- 1Center of Clinical Laboratory, Zhongshan Hospital, School of Medicine, Xiamen University, Xiamen, China
- 2Xiamen Hospital of Traditional Chinese Medicine, Xiamen, China
- 3Shaoxing Center for Disease Control and Prevention, Shaoxing, China
- 4Institute of Infectious Disease, School of Medicine, Xiamen University, Xiamen, China
Background: This study aimed to evaluate real-time polymerase chain reaction coupled with multiplex probe melting curve analysis (PCR-MCA) for pathogen detection in patients with suspected bloodstream infections (BSIs).
Methods: A PCR-MCA assay was developed for simultaneous identification of 28 kinds of the most common pathogens and two resistance genes within a few hours. The diagnostic performance of the PCR-MCA assay was determined and compared to the results of blood culture.
Results: A total of 2,844 consecutive new episodes of suspected BSIs in 2,763 patients were included in this study. There were 269 episodes of pathogens identified by blood culture. For all the pathogens tested, the PCR-MCA assay exhibited a sensitivity of 88.8% (239/269), specificity of 100% (2,575/2,575), and agreement of 98.9% (2,814/2,844). For the pathogens on the PCR-MCA list, the PCR-MCA results had a sensitivity of 99.2% (239/241), specificity of 100% (2,575/2,575), and agreement of 99.9% (2,814/2,816) compared with the results of blood culture. For seven samples with multiple pathogens identified simultaneously during one blood culture investigation, the PCR-MCA assay verified the results of the blood culture, with an agreement rate of 100% for each.
Conclusion: The PCR-MCA assay could discover 88.8% of the pathogens in clinical practice, showing excellent diagnostic performance vs. that of blood culture for pathogen detection in patients with suspected BSIs, and would contribute to rapid diagnosis and correct antibiotic administration.
Introduction
Sepsis could be called a severe complication of bloodstream infections (BSIs). The reported incidence of sepsis is increasing, and conservative estimates indicate that sepsis is a leading cause of mortality and critical illness worldwide (Singer et al., 2016). Early recognition of sepsis and accurate administration of antibiotics are important for improving outcomes. According to international guidelines (Singer et al., 2016), empirical antibiotic treatment should be given within 1 h once sepsis is suspected, and broad-spectrum antibiotics are often recommended. However, as early empirical treatment may be ineffective more often than in the past because of the worldwide rise and broadening of bacterial multidrug resistance (Gustinetti and Mikulska, 2016), precise diagnosis and antibiotic treatment targeting the causative pathogen of sepsis are urgently need.
Blood cultures, aiming to detect viable microorganisms in blood, are still considered as the “gold standard” for the microbiological diagnosis of BSIs. Suspected BSIs can be identified if the culture is obtained first and the antibiotic is concomitantly administered within 72 h, whereas if the antibiotic is first and the culture is acquired within 24 h (Singer et al., 2016). However, when antibiotics are administered before the culture is obtained, sensitivity is greatly reduced, and slow-growing, fastidious microorganisms are difficult to detect by blood culture (Opota et al., 2015). Studies have shown that only approximately half of the patients experiencing sepsis and septic shock have positive blood cultures (Bochud et al., 2001). Furthermore, ~24–72 h is required to identify the pathogen and obtain information on antibiotic sensitivity (Peters et al., 2004). As a consequence, empirical antibiotic therapy is more commonly guided by clinical response than by the result of a blood culture.
To achieve faster and more accurate diagnosis of sepsis, rapid molecular diagnostic tests have been developed for BSI diagnosis. The U.S. Food and Drug Administration has cleared several multiplex molecular assays that can detect a wide range of microorganisms concurrently with specific resistance genes directly in blood samples, and some of these assays have been made commercially available (Rello et al., 2018). One of the most developed technologies is the multiplex polymerase chain reaction (PCR) assay, which can detect the DNA of as many as 20 pathogen species directly in blood samples within hours (Galiana et al., 2017; Makristathis et al., 2018), even more kinds of microorganisms concurrently with several resistance genes in positive blood cultures (Ramanan et al., 2018). Recently, multiplex probe melting curve analysis (MCA) based on real-time PCR assays has also been applied in pathogen identification (Jiang et al., 2017), allowing simultaneous identification of ten bacterial pathogens in gastroenteritis and wound infections via a single reaction. On this basis, we developed a real-time PCR coupled with multiplex probe MCA (PCR-MCA) assay for simultaneous identification of 28 kinds of the most common pathogens and two resistance genes in BSIs. After the multiplex ligation reaction, linear-after-the-exponential PCR was carried out with a real-time PCR instrument by using the fluorophore and melting temperature (Tm) value as dual indicators, and MCA yielded a unique Tm code by which a certain pathogenic bacterium could be identified (Liao et al., 2013). In this study, we aimed to evaluate the clinical performance of this assay compared with that of blood culture for pathogen detection in patients with suspected BSIs.
Materials and Methods
Study Population and Ethics Statement
This study was conducted at Zhongshan Hospital, School of Medicine, Xiamen University, from October 2016 to June 2017. The inclusion criteria for the study population were as follows: clinical suspicion of the development of life-threatening organ dysfunction caused by a BSI identified by a treating clinician and an urgent need for blood cultures. For clinical operationalization, organ dysfunction can be represented by an increase of 2 points or more in the sepsis-related organ failure assessment score (Singer et al., 2016). Patients who had been administered with antibiotics (such as some postoperative or transferred patients) were excluded from the study. In this study, the observation unit was an episode of suspected BSI, the results were evaluated as follows: once a bacterial or fungal pathogen was isolated from at least one blood culture, a positive BSI episode was recorded, and the consecutive duplicate results were merged; a BSI episode was considered new if it was detected at least 14 days from the previous infection with positive blood culture or if a different microorganism was isolated from a new blood sample (Quiles et al., 2019); consecutive repeated negative blood culture results from one patient during one hospitalization was recorded as a negative BSI episode. A total of 2,763 patients with 2,844 consecutive new episodes of suspected BSIs were recruited. This study was approved by the Institutional Ethics Committee of Zhongshan Hospital, School of Medicine, Xiamen University, and was in compliance with national legislation and the Declaration of Helsinki guidelines. Written informed consent was obtained from the patient or, from next of kin or an independent patient advocate if the patient lacked this capacity.
Laboratory Procedures
Blood samples were obtained from eligible patients as follows. First, two blood samples were collected simultaneously from peripheral venepuncture (8–10 mL for adults and 1 mL for children) and inoculated into paired culture bottles (Plus Aerobic, Plus Anaerobic; BD BACTECTM). Blood culture entered the standard clinical pathway, and the results were returned directly to the clinical service at each center. Second, research blood samples (5 mL for adults and 1 mL for children) were obtained from the same venepuncture sites at the same time and were placed into sterile EDTA tubes for PCR-MCA analysis of pathogen DNA in whole blood. The research blood samples were transferred to a single molecular laboratory and kept for no more than 72 h at 4°C prior to PCR analysis.
Blood Culture
Blood culture bottles were incubated for 5 days in an automated blood culture instrument (BD BactecTM FX). When bacterial growth was noted, the positive culture sample was inoculated into blood agar, MacConkey agar and chocolate agar and then cultured overnight in a 37°C electro-heating standing-temperature cultivator. A single colony of fresh overnight culture was smeared directly onto a ground-steel matrix-assisted laser desorption/ionization (MALDI) target plate, covered with 1.5 μL of saturated α-cyano-4-hydroxycinnamic acid matrix in 50% acetonitrile-2.5% trifluoroacetic acid and allowed to dry. The plate was inserted into the source of a Bruker Microflex LT/SH MALDI-TOF-MS instrument (Bruker MALDI Biotyper) for identification. The blood culture was considered negative if no bacterial growth was detected within 5 days and was not evaluated by the Bruker MALDI Biotyper.
DNA Preparation
The blood was cultured with a pipette at 1 mL in an EP tube and centrifuged at 13,000 × g for 2 min to precipitate the bacteria. After the supernatant was removed by pipetting, 100 μL of 1 × TE buffer (10 mM Tris base, 1 mM EDTA, pH 8.5) was added to the bacterial precipitate. The EP tube was heated at 99°C for 10 min and centrifuged at 13,000 × g for 10 min. The supernatant was removed into the new EP tube and stored in a −20°C freezer for PCR-MCA.
PCR-MCA
In this study, tagged primers were used, which were different from universal primers in that they contained a common tag sequence at the 5′ ends of specific primers. The primer sequences (Sangon Biotech (Shanghai) Co., Ltd.) are shown in Table 1; these primers target 12 kinds of gram-positive bacteria, 11 kinds of gram-negative bacteria, five kinds of fungi, and two resistance genes, namely, a vancomycin resistance-encoding gene for Enterococcus species (vanA) and a beta-lactam resistance-encoding gene for Staphylococcus species (mecA). The probes in this study were composed of media probes and universal probes. The secondary structures of the probes were predicted using mfold Web Server online analysis software (http://mfold.rna.albany.edu/?q=mfold/DNA-Folding-Form). The fluorophores FAM, ROX, CY5, and HEX were marked with a 5′ universal probe, and the quenchers BHQ1 and BHQ2 were marked with a 3′ probe. The probe sequences have not been shown here as the patent is pending. The Tm values of the probes were predicted using Tm Utility v1.3 software. The homology of the designed primers and probes was tested using BLAST software (https://blast.ncbi.nlm.nih.gov/Blast.cgi).
When the uniplex PCR assay was established, three pairs of primers were designed for each testing object first, and primers were screened by pairwise combination to select the optimal primer combination. Second, the PCR components were optimized. The final 25-μL uniplex PCR assay included 5.0 mM MgCl2, 1× buffer (67 mM Tris-HCl, 16.6 mM (NH4)2SO4, 6.7 μM EDTA, and 0.085 mg/mL BSA), 0.2 mM dNTPs, 2.0 U of Taq01 DNA polymerase, 0.01 U of UNG enzyme, 0.05 μM forward/reverse primers, 0.4 μM universal primers, 0.3 μM media probes, 0.08 μM universal probes, and 5 μL of template DNA. After the PCR procedure was optimized, the procedure used for the uniplex PCR assay for BSIs was as follows: amplification stage: 50°C for 2 min, predenaturation at 95°C for 5 min, 95°C for 20 s and 60°C for 1 min (for a total of 50 cycles), and final extension at 35°C for 25 min; melting stage: denaturation at 95°C for 2 min and heat preservation at 45°C for 2 min, followed by probe melting curve analysis via a temperature ramp from 45 to 95°C with a heating rate of 0.04°C/s. Fluorescence signals in the corresponding detection channels were collected during the whole melting process.
On the basis of the uniplex PCR assay for BSIs, all primers and probes were combined to establish the multiplex PCR assay. The PCR components were optimized, and the final 25-μL multiplex PCR assay included 5.0 mM MgCl2, 1× buffer, 0.25 mM dNTPs, 2.5 U of Taq01 DNA polymerase, 0.01 U of UNG enzyme, 0.7 μM universal primers, 7 universal probes at 0.1 μM each, and 5 μL of template DNA. After the PCR procedure was optimized, the procedure used for the multiplex PCR assay for BSIs was the same as that for the uniplex PCR assay, and the fluorescence signals in the corresponding detection channels were collected during the whole melting process. The uniplex and multiplex experiments were carried out on an SLAN 96S real-time fluorescence PCR instrument (Shanghai Hongshi Medical Technology Co., Ltd.). Discordant results between the PCR-MCA assay and blood culture were further verified using a uniplex PCR assay.
Analytical Evaluation
To evaluate analytical sensitivity, first, plasmids of 28 kinds of pathogens and two resistance genes were constructed, and serial dilutions of the DNA were prepared from 104 copies/μL to 100 copies/μL. Second, suspensions of reference strains of the 28 kinds of pathogens were prepared with serial 10-fold dilutions in 0.9% NaCl. Each dilution, the concentration was determined by plate counts (CFU/mL), was subjected to DNA extraction. The limit of detection (LOD) was defined as the lowest concentration that yielded no more than one negative result in 20 replicates (i.e., positive rate, ≥95%).
To evaluate analytical specificity, each target pathogen or resistance gene in the detection system was used as a template for the PCR. In addition, a blind test of the bacterial samples, including the 28 targeted pathogens and non-targeted pathogens, was performed.
The reproducibility was evaluated using the constructed plasmids for each pathogen or resistant gene as templates (concentration 103 copies/μL). The experiments were carried out three times on the same instrument, with three duplicates of each template for each experiment. The Tm values of the melting peaks of each test object in different experiments and parallel tubes were analyzed by calculating the standard deviations (SDs) and coefficient of variation (CV).
Statistical Analysis
The results of blood culture for identification of pathogens were used as the gold standard in this study. The diagnostic performance of the PCR-MCA assay was determined and compared to the results of blood culture, using sensitivity, specificity, positive predictive value, negative predictive value (NPV), agreement rate and Kappa value, according to the Yerushalmy model (Niu et al., 2015). The sensitivity was calculated as the number of episodes in which both blood culture and PCR-MCA were positive divided by the sum of these episodes and the number of episodes in which blood culture was positive but PCR-MCA were negative. The specificity was calculated as the number of episodes in which both blood culture and PCR-MCA were negative divided by the sum of these episodes and the number of episodes in which blood culture was negative but PCR-MCA were positive. The statistical analyses were performed using SPSS 19.0 for Windows (SPSS Inc., Chicago, Illinois, USA).
Results
Characteristics of the Study Participants
A total of 2,763 patients with 2,844 consecutive new episodes of suspected BSIs were recruited in this study. The median age of patient episodes was 44 years (interquartile range, 1–67 years), including 1,658 male episodes (58.3%) and 1,186 female episodes (41.7%). The main proportion of participants came from the pediatrics department (33.5%), followed by the pulmonology department (16.7%), general surgery department (10.2%), hematology department (6.6%), gastroenterology department (6.2%), nephrology department (5.3%), etc (Table 2). The prevalence of BSIs identified by blood culture was 26.5% (148/558) in surgery, 7.5% (93/1,248) in internal medicine, 11.9% (10/84) in obstetrics and gynecology, and 1.9% (18/954) in pediatrics.
Performance of the PCR-MCA Assay for Identification of Pathogens Compared to That of Blood Culture
The analytical sensitivity, specificity and reproducibility of the PCR-MCA assay were evaluated. The results showed that the analytical sensitivity reached 50 to 5 copies per reaction. The 28 targeted pathogens gave reproducible readouts in 20 replicates when the concentration was equal to or higher than 300 CFU/mL; therefore, the PCR-MCA assay LOD was determined to be 300 CFU/mL (data not shown). The analytical specificity results showed that the PCR-MCA assay could correctly detect all 28 targeted pathogens and exhibited no cross-reactivity with non-targeted pathogens. The relevant melting signal peak of each pathogen or resistance gene was achieved, and no interference existed among the signals. The reproducibility results showed that the SDs of the Tm values were no more than 1°C and that the CVs were no more than 1%, indicating a high reproducibility of this assay.
Among the 2,844 episodes of suspected BSIs, 269 were identified for the presence of pathogens with the results of blood culture. The most common gram-negative pathogen was Escherichia coli (n = 95, 35.3%), followed by Klebsiella pneumoniae (n = 40, 14.9%), Acinetobacter baumannii (n = 9, 3.3%), Pseudomonas aeruginosa (n = 9, 3.3%), Enterobacter cloacae (n = 5, 1.9%), etc. The most common gram-positive pathogen was Staphylococcus aureus (n = 22, 8.2%), followed by Staphylococcus epidermidis (n = 16, 5.9%), Streptococcus pneumoniae (n = 9, 3.3%), Staphylococcus capitis (n = 7, 2.6%), Enterococcus faecium (n = 6, 2.2%), Enterococcus faecalis (n = 6, 2.2%), etc. The most common fungus was Candida albicans (n = 8, 3.0%). Among the 2,844 episodes of suspected BSIs, 24 episodes were identified with Staphylococcus species (including 11 with S. epidermidis, five with S. capitis, four with S. aureus, two with S. haemolyticus, one with S. hominis, and one with S. lugdunensis) resistant to all beta-lactam antibiotics in phenotypic susceptibility tests. However, the mecA gene was not detected in any of these species using the PCR-MCA assay. In addition, no vancomycin-resistant Enterococcus species were identified in phenotypic susceptibility tests, consistent with the PCR-MCA result showing that the vanA gene was not detected. The performance of the PCR-MCA assay compared to that of blood culture for identification of each pathogen is shown in Table 3. For all the pathogens tested, the PCR-MCA assay discovered 88.8% (239/269) of the pathogens discovered by blood culture, with a sensitivity of 88.8%, specificity of 100% (2,575/2,575), and agreement of 98.9% (2,814/2,844). Among the 269 episodes of pathogens identified by blood culture, 241 were identified with a pathogen on the PCR-MCA list, while 28 were not. For the pathogens on the PCR-MCA list, the PCR-MCA assay had a sensitivity of 99.2% (239/241), specificity of 100% (2,575/2,575), and agreement of 99.9% (2,814/2,816) compared with the results of blood culture.
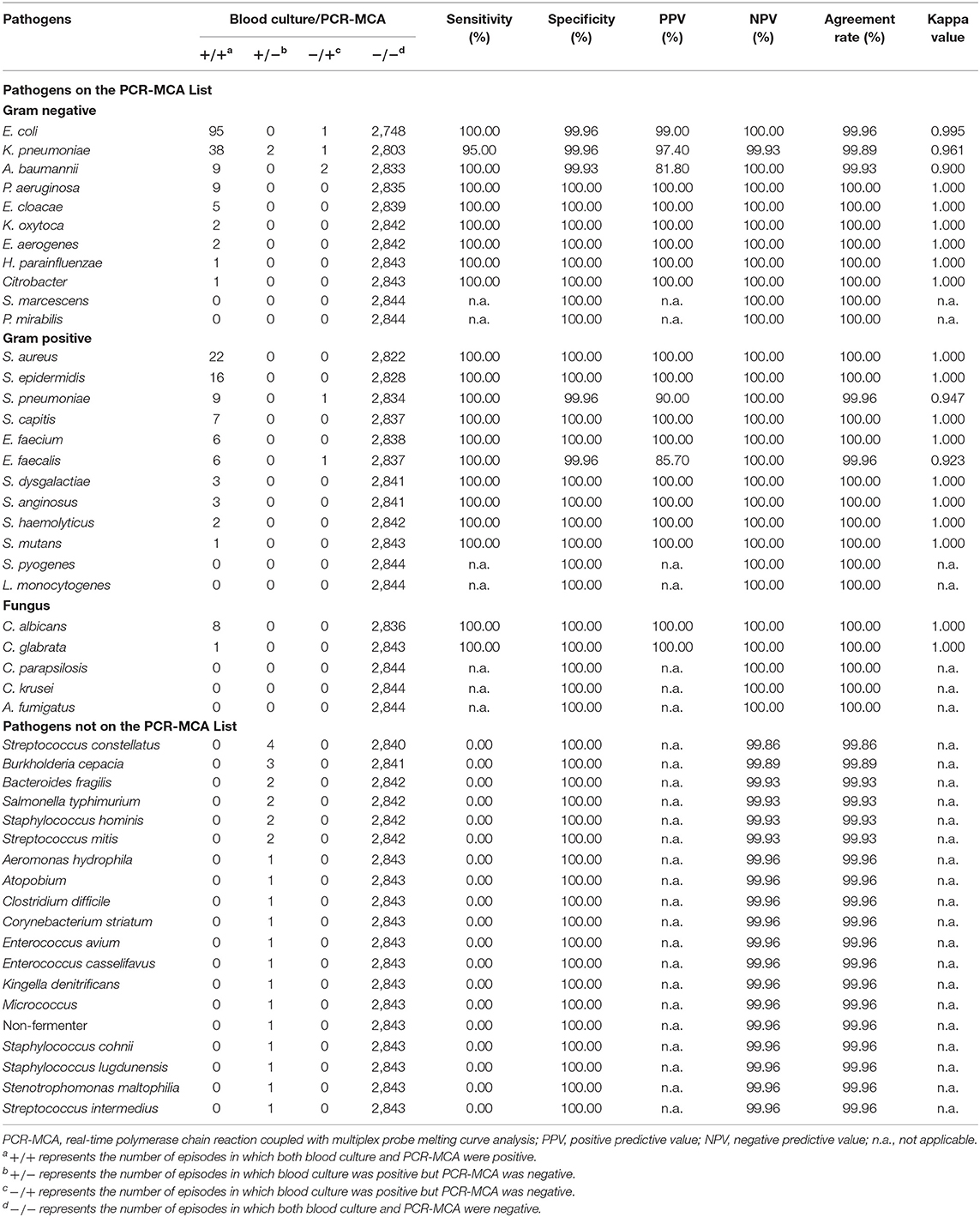
Table 3. Performance of the PCR-MCA assay for identification of pathogens compared to the results of blood culture.
Results of the Blood Culture and PCR-MCA Assay for the Identification of Multiple Infections
Among the 2,763 patients recruited, there were seven cases in whose blood samples two kinds of pathogens were identified simultaneously during one blood culture investigation. For these samples, the PCR-MCA assay verified the results of blood culture, with an agreement rate of 100% for each case. The results of blood culture and the PCR-MCA assay for the identification of multiple infections are shown in Table 4. In addition, there were eight subjects who underwent two or more sequential episodes of BSIs at different times during one hospitalization. In five cases (cases number 10, 82, 91, 94, and 133), the results of the PCR-MCA assay for identification of pathogens were consistent with those of blood culture in every infection episode. For case No. 9, the PCR-MCA assay verified the results of blood culture for the second and third infection episodes but showed negative results for the first episode, while K. pneumoniae was detected by blood culture. For case No. 176, the PCR-MCA assay verified the results of blood culture for the first infection episode but showed negative results for the second and third episodes because the pathogens identified by blood culture were not on the PCR-MCA list. For case No. 334, the result of the PCR-MCA assay was the same as that of blood culture for the first infection episode but detected one more pathogen than blood culture for the second infection episode.
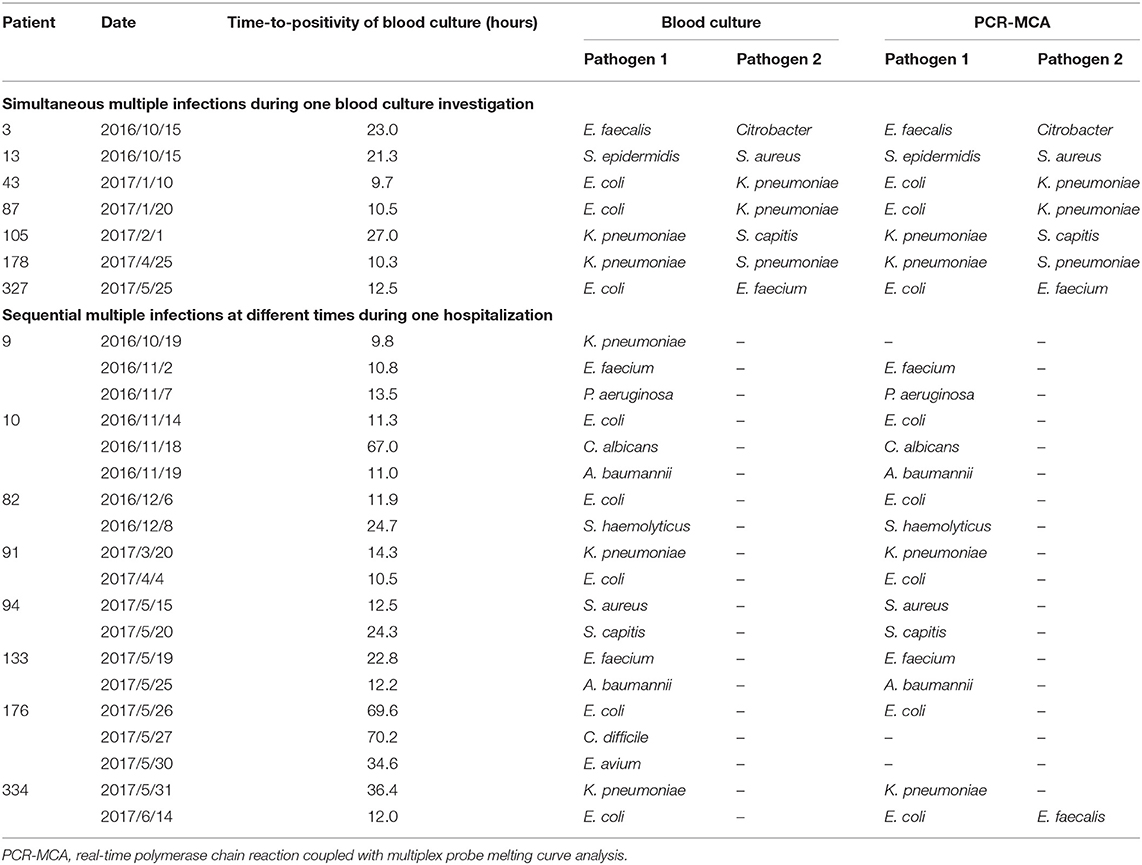
Table 4. Results of blood culture and the PCR-MCA assay for the identification of multiple infections.
Clinical Characteristics of Patients With Discordant Results Between Blood Culture and the PCR-MCA Assay
Among the 2,844 episodes of suspected BSIs, there were eight episodes from eight cases for which the results of the PCR-MCA assay were inconsistent with those of blood culture. The clinical characteristics of these cases are shown in Table 5. Two cases (cases number 9 and 42) were identified with K. pneumoniae infection by blood culture, but the results of the PCR-MCA assay were negative. These two cases both came from surgical wards and had increased C-reactive protein levels; case No. 42 also had a decreased white blood cell counts. Six cases (cases number 121, 229, 314, 334, 354, and 355) were identified as having single infection by blood culture, but the results of the PCR-MCA assay showed multiplex DNAemia from two pathogens. These six cases both had increased procalcitonin levels, and cases number 354 and 355 also had increased white blood cell counts, while cases number 314, 334 and 355 had increased C-reactive protein levels. The time-to-positivity (TTP) of blood culture was 9.8 h in cases number 9 and 42, and 13.0, 25.0, 18.3, 12.0, 13.7, 6.5 h in cases number 121, 229, 314, 334, 354, and 355, respectively. These discordant results were further confirmed using a uniplex PCR assay and showed the same results as the PCR-MCA assay.
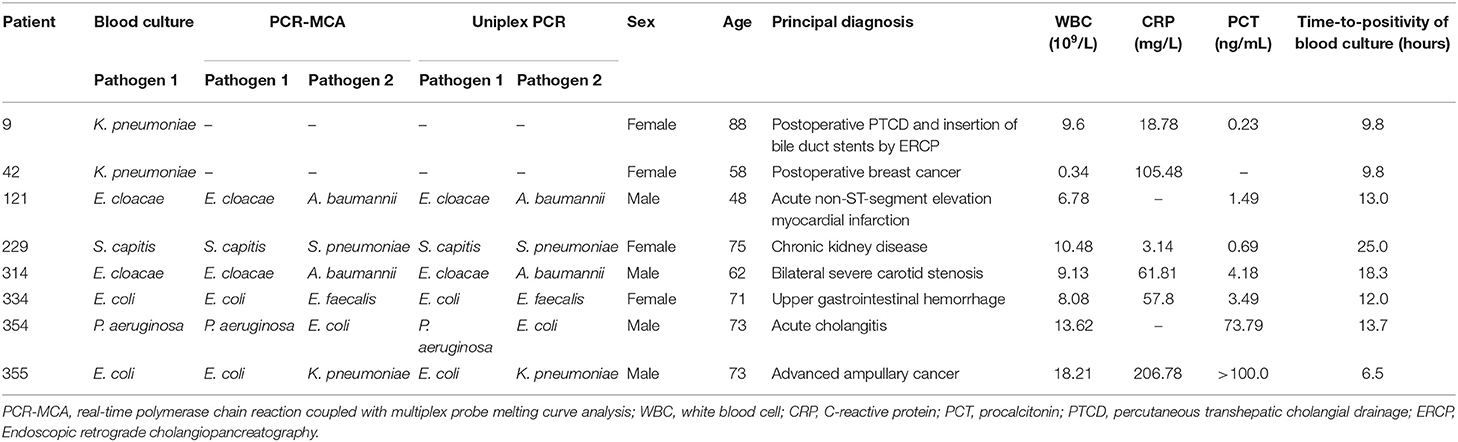
Table 5. Clinical characteristics of patients with discordant results between blood culture and the PCR-MCA assay.
Discussion
Delayed diagnosis of a pathogenic infection may increase the mortality rate of fatal sepsis. The earlier specific antibiotic administration is performed, the better the effect of the treatment and benefit to the patients (Dellinger et al., 2008). To date, the routine method for pathogen identification was blood culture, the whole procedure for which requires more than 2 days to perform and includes blood culture, Gram staining, plate culture and systematic microbial mass spectrometric identification. Because of the long duration of blood culture, empirical antibiotic therapy is administered by necessity (Singer et al., 2016), enhancing the problem of antibiotic resistance. In recent years, molecular biological methods have gradually been applied in pathogen detection in sepsis, reducing the detection duration while demonstrating excellent diagnostic performance (Rello et al., 2018).
The present study included 2,763 patients with 2,844 consecutive new episodes of suspected BSIs to evaluate a PCR-MCA assay for pathogen detection. The most common pathogen identified by blood culture was E. coli, followed by K. pneumoniae, S. aureus, S. epidermidis, A. baumannii, P. aeruginosa, S. pneumoniae, C. albicans, etc. The PCR-MCA assay could identify 11 kinds of gram-positive bacteria, 12 kinds of gram-negative bacteria, five kinds of fungi and two types of resistance genes. For the pathogens on the PCR-MCA list, the PCR-MCA assay discovered 99.2% (239/241) of the pathogens discovered by blood culture, with a specificity of 100% (2,575/2,575) and agreement of 99.9% (2,814/2,816) compared with the results of blood culture, suggesting that the assay presented excellent diagnostic performance compared with blood culture. For all the pathogens tested in clinical practice, the PCR-MCA assay also presented a good sensitivity of 88.8% (239/269) with a specificity of 100% (2,575/2,575) and agreement of 98.9% (2,814/2,844). For some prevalent pathogens, e.g., E. coli, A. baumannii, P. aeruginosa, S. aureus, S. epidermidis, and S. pneumoniae, the sensitivity of the PCR-MCA assay could reach 100%, while the specificity reached over 99%. Notably, for some samples with multiple pathogens identified simultaneously during one blood culture investigation, the PCR-MCA assay verified the results of blood culture, with an agreement rate of 100% for each. This finding may indicate that the PCR-MCA assay could be used to obtain early indication of multiple infections and help clinicians adopt the corresponding treatment strategy in a timely manner.
Discordance was observed in eight episodes of BSIs from eight cases. Two of them had negative results in the PCR-MCA assay but were identified with K. pneumoniae infection by blood culture. The TTP of both these cases was 9.8 h, much shorter than the average TTP of K. pneumoniae of 22 h (Ning et al., 2016). The TTP of an automated continuous blood culture system may indicate the original concentration of the organism (Da et al., 2016). It could be inferred that the original concentration of K. pneumoniae was sufficient, but the failure of the PCR-MCA assay to detect this organism may be due to the low sensitivity of this method compared to blood culture for K. pneumoniae detection. Samples of six other episodes were identified with one more pathogen than what was identified by blood culture. The TTP of blood culture of all these samples, which is defined as the length of time from the beginning of culture incubation to the detection of bacterial growth by an automated system (Tang et al., 2017), was ≤ 25 h. It could be inferred that the pathogen identified by blood culture was in dominance, which had a higher initial inoculum concentration or speed of growth, and its growth competitively inhibited others, known as “Jameson effect” (Mellefont et al., 2008). Non-dominant pathogens could also cause BSIs or originate from contamination.
The PCR-MCA assay is more advanced in some aspects than the existing blood culture techniques. First, <4 h were required to perform an entire PCR-MCA procedure. Pathogens of suspected BSIs could be identified within a short time, which will guide clinicians to adjust antibiotic therapy in a timely manner. In this way, the mortality of sepsis could be reduced, as well as the trends of resistance development. Second, the PCR-MCA assay could detect 28 kinds of pathogens simultaneously, which could prevent interference by the “Jameson effect” in mixed infection. In the blood culture process, identification and analysis of pathogens followed cultivation. When two or more pathogens are present in one sample, once the growth of dominant pathogens competitively inhibits others, the results of blood culture may falsely indicate single infection. Moreover, the PCR-MCA assay could detect two types of resistance genes, namely, vanA and mecA, along with pathogen detection, although none was identified in the present study. Third, the NPV of the PCR-MCA assay could reach 100% in detection of 27 kinds of pathogens on the panel except for K. pneumoniae. Even for pathogens not on the PCR-MCA list, the NPV reached over 99%. Since a sufficiently high NPV could provide high odds to exclude uninfected patients, unnecessary empirical antibiotic therapy could be avoided.
This study has several limitations that need to be addressed. First, not all of the pathogens that cause BSIs were included in this assay, and only 88.8% of the prevalent pathogens were identified, limited by the panel of detectable germs. Additional kinds of prevalent pathogens should be incorporated into this assay before clinical application. Second, the number of targeted pathogens, i.e., Serratia marcescens, Proteus mirabilis, Streptococcus pyogenes, Listeria monocytogenes, Candida parapsilosis, Candida krusei, and Aspergillus fumigatus, was insufficient for evaluation of diagnostic performance. The detection rate of the resistance genes mecA was 0, relatively low compared with the data reported by the China Antimicrobial Surveillance Network (http://www.chinets.com/), in which the prevalence of methicillin-resistant Staphylococcus aureus (MRSA) could be as high as 45.8% and the detection rate of mecA in MRSA could be 93.6% (Chen et al., 2012). That may be explained by the small number of MRSA (n = 4) in our study site, as well as the probability of a mecA-negative strain of Staphylococcus species with high-level beta-lactam resistance which has been reported (Banerjee et al., 2010). In addition, no vancomycin-resistant Enterococcus (VRE) species was identified in phenotypic susceptibility tests, consistent with the PCR-MCA result showing that the vanA gene was not detected. According to the China Antimicrobial Surveillance Network, the prevalence of VRE was 2.3%, and the detection rate of vanA in VRE could be 73.9% (Zhao et al., 2012). Larger samples are needed to verify the efficiency and accuracy of the PCR-MCA assay in future studies. Third, the resistance genes vanA and mecA included in the PCR-MCA assay were related to only gram-positive bacteria, so additional kinds of prevalent resistance genes should be incorporated into this assay for further study. Finally, samples with discordant results were further verified using a uniplex PCR assay and showed the same results as the PCR-MCA assay. Since the uniplex PCR detection system was built following the same principle as the PCR-MCA assay, the confirmation results may not be sufficiently objective, and a third detection method is necessary for evaluation. In addition, several positive PCR-MCA results but negative blood culture results were observed in this study, though the agreement rates of these two assays were high in general. Cross-reaction with other potential microorganisms in this assay should be investigated more extensively to promote clinical application.
In conclusion, our study demonstrated that the PCR-MCA assay is valuable proof of principle, simultaneously identifying 28 kinds of pathogens and two resistance genes in patients with suspected BSIs within a few hours. This novel assay exhibited considerable sensitivity and specificity and covered 88.8% of the pathogens in clinical practice. Rapid identification of pathogens involved in BSIs could help clinicians to make the correct diagnosis and guide antibiotic administration. Furthermore, additional kinds of prevalent pathogens could be selected to incorporate into this assay, which could be applied in clinical practice someday.
Data Availability Statement
All datasets generated for this study are included in the manuscript/supplementary files.
Ethics Statement
This study was approved by the Institutional Ethics Committee of Zhongshan Hospital, School of Medicine, Xiamen University, and was in compliance with national legislation and the Declaration of Helsinki guidelines. Written informed consent was obtained from the patient or, from next of kin or an independent patient advocate if the patient lacked this capacity.
Author Contributions
YX and XS wrote the main manuscript text. Q-FZ and Y-HY contributed to samples collection and laboratory tests. T-CY performed the statistical analysis. J-JN conceived the study and revised the manuscript.
Funding
This work was supported by the Guiding Projects of Fujian Science and Technology Program [grant number 2019D008], the Major Special Projects for Serious Illness of Xiamen [grant number 3502Z20179045], the Projects of Xiamen Science and Technology Program [grant number 3502Z20184057], and the Youth Foundation Projects of Fujian Provincial Health Department [grant number 2017-2-105]. The funders played no role in the study design, data collection, or analyses, the decision to publish, or manuscript preparation.
Conflict of Interest
The authors declare that the research was conducted in the absence of any commercial or financial relationships that could be construed as a potential conflict of interest.
Acknowledgments
We acknowledge Professor Qing-Ge Li and his colleagues from Engineering Research Center of Molecular Diagnostics at Xiamen University, Ministry of Education for their assistance in the construction of PCR-MCA assay.
References
Banerjee, R., Gretes, M., Harlem, C., Basuino, L., and Chambers, H. F. (2010). A mecA-negative strain of methicillin-resistant Staphylococcus aureus with high-level beta-lactam resistance contains mutations in three genes. Antimicrob. Agents Chemother. 54, 4900–4902. doi: 10.1128/aac.00594-10
Bochud, P. Y., Glauser, M. P., Calandra, T., and International Sepsis, F. (2001). Antibiotics in sepsis. Intensive Care Med. 27, S33–S48. doi: 10.1007/pl00003796
Chen, X., Yang, H. H., Huangfu, Y. C., Wang, W. K., Liu, Y., Ni, Y. X., et al. (2012). Molecular epidemiologic analysis of Staphylococcus aureus isolated from four burn centers. Burns 38, 738–742. doi: 10.1016/j.burns.2011.12.023
Da, R., Wu, Y., Liu, W., Shi, H., and Wang, W. (2016). Rapid time to positivity of cerebrospinal fluid culture with coagulase-negative Staphylococcus is more likely to reflect a true infection than contamination. World Neurosurg. 93, 330–335. doi: 10.1016/j.wneu.2016.06.056
Dellinger, R. P., Levy, M. M., Carlet, J. M., Bion, J., Parker, M. M., Jaeschke, R., et al. (2008). Surviving Sepsis Campaign: international guidelines for management of severe sepsis and septic shock: 2008. Crit. Care Med. 36, 296–327. doi: 10.1097/01.CCM.0000298158.12101.41
Galiana, A., Coy, J., Gimeno, A., Guzman, N. M., Rosales, F., Merino, E., et al. (2017). Evaluation of the Sepsis Flow Chip assay for the diagnosis of blood infections. PLoS ONE 12:e0177627. doi: 10.1371/journal.pone.0177627
Gustinetti, G., and Mikulska, M. (2016). Bloodstream infections in neutropenic cancer patients: a practical update. Virulence 7, 280–297. doi: 10.1080/21505594.2016.1156821
Jiang, Y., He, L., Wu, P., Shi, X., Jiang, M., Li, Y., et al. (2017). Simultaneous identification of ten bacterial pathogens using the multiplex ligation reaction based on the probe melting curve analysis. Sci. Rep. 7:5902. doi: 10.1038/s41598-017-06348-z
Liao, Y., Wang, X., Sha, C., Xia, Z., Huang, Q., and Li, Q. (2013). Combination of fluorescence color and melting temperature as a two-dimensional label for homogeneous multiplex PCR detection. Nucleic Acids Res. 41:e76. doi: 10.1093/nar/gkt004
Makristathis, A., Harrison, N., Ratzinger, F., Kussmann, M., Selitsch, B., Forstner, C., et al. (2018). Substantial diagnostic impact of blood culture independent molecular methods in bloodstream infections: superior performance of PCR/ESI-MS. Sci. Rep. 8:16024. doi: 10.1038/s41598-018-34298-7
Mellefont, L. A., McMeekin, T. A., and Ross, T. (2008). Effect of relative inoculum concentration on Listeria monocytogenes growth in co-culture. Int. J. Food Microbiol. 121, 157–168. doi: 10.1016/j.ijfoodmicro.2007.10.010
Ning, Y., Hu, R., Yao, G., and Bo, S. (2016). Time to positivity of blood culture and its prognostic value in bloodstream infection. Eur. J. Clin. Microbiol. Infect. Dis. 35, 619–624. doi: 10.1007/s10096-016-2580-5
Niu, J. J., Zhang, Q., Chen, F. Y., Liu, L., Liu, L. L., and Yang, T. C. (2015). The evaluation method for assessing a diagnostic test of HIV: some noteworthy issues. J. Clin. Virol. 65, 74–75. doi: 10.1016/j.jcv.2015.02.013
Opota, O., Jaton, K., and Greub, G. (2015). Microbial diagnosis of bloodstream infection: towards molecular diagnosis directly from blood. Clin. Microbiol. Infect. 21, 323–331. doi: 10.1016/j.cmi.2015.02.005
Peters, R. P., van Agtmael, M. A., Danner, S. A., Savelkoul, P. H., and Vandenbroucke-Grauls, C. M. (2004). New developments in the diagnosis of bloodstream infections. Lancet Infect. Dis. 4, 751–760. doi: 10.1016/S1473-3099(04)01205-8
Quiles, M. G., Boettger, B. C., Inoue, F. M., Monteiro, J., Santos, D. W., Ponzio, V., et al. (2019). Direct matrix-assisted laser desorption ionization time-of-flight mass spectrometry and real-time PCR in a combined protocol for diagnosis of bloodstream infections: a turnaround time approach. Braz. J. Infect. Dis. 23, 164–172. doi: 10.1016/j.bjid.2019.05.005
Ramanan, P., Bryson, A. L., Binnicker, M. J., Pritt, B. S., and Patel, R. (2018). Syndromic panel-based testing in clinical microbiology. Clin. Microbiol. Rev. 31:e00024–17. doi: 10.1128/CMR.00024-17
Rello, J., van Engelen, T. S. R., Alp, E., Calandra, T., Cattoir, V., Kern, W. V., et al. (2018). Towards precision medicine in sepsis: a position paper from the European Society of Clinical Microbiology and Infectious Diseases. Clin. Microbiol. Infect. 24, 1264–1272. doi: 10.1016/j.cmi.2018.03.011
Singer, M., Deutschman, C. S., Seymour, C. W., Shankar-Hari, M., Annane, D., Bauer, M., et al. (2016). The third international consensus definitions for sepsis and septic shock (sepsis-3). JAMA 315, 801–810. doi: 10.1001/jama.2016.0287
Tang, P. C., Lee, C. C., Li, C. W., Li, M. C., Ko, W. C., and Lee, N. Y. (2017). Time-to-positivity of blood culture: an independent prognostic factor of monomicrobial Pseudomonas aeruginosa bacteremia. J. Microbiol. Immunol. Infect. 50, 486–493. doi: 10.1016/j.jmii.2015.08.014
Zhao, C., Sun, H., Wang, H., Liu, Y., Hu, B., Yu, Y., et al. (2012). Antimicrobial resistance trends among 5608 clinical Gram-positive isolates in China: results from the Gram-Positive Cocci Resistance Surveillance program (2005–2010). Diagn. Microbiol. Infect. Dis. 73, 174–181. doi: 10.1016/j.diagmicrobio.2012.03.003
Keywords: PCR-MCA, bloodstream infections, pathogen detection, diagnostic performance, blood culture
Citation: Xiao Y, Shen X, Zhao Q-F, Yao Y-H, Yang T-C and Niu J-J (2019) Evaluation of Real-Time PCR Coupled With Multiplex Probe Melting Curve Analysis for Pathogen Detection in Patients With Suspected Bloodstream Infections. Front. Cell. Infect. Microbiol. 9:361. doi: 10.3389/fcimb.2019.00361
Received: 08 July 2019; Accepted: 07 October 2019;
Published: 22 October 2019.
Edited by:
Caspar Yvan, Centre Hospitalier Universitaire de Grenoble, FranceReviewed by:
Tam T. Van, Harbor–UCLA Medical Center, United StatesKrisztina M. Papp-Wallace, Louis Stokes Cleveland VA Medical Center, United States
Vittorio Sambri, University of Bologna, Italy
Copyright © 2019 Xiao, Shen, Zhao, Yao, Yang and Niu. This is an open-access article distributed under the terms of the Creative Commons Attribution License (CC BY). The use, distribution or reproduction in other forums is permitted, provided the original author(s) and the copyright owner(s) are credited and that the original publication in this journal is cited, in accordance with accepted academic practice. No use, distribution or reproduction is permitted which does not comply with these terms.
*Correspondence: Jian-Jun Niu, bml1amlhbmp1bjIxMUB4bXUuZWR1LmNu
†These authors have contributed equally to this work