- 1King Saud Bin Abdulaziz University for Health Sciences, Riyadh, Saudi Arabia
- 2Infectious Diseases Research Department, King Abdullah International Medical Research Center, Riyadh, Saudi Arabia
- 3Vaccine and Infectious Disease Organization–International Vaccine Center, University of Saskatchewan, Saskatoon, SK, Canada
- 4John H. Stroger Junior Hospital of Cook County, Chicago, IL, United States
- 5Ministry of National Guard-Health Affairs, Riyadh, Saudi Arabia
Non-typhoidal Salmonella (NTS) strains are Gram negative bacterial pathogens that are associated with foodborne illness worldwide. During the process of infection, Salmonella uses two molecular injectisomes known as Type 3 Secretion Systems (T3SS) to secrete virulence factors that are encoded by Salmonella Pathogenicity Island-1 (SPI-1) and SPI-2 into host cells. These secretion systems play a major role in virulence, as shown in various animal models, but little is known about their role in human infections. In Saudi Arabia, NTS strains frequently cause human infections but data regarding these pathogenic strains is fairly limited. The aim of this study was to characterize Salmonella human clinical isolates in Riyadh, Saudi Arabia, by determining their serotype, testing for the presence of SPI-1 and SPI-2 genes and to determine the antibiotic resistance profiles of these strains. Using the rapid Check and Trace Salmonella™ (CTS) system our results demonstrate that S. Enteritidis and S. Typhimurium were the predominant serovars, followed by S. Livingstone, S. Kentucky and S. Poona among a list of 36 serovars reported for the first time in the country. In addition, SPI-1 genes were detected in 99% of the isolates, while the sifA gene (SPI-2) was not detected in 13.5% of the isolates. These results suggest that both the SPI-1 and SPI-2 virulence determinants are important for human infection. Moreover, we report the presence of a Multi-Drug (MDR) carbapenem resistant S. Kentucky isolate harboring the blaOXA−48 gene not reported previously in Saudi Arabia.
Introduction
Salmonella enterica is a Gram negative facultative intracellular bacterial pathogen that is capable of causing disease in a variety of hosts. Salmonella enterica consists of more than 2600 serovars of which S. enterica subspecies enterica is comprised of serovars like S. Typhi that are host-specific, while others like S. Typhimurium are generalists (Porwollik et al., 2004; Gal-Mor, 2019). Typhoid fever and invasive disease are typical symptoms that are associated with human infections caused by S. Typhi (Dougan and Baker, 2014). Non-typhoidal Salmonella (NTS) species mostly cause self-limiting gastrointestinal illness which may lead to hospitalization (requiring antibiotic treatment) and death. However, in sub-Saharan Africa, NTS species cause invasive disease (iNTS) that manifests as bacteremia in 8–45% of all community-acquired blood stream infections resulting in an overall case fatality rate of 20.6% (Haselbeck et al., 2017; Uche et al., 2017). The main source of NTS infections are the consumption of contaminated poultry products, though recently many outbreaks have been linked to fresh produce (Hanning et al., 2009). The global burden of NTS is estimated at 93.8 million cases of human infections resulting in 155,000 deaths annually (Ao et al., 2015). According to the CDC, NTS species are the second leading cause of foodborne illness in the U.S. which is estimated at 1.2 million annual cases of Salmonellosis. This results in 19,336 hospitalizations, 17,000 quality adjusted life years lost and USD $3.3 billion in total medical expenditures and lost productivity annually (Cummings et al., 2012). In addition, S. Enteritidis and S. Typhimurium are the most common serotypes associated with human infection in Africa, Asia, Europe, Latin America and the Caribbean, and in North America (Galanis et al., 2006). However, in Saudi Arabia very little is known about the prevalence of Salmonella serovars or their virulence properties associated with human infection. This information is vital for determining the source of these infections in order to develop intervention strategies aimed at reducing the levels of Salmonella species across the country (Gast, 2007). Hence, NTS human infections translate into a significant impact on both the healthcare system and the economy of a given country (Ghunaim and Desin, 2015).
During the process of infection, Salmonella uses two specialized nanomachines known as Type 3 Secretion Systems (T3SS) that are encoded by Salmonella Pathogenicity Island-1 (SPI-1) (Collazo and Galán, 1997) and SPI-2 (Hensel, 2000), respectively, to inject virulence factors directly into host cells. Traditionally, the SPI-1 T3SS has been associated with bacterial invasion of the host intestinal epithelial cell surface, while the SPI-2 T3SS has been linked to intracellular survival and maintenance of the Salmonella Containing Vacuole (SCV) (Galán, 2001). However, there has been recent evidence suggesting that there is a certain level of cross-talk between the two T3SS, indicating that the two systems are closely linked in their virulence functions (Brown et al., 2005; Coburn et al., 2005; Coombes et al., 2005). Many in-vitro and in-vivo studies have shown that the two Salmonella T3SS are important virulence factors as the deletion of these systems results in attenuation (Zhang et al., 2002; Hapfelmeier et al., 2004; Raffatellu et al., 2005; Desin et al., 2009; Wisner et al., 2010; Buckner et al., 2011). In human infections, the roles of SPI-1 and SPI-2 have not been defined as clearly, though a study in China has indicated that clinical isolates from a food-borne disease outbreak of S. Senftenberg lacking SPI-1 were still able to cause gastroenteritis (Hu et al., 2008). Several genes that encode for T3SS components which consist of structural or secreted effectors including invA (SPI-1 structure), sipA (SPI-1 effector), sopE2 (SPI-1 effector), sifA (SPI-2 effector), and ssaR (SPI-2 structure) have been used as markers to test for the presence of these pathogenicity islands (Hu et al., 2008).
The emergence of antibiotic resistant bacteria worldwide is a significant public health concern resulting in 23,000 deaths per year in the U.S. at a cost of over $20 billion (Centers for Disease Control and Prevention, 2013). Moreover, drug-resistant NTS have been classified as a serious threat by the CDC with reports of increasing resistance to ceftriaxone and ciprofloxacin which are used as first-line antibiotic treatment. Alternative antimicrobials such as aminoglycosides, folic acid pathway inhibitors or the carbapenems are used for treatment of resistant NTS infections (Frye and Jackson, 2013). Therefore, from a patient safety perspective, it is critical to monitor antibiotic resistance patterns associated with human Salmonella infections. The objective of this study was to determine the prevalence of Salmonella serovars associated with human infections in Saudi Arabia using the rapid CTS system and to determine if the isolates contain SPI-1 and SPI-2 as well as characterizing the associated antimicrobial resistance.
Materials and Methods
Salmonella Clinical Isolates
NTS clinical isolates (n = 200) collected between May 2015 and Nov 2017 were obtained from the clinical microbiology laboratory at King Fahad Hospital (National Guard Health Affairs, King Abdulaziz Medical City, Riyadh). Isolates were from patients that presented with a variety of clinical symptoms ranging from gastrointestinal illness to systemic infection and were used in accordance with the ethics approval from the KAIMRC Institution Review Board (IRBC/040/16, IRBC/265/17, and IRBC/0768/18).
PCR and Sequencing
Gene amplifications were performed with the MegaMIX Blue master mix (Gel Company, USA) using bacterial genomic DNA extracted from overnight cultures with the MagNa Pure LC 2.0 nucleic acid purification system (Roche), unless stated otherwise. Sanger sequencing was performed using an ABI 3730xL Genetic Analyzer (Applied Biosystems) at the KAIMRC core facility.
Molecular Serotyping
Salmonella isolates were serotyped with the rapid CTS system (Checkpoints, Netherlands) (Wattiau et al., 2008b). The strains were streaked on Tryptic Soy Agar (TSA). Genomic DNA was extracted by resuspending a single colony from an agar plate into 100 μl Lysis buffer (CTS Kit) and incubated at 99°C for 15 min. The samples were serotyped using the PCR based approach described in the CTS manual as published previously (Ferrato et al., 2017). The samples were probed using specific DNA probes that correspond to an array pattern analyzed by the CTS software which assigns a Salmonella serovar to the unknown sample based on the available serovars in the CTS database. Serotypes of seven isolates that were not recognized by the CTS system were identified using Multi-Locus Sequence Typing (MLST) as an alternative serotyping method (Achtman et al., 2012). Briefly, the seven housekeeping genes, aroC, dnaN, hemD, hisD, purE, sucA, and thrA were amplified and sequenced. Samples were assigned alleles, sequence types (ST), and serotypes using the Achtman MLST database in Enterobase (http://enterobase.warwick.ac.uk) (Alikhan et al., 2018).
Detection of SPI-1 and SPI-2 Genes
In order to detect the presence of the two major SPIs in the Salmonella isolates as described previously (Hu et al., 2008), primers to amplify SPI-1 (invA, sipA, sopE2) and SPI-2 (sifA and ssaR) genes (Table 1) were designed using the NCBI Primer-BLAST tool corresponding to the S. Typhimurium LT2 genome. The reference strain, S. Typhimurium strain SL 1344 (provided by VIDO-InterVac, University of Saskatchewan, Canada) was used as a positive control. Randomly selected amplicons representing all the 5 genes were sequenced for further confirmation.
Antibiotic Resistance Determination
Minimum Inhibitory Concentrations (MICs) to Ampicillin (AMP), Amoxicillin/Clavulanic acid (AMC), Piperacillin/Tazobactam (TZP), Cefalotin (CF), Cefoxitin (FOX), Ceftazidime (CAZ), Ceftriaxone (CRO), Cefepime (FEP), Imipenem (IPM), Meropenem (MEM), Amikacin (AMK), Gentamicin (GEN), Ciprofloxacin (CIP), Tigecycline (TGC), Nitrofurantoin (NIT), and Trimethoprim/Sulfamethoxazole (SXT) were determined using the Antimicrobial Susceptibility Test (AST) card AST-N291 for Gram negative bacteria for VITEK® 2 Systems (BioMérieux) as described elsewhere (MacLowry and Marsh, 1968; Tilton et al., 1973; Bobenchik et al., 2017). The MICs were interpreted according to Clinical and Laboratory Standards Institute (CLSI) breakpoints (CLSI M100-ED28, 2018).
Amplification of Antibiotic Resistance Genes
The detection of antibiotic resistance determinants was performed by PCR and sequencing using primers (Table 1) and amplification conditions as described by others (Weill et al., 2006; Lynne et al., 2008; Wang et al., 2017). Genes encoding CTX-M enzymes of groups 1, 2, and 9, plasmid-mediated AmpC of groups ACC, CIT, DHA, ENT/ENC, FOX and MOX, carbapenemase NDM, OXA-48-like, IMP, VIM as well as TEM, SHV, OXA-1, PSE-1/CARB-2 were sought by PCR in isolates showing resistance to one of the tested β-lactams. Quinolone resistance determinant regions (QRDRs) from gyrA and parC genes were sequenced and analyzed for point mutations that confer ciprofloxacin resistance (Hopkins et al., 2005).
Results
S. Enteritidis and S. Typhimurium Are the Predominant Serotypes
The majority (99%, 198/200) of the Salmonella species tested in this study belonged to S. enterica subspecies enterica and were comprised of 36 different serotypes (Table 2). The serotyping was mainly determined by the CTS system, while serotypes of 7 isolates that were untypeable using this approach were inferred from MLST. S. Enteritidis (40%, 80/200) was by far the most frequently isolated serovar, followed to a lesser extent by S. Typhimurium (13.5%, 27/200), S. Livingstone (5%, 10/200), S. Kentucky, S. Poona (4.5%, 9/200), S. Agona and S. Newport (2.5%, 5/200), and S. Give (2%, 4/200). The remaining serotypes had a prevalence ranging from one to three isolates each. Serotypes identified by MLST were S. Corvallis (ST2542, 2/200), S. Telhashomer (ST2399, 1/200) and S. Obogu (ST3335, 2/200), while one isolate could not be matched to any serotype in the database (ST3774) and one could not be identified.
Systemic Infection Was Mainly Associated With S. Enteritidis and S. Typhimurium
The human clinical isolates (n = 170) were mainly isolated from stool samples and were comprised of 34 serotypes, with S. Enteritidis (38.8%, 66/170) and S. Typhimurium (12.4%, 21/170) being the most common (Table 3). The remaining strains were from the blood (n = 16), urine (n = 7), wound (n = 4), abdominal fluid (n = 2) or tissue (n = 1). Interestingly, 11.3% (9/80) of the S. Enteritidis strains were isolated from the blood, while only 7.4 % (2/27) of the S. Typhimurium strains were isolated from the same site. In addition to the aforementioned serotypes, non-fecal isolates were also isolated from the urine (S. Poona), wound (S. Agona and S. Minnesota), and blood (S. Poona and S. Urbana). However, S. Livingstone and S. Kentucky, despite being the third and fourth most prevalent serovars (Table 2), were exclusively isolated from stool samples. Taken together, this may suggest that S. Enteritidis may have a higher tendency of causing system infection relative to the other serotypes.
SPI Genes Were Present in the Majority of Clinical Isolates
The SPI-1 (invA, sipA, sopE2) and SPI-2 (sifA, ssaR) genes were present in 84.5% (169/200) of the Salmonella strains (Table 4). The pathogenicity island genes were not detected in 15.5% (31/200) of the isolates in the form of either a SPI-1 gene (1%, 2/200 isolates), SPI-2 gene (13.5%, 27/200 isolates) or both SPI-1 and SPI-2 genes (1%, 2/200 isolates). The sopE2 gene (SPI-1) was absent in a single isolate each belonging to S. Schwarzengrund and S. Kentucky. In contrast, the SPI-2 gene, sifA, was not detected in 13.5% (27/200) strains corresponding to various serotypes. The ssaR gene (SPI-2) was present in all S. enterica subspecies tested, except for the non-enterica subspecies, which were also missing other SPI-1 and SPI-2 genes. Additionally, it is noteworthy to highlight that 97% (28/29) of the Salmonella isolates lacking the sifA gene were exclusively isolated from fecal samples suggesting that SPI-2 may be important in human systemic infection.
Antibiotic Resistance Profiles of the Salmonella Strains
Overall, the Salmonella strains demonstrated the highest level of antibiotic resistance against nitrofurantoin (17%, 34/200) (Table 5) with the majority being associated with S. Enteritidis (88.2%, 30/34) (Table 6). This was followed by resistance to ampicillin (13.5%, 27/200), cefalotin (10%, 20/200), tigecycline (10%, 20/200), ciprofloxacin (8.5%, 17/200), and trimethoprim/sulfamethoxazole (8%, 16/200) (Table 5). Resistance to other β-lactams including cephalosporins and carbapenems, as well as aminoglycosides, was below 5%. However, a significant number of isolates (61.5%, 123/200) were fully susceptible to all the tested antibiotics (Table 6). Interestingly, 13 strains identified as S. Agona (n = 3), S. Blockley (n = 1), S. Enteritidis (n = 1), S. Havana (n = 1), S. Kentucky (n = 1), S. Minnesota (n = 2), S. Newport (n = 1), and S. Typhimurium (n = 3) were Multi-Drug Resistant (MDR) strains as they demonstrated resistance to more than three antibiotic classes. In addition, one single isolate of S. Kentucky conferred resistance to carbapenem (imipenem and meropenem) and to ten other antibiotics (AMP, AMC, TZP, CF, CAZ, CRO, FEP, CIP, TGC, and SXT) making this strain a unique MDR isolate in the region.
Characterization of Resistance to β-Lactam Antibiotics
Twenty-seven isolates (13.5%) (Table 7), which were mainly associated with S. Typhimurium (41%, 11/27), that had resistance to at least one of the β-lactam antibiotics were tested for the presence of β-lactam genes. The majority (66.6%, 18/27) of these strains were only resistant to ampicillin and carried the blaTEM−1 (n = 16) or blaPSE−1 (n = 2) genes. Of the remainder, three isolates had resistance to ceftazidime of which two were also resistant to piperacillin/tazobactam and amoxicillin/clavulanic acid and carried the blaCMY−2 gene, while one isolate remained susceptible to the two β-lactam/β-lactam inhibitor combinations and harbored the blaTEM−1 and blaCTX−M−1−group genes. In addition, three other isolates had resistance to the β-lactam/β-lactam inhibitors but remained susceptible to cephalosporins with one strain carrying the blaOXA−1 gene and the two others only containing the blaTEM−1 genes. The MDR S. Kentucky isolate described above that conferred resistance to all the β-lactam antibiotics, including carbapenem, contained the blaCTX−M−9−group and blaOXA−48−like carbapenemase genes.
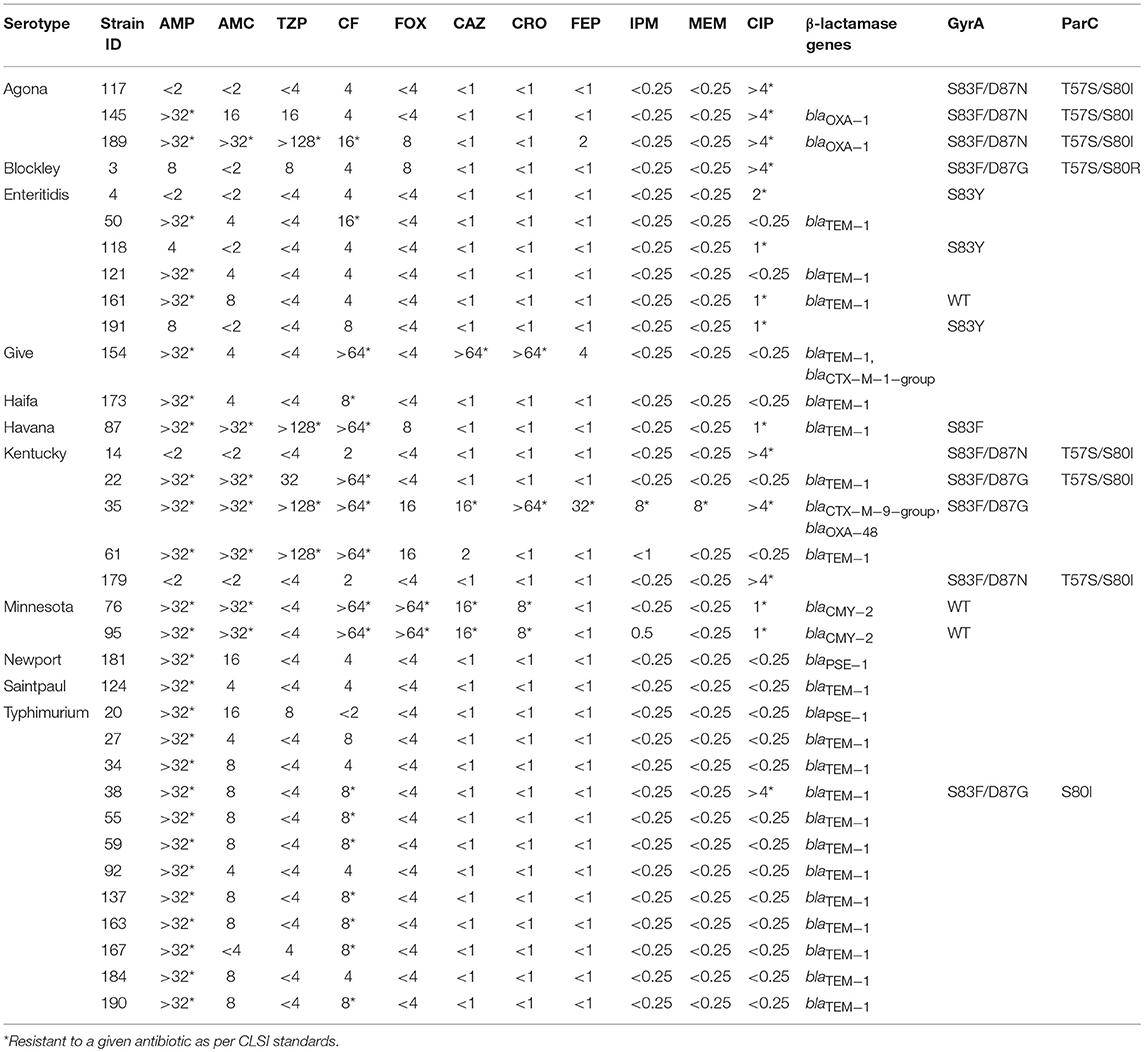
Table 7. Genotypes for Salmonella strains with resistance to β-lactam antibiotics and Ciprofloxacin.
Characterization of Genes Encoding Resistance to Ciprofloxacin
Sixteen (8%) Salmonella strains had reduced susceptibility to ciprofloxacin with MICs ranging from 1 to ≥ 4 μg/ml (Table 7). Resistance was primarily seen in S. Agona (60%, 3/5 isolates), S. Kentucky (40%, 4/10) and S. Enteritidis (5%, 4/80). In order to identify mutations encoding for ciprofloxacin resistance, PCR was performed on the gyrA and parC genes. Eight isolates with an MIC ≥ 4 μg/ml had mutations in GyrA (S83F/Y and D87G/N) and ParC (T57S and S80I/R) amino acid sequences. In contrast, five of the isolates with MICs between 1 and 2 μg/ml had only a single mutation in the GyrA (S83Y/F) amino acid sequence, while three strains had no detectable mutations.
Discussion
NTS species are a public health concern as they are prevalent worldwide causing a significant number of human infections (Gal-Mor, 2019). Rapid identification of Salmonella species associated with human infections is a critical component of a Salmonella control program since this allows for epidemiological investigations that can assist in determining the source of the infections (Ferrato et al., 2017). In addition, the presence of important virulence factors such as SPI-1 and SPI-2 enhance our understanding of the virulence potential of these strains (Dos Santos et al., 2019). Similarly, characterization of antimicrobial resistance associated with these strains can further assist in reducing the spread of antibiotic resistance mechanisms within Salmonella species (Parisi et al., 2018). The aim of the present study was to serotype Salmonella species associated with human infections in Saudi Arabia using a rapid method (CTS system) and to characterize the clinical isolates by testing for the presence of SPI-1 and SPI-2 virulence determinants as well as determining antimicrobial resistance patterns.
We chose to use the CTS system since it is a rapid technique that can be used for serotyping Salmonella species with a turnaround time of 8 hours. This system has been shown to be highly effective and accurate in determining Salmonella serotypes (Wattiau et al., 2008a; Ferrato et al., 2017). The CTS system was able to identify 96.5% of the Salmonella clinical isolates used in the current study. This establishes a fast and reliable method for the identification of Salmonella species associated with human infections in Saudi Arabia and in the region. The remaining isolates were identified using MLST which has been used successfully for the serotyping of Salmonella species (Achtman et al., 2012). The results from MLST imply that some of the serotypes not identified by the CTS were rare serotypes like S. Obogu and S. Telhashomer that are not present in the CTS database. In addition, ST3774 and the strain that was not identified by MLST could possibly be novel serotypes that are associated with human infection in Saudi Arabia. Our results indicate that the predominant serovars associated with human infections in Riyadh are S. Enteritidis and S. Typhimurium, followed by S. Livingstone, S. Kentucky and S. Poona, respectively. This is the first report in the region which demonstrates that besides S. Enteritidis and S. Typhimurium being the leading cause of human infections, serotypes S. Livingstone, S. Kentucky, and S. Poona are also significant. Moreover, the data also show that 36 unique Salmonella serotypes were circulating among the human population in Riyadh (Saudi Arabia). The fact that S. Enteritidis and S. Typhimurium are the most common serotypes is in agreement with surveillance data from the U.S. reported by FoodNET (Marder Mph et al., 2018) and the European Union (Gal-Mor, 2019). These results are also supported by a preliminary study performed in Saudi Arabia where the authors examined 33 isolates which included Typhoidal Salmonella species (El-Tayeb et al., 2017). Our results differ with respect to the third, fourth and fifth most common serovars since FoodNET has indicated that they are S. Newport, S. Javiana and the monophasic variant of S. Typhimurium (I 4, [5],12:i:-) (Marder Mph et al., 2018), in decreasing order, while in the European Union they are monophasic S. Typhimurium, S. Infantis and S. Derby (Gal-Mor, 2019). The differences seen in the list of prevalent serovars may arise due to differences in food consumption habits, geographical locations and climate which may give certain serovars advantages over others. As well, a larger sample size of Salmonella strains in Saudi Arabia may reveal a different distribution of serotypes across the country.
The main Salmonella serovars identified in this study, S. Enteritidis and S. Typhimurium, were also isolated from non-fecal samples such as the blood. This may suggest that these strains, especially S. Enteritidis, are more invasive and have the potential to cause systemic infection compared to the other serotypes. However, this conclusion has to be confirmed with a larger sample size that includes regions from across the country as it is possible that this observation is only being seen since the aforementioned serotypes are more frequently isolated. As well, we are unable to determine if any of the isolates caused iNTS at this time as there is no data available on the presence of these strains in Saudi Arabia or in the region.
Traditionally, the prevailing view was that SPI-1 was mainly associated with intestinal invasion of epithelial cells, while SPI-2 was involved in systemic infection (Hansen-Wester and Hensel, 2001). The observation that there is a certain level of cross talk between the two secretion systems has led to an understanding that both SPI-1 and SPI-2 are important virulence factors during the process of infection (Moest and Méresse, 2013). However, the majority of the data has been derived from cell culture models or animal models of infection. Relatively, the role of SPI-1 and SPI-2 in human infections is still not clearly defined. The present study was an attempt to determine if SPI-1 and SPI-2 are present in clinical isolates associated with human disease in Saudi Arabia. Our data clearly show that SPI-1 was present in the majority of the Salmonella strains and that strains missing SPI-1 genes only had a single missing gene like sopE2. The absence of the aforementioned SPI-1 genes indicates that these strains are capable of forming a fully functional SPI-1 T3SS with a minimal effect, if any, on virulence since the effector proteins encoded by these genes are known to have important but redundant roles (Zhang et al., 2018). On the other hand, the SPI-2 gene sifA was not detected in several isolates, while the ssaR gene (SPI-2) was present in all the samples. This demonstrates that an intact SPI-2 T3SS was likely present in the sifA deficient isolates, since this gene is located outside SPI-2 and plays a role in the formation of Salmonella induced filaments and maintenance of the Salmonella containing vacuole (Stein et al., 1996). However, sifA mutants are known to be highly attenuated in systemic infection and intracellular replication in mice (Beuzón et al., 2000; Rajashekar et al., 2014), thereby suggesting that isolates with these genotypic characteristics might have a reduced systemic infection potential in humans, though the exact role of SPI-2 in human infection remains to be elucidated. Our SPI-1 results are not in agreement with a study in China which indicated that SPI-1 was not required for human gastroenteritis since two S. Senftenberg strains isolated from a food-borne disease outbreak were SPI-1 deficient (Hu et al., 2008). However, our finding that SPI-2 genes, with the exception of sifA, were present in all the test strains imply that SPI-2 is associated with human disease and are in accordance with the findings of the former study (Hu et al., 2008). Another noteworthy observation was that 97% (28/29) of the sifA deficient strains were only isolated from stool samples (data not shown), implying that SPI-2 is important in human infection. However, a detailed analysis of the exact role of the individual SPI-1 and SPI-2 effectors during human enterocolitis remains to be elucidated.
Antibiotic resistance has been reported in Salmonella species since the 1990's (Threlfall et al., 2000) and it appears that resistance is on a rise (Frye and Jackson, 2013). Our findings indicate that antibiotic resistance among the strains tested were the highest to nitrofurantoin, followed by ampicillin, cefalotin, tigecycline, ciprofloxacin, and trimethoprim/sulfamethoxazole. The rate of resistance to ampicillin is in agreement with a previous report from the U.S. where resistance was 14% among 23,585 human Salmonella isolates (Frye and Jackson, 2013). However, Ciprofloxacin resistance in our study (8%) was significantly higher and in contrast to the findings of this report (0.11%), but in agreement with data from countries in the Arab League (Moghnieh et al., 2018). The U.S. study did not assess resistance to Nitrofurantoin, Cefalotin or Tigecycline making the comparison with our data difficult (Frye and Jackson, 2013). Nevertheless, resistance to other antibiotics in our study like Amikacin, Gentamicin, Amoxicillin/Clavulanic acid and Ceftriaxone were similar to those reported. Additionally, our data also suggest that S. Agona, S. Give, S. Havana, S. Kentucky, and S. Minnesota are MDR strains, which is in line with similar observations by others for serotypes like S. Agona (Kuang et al., 2014; Irfan et al., 2015), S. Kentucky (Le Hello et al., 2013; Seiffert et al., 2014), and S. Minnesota (Campos et al., 2018), while it is rare for S. Give and S. Havana (Bekal et al., 2013).
Our observation that 70% of our test strains conferring β-lactam resistance contained the blaTEM−1 gene while very few strains contained blaPSE−1/blaCARB−2 and blaOXA−1 is also supported by a report from the UK where the authors examined 3,491 Salmonella isolates (Neuert et al., 2018). These results are partially in agreement with a study performed in Shanghai where β-lactam resistance was distributed between the blaTEM and blaOXA genes (Wang et al., 2017). However, our results are in contrast to data from France where the blaPSE−1 gene was predominantly associated with β-lactam resistance whereas the blaTEM gene was detected at low levels (Weill et al., 2006). The latter two studies may not be an accurate comparison with our work since they focused on S. Typhimurium. The presence of CTX-M-type extended spectrum β-lactamases was detected in only 2 isolates (S. Give and S. Kentucky) in our study and is similar to what has been previously published (Wang et al., 2017; Neuert et al., 2018). Our finding that the MDR carbapenem S. Kentucky strain contained the blaOXA−48 gene is a significant finding and the first report of its kind in Saudi Arabia. The association of this strain with human infections is concerning due to the fact that an increase in the number of such strains can lead to new challenges faced by physicians who encounter patients infected with this strain. Similar findings associated with S. Kentucky are rare, but have been reported previously in other countries (Le Hello et al., 2013; Seiffert et al., 2014), implying that this serotype may be an emerging MDR pathogen and may require increased surveillance in order to minimize outbreaks related to such strains. The association of mutations with the gyrA and parC genes when associated with ciprofloxacin resistance is evident from our data since an MIC >4 μg/ml resulted in two point mutations in the gyrA gene (codons 83 and 87) and parC gene (codons 57 and 80) as shown elsewhere (Weill et al., 2006; Wang et al., 2017; Neuert et al., 2018).
Taken together, we have established that the CTS system can be used to rapidly serotype Salmonella species in Riyadh. We have demonstrated that S. Enteritidis and S. Typhimurium are the most prevalent serovars associated with human infection in Riyadh (Saudi Arabia) and that S. Livingstone, S. Kentucky and S. Poona are also significant among a list of 36 serovars. Our data further suggest that important virulence factors like SPI-1 and SPI-2 were present in these isolates and strains lacking sifA were not associated with systemic infection. However, extensive work is still required to determine the roles of these T3SS in human enterocolitis and systemic infection. We also report the finding of a unique MDR resistant S. Kentucky isolate conferring carbapenem resistance. Further, our results indicate that there is a rising trend of ciprofloxacin and nitrofurantoin resistance among Salmonella species in the region.
Data Availability
The raw data supporting the conclusions of this manuscript will be made available by the authors, without undue reservation, to any qualified researcher.
Author Contributions
TD, AM, SA, HB designed the study. TD, AM, and SA assisted in sample collection. MA did the majority of the experimental work, while MAZ was instrumental in MLST PCR and confirming carbapenem resistance. TD analyzed all the serotyping data. MFA analyzed all the MLST Data and the sequencing results. MD and TD led the AMR work and analysis. TD and WK led the detection of SPI-1 and SPI-2 and analyzed the results. TD wrote the manuscript with major contributions from MA, MD, and WK. AM, SA, and HB critically read the manuscript with important feedback and input. All the authors contributed to, read and approved the final manuscript.
Conflict of Interest Statement
The authors declare that the research was conducted in the absence of any commercial or financial relationships that could be construed as a potential conflict of interest.
Acknowledgments
We would like to thank Ms. Grace Lavadia and other members of the Clinical Microbiology Laboratory at the National Guard Health Affairs Hospital (Riyadh) for kindly providing us with the Salmonella strains. Special thanks to Dr. Aneta Karczmerac and Mr. Joost Thijssen at Checkpoints for assistance with serotyping. Dr. Linda Chui at the Alberta Provincial Health Laboratory (Canada) was very supportive of this work. The S. Typhimurium SL1344 control strain was provided to us by VIDO-InterVac at the University of Saskatchewan (Canada). We also thank all the members of the Infectious Diseases Research Department at KAIMRC for their assistance and support throughout this project. The KAIMRC Contracts Manager, Mr. Bandar Ahmed, was very helpful with logistical support required for this project. We thank KAIMRC (Riyadh) for funding this project as RC 15/088.
References
Achtman, M., Wain, J., Weill, F. X., Nair, S., Zhou, Z., Sangal, V., et al. (2012). Multilocus sequence typing as a replacement for serotyping in Salmonella enterica. PLoS Pathog. 8:e1002776. doi: 10.1371/journal.ppat.1002776
Alikhan, N. F., Zhou, Z., Sergeant, M. J., and Achtman, M. (2018). A genomic overview of the population structure of Salmonella. PLoS Genet. 14:e1007261. doi: 10.1371/journal.pgen.1007261
Ao, T. T., Feasey, N. A., Gordon, M. A., Keddy, K. H., Angulo, F. J., and Crump, J. A. (2015). Global burden of invasive nontyphoidal Salmonella disease, 2010. Emerg. Infect. Dis. 21, 941–949. doi: 10.3201/eid2106.140999
Bekal, S., Lefebvre, B., Bergevin, M., and Tremblay, C. (2013). CTX-M-15 type ESBL-producing Salmonella Havana associated with international adoption in Canada. Can. J. Microbiol. 59:57. doi: 10.1139/cjm-2012-0667
Beuzón, C. R., Méresse, S., Unsworth, K. E., Ruíz-Albert, J., Garvis, S., Waterman, S. R., et al. (2000). Salmonella maintains the integrity of its intracellular vacuole through the action of SifA. EMBO J. 19, 3235–3249. doi: 10.1093/emboj/19.13.3235
Bobenchik, A. M., Deak, E., Hindler, J. A., Charlton, C. L., and Humphries, R. M. (2017). Performance of vitek 2 for antimicrobial susceptibility testing of Acinetobacter baumannii, Pseudomonas aeruginosa, and Stenotrophomonas maltophilia with Vitek 2 (2009 FDA) and CLSI M100S 26th edition breakpoints. J. Clin. Microbiol. 55, 450–456. doi: 10.1128/JCM.01859-16
Brown, N. F., Vallance, B. A., Coombes, B. K., Valdez, Y., Coburn, B. A., and Finlay, B. B. (2005). Salmonella pathogenicity island 2 is expressed prior to penetrating the intestine. PLoS Pathog. 1:e32. doi: 10.1371/journal.ppat.0010032
Buckner, M. M., Croxen, M. A., Arena, E. T., and Finlay, B. B. (2011). A comprehensive study of the contribution of Salmonella enterica serovar Typhimurium SPI2 effectors to bacterial colonization, survival, and replication in typhoid fever, macrophage, and epithelial cell infection models. Virulence 2, 208–216. doi: 10.4161/viru.2.3.15894
Campos, J., Mourão, J., Silveira, L., Saraiva, M., Correia, C. B., Maçãs, A. P., et al. (2018). Imported poultry meat as a source of extended-spectrum cephalosporin-resistant CMY-2-producing Salmonella Heidelberg and Salmonella Minnesota in the European Union, 2014-2015. Int. J. Antimicrob. Agents 51, 151–154. doi: 10.1016/j.ijantimicag.2017.09.006
Centers for Disease Control and Prevention (2013). Antibiotic Resistance Threats in the United States. Centers for Disease Control and Prevention.
Coburn, B., Li, Y., Owen, D., Vallance, B. A., and Finlay, B. B. (2005). Salmonella enterica serovar Typhimurium pathogenicity island 2 is necessary for complete virulence in a mouse model of infectious enterocolitis. Infect. Immun. 73, 3219–3227. doi: 10.1128/IAI.73.6.3219-3227.2005
Collazo, C. M., and Galán, J. E. (1997). The invasion-associated type-III protein secretion system in Salmonella–a review. Gene 192, 51–59. doi: 10.1016/S0378-1119(96)00825-6
Coombes, B. K., Coburn, B. A., Potter, A. A., Gomis, S., Mirakhur, K., Li, Y., et al. (2005). Analysis of the contribution of Salmonella pathogenicity islands 1 and 2 to enteric disease progression using a novel bovine ileal loop model and a murine model of infectious enterocolitis. Infect. Immun. 73, 7161–7169. doi: 10.1128/IAI.73.11.7161-7169.2005
Cummings, P. L., Sorvillo, F., and Kuo, T. (2012). “The burden of salmonellosis in the United States,” in Salmonella-A Dangerous Food Pathogen, ed B. S. M. Mahmoud (London, UK: Intech), 1–20.
Desin, T. S., Lam, P. K., Koch, B., Mickael, C., Berberov, E., Wisner, A. L., et al. (2009). Salmonella enterica serovar enteritidis pathogenicity island 1 is not essential for but facilitates rapid systemic spread in chickens. Infect. Immun. 77, 2866–2875. doi: 10.1128/IAI.00039-09
Dolejska, M., Masarikova, M., Dobiasova, H., Jamborova, I., Karpiskova, R., Havlicek, M., et al. (2016). High prevalence of Salmonella and IMP-4-producing Enterobacteriaceae in the silver gull on Five Islands, Australia. J. Antimicrob. Chemother. 71, 63–70. doi: 10.1093/jac/dkv306
Dos Santos, A. M. P., Ferrari, R. G., and Conte-Junior, C. A. (2019). Virulence factors in Salmonella Typhimurium: the sagacity of a bacterium. Curr. Microbiol. 76, 762–773. doi: 10.1007/s00284-018-1510-4
Dougan, G., and Baker, S. (2014). Salmonella enterica serovar Typhi and the pathogenesis of typhoid fever. Annu. Rev. Microbiol. 68, 317–336. doi: 10.1146/annurev-micro-091313-103739
El-Tayeb, M. A., Ibrahim, A. S. S., Al-Salamah, A. A., Almaary, K. S., and Elbadawi, Y. B. (2017). Prevalence, serotyping and antimicrobials resistance mechanism of Salmonella enterica isolated from clinical and environmental samples in Saudi Arabia. Braz. J. Microbiol. 48, 499–508. doi: 10.1016/j.bjm.2016.09.021
Ferrato, C., Chui, L., King, R., and Louie, M. (2017). Utilization of a molecular serotyping method for Salmonella enterica in a routine laboratory in Alberta Canada. J. Microbiol. Methods 135, 14–19. doi: 10.1016/j.mimet.2017.01.018
Frye, J. G., and Jackson, C. R. (2013). Genetic mechanisms of antimicrobial resistance identified in Salmonella enterica, Escherichia coli, and Enteroccocus spp. isolated from U.S. food animals. Front. Microbiol. 4:135. doi: 10.3389/fmicb.2013.00135
Galán, J. E. (2001). Salmonella interactions with host cells: type III secretion at work. Annu. Rev. Cell Dev. Biol. 17, 53–86. doi: 10.1146/annurev.cellbio.17.1.53
Galanis, E., Lo Fo Wong, D. M., Patrick, M. E., Binsztein, N., Cieslik, A., Chalermchikit, T., et al. (2006). Web-based surveillance and global Salmonella distribution, 2000-2002. Emerg. Infect. Dis. 12, 381–388. doi: 10.3201/eid1205.050854
Gal-Mor, O. (2019). Persistent infection and long-term carriage of typhoidal and nontyphoidal salmonellae. Clin. Microbiol. Rev. 32, 1–31. doi: 10.1128/CMR.00088-18
Gast, R. K. (2007). Serotype-specific and serotype-independent strategies for preharvest control of food-borne Salmonella in poultry. Avian Dis. 51, 817–828. doi: 10.1637/8090-081807.1
Ghunaim, H., and Desin, T. S. (2015). Potential impact of food safety vaccines on health care costs. Foodborne Pathog. Dis. 12, 733–740. doi: 10.1089/fpd.2014.1924
Hanning, I. B., Nutt, J. D., and Ricke, S. C. (2009). Salmonellosis outbreaks in the United States due to fresh produce: sources and potential intervention measures. Foodborne Pathog. Dis. 6, 635–648. doi: 10.1089/fpd.2008.0232
Hansen-Wester, I., and Hensel, M. (2001). Salmonella pathogenicity islands encoding type III secretion systems. Microbes Infect. 3, 549–559. doi: 10.1016/S1286-4579(01)01411-3
Hapfelmeier, S., Ehrbar, K., Stecher, B., Barthel, M., Kremer, M., and Hardt, W. D. (2004). Role of the Salmonella pathogenicity island 1 effector proteins SipA, SopB, SopE, and SopE2 in Salmonella enterica subspecies 1 serovar Typhimurium colitis in streptomycin-pretreated mice. Infect. Immun. 72, 795–809. doi: 10.1128/IAI.72.2.795-809.2004
Haselbeck, A. H., Panzner, U., Im, J., Baker, S., Meyer, C. G., and Marks, F. (2017). Current perspectives on invasive nontyphoidal Salmonella disease. Curr. Opin. Infect. Dis. 30, 498–503. doi: 10.1097/QCO.0000000000000398
Hensel, M. (2000). Salmonella pathogenicity island 2. Mol. Microbiol. 36, 1015–1023. doi: 10.1046/j.1365-2958.2000.01935.x
Hopkins, K. L., Davies, R. H., and Threlfall, E. J. (2005). Mechanisms of quinolone resistance in Escherichia coli and Salmonella: recent developments. Int. J. Antimicrob. Agents 25, 358–373. doi: 10.1016/j.ijantimicag.2005.02.006
Hu, Q., Coburn, B., Deng, W., Li, Y., Shi, X., Lan, Q., et al. (2008). Salmonella enterica serovar Senftenberg human clinical isolates lacking SPI-1. J. Clin. Microbiol. 46, 1330–1336. doi: 10.1128/JCM.01255-07
Hujer, K. M., Hujer, A. M., Hulten, E. A., Bajaksouzian, S., Adams, J. M., Donskey, C. J., et al. (2006). Analysis of antibiotic resistance genes in multidrug-resistant Acinetobacter sp. isolates from military and civilian patients treated at the Walter Reed Army Medical Center. Antimicrob. Agents Chemother. 50, 4114–4123. doi: 10.1128/AAC.00778-06
Irfan, S., Khan, E., Jabeen, K., Bhawan, P., Hopkins, K. L., Day, M., et al. (2015). Clinical isolates of Salmonella enterica serovar Agona producing NDM-1 metallo-beta-lactamase: first report from Pakistan. J. Clin. Microbiol. 53, 346–348. doi: 10.1128/JCM.02396-14
Kuang, D., Zhang, J., Meng, J., Yang, X., Jin, H., Shi, W., et al. (2014). Antimicrobial susceptibility and molecular typing of Salmonella agona isolated from humans and other sources. Foodborne Pathog. Dis. 11, 844–849. doi: 10.1089/fpd.2014.1776
Le Hello, S., Harrois, D., Bouchrif, B., Sontag, L., Elhani, D., Guibert, V., et al. (2013). Highly drug-resistant Salmonella enterica serotype Kentucky ST198-X1: a microbiological study. Lancet Infect. Dis. 13, 672–679. doi: 10.1016/S1473-3099(13)70124-5
Lynne, A. M., Rhodes-Clark, B. S., Bliven, K., Zhao, S., and Foley, S. L. (2008). Antimicrobial resistance genes associated with Salmonella enterica serovar newport isolates from food animals. Antimicrob. Agents Chemother. 52, 353–356. doi: 10.1128/AAC.00842-07
MacLowry, J. D., and Marsh, H. H. (1968). Semiautomatic microtechnique for serial dilution-antibiotic sensitivity testing in the clinical laboratory. J. Lab. Clin. Med. 72, 685–687.
Marder Mph, E. P., Griffin, P. M., Cieslak, P. R., Dunn, J., Hurd, S., Jervis, R., et al. (2018). Preliminary incidence and trends of infections with pathogens transmitted commonly through food - foodborne diseases active surveillance network, 10 U.S. sites, 2006-2017. Morb. Mortal Wkly. Rep. 67, 324–328. doi: 10.15585/mmwr.mm6711a3
Moest, T. P., and Méresse, S. (2013). Salmonella T3SSs: successful mission of the secret(ion) agents. Curr. Opin. Microbiol. 16, 38–44. doi: 10.1016/j.mib.2012.11.006
Moghnieh, R. A., Kanafani, Z. A., Tabaja, H. Z., Sharara, S. L., Awad, L. S., and Kanj, S. S. (2018). Epidemiology of common resistant bacterial pathogens in the countries of the Arab League. Lancet Infect. Dis. 18, e379–e394. doi: 10.1016/S1473-3099(18)30414-6
Neuert, S., Nair, S., Day, M. R., Doumith, M., Ashton, P. M., Mellor, K. C., et al. (2018). Prediction of phenotypic antimicrobial resistance profiles from whole genome sequences of non-typhoidal Salmonella enterica. Front. Microbiol. 9:592. doi: 10.3389/fmicb.2018.00592
Parisi, A., Crump, J. A., Glass, K., Howden, B. P., Furuya-Kanamori, L., Vilkins, S., et al. (2018). Health outcomes from multidrug-resistant salmonella infections in high-income countries: a systematic review and meta-analysis. Foodborne Pathog. Dis. 15, 428–436. doi: 10.1089/fpd.2017.2403
Perez-Perez, F. J., and Hanson, N. D. (2002). Detection of plasmid-mediated AmpC beta-lactamase genes in clinical isolates by using multiplex PCR. J. Clin. Microbiol. 40, 2153–2162. doi: 10.1128/JCM.40.6.2153-2162.2002
Poirel, L., Héritier, C., Tolün, V., and Nordmann, P. (2004). Emergence of oxacillinase-mediated resistance to imipenem in Klebsiella pneumoniae. Antimicrob. Agents Chemother. 48, 15–22. doi: 10.1128/AAC.48.1.15-22.2004
Poirel, L., Walsh, T. R., Cuvillier, V., and Nordmann, P. (2011). Multiplex PCR for detection of acquired carbapenemase genes. Diagn. Microbiol. Infect. Dis. 70, 119–123. doi: 10.1016/j.diagmicrobio.2010.12.002
Porwollik, S., Boyd, E. F., Choy, C., Cheng, P., Florea, L., Proctor, E., et al. (2004). Characterization of Salmonella enterica subspecies I genovars by use of microarrays. J. Bacteriol. 186, 5883–5898. doi: 10.1128/JB.186.17.5883-5898.2004
Raffatellu, M., Wilson, R. P., Chessa, D., Andrews-Polymenis, H., Tran, Q. T., Lawhon, S., et al. (2005). SipA, SopA, SopB, SopD, and SopE2 contribute to Salmonella enterica serotype typhimurium invasion of epithelial cells. Infect. Immun. 73, 146–154. doi: 10.1128/IAI.73.1.146-154.2005
Rajashekar, R., Liebl, D., Chikkaballi, D., Liss, V., and Hensel, M. (2014). Live cell imaging reveals novel functions of Salmonella enterica SPI2-T3SS effector proteins in remodeling of the host cell endosomal system. PLoS ONE 9:e115423. doi: 10.1371/journal.pone.0115423
Seiffert, S. N., Perreten, V., Johannes, S., Droz, S., Bodmer, T., and Endimiani, A. (2014). OXA-48 carbapenemase-producing Salmonella enterica serovar Kentucky isolate of sequence type 198 in a patient transferred from Libya to Switzerland. Antimicrob. Agents Chemother. 58, 2446–2449. doi: 10.1128/AAC.02417-13
Stein, M. A., Leung, K. Y., Zwick, M., Garcia-del Portillo, F., and Finlay, B. B. (1996). Identification of a Salmonella virulence gene required for formation of filamentous structures containing lysosomal membrane glycoproteins within epithelial cells. Mol. Microbiol. 20, 151–164. doi: 10.1111/j.1365-2958.1996.tb02497.x
Threlfall, E. J., Ward, L. R., Skinner, J. A., and Graham, A. (2000). Antimicrobial drug resistance in non-typhoidal salmonellas from humans in England and Wales in 1999: decrease in multiple resistance in Salmonella enterica serotypes Typhimurium, Virchow, and Hadar. Microb. Drug Resist. 6, 319–325. doi: 10.1089/mdr.2000.6.319
Tilton, R. C., Lieberman, L., and Gerlach, E. H. (1973). Microdilution antibiotic susceptibility test: examination of certain variables. Appl. Microbiol. 26, 658–665.
Uche, I. V., MacLennan, C. A., and Saul, A. (2017). A systematic review of the incidence, risk factors and case fatality rates of invasive nontyphoidal Salmonella (iNTS) disease in Africa (1966 to 2014). PLoS Negl. Trop. Dis. 11:e0005118. doi: 10.1371/journal.pntd.0005118
Wang, J., Li, Y., Xu, X., Liang, B., Wu, F., Yang, X., et al. (2017). Antimicrobial resistance of Salmonella enterica Serovar Typhimurium in Shanghai, China. Front. Microbiol. 8:510. doi: 10.3389/fmicb.2017.00510
Wattiau, P., Van Hessche, M., Schlicker, C., Vander Veken, H., and Imberechts, H. (2008a). Comparison of classical serotyping and PremiTest assay for routine identification of common Salmonella enterica serovars. J. Clin. Microbiol. 46, 4037–4040. doi: 10.1128/JCM.01405-08
Wattiau, P., Weijers, T., Andreoli, P., Schliker, C., Veken, H. V., Maas, H. M., et al. (2008b). Evaluation of the Premi Test Salmonella, a commercial low-density DNA microarray system intended for routine identification and typing of Salmonella enterica. Int. J. Food Microbiol. 123, 293–298. doi: 10.1016/j.ijfoodmicro.2008.01.006
Weill, F. X., Guesnier, F., Guibert, V., Timinouni, M., Demartin, M., Polomack, L., et al. (2006). Multidrug resistance in Salmonella enterica serotype Typhimurium from humans in France (1993 to 2003). J. Clin. Microbiol. 44, 700–708. doi: 10.1128/JCM.44.3.700-708.2006
Wisner, A. L., Desin, T. S., Koch, B., Lam, P. K., Berberov, E. M., Mickael, C. S., et al. (2010). Salmonella enterica subspecies enterica serovar Enteritidis salmonella pathogenicity island 2 type III secretion system: role in intestinal colonization of chickens and systemic spread. Microbiology 156(Pt 9), 2770–2781. doi: 10.1099/mic.0.038018-0
Zhang, K., Riba, A., Nietschke, M., Torow, N., Repnik, U., Pütz, A., et al. (2018). Minimal SPI1-T3SS effector requirement for Salmonella enterocyte invasion and intracellular proliferation in vivo. PLoS Pathog. 14:e1006925. doi: 10.1371/journal.ppat.1006925
Zhang, S., Santos, R. L., Tsolis, R. M., Stender, S., Hardt, W. D., Baumler, A. J., et al. (2002). The Salmonella enterica serotype typhimurium effector proteins SipA, SopA, SopB, SopD, and SopE2 act in concert to induce diarrhea in calves. Infect. Immun. 70, 3843–3855. doi: 10.1128/IAI.70.7.3843-3855.2002
Keywords: Salmonella, SP-1, SPI-2, Type 3 secretion systems, serotyping
Citation: Alghoribi MF, Doumith M, Alrodayyan M, Al Zayer M, Köster WL, Muhanna A, Aljohani SM, Balkhy HH and Desin TS (2019) S. Enteritidis and S. Typhimurium Harboring SPI-1 and SPI-2 Are the Predominant Serotypes Associated With Human Salmonellosis in Saudi Arabia. Front. Cell. Infect. Microbiol. 9:187. doi: 10.3389/fcimb.2019.00187
Received: 07 March 2019; Accepted: 15 May 2019;
Published: 31 May 2019.
Edited by:
Margaret E. Bauer, Indiana University School of Medicine, Bloomington, United StatesReviewed by:
Steve Carlson, Iowa State University College of Veterinary Medicine, United StatesBrian Ahmer, The Ohio State University, United States
Copyright © 2019 Alghoribi, Doumith, Alrodayyan, Al Zayer, Köster, Muhanna, Aljohani, Balkhy and Desin. This is an open-access article distributed under the terms of the Creative Commons Attribution License (CC BY). The use, distribution or reproduction in other forums is permitted, provided the original author(s) and the copyright owner(s) are credited and that the original publication in this journal is cited, in accordance with accepted academic practice. No use, distribution or reproduction is permitted which does not comply with these terms.
*Correspondence: Taseen S. Desin, taseen.desin@usask.ca