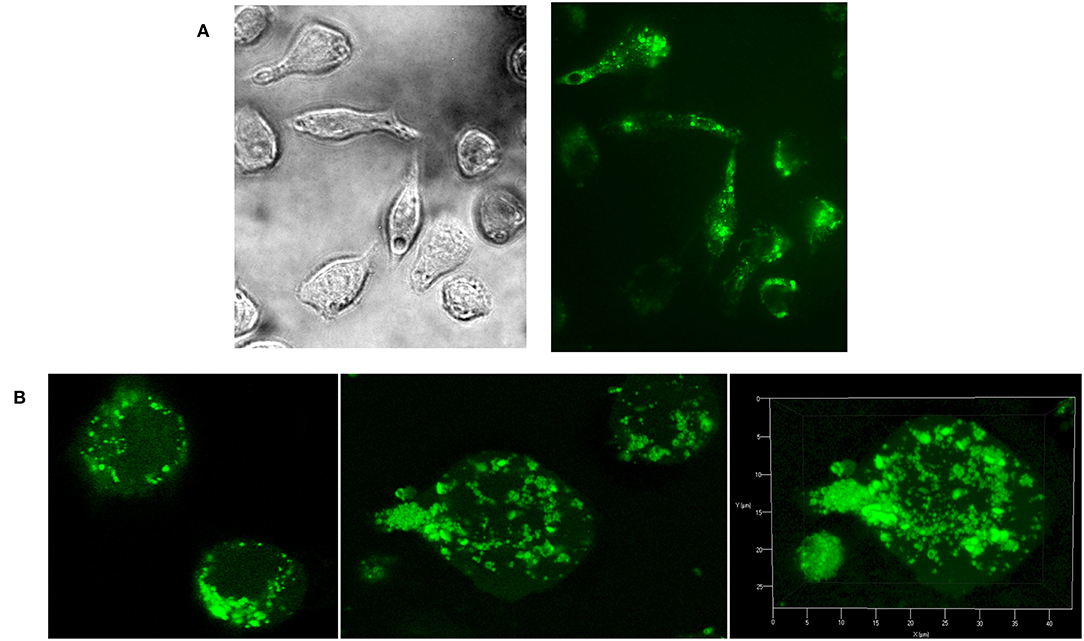
Figure 3. Evaluation of Raw 264.7 cellular interactions with C. albicans mannan- FITC conjugated complex. (A) light and fluorescence microscopy (magnification 630x); (B) confocal microscopy.
Interactions of Natural Mannan and Glycoconjugate Formulas 1–8 With Murine Macrophage Cell Line RAW 264.7, and Influence on Cell Proliferation
The effect of glycoconjugate formulas 1–8 on macrophage cell line RAW 264.7 proliferation was monitored by adenosine triphosphate (ATP) bioluminescence as a marker of cell viability (Figure 4). The lower concentration of glycoconjugate formulas 1–4 (10 μg/mL, Figure 4A) slightly decreased the proliferation of RAW 264.7 macrophages. Improved proliferation was observed for formula 1, which is comprised of three α-1,2-Man units (24 h treatment). The higher concentration of formulas 1–4 (100 μg/mL, Figure 4A), which are comprised exclusively of α-linkages between Man residues, significantly decreased the proliferation of RAW 264.7 macrophages (between 94 and 98% reduction). As opposed to the α-mannooligosaccharides, treatment of RAW 264.7 macrophages with formulas 5–8, which also contain β-1,2-linked Man units, slightly increased proliferation after 24 h, and the increase was more significant after 48 h stimulation (Figure 4B). The highest proliferations were observed for the 10 μg/mL concentration of tetramer formula 5, which contains one terminal β-1,2-linked Man unit (2.1 times higher than control), and hexamer formula 8, which contains a tetrameric block of β-1,2-linked Man units (2.2 times higher than control). The proliferation of RAW 264.7 macrophages treated by glycoconjugates for 48 h was significantly lower (formulas 1–4: P < 0.01, formulas 5–8: P < 0.01) compared with natural C. albicans mannan (M, Figure 4C).
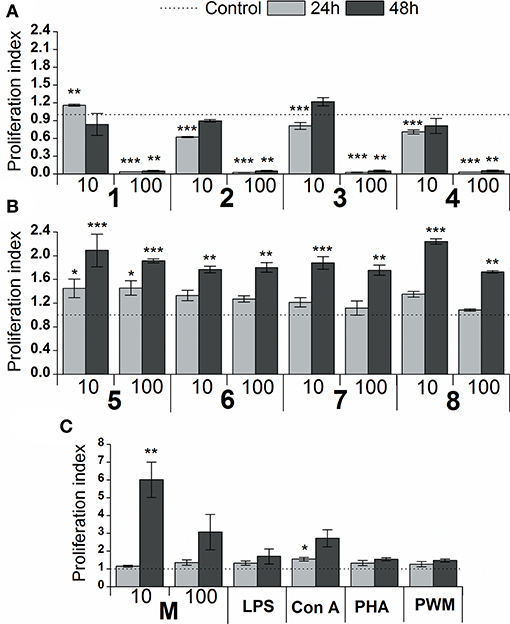
Figure 4. Effect of glycoconjugate formulas 1–8 on proliferation of RAW264.7 macrophages. (A): glycoconjugate formulas 1, 2, 3, and 4; (B): glycoconjugate formulas 5, 6, 7, and 8; 10 and 100 μg/mL); (C): C. albicans mannan (M, 10 and 100 μg/mL), lipopolysaccharide (LPS, 1 μg/mL), Concanavalin A (Con A, 10 μg/mL), phytohemagglutinin (PHA, 10 μg/mL) and pokeweed mitogen (PWM, 1 μg/mL) for 24 and 48 h. Each value represents the mean ± SD of the proliferation index. The statistical significance of differences between stimulated cells and untreated cells are expressed: *0.01 < P < 0.05, **0.001 < P < 0.01, ***P < 0.001.
Cytokine Responses of RAW 264.7 Macrophages in vitro to Glycoconjugate Formulas 1–8
The in vitro stimulatory effect of glycoconjugate formulas 1–8 on RAW 264.7 macrophage cytokine production was determined by the levels of pro-inflammatory cytokines TNFα, IL-6, IL-17, IL-12, anti-inflammatory cytokine IL-10, and haemopoietic growth factor GM-CSF in supernatants obtained from cultures of RAW264.7 macrophages after 24 or 48 h treatments [not normalized raw cytokine concentrations (Supplementary Figures 1, 2) and cytokine concentrations normalized to viable cell counts of untreated control RAW 264.7 cells (Figures 5, 6)].
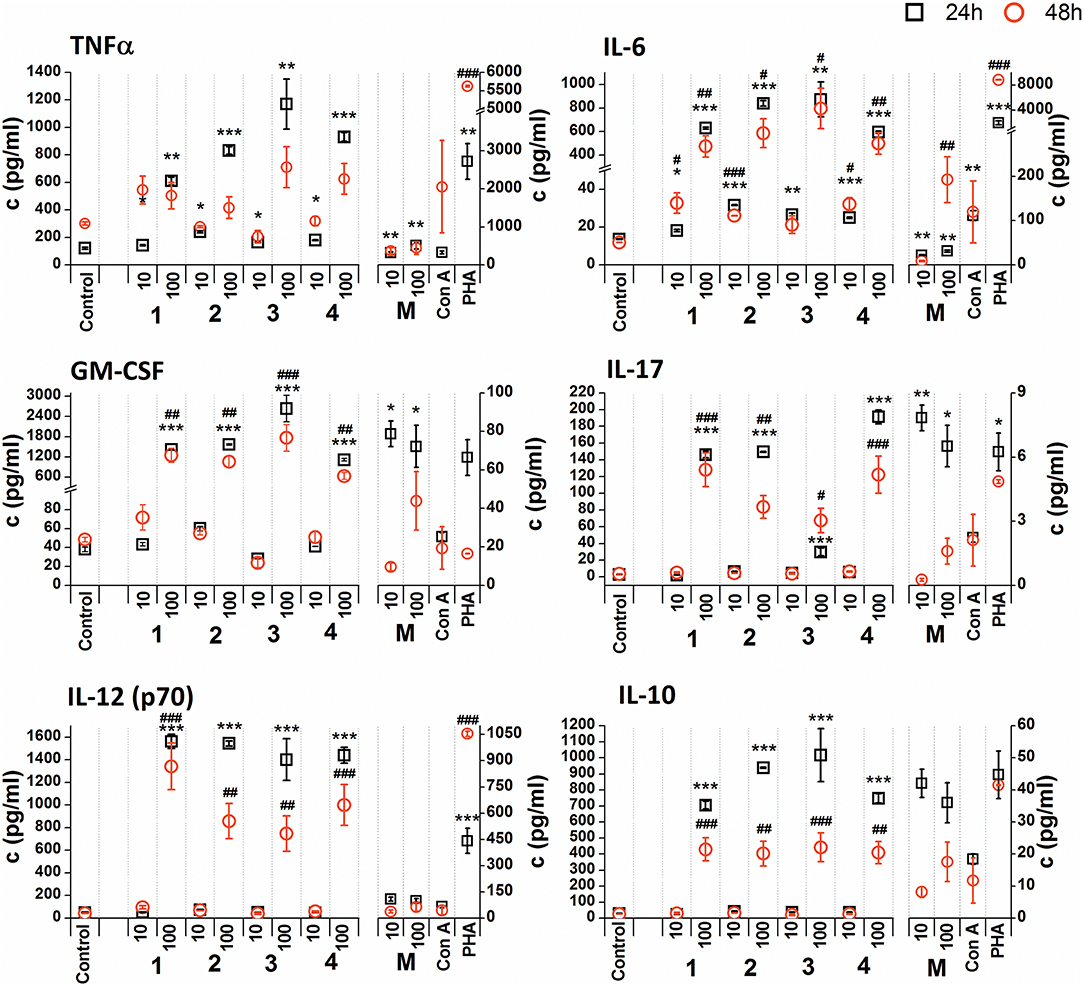
Figure 5. Effect of glycoconjugate formulas 1–4 on RAW 264.7 macrophage cytokine production. Negative control represents untreated cells (Control); positive controls: C. albicans mannan (M, 10 or 100 μg/mL), Concanavaline A (Con A, 10 μg/mL) and phytohemagglutinin (PHA, 10 μg/mL). All data were normalized to the viable cells' count of untreated control cells of each experiment and are presented as Mean ± SD. Tests were carried out in triplicate. The statistical significance of differences between untreated cells and stimulated cells are expressed: 24 h treatment: ***P < 0.001, **0.001 < P < 0.01, *0.01 < P < 0.05; 48 h treatment: ###P < 0.001, ##0.001 < P < 0.01, #0.01 < P < 0.05.
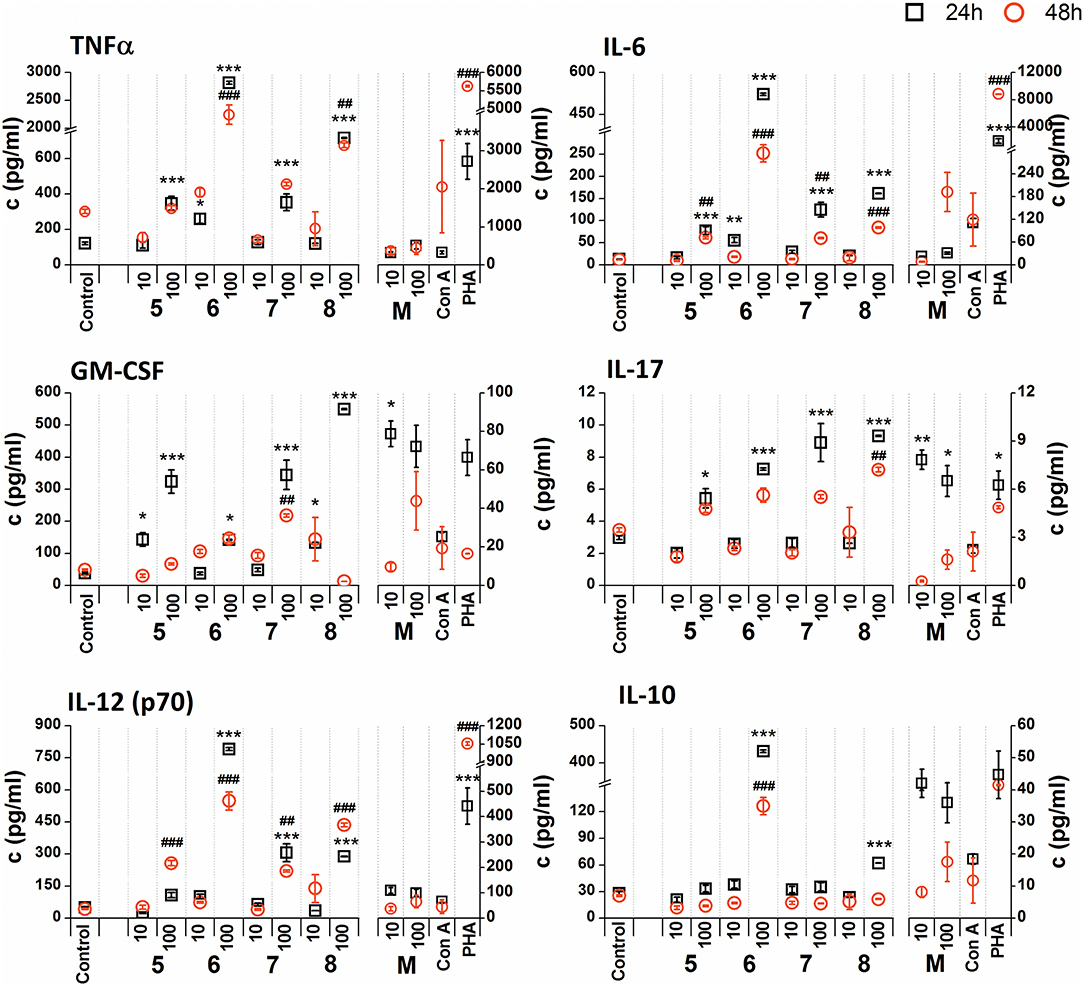
Figure 6. Effect of glycoconjugate formulas 5–8 on RAW 264.7 macrophage cytokine production. Negative control represents untreated cells (Control); positive controls: C. albicans mannan (M, or 100 μg/mL), Concanavaline A (Con A, 10 μg/mL) and phytohemagglutinin (PHA, 10 μg/mL). All data were normalized to the viable cells' count of untreated control cells of each experiment and are presented as mean ± SD. Tests were carried out in triplicate. The statistical significance of differences between untreated cells and stimulated cells are expressed as follows: 24 h treatment: ***P < 0.001, **0.001 < P < 0.01, *0.01 < P < 0.05; 48 h treatment: ###P < 0.001, ##0.001 < P < 0.01, #0.01 < P < 0.05.
Non-normalized raw cytokine concentrations data showed that stimulation of RAW 264.7 cells with the lower concentration of glycoconjugate formulas 1–4 (10 μg/mL), which contain linked Man residues, resulted in a slight increase of TNFα production; maximal effect was observed for formula 1 (24 h treatment: 1.37-fold increase and 48 h treatment: 1.48-fold increase, Supplementary Figure 1). The stimulation of RAW 264.7 macrophages with the higher concentration of glycoconjugate formulas (100 μg/mL) significantly decreased TNFα production (more than 70% decrease compared to the control). However, IL-6 and GM-CSF production showed different concentration dependencies. The higher concentration of glycoconjugate formulas 1–4 (100 μg/mL) induced comparable or higher IL-6 and GM-CSF secretion than the lower concentration (10 μg/mL) (Supplementary Figure 1). The highest IL-6 and GM-CSF release was observed for glycoconjugate formula 3 (IL-6: 3.2-fold increase, GM-CSF: 1.9-fold increase).
Glycoconjugate formulas 1–4 induced increased IL-17 production (Supplementary Figure 1). The higher concentration of glycoconjugate formulas 1–4 (100 μg/mL) induced higher IL-17 secretion, except for glycoconjugate formula 3, for which IL-17 production declined with increasing glycoconjugate concentration (Supplementary Figure 1). Production of IL-12 showed a structure related dependency (Supplementary Figure 1). The most effective IL-12 inducer was glycoconjugate formula 1, and induction efficacy declined slightly with increasing number of mannose units in glycoconjugate formulas 1–4 (Supplementary Figure 1). Glycoconjugate formulas 1–4 did not significantly influence IL-10 production (non-normalized data, Supplementary Figure 1). The results indicated a higher proinflammatory response associated with glycoconjugate formulas 1–4, containing linked Man residues, with significant reduction of RAW 264.7 macrophage proliferation.
The stimulation of RAW 264.7 macrophages with glycoconjugate formulas 5–8 showed a different impact on TNFα production compared to glycoconjugate formulas 1–4 (Supplementary Figure 2). Higher TNFα production was observed during the shorter exposure period (24 h). The higher tested concentration (100 μg/mL) significantly increased TNFα production, with maximal efficacy for glycoconjugate formula 6 (24 h: 29.4-fold increase, 48 h: 13.4-fold increase compared to the control). Production of IL-6, GM-CSF, IL-17, and IL-12 also showed a concentration dependency, with higher efficacy for the higher concentrations of glycoconjugate formulas 5–8 (100 μg/mL). The highest IL-6 secretion was induced by glycoconjugate formula 6 (Supplementary Figure 2, 24 h: 48.8-fold increase, 48 h: 40.0-fold increase, compared to the control). Additionally, glycoconjugate formula 6 induced a strong increase in IL-17, IL-12, and IL-10 production (Supplementary Figure 2). Stimulation with β-mannooligosaccharides 6 and 8 for 24 h markedly increased the production of TNFα (100 μg/mL, P < 0.001), IL-6 (100 μg/mL, P < 0.001), IL-12 (100 μg/mL, P < 0.001), and IL10 (100 μg/mL, P < 0.001) compared with natural C. albicans mannan.
Due to the tested glycoconjugates having a significant effect on RAW 264.7 macrophage proliferation, especially for glycoconjugate formulas 1–4 that contain α-linked Man residues, the raw data of cytokine concentrations in the culture supernatants were normalized to the viable cell counts of untreated control RAW 264.7 cells for each experiment. We observed that the normalization of cytokine concentration data showed no significant trend change for stimulation of RAW 264.7 macrophages with the β-mannooligosaccharide glycoconjugates (formulas 5–8) (Figure 6). Out of all tested β-mannooligosaccharide glycoconjugates, the most effective cytokine inducers were glycoconjugate formulas 6 and 8. The highest TNFα (24 h: 23.2-fold increase), IL-6 (24 h, 38.5-fold increase), IL-12 (24 h: 15.6-fold increase), and IL-10 (24 h: 15.3-fold increase) secretion was induced by glycoconjugate formula 6. The GM-CSF (24 h: 14.5-fold increase) and IL-17 (24 h: 3.1-fold increase) was most effectively induced by β-mannooligosaccharide glycoconjugate formula 8. Normalization of the cytokine concentration data after stimulation with α-mannooligosaccharide glycoconjugates (formulas 1–4) (Figure 5) accentuated the release of cytokines induced by the higher concentration of glycoconjugates (100 μg/mL). We observed significant capability to induce TNFα, IL-6, GM-CSF, IL-17, and IL-12 production accompanied by an increase of IL-10 after stimulation with all α-mannooligosaccharide glycoconjugates, induced especially with higher 100 μg/mL concentration, that strongly reduced the proliferation of RAW 264.7 cells. The highest production of TNFα, IL-6, GM-CSF, and IL-10 was observed after the shorter exposure time (24 h) with glycoconjugate formula 3.
The influence of glycoconjugate formulas 1–8 on Th1 and Th2 polarization was revealed based on the TNFα (Th1) to IL-10 (Th2) and IL-6 (Th2) to IL-10 (Th2) ratios (Figure 7). Th1 dominance was represented by a higher ratio, while a lower ratio expressed a Th2 dominated environment. Concerning the ratios following 24 and 48 h exposures with 100 and 10 μg/mL of glycoconjugate formulas 1–4, Th1 dominance based on the TNFα/IL-10 ratio was revealed for conjugate formulas 1 and 4, while conjugate formulas 2 and 3 exerted Th1 dominance with higher TNFα/IL-10 ratios over IL-6/IL-10 ratios only at the lower concentration (10 μg/mL) after 48 h exposure. For conjugate formulas 5–8, the values of the TNFα/IL-10 ratios overcame the values of the IL-6/IL-10 ratios following 24 and 48 h exposures with both concentrations for all conjugates, reflecting Th1 dominance.
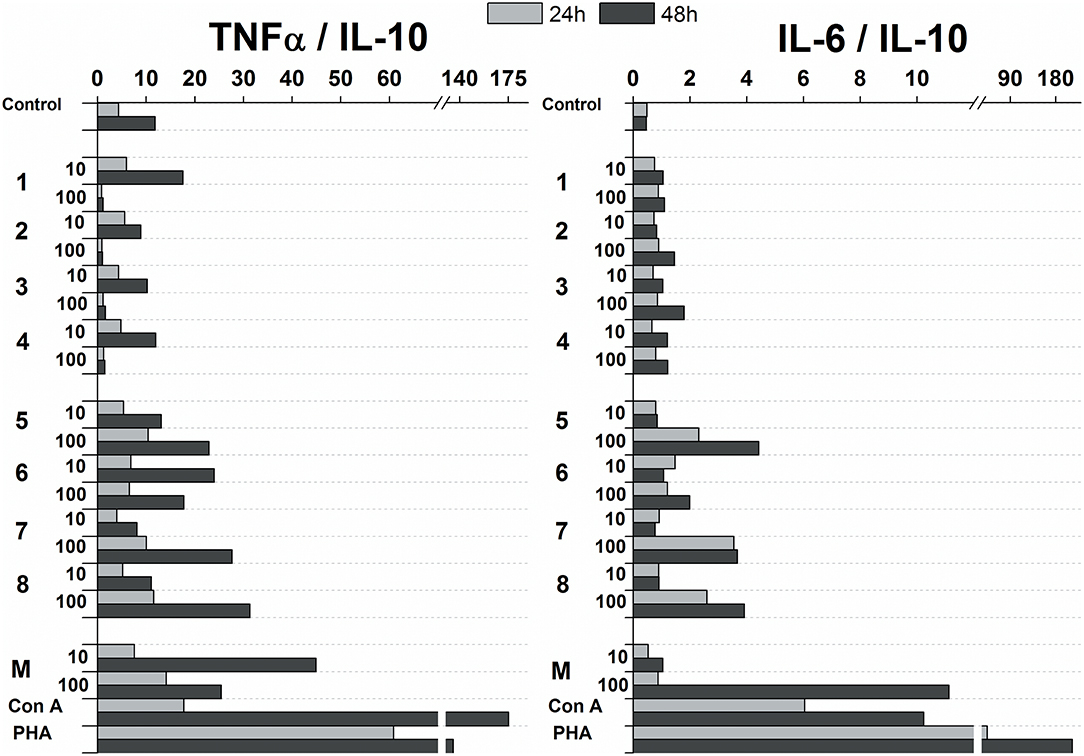
Figure 7. Influence of glycoconjugate formulas 1–8 on Th1 and Th2 polarization based on TNFα to IL-10 and IL-6 to IL-10 ratios.
The resulting in vitro proinflammatory effect of glycoconjugate formulas 5-8, containing terminal β-mannosyls, overcame that of the α-mannooligosaccharides. This was supported by statistically insignificant correlations between the release of proinflammatory cytokines following 24 and 48 h exposures with α-mannooligosaccharides. Significant overall correlations were determined between the release of proinflammatory cytokines induced by individual β-mannooligosaccharides glycoconjugate formulas following 24 h exposure: TNFα and IL-6 (R = 0.994 p = 5.38 ×10−7), TNFα and IL-12 (R = 0.969 p = 6.71 ×10−5), and IL-12 and IL-6 (R = 0.989 p = 2.61 ×10−6). After 48 h, a significant correlation was also revealed between IL-17 and IL-12 (R = 0.877 p = 0.0042).
Discussion
Several attempts have been made to synthesize relevant mannan epitopes with immunobiological effectiveness. Synthetically prepared mannooligosaccharides mimicking Candida antigenic factors (Karelin et al., 2010, 2015, 2016, 2017) represent promising study models to establish the immunomodulating activity of such formulas on humoral and cellular immunity for subcellular anti-Candida vaccine construction (Paulovicova et al., 2010, 2012, 2014; Paulovicova L. et al., 2013).
The immunobiological importance and vaccination potency of synthetically prepared β-1,2-mannopyranosyl trisaccharide mimicking the structure of the C. albicans cell surface epitope has previously been studied (Xin et al., 2008, 2012; Costello and Bundle, 2012; Cartmell et al., 2015; Bundle et al., 2018). Next, a novel tetrasaccharide construct consisting of β-1,2-mannopyranosyl trisaccharide and α-mannopyranoside was designed and suggested as a model of the C. albicans phosphodiester epitope (Dang et al., 2012). Glycoarrays formed by biotinylated oligosaccharides loaded on streptavidin-coated surfaces were previously shown to be indispensable instruments for the investigation of carbohydrate antigen recognition by immune cells (Komarova et al., 2015, 2018; Akhmatova et al., 2016; Paulovicova et al., 2016, 2017; Kurbatova et al., 2017; Argunov et al., 2019; Schubert et al., 2019).
Moreover, the sera reactivity and determination of antigen-specific isotypic antibodies against synthetically prepared mannooligosaccharides were evaluated in a cohort of patients with vulvovaginal candidosis (Karelin et al., 2016; Paulovicova et al., 2016, 2017). Postvaccination antisynthetic heptamannoside polyclonal sera inhibited growth of the azole-resistant clinical strain C. albicans CCY 29-3-164 and reduced the number of colony-forming units throughout an experimental mucosal infection (Paulovicova E. et al., 2013). Next, sera cytokine patterns of Th1/Th2/Th17 polarization of immune responses by synthetic oligosaccharide–BSA conjugates revealed a tight structure-activity relationship (Paulovicova E. et al., 2013; Paulovicova et al., 2017).
Here, the proliferating and cytokine-inducing activities of a series of synthetically prepared nanopolymers mimicking native C. albicans cell wall immunogenic moieties were studied using RAW264.7 cell exposure (Figure 4). The cell proliferation results revealed almost immunoinhibitory activity of α- mannoside formulas 1–4 (trisaccharide through hexasaccharide), with more pronounced activity with increasing concentration (P < 0.001), in contrast with native C. albicans cell wall mannan (Figure 4). These findings concur with previously published studies (Podzorski et al., 1989, 1990) that reported the immunoinhibitory influence of members of a family of mannose oligosaccharides (disaccharide through hexasaccharide) derived from cetyltrimethylammonium bromide (CTAB) mannan (native C. albicans mannan prepared by complexation with CTAB). CTAB mannan was a potent stimulator of lymphoproliferative when added to human peripheral blood mononuclear cells (PMBCs) from donors responsive to Candida; it had no inhibitory influence on lymphoproliferation induced by Candida or other antigens. Two major oligomannosyl components, mannobiose and mannotriose, of CTAB mannan with an inhibitory effect on cell proliferation were demonstrated to be bound mainly through α(1,2) linkages (Hayette et al., 1992). In contrast, synthetically prepared mannooligosaccharide formulas 5–8 with terminal β-mannosyl units (Figure 3) exerted a stimulatory effect on RAW264.7 cell proliferation (P < 0.001). Thus, the immunobiological properties of the studied mannooligosaccharides are dose- and structure-dependent. Cell release of interleukins and growth factors associated with inflammation and proliferation was induced by mannooligomers to different extents depending on the oligomer structures (normalized data: Figures 5, 6, not normalized data: Supplementary Figures 1, 2).
Upregulation of cytokines such as TNFα, IL-6, IL-12, GM-CSF was more evident with mannooligosaccharides with terminal β-mannosyl units. Acceleration of secretion of anti-inflammatory cytokine IL-10 with Th1-inhibiting properties was also revealed with β-mannooligosaccharides (Supplementary Figure 2). Association between pro- and anti-inflammatory cytokines, in addition to Th1, Th2 and Th17 polarization, is an important prerequisite for the assessment of immunogenic substance behavior. The influence of glycoconjugate formulas 1–8 on Th1 and Th2 polarization, based on TNFα to IL-10 and IL-6 to IL-10 ratios (Figure 7), resulted in a predominant Th1 immune response. Th1 dominance, represented by higher TNFα/IL-10 and IL-6/IL-10 ratios with higher dose and following prolonged treatment, was revealed with tested 1–8 formulas, and was more evident for formulas 5–8 with β-terminal mannosyls; the Th2 dominated environment was not determined. The observed immune response was tightly associated with dose, exposure time, and selected signature cytokines. The TNFα/IL-10 ratio was more descriptive than the IL-6/IL-10 ratio, presumably due to dual IL-6 roles, i.e., anti-inflammatory activities of IL-6 are mediated by classic signaling, whereas proinflammatory responses of IL-6 are mediated by trans-signaling (Scheller et al., 2011). Saijo et al. reported induced release of cytokines, such as IL-12p40, IL-6, TNFα, and IL-10, from wild-type bone marrow-derived dendritic cells (BMDCs) by treatment with C. albicans water soluble fraction (CAWs) and C. albicans mannans (Saijo et al., 2010). Moreover, they also observed the secretion of yeast- and hyphae-specific cytokines following cell exposure with both C. albicans morphoforms. C. albicans mannan, glucomannoprotein and phospholipomannan, containing β-1,2 oligomannosides, induced TNFα in association with degree of polymerization (DP). Jouault et al. noted that TNFα-release occurred most in the presence of relatively long chains of β-oligomannosides (i.e., an oligomannoside comprised of eight mannose units was superior to shorter chains, and oligomannosides of less than four mannose units were not active) (Jouault et al., 1995). Evidently a minimal DP of 4 was necessary to induce production of cytokine (Poulain et al., 1997). With synthetically prepared α- and β- oligomannosides, effective TNFα release was triggered by trimannosides. The ability of β-oligomannosides to induce release of TNFα was also demonstrated by Cutler (2001).
Additionally, cell exposure to conjugate formula 6, which comprises 2 β and 3α mannosyls, exerted the highest media release of IL-12p70, IL-6, TNFα, and IL-10 (Figure 6). Interestingly, conjugate formula 8, with 4β and 2α-linked mannosyls, induced higher IL-17 and regulatory GM-CSF cell release than the other β-oligomannosides (Figure 6).
Conclusions
Our data suggest an immunobiological role for synthesized mannooligosaccharides that closely resemble Candida cell wall mannooligomers. The observed Th1/Th2/Th17 immune responses were tightly associated with structure, dose, exposure time, and selected signature cytokines. Glycoconjugate formulas 5–8, with terminal β-mannosyl-units, tended to be more potent than glycoconjugate formulas 1–4 in terms of Candida relevant cytokines IL-12 p70, IL-17, GM-CSF, IL-6, and TNFα induction and cell proliferation, and this tendency was associated with structural differences between the studied glycoconjugate formulas. Obtained results warrant further systematic investigation of the immunological properties of carbohydrate antigens of the Candida cell wall toward the selection of efficient structures suitable for application as immunomodulative agents either for in vitro Candida diagnostics or prospectively for subcellular anti-Candida vaccine design.
Data Availability Statement
All datasets generated for this study are included in the article/Supplementary Material.
Author Contributions
EP, LP, and NN contributed to the conception and design of the study, performed the immunobiological research and analyzed data, acquired funding, and prepared the original draft. PF performed the modification and characterization of mannan. AK, YT, and VK performed the chemical syntheses and analyzed data. All authors contributed to manuscript revision, read, and approved the submitted version.
Funding
The synthetic part of this work was supported by the Russian Science Foundation (grant 19-73-30017), while the immunological part of the work was supported by the Scientific Grant Agency of the Slovak Republic VEGA (project 2/0098/17) and the Slovak Research and Development Agency (contract N° APVV-15-0161).
Conflict of Interest
The authors declare that the research was conducted in the absence of any commercial or financial relationships that could be construed as a potential conflict of interest.
Supplementary Material
The Supplementary Material for this article can be found online at: https://www.frontiersin.org/articles/10.3389/fcimb.2019.00378/full#supplementary-material
References
Adiguzel, N., Karakurt, Z., Gungor, G., Yazicioglu Mocin, O., Acarturk, E., Sogukpinar, O., et al. (2010). Mortality rates and risk factors associated with nosocomial Candida infection in a respiratory intensive care unit. Tuberkuloz ve toraks 58, 35–43.
Akhmatova, N. K., Kurbatova, E. A., Akhmatov, E. A., Egorova, N. B., Logunov, D. Y., Gening, M. L., et al. (2016). The effect of a BSA conjugate of a synthetic hexasaccharide related to the fragment of capsular polysaccharide of Streptococcus pneumoniae type 14 on the activation of innate and adaptive immune responses. Front. Immunol. 7:248. doi: 10.3389/fimmu.2016.00248
Angiolella, L., Stringaro, A. R., De Bernardis, F., Posteraro, B., Bonito, M., Toccacieli, L., et al. (2008). Increase of virulence and its phenotypic traits in drug-resistant strains of Candida albicans. Antimicrob. Agents Chemother. 52, 927–936. doi: 10.1128/AAC.01223-07
Apostolopoulos, V., Pietersz, G. A., Tsibanis, A., Tsikkinis, A., Drakaki, H., Loveland, B. E., et al. (2006). Pilot phase III immunotherapy study in early-stage breast cancer patients using oxidized mannan-MUC1 [ISRCTN71711835]. Breast Cancer Res. 8:R27. doi: 10.1186/bcr1505
Argunov, D. A., Trostianetskaia, A. S., Krylov, V. B., Kurbatova, E. A., and Nifantiev, N. E. (2019). Convergent synthesis of oligosaccharides structurally related to galactan I and galactan II of Klebsiella pneumoniae and their use in screening of antibody specificity. Eur. J. Org. Chem. 2019, 4226–4232 doi: 10.1002/ejoc.201900389
Ashman, R. B., and Papadimitriou, J. M. (1995). Production and function of cytokines in natural and acquired immunity to Candida albicans infection. Microbiol. Rev. 59, 646–672.
Bartheldyova, E., Turanek Knotigova, P., Zachova, K., Masek, J., Kulich, P., Effenberg, R., et al. (2019). N-Oxy lipid-based click chemistry for orthogonal coupling of mannan onto nanoliposomes prepared by microfluidic mixing: synthesis of lipids, characterisation of mannan-coated nanoliposomes and in vitro stimulation of dendritic cells. Carbohydr. Polym. 207, 521–532. doi: 10.1016/j.carbpol.2018.10.121
Beenhouwer, D. O. (2018). “Chapter 17 - Molecular basis of diseases of immunity,” in Molecular Pathology, 2nd Edn. eds W. B. Coleman and G. J. Tsongalis (London: Academic Press), 329–345. doi: 10.1016/B978-0-12-802761-5.00017-1
Brown, G. D. (2006). Dectin-1: a signalling non-TLR pattern-recognition receptor. Nat. Rev. Immunol. 6, 33–43. doi: 10.1038/nri1745
Brown, G. D., and Gordon, S. (2001). Immune recognition. A new receptor for beta-glucans. Nature 413, 36–37. doi: 10.1038/35092620
Brown, G. D., Herre, J., Williams, D. L., Willment, J. A., Marshall, A. S., and Gordon, S. (2003). Dectin-1 mediates the biological effects of beta-glucans. J. Exp. Med. 197, 1119–1124. doi: 10.1084/jem.20021890
Brown, G. D., Taylor, P. R., Reid, D. M., Willment, J. A., Williams, D. L., Martinez-Pomares, L., et al. (2002). Dectin-1 is a major beta-glucan receptor on macrophages. J. Exp. Med. 196, 407–412. doi: 10.1084/jem.20020470
Bundle, D. R., Paszkiewicz, E., Elsaidi, H. R. H., Mandal, S. S., and Sarkar, S. (2018). A three component synthetic vaccine containing a beta-Mannan T-cell peptide epitope and a beta-Glucan dendritic cell ligand. Molecules 23:E1961. doi: 10.3390/molecules23081961
Cartmell, J., Paszkiewicz, E., Dziadek, S., Tam, P. H., Luu, T., Sarkar, S., et al. (2015). Synthesis of antifungal vaccines by conjugation of beta-1,2 trimannosides with T-cell peptides and covalent anchoring of neoglycopeptide to tetanus toxoid. Carbohydr. Res. 403, 123–134. doi: 10.1016/j.carres.2014.06.024
Collot, M., Loukou, C., and Mallet, J.-M. N.E N. (2009). “Chemical synthesis of the oligosaccharidic fragments of yeast mannans,” in Progress in the Synthesis of Complex Carbohydrate Chains of Plant and Microbial Polysaccharides, ed N. E. Nifantiev (Trivandrum: Transworld Research Network, 371–398.
Cortés, J. A., and Corrales, F. I. (2018). “Invasive candidiasis: epidemiology and risk factors,” in Fungal Infection, eds É. S. d. Loreto and J. S. M. Tondolo (London: IntechOpe).
Costello, C., and Bundle, D. R. (2012). Synthesis of three trisaccharide congeners to investigate frame shifting of beta1,2-mannan homo-oligomers in an antibody binding site. Carbohydr. Res. 357, 7–15. doi: 10.1016/j.carres.2012.03.019
Cunha, C., and Carvalho, A. (2012). Host genetics and invasive fungal diseases: towards improved diagnosis and therapy? Expert Rev. Anti Infect. Ther. 10, 257–259. doi: 10.1586/eri.12.3
Cutler, J. E. (2001). N-glycosylation of yeast, with emphasis on Candida albicans. Med. Mycol. 39 (Suppl. 1), 75–86. doi: 10.1080/mmy.39.1.75.86
Cutler, J. E. (2005). Defining criteria for anti-mannan antibodies to protect against candidiasis. Curr. Mol. Med. 5, 383–392. doi: 10.2174/1566524054022576
Da Silva, C. A., Chalouni, C., Williams, A., Hartl, D., Lee, C. G., and Elias, J. A. (2009). Chitin is a size-dependent regulator of macrophage TNF and IL-10 production. J. Immunol. 182, 3573–3582. doi: 10.4049/jimmunol.0802113
Da Silva, C. A., Hartl, D., Liu, W., Lee, C. G., and Elias, J. A. (2008). TLR-2 and IL-17A in chitin-induced macrophage activation and acute inflammation. J. Immunol. 181, 4279–4286. doi: 10.4049/jimmunol.181.6.4279
Dang, A. T., Johnson, M. A., and Bundle, D. R. (2012). Synthesis of a Candida albicans tetrasaccharide spanning the beta1,2-mannan phosphodiester alpha-mannan junction. Org. Biomol. Chem. 10, 8348–8360. doi: 10.1039/c2ob26355f
Dennehy, K. M., Ferwerda, G., Faro-Trindade, I., Pyz, E., Willment, J. A., Taylor, P. R., et al. (2008). Syk kinase is required for collaborative cytokine production induced through Dectin-1 and Toll-like receptors. Eur. J. Immunol. 38, 500–506. doi: 10.1002/eji.200737741
Ernst, J. F., and Pla, J. (2011). Signaling the glycoshield: maintenance of the Candida albicans cell wall. Int. J. Med. Microbiol. 301, 378–383. doi: 10.1016/j.ijmm.2011.04.003
Erwig, L. P., and Gow, N. A. (2016). Interactions of fungal pathogens with phagocytes. Nat. Rev. Microbiol. 14, 163–176. doi: 10.1038/nrmicro.2015.21
Esteban, A., Popp, M. W., Vyas, V. K., Strijbis, K., Ploegh, H. L., and Fink, G. R. (2011). Fungal recognition is mediated by the association of dectin-1 and galectin-3 in macrophages. Proc. Natl. Acad. Sci. U.S.A. 108, 14270–14275. doi: 10.1073/pnas.1111415108
Forsberg, K., Woodworth, K., Walters, M., Berkow, E. L., Jackson, B., Chiller, T., et al. (2019). Candida auris: the recent emergence of a multidrug-resistant fungal pathogen. Med. Mycol. 57, 1–12. doi: 10.1093/mmy/myy054
Fukazawa, Y., Kagaya, K., Suzuki, M., and Shinoda, T. (1997). “Serological specificity and biological activity of cell wall polysaccharides of medically important yeasts,” in Fungal Cells in Biodefense Mechanism, eds S. Suzuki and M. Suzuki (Tokyo: Saigon Publishing, 17–24.
Gantner, B. N., Simmons, R. M., Canavera, S. J., Akira, S., and Underhill, D. M. (2003). Collaborative induction of inflammatory responses by dectin-1 and Toll-like receptor 2. J. Exp. Med. 197, 1107–1117. doi: 10.1084/jem.20021787
Gow, N. A. R., Latge, J. P., and Munro, C. A. (2017). The fungal cell wall: structure, biosynthesis, and function. Microbiol. Spectr. 5, 1–25. doi: 10.1128/microbiolspec.FUNK-0035-2016
Hall, R. A., Bates, S., Lenardon, M. D., Maccallum, D. M., Wagener, J., Lowman, D. W., et al. (2013). The Mnn2 mannosyltransferase family modulates mannoprotein fibril length, immune recognition and virulence of Candida albicans. PLoS Pathog. 9:e1003276. doi: 10.1371/journal.ppat.1003276
Hall, R. A., and Gow, N. A. (2013). Mannosylation in Candida albicans: role in cell wall function and immune recognition. Mol. Microbiol. 90, 1147–1161. doi: 10.1111/mmi.12426
Han, Y., Ulrich, M. A., and Cutler, J. E. (1999). Candida albicans mannan extract-protein conjugates induce a protective immune response against experimental candidiasis. J. Infect. Dis. 179, 1477–1484. doi: 10.1086/314779
Harris, M., Mora-Montes, H. M., Gow, N. A., and Coote, P. J. (2009). Loss of mannosylphosphate from Candida albicans cell wall proteins results in enhanced resistance to the inhibitory effect of a cationic antimicrobial peptide via reduced peptide binding to the cell surface. Microbiology 155, 1058–1070. doi: 10.1099/mic.0.026120-0
Hayette, M. P., Strecker, G., Faille, C., Dive, D., Camus, D., Mackenzie, D. W., et al. (1992). Presence of human antibodies reacting with Candida albicans O-linked oligomannosides revealed by using an enzyme-linked immunosorbent assay and neoglycolipids. J. Clin. Microbiol. 30, 411–417.
Ito, J. I. (2011). T cell immunity and vaccines against invasive fungal diseases. Immunol. Invest. 40, 825–838. doi: 10.3109/08820139.2011.595472
Jouault, T., El Abed-El Behi, M., Martinez-Esparza, M., Breuilh, L., Trinel, P. A., Chamaillard, M., et al. (2006). Specific recognition of Candida albicans by macrophages requires galectin-3 to discriminate Saccharomyces cerevisiae and needs association with TLR2 for signaling. J. Immunol. 177, 4679–4687. doi: 10.4049/jimmunol.177.7.4679
Jouault, T., Lepage, G., Bernigaud, A., Trinel, P. A., Fradin, C., Wieruszeski, J. M., et al. (1995). Beta-1,2-linked oligomannosides from Candida albicans act as signals for tumor necrosis factor alpha production. Infect. Immun. 63, 2378–2381.
Karelin, A. A., Tsvetkov, Y. E., Kogan, G., Bystricky, S., and Nifantiev, N. E. (2007). Synthesis of oligosaccharide fragments of mannan from Candida albicans cell wall and their BSA conjugates. Russ. J. Bioorg. Chem. 33, 110–121. doi: 10.1134/S106816200701013X
Karelin, A. A., Tsvetkov, Y. E., and Nifantiev, N. E. (2017). Synthesis of oligosaccharides related to cell wall polysaccharides of the fungi Candida and Aspergillus. Russ. Chem. Rev. 86, 1073–1126. doi: 10.1070/RCR4750
Karelin, A. A., Tsvetkov, Y. E., Paulovičová, E., Paulovičová, L., and Nifantiev, N. E. (2015). Blockwise synthesis of a pentasaccharide structurally related to the mannan fragment from the Candida albicans cell wall corresponding to the antigenic factor 6. Russ. Chem. Bull. 64, 2942–2948. doi: 10.1007/s11172-015-1251-5
Karelin, A. A., Tsvetkov, Y. E., Paulovicova, E., Paulovicova, L., and Nifantiev, N. E. (2016). A blockwise approach to the synthesis of (1 -> 2)-linked oligosaccharides corresponding to fragments of the acid-stable beta-mannan from the Candida albicans cell wall. Eur. J. Org. Chem. 2016, 1173–1181. doi: 10.1002/ejoc.201501464
Karelin, A. A., Tsvetkov, Y. E., Paulovicova, L., Bystricky, S., Paulovicova, E., and Nifantiev, N. E. (2010). Synthesis of 3,6-branched oligomannoside fragments of the mannan from Candida albicans cell wall corresponding to the antigenic factor 4. Carbohydr. Res. 345, 1283–1290. doi: 10.1016/j.carres.2009.11.012
Kavishwar, A., and Shukla, P. K. (2006). Candidacidal activity of a monoclonal antibody that binds with glycosyl moieties of proteins of Candida albicans. Med. Mycol. 44, 159–167. doi: 10.1080/13693780500266038
Klis, F. M., de Groot, P., and Hellingwerf, K. (2001). Molecular organization of the cell wall of Candida albicans. Med. Mycol. 39 (Suppl. 1), 1–8. doi: 10.1080/744118876
Kogan, G., Pavliak, V., and Masler, L. (1988). Structural studies of mannans from the cell-walls of the pathogenic yeasts Candida albicans Serotype-A and serotype-B and Candidia parapsilosis. Carbohydr. Res. 172, 243–253. doi: 10.1016/S0008-6215(00)90858-9
Komarova, B. S., Orekhova, M. V., Tsvetkov, Y. E., Beau, R., Aimanianda, V., Latge, J. P., et al. (2015). Synthesis of a pentasaccharide and neoglycoconjugates related to fungal alpha-(1 -> 3)-glucan and their use in the generation of antibodies to trace Aspergillus fumigatus cell wall. Chem. A Eur. J. 21, 1029–1035. doi: 10.1002/chem.201404770
Komarova, B. S., Wong, S. S. W., Orekhova, M. V., Tsvetkov, Y. E., Krylov, V. B., Beauvais, A., et al. (2018). Chemical synthesis and application of biotinylated oligo-alpha-(1 –> 3)-d-glucosides to study the antibody and cytokine response against the cell wall alpha-(1 –> 3)-d-glucan of Aspergillus fumigatus. J. Org. Chem. 83, 12965–12976. doi: 10.1021/acs.joc.8b01142
Krylov, V. B., Paulovicova, L., Paulovicova, E., Tsvetkov, Y. E., and Nifantiev, N. E. (2017). Recent advances in the synthesis of fungal antigenic oligosaccharides. Pure Appl. Chem. 89, 885–898. doi: 10.1515/pac-2016-1011
Krylov, V. B., Petruk, M. I., Grigoryev, I. V., Lebedin, Y. S., Glushko, N. I., Khaldeeva, E. V., et al. (2018a). Study of the carbohydrate specificity of antibodies against Aspergillus fumigatus using the library of synthetic mycoantigens. Russ. J. Bioorg. Chem. 44, 80–89. doi: 10.1134/S1068162017060073
Krylov, V. B., Petruk, M. I., Karelin, A. A., Yashunuskii, D. V., Tsvetkov, Y. E., Glushko, N. I., et al. (2018b). Carbohydrate specificity of antibodies against yeast preparations of Saccharomyces cerevisiae and Candida krusei. Appl. Biochem. Microbiol. 54, 665–669. doi: 10.1134/S0003683818060108
Kurbatova, E. A., Akhmatova, N. K., Akhmatova, E. A., Egorova, N. B., Yastrebova, N. E., Sukhova, E. V., et al. (2017). Neoglycoconjugate of tetrasaccharide representing one repeating unit of the Streptococcus pneumoniae type 14 capsular polysaccharide induces the production of opsonizing IgG1 antibodies and possesses the highest protective activity as compared to hexa- and octasaccharide conjugates. Front. Immunol. 8:659. doi: 10.3389/fimmu.2017.00659
Li, X., Hou, Y., Yue, L., Liu, S., Du, J., and Sun, S. (2015). Potential targets for antifungal drug discovery based on growth and virulence in Candida albicans. Antimicrob. Agents Chemother. 59, 5885–5891. doi: 10.1128/AAC.00726-15
Linden, J. R., De Paepe, M. E., Laforce-Nesbitt, S. S., and Bliss, J. M. (2013). Galectin-3 plays an important role in protection against disseminated candidiasis. Med. Mycol. 51, 641–651. doi: 10.3109/13693786.2013.770607
Lowman, D. W., Ensley, H. E., Greene, R. R., Knagge, K. J., Williams, D. L., and Kruppa, M. D. (2011). Mannan structural complexity is decreased when Candida albicans is cultivated in blood or serum at physiological temperature. Carbohydr. Res. 346, 2752–2759. doi: 10.1016/j.carres.2011.09.029
Magliani, W., Conti, S., Salati, A., Vaccari, S., Ravanetti, L., Maffei, D. L., et al. (2004). Therapeutic potential of yeast killer toxin-like antibodies and mimotopes. FEMS Yeast Res. 5, 11–18. doi: 10.1016/j.femsyr.2004.06.010
McKenzie, C. G., Koser, U., Lewis, L. E., Bain, J. M., Mora-Montes, H. M., Barker, R. N., et al. (2010). Contribution of Candida albicans cell wall components to recognition by and escape from murine macrophages. Infect. Immun. 78, 1650–1658. doi: 10.1128/IAI.00001-10
Moragues, M. D., Omaetxebarria, M. J., Elguezabal, N., Sevilla, M. J., Conti, S., Polonelli, L., et al. (2003). A monoclonal antibody directed against a Candida albicans cell wall mannoprotein exerts three anti-C. albicans activities. Infect. Immun. 71, 5273–5279. doi: 10.1128/IAI.71.9.5273-5279.2003
Moyes, D. L., and Naglik, J. R. (2011). Mucosal immunity and Candida albicans infection. Clin. Dev. Immunol. 2011:346307. doi: 10.1155/2011/346307
Moyes, D. L., Richardson, J. P., and Naglik, J. R. (2015). Candida albicans-epithelial interactions and pathogenicity mechanisms: scratching the surface. Virulence 6, 338–346. doi: 10.1080/21505594.2015.1012981
Netea, M. G., Brown, G. D., Kullberg, B. J., and Gow, N. A. (2008). An integrated model of the recognition of Candida albicans by the innate immune system. Nat. Rev. Microbiol. 6, 67–78. doi: 10.1038/nrmicro1815
Netea, M. G., Gow, N. A., Munro, C. A., Bates, S., Collins, C., Ferwerda, G., et al. (2006). Immune sensing of Candida albicans requires cooperative recognition of mannans and glucans by lectin and Toll-like receptors. J. Clin. Invest. 116, 1642–1650. doi: 10.1172/JCI27114
Netea, M. G., Joosten, L. A., van der Meer, J. W., Kullberg, B. J., and van de Veerdonk, F. L. (2015). Immune defence against Candida fungal infections. Nat. Rev. Immunol. 15, 630–642. doi: 10.1038/nri3897
Nishikawa, A., Shinoda, T., and Fukazawa, Y. (1982). Immunochemical determinant and serological specificity of Candida krusei. Mol. Immunol. 19, 367–373. doi: 10.1016/0161-5890(82)90202-4
Pashov, A., Monzavi-Karbassi, B., and Kieber-Emmons, T. (2011). Glycan mediated immune responses to tumor cells. Hum. Vaccin. 7, 156–165. doi: 10.4161/hv.7.0.14578
Paulovicova, E., Paulovicova, L., Hrubisko, M., Krylov, V. B., Argunov, D. A., and Nifantiev, N. E. (2017). Immunobiological activity of synthetically prepared immunodominant galactomannosides structurally mimicking Aspergillus galactomannan. Front. Immunol. 8:1273. doi: 10.3389/fimmu.2017.01273
Paulovicova, E., Paulovicova, L., Pilisiova, R., Bystricky, S., Yashunsky, D. V., Karelin, A. A., et al. (2013). Synthetically prepared glycooligosaccharides mimicking Candida albicans cell wall glycan antigens–novel tools to study host-pathogen interactions. FEMS Yeast Res. 13, 659–673. doi: 10.1111/1567-1364.12065
Paulovicova, E., Paulovicova, L., Pilisiova, R., Jancinova, V., Yashunsky, D. V., Karelin, A. A., et al. (2016). The evaluation of beta-(1 –> 3)-nonaglucoside as an anti-Candida albicans immune response inducer. Cell. Microbiol. 18, 1294–1307. doi: 10.1111/cmi.12631
Paulovicova, L., Bystricky, S., Paulovicova, E., Karelin, A. A., Tsvetkov, Y. E., and Nifantiev, N. E. (2010). Model alpha-mannoside conjugates: immunogenicity and induction of candidacidal activity. FEMS Immunol. Med. Microbiol. 58, 307–313. doi: 10.1111/j.1574-695X.2009.00642.x
Paulovicova, L., Paulovicova, E., and Bystricky, S. (2014). Immunological basis of anti-Candida vaccines focused on synthetically prepared cell wall mannan-derived manno-oligomers. Microbiol. Immunol. 58, 545–551. doi: 10.1111/1348-0421.12195
Paulovicova, L., Paulovicova, E., Karelin, A. A., Tsvetkov, Y. E., Nifantiev, N. E., and Bystricky, S. (2012). Humoral and cell-mediated immunity following vaccination with synthetic Candida cell wall mannan derived heptamannoside-protein conjugate Immunomodulatory properties of heptamannoside-BSA conjugate. Int. Immunopharmacol. 14, 179–187. doi: 10.1016/j.intimp.2012.07.004
Paulovicova, L., Paulovicova, E., Karelin, A. A., Tsvetkov, Y. E., Nifantiev, N. E., and Bystricky, S. (2013). Effect of branched -oligomannoside structures on induction of anti-Candida humoral immune response. Scand. J. Immunol. 77, 431–441. doi: 10.1111/sji.12044
Peat, S., Whelan, W. J., and Edwards, T. E. (1961). 6. Polysaccharides of baker's yeast. Part IV. Mannan. J. Chem. Soc. 29–34. doi: 10.1039/jr9610000029
Perez-Garcia, L. A., Diaz-Jimenez, D. F., Lopez-Esparza, A., and Mora-Montes, H. M. (2011). Role of cell wall polysaccharides during recognition of Candida albicans by the innate immune system. J. Glycobiol. 1, 1–7. doi: 10.4172/jgb.1000102
Pfaller, M. A., Messer, S. A., Hollis, R. J., and Jones, R. N. (2002). Antifungal activities of posaconazole, ravuconazole, and voriconazole compared to those of itraconazole and amphotericin B against 239 clinical isolates of Aspergillus spp. and other filamentous fungi: report from SENTRY Antimicrobial Surveillance Program, 2000. Antimicrob. Agents Chemother. 46, 1032–1037. doi: 10.1128/AAC.46.4.1032-1037.2002
Piccione, D., Mirabelli, S., Minto, N., and Bouklas, T. (2019). Difficult but not impossible: in search of an anti-Candida vaccine. Curr. Trop. Med. Rep. 6, 42–49. doi: 10.1007/s40475-019-00173-2
Pichard, D. C., Freeman, A. F., and Cowen, E. W. (2015). Primary immunodeficiency update: part II. Syndromes associated with mucocutaneous candidiasis and noninfectious cutaneous manifestations. J. Am. Acad. Dermatol. 73, 367–381; quiz 381–362. doi: 10.1016/j.jaad.2015.01.055
Pikman, R., and Ben-Ami, R. (2012). Immune modulators as adjuncts for the prevention and treatment of invasive fungal infections. Immunotherapy 4, 1869–1882. doi: 10.2217/imt.12.127
Podzorski, R. P., Gray, G. R., and Nelson, R. D. (1990). Different effects of native Candida albicans mannan and mannan-derived oligosaccharides on antigen-stimulated lymphoproliferation in vitro. J. Immunol. 144, 707–716.
Podzorski, R. P., Herron, M. J., Fast, D. J., and Nelson, R. D. (1989). Pathogenesis of candidiasis. Immunosuppression by cell wall mannan catabolites. Arch. Surg. 124, 1290–1294. doi: 10.1001/archsurg.1989.01410110044009
Poulain, D., Jouault, T., and Trinel, P. A. (1997). “Immunoreactivity of Candida albicans β-1,2 linked oligomannosides and phospholipomannan,” in Fungal Cells in Biodefense Mechanism, eds. S. Suzuki and M. Suzuki (Tokyo: Saigon Publishing, 175–181.
Richardson, J. P., and Moyes, D. L. (2015). Adaptive immune responses to Candida albicans infection. Virulence 6, 327–337. doi: 10.1080/21505594.2015.1004977
Richter, S. S., Galask, R. P., Messer, S. A., Hollis, R. J., Diekema, D. J., and Pfaller, M. A. (2005). Antifungal susceptibilities of Candida species causing vulvovaginitis and epidemiology of recurrent cases. J. Clin. Microbiol. 43, 2155–2162. doi: 10.1128/JCM.43.5.2155-2162.2005
Rizzetto, L., Kuka, M., De Filippo, C., Cambi, A., Netea, M. G., Beltrame, L., et al. (2010). Differential IL-17 production and mannan recognition contribute to fungal pathogenicity and commensalism. J. Immunol. 184, 4258–4268. doi: 10.4049/jimmunol.0902972
Romani, L. (2003). “Cytokines of innate and adaptive immunity to Candida albicans,” in Cytokines and Chemokines in Infectious Diseases Handbook, eds. M. Kotb and T. Calandra (Totowa, NJ: Humana Press), 227–241. doi: 10.1385/1-59259-309-7:227
Romani, L. (2011). Immunity to fungal infections. Nat. Rev. Immunol. 11, 275–288. doi: 10.1038/nri2939
Saijo, S., Ikeda, S., Yamabe, K., Kakuta, S., Ishigame, H., Akitsu, A., et al. (2010). Dectin-2 recognition of alpha-mannans and induction of Th17 cell differentiation is essential for host defense against Candida albicans. Immunity 32, 681–691. doi: 10.1016/j.immuni.2010.05.001
Salek-Ardakani, S., Cota, E., and Bignell, E. (2012). Host-fungal interactions: key players of antifungal immunity. Expert Rev. Anti Infect. Ther. 10, 149–151. doi: 10.1586/eri.11.169
Sandini, S., La Valle, R., De Bernardis, F., Macrì, C., and Cassone, A. (2007). The 65 kDa mannoprotein gene of Candida albicans encodes a putative β-glucanase adhesin required for hyphal morphogenesis and experimental pathogenicity. Cell. Microbiol. 9, 1223–1238. doi: 10.1111/j.1462-5822.2006.00862.x
Scheller, J., Chalaris, A., Schmidt-Arras, D., and Rose-John, S. (2011). The pro- and anti-inflammatory properties of the cytokine interleukin-6. Biochim. Biophys. Acta 1813, 878–888. doi: 10.1016/j.bbamcr.2011.01.034
Schubert, M., Xue, S., Ebel, F., Vaggelas, A., Krylov, V. B., Nifantiev, N. E., et al. (2019). Monoclonal antibody AP3 binds galactomannan antigens displayed by the pathogens Aspergillus flavus, A. fumigatus, and A. parasiticus. Front. Cell. Infect. Microbiol. 9:234. doi: 10.3389/fcimb.2019.00234
Sears, D., and Schwartz, B. S. (2017). Candida auris: an emerging multidrug-resistant pathogen. Int. J. Infect. Dis. 63, 95–98. doi: 10.1016/j.ijid.2017.08.017
Shibata, N., Ikuta, K., Imai, T., Satoh, Y., Satoh, R., Suzuki, A., et al. (1995). Existence of branched side chains in the cell wall mannan of pathogenic yeast, Candida albicans. Structure-antigenicity relationship between the cell wall mannans of Candida albicans and Candida parapsilosis. J. Biol. Chem. 270, 1113–1122. doi: 10.1074/jbc.270.3.1113
Shibata, N., Kobayashi, H., and Suzuki, S. (2012). Immunochemistry of pathogenic yeast, Candida species, focusing on mannan. Proc. Jpn. Acad. Ser. B Phys. Biol. Sci. 88, 250–265. doi: 10.2183/pjab.88.250
Shibata, N., Suzuki, A., Kobayashi, H., and Okawa, Y. (2007). Chemical structure of the cell-wall mannan of Candida albicans serotype A and its difference in yeast and hyphal forms. Biochem. J. 404, 365–372. doi: 10.1042/BJ20070081
Snarr, B. D., Qureshi, S. T., and Sheppard, D. C. (2017). Immune recognition of fungal polysaccharides. J. Fungi 3:47. doi: 10.3390/jof3030047
Suzuki, M., and Fukazawa, Y. (1982). Immunochemical characterization of Candida albicans cell wall antigens: specific determinant of Candida albicans serotype A mannan. Microbiol. Immunol. 26, 387–402. doi: 10.1111/j.1348-0421.1982.tb00189.x
Suzuki, S. (1997). “Structural investigation of mannans of medicinally relevant Candida species; Determination of chemical structures of antigenic factors, 1, 4, 5, 6, 9 and 13b,” in Fungal Cells in Biodefense Mechanism, eds S. Suzuki and M. Suzuki (Tokyo: Saigon Publishing, 1–16.
Tang, C. K., Sheng, K. C., Esparon, S. E., Proudfoot, O., Apostolopoulos, V., and Pietersz, G. A. (2009). Molecular basis of improved immunogenicity in DNA vaccination mediated by a mannan based carrier. Biomaterials 30, 1389–1400. doi: 10.1016/j.biomaterials.2008.11.010
Taylor, P. R., Tsoni, S. V., Willment, J. A., Dennehy, K. M., Rosas, M., Findon, H., et al. (2007). Dectin-1 is required for beta-glucan recognition and control of fungal infection. Nat. Immunol. 8, 31–38. doi: 10.1038/ni1408
Torosantucci, A., Bromuro, C., Chiani, P., De Bernardis, F., Berti, F., Galli, C., et al. (2005). A novel glyco-conjugate vaccine against fungal pathogens. J. Exp. Med. 202, 597–606. doi: 10.1084/jem.20050749
Trinel, P. A., Faille, C., Jacquinot, P. M., Cailliez, J. C., and Poulain, D. (1992). Mapping of Candida albicans oligomannosidic epitopes by using monoclonal antibodies. Infect. Immun. 60, 3845–3851.
Tso, G. H. W., Reales-Calderon, J. A., and Pavelka, N. (2018). The elusive anti-Candida vaccine: lessons from the past and opportunities for the future. Front. Immunol. 9:897. doi: 10.3389/fimmu.2018.00897
Tsvetkov, Y. E., Burg-Roderfeld, M., Loers, G., Arda, A., Sukhova, E. V., Khatuntseva, E. A., et al. (2012). Synthesis and molecular recognition studies of the HNK-1 trisaccharide and related oligosaccharides. The specificity of monoclonal anti-HNK-1 antibodies as assessed by surface plasmon resonance and STD NMR. J. Am. Chem. Soc. 134, 426–435. doi: 10.1021/ja2083015
van de Veerdonk, F. L., and Netea, M. G. (2010). T-cell subsets and antifungal host defenses. Curr. Fungal Infect. Rep. 4, 238–243. doi: 10.1007/s12281-010-0034-6
Varki, A., Cummings, R., Esko, J., Freeze, H., Hart, G., and Marth, J. (1999). Essentials of Glycobiology. New York, NY: Cold Spring Harbor.
Vinh, D. C. (2011). Insights into human antifungal immunity from primary immunodeficiencies. Lancet Infect. Dis. 11, 780–792. doi: 10.1016/S1473-3099(11)70217-1
Wagener, J., Malireddi, R. K., Lenardon, M. D., Koberle, M., Vautier, S., MacCallum, D. M., et al. (2014). Fungal chitin dampens inflammation through IL-10 induction mediated by NOD2 and TLR9 activation. PLoS Pathog. 10:e1004050. doi: 10.1371/journal.ppat.1004050
West, L., Lowman, D. W., Mora-Montes, H. M., Grubb, S., Murdoch, C., Thornhill, M. H., et al. (2013). Differential virulence of Candida glabrata glycosylation mutants. J. Biol. Chem. 288, 22006–22018. doi: 10.1074/jbc.M113.478743
Xin, H., Cartmell, J., Bailey, J. J., Dziadek, S., Bundle, D. R., and Cutler, J. E. (2012). Self-adjuvanting glycopeptide conjugate vaccine against disseminated candidiasis. PLoS ONE 7:e35106. doi: 10.1371/journal.pone.0035106
Xin, H., Dziadek, S., Bundle, D. R., and Cutler, J. E. (2008). Synthetic glycopeptide vaccines combining beta-mannan and peptide epitopes induce protection against candidiasis. Proc. Natl. Acad. Sci. U.S.A. 105, 13526–13531. doi: 10.1073/pnas.0803195105
Keywords: Candida, oligomannosides, RAW 264.7, cytokines, proliferation
Citation: Paulovičová E, Paulovičová L, Farkaš P, Karelin AA, Tsvetkov YE, Krylov VB and Nifantiev NE (2019) Importance of Candida Antigenic Factors: Structure-Driven Immunomodulation Properties of Synthetically Prepared Mannooligosaccharides in RAW264.7 Macrophages. Front. Cell. Infect. Microbiol. 9:378. doi: 10.3389/fcimb.2019.00378
Received: 04 July 2019; Accepted: 21 October 2019;
Published: 08 November 2019.
Edited by:
Laura Alcazar-Fuoli, Carlos III Health Institute, SpainReviewed by:
Jill R. Blankenship, University of Nebraska Omaha, United StatesIan A. Cleary, Grand Valley State University, United States
Copyright © 2019 Paulovičová, Paulovičová, Farkaš, Karelin, Tsvetkov, Krylov and Nifantiev. This is an open-access article distributed under the terms of the Creative Commons Attribution License (CC BY). The use, distribution or reproduction in other forums is permitted, provided the original author(s) and the copyright owner(s) are credited and that the original publication in this journal is cited, in accordance with accepted academic practice. No use, distribution or reproduction is permitted which does not comply with these terms.
*Correspondence: Ema Paulovičová, ZW1hLnBhdWxvdmljb3ZhQHNhdmJhLnNr; Nikolay E. Nifantiev, bmVuQGlvYy5hYy5ydQ==